- 1The Reproduction Center, The Third Affiliated Hospital of Zhengzhou University, Zhengzhou, China
- 2Office of Scientific Research, The Third Affiliated Hospital of Zhengzhou University, Zhengzhou, China
Objective: This study aimed to determine whether controlled ovarian hyperstimulation (COH) parameters influence the incidence of de novo chromosomal abnormalities (> 4 Mb) in blastocysts and, thus, clinical pregnancy outcomes in preimplantation genetic testing (PGT).
Methods: Couples who underwent preimplantation genetic testing for structural chromosome rearrangements (PGT-SR) and monogenic disorders (PGT-M) were included in this study. The relationships of maternal age, paternal age, stimulation protocol, exogenous gonadotropin dosage, duration of stimulation, number of oocytes retrieved and estradiol (E2) levels on human chorionic gonadotropin (hCG) trigger day with the incidence of de novo chromosomal abnormalities were assessed. Blastocysts were biopsied, and nuclear DNA was sequenced using next-generation sequencing (NGS). Clinical pregnancy outcomes after single euploid blastocyst transfers under different COH parameters were assessed.
Results: A total of 1,710 and 190 blastocysts were biopsied for PGT-SR and PGT-M, respectively. The rate of de novo chromosomal abnormalities was found to increase with maternal age (p< 0.001) and paternal age (p = 0.019) in the PGT-SR group. No significant differences in the incidence of de novo chromosomal abnormalities were seen for different maternal or paternal age groups between the PGT-SR and PGT-M groups (p > 0.05). Stratification analysis by gonadotropin dosage, stimulation protocol, duration of stimulation, number of retrieved oocytes and E2 levels on hCG trigger day revealed that de novo chromosomal abnormalities and clinical pregnancy outcomes were not correlated with COH parameters after adjusting for various confounding factors.
Conclusion: The rate of de novo chromosomal abnormalities was found to increase with maternal or paternal age. COH parameters were found to not influence the incidence of de novo chromosomal abnormalities or clinical pregnancy outcomes.
Introduction
In assisted reproductive technology (ART), aneuploidy is one of the most significant causes of pregnancy failure and miscarriage. Aneuploidy occurs due to meiotic errors during gametogenesis, the fertilization of unbalanced gametes or mitotic errors during embryonic development (1, 2). Therefore, a major goal of controlled ovulation hyperstimulation (COH) is to achieve maximal follicular development during a single menstrual cycle. It is thought that aneuploidy can be avoided through the collection of many oocytes, thereby increasing the likelihood of obtaining euploid embryos. However, embryonic chromosomal abnormalities are thought to also occur due to iatrogenic factors (3), with the in vitro fertilization (IVF) process itself and associated COH increasing the risk of meiotic or mitotic errors. It has been speculated that the use of exogenous gonadotropins (Gn) to stimulate multifollicular development can interfere with the natural selection of dominant follicles, thereby increasing the retention of aneuploid oocytes (4, 5). Moreover, COH has been hypothesized to increase oocyte division errors and affect genomic imprinting (6). However, the specific nature of such adverse effects of COH remains unclear.
As preimplantation genetic testing (PGT) is an important prenatal diagnostic method used to detect aneuploid embryos (7), it can prevent the transmission of pathogenic genetic mutations or an unbalanced chromosome to the offspring, increasing the chances of a successful, healthy pregnancy. Therefore, PGT is widely used in clinical practice (7, 8). Many studies have explored the relationship between COH and aneuploid, but have yielded inconclusive results. It has been suggested that higher Gn dosage increases the incidence of aneuploid (9, 10). In contrast, other studies have reported that the incidence of embryonic aneuploidy was not affected by exogenous Gn, with equivalent aneuploidy rates across unstimulated and stimulated IVF cycles, and different Gn dosages (11, 12). However, most studies have focused on the relationship between ovulation induction and aneuploidy (6, 13), although many other intrinsic factors can influence ploidy. Aneuploidy can be inherited from a parental carrier of genetic abnormalities (14), or it can occur de novo (15, 16). PGT for aneuploidy (PGT-A) is typically carried out following recurrent miscarriages and repeated implantation failures or in cases of advanced maternal age. In contrast, PGT for structural chromosome rearrangements (PGT-SR) is carried out for individuals known to have high rates of unbalanced gametes after meiotic segregation, resulting in embryos with abnormal chromosomal composition. Therefore, we sought to study the effect of COH on the rate of de novo chromosomal abnormalities identified by PGT-SR. As patients undergoing PGT for monogenic disorders (PGT-M) have known monogenetic mutations but normal karyotypes, they typically do not have fertility issues and are not at risk of elevated embryonic aneuploidy rates. We therefore used patients undergoing PGT-M as our control group.
In this study, we aimed to determine whether COH parameters are associated with de novo chromosomal abnormality rates in the Chinese population served by our reproductive center at The Third Affiliated Hospital of Zhengzhou University. We compared patients undergoing unstimulated and stimulated PGT-SR and PGT-M and explored various COH parameters for their associations with aneuploidy rates and clinical pregnancy outcomes. To our knowledge, this is the first systematic evaluation of the effects of specific COH parameters on de novo chromosomal abnormalities rates and clinical pregnancy outcomes involving PGT using next-generation sequencing (NGS) techniques. This research will inform the development of improved stimulation protocols that may reduce the occurrence of de novo aneuploidy following COH.
Materials and methods
Study population
This retrospective study involved 430 patients who underwent PGT-SR and 47 patients who underwent PGT-M from January 2017 to December 2021 (Table 1). Anonymous data were obtained from the Center for Reproductive Medicine at The Third Affiliated Hospital of Zhengzhou University. Chromosomal karyotyping of all participants was performed by standard Giemsa banding techniques prior to ovarian stimulation. Couples where at least one partner was a known carrier of a reciprocal translocation (REC), Robertsonian translocation (ROB) or inversion (INV) were assigned to the PGT-SR group. Patients with monogenetic mutations but normal karyotypes were assigned to the PGT-M group. Basic patient information was gathered, and other parameters, including the number of oocytes retrieved, fertilization rate, embryo formation rate and blastocyst formation rate, were recorded.
Ethics approval
The study was reviewed and approved by the Ethics Committee of the Third Affiliated Hospital of Zhengzhou University, and all patients underwent genetic counseling. Written informed consent for participation was not required for this study, in accordance with national legislation and institutional requirements.
Basic clinical characteristics
Baseline demographic information was collected, including maternal and paternal age (years, y) and maternal and paternal body mass index (BMI; kg/m2). Basal plasma follicle stimulating hormone (FSH; mIU/mL), luteinizing hormone (LH; mIU/mL) and estradiol (E2; pmol/L) concentrations were measured on day two or three of the menstrual cycle, and anti-Mullerian hormone (AMH; pmol/L) concentrations were measured on any day of the menstrual cycle. COH parameters, including the ovulation stimulation protocol used, Gn dosage (IU), duration of ovarian stimulation (days, d), number of retrieved oocytes and peak E2 concentrations (pmol/L), were documented for all patients.
Controlled ovarian hyperstimulation
Ovarian stimulation and gonadotrophin (Gn) administration was performed by experienced clinicians taking into consideration maternal age, antral follicle count (AFC), basal FSH concentration, the cause of infertility and ovarian reserve function. COH cycles for PGT require the administration of more Gn to ensure that more oocytes are collected and enough transferable embryos remain after testing. Three stimulation protocols were used: a gonadotropin-releasing hormone (GnRH) antagonist, a GnRH agonist and progestin-primed ovarian stimulation (PPOS). Gn dosing and induction duration were adjusted with ovarian response, as monitored using transvaginal ultrasound and circulating E2. Patients were categorized into different groups according to the total amount of Gn administered (< 2,000, 2,000–3,000 and > 3,000 IU), stimulation duration (< 10, 10–12 and > 12 d), number of oocytes retrieved (< 10, 10–15 and > 15 oocytes) and peak E2 concentrations (< 10,000, 10,000–15,000 and > 15,000 pmol/L). hCG was administered by injection to promote oocyte maturation when the diameter of at least two follicles exceeded 18 mm.
Oocyte collection, intracytoplasmic sperm injection (ICSI), embryo culture, and blastocyst biopsy
Transvaginal ultrasonography-assisted oocyte aspiration was performed approximately 36 hours after the hCG injection. After oocyte retrieval, cumulus-oocyte complexes were cultured for 4 h and then inseminated by intracytoplasmic sperm injection (ICSI). If the oocytes were at the metaphase-I (MI) or germinal vesicle (GV) stage, they were cultured in vitro until mature for an additional 24 h and then fertilized. Fertilization was confirmed by the presence of two pronuclei (2PN) 17–18 h post-insemination. Embryo cleavage was evaluated 41–44 h (Day 2) and 65–68 h (Day 3) after ICSI. For the first 3 days post-ICSI, the embryos were cultured in G1™ plus (Vitrolife, Sweden) in a humidified incubator with 5% O2 and 6% CO2. All cleavage embryos were transferred into G2™ plus (Vitrolife, Sweden) sequential media and cultured until they reached the blastocyst stage. Blastocysts were scored using the Gardner grading system. Blastocysts graded above 3BC were used for subsequent biopsies. Trophectoderm biopsies were performed from day 5 to 7 of development, based on the time of gastrulation, using the laser method. For genetic analysis, 5–8 cells were biopsied and analyzed using NGS (17).
Sample preparation and NGS analysis
The biopsied samples were washed with G-MOPS™ plus medium and placed in 0.2-mL polymerase chain reaction (PCR) tubes with 2 μL PBS. NGS allows direct quantification of the sequenced DNA fragments based on read numbers. In accordance with the Illumina NGS protocol, raw data were further processed using computational bioinformatic algorithms to map and align the short sequence reads to a linear human reference genome sequence. A small minority of cases, for which DNA amplification failed, were excluded from the study. The minimum detection range was 4 Mb. An embryo was considered “abnormal” when the result deviated from the reference baseline. Embryos with< 20%, 20%–80% and > 80% aneuploid cells were classified as euploid, mosaic and aneuploid, respectively. In the case of aneuploid embryos, if the chromosome involved was the same as that of an affected parent, it was classified as a genetic abnormality. In contrast, if the imbalanced chromosome was normal in the parents, it was classified as a de novo abnormality.
Endometrium preparation and frozen embryo transfer
Natural cycle tracking, hormone replacement therapy and ovarian stimulation were used for endometrium preparation. Luteal-phase support was initiated when endometrial thickness reached at least 7 mm and was continued until 3 months of gestation. A single euploid embryo was chosen for transfer. Biochemical pregnancy was defined as a serum concentration of ß-hCG > 30 mIU/ml measured 2 weeks post-embryo transfer. Clinical pregnancy was confirmed by the ultrasonographic observation of a gestational sac 35 days post-embryo transfer. Spontaneous abortion was defined as a pregnancy with a gestational sac that did not result in a live birth. Live birth was defined as the delivery of at least one live birth at ≥ 28 weeks of gestation.
Statistical analysis
Statistical analyses were performed using SPSS 24.0. Continuous variables are presented as the mean ± standard deviation (SD). Categorical variables are presented as absolute values and percentage frequencies.
The chi-square test was used to compare differences in categorical variables, and one-way analysis of variance (ANOVA) to compare differences in continuous variables, between the groups. P< 0.05 was considered statistically significant. The relationships between various COH parameters and the incidence of de novo chromosomal abnormalities and clinical pregnancy outcomes were analyzed using logistic regression. ap-values were calculated using a mixed logistic model adjusted for maternal age, maternal BMI and blastocyst quality. bp-values were calculated using a mixed logistic model adjusted for maternal age, maternal BMI, method of endometrial preparation, endometrial thickness transfer day of blastocyst and blastocyst quality.
Results
General characteristics of study subjects
From January 2017 to December 2021, 477 PGT cycles were initiated at the study center for which blastocysts were subsequently biopsied. No significant differences were seen between the PGT-M and PGT-SR groups in terms of maternal age or BMI, paternal age or BMI, basal FSH, E2 and LH or AMH. A total of 7,632 oocytes were collected, and 6,071 MII oocytes were subsequently used for ICSI. In the PGT-SR and PGT-M groups, 81.77% and 81.58% of MII oocytes were successfully fertilized and developed into normally fertilized oocytes with two pronuclei (2PN), of which 49.63% and 61.01% fertilized oocytes developed into blastocysts suitable for biopsy, respectively. In the PGT-SR group, 1,710 (98.62%) of blastocysts had genetic results, of which 575 (33.63%) were euploid, 941 (55.03%) were aneuploid, 194 (11.34%) were mosaic and 393 (22.98%) had de novo chromosomal abnormalities. In the PGT-M group, 190 blastocysts had genetic results, of which 115 (60.53%) were euploid, 45 (23.68%) were aneuploid, 30 (15.79%) were mosaic and 43 (23.63%) had de novo chromosomal abnormalities. The total number of blastocysts, number of euploid blastocysts and number of aneuploid blastocysts were significantly different in the PGT-SR and PGT-M groups (all p< 0.05), as shown in Table 1.
Parent’s age and the occurrence of de novo chromosomal abnormalities
As shown in Table 2, no significant differences were seen between the PGT-SR and PGT-M groups in the incidence of de novo chromosomal abnormalities in each maternal age group and paternal age group. However, within the PGT-SR group, there was a statistically significant increase in such abnormalities with increasing maternal age (p< 0.001). Similarly, the rate of de novo chromosomal abnormalities was found to increase with paternal age in the PGT-SR group (p = 0.019). In contrast, in the PGT-M group, the rate of blastocysts with de novo chromosomal abnormalities increased with maternal or paternal age, but these differences were not statistically significant (p > 0.05).
COH parameters and the incidence of de novo chromosomal abnormalities
Logistic regression analysis was used to analyze the association between ovarian stimulation factors and the incidence of de novo chromosomal abnormalities by adjusting for maternal age and BMI and blastocyst quality (Table 3). No significant differences were identified in different stimulation protocols, Gn doses, stimulation durations, number of retrieved oocytes or maximal E2 concentrations on hCG trigger day between the PGT-SR and PGT-M groups after adjusting for confounding factors.
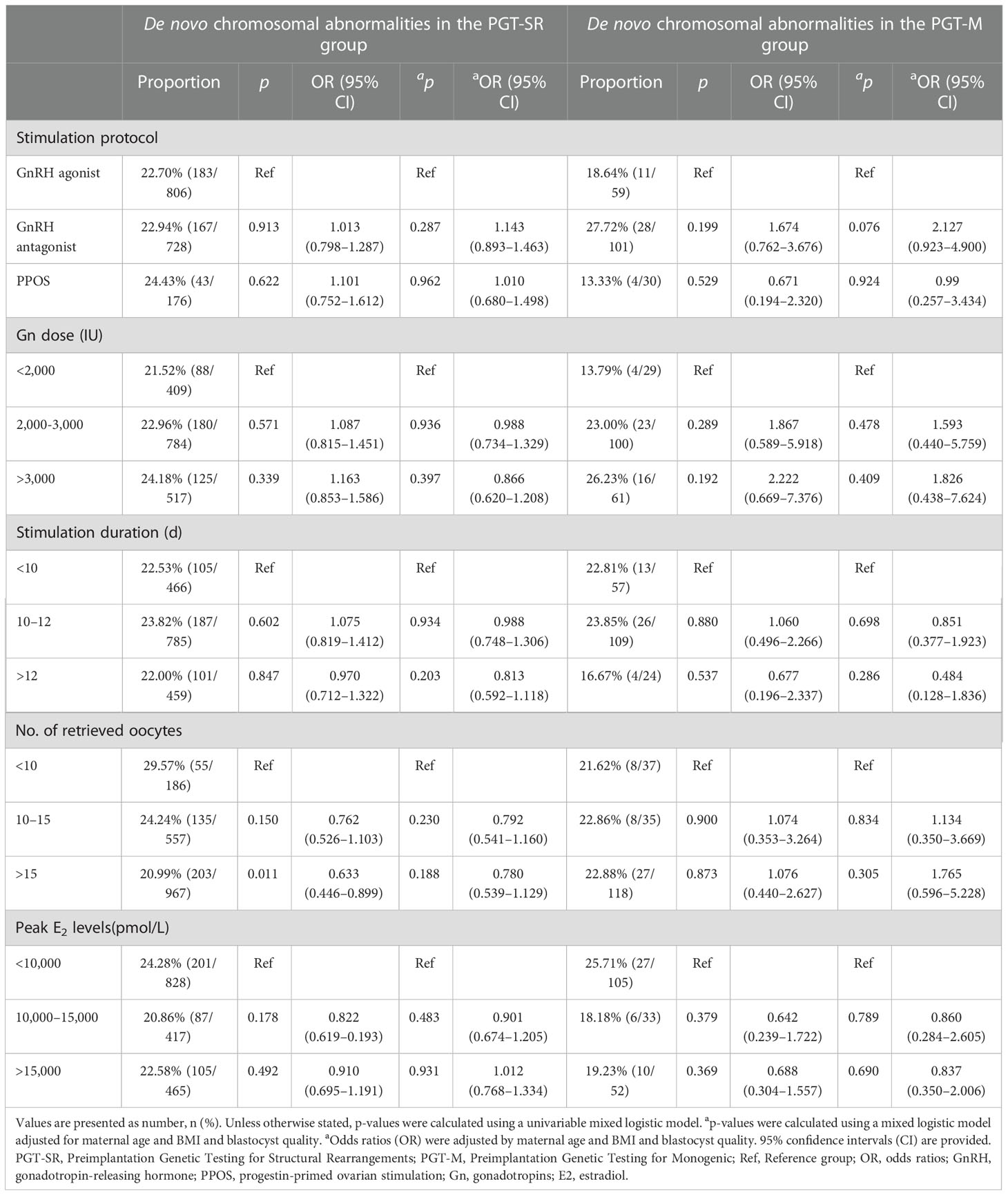
Table 3 Incidence of de novo chromosomal abnormalities for different ovarian hyperstimulation parameters.
COH parameters and clinical pregnancy outcomes
After adjusting for confounding factors, we found that COH parameters were not associated with clinical pregnancy outcomes (Figures 1–5). Clinical pregnancy rates ranged from 60.54% to 73.53% (bp = 0.293) for the different stimulation methods, from 63.79% to 69.66% (bp = 0.677) for different Gn dosages, from 63.53% to 67.86% (bp = 0.827) for different stimulation durations, from 65.04% to 68.09% (bp = 0.816) for different numbers of retrieved oocytes and from 63.01% to 73.40% (bp = 0.432) for different maximal E2 concentrations. Corresponding live birth rates (LBRs) were 52.38%–64.71% (bp = 0.320), 56.52%–58.43% (bp = 0.969), 54.12%–59.69% (bp = 0.605), 56.87%–61.70% (bp = 0.600) and 55.25%–60.64% (bp = 0.959), respectively. Similarly, no significant linear trends of relationship were seen between any of these factors and the rates of miscarriage (12.00%–13.48%, bp = 0.780; 9.01%–16.13%, bp = 0.358; 11.69%–14.82%, bp = 0.393; 9.38%–13.67%, bp = 0.498; and 7.02%–17.39%, bp = 0.089, respectively). The original data can be found in Supplementary Tables 1, 2.
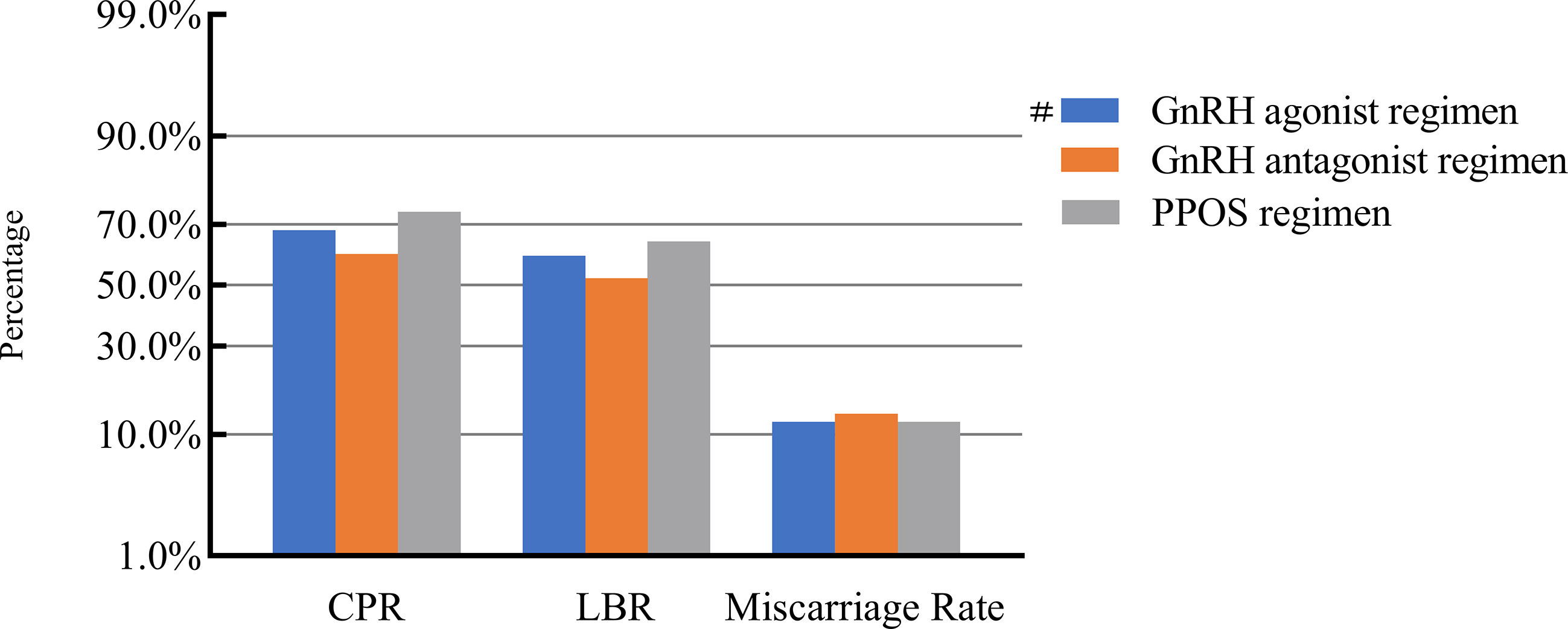
Figure 1 Association between stimulation protocols and pregnancy outcomes. #, Reference group bp-values were calculated using a mixed logistic model adjusted for maternal age and BMI, method of endometrial preparation, endometrial thickness, transfer day of blastocyst and blastocyst quality. CPR, clinical pregnancy rate; LBR, live birth rate; GnRH, gonadotropin-releasing hormone; PPOS, progestin-primed ovarian stimulation.
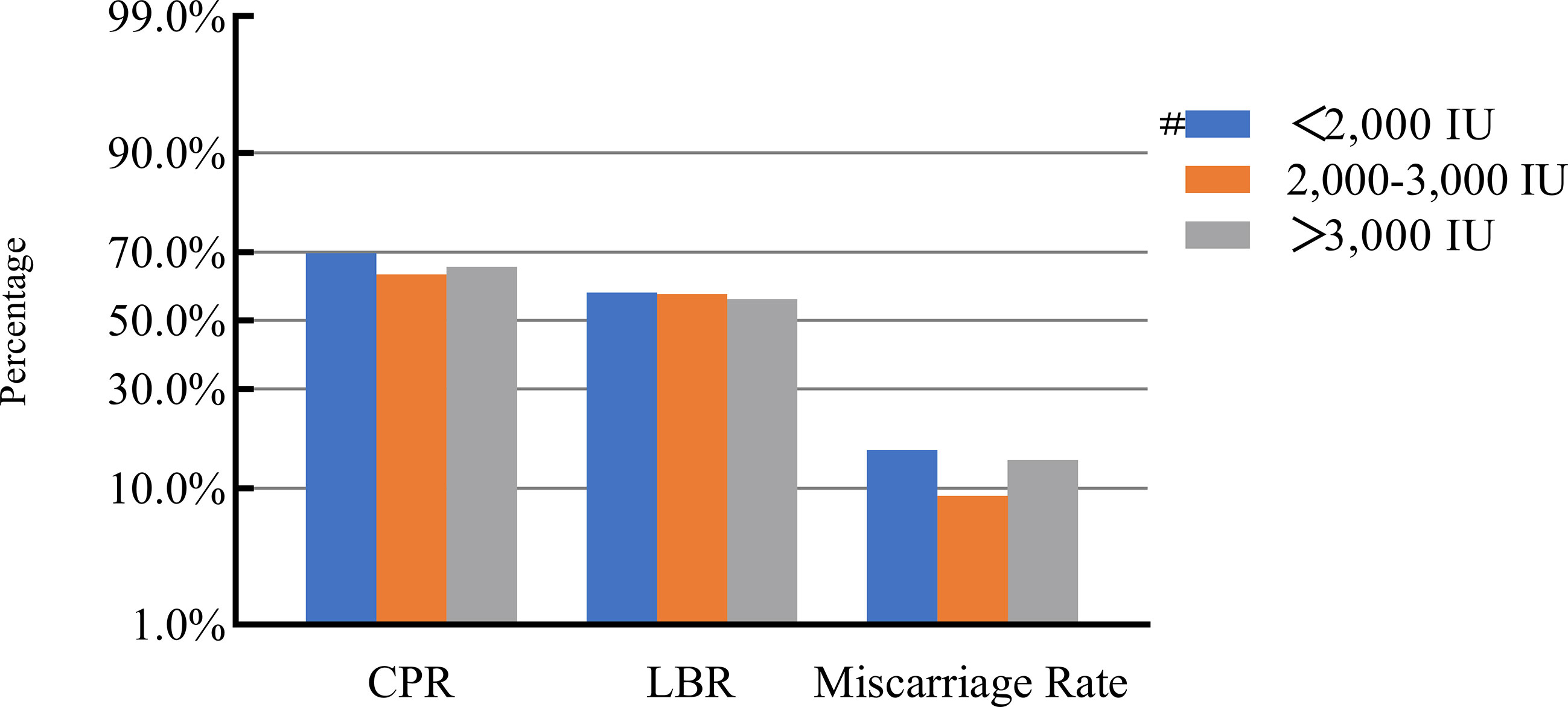
Figure 2 Association between gonadotropin dosage and pregnancy outcomes. #, Reference group bp-values were calculated using a mixed logistic model adjusted for maternal age and BMI, method of endometrial preparation, endometrial thickness, transfer day of blastocyst and blastocyst quality. CPR, clinical pregnancy rate; LBR, live birth rate.
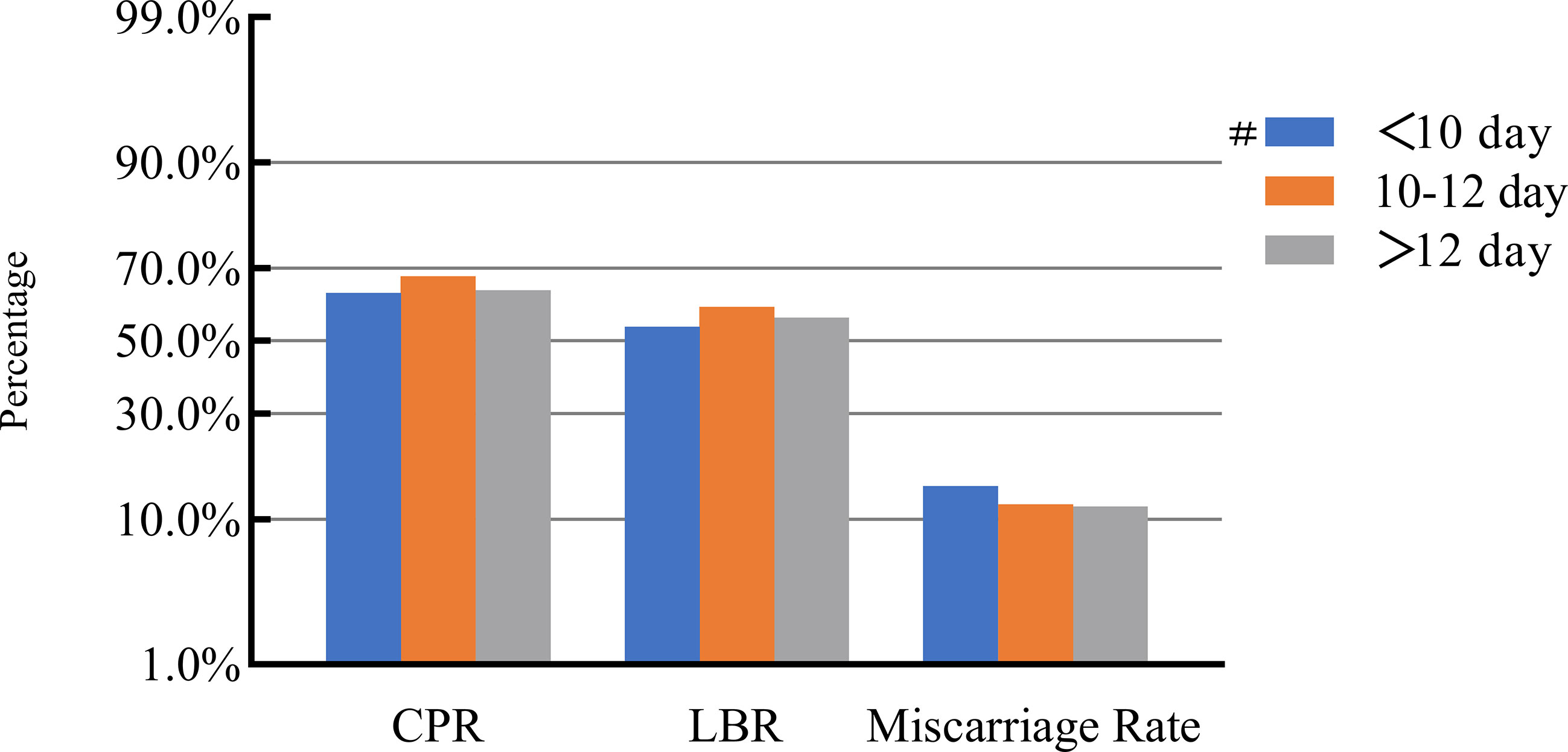
Figure 3 Association between stimulation duration and pregnancy outcomes. #, Reference group bp-values were calculated using a mixed logistic model adjusted for maternal age and BMI, method of endometrial preparation, endometrial thickness, transfer day of blastocyst and blastocyst quality. CPR, clinical pregnancy rate; LBR, live birth rate.
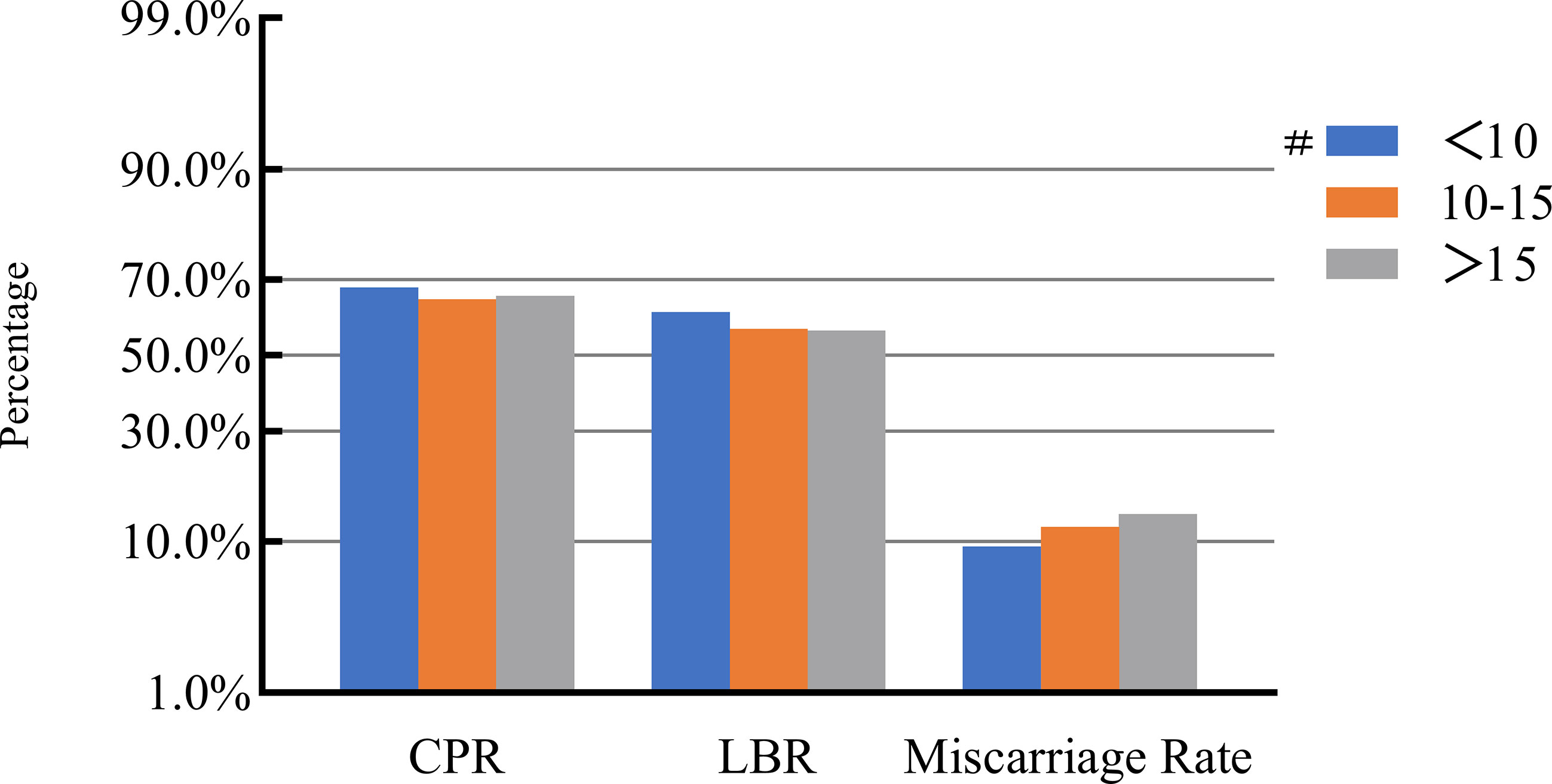
Figure 4 Association between the number of retrieved oocytes and pregnancy outcomes. #, Reference group bp-values were calculated using a mixed logistic model adjusted for maternal age and BMI, method of endometrial preparation, endometrial thickness, transfer day of blastocyst and blastocyst quality. CPR, clinical pregnancy rate; LBR, live birth rate.
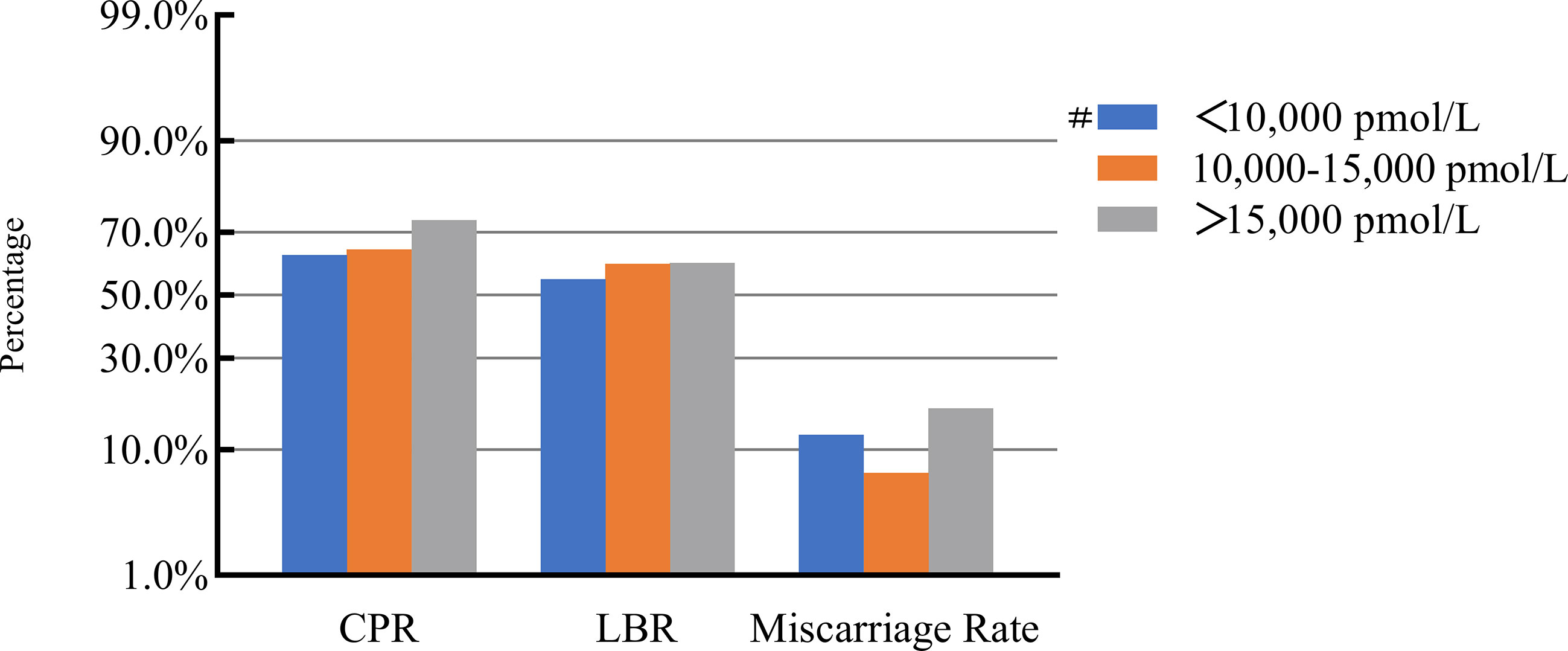
Figure 5 Association between maximal E2 levels and pregnancy outcomes. #, Reference group bp-values were calculated using a mixed logistic model adjusted for maternal age and BMI, method of endometrial preparation, endometrial thickness, transfer day of blastocyst and blastocyst quality. CPR, clinical pregnancy rate; LBR, live birth rate; E2, estradiol.
Discussion
The study comprehensively evaluated the incidence of de novo chromosomal abnormalities in blastocysts and clinical pregnancy outcomes for different COH parameters across 477 PGT cycles. The incidence of de novo chromosomal abnormalities was 22.98% in PGT-SR cycles and 22.63% in PGT-M cycles. Such abnormalities were found to increase with maternal or paternal age. It was found that the stimulation protocol, Gn dosage, stimulation duration, number of oocytes retrieved and peak E2 concentrations did not affect de novo aneuploidy rates or clinical pregnancy outcomes. We identified a significant age-dependent increase in de novo chromosomal abnormalities in the PGT-SR group. To our knowledge, this is the first study examining the relationships between COH parameters and de novo chromosomal abnormalities and clinical pregnancy outcomes following PGT.
In terms of ART, the high incidence of chromosomal abnormalities in the resultant embryos and the impact of such abnormalities on implantation have been widely discussed (18–20). Though PGT-A candidates have been studied frequently in previous studies, such individuals often harbor unexplained confounding factors that lead to high aneuploidy rates, thereby resulting in recurrent miscarriages and repeated implantation failures that necessitate PGT-A (21). Therefore, PGT-A samples were not suitable for accurately examining the relationship between ovarian stimulation and aneuploidy. Additionally, gametes produced by meiotic segregation in patients with unbalanced translocations potentially have an increased risk of aneuploidy. In addition to the direct effects of such translocations on the unbalanced chromosome, they may also affect the meiosis of other structurally normal chromosomes, leading to an increased risk of additional aneuploid gametes. This phenomenon is known as the inter chromosomal effect (ICE) (22). Many studies have attempted to confirm the ICE hypothesis, but it remains disputed (22–24). Therefore, we focused our study on de novo chromosomal abnormalities rather than pre-existing translocations or inversions. Additionally, patients undergoing PGT-M were chosen as a control group to verify whether ICE occurs.
It is well known that increased maternal age is an independent factor that negatively impacts the probability of obtaining a euploid embryo (2, 25). In line with this, we found that the incidence of de novo chromosomal abnormalities increased with maternal age. This might be because the risk of chromosomal abnormalities in oocytes and embryos increases with maternal age (26) largely due to recombination errors in early meiosis, defective spindle assembly checkpoints at MI and centromere cohesion loss (27). Similarly, our study found that older paternal age also has been associated with an increased incidence of de novo chromosomal abnormalities, which is consistent with previous studies. The association between aneuploidy rate and paternal age may be related to the level of sperm DNA fragmentation, which is theoretically believed that post-fertilization sperm DNA strand breaks can be repaired within the oocyte. However, the level of sperm DNA fragmentation deteriorates with age and the repair capacity of the embryo may not be able to compensate for the decrease in DNA quality, possibly leading to poorer embryo quality and aneuploidy rate increased (28, 29). In contrast, this study found no statistical differences between the PGT-SR and PGT-M groups in terms of the incidence of de novo chromosomal abnormalities across different age groups, suggesting that the ICE phenomenon does not occur. Therefore, despite finding evidence that increased de novo chromosomal abnormalities can occur in these conditions, they cannot be attributed to ICE.
Other than maternal or paternal age, chromosomal abnormalities have been thought to occur due to various aspects of the ART process, such as the ovarian stimulation protocols used for IVF. Undoubtedly, the goal of ovarian stimulation is to induce the ongoing development of multiple dominant follicles to obtain multiple mature oocytes (30, 31). Such ovarian stimulation is a critical aspect of IVF, particularly PGT cycles. In general, approximately 10–15 days of ovarian stimulation is required, during which E2 levels increase 10–20-fold compared with natural cycles. Some studies have proposed that oocytes obtained via natural or modified natural cycles are superior to those obtained via induced ovulation cycles (5). It is also believed that altered regulation of meiotic spindle alignment may occur due to the use of exogenous gonadotropins to stimulate the development of multiple follicles (32). Therefore, we examined whether varied COH parameters are correlated with an increase in de novo chromosomal abnormalities and worse pregnancy outcomes.
Our study demonstrated that the incidence of de novo chromosomal abnormalities and clinical pregnancy outcomes are not affected by the COH stimulation protocol used; a result consistent with previous findings (33). We found that the rate of de novo chromosomal abnormalities and miscarriages in those treated with a GnRH antagonist was slightly higher than in those treated with a GnRH agonist. However, this difference was not statistically different. Similarly, a Chinese birth cohort study of ART and birth defects reported that GnRH antagonist-based stimulatory regimens were associated with an increased risk of birth defects and that aneuploidy was a major factor resulting in these birth defects (34). Another study revealed that GnRH antagonist treatment was associated with higher aneuploidy rates in early aborted tissues and blastocysts than GnRH agonist treatment (35). These conflicting results may result from heterogenous study populations or variation in the specific methods used by different physicians in different IVF centers. Therefore, a strength of this study is that we focused on de novo chromosomal abnormalities rather than aneuploidy.
Previous theoretical studies have speculated that the administration of supraphysiological exogenous Gn interferes with natural follicle selection, potentially leading to chromosomal dysfunction and poor quality oocytes (36). It has also been proposed that low-dose Gn is associated with reduced aneuploidy rates in human preimplantation embryos (9, 37). In contrast, our findings indicate that the Gn dose, duration of ovarian stimulation treatment and peak of estrogen on hCG trigger day were not associated with the incidence of de novo chromosomal abnormality and clinical pregnancy outcomes. Other studies have demonstrated that different Gn dosages and the number of oocytes retrieved were not relevant to embryonic aneuploidy rates and pregnancy rates (38, 39). Moreover, another study reported that high-dose Gn leads to an increased risk of embryonic aneuploidy, but only in those with reduced ovarian reserve (6). Such reports of high-dose exogenous Gn leading to increased meiotic segregation errors in oocytes and increasing aneuploidy rates have not been confirmed. Other studies have reported that the duration of ovarian stimulation significantly affects LBRs of fresh cycles, potentially due to the adverse effects of high levels of Gn on endometrium receptivity - effects that are potentially reduced or eliminated in the case of frozen cycles (40). Therefore, in the absence of an alternative, milder method by which to stimulate ovarian maturation, conventional ovarian stimulation remains the recommended approach for most patients undergoing PGT. Our study reinforces previous findings that pregnancy rates are independent of the Gn dosage in frozen embryo transfer cycles.
In the study, we found that the duration of ovarian stimulation was not correlated with the incidence of de novo chromosomal abnormalities, while the > 12d group is lowest. It might because longer stimulation times are more conducive to the physiological process of cellular self-repair (41). This hypothesis has been corroborated in this study, as the incidence of de novo chromosomal abnormalities was reduced for the longer GnRH agonist protocol. Response to ovarian stimulation was assessed by the number of oocytes retrieved and maximal E2 concentrations on hCG trigger day. Supraphysiological levels of E2 are detected due to the maturation of multiple follicles, but more oocytes, and subsequently higher quality blastocysts, are required to obtain at least one euploid embryo for implantation. Clinicians have expressed concern that COH might affect the development and quality of oocytes, as multiple small, nondominant follicles will mature following exogenous Gn treatment (11). Labarta et al. reported (42) that a greater ovarian response, and thus number of oocytes retrieved, results in a greater number of resultant euploid embryos. Moreover, we found that the number of oocytes retrieved and maximal E2 concentrations on hCG trigger day were not correlated with de novo chromosomal abnormality rates and clinical pregnancy outcomes. These findings reinforce the lack of association between E2 concentrations and embryo quality or pregnancy outcomes seen in previous studies (43, 44). It had been suggested that a reduced oocyte yield represents an appropriate response to some forms of ovarian stimulation, as only the most competent follicles and oocytes can develop. However, older patients and those with a poor ovarian reserve should also be considered.
Conclusion
In conclusion, this study revealed that total Gn dosage, ovarian stimulation duration, peak E2 concentrations and number of oocytes retrieved did not influence de novo chromosomal abnormality rates or clinical pregnancy outcomes. This study will provide clinicians with valuable information to consider when choosing an ovarian stimulation protocol. In addition to maternal age, AFC, baseline FSH levels and known causes of infertility, it is important to consider the potential to retrieve as many oocytes as possible to provide more embryos for biopsy. However, we do not advocate the use of high-dosage stimulation protocols, especially for patients at an increased risk of complications such as ovarian torsion and hyperstimulation syndrome. This is the first clinical study to examine the role of COH parameters in determining the incidence of de novo chromosomal abnormalities in Chinese IVF patients. Our findings may inform the development of novel strategies for ovarian stimulation during ART that promote both safety and efficacy. However, the mechanisms underlying de novo chromosomal abnormalities that occur during PGT cycles remain unclear.
Limitations
This study was retrospective in nature, had a modest sample size and had a heterogenous study population. As such, larger prospective, multicenter and randomized controlled trials should be carried out to confirm our findings and further explore the mechanisms underlying the differential effects of different ovarian stimulation protocols.
Data availability statement
The original contributions presented in the study are included in the article/supplementary files, further inquiries can be directed to the corresponding author.
Ethics statement
The studies involving human participants were reviewed and approved by The review board of the Third Affiliated Hospital of Zhengzhou University. Written informed consent for participation was not required for this study in accordance with the national legislation and the institutional requirements.
Author contributions
YL, RY and YG designed the study. PZ, JS and YZ were involved in the data extraction and analyses. YL and JS were involved in drafting this article. RP, JZ reviewed the data and article. All authors contributed to the article and approved the submitted version.
Funding
This research was funded by Henan Provincial Science and Technology Research Project (222102310329) and Henan Provincial High-Level Talent International Training Grant Program (2022050706).
Acknowledgments
The authors particularly appreciate the patients who participated in this study. We also thank AsiaEdit for their professional manuscript editing services.
Conflict of interest
The authors declare that the research was conducted in the absence of any commercial or financial relationships that could be construed as a potential conflict of interest.
Publisher’s note
All claims expressed in this article are solely those of the authors and do not necessarily represent those of their affiliated organizations, or those of the publisher, the editors and the reviewers. Any product that may be evaluated in this article, or claim that may be made by its manufacturer, is not guaranteed or endorsed by the publisher.
Supplementary material
The Supplementary Material for this article can be found online at: https://www.frontiersin.org/articles/10.3389/fendo.2022.1080843/full#supplementary-material
References
1. Babariya D, Fragouli E, Alfarawati S, Spath K, Wells D. The incidence and origin of segmental aneuploidy in human oocytes and preimplantation embryos. Hum Reprod (2017) 32(12):2549–60. doi: 10.1093/humrep/dex324
2. Nair J, Shetty S, Kasi CI, Thondehalmath N, Ganesh D, Bhat VR, et al. Preimplantation genetic testing for aneuploidy (Pgt-a)-a single-center experience. J Assist Reprod Genet (2022) 39(3):729–38. doi: 10.1007/s10815-022-02413-3
3. Munne S, Alikani M, Ribustello L, Colls P, Martinez-Ortiz PA, McCulloh DH, et al. Euploidy rates in donor egg cycles significantly differ between fertility centers. Hum Reprod (2017) 32(4):743–9. doi: 10.1093/humrep/dex031
4. Baart EB, Martini E, Eijkemans MJ, Van Opstal D, Beckers NG, Verhoeff A, et al. Milder ovarian stimulation for in-vitro fertilization reduces aneuploidy in the human preimplantation embryo: A randomized controlled trial. Hum Reprod (2007) 22(4):980–8. doi: 10.1093/humrep/del484
5. Drakopoulos P, Romito A, Errazuriz J, Santos-Ribeiro S, Popovic-Todorovic B, Racca A, et al. Modified natural cycle ivf versus conventional stimulation in advanced-age Bologna poor responders. Reprod BioMed Online (2019) 39(4):698–703. doi: 10.1016/j.rbmo.2019.05.009
6. Sekhon L, Shaia K, Santistevan A, Cohn KH, Lee JA, Beim PY, et al. The cumulative dose of gonadotropins used for controlled ovarian stimulation does not influence the odds of embryonic aneuploidy in patients with normal ovarian response. J Assist Reprod Genet (2017) 34(6):749–58. doi: 10.1007/s10815-017-0909-3
7. Zegers-Hochschild F, Adamson GD, Dyer S, Racowsky C, de Mouzon J, Sokol R, et al. The international glossary on infertility and fertility care, 2017. Fertil Steril (2017) 108(3):393–406. doi: 10.1016/j.fertnstert.2017.06.005
8. Committee EPCS, Carvalho F, Coonen E, Goossens V, Kokkali G, Rubio C, et al. Eshre pgt consortium good practice recommendations for the organisation of pgt. Hum Reprod Open (2020) 2020(3):hoaa021. doi: 10.1093/hropen/hoaa021
9. Sachdeva K, Upadhyay D, Discutido R, Varghese MM, Albuz F, Almekosh R, et al. Low gonadotropin dosage reduces aneuploidy in human preimplantation embryos: First clinical study in a uae population. Genet Test Mol Biomarkers (2018) 22(10):630–4. doi: 10.1089/gtmb.2018.0063
10. Rubio C, Mercader A, Alama P, Lizan C, Rodrigo L, Labarta E, et al. Prospective cohort study in high responder oocyte donors using two hormonal stimulation protocols: Impact on embryo aneuploidy and development. Hum Reprod (2010) 25(9):2290–7. doi: 10.1093/humrep/deq174
11. Hong KH, Franasiak JM, Werner MM, Patounakis G, Juneau CR, Forman EJ, et al. Embryonic aneuploidy rates are equivalent in natural cycles and gonadotropin-stimulated cycles. Fertil Steril (2019) 112(4):670–6. doi: 10.1016/j.fertnstert.2019.05.039
12. Irani M, Canon C, Robles A, Maddy B, Gunnala V, Qin X, et al. No effect of ovarian stimulation and oocyte yield on euploidy and live birth rates: An analysis of 12 298 trophectoderm biopsies. Hum Reprod (2020) 35(5):1082–9. doi: 10.1093/humrep/deaa028
13. McCulloh DH, Alikani M, Norian J, Kolb B, Arbones JM, Munne S. Controlled ovarian hyperstimulation (Coh) parameters associated with euploidy rates in donor oocytes. Eur J Med Genet (2019) 62(8):103707. doi: 10.1016/j.ejmg.2019.103707
14. Vera-Rodriguez M, Michel CE, Mercader A, Bladon AJ, Rodrigo L, Kokocinski F, et al. Distribution patterns of segmental aneuploidies in human blastocysts identified by next-generation sequencing. Fertil Steril (2016) 105(4):1047–55 e2. doi: 10.1016/j.fertnstert.2015.12.022
15. Zhou S, Cheng D, Ouyang Q, Xie P, Lu C, Gong F, et al. Prevalence and authenticity of de-Novo segmental aneuploidy (>16 Mb) in human blastocysts as detected by next-generation sequencing. Reprod BioMed Online (2018) 37(5):511–20. doi: 10.1016/j.rbmo.2018.08.006
16. Garcia-Herrero S, Cervero A, Mateu E, Mir P, Poo ME, Rodrigo L, et al. Genetic analysis of human preimplantation embryos. Curr Top Dev Biol (2016) 120:421–47. doi: 10.1016/bs.ctdb.2016.04.009
17. Harton GL, Magli MC, Lundin K, Montag M, Lemmen J, Harper JC, et al. Eshre pgd Consortium/Embryology special interest group–best practice guidelines for polar body and embryo biopsy for preimplantation genetic Diagnosis/Screening (Pgd/Pgs). Hum Reprod (2011) 26(1):41–6. doi: 10.1093/humrep/deq265
18. Tiegs AW, Tao X, Zhan Y, Whitehead C, Kim J, Hanson B, et al. A multicenter, prospective, blinded, nonselection study evaluating the predictive value of an aneuploid diagnosis using a targeted next-generation sequencing-based preimplantation genetic testing for aneuploidy assay and impact of biopsy. Fertil Steril (2021) 115(3):627–37. doi: 10.1016/j.fertnstert.2020.07.052
19. Gruhn JR, Zielinska AP, Shukla V, Blanshard R, Capalbo A, Cimadomo D, et al. Chromosome errors in human eggs shape natural fertility over reproductive life span. Science (2019) 365(6460):1466–9. doi: 10.1126/science.aav7321
20. Colley E, Hamilton S, Smith P, Morgan NV, Coomarasamy A, Allen S. Potential genetic causes of miscarriage in euploid pregnancies: A systematic review. Hum Reprod Update (2019) 25(4):452–72. doi: 10.1093/humupd/dmz015
21. Kort JD, McCoy RC, Demko Z, Lathi RB. Are blastocyst aneuploidy rates different between fertile and infertile populations? J Assist Reprod Genet (2018) 35(3):403–8. doi: 10.1007/s10815-017-1060-x
22. Alfarawati S, Fragouli E, Colls P, Wells D. Embryos of robertsonian translocation carriers exhibit a mitotic interchromosomal effect that enhances genetic instability during early development. PloS Genet (2012) 8(10):e1003025. doi: 10.1371/journal.pgen.1003025
23. Xie Y, Xu Y, Wang J, Miao B, Zeng Y, Ding C, et al. Preliminary analysis of numerical chromosome abnormalities in reciprocal and robertsonian translocation preimplantation genetic diagnosis cases with 24-chromosomal analysis with an Acgh/Snp microarray. J Assist Reprod Genet (2018) 35(1):177–86. doi: 10.1007/s10815-017-1045-9
24. Young D, Klepacka D, McGarvey M, Schoolcraft WB, Katz-Jaffe MG. Infertility patients with chromosome inversions are not susceptible to an inter-chromosomal effect. J Assist Reprod Genet (2019) 36(3):509–16. doi: 10.1007/s10815-018-1376-1
25. Demko ZP, Simon AL, McCoy RC, Petrov DA, Rabinowitz M. Effects of maternal age on euploidy rates in a Large cohort of embryos analyzed with 24-chromosome single-nucleotide polymorphism-based preimplantation genetic screening. Fertil Steril (2016) 105(5):1307–13. doi: 10.1016/j.fertnstert.2016.01.025
26. Thomas C, Cavazza T, Schuh M. Aneuploidy in human eggs: Contributions of the meiotic spindle. Biochem Soc Trans (2021) 49(1):107–18. doi: 10.1042/BST20200043
27. Chiang T, Schultz RM, Lampson MA. Meiotic origins of maternal age-related aneuploidy. Biol Reprod (2012) 86(1):1–7. doi: 10.1095/biolreprod.111.094367
28. Yang H, Li G, Jin H, Guo Y, Sun Y. The effect of sperm DNA fragmentation index on assisted reproductive technology outcomes and its relationship with semen parameters and lifestyle. Transl Androl Urol (2019) 8(4):356–65. doi: 10.21037/tau.2019.06.22
29. Petersen CG, Mauri AL, Vagnini LD, Renzi A, Petersen B, Mattila M, et al. The effects of Male age on sperm DNA damage: An evaluation of 2,178 semen samples. JBRA Assist Reprod (2018) 22(4):323–30. doi: 10.5935/1518-0557.20180047
30. Lambertini M, Pescio MC, Viglietti G, Goldrat O, Del Mastro L, Anserini P, et al. Methods of controlled ovarian stimulation for Embryo/Oocyte cryopreservation in breast cancer patients. Expert Rev Qual Life Cancer Care (2017) 2(1):47–59. doi: 10.1080/23809000.2017.1270760
31. Cascales A, Lledo B, Ortiz JA, Morales R, Ten J, Llacer J, et al. Effect of ovarian stimulation on embryo aneuploidy and mosaicism rate. Syst Biol Reprod Med (2021) 67(1):42–9. doi: 10.1080/19396368.2020.1850908
32. Patrizio P, Sakkas D. From oocyte to baby: A clinical evaluation of the biological efficiency of in vitro fertilization. Fertil Steril (2009) 91(4):1061–6. doi: 10.1016/j.fertnstert.2008.01.003
33. Wang R, Lin S, Wang Y, Qian W, Zhou L. Comparisons of gnrh antagonist protocol versus gnrh agonist long protocol in patients with normal ovarian reserve: A systematic review and meta-analysis. PloS One (2017) 12(4):e0175985. doi: 10.1371/journal.pone.0175985
34. Lv H, Diao F, Du J, Chen T, Meng Q, Ling X, et al. Assisted reproductive technology and birth defects in a Chinese birth cohort study. Lancet Reg Health West Pac (2021) 7:100090. doi: 10.1016/j.lanwpc.2020.100090
35. Wang J, Zhang J, Zhao N, Ma Y, Wang X, Gou X, et al. The effect of ovarian stimulation on aneuploidy of early aborted tissues and preimplantation blastocysts: Comparison of the gnrh agonist long protocol with the gnrh antagonist protocol. J Assist Reprod Genet (2022) 39(8):1927–36. doi: 10.1007/s10815-022-02557-2
36. Nagaoka SI, Hassold TJ, Hunt PA. Human aneuploidy: Mechanisms and new insights into an age-old problem. Nat Rev Genet (2012) 13(7):493–504. doi: 10.1038/nrg3245
37. Katz-Jaffe MG, Trounson AO, Cram DS. Chromosome 21 mosaic human preimplantation embryos predominantly arise from diploid conceptions. Fertil Steril (2005) 84(3):634–43. doi: 10.1016/j.fertnstert.2005.03.045
38. Barash OO, Hinckley MD, Rosenbluth EM, Ivani KA, Weckstein LN. High gonadotropin dosage does not affect euploidy and pregnancy rates in ivf pgs cycles with single embryo transfer. Hum Reprod (2017) 32(11):2209–17. doi: 10.1093/humrep/dex299
39. Wu Q, Li H, Zhu Y, Jiang W, Lu J, Wei D, et al. Dosage of exogenous gonadotropins is not associated with blastocyst aneuploidy or live-birth rates in pgs cycles in Chinese women. Hum Reprod (2018) 33(10):1875–82. doi: 10.1093/humrep/dey270
40. Gerber RS, Fazzari M, Kappy M, Cohen A, Galperin S, Lieman H, et al. Differential impact of controlled ovarian hyperstimulation on live birth rate in fresh versus frozen embryo transfer cycles: A society for assisted reproductive technology clinic outcome system study. Fertil Steril (2020) 114(6):1225–31. doi: 10.1016/j.fertnstert.2020.06.021
41. Vanneste E, Voet T, Melotte C, Debrock S, Sermon K, Staessen C, et al. What next for preimplantation genetic screening? high mitotic chromosome instability rate provides the biological basis for the low success rate. Hum Reprod (2009) 24(11):2679–82. doi: 10.1093/humrep/dep266
42. Labarta E, Bosch E, Mercader A, Alama P, Mateu E, Pellicer A. A higher ovarian response after stimulation for ivf is related to a higher number of euploid embryos. BioMed Res Int (2017) 2017:5637923. doi: 10.1155/2017/5637923
43. Hu D, Dong X, Xiong M, Xiong T, Huang B, Zeng D, et al. Can the peak E2/Follicle ratio be a quantitative indicator of pregnancy outcomes following assisted reproductive cycles? a retrospective study. Int J Clin Exp Med (2015) 8(7):10964–70.
44. Taskin EA, Atabekoglu CS, Musali N, Oztuna D, Sonmezer M. Association of serum estradiol levels on the day of hcg administration with pregnancy rates and embryo scores in fresh Icsi/Et cycles down regulated with either gnrh agonists or gnrh antagonists. Arch Gynecol Obstet (2014) 289(2):399–405. doi: 10.1007/s00404-013-2984-8
Keywords: controlled ovarian hyperstimulation (COH), in vitro fertilization, preimplantation genetic testing (PGT), de novo chromosomal abnormality, clinical pregnancy outcomes
Citation: Liu Y, Shen J, Zhang Y, Peng R, Zhao J, Zhou P, Yang R and Guan Y (2023) Controlled ovarian hyperstimulation parameters are not associated with de novo chromosomal abnormality rates and clinical pregnancy outcomes in preimplantation genetic testing. Front. Endocrinol. 13:1080843. doi: 10.3389/fendo.2022.1080843
Received: 26 October 2022; Accepted: 23 December 2022;
Published: 12 January 2023.
Edited by:
Richard Ivell, University of Nottingham, United KingdomReviewed by:
Jianzhen Li, Northwest Normal University, ChinaJiaojiao Huang, Qingdao Agricultural University, China
Copyright © 2023 Liu, Shen, Zhang, Peng, Zhao, Zhou, Yang and Guan. This is an open-access article distributed under the terms of the Creative Commons Attribution License (CC BY). The use, distribution or reproduction in other forums is permitted, provided the original author(s) and the copyright owner(s) are credited and that the original publication in this journal is cited, in accordance with accepted academic practice. No use, distribution or reproduction is permitted which does not comply with these terms.
*Correspondence: Yichun Guan, lisamayguan@163.com
†ORCID: Yichun Guan, orcid.org/0000-0002-6255-2551