- 1Department of Pediatrics, Nanfang Hospital, Southern Medical University, Guangzhou, China
- 2Department of Endocrinology, Affiliated Baoan Hospital of Shenzhen, Southern Medical University, Shenzhen, China
Chemotherapy is a critical treatment for endocrine-related cancers; however, chemoresistance and disease recurrence remain a challenge. The interplay between cancer cells and the tumor microenvironment via cell adhesion molecules (CAMs) promotes drug resistance, known as cell adhesion-mediated drug resistance (CAM-DR). CAMs are cell surface molecules that facilitate cell-to-cell or cell-to-extracellular matrix binding. CAMs exert an adhesion effect and trigger intracellular signaling that regulates cancer cell stemness maintenance, survival, proliferation, metastasis, epithelial–mesenchymal transition, and drug resistance. To understand these mechanisms, this review focuses on the role of CD44, cadherins, selectins, and integrins in CAM-DR in endocrine-related cancers.
Introduction
Multidrug resistance and disease recurrence are challenging in the treatment of endocrine-related cancers, including breast, ovarian, prostate, pancreatic, and thyroid cancers. Breast cancer is one of the most common cancers in women worldwide and is the leading cause of cancer-related deaths (1). The 5-year survival rate of patients with metastatic breast cancer is approximately 26% (2). The ER/PR/HER2-negative subtype, known as triple-negative breast cancer (TNBC), accounts for approximately 10%–15% of all breast cancers and constitutes the most aggressive breast cancer (3). TNBC has a high metastatic capacity and poor outcome owing to increased recurrence rates, regardless of disease stage and resistance to conventional therapies (4). Ovarian cancer may arise from the histological portions of the ovary, including the epithelium, stroma, or germ cells. Epithelial ovarian cancers are diagnosed at advanced stages and are treated with surgery and chemotherapy (5). Of these, high-grade serous carcinoma arising from the epithelium of the ovary is the most common (5). The clinical management and prognosis of ovarian cancer depend on the cancer stage. Most patients are diagnosed at an advanced stage with widespread peritoneal dissemination and malignant ascites. Since rapidly proliferating tumors compress visceral organs and are only temporarily chemosensitive, ovarian carcinoma is a deadly disease with a cure rate of only 30% (6). Although the incidence rates for pancreatic ductal adenocarcinoma (PDAC) are currently low, about half of the patients have advanced disease at diagnosis, and it is estimated that it will be the second leading cause of cancer-related mortality by 2030. Nearly half of the patients with PDAC have progressive disease at diagnosis, and multiagent chemotherapy regimens only provide a survival benefit of 2–6 months compared with single-agent gemcitabine for advanced patients (7). Metastatic castration-resistant prostate cancer (8) and persistent or recurrent thyroid cancer are highly treatment-refractory and have poor prognoses (9, 10).
Cell adhesion molecules (CAMs) are transmembrane receptor proteins involved in cell-to-cell or cell-to-extracellular matrix (ECM) binding (11). Based on their structures, CAMs can be classified into four major groups: integrins, cadherins, selectins, and the immunoglobulin superfamily CAM (IgCAM) (12, 13). CD44 is an essential cell surface glycoprotein involved in cell-to-cell adhesion (14). Therefore, we considered CD44 as a CAM in this context. CAMs play a role in maintaining cell adhesion and exert crucial cellular functions, such as cell proliferation, survival, migration, and oncogenesis (14–16). Moreover, the cell adhesion-mediated resistance (CAM-DR) restricts the success of cancer therapies and is an enormous obstacle to combat in the clinic (13, 17). This review describes the role of integrins, cadherins, selectins, and CD44 in endocrine-related cancer.
This review attempts to concisely summarize the advances made in this context, emphasizing endocrine-related cancers and the avenues for future progress to target mitotic mechanisms to overcome these dreadful cancers.
Structures and Function of CAMs
Integrins
Integrins are calcium-independent heterodimeric transmembrane proteins with a shared structure of the extracellular, transmembrane, and cytoplasmic domains (Figure 1A). To date, 24 functionally distinct integrin heterodimers have comprised 18 integrin α and 8 integrin β subunits (18). Integrins interact with specific ECM ligands via bidirectional signaling pathways. Inside-out signals mediate talin binding to integrin β-tails and thus tightly control integrin affinity for ECM ligands. Subsequent ECM binding triggers the recruitment of protein complexes to integrin cytoplasmic tails to facilitate integrin downstream signaling, known as outside-in signaling (19). In addition, integrins can be internalized and recycled, thereby controlling the availability of integrin heterodimers in the plasma membrane (13). Integrins and their ligands play critical roles in cell survival, proliferation, motility, differentiation, and ensuring appropriate cell localization (20, 21).
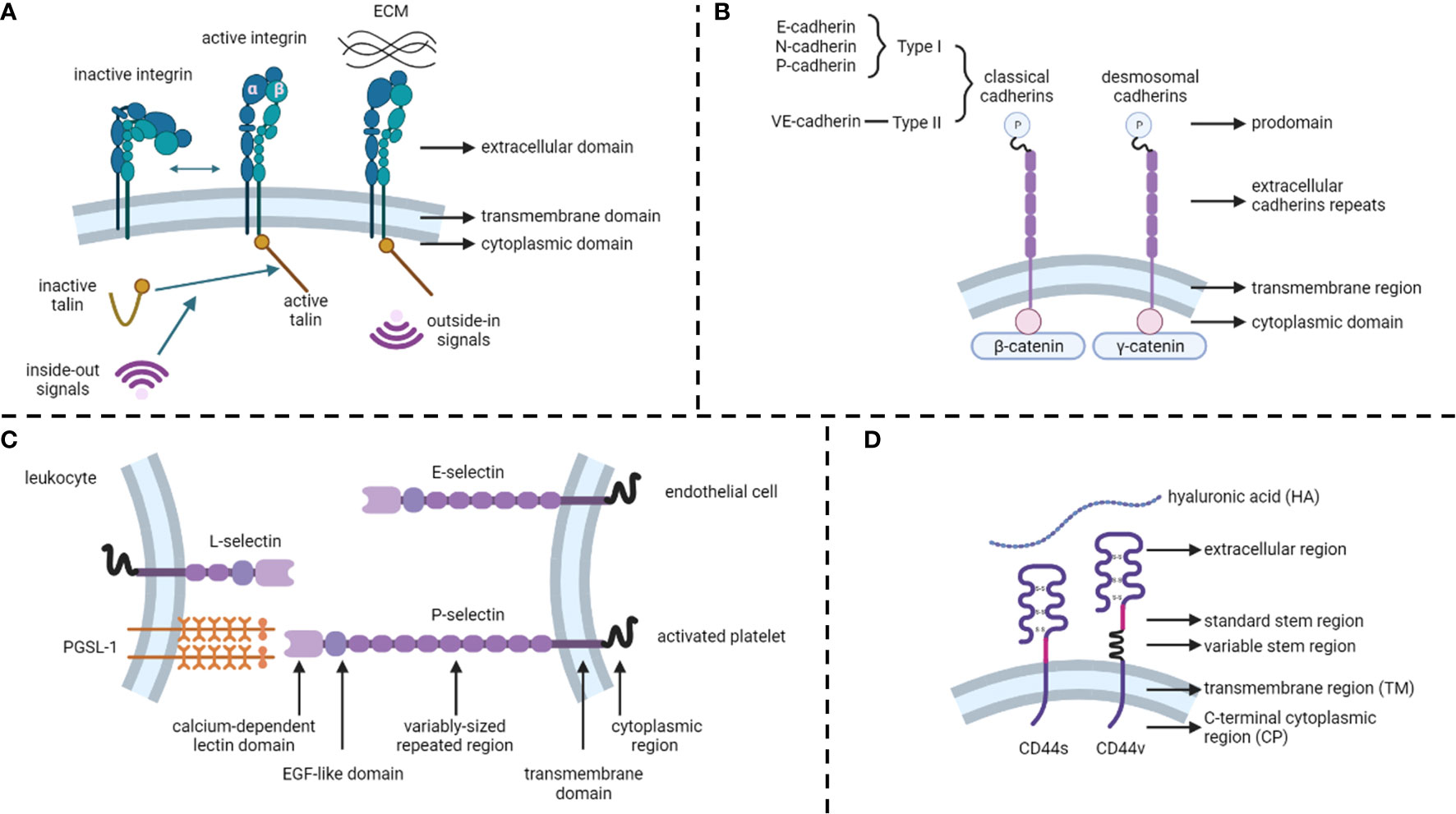
Figure 1 Structures of cell adhesion molecules. (A) Integrins. (B) Cadherins. (C) Selectins. (D) CD44. ECM, extracellular matrix.
Cadherins
Unlike integrins, cadherins are calcium-dependent transmembrane proteins (22) that constitute type I and type II classical cadherins, desmosomal cadherins, proto-cadherins, seven-pass transmembrane cadherins, and FAT and dachsous cadherins (13) (Figure 1B). These proteins contain a prodomain (P) immediately after the removal of the signal sequence by proteolysis. Mature classical and desmosomal cadherins have ectodomains composed of five extracellular cadherin repeats, a single transmembrane region, and a cytoplasmic domain that interacts with either β-catenin (classical cadherin) or γ-catenin (desmosomal cadherin) (22). Moreover, E-cadherin (CDH1), N-cadherin, and P-cadherin are classified as type I, whereas VE-cadherins are type II classical cadherins (13). Cadherins regulate cell-to-cell cohesion in all tissues and are indispensable for morphogenesis, maintaining tissue barriers, and regulating tissue remodeling (16, 23).
Selectins
Selectins (CD62) are single-chain transmembrane glycoproteins that regulate cell-to-cell adhesion via carbohydrate-binding in a calcium-dependent manner (24). There are three major types of selectins: L-selectins (primarily expressed on leukocytes), E-selectins (expressed on endothelial cells), and P-selectins (expressed on activated platelets) (25) (Figure 1C). The structures of selectins consist of a calcium-dependent lectin domain, an epidermal growth factor (EGF)-like domain, a variably sized repeated region, a transmembrane domain, and a cytoplasmic domain (26). The primary function of selectins is to promote leukocyte rolling along with endothelial cells, which is an initial step in the transmigration of leukocytes through the endothelial barrier (27, 28).
CD44
The CD44 protein consists of four primary regions: the extracellular region, stem region (standard stem region and variable stem region), transmembrane region (TM), and C-terminal cytoplasmic (CP) region (14) (Figure 1D). The CD44 variant (CD44v) differs from the CD44 standard isoform (CD44s) by the insertion or excision of alternatively spliced exons between the N-terminal and C-terminal domains (29). CD44s are distributed in tissues, such as lymphocytes, the central nervous system, lungs, epidermis, pancreas, intestines, kidneys, urinary bladder, and cervix. CD44v is dispersed on keratinocytes, lymphocytes, macrophages, and epithelial cells in the bladder, stomach, and cervix (30). While binding to its primary ligand hyaluronic acid (HA), CD44 has extensive functions, such as cell adhesion, hyaluronate degradation, lymphocyte activation, lymphocyte homing, lymphopoiesis, myelopoiesis, angiogenesis, and release of cytokines (31, 32).
CAMs in Cancers and Their Microenvironment
CAMs mediate the adhesion of cancer cells not only to each other but also to stromal cells and ECM, namely, the tumor microenvironment (TME). The TME mainly comprises stable nonmalignant cells, including surrounding immune cells, extracellular matrix, fibroblasts, endothelial cells (ECs), blood vessels, and stromal cells (33).
Notably, there is a population of cancer cells named cancer stem cells (CSCs), identified as cells within a tumor with self-renewal capacity and tumorigenic potential (34). In previous studies, CSCs were isolated from various cancers and successfully identified using surface markers, including CD44, CD24, CD133, and CD166 (34, 35), with CD44 being the most common marker (36). For example, a population of cells expressing CD44 high/CD24 low was identified as CSCs in breast cancer (37). Moreover, when CD44 binds to HA, it plays a pivotal role in cancer invasiveness (38). In a previous study, the invasiveness of the human breast cancer cell line MDA-MB-468 was increased by 45% with high-molecular-weight HA and was inhibited by anti-CD44s or HAoligo-6 (39).
CSCs also have characteristics associated with cells undergoing epithelial–mesenchymal transition (EMT) (40). In metastatic epithelial tumors, downregulation of CDH1, compensated by the expression of N-cadherin, is a hallmark of EMT (41). The transforming growth factor-β (TGF-β) pathway plays a central role in activating EMT in several cancers (42, 43). EMT-inducing transcription factors (EMT-TFs), such as Snail, ZEB1, TWIST, and Slug, endow resistance to cisplatin- and oxaliplatin-based chemotherapies in breast, ovarian, and pancreatic cancers and CSC maintenance (44, 45). The invasion and metastasis of cancer implicate the latest steps in malignant progression and most cancer-associated deaths (46). Interestingly, cadherins mediate cancer cell-to-cancer cell adhesion and, subsequently, form collective migration, defined as two or more cells moving together rather than single cancer cell migration (47).
After cancer cells undergo EMT, selectins confer cancer cells that express selectin ligand implantation from blood-borne metastasis. For instance, it was shown that overexpressing c-FOS ovarian cancer cells had lower selectin ligands sLea and sLex and diminished adhesion to E-selectin, thereby reducing metastasis in an intraperitoneal xenograft mouse model (48). Regarding P-selectin, there was a report showing that P-selectin mediated rolling and adhesion of ovarian cancer cells expressing sLex to mesothelial cells (49). Similarly, Gebauer et al. demonstrated that the interaction of E-selectin, P-selectin, and mesothelial cells in pancreatic cancer cells was in a shear stress-dependent manner (50).
This initial interaction between endothelial or mesothelial cells expressing selectins and cancer cells results in the activation of integrins, which mediate firm adhesion to the ECM and stromal cells. Cancer-associated fibroblasts (CAFs) are derived from various cell types including ECs (51), adipocytes (52), pericytes (53), and mesenchymal stromal cells (MSCs) (54). In addition, integrin α11 in breast CAFs originating from MSCs (54) interacts with platelet-derived growth factor receptor beta (PDGFRβ), promotes invasiveness, and produces a matricellular protein (55). Another role of integrins is the maintenance of CSC characteristics, such as CD44 marker. For example, integrin β1 is a CSC marker and its expression is critical for initiating breast cancer tumorigenesis in vivo, which is discussed in the following section (56).
CSCs or cancer cells contact the TME through cell-to-cell and cell-to-ECM by CAMs, leading to the initiation of survival and proliferation signaling pathways in cancer cells and secretion of cytokines and growth factors from both cancer cells and stromal cells. Furthermore, this mutual beneficial effect regulates conventional treatment failure, called cell adhesion-mediated drug resistance (CAM-DR), in cancer cells.
CD44 and Endocrine-Related Cancers
CD44-positive expression was predominantly correlated with a high TMN and worse 5-year overall survival in ovarian cancers in a meta-analysis of 18 publications including 2,161 patients (57). CD44 is the most common cancer stem cell receptor, and its overexpression is associated with metastasis, resistance, and tumor recurrence in endocrine-related cancers (38, 58, 59). Although many cells express CD44s on their cell surface, cancerous cells express both CD44s and CD44v on their cell membranes (60). HA interacts with various cell surface receptors, including those involved in intracellular signaling pathways, such as the tyrosine kinase pathway and the hyaluronan-mediated motility receptor CD44, to increase proliferation, survival, and resistance to cancer cells (61) (Figure 2).
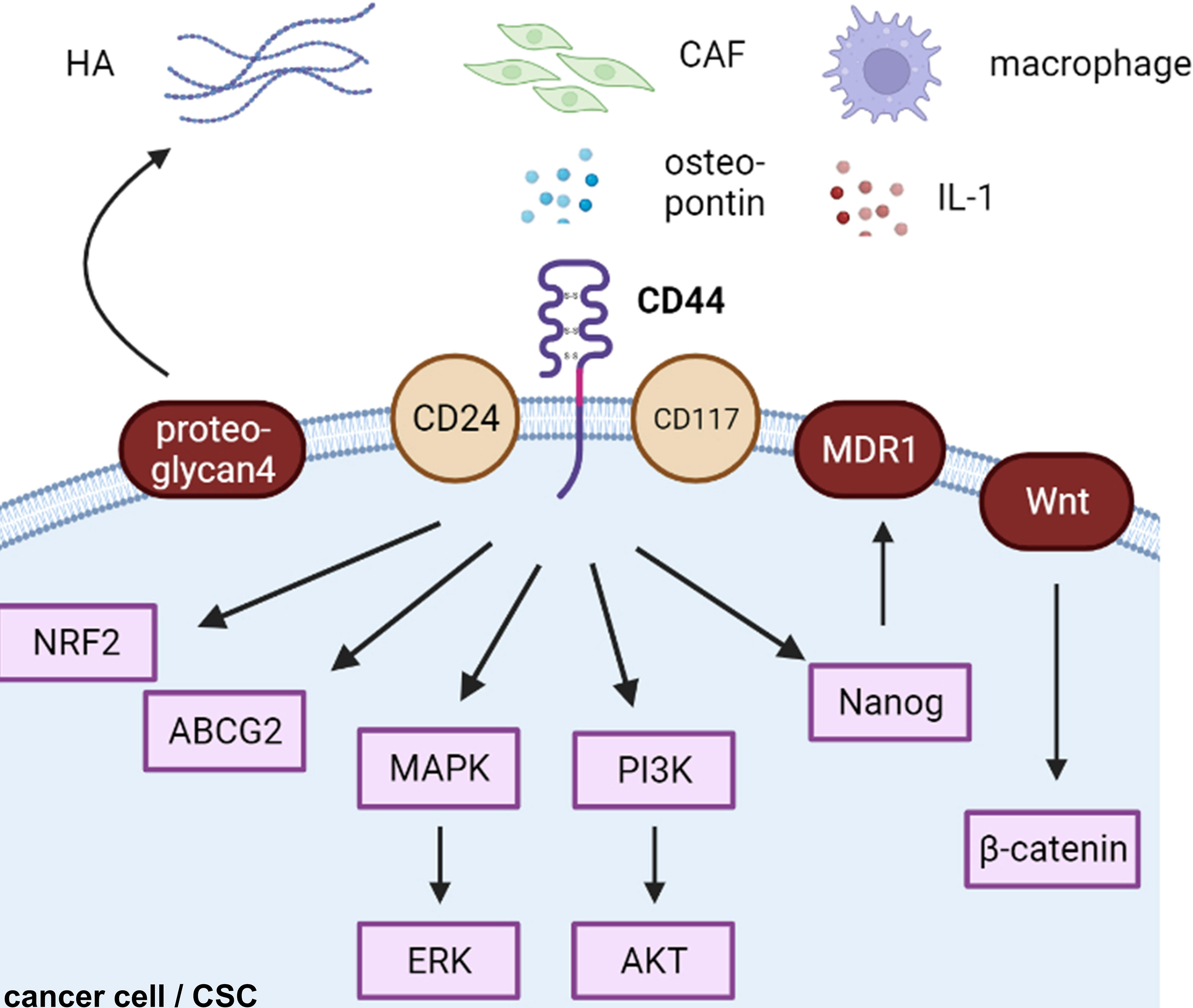
Figure 2 CD44-related cascades in cancer cells. HA, hyaluronic acid; CAF, cancer-associated fibroblast; CSC, cancer stem cell.
CD44 and CSCs
Based on clinical evidence, increased CD44high/CD24low expression was found in a subpopulation of chemoresistant breast CSCs (62). The CD44+/CD117+ subpopulation is known as ovarian CSCs (63). CD44s, rather than CD44v, initiates PDGFRβ/Stat3 signaling to facilitate CSC properties in breast cancer (64). Stress tolerance caused by reactive oxygen species is a characteristic of drug resistance. CD44v enhances protection against ROS and promotes chemoresistance (65). Moreover, long-term CAF-conditioned media facilitates the enrichment of the stemness population of prostate cancer cells and their drug resistance via the osteopontin/CD44 axis (66). For example, high CD44 expression results in p62-associated NRF2 activation in breast CSCs. CD44/NRF2 activation contributes to doxorubicin resistance in CD44high breast CSCs (67). Proteoglycan 4, a mucin-like glycoprotein, induces TGF-β and HA expression in breast cancer (68). Therefore, proteoglycan 4 acts as a CD44/HA regulator and promotes metastasis (68). Recently, a study showed that CD44 promoted PD-L1 expression, eliciting an immunosuppressive effect in TNBC using the FACS-assisted shRNA screening method (69). MicroRNA-199a could target CD44, restore chemosensitivity to paclitaxel, cisplatin, and adriamycin, and reduce ABCG2 expression, a multidrug resistance gene in in vitro and in vivo ovarian cancer models (63).
HA/CD44/MDR1 Axis
Following the interaction of CD44 with HA, ankyrin binds to multidrug resistance mutation 1 (MDR1) leading to doxorubicin efflux and paclitaxel and chemoresistance in both breast and ovarian cancer cells (70). Moreover, this activation of MDR1 is mediated by CD44/Nanog/Stat-3 signaling (70). Further study demonstrated that inhibitors of apoptosis proteins are also elevated by the CD44/HA/Nanog interaction (71). This process leads to microRNA-21 production and protein programmed cell death 4 reductions (71). HA-CD44-PI3K-ErbB2 constitutes a positive feedback loop in an Akt-independent manner (72). This loop amplifies MDR1 expression and regulates drug resistance in doxorubicin-resistant breast cancer cells MCF-7 (73) and pancreatic cancer cells (73). CD44/HA/MDR1 siRNA NPs have been successfully evaluated in ovarian cancer (74). HA-engineered nanomicelles are taken up by triple-marker positive (CD44+/CD133+/EpCAM+) pancreatic CSCs and induce a cell killing effect (75). HA-modified nano-complexes target CD44 receptor-induced apoptosis of drug-resistant cells via mTOR signaling in ovarian cancer SKOV3 cells in vivo (76).
CD44/PI3K, CD44/MAPK, and CD44/Wnt Axis
Increased CD44v6 expression is found in prostate cancer surrounding stromal cells (77) and indicates poor clinical outcomes in pancreatic ductal adenocarcinomas (78). Furthermore, knockdown of CD44v6 suppresses prostate cancer proliferation, reduces EMT, and augments chemosensitivity via downregulation of Wnt/β-catenin and PI3K/Akt/mTOR signaling (77). Another study determined that CD44v3 induces MDR1 overexpression, thereby activating P300/β-catenin and NF-κB (60). Calreticulin maintains the breast CSC properties of CD24-/CD44+ and ALDH+ and promotes breast cancer progression via Wnt/β-catenin signaling in an HIF-1-dependent manner (79). SRGN is an ECM factor that binds to CD44 in an autocrine manner and acts by triggering MAPK and Wnt/β-catenin signaling in breast CSCs (80). Thus, CD44 is closely regulated by Wnt signaling (33, 81).
Phospho (p)-AKT expression is more likely found in CD44v6-positive breast cancer tissues. Moreover, overexpression of CD44v6 and p-AKT is correlated with poor clinical outcomes (82). Osteopontin/CD44 cascade promotes ovarian cancer cell chemoresistance via PI3K/AKT signaling and drug efflux mechanisms (83). Rho GTPase activation has been linked to the prostate cancer cell line PC3 and osteopontin adhesion. Subsequently, surface CD44 and MMP-9 expression increases (84). Furthermore, CD44 has been linked to aggressive pancreatic cancer by the PI3K/AKT or MAPK/ERK pathways in clinical data (85).
Recently, several studies have implied that some interleukins (ILs) also regulate CD44 signaling. IL1β, a pivotal regulator of the systemic inflammatory response, is produced by macrophages in breast cancer. This process is mediated by the breast cancer cell membrane-derived soluble CD44. Therefore, neutralization of CD44 by antibody decreased IL1β production in macrophages and attenuated the growth of primary breast cancer (86). Another similar target strategy using an IL1R2 neutralizing antibody suppressed breast CSC progression (87). IL-6 can generate CD44+ CSCs via the induction of EMT in breast cancer cell T47D (88).
Cadherins and Endocrine-Related Cancers
In recent decades, studies have shown that mechanical EMT mediated by cadherin switching leads to drug resistance in breast cancer. Decreased CDH1 expression is significantly correlated with a higher-grade, triple-negative receptor status, and poor prognosis in invasive breast carcinoma (89). Upregulation of cyclin D1, β-catenin, N-cadherin, MMP-2, MMP-9, and ICAM-1 and downregulation of CDH1 were found in doxorubicin-resistant TNBC cells (90). CDH1 is crucial for epithelial polarization and differentiation. Deregulation of CDH1 function plays a fundamental role in breast cancer metastasis and is associated with a worse prognosis (91). Over the past decade, many mechanisms have been identified to cause CDH1 inactivation in breast cancer (92, 93). In the 1990s, a correlation was found between the absence of CDH1 and the lobular subtype harboring CDH1 genetic alterations (94, 95). Subsequently, it is well-known that approximately half of the invasive lobular breast cancers show loss of heterozygosity, which is crucial for CDH1 dysfunction and loss of expression (93, 96). In addition, epigenetic alterations have emerged as a possible cause of aberrant CDH1 expression and function, such as hypermethylation of the CDH1 promoter site (97, 98). Abnormal glycosylation is also a potential cause of CDH1 dysfunction, because CDH1 is post-translationally modified by oxygen and nitrogen glycosylation (99, 100).
Cadherins and EMT
Many recent studies have focused on signaling changes in EMT in endocrine-related cancers (101–103). In this review, we have taken breast cancer as an example to illustrate recent EMT-related signaling (Figure 3). β-catenin, a critical cytoplasmic protein, confers cadherin-mediated cell-to-cell adhesion due to the transcriptional output of Wnt signaling, which leads to breast cancer tumor progression (104). Krüppel-like factor 9 (KLF9) blocks lung metastasis of breast cancer and increases CDH1 expression in 4T1 cells in vivo, indicating that the KLF9/CDH1 axis strongly contributes to breast cancer invasion and metastasis (105). N-cadherin-dependent cell-to-cell adhesion is required for migration mediated by bone marrow-derived MSCs towards MDA-MB-231 breast cancer cells via canonical TGF-β signaling (106). A previous study reported that aging breast ECM alone was sufficient to drive normal human mammary epithelial cells (KTB21) to an invasive and cancer-like phenotype that led to a loss of CDH1 in vivo (107). CDH1 facilitates metastasis in phenotypically sorted subpopulations of breast cancer cells, enabling clustering of circulating tumor cells (108). Snail exerts invasion, migration, and adhesion effects via regulation of N-cadherin and CDH1 in drug-resistant MCF-7/A cells (109). The authors also found that miR34a combined with doxorubicin suppressed the expression of Snail by inhibiting the Notch/NF-κB and RAS/RAF/MEK/ERK pathways in MCF-7/A cells (109).
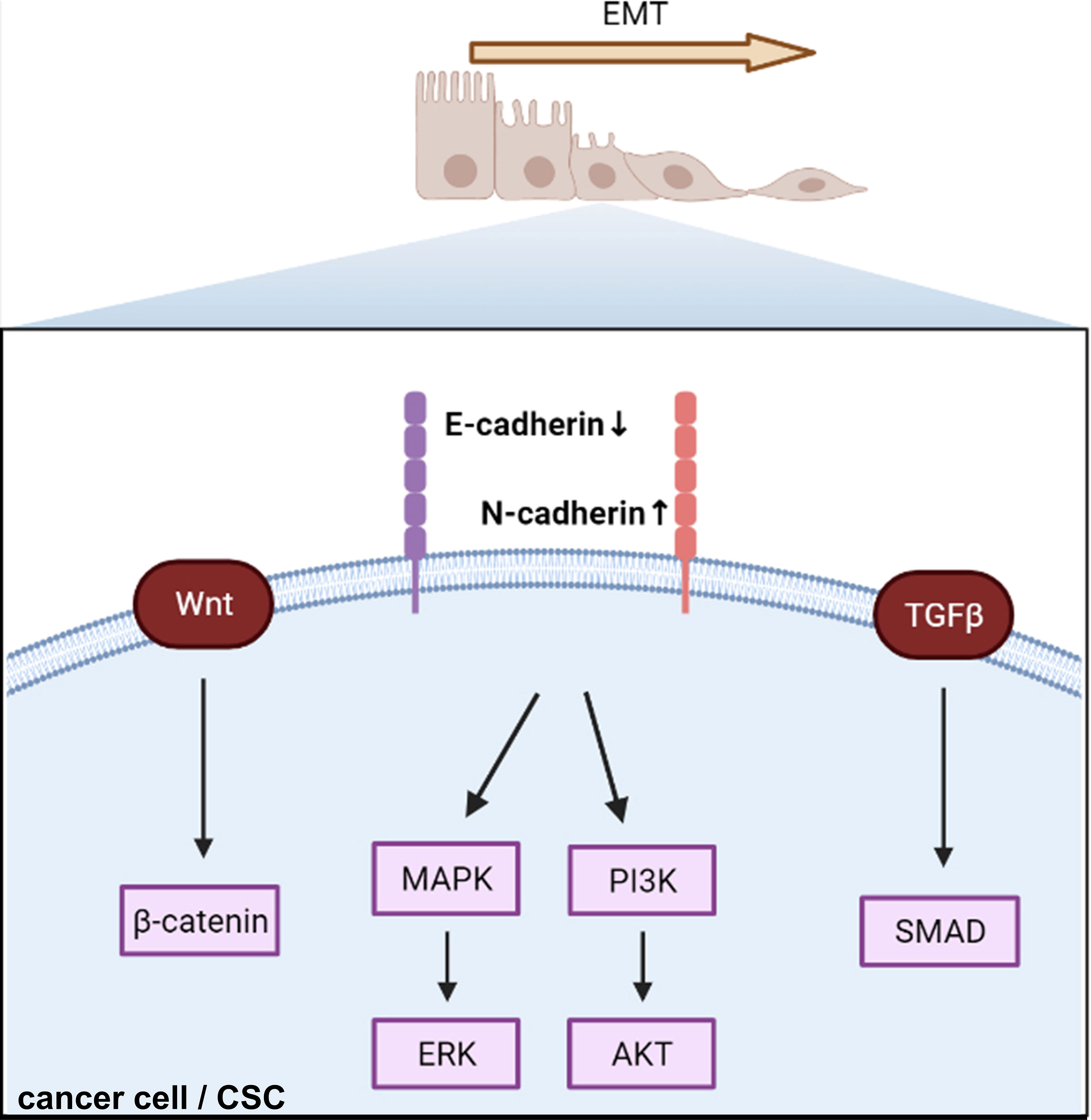
Figure 3 Cadherin regulates epithelial–mesenchymal transition and triggers intracellular signaling. EMT, epithelial–mesenchymal transition; CSC, cancer stem cell.
Cadherins and CAM-DR
Furthermore, the combination of miR34a and doxorubicin significantly repressed tumor growth in the MCF-7/A nude mouse xenograft model compared to doxorubicin alone (109). Cell motility was mediated by ERK1/2 and CDH1 was correlated with lactate supplementation (110). A study showed that activated ERK signaling is involved in radiotherapy-resistant breast cancer using MDA-MB-231 cells. CDH1/TGF-β/p-Smad2/3 signaling was found to be a survival axis in breast cancer by inhibiting reactive oxygen-dependent endogenous mitochondrial apoptosis (111). F-box and leucine-rich repeat protein 10 (FBXL10) interacting with SNAI1 promoted the migration and invasion of breast cancer cells by inhibiting CDH1 expression and inducing EMT (112). AKR1B10 downregulated CDH1 expression via the PI3K/AKT/NF-κB p65 signaling pathway in MCF-7 cells (113). Moreover, taxane therapy resistance was related to reduced PRP4K expression, depletion of PRP4K in TNBC cells (MDA-MB-231), and enhanced 2D migration, and 3D invasion correlated with higher fibronectin levels rather than changes in CDH1 (114). Connexins linked to N-cadherin, vimentin, Snail, and Zeb1 modulate CSC and EMT properties in breast cancer cells (90).
At the RNA level, overexpression of TPA diminished the expression of CDH1 and increased the expression of vimentin, fibronectin, and TGF-β1 (115). Circular RNA studies have shown that circNOLC1 (116) and circSCRIB (117) contribute to BC cell invasion and migration ability by reducing CDH1 and facilitating the tumorigenesis of breast cancer (118). Extracellular vesicles of insulin-like growth factor-1 (IGF-1), which is a crucial regulatory factor of mammary glands, promote the downregulation of CDH1 and upregulation of vimentin, N-cadherin, and MMP-9 in MDA-MB-231 breast cancer cells (119).
Interestingly, among environmental contaminants, including long-chain per- and polyfluoroalkyl substances (PFASs), a study has found that PFHxS can induce cell malignancy by reducing the levels of CDH1 and β-integrin and promoting cell migration and invasion in normal human breast epithelial cells (MCF-10A) (120).
Apart from CDH1 and N-cadherin, VE-cadherin promotes the attachment of breast cancer cells to the endothelial layer and initiates the incorporation phase instead of transmigration (121). As a result, cadherins mainly mediate CAM-DR via the EMT pathway and promote long-distance metastasis of breast cancer.
Targeting of Cadherins
To date, there are few targeted therapies for cadherins. However, one potential practical strategy is the reversal of EMT by restoring CDH1 expression. For example, CTI-2 increased the expression level of CDH1 and decreased the expression levels of N-cadherin and vimentin through inactivation of the MAPK signaling pathway (122). MTDH, an miR-9-3p inhibitor, promotes the effect of gemcitabine on inducing apoptosis and inhibiting cell migration, invasion, and growth in breast cancer, suggesting that miR-9-3p regulated gemcitabine drug resistance (123). Interestingly, atorvastatin, an HMG-CoA reductase inhibitor, enhanced partial cancer-associated mesenchymal-to-epithelial reverting transition, and facilitated chemotherapy effects in metastatic TNBC (124). The sulforaphane-cisplatin combination restored CDH1 expression by altering chromatin modification and attenuated the metastatic potential of TNBCs by downregulating the SIRT-mediated EMT signaling axis (125). This study demonstrated that sulforaphane-cisplatin could overcome cisplatin resistance (125). The tumor-suppressive role of miR-205, suppressed by the CLDN11 gene, regulates EMT in breast cancer (126). Co-treatment with miR34a and doxorubicin slowed tumor growth in MCF-7/A cells via the Snail/CDH1 pathway in vivo (109). Intriguingly, many herbal or traditional medicines are available. JI017 in combination with paclitaxel can overcome paclitaxel resistance (127). Triptonide, a small molecule from the traditional Chinese medicinal herb, promotes the downregulation of N-cadherin, VE-cadherin, and VEGFR2 (128). Rhoifolin (RFL), a flavonoid from C. nudiflora, is a Chinese medicinal herb that affects CDH1, vimentin, Snail, and Slug, thereby eliciting anti-motile properties (129). Ethanol-based garlic extract prevents breast cancer evolution driven by a hypoxia-induced decrease in CDH1 and increased vimentin and motility (130).
Selectins and Endocrine-Related Cancers
The role of selectins in cancer implantation and metastasis is well-established (131). Selectin ligands on cancer cells interact with selectins on endothelial or mesothelial cells, leading to extravasation of cancer cells into the blood, which is critical for hematogenous metastasis (132) (Figure 4). In addition to blood-borne metastasis, selectins mediate peritoneal dissemination in endocrine-related cancers (133). Platelets and leukocytes are also involved in metastasis via selective regulation (131, 134, 135). Selectins, particularly E-selectin, are involved in cancer drug resistance in acute myeloid leukemia (AML).
Selectins and Their Ligands in Cancers
Inhibition of the interplay between selectins and their ligands has been studied as a potential strategy to provide therapeutic benefit in cancer. P-selectin glycoprotein ligand 1 (PSGL-1), a well-known selectin ligand, promotes the physiological and pathological progression of endocrine-related cancers (136). CD24 is another well-known P-selectin ligand that confers ovarian cancer progression by directly mediating ovarian cancer adhesion to tumor–mesothelial (49). MIP-1β secreted by alternatively activated macrophages elevated the expression of P-selectin on the mesothelial cell surface through CCR5/PI3K signaling, and ovarian cancer cells adhered to the de novo P-selectin via CD24 (49). MUC16 (CA125) is a selectin ligand expressed in metastatic pancreatic cancer cells (137) and epithelial ovarian cancer cells (138). Another mucin, called CA19-9, accelerates pancreatic cancer progression by binding to E-selectin, promoting angiogenesis, and regulating immunological response (139). Overexpression of MUC1, MUC9, and MUC13 has also been observed in ovarian cancer cells (140, 141). Two E-selectin ligands, BST-2 and LGALS3BP, are correlated with high liver and brain metastasis potential and poor survival in ER-negative breast cancer (142). Growth of pancreatic cancer cells was slowed down by E-selectin-dependent rolling and E-selectin-HA binding (143). Sialyl Lewis X on bone-metastatic prostate tumor cells exerted robust E-selectin binding activity by shear-flow manner (144). Contrarily, c-FOS, a negative regulator of E-selectin, significantly reduced ovarian cancer growth both in vitro and in vivo (48).
Selectins and Metastasis
Breast cancer cells are anchored to the bone marrow microenvironment through the stromal-derived factor 1 (SDF-1)/E-selectin pathway (145). E-selectin also induces mesenchymal–epithelial transition and Wnt activation in cancer cells to promote bone metastasis (146). Furthermore, E-selectin plays a critical role in allowing breast cancer cells to infiltrate the bone marrow, and inhibition of the SDF-1/CXCR4 axis induces the mobilization of dormant cancer cells into circulation (147). Following lung-derived selectin inhibition, decreased lung migration and reduced proliferation were observed in TNBC through a 3D ex vivo pulmonary metastasis assay and lung-conditioned media in an in vitro model (148). In host mice, E- and P-selectin depletion inhibits intraperitoneal metastasis of PDA cells (149). P-selectin mediates platelet activation simultaneously in a combination of tissue factor-induced thrombin formation by pancreatic cancer cells (150) and breast cancer cells (151).
Selectins and CAM-DR
Selectins, especially E-selectin, are also involved in cancer drug resistance in AML. E-selectin promotes endocrine-related cancer cell adhesion, chemotaxis, trans-endothelial migration, and stroma-induced drug resistance. Blocking E-selectin results in cancer cell mobilization from the TME to circulation and resensitizes cancer cells to chemotherapies (152). For example, uproleselan (GMI-1271), an E-selectin antagonist, received FDA approval for adult relapsed/refractory AML in 2017 and a phase 3 study is ongoing (153). A preclinical study demonstrated that AML cells have high E-selectin binding potential with chemotherapy resistance, resulting in a high leukemia relapse incidence. Chemoresistance of AML cells was resensitized to chemotherapy by both E-selectin host knockout (sele-/-) and uproleselan (154). GMI-1359, a dual CXCR4/E-selectin antagonist, reduced growth, enhanced docetaxel treatment, and restored docetaxel effectiveness in docetaxel-resistant prostate cancer cells (155). High expression of E-selectin with substantial CD45+ immune cell density adjacency was observed in doxorubicin-treated residual human breast tumors. Moreover, the functional blockade of E-selectin with an anti-E-selectin aptamer decreased the residual tumor burden and metastasis by inhibiting the TH2 shift (156).
Since L-selectin assists lymphocyte homing to lymph nodes, OX40L promotes effector T-cell expansion and inhibits regulatory T cells. A type of intelligent exosome engineered with L-selectin and OX40L was developed in a previous study (157). The exosome surface was functionally engineered with CD62L (L-selectin, a gene for lymphocyte homing to lymph nodes) and OX40L (CD134L, a gene for effector T-cell expansion and regulatory T-cell inhibition) by forced expression of the genes in the donor cells. These engineered smart exosomes were tested, and an improved outcome was observed in a breast cancer 4T1 xenograft model (157). As ovarian cancer cells can bind to L-selectin in vitro, RO-heparin, which has low anticoagulant activity, can inhibit L-selectin-mediated cell adhesion and prevent cancer metastasis (158).
Granulocytes are crucial factors in cancer metastasis. Cancer-induced expansion of immunosuppressive myeloid-derived suppressor cells (MDSCs) has been well described. Lu et al. first reported that PSGL-1 is expressed on the surface of MDSCs in pancreatic tumor tissues (159). They found that low-molecular-weight heparin (LMWH) could attenuate the early stage of adhesion between vascular ECs and MDSCs by inhibiting P-selectin/PSGL-1 binding. Therefore, fewer MDSCs are recruited to pancreatic tumor tissues (159). Furthermore, they reported that hydrophilic LMWH attenuated lung metastasis and alleviated the immunosuppressive TME through P-selectin blockage, thereby decreasing the recruitment of MDSCs to the lung (160). In addition to MDSCs, aged neutrophils regulate metastasis (161). Compared to CXCR4 low/CD62L high immature neutrophils, aged neutrophils facilitate breast cancer migration and mediate metastasis through increased release of neutrophil extracellular traps (161).
Fucoidan, a sulfated polysaccharide, has shown a strong affinity towards P-selectin and is widely used for its anticoagulant, antitumor, and anti-inflammatory effects; therefore, fucoidan-Dox NPs (FU-Dox NPs) were developed. The MDA-MB-231 cell line with high P-selectin expression is more sensitive to FU-Dox NPs than the MDA-MB-468 cell line that exerts low P-selectin expression (162). Interestingly, P-selectin-mediated adhesion is upregulated by sulfatide in a breast cancer model. However, increased sulfatide synthesis sensitizes cancer cells to hypoxia and doxorubicin (163).
Integrin and Endocrine-Related Cancers
According to their bidirectional signaling character, integrins present two conformational states that determine the receptor affinity of ECM proteins: a bent integrin (inactive form) shows a low affinity for ECM ligands, whereas an extended integrin (active form) elicits the activation of downstream signaling (Figure 1A). Abnormal regulation of integrins impacts cancer cell and stroma crosstalk, maintains cancer stemness characteristics, facilitates the formation of metastases, and induces drug resistance (164, 165) (Figure 5). For example, Kim et al. systemically demonstrated integrin α4 and α6 mediated drug resistance in B-cell acute lymphoblastic leukemia, and either integrin α4 or α6 inhibition led to increased chemosensitivity (166–168).
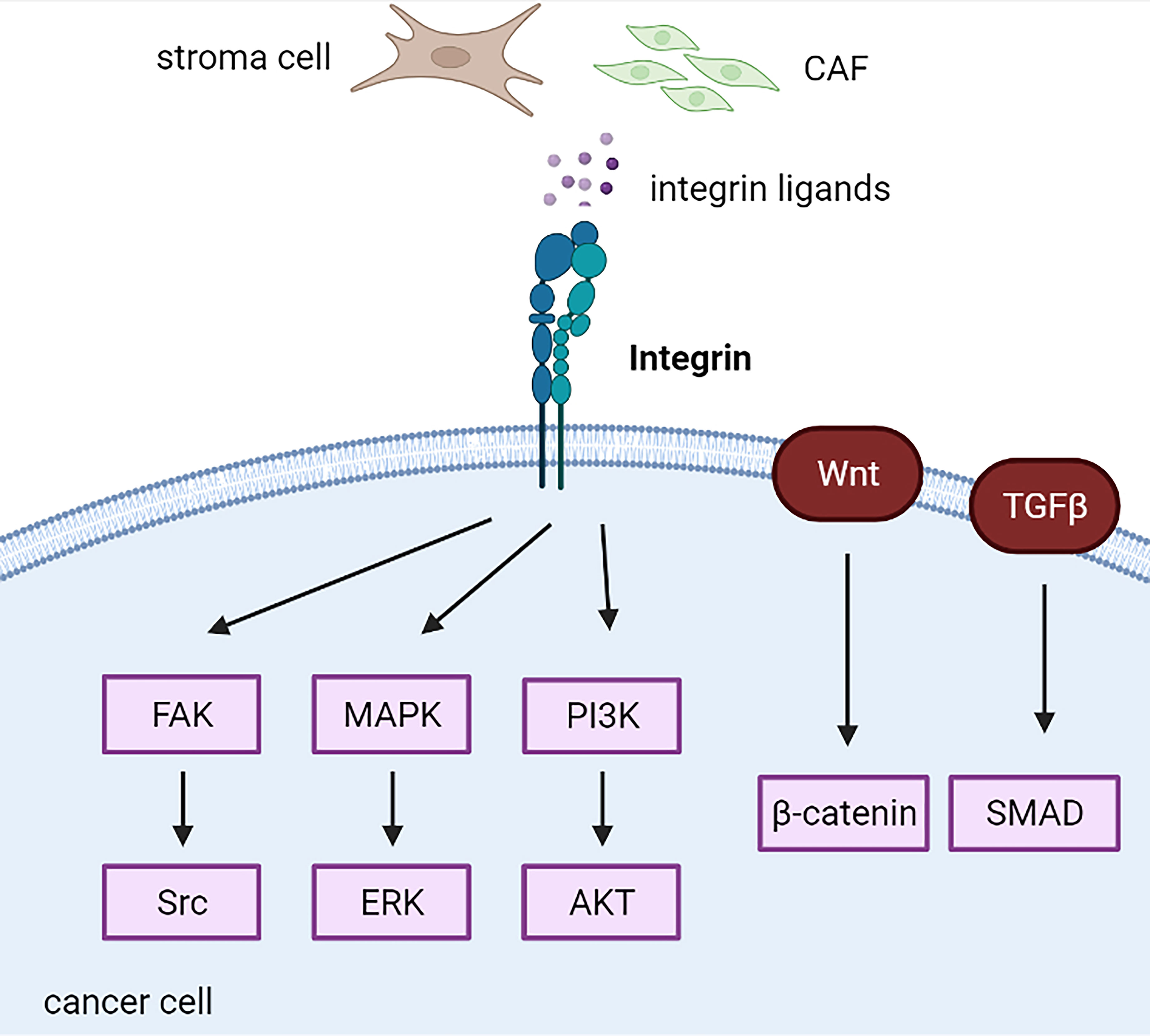
Figure 5 Integrin-related cascades in cancer cells. CAF, cancer-associated fibroblast; CSC, cancer stem cell.
Increased integrin expression mainly indicates poor outcomes in endocrine-related cancers. For example, integrin α3 is highly correlated with unfavorable prognosis in papillary thyroid carcinomas (169). Increased expression of integrins α5 and β1 is associated with the development of chemotherapy resistance and is an independent predictor of worse overall survival in epithelial ovarian cancer (170). Increased expression of integrin αV in PDAC cells has been associated with decreased patient survival (149). High expression of integrin α2β1 co-expressing HGFR or CD44v6 indicated worse progression-free survival in primary ovarian cancer and supported the hypothesis of mediating platinum resistance mediated by integrin α2β1 (171). Oxysterol-binding protein-like (OSBPL) family members potentially bound to integrins are highly upregulated in PDAC with poor outcomes in pancreatic ductal adenocarcinoma PDAC (172). Furthermore, increased integrin β1/lnc005620 regulated chemoresistance to epirubicin in TNBC in vitro and in vivo (139). However, integrin α3 is a favorable prognostic biomarker in breast cancer via integrin α3-ECM mediated immune cell infiltration (173). Increased integrin α5 expression induces lower aggressiveness in tamoxifen-resistant breast cancer (174).
Integrins and CSCs
Several studies have implicated integrins as vital regulators of CSCs. Integrin β1, specifically integrin α3β1, is necessary for mammary tumorigenesis (175–177). Apart from stemness, integrin β1 promotes proliferation by increasing the expression of TGF-β receptor 2 and kindlin-2 signaling in the MIA PaCa-2 pancreatic cancer cell line (178). Knockout of integrin β1 decreased the expression of integrin α5, which resulted in impaired cell metastasis and adhesion to vitronectin and fibronectin (178). Integrin α5β1-targeted micellar paclitaxel (ATN-MPTX) successfully selectively inhibited immunogenic cell death using chemo-immunotherapy in TNBC cells (132). Interestingly, downregulation of integrin β1 is compensated for by increased β3 integrin expression (179).
Integrin β3 regulates breast cancer stemness through the Wnt/β-catenin/HOXD3 pathway and mediates drug resistance (180, 181). In addition, integrin αvβ3 is a potential marker of breast and pancreatic cancers with stem-like properties and high resistance to receptor tyrosine kinase inhibitors, such as erlotinib and other chemotherapies, through the KRAS-RalB-NF-κB pathway and the mTOR/mTORC1 axis (182, 183). Moreover, integrin αvβ3 mediated PARP inhibitors’ resistance in breast cancer cells via the TGFBI-ZEB1 pathway using the CRISPR deletion method (184). Furthermore, TGFBI produced by macrophages during immunosuppression in early ovarian cancer cells may be an effector of the tumor-promoting factor TGF-β (185). Integrin αv activates latent TGF-β and drives EMT in PDAC cells (149). Similarly, integrin α6 induced EMT with the upregulation of N-cadherin and downregulation of CDH1 in ovarian cancer spheroids via the TGF-β1/Smad3 pathway (186). Interestingly, TGF-β1 promotes the expression of SMYD3 and ITGB6 as feedback mechanisms (186). Integrin α6 is enriched and enhances stem cell phenotypes in breast CSCs, especially in TNBC cells (187, 188). Integrin α2, a direct target of miR-206, promotes TNBC self-renewal-related mammosphere formation, where canonical Wnt signaling is involved (189). Recently, a study demonstrated that upregulation of integrin β8 mediated by lnc-TCF7 leads to ovarian cancer stemness (190). Integrin αvβ8, a key activator of TGF-β, regulates immunotherapy and radiotherapy resistance, which may be reversed by a potent blocking monoclonal antibody against integrin αvβ8 (ADWA-11) in prostate cancer (191). The anti-integrin β8 antibody treatment mechanism restores the in vitro cytotoxic effect of tumor CD8 T cells by inhibiting Treg cells (191, 192).
Integrins and FAK/Src/PI3K Axis
FAK is a downstream protein that is highly phosphorylated in response to integrin activation and contributes to cancer cell behaviors, including resistance to anoikis and maintenance of an immunosuppressive TME (193, 194). Therefore, FAK inhibition increased immune surveillance in immunosuppressive pancreatic ductal adenocarcinoma TME and restored tumor response to immunotherapy, such as PD-1 antagonists (194). Integrin β1/LRRC15 exerts a metastatic invasion role via activation of FAK in patient-derived ovarian cancer xenograft models, inhibited by ABBV-085, an antibody–drug conjugate of LRRC15 (195). Following binding to integrin, PCMT1 was released from ovarian cancer cells, leading to activated FAK-Src signaling to promote cancer progression and anoikis resistance (196). Moreover, stiffness-induced autophagy in stromal cells mediated by integrin αV-PTK-AMPKα promotes the growth of adjacent pancreatic stellate cells in vitro and in vivo (197). Integrins α3 and β5 were increased in highly metastatic breast cancer cell lines, such as MDA-MB-231 and MDA-MB-231BO, while decreased in poorly metastatic MCF-7 cells. Knockdown of integrin α3 and β5 leads to the inhibition of migration and invasion of breast cancer cells through the FAK/Src/Rac1 pathway (198). Increased integrin β1 contributes to resistance to anti-HER2 (trastuzumab or lapatinib) and anti-PI3K (pertuzumab) via the activation of FAK/Src signaling in HER2-positive breast cancer (199, 200).
Integrins also play an essential role in stimulating the activity of Ras, which in turn activates the MAPK/ERK and PI3K/Akt signaling pathways (201, 202). Both the MAPK/ERK and PI3K/Akt signaling pathways are critical cascades that regulate cancer cell proliferation, survival, and drug resistance, which have been well investigated in the past decades (203). CAF-derived THBS2 bound to integrin αvβ3 promotes cancer cell growth and adhesion via the MAPK pathway in PDAC cells in vitro and in vivo (204). In addition, serial studies have implicated that inhibition of integrins efficiently sensitizes breast cancer cells to conventional therapies, such as radiotherapy. Integrin β1 inhibition by AIIB2 in combination with post-ionizing radiation increases apoptosis compared with radiation alone by downregulating Akt in breast cancer (205). Integrin α6 mediates radiation resistance via PI3K/Akt and MEK/ERK signaling (206). Therefore, the PI3K inhibitor LY294002 and MEK inhibitor U0126 reverse radiation resistance was mediated by integrin α6 in breast cancer cells (206). Conversely, integrin α6β1 mediates resistance to PI3K inhibitors in PTEN-negative prostate cancer by inducing PIM kinases and oxidative stress (207). Recently, the upregulation of IGF1R-integrin α6-S100A4 signaling was found to promote chemoresistance to metastasis of epithelial ovarian cancer cells (208). S100A4 is secreted from lung fibroblasts and can reciprocally activate them (208). Integrin β1 is regulated by cysteine-rich angiogenic inducer 61 (CYR61), a matricellular protein, in TNBC (209). In addition, CYR61 integrin β1-AMPKα mediates lung metastasis, anoikis resistance, and extravasation of TNBC cells in an AKT-, FAK-, and ERK1/2-independent manner (209).
Outlook
CAMs contribute to the attachment of cancer cells to the TME via cell-to-cell and cell-to-ECM interactions. Remodeled TME mediates drug resistance in cancer cells, resulting in altered expression of CAMs (Figure 6). Therefore, CAMs may be an attractive therapeutic target for cancer intervention. Further mechanisms of CAM-DR in endocrine-related cancers are warranted to open new avenues for more effective treatments in the future.
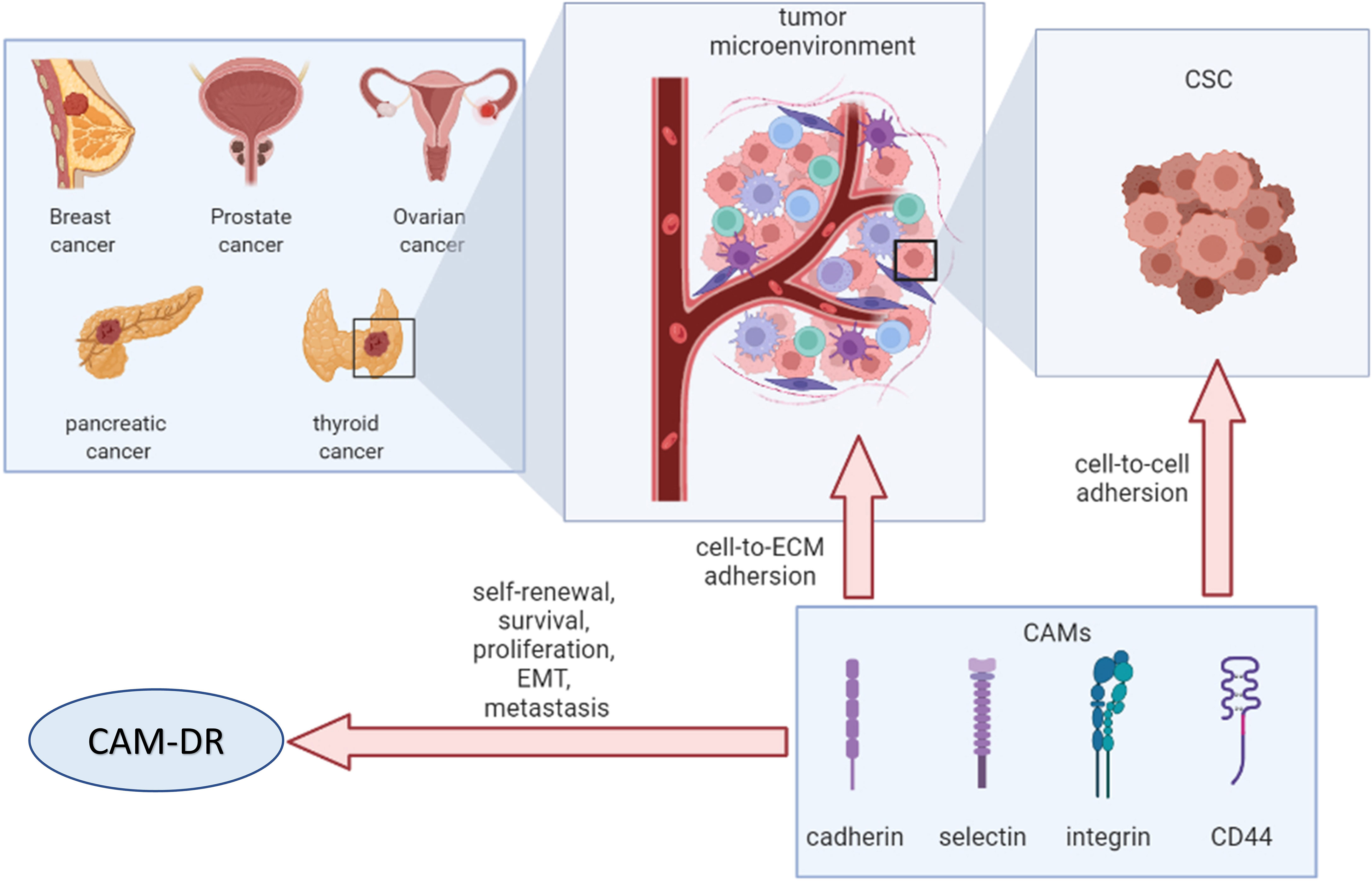
Figure 6 Overview of cell adhesion molecules in endocrine-related cancers. CSC, cancer stem cell; ECM, extracellular matrix; CAMs, cell adhesion molecules; EMT, epithelial–mesenchymal transition; CAM-DR, cell adhesion-mediated drug resistance.
Author Contributions
YR conceptualized the study. YR, LC, and DX wrote the original draft. YR, LC, DX, XF, and XW wrote, reviewed, and edited the manuscript. YR and XW supervised the study and YR acquired funding. All authors have contributed to the manuscript and approved the submitted version.
Funding
This study was supported by the GuangDong Basic and Applied Basic Research Foundation (Grant No.2022A1515010012), the President Foundation of Nanfang Hospital, Southern Medical University (Grant No. 2020C003), and the State Scholarship Fund from the China Scholarship Council (File No. 201809315008).
Conflict of Interest
The authors declare that the research was conducted in the absence of any commercial or financial relationships that could be construed as a potential conflict of interest.
Publisher’s Note
All claims expressed in this article are solely those of the authors and do not necessarily represent those of their affiliated organizations, or those of the publisher, the editors and the reviewers. Any product that may be evaluated in this article, or claim that may be made by its manufacturer, is not guaranteed or endorsed by the publisher.
Acknowledgments
All figures were created with https://biorender.com/.
References
1. Siegel RL, Miller KD, Fuchs HE, Jemal A. Cancer Statistics, 2021. CA Cancer J Clin (2021) 71(1):7–33. doi: 10.3322/caac.21654
3. Foulkes WD, Smith IE, Reis-Filho JS. Triple-Negative Breast Cancer. N Engl J Med (2010) 363(20):1938–48. doi: 10.1056/NEJMra1001389
4. Retecki K, Seweryn M, Graczyk-Jarzynka A, Bajor M. The Immune Landscape of Breast Cancer: Strategies for Overcoming Immunotherapy Resistance. Cancers (Basel) (2021) 13(23):6012. doi: 10.3390/cancers13236012
5. O’Shea AS. Clinical Staging of Ovarian Cancer. Methods Mol Biol (2022) 2424:3–10. doi: 10.1007/978-1-0716-1956-8_1
6. Lengyel E. Ovarian Cancer Development and Metastasis. Am J Pathol (2010) 177(3):1053–64. doi: 10.2353/ajpath.2010.100105
7. Park W, Chawla A, O’Reilly EM. Pancreatic Cancer: A Review. JAMA (2021) 326(9):851–62. doi: 10.1001/jama.2021.13027
8. Sandhu S, Moore CM, Chiong E, Beltran H, Bristow RG, Williams SG. Prostate Cancer. Lancet (2021) 398(10305):1075–90. doi: 10.1016/S0140-6736(21)00950-8
9. Valerio L, Pieruzzi L, Giani C, Agate L, Bottici V, Lorusso L, et al. Targeted Therapy in Thyroid Cancer: State of the Art. Clin Oncol (R Coll Radiol) (2017) 29(5):316–24. doi: 10.1016/j.clon.2017.02.009
10. Coperchini F, Croce L, Marinò M, Chiovato L, Rotondi M. Role of Chemokine Receptors in Thyroid Cancer and Immunotherapy. Endocr Relat Cancer (2019) 26(8):R465–R78. doi: 10.1530/ERC-19-0163
11. Makrilia N, Kollias A, Manolopoulos L, Syrigos K. Cell Adhesion Molecules: Role and Clinical Significance in Cancer. Cancer Invest (2009) 27(10):1023–37. doi: 10.3109/07357900902769749
12. Frenette PS, Wagner DD. Adhesion Molecules–Part 1. N Engl J Med (1996) 334(23):1526–9. doi: 10.1056/NEJM199606063342308
13. Kim HN, Ruan Y, Ogana H, Kim YM. Cadherins, Selectins, and Integrins in Cam-Dr in Leukemia. Front Oncol (2020) 10:592733. doi: 10.3389/fonc.2020.592733
14. Hassn Mesrati M, Syafruddin SE, Mohtar MA, Syahir A. Cd44: A Multifunctional Mediator of Cancer Progression. Biomolecules (2021) 11(12):1850. doi: 10.3390/biom11121850
15. Windisch R, Pirschtat N, Kellner C, Chen-Wichmann L, Lausen J, Humpe A, et al. Oncogenic Deregulation of Cell Adhesion Molecules in Leukemia. Cancers (Basel) (2019) 11(3):311. doi: 10.3390/cancers11030311
16. Janiszewska M, Primi MC, Izard T. Cell Adhesion in Cancer: Beyond the Migration of Single Cells. J Biol Chem (2020) 295(8):2495–505. doi: 10.1074/jbc.REV119.007759
17. Huang Y, Wang Y, Tang J, Qin S, Shen X, He S, et al. Cam-Dr: Mechanisms, Roles and Clinical Application in Tumors. Front Cell Dev Biol (2021) 9:698047. doi: 10.3389/fcell.2021.698047
18. Bachmann M, Kukkurainen S, Hytonen VP, Wehrle-Haller B. Cell Adhesion by Integrins. Physiol Rev (2019) 99(4):1655–99. doi: 10.1152/physrev.00036.2018
19. Moreno-Layseca P, Icha J, Hamidi H, Ivaska J. Integrin Trafficking in Cells and Tissues. Nat Cell Biol (2019) 21(2):122–32. doi: 10.1038/s41556-018-0223-z
20. Hynes RO. Integrins: Bidirectional, Allosteric Signaling Machines. Cell (2002) 110(6):673–87. doi: 10.1016/s0092-8674(02)00971-6
21. Aksorn N, Chanvorachote P. Integrin as a Molecular Target for Anti-Cancer Approaches in Lung Cancer. Anticancer Res (2019) 39(2):541–8. doi: 10.21873/anticanres.13146
22. Shapiro L, Weis WI. Structure and Biochemistry of Cadherins and Catenins. Cold Spring Harb Perspect Biol (2009) 1(3):a003053. doi: 10.1101/cshperspect.a003053
23. Shashikanth N, Petrova YI, Park S, Chekan J, Maiden S, Spano M, et al. Allosteric Regulation of E-Cadherin Adhesion. J Biol Chem (2015) 290(35):21749–61. doi: 10.1074/jbc.M115.657098
24. Patel KD, Cuvelier SL, Wiehler S. Selectins: Critical Mediators of Leukocyte Recruitment. Semin Immunol (2002) 14(2):73–81. doi: 10.1006/smim.2001.0344
25. Borsig L. Selectins in Cancer Immunity. Glycobiology (2018) 28(9):648–55. doi: 10.1093/glycob/cwx105
26. Cummings RD, Smith DF. The Selectin Family of Carbohydrate-Binding Proteins: Structure and Importance of Carbohydrate Ligands for Cell Adhesion. Bioessays (1992) 14(12):849–56. doi: 10.1002/bies.950141210
27. Springer TA. Adhesion Receptors of the Immune System. Nature (1990) 346(6283):425–34. doi: 10.1038/346425a0
28. Kansas GS. Selectins and Their Ligands: Current Concepts and Controversies. Blood (1996) 88(9):3259–87. doi: 10.1182/blood.V88.9.3259.bloodjournal8893259
29. Goodison S, Urquidi V, Tarin D. Cd44 Cell Adhesion Molecules. Mol Pathol (1999) 52(4):189–96. doi: 10.1136/mp.52.4.189
30. Mishra MN, Chandavarkar V, Sharma R, Bhargava D. Structure, Function and Role of Cd44 in Neoplasia. J Oral Maxillofac Pathol (2019) 23(2):267–72. doi: 10.4103/jomfp.JOMFP_246_18
31. Huet S, Groux H, Caillou B, Valentin H, Prieur AM, Bernard A. Cd44 Contributes to T Cell Activation. J Immunol (1989) 143(3):798–801.
32. Trochon V, Mabilat C, Bertrand P, Legrand Y, Smadja-Joffe F, Soria C, et al. Evidence of Involvement of Cd44 in Endothelial Cell Proliferation, Migration and Angiogenesis In Vitro. Int J Cancer (1996) 66(5):664–8. doi: 10.1002/(SICI)1097-0215(19960529)66:5<664::AID-IJC14>3.0.CO;2-4
33. Ruan Y, Ogana H, Gang E, Kim HN, Kim Y-M. Wnt Signaling in the Tumor Microenvironment. Adv Exp Med Biol (2021) 1270:107–21. doi: 10.1007/978-3-030-47189-7_7
34. Clarke MF, Dick JE, Dirks PB, Eaves CJ, Jamieson CH, Jones DL, et al. Cancer Stem Cells–Perspectives on Current Status and Future Directions: Aacr Workshop on Cancer Stem Cells. Cancer Res (2006) 66(19):9339–44. doi: 10.1158/0008-5472.CAN-06-3126
35. Senel F, Kokenek Unal TD, Karaman H, Inanc M, Aytekin A. Prognostic Value of Cancer Stem Cell Markers Cd44 and Aldh1/2 in Gastric Cancer Cases. Asian Pac J Cancer Prev (2017) 18(9):2527–31. doi: 10.22034/APJCP.2017.18.9.2527
36. Ailles LE, Weissman IL. Cancer Stem Cells in Solid Tumors. Curr Opin Biotechnol (2007) 18(5):460–6. doi: 10.1016/j.copbio.2007.10.007
37. Al-Hajj M, Wicha MS, Benito-Hernandez A, Morrison SJ, Clarke MF. Prospective Identification of Tumorigenic Breast Cancer Cells. Proc Natl Acad Sci USA (2003) 100(7):3983–8. doi: 10.1073/pnas.0530291100
38. Basakran NS. Cd44 as a Potential Diagnostic Tumor Marker. Saudi Med J (2015) 36(3):273–9. doi: 10.15537/smj.2015.3.9622
39. Afify A, Purnell P, Nguyen L. Role of Cd44s and Cd44v6 on Human Breast Cancer Cell Adhesion, Migration, and Invasion. Exp Mol Pathol (2009) 86(2):95–100. doi: 10.1016/j.yexmp.2008.12.003
40. Chaffer CL, San Juan BP, Lim E, Weinberg RA. Emt, Cell Plasticity and Metastasis. Cancer Metastasis Rev (2016) 35(4):645–54. doi: 10.1007/s10555-016-9648-7
41. Dongre A, Weinberg RA. New Insights Into the Mechanisms of Epithelial-Mesenchymal Transition and Implications for Cancer. Nat Rev Mol Cell Biol (2019) 20(2):69–84. doi: 10.1038/s41580-018-0080-4
42. Xu J, Lamouille S, Derynck R. Tgf-Beta-Induced Epithelial to Mesenchymal Transition. Cell Res (2009) 19(2):156–72. doi: 10.1038/cr.2009.5
43. Lamouille S, Xu J, Derynck R. Molecular Mechanisms of Epithelial-Mesenchymal Transition. Nat Rev Mol Cell Biol (2014) 15(3):178–96. doi: 10.1038/nrm3758
44. Guo W, Keckesova Z, Donaher JL, Shibue T, Tischler V, Reinhardt F, et al. Slug and Sox9 Cooperatively Determine the Mammary Stem Cell State. Cell (2012) 148(5):1015–28. doi: 10.1016/j.cell.2012.02.008
45. Lim S, Becker A, Zimmer A, Lu J, Buettner R, Kirfel J. Snai1-Mediated Epithelial-Mesenchymal Transition Confers Chemoresistance and Cellular Plasticity by Regulating Genes Involved in Cell Death and Stem Cell Maintenance. PloS One (2013) 8(6):e66558. doi: 10.1371/journal.pone.0066558
46. Lambert AW, Pattabiraman DR, Weinberg RA. Emerging Biological Principles of Metastasis. Cell (2017) 168(4):670–91. doi: 10.1016/j.cell.2016.11.037
47. Ilina O, Friedl P. Mechanisms of Collective Cell Migration at a Glance. J Cell Sci (2009) 122(Pt 18):3203–8. doi: 10.1242/jcs.036525
48. Oliveira-Ferrer L, Rossler K, Haustein V, Schroder C, Wicklein D, Maltseva D, et al. C-Fos Suppresses Ovarian Cancer Progression by Changing Adhesion. Br J Cancer (2014) 110(3):753–63. doi: 10.1038/bjc.2013.774
49. Carroll MJ, Fogg KC, Patel HA, Krause HB, Mancha AS, Patankar MS, et al. Alternatively-Activated Macrophages Upregulate Mesothelial Expression of P-Selectin to Enhance Adhesion of Ovarian Cancer Cells. Cancer Res (2018) 78(13):3560–73. doi: 10.1158/0008-5472.CAN-17-3341
50. Gebauer F, Wicklein D, Stubke K, Nehmann N, Schmidt A, Salamon J, et al. Selectin Binding Is Essential for Peritoneal Carcinomatosis in a Xenograft Model of Human Pancreatic Adenocarcinoma in Pfp–/Rag2– Mice. Gut (2013) 62(5):741–50. doi: 10.1136/gutjnl-2011-300629
51. Yeon JH, Jeong HE, Seo H, Cho S, Kim K, Na D, et al. Cancer-Derived Exosomes Trigger Endothelial to Mesenchymal Transition Followed by the Induction of Cancer-Associated Fibroblasts. Acta Biomater (2018) 76:146–53. doi: 10.1016/j.actbio.2018.07.001
52. Bochet L, Lehuede C, Dauvillier S, Wang YY, Dirat B, Laurent V, et al. Adipocyte-Derived Fibroblasts Promote Tumor Progression and Contribute to the Desmoplastic Reaction in Breast Cancer. Cancer Res (2013) 73(18):5657–68. doi: 10.1158/0008-5472.CAN-13-0530
53. Ning X, Zhang H, Wang C, Song X. Exosomes Released by Gastric Cancer Cells Induce Transition of Pericytes Into Cancer-Associated Fibroblasts. Med Sci Monit (2018) 24:2350–9. doi: 10.12659/msm.906641
54. Raz Y, Cohen N, Shani O, Bell RE, Novitskiy SV, Abramovitz L, et al. Bone Marrow-Derived Fibroblasts Are a Functionally Distinct Stromal Cell Population in Breast Cancer. J Exp Med (2018) 215(12):3075–93. doi: 10.1084/jem.20180818
55. Primac I, Maquoi E, Blacher S, Heljasvaara R, Van Deun J, Smeland HY, et al. Stromal Integrin Alpha11 Regulates Pdgfr-Beta Signaling and Promotes Breast Cancer Progression. J Clin Invest (2019) 129(11):4609–28. doi: 10.1172/JCI125890
56. White DE, Kurpios NA, Zuo D, Hassell JA, Blaess S, Mueller U, et al. Targeted Disruption of Beta1-Integrin in a Transgenic Mouse Model of Human Breast Cancer Reveals an Essential Role in Mammary Tumor Induction. Cancer Cell (2004) 6(2):159–70. doi: 10.1016/j.ccr.2004.06.025
57. Lin J, Ding D. The Prognostic Role of the Cancer Stem Cell Marker Cd44 in Ovarian Cancer: A Meta-Analysis. Cancer Cell Int (2017) 17:8. doi: 10.1186/s12935-016-0376-4
58. Chen C, Zhao S, Karnad A, Freeman JW. The Biology and Role of Cd44 in Cancer Progression: Therapeutic Implications. J Hematol Oncol (2018) 11(1):64. doi: 10.1186/s13045-018-0605-5
59. Long J, Zhang Y, Yu X, Yang J, LeBrun DG, Chen C, et al. Overcoming Drug Resistance in Pancreatic Cancer. Expert Opin Ther Targets (2011) 15(7):817–28. doi: 10.1517/14728222.2011.566216
60. Zöller M. Cd44, Hyaluronan, the Hematopoietic Stem Cell, and Leukemia-Initiating Cells. Front Immunol (2015) 6:235. doi: 10.3389/fimmu.2015.00235
61. Price ZK, Lokman NA, Ricciardelli C. Differing Roles of Hyaluronan Molecular Weight on Cancer Cell Behavior and Chemotherapy Resistance. Cancers (2018) 10(12):482. doi: 10.3390/cancers10120482
62. Li X, Lewis MT, Huang J, Gutierrez C, Osborne CK, Wu M-F, et al. Intrinsic Resistance of Tumorigenic Breast Cancer Cells to Chemotherapy. J Natl Cancer Inst (2008) 100(9):672–9. doi: 10.1093/jnci/djn123
63. Cheng W, Liu T, Wan X, Gao Y, Wang H. Microrna-199a Targets Cd44 to Suppress the Tumorigenicity and Multidrug Resistance of Ovarian Cancer-Initiating Cells. FEBS J (2012) 279(11):2047–59. doi: 10.1111/j.1742-4658.2012.08589.x
64. Zhang H, Brown RL, Wei Y, Zhao P, Liu S, Liu X, et al. Cd44 Splice Isoform Switching Determines Breast Cancer Stem Cell State. Genes Dev (2019) 33(3-4):166–79. doi: 10.1101/gad.319889.118
65. Nagano O, Okazaki S, Saya H. Redox Regulation in Stem-Like Cancer Cells by Cd44 Variant Isoforms. Oncogene (2013) 32(44):5191–8. doi: 10.1038/onc.2012.638
66. Nallasamy P, Nimmakayala RK, Karmakar S, Leon F, Seshacharyulu P, Lakshmanan I, et al. Pancreatic Tumor Microenvironment Factor Promotes Cancer Stemness Via Spp1-Cd44 Axis. Gastroenterology (2021) 161(6):1998–2013. doi: 10.1053/j.gastro.2021.08.023
67. Ryoo I-G, Choi B-H, Ku S-K, Kwak M-K. High Cd44 Expression Mediates P62-Associated Nfe2l2/Nrf2 Activation in Breast Cancer Stem Cell-Like Cells: Implications for Cancer Stem Cell Resistance. Redox Biol (2018) 17:246–58. doi: 10.1016/j.redox.2018.04.015
68. Sarkar A, Chanda A, Regmi SC, Karve K, Deng L, Jay GD, et al. Recombinant Human Prg4 (Rhprg4) Suppresses Breast Cancer Cell Invasion by Inhibiting Tgfβ-Hyaluronan-Cd44 Signalling Pathway. PloS One (2019) 14(7):e0219697. doi: 10.1371/journal.pone.0219697
69. Kong T, Ahn R, Yang K, Zhu X, Fu Z, Morin G, et al. Cd44 Promotes Pd-L1 Expression and Its Tumor-Intrinsic Function in Breast and Lung Cancers. Cancer Res (2020) 80(3):444–57. doi: 10.1158/0008-5472.CAN-19-1108
70. Bourguignon LYW, Peyrollier K, Xia W, Gilad E. Hyaluronan-Cd44 Interaction Activates Stem Cell Marker Nanog, Stat-3-Mediated Mdr1 Gene Expression, and Ankyrin-Regulated Multidrug Efflux in Breast and Ovarian Tumor Cells. J Biol Chem (2008) 283(25):17635–51. doi: 10.1074/jbc.M800109200
71. Bourguignon LYW, Spevak CC, Wong G, Xia W, Gilad E. Hyaluronan-Cd44 Interaction With Protein Kinase C(Epsilon) Promotes Oncogenic Signaling by the Stem Cell Marker Nanog and the Production of Microrna-21, Leading to Down-Regulation of the Tumor Suppressor Protein Pdcd4, Anti-Apoptosis, and Chemotherapy Resistance in Breast Tumor Cells. J Biol Chem (2009) 284(39):26533–46. doi: 10.1074/jbc.M109.027466
72. Misra S, Ghatak S, Toole BP. Regulation of Mdr1 Expression and Drug Resistance by a Positive Feedback Loop Involving Hyaluronan, Phosphoinositide 3-Kinase, and Erbb2. J Biol Chem (2005) 280(21):20310–5. doi: 10.1074/jbc.M500737200
73. Hong SP, Wen J, Bang S, Park S, Song SY. Cd44-Positive Cells Are Responsible for Gemcitabine Resistance in Pancreatic Cancer Cells. Int J Cancer (2009) 125(10):2323–31. doi: 10.1002/ijc.24573
74. Yang X, Iyer AK, Singh A, Choy E, Hornicek FJ, Amiji MM, et al. Mdr1 Sirna Loaded Hyaluronic Acid-Based Cd44 Targeted Nanoparticle Systems Circumvent Paclitaxel Resistance in Ovarian Cancer. Sci Rep (2015) 5:8509. doi: 10.1038/srep08509
75. Kesharwani P, Banerjee S, Padhye S, Sarkar FH, Iyer AK. Hyaluronic Acid Engineered Nanomicelles Loaded With 3,4-Difluorobenzylidene Curcumin for Targeted Killing of Cd44+ Stem-Like Pancreatic Cancer Cells. Biomacromolecules (2015) 16(9):3042–53. doi: 10.1021/acs.biomac.5b00941
76. Wang X, Xiong T, Cui M, Li N, Li Q, Zhu L, et al. A Novel Targeted Co-Delivery Nanosystem for Enhanced Ovarian Cancer Treatment Via Multidrug Resistance Reversion and Mtor-Mediated Signaling Pathway. J Nanobiotechnol (2021) 19(1):444. doi: 10.1186/s12951-021-01139-1
77. Ni J, Cozzi PJ, Hao JL, Beretov J, Chang L, Duan W, et al. Cd44 Variant 6 Is Associated With Prostate Cancer Metastasis and Chemo-/Radioresistance. Prostate (2014) 74(6):602–17. doi: 10.1002/pros.22775
78. Xie Z, Gao Y, Ho C, Li L, Jin C, Wang X, et al. Exosome-Delivered Cd44v6/C1qbp Complex Drives Pancreatic Cancer Liver Metastasis by Promoting Fibrotic Liver Microenvironment. Gut (2021) 71(3):568–79. doi: 10.1136/gutjnl-2020-323014
79. Liu X, Xie P, Hao N, Zhang M, Liu Y, Liu P, et al. Hif-1-Regulated Expression of Calreticulin Promotes Breast Tumorigenesis and Progression Through Wnt/β-Catenin Pathway Activation. Proc Natl Acad Sci USA (2021) 118(44):e2109144118. doi: 10.1073/pnas.2109144118
80. Cao L, Luo F-F, Huang H-B, Huang T-J, Hu H, Zheng L-S, et al. The Autoregulatory Serglycin/Cd44 Axis Drives Stemness-Like Phenotypes in Tnbc in a β-Catenin-Dependent Manner. Clin Transl Med (2021) 11(2):e311. doi: 10.1002/ctm2.311
81. Ruan Y, Kim HN, Ogana H, Kim Y-M. Wnt Signaling in Leukemia and Its Bone Marrow Microenvironment. Int J Mol Sci (2020) 21(17):6247. doi: 10.3390/ijms21176247
82. Yu P, Zhou L, Ke W, Li K. Clinical Significance of Pakt and Cd44v6 Overexpression With Breast Cancer. J Cancer Res Clin Oncol (2010) 136(8):1283–92. doi: 10.1007/s00432-010-0779-x
83. Qian J, LeSavage BL, Hubka KM, Ma C, Natarajan S, Eggold JT, et al. Cancer-Associated Mesothelial Cells Promote Ovarian Cancer Chemoresistance Through Paracrine Osteopontin Signaling. J Clin Invest (2021) 131(16):e146186. doi: 10.1172/JCI146186
84. Desai B, Rogers MJ, Chellaiah MA. Mechanisms of Osteopontin and Cd44 as Metastatic Principles in Prostate Cancer Cells. Mol Cancer (2007) 6:18. doi: 10.1186/1476-4598-6-18
85. Li X-P, Zhang X-W, Zheng L-Z, Guo W-J. Expression of Cd44 in Pancreatic Cancer and Its Significance. Int J Clin Exp Pathol (2015) 8(6):6724–31.
86. Jang J-H, Kim D-H, Lim JM, Lee JW, Jeong SJ, Kim KP, et al. Breast Cancer Cell-Derived Soluble Cd44 Promotes Tumor Progression by Triggering Macrophage Il1β Production. Cancer Res (2020) 80(6):1342–56. doi: 10.1158/0008-5472.CAN-19-2288
87. Zhang L, Qiang J, Yang X, Wang D, Rehman AU, He X, et al. Il1r2 Blockade Suppresses Breast Tumorigenesis and Progression by Impairing Usp15-Dependent Bmi1 Stability. Advanced Sci (Weinheim Baden-Wurttemberg Germany) (2020) 7(1):1901728. doi: 10.1002/advs.201901728
88. Xie G, Yao Q, Liu Y, Du S, Liu A, Guo Z, et al. Il-6-Induced Epithelial-Mesenchymal Transition Promotes the Generation of Breast Cancer Stem-Like Cells Analogous to Mammosphere Cultures. Int J Oncol (2012) 40(4):1171–9. doi: 10.3892/ijo.2011.1275
89. Burandt E, Lubbersmeyer F, Gorbokon N, Buscheck F, Luebke AM, Menz A, et al. E-Cadherin Expression in Human Tumors: A Tissue Microarray Study on 10,851 Tumors. Biomark Res (2021) 9(1):44. doi: 10.1186/s40364-021-00299-4
90. Acuna RA, Varas-Godoy M, Herrera-Sepulveda D, Retamal MA. Connexin46 Expression Enhances Cancer Stem Cell and Epithelial-To-Mesenchymal Transition Characteristics of Human Breast Cancer Mcf-7 Cells. Int J Mol Sci (2021) 22(22):12604. doi: 10.3390/ijms222212604
91. Corso G, Figueiredo J, De Angelis SP, Corso F, Girardi A, Pereira J, et al. E-Cadherin Deregulation in Breast Cancer. J Cell Mol Med (2020) 24(11):5930–6. doi: 10.1111/jcmm.15140
92. Hanby AM, Hughes TA. In Situ and Invasive Lobular Neoplasia of the Breast. Histopathology (2008) 52(1):58–66. doi: 10.1111/j.1365-2559.2007.02891.x
93. Droufakou S, Deshmane V, Roylance R, Hanby A, Tomlinson I, Hart IR. Multiple Ways of Silencing E-Cadherin Gene Expression in Lobular Carcinoma of the Breast. Int J Cancer (2001) 92(3):404–8. doi: 10.1002/ijc.1208
94. De Leeuw WJ, Berx G, Vos CB, Peterse JL, Van de Vijver MJ, Litvinov S, et al. Simultaneous Loss of E-Cadherin and Catenins in Invasive Lobular Breast Cancer and Lobular Carcinoma In Situ. J Pathol (1997) 183(4):404–11. doi: 10.1002/(SICI)1096-9896(199712)183:4<404::AID-PATH1148>3.0.CO;2-9
95. Huiping C, Sigurgeirsdottir JR, Jonasson JG, Eiriksdottir G, Johannsdottir JT, Egilsson V, et al. Chromosome Alterations and E-Cadherin Gene Mutations in Human Lobular Breast Cancer. Br J Cancer (1999) 81(7):1103–10. doi: 10.1038/sj.bjc.6690815
96. Berx G, Cleton-Jansen AM, Strumane K, de Leeuw WJ, Nollet F, van Roy F, et al. E-Cadherin Is Inactivated in a Majority of Invasive Human Lobular Breast Cancers by Truncation Mutations Throughout Its Extracellular Domain. Oncogene (1996) 13(9):1919–25.
97. Mielnicki LM, Asch HL, Asch BB. Genes, Chromatin, and Breast Cancer: An Epigenetic Tale. J Mammary Gland Biol Neoplasia (2001) 6(2):169–82. doi: 10.1023/a:1011356623442
98. Bhagat R, Premalata CS, Shilpa V, Pallavi VR, Ramesh G, Vijay CR, et al. Altered Expression of Beta-Catenin, E-Cadherin, and E-Cadherin Promoter Methylation in Epithelial Ovarian Carcinoma. Tumour Biol (2013) 34(4):2459–68. doi: 10.1007/s13277-013-0797-9
99. Vajaria BN, Patel PS. Glycosylation: A Hallmark of Cancer? Glycoconj J (2017) 34(2):147–56. doi: 10.1007/s10719-016-9755-2
100. Pinho SS, Seruca R, Gartner F, Yamaguchi Y, Gu J, Taniguchi N, et al. Modulation of E-Cadherin Function and Dysfunction by N-Glycosylation. Cell Mol Life Sci (2011) 68(6):1011–20. doi: 10.1007/s00018-010-0595-0
101. Zhu X, Wang X, Gong Y, Deng J. E-Cadherin on Epithelial-Mesenchymal Transition in Thyroid Cancer. Cancer Cell Int (2021) 21(1):695. doi: 10.1186/s12935-021-02344-6
102. Kvokackova B, Remsik J, Jolly MK, Soucek K. Phenotypic Heterogeneity of Triple-Negative Breast Cancer Mediated by Epithelial-Mesenchymal Plasticity. Cancers (Basel) (2021) 13(9):2188. doi: 10.3390/cancers13092188
103. Loret N, Denys H, Tummers P, Berx G. The Role of Epithelial-To-Mesenchymal Plasticity in Ovarian Cancer Progression and Therapy Resistance. Cancers (Basel) (2019) 11(6):838. doi: 10.3390/cancers11060838
104. Buechel D, Sugiyama N, Rubinstein N, Saxena M, Kalathur RKR, Luond F, et al. Parsing Beta-Catenin’s Cell Adhesion and Wnt Signaling Functions in Malignant Mammary Tumor Progression. Proc Natl Acad Sci USA (2021) 118(34):e2020227118. doi: 10.1073/pnas.2020227118
105. Bai X, Jiang X, Liu Y, Wang Y, Jiang X, Song G, et al. Kruppel-Like Factor 9 Upregulates E-Cadherin Transcription and Represses Breast Cancer Invasion and Metastasis. Am J Cancer Res (2021) 11(7):3660–73.
106. Choi S, Yu J, Kim W, Park KS. N-Cadherin Mediates the Migration of Bone Marrow-Derived Mesenchymal Stem Cells Toward Breast Tumor Cells. Theranostics (2021) 11(14):6786–99. doi: 10.7150/thno.59703
107. Bahcecioglu G, Yue X, Howe E, Guldner I, Stack MS, Nakshatri H, et al. Aged Breast Extracellular Matrix Drives Mammary Epithelial Cells to an Invasive and Cancer-Like Phenotype. Adv Sci (Weinh) (2021) 8(22):e2100128. doi: 10.1002/advs.202100128
108. Hapach LA, Carey SP, Schwager SC, Taufalele PV, Wang W, Mosier JA, et al. Phenotypic Heterogeneity and Metastasis of Breast Cancer Cells. Cancer Res (2021) 81(13):3649–63. doi: 10.1158/0008-5472.CAN-20-1799
109. Yang X, Shang P, Yu B, Jin Q, Liao J, Wang L, et al. Combination Therapy With Mir34a and Doxorubicin Synergistically Inhibits Dox-Resistant Breast Cancer Progression Via Down-Regulation of Snail Through Suppressing Notch/Nf-Kappab and Ras/Raf/Mek/Erk Signaling Pathway. Acta Pharm Sin B (2021) 11(9):2819–34. doi: 10.1016/j.apsb.2021.06.003
110. Khajah MA, Khushaish S, Luqmani YA. Lactate Dehydrogenase a or B Knockdown Reduces Lactate Production and Inhibits Breast Cancer Cell Motility In Vitro. Front Pharmacol (2021) 12:747001. doi: 10.3389/fphar.2021.747001
111. Zhu M, Tang X, Gong Z, Tang W, Zhang Y. Tad1822-7 Induces Ros-Mediated Apoptosis of Her2 Positive Breast Cancer by Decreasing E-Cadherin in an Ephb4 Dependent Manner. Life Sci (2021) 285:119954. doi: 10.1016/j.lfs.2021.119954
112. Yang Y, Zhao B, Lv L, Yang Y, Li S, Wu H. Fbxl10 Promotes Emt and Metastasis of Breast Cancer Cells Via Regulating the Acetylation and Transcriptional Activity of Snai1. Cell Death Discov (2021) 7(1):328. doi: 10.1038/s41420-021-00722-7
113. Qu J, Li J, Zhang Y, He R, Liu X, Gong K, et al. Akr1b10 Promotes Breast Cancer Cell Proliferation and Migration Via the Pi3k/Akt/Nf-Kappab Signaling Pathway. Cell Biosci (2021) 11(1):163. doi: 10.1186/s13578-021-00677-3
114. Clarke LE, Cook A, Mathavarajah S, Bera A, Salsman J, Habib E, et al. Haploinsufficient Tumor Suppressor Prp4k Is Negatively Regulated During Epithelial-To-Mesenchymal Transition. FASEB J (2021) 35(11):e22001. doi: 10.1096/fj.202001063R
115. Li Q, Mo W, Ding Y, Ding X. Study of Lncrna Tpa in Promoting Invasion and Metastasis of Breast Cancer Mediated by Tgf-Beta Signaling Pathway. Front Cell Dev Biol (2021) 9:688751. doi: 10.3389/fcell.2021.688751
116. Liu YP, Heng JY, Zhao XY, Li EY. The Inhibition of Circular Rna Circnolc1 by Propofol/Stat3 Attenuates Breast Cancer Stem Cells Function Via Mir-365a-3p/Stat3 Signaling. J Transl Med (2021) 19(1):467. doi: 10.1186/s12967-021-03133-5
117. Ma J, Du WW, Zeng K, Wu N, Fang L, Lyu J, et al. An Antisense Circular Rna Circscrib Enhances Cancer Progression by Suppressing Parental Gene Splicing and Translation. Mol Ther (2021) 29(9):2754–68. doi: 10.1016/j.ymthe.2021.08.002
118. Paramanantham A, Jung EJ, Go SI, Jeong BK, Jung JM, Hong SC, et al. Activated Erk Signaling Is One of the Major Hub Signals Related to the Acquisition of Radiotherapy-Resistant Mda-Mb-231 Breast Cancer Cells. Int J Mol Sci (2021) 22(9):4940. doi: 10.3390/ijms22094940
119. Leal-Orta E, Ramirez-Ricardo J, Garcia-Hernandez A, Cortes-Reynosa P, Salazar EP. Extracellular Vesicles From Mda-Mb-231 Breast Cancer Cells Stimulated With Insulin-Like Growth Factor 1 Mediate an Epithelial-Mesenchymal Transition Process in Mcf10a Mammary Epithelial Cells. J Cell Commun Signal (2021). doi: 10.1007/s12079-021-00638-y
120. Pierozan P, Cattani D, Karlsson O. Tumorigenic Activity of Alternative Per- and Polyfluoroalkyl Substances (Pfas): Mechanistic In Vitro Studies. Sci Total Environ (2021) 808:151945. doi: 10.1016/j.scitotenv.2021.151945
121. Brock T, Boudriot E, Klawitter A, Grosser M, Nguyen TTP, Giebe S, et al. The Influence of Ve-Cadherin on Adhesion and Incorporation of Breast Cancer Cells Into Vascular Endothelium. Int J Mol Sci (2021) 22(11):6049. doi: 10.3390/ijms22116049
122. Ke J, Han W, Meng F, Guo F, Wang Y, Wang L. Cti-2 Inhibits Metastasis and Epithelial-Mesenchymal Transition of Breast Cancer Cells by Modulating Mapk Signaling Pathway. Int J Mol Sci (2021) 22(22):12229. doi: 10.3390/ijms222212229
123. Wang Y, Dong L, Wan F, Chen F, Liu D, Chen D, et al. Mir-9-3p Regulates the Biological Functions and Drug Resistance of Gemcitabine-Treated Breast Cancer Cells and Affects Tumor Growth Through Targeting Mtdh. Cell Death Dis (2021) 12(10):861. doi: 10.1038/s41419-021-04145-1
124. Marti JLG, Beckwitt CH, Clark AM, Wells A. Atorvastatin Facilitates Chemotherapy Effects in Metastatic Triple-Negative Breast Cancer. Br J Cancer (2021) 125(9):1285–98. doi: 10.1038/s41416-021-01529-0
125. Sinha S, Sharma S, Sharma A, Vora J, Shrivastava N. Sulforaphane-Cisplatin Combination Inhibits the Stemness and Metastatic Potential of Tnbcs Via Down Regulation of Sirtuins-Mediated Emt Signaling Axis. Phytomedicine (2021) 84:153492. doi: 10.1016/j.phymed.2021.153492
126. Shen Y, Xu Y, Huang L, Chi Y, Meng L. Mir-205 Suppressed the Malignant Behaviors of Breast Cancer Cells by Targeting Cldn11 Via Modulation of the Epithelial-To-Mesenchymal Transition. Aging (Albany NY) (2021) 13(9):13073–86. doi: 10.18632/aging.202988
127. Kim TW, Ko SG. The Herbal Formula Ji017 Induces Er Stress Via Nox4 in Breast Cancer Cells. Antioxid (Basel) (2021) 10(12):1881. doi: 10.3390/antiox10121881
128. Zhang M, Meng M, Liu Y, Qi J, Zhao Z, Qiao Y, et al. Triptonide Effectively Inhibits Triple-Negative Breast Cancer Metastasis Through Concurrent Degradation of Twist1 and Notch1 Oncoproteins. Breast Cancer Res (2021) 23(1):116. doi: 10.1186/s13058-021-01488-7
129. Xiong L, Lu H, Hu Y, Wang W, Liu R, Wan X, et al. In Vitro Anti-Motile Effects of Rhoifolin, a Flavonoid Extracted From Callicarpa Nudiflora on Breast Cancer Cells Via Downregulating Podocalyxin-Ezrin Interaction During Epithelial Mesenchymal Transition. Phytomedicine (2021) 93:153486. doi: 10.1016/j.phymed.2021.153486
130. Brugnoli F, Tedeschi P, Grassilli S, Maietti A, Brandolini V, Bertagnolo V. Ethanol-Based Garlic Extract Prevents Malignant Evolution of Non-Invasive Breast Tumor Cells Induced by Moderate Hypoxia. BioMed Pharmacother (2021) 142:112052. doi: 10.1016/j.biopha.2021.112052
131. Witz IP. The Selectin-Selectin Ligand Axis in Tumor Progression. Cancer Metastasis Rev (2008) 27(1):19–30. doi: 10.1007/s10555-007-9101-z
132. Läubli H, Borsig L. Selectins Promote Tumor Metastasis. Semin Cancer Biol (2010) 20(3):169–77. doi: 10.1016/j.semcancer.2010.04.005
133. Hassan AA, Artemenko M, Tang MKS, Wong AST. Selectins: An Important Family of Glycan-Binding Cell Adhesion Molecules in Ovarian Cancer. Cancers (2020) 12(8):2238. doi: 10.3390/cancers12082238
134. Pang JH, Coupland LA, Freeman C, Chong BH, Parish CR. Activation of Tumour Cell Ecm Degradation by Thrombin-Activated Platelet Membranes: Potentially a P-Selectin and Gpiib/Iiia-Dependent Process. Clin Exp Metastasis (2015) 32(5):495–505. doi: 10.1007/s10585-015-9722-5
135. Kang S-A, Blache CA, Bajana S, Hasan N, Kamal M, Morita Y, et al. The Effect of Soluble E-Selectin on Tumor Progression and Metastasis. BMC Cancer (2016) 16:331. doi: 10.1186/s12885-016-2366-2
136. Kappelmayer J, Nagy B. The Interaction of Selectins and Psgl-1 as a Key Component in Thrombus Formation and Cancer Progression. BioMed Res Int (2017) 2017:6138145. doi: 10.1155/2017/6138145
137. Chen S-H, Dallas MR, Balzer EM, Konstantopoulos K. Mucin 16 Is a Functional Selectin Ligand on Pancreatic Cancer Cells. FASEB J: Off Publ Fed Am Societies Exp Biol (2012) 26(3):1349–59. doi: 10.1096/fj.11-195669
138. Thériault C, Pinard M, Comamala M, Migneault M, Beaudin J, Matte I, et al. Muc16 (Ca125) Regulates Epithelial Ovarian Cancer Cell Growth, Tumorigenesis and Metastasis. Gynecol Oncol (2011) 121(3):434–43. doi: 10.1016/j.ygyno.2011.02.020
139. Wang F, Yang S, Lv M, Chen F, Yin H, Gao S, et al. Novel Long Noncoding Rna 005620 Induces Epirubicin Resistance in Triple-Negative Breast Cancer by Regulating Itgb1 Expression. Front Oncol (2021) 11:592215. doi: 10.3389/fonc.2021.592215
140. Wang R, Yu C, Zhao D, Wu M, Yang Z. The Mucin-Type Glycosylating Enzyme Polypeptide N-Acetylgalactosaminyltransferase 14 Promotes the Migration of Ovarian Cancer by Modifying Mucin 13. Oncol Rep (2013) 30(2):667–76. doi: 10.3892/or.2013.2493
141. Maines-Bandiera S, Woo MMM, Borugian M, Molday LL, Hii T, Gilks B, et al. Oviductal Glycoprotein (Ovgp1, Muc9): A Differentiation-Based Mucin Present in Serum of Women With Ovarian Cancer. Int J Gynecol Cancer (2010) 20(1):16–22. doi: 10.1111/IGC.0b013e3181bcc96d
142. Woodman N, Pinder SE, Tajadura V, Le Bourhis X, Gillett C, Delannoy P, et al. Two E-Selectin Ligands, Bst-2 and Lgals3bp, Predict Metastasis and Poor Survival of Er-Negative Breast Cancer. Int J Oncol (2016) 49(1):265–75. doi: 10.3892/ijo.2016.3521
143. Shea DJ, Li YW, Stebe KJ, Konstantopoulos K. E-Selectin-Mediated Rolling Facilitates Pancreatic Cancer Cell Adhesion to Hyaluronic Acid. FASEB J: Off Publ Fed Am Societies Exp Biol (2017) 31(11):5078–86. doi: 10.1096/fj.201700331R
144. Dimitroff CJ, Lechpammer M, Long-Woodward D, Kutok JL. Rolling of Human Bone-Metastatic Prostate Tumor Cells on Human Bone Marrow Endothelium Under Shear Flow Is Mediated by E-Selectin. Cancer Res (2004) 64(15):5261–9. doi: 10.1158/0008-5472.CAN-04-0691
145. Price TT, Sipkins DA. E-Selectin and Sdf-1 Regulate Metastatic Trafficking of Breast Cancer Cells Within the Bone. Mol Cell Oncol (2017) 4(4):e1214771. doi: 10.1080/23723556.2016.1214771
146. Esposito M, Mondal N, Greco TM, Wei Y, Spadazzi C, Lin S-C, et al. Bone Vascular Niche E-Selectin Induces Mesenchymal-Epithelial Transition and Wnt Activation in Cancer Cells to Promote Bone Metastasis. Nat Cell Biol (2019) 21(5):627–39. doi: 10.1038/s41556-019-0309-2
147. Price TT, Burness ML, Sivan A, Warner MJ, Cheng R, Lee CH, et al. Dormant Breast Cancer Micrometastases Reside in Specific Bone Marrow Niches That Regulate Their Transit to and From Bone. Sci Transl Med (2016) 8(340):340ra73. doi: 10.1126/scitranslmed.aad4059
148. Khan SU, Xia Y, Goodale D, Schoettle G, Allan AL. Lung-Derived Selectins Enhance Metastatic Behavior of Triple Negative Breast Cancer Cells. Biomedicines (2021) 9(11):1580. doi: 10.3390/biomedicines9111580
149. Kemper M, Schiecke A, Maar H, Nikulin S, Poloznikov A, Galatenko V, et al. Integrin Alpha-V Is an Important Driver in Pancreatic Adenocarcinoma Progression. J Exp Clin Cancer Res (2021) 40(1):214. doi: 10.1186/s13046-021-01946-2
150. Haschemi R, Gockel LM, Bendas G, Schlesinger M. A Combined Activity of Thrombin and P-Selectin Is Essential for Platelet Activation by Pancreatic Cancer Cells. Int J Mol Sci (2021) 22(7):3323. doi: 10.3390/ijms22073323
151. Ye H, Wang K, Wang M, Liu R, Song H, Li N, et al. Bioinspired Nanoplatelets for Chemo-Photothermal Therapy of Breast Cancer Metastasis Inhibition. Biomaterials (2019) 206:1–126. doi: 10.1016/j.biomaterials.2019.03.024
152. Muz B, Abdelghafer A, Markovic M, Yavner J, Melam A, Salama NN, et al. Targeting E-Selectin to Tackle Cancer Using Uproleselan. Cancers (2021) 13(2):335. doi: 10.3390/cancers13020335
153. DeAngelo DJ, Jonas BA, Liesveld JL, Bixby DL, Advani AS, Marlton P, et al. Phase 1/2 Study of Uproleselan Added to Chemotherapy in Patients With Relapsed or Refractory Acute Myeloid Leukemia. Blood (2021) 139(8):1135–46. doi: 10.1182/blood.2021010721
154. Barbier V, Erbani J, Fiveash C, Davies JM, Tay J, Tallack MR, et al. Endothelial E-Selectin Inhibition Improves Acute Myeloid Leukaemia Therapy by Disrupting Vascular Niche-Mediated Chemoresistance. Nat Commun (2020) 11(1):2042. doi: 10.1038/s41467-020-15817-5
155. Festuccia C, Mancini A, Gravina GL, Colapietro A, Vetuschi A, Pompili S, et al. Dual Cxcr4 and E-Selectin Inhibitor, Gmi-1359, Shows Anti-Bone Metastatic Effects and Synergizes With Docetaxel in Prostate Cancer Cell Intraosseous Growth. Cells (2019) 9(1):32. doi: 10.3390/cells9010032
156. Morita Y, Leslie M, Kameyama H, Lokesh GLR, Ichimura N, Davis R, et al. Functional Blockade of E-Selectin in Tumor-Associated Vessels Enhances Anti-Tumor Effect of Doxorubicin in Breast Cancer. Cancers (2020) 12(3):725. doi: 10.3390/cancers12030725
157. Ji P, Yang Z, Li H, Wei M, Yang G, Xing H, et al. Smart Exosomes With Lymph Node Homing and Immune-Amplifying Capacities for Enhanced Immunotherapy of Metastatic Breast Cancer. Mol Ther Nucleic Acids (2021) 26:987–96. doi: 10.1016/j.omtn.2021.10.009
158. Chen Z, Jing Y, Song B, Han Y, Chu Y. Chemically Modified Heparin Inhibits In Vitro L-Selectin-Mediated Human Ovarian Carcinoma Cell Adhesion. Int J Gynecol Cancer (2009) 19(4):540–6. doi: 10.1111/IGC.0b013e3181a44bc8
159. Lu Z, Long Y, Wang Y, Wang X, Xia C, Li M, et al. Phenylboronic Acid Modified Nanoparticles Simultaneously Target Pancreatic Cancer and Its Metastasis and Alleviate Immunosuppression. Eur J Pharm Biopharm (2021) 165:164–73. doi: 10.1016/j.ejpb.2021.05.014
160. Lu Z, Long Y, Li J, Li J, Ren K, Zhao W, et al. Simultaneous Inhibition of Breast Cancer and Its Liver and Lung Metastasis by Blocking Inflammatory Feed-Forward Loops. J Control Release (2021) 338:662–79. doi: 10.1016/j.jconrel.2021.08.047
161. Peng Z, Liu C, Victor AR, Cao D-Y, Veiras LC, Bernstein EA, et al. Tumors Exploit Cxcr4cd62l Aged Neutrophils to Facilitate Metastatic Spread. Oncoimmunology (2021) 10(1):1870811. doi: 10.1080/2162402X.2020.1870811
162. Jafari M, Sriram V, Xu Z, Harris GM, Lee J-Y. Fucoidan-Doxorubicin Nanoparticles Targeting P-Selectin for Effective Breast Cancer Therapy. Carbohydr Polym (2020) 249:116837. doi: 10.1016/j.carbpol.2020.116837
163. Suchanski J, Grzegrzolka J, Owczarek T, Pasikowski P, Piotrowska A, Kocbach B, et al. Sulfatide Decreases the Resistance to Stress-Induced Apoptosis and Increases P-Selectin-Mediated Adhesion: A Two-Edged Sword in Breast Cancer Progression. Breast Cancer Res: BCR (2018) 20(1):133. doi: 10.1186/s13058-018-1058-z
164. Hamidi H, Pietila M, Ivaska J. The Complexity of Integrins in Cancer and New Scopes for Therapeutic Targeting. Br J Cancer (2016) 115(9):1017–23. doi: 10.1038/bjc.2016.312
165. Seguin L, Desgrosellier JS, Weis SM, Cheresh DA. Integrins and Cancer: Regulators of Cancer Stemness, Metastasis, and Drug Resistance. Trends Cell Biol (2015) 25(4):234–40. doi: 10.1016/j.tcb.2014.12.006
166. Gang EJ, Kim HN, Hsieh Y-T, Ruan Y, Ogana HA, Lee S, et al. Integrin α6 Mediates the Drug Resistance of Acute Lymphoblastic B-Cell Leukemia. Blood (2020) 136(2):210–23. doi: 10.1182/blood.2019001417
167. Ruan Y, Kim HN, Ogana HA, Gang EJ, Li S, Liu H-C, et al. And Effects of Ava4746, a Novel Competitive Antagonist of the Ligand Binding of Vla-4, in B-Cell Acute Lymphoblastic Leukemia. Exp Ther Med (2022) 23(1):47. doi: 10.3892/etm.2021.10969
168. Hsieh Y-T, Gang EJ, Geng H, Park E, Huantes S, Chudziak D, et al. Integrin Alpha4 Blockade Sensitizes Drug Resistant Pre-B Acute Lymphoblastic Leukemia to Chemotherapy. Blood (2013) 121(10):1814–8. doi: 10.1182/blood-2012-01-406272
169. Mautone L, Ferravante C, Tortora A, Tarallo R, Giurato G, Weisz A, et al. Higher Integrin Alpha 3 Beta1 Expression in Papillary Thyroid Cancer Is Associated With Worst Outcome. Cancers (2021) 13(12):2937. doi: 10.3390/cancers13122937
170. Zhu LC, Gao J, Hu ZH, Schwab CL, Zhuang HY, Tan MZ, et al. Membranous Expressions of Lewis Y and Cam-Dr-Related Markers Are Independent Factors of Chemotherapy Resistance and Poor Prognosis in Epithelial Ovarian Cancer. Am J Cancer Res (2015) 5(2):830–43.
171. Dötzer K, Schlüter F, Koch F, Brambs CE, Anthuber S, Frangini S, et al. Integrin α2β1 Represents a Prognostic and Predictive Biomarker in Primary Ovarian Cancer. Biomedicines (2021) 9(3):289. doi: 10.3390/biomedicines9030289
172. Chou C-W, Hsieh Y-H, Ku S-C, Shen W-J, Anuraga G, Khoa Ta HD, et al. Potential Prognostic Biomarkers of Osbpl Family Genes in Patients With Pancreatic Ductal Adenocarcinoma. Biomedicines (2021) 9(11):1601. doi: 10.3390/biomedicines9111601
173. Li Y, Li F, Bai X, Li Y, Ni C, Zhao X, et al. Is Associated With Immune Cell Infiltration and Serves as a Favorable Prognostic Biomarker for Breast Cancer. Front Oncol (2021) 11:658547. doi: 10.3389/fonc.2021.658547
174. Ricci E, Fava M, Rizza P, Pellegrino M, Bonofiglio D, Casaburi I, et al. Foxo3a Inhibits Tamoxifen-Resistant Breast Cancer Progression by Inducing Integrin α5 Expression. Cancers (2022) 14(1):214. doi: 10.3390/cancers14010214
175. Cagnet S, Faraldo MM, Kreft M, Sonnenberg A, Raymond K, Glukhova MA. Signaling Events Mediated by α3β1 Integrin Are Essential for Mammary Tumorigenesis. Oncogene (2014) 33(34):4286–95. doi: 10.1038/onc.2013.391
176. Barnawi R, Al-Khaldi S, Colak D, Tulbah A, Al-Tweigeri T, Fallatah M, et al. β1 Integrin Is Essential for Fascin-Mediated Breast Cancer Stem Cell Function and Disease Progression. Int J Cancer (2019) 145(3):830–41. doi: 10.1002/ijc.32183
177. Bui T, Gu Y, Ancot F, Sanguin-Gendreau V, Zuo D, Muller WJ. Emergence of β1 Integrin-Deficient Breast Tumours From Dormancy Involves Both Inactivation of P53 and Generation of a Permissive Tumour Microenvironment. Oncogene (2021) 41(4):527–37. doi: 10.1038/s41388-021-02107-7
178. Mia MS, Jarajapu Y, Rao R, Mathew S. Integrin β1 Promotes Pancreatic Tumor Growth by Upregulating Kindlin-2 and Tgf-β Receptor-2. Int J Mol Sci (2021) 22(19):10599. doi: 10.3390/ijms221910599
179. Parvani JG, Galliher-Beckley AJ, Schiemann BJ, Schiemann WP. Targeted Inactivation of β1 Integrin Induces β3 Integrin Switching, Which Drives Breast Cancer Metastasis by Tgf-β. Mol Biol Cell (2013) 24(21):3449–59. doi: 10.1091/mbc.E12-10-0776
180. Zhang Y, Zhang Q, Cao Z, Huang Y, Cheng S, Pang D. Hoxd3 Plays a Critical Role in Breast Cancer Stemness and Drug Resistance. Cell Physiol Biochem (2018) 46(4):1737–47. doi: 10.1159/000489249
181. Desgrosellier JS, Lesperance J, Seguin L, Gozo M, Kato S, Franovic A, et al. Integrin αvβ3 Drives Slug Activation and Stemness in the Pregnant and Neoplastic Mammary Gland. Dev Cell (2014) 30(3):295–308. doi: 10.1016/j.devcel.2014.06.005
182. Seguin L, Kato S, Franovic A, Camargo MF, Lesperance J, Elliott KC, et al. An Integrin Beta(3)-Kras-Ralb Complex Drives Tumour Stemness and Resistance to Egfr Inhibition. Nat Cell Biol (2014) 16(5):457–68. doi: 10.1038/ncb2953
183. Fox GC, Su X, Davis JL, Xu Y, Kwakwa KA, Ross MH, et al. Targeted Therapy to β3 Integrin Reduces Chemoresistance in Breast Cancer Bone Metastases. Mol Cancer Ther (2021) 20(6):1183–98. doi: 10.1158/1535-7163.MCT-20-0931
184. Sun Q, Wang Y, Officer A, Pecknold B, Lee G, Harismendy O, et al. Stem-Like Breast Cancer Cells in the Activated State Resist Genetic Stress Via Tgfbi-Zeb1. NPJ Breast Cancer (2022) 8(1):5. doi: 10.1038/s41523-021-00375-w
185. Lecker LSM, Berlato C, Maniati E, Delaine-Smith R, Pearce OMT, Heath O, et al. Tgfbi Production by Macrophages Contributes to an Immunosuppressive Microenvironment in Ovarian Cancer. Cancer Res (2021) 81(22):5706–19. doi: 10.1158/0008-5472.CAN-21-0536
186. Jiang Y, Zhou T, Shi Y, Feng W, Lyu T. A Smyd3/Itgb6/Tgfβ1 Positive Feedback Loop Promotes the Invasion and Adhesion of Ovarian Cancer Spheroids. Front Oncol (2021) 11:690618. doi: 10.3389/fonc.2021.690618
187. Martin TA, Jiang WG. Evaluation of the Expression of Stem Cell Markers in Human Breast Cancer Reveals a Correlation With Clinical Progression and Metastatic Disease in Ductal Carcinoma. Oncol Rep (2014) 31(1):262–72. doi: 10.3892/or.2013.2813
188. Parker KA, Gooding AJ, Valadkhan S, Schiemann WP. Lncrna Borg : Trim28 Complexes Drive Metastatic Progression by Inducing α6 Integrin/Cd49f Expression in Breast Cancer Stem Cells. Mol Cancer Res (2021) 19(12):2068–80. doi: 10.1158/1541-7786.MCR-21-0137
189. Adorno-Cruz V, Hoffmann AD, Liu X, Dashzeveg NK, Taftaf R, Wray B, et al. Promotes Expression of and in Enhancing Breast Cancer Stemness and Metastasis. Genes Dis (2021) 8(4):493–508. doi: 10.1016/j.gendis.2020.01.015
190. Su C, Huang K. Lncrna Tcf7 Promotes Epithelial Ovarian Cancer Viability, Mobility and Stemness Regulating Itgb8. Front Oncol (2021) 11:649655. doi: 10.3389/fonc.2021.649655
191. Dodagatta-Marri E, Ma H-Y, Liang B, Li J, Meyer DS, Chen S-Y, et al. Integrin αvβ8 on T Cells Suppresses Anti-Tumor Immunity in Multiple Models and Is a Promising Target for Tumor Immunotherapy. Cell Rep (2021) 36(1):109309. doi: 10.1016/j.celrep.2021.109309
192. Lainé A, Labiad O, Hernandez-Vargas H, This S, Sanlaville A, Léon S, et al. Regulatory T Cells Promote Cancer Immune-Escape Through Integrin αvβ8-Mediated Tgf-β Activation. Nat Commun (2021) 12(1):6228. doi: 10.1038/s41467-021-26352-2
193. Lee BY, Timpson P, Horvath LG, Daly RJ. Fak Signaling in Human Cancer as a Target for Therapeutics. Pharmacol Ther (2015) 146:132–49. doi: 10.1016/j.pharmthera.2014.10.001
194. Jiang H, Hegde S, Knolhoff BL, Zhu Y, Herndon JM, Meyer MA, et al. Targeting Focal Adhesion Kinase Renders Pancreatic Cancers Responsive to Checkpoint Immunotherapy. Nat Med (2016) 22(8):851–60. doi: 10.1038/nm.4123
195. Ray U, Jung D-B, Jin L, Xiao Y, Dasari S, Sarkar Bhattacharya S, et al. Targeting Lrrc15 Inhibits Metastatic Dissemination of Ovarian Cancer. Cancer Res (2021) 82(6):1038–54. doi: 10.1158/0008-5472.CAN-21-0622
196. Zhang J, Li Y, Liu H, Zhang J, Wang J, Xia J, et al. Genome-Wide Crispr/Cas9 Library Screen Identifies Pcmt1 as a Critical Driver of Ovarian Cancer Metastasis. J Exp Clin Cancer Res (2022) 41(1):24. doi: 10.1186/s13046-022-02242-3
197. Hupfer A, Brichkina A, Koeniger A, Keber C, Denkert C, Pfefferle P, et al. Matrix Stiffness Drives Stromal Autophagy and Promotes Formation of a Protumorigenic Niche. Proc Natl Acad Sci USA (2021) 118(40). doi: 10.1073/pnas.2105367118
198. Chen Y-Q, Song H-Y, Zhou Z-Y, Ma J, Luo Z-Y, Zhou Y, et al. Osthole Inhibits the Migration and Invasion of Highly Metastatic Breast Cancer Cells by Suppressing Itgα3/Itgβ5 Signaling. Acta Pharmacol Sin (2021). doi: 10.1038/s41401-021-00757-7
199. Hanker AB, Estrada MV, Bianchini G, Moore PD, Zhao J, Cheng F, et al. Extracellular Matrix/Integrin Signaling Promotes Resistance to Combined Inhibition of Her2 and Pi3k in Her2(+) Breast Cancer. Cancer Res (2017) 77(12):3280–92. doi: 10.1158/0008-5472.CAN-16-2808
200. Huang C, Park CC, Hilsenbeck SG, Ward R, Rimawi MF, Wang YC, et al. Beta1 Integrin Mediates an Alternative Survival Pathway in Breast Cancer Cells Resistant to Lapatinib. Breast Cancer Res (2011) 13(4):R84. doi: 10.1186/bcr2936
201. Cary LA, Guan JL. Focal Adhesion Kinase in Integrin-Mediated Signaling. Front Biosci (1999) 4:D102–13. doi: 10.2741/Cary
202. Wu C, You J, Fu J, Wang X, Zhang Y. Phosphatidylinositol 3-Kinase/Akt Mediates Integrin Signaling to Control Rna Polymerase I Transcriptional Activity. Mol Cell Biol (2016) 36(10):1555–68. doi: 10.1128/MCB.00004-16
203. Sakamoto H, Attiyeh MA, Gerold JM, Makohon-Moore AP, Hayashi A, Hong J, et al. The Evolutionary Origins of Recurrent Pancreatic Cancer. Cancer Discov (2020) 10(6):792–805. doi: 10.1158/2159-8290.CD-19-1508
204. Nan P, Dong X, Bai X, Lu H, Liu F, Sun Y, et al. Tumor-Stroma Tgf-β1-Thbs2 Feedback Circuit Drives Pancreatic Ductal Adenocarcinoma Progression Via Integrin αβ/Cd36-Mediated Activation of the Mapk Pathway. Cancer Lett (2022) 528:59–75. doi: 10.1016/j.canlet.2021.12.025
205. Park CC, Zhang HJ, Yao ES, Park CJ, Bissell MJ. Beta1 Integrin Inhibition Dramatically Enhances Radiotherapy Efficacy in Human Breast Cancer Xenografts. Cancer Res (2008) 68(11):4398–405. doi: 10.1158/0008-5472.CAN-07-6390
206. Hu T, Zhou R, Zhao Y, Wu G. Integrin Alpha6/Akt/Erk Signaling Is Essential for Human Breast Cancer Resistance to Radiotherapy. Sci Rep (2016) 6:33376. doi: 10.1038/srep33376
207. Toth RK, Tran JD, Muldong MT, Nollet EA, Schulz VV, Jensen CC, et al. Hypoxia-Induced Pim Kinase and Laminin-Activated Integrin Alpha6 Mediate Resistance to Pi3k Inhibitors in Bone-Metastatic Crpc. Am J Clin Exp Urol (2019) 7(4):297–312.
208. Deo AN, Thorat R, Dhadve AC, De A, Rekhi B, Ray P. Igf1r-α6 Integrin-S100a4 Network Governs the Organ-Specific Metastasis of Chemoresistant Epithelial Ovarian Cancer Cells. Biochim Biophys Acta Mol Basis Dis (2022) 1868(1):166282. doi: 10.1016/j.bbadis.2021.166282
Keywords: cell adhesion-mediated drug resistance, endocrine-related cancers, tumor microenvironment, cell adhesion molecules, chemoresistance
Citation: Ruan Y, Chen L, Xie D, Luo T, Xu Y, Ye T, Chen X, Feng X and Wu X (2022) Mechanisms of Cell Adhesion Molecules in Endocrine-Related Cancers: A Concise Outlook. Front. Endocrinol. 13:865436. doi: 10.3389/fendo.2022.865436
Received: 29 January 2022; Accepted: 09 March 2022;
Published: 07 April 2022.
Edited by:
Francesca Coperchini, University of Pavia, ItalyReviewed by:
Valentina Vicennati, University of Bologna, ItalyCarmela Ricciardelli, University of Adelaide, Australia
Copyright © 2022 Ruan, Chen, Xie, Luo, Xu, Ye, Chen, Feng and Wu. This is an open-access article distributed under the terms of the Creative Commons Attribution License (CC BY). The use, distribution or reproduction in other forums is permitted, provided the original author(s) and the copyright owner(s) are credited and that the original publication in this journal is cited, in accordance with accepted academic practice. No use, distribution or reproduction is permitted which does not comply with these terms.
*Correspondence: Yongsheng Ruan, dmltbWVyQDEyNi5jb20=; Xuedong Wu, eHVlZG9uZ3d1QDE2My5jb20=
†These authors have contributed equally to this work