- 1The First Clinical Medical College of Lanzhou University, Lanzhou, China
- 2Department of Endocrinology, The First Hospital of Lanzhou University, Lanzhou, China
Osteoporosis is a systemic bone metabolism disease that often causes complications, such as fractures, and increases the risk of death. The nucleotide-binding oligomerization domain-like-receptor family pyrin domain-containing 3 (NLRP3) inflammasome is an intracellular multiprotein complex that regulates the maturation and secretion of Caspase-1 dependent proinflammatory cytokines interleukin (IL)-1β and IL-18, mediates inflammation, and induces pyroptosis. The chronic inflammatory microenvironment induced by aging or estrogen deficiency activates the NLRP3 inflammasome, promotes inflammatory factor production, and enhances the inflammatory response. We summarize the related research and demonstrate that the NLRP3 inflammasome plays a vital role in the pathogenesis of osteoporosis by affecting the differentiation of osteoblasts and osteoclasts. IL-1β and IL-18 can accelerate osteoclast differentiation by expanding inflammatory response, and can also inhibit the expression of osteogenic related proteins or transcription factors. In vivo and in vitro experiments showed that the overexpression of NLRP3 protein was closely related to aggravated bone resorption and osteogenesis deficiency. In addition, abnormal activation of NLRP3 inflammasome can not only produce inflammation, but also lead to pyroptosis and dysfunction of osteoblasts by upregulating the expression of Caspase-1 and gasdermin D (GSDMD). In conclusion, NLRP3 inflammasome overall not only accelerates bone resorption, but also inhibits bone formation, thus increasing the risk of osteoporosis. Thus, this review highlights the recent studies on the function of NLRP3 inflammasome in osteoporosis, provides information on new strategies for managing osteoporosis, and investigates the ideal therapeutic target to treat osteoporosis.
Introduction
Osteoporosis (OP) is a chronic disease characterized by changes in bone mass, bone microstructure, and fractures and is a causative factor of morbidity and death in senior adults (1). According to the National Health and Nutrition Examination Survey in 2010, more than 50% of seniors in the USA were affected by OP and low bone mass (2). Furthermore, severe medical and social issues caused by OP are accelerated with age (3).
Previous studies have suggested that estrogen is the main hormone regulator of bone metabolism. Estrogen deficiency can not only directly promote osteoclasts differentiation through estrogen receptors on osteoclasts, but also indirectly stimulate nuclear factor kappa-β ligand (RANKL) on osteoblasts, T cells and B cells to promote bone resorption (4). In addition, estrogen deficiency increases osteoblast apoptosis and inhibits osteoblast differentiation by increasing reactive oxygen species (ROS) and nuclear factor kappa-B (NF-κB) pathway, causing a relative deficiency of bone formation (5, 6). As a result, the balance between osteoblasts and osteoclasts is broken while the rate of bone formation cannot keep up with the rate of bone resorption, leading to net bone loss. Therefore, the therapeutic effect of estrogen achieves in OP mainly through four effector cells such as osteoblasts, osteoclasts, osteocytes and T cells (7). Besides the effects of hormones on bone metabolism, chronic inflammation promotes bone loss and the onset of OP (8). The nucleotide-binding oligomerization domain-like-receptor family pyrin domain-containing 3 (NLRP3) inflammasome is an intracellular protein complex that mediates the systemic innate immune response and inflammation. The NLRP3 inflammasome mediates the activation of inflammatory caspase 1 (Caspase-1), interleukin (IL)-1β, and IL-18, causing inflammation and inducing inflammatory cell death.
It has been demonstrated that the abnormal activation of the NLRP3 inflammasome is closely related to multiple metabolic diseases driven by aging and chronic inflammation, such as diabetes, obesity, and gout (9–12). Here, we briefly summarize recent studies on the mechanism of NLRP3 inflammasome in OP, provide information on new strategies for preventing and treating the disease, and investigate the ideal therapeutic target to treat osteoporosis.
Concept and Structure of NLRP3 Inflammasome
The maturation and activation of IL-1β, a critical molecule involved in inflammation, was known to be mediated by Caspase-1; however, the underlying mechanism remained unclear until the discovery of the NLRP1 inflammasome that suggested the modulation of the process in monocytes (13). The NLRP3 inflammasome, a supramolecular complex concentrated in the cytoplasm, affects innate immunity and inflammation, responds to pathogen- and injury-related signals, and mediates the activity of Caspase-1 and IL-1β (14). Additionally, various sensors are known to assemble into classic inflammasomes such as NLRP1, NLRP3, NLRC4, AIM2, and Pyrin (15).
The NLRP3 inflammasome is mainly composed of a signal sensor component (NLRP3) and an adaptor, apoptosis-associated speck-like protein containing a CARD (ASC). The ASC can recruit the proinflammatory caspase, Caspase-1 (16), or the non-classical caspases, Caspase-4 or Caspase-5 (17). In the inflammasome complex, Caspase-1 is composed of CARD and two subunits, p10 and p20, at the N-terminal. NLRP3 and ASC interact with their respective PYRIN domains (PYD), whereas clustered ASC and Caspase-1 interact with their respective caspase recruitment domains (CARD). The NLRP3 inflammasome acts as the molecular platform for Caspase-1 lysis and activation, and the two subunits form the activated Caspase-1 tetramer (18). The activated Caspase-1 processes the precursors IL-1β and IL-18, resulting in the release of mature cytokines and the induction of the inflammatory response in the extracellular environment (Figure 1).
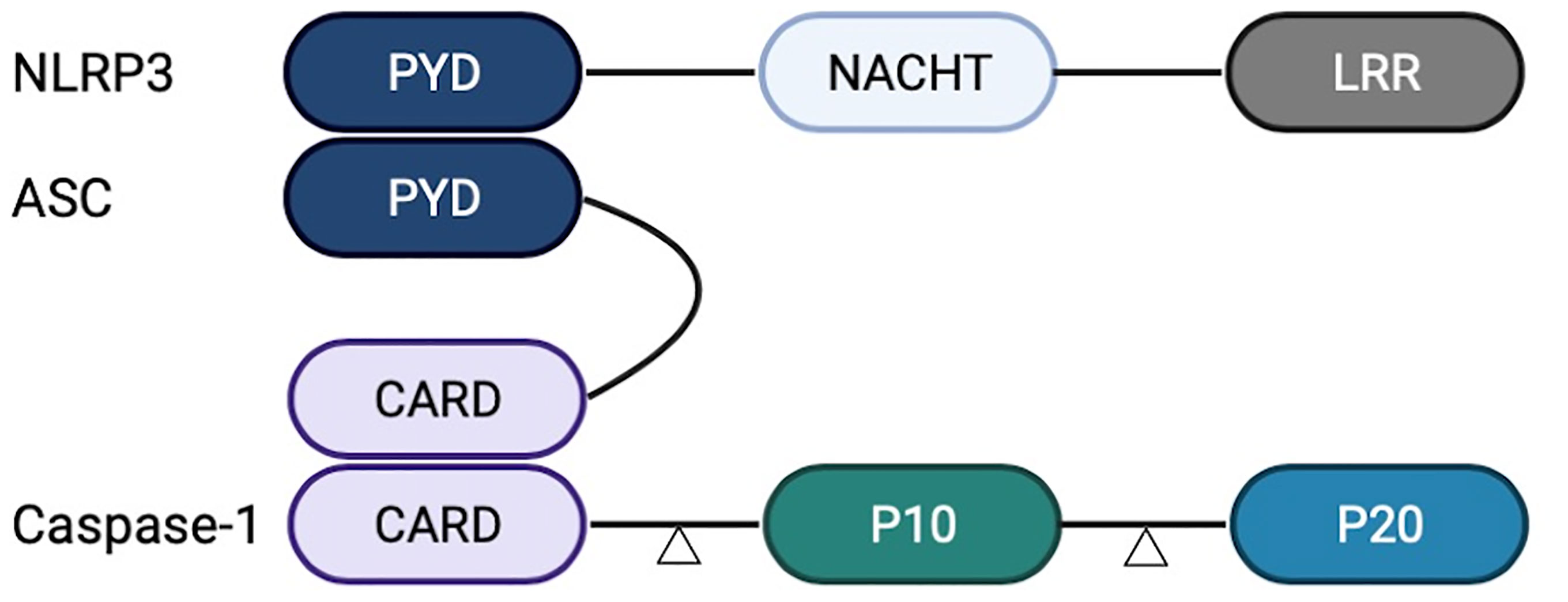
Figure 1 Structure of nucleotide-binding oligomerization domain-like-receptor family pyrin domain-containing 3 (NLRP3) inflammasome. NLRP3 inflammasome comprises a leucine-rich repeat (LRR) domain, an N-terminal Pyrin domain (PYD), and a central adenosine triphosphatase (ATPase) domain known as NACHT. Caspase-1 comprises CARD and two subunits, p10 and p20. NLRP3 and ASC interact with their respective PYDs. ASC and Caspase-1 interact with their respective CARDs.
Regulatory Mechanism of NLRP3 Inflammasome
The recognition of activation signals by inflammasomes is the first step in inflammatory reactions. NLRP3 recognizes two types of extracellular stimulators, pathogen-associated molecular models (PAMPs), such as bacteria and viruses, and danger-associated molecular models (DAMPs), such as uric acid crystals, saturated fatty acids, and cholesterol (19). Next, it induces uniform downstream host-derived cellular events, including K+ efflux, Ca2+ efflux, ROS generation, and lysosomal damage (20). The activation of the NLRP3 inflammasome involves priming and activation. It is believed that the transcription of IL-1β and NLRP3, mediated by NF-κB, is the main event caused by the inflammasomes. In addition, post-translational modification of NLRP3, such as phosphorylation induced by JNK1, is a critical event during the initiation process (21). During inflammasome assembly, NLRP3 interacts with a NIMA-related enzyme (NEK7) through the NACHT domain to form large oligomers that form the basis of its activation (22). Finally, NLRP3 recruits Caspase-1 precursor (pro-Caspase-1) through ASC and promotes the processing of Caspase-1 and the subsequent maturation and secretion of the proinflammatory cytokines IL-1β and IL-18, causing inflammation (23) (Figure 2).
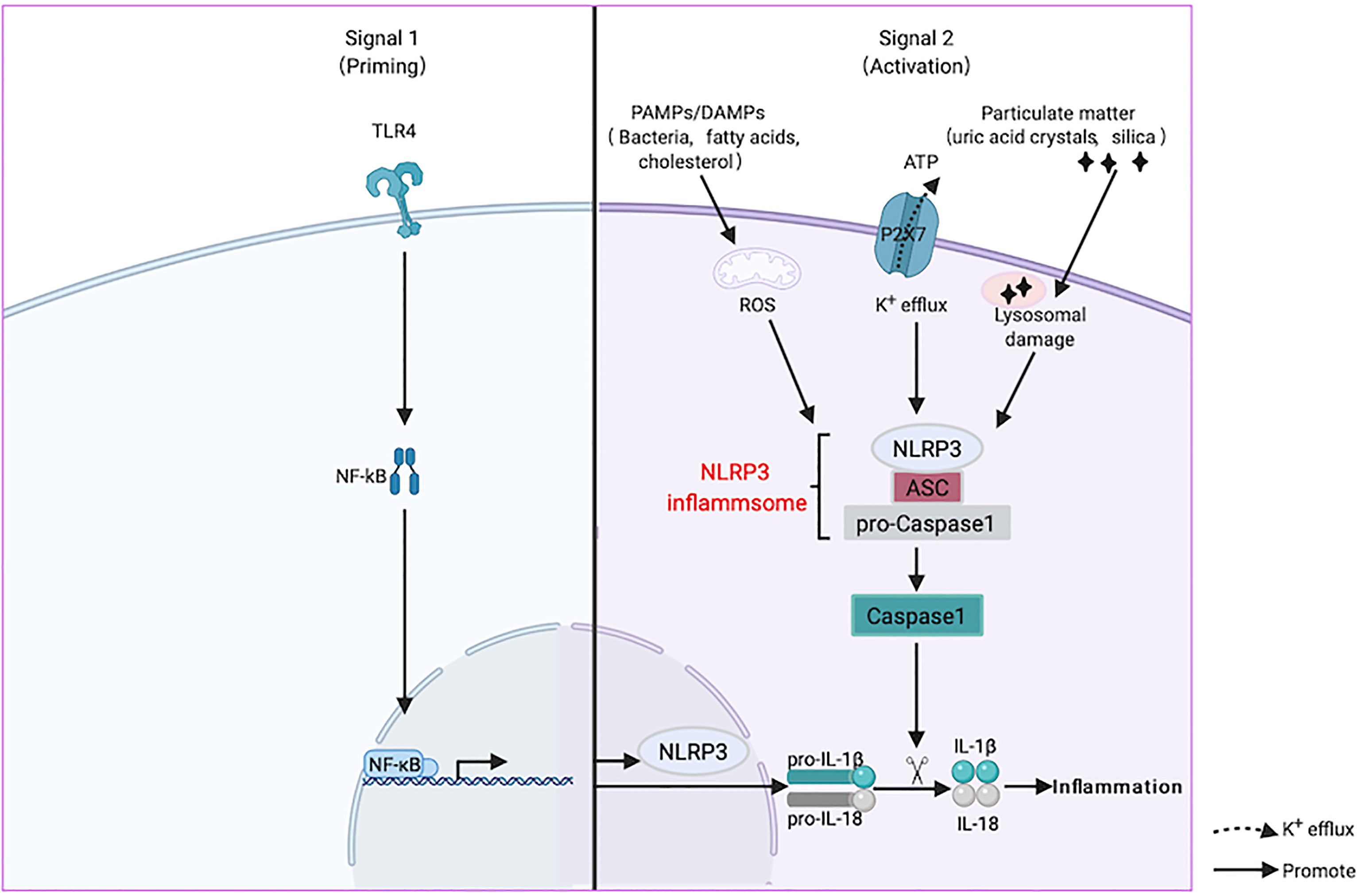
Figure 2 Activation mechanism of nucleotide-binding oligomerization domain-like-receptor family pyrin domain-containing 3 (NLRP3) inflammasome. NLRP3 is activated by two signals when it senses the stimulation of aging or estrogen deficiency through toll like receptors (TLRs). The first priming process (Signal 1) is the expression of NLRP3 and inflammatory factors under the action of the NF-κB transcription factor. Next, it induces uniform downstream host-derived cellular events, including K+ efflux, Ca2+ efflux, reactive oxygen species (ROS) generation, and lysosomal damage. ASC is an adaptor molecule responsible for connecting NLRP3 and caspase-1 precursors, and then recruits the precursor caspase-1 into an activated form (Signal 2). Activated caspase-1 cleaves the precursors of IL-1β and IL-18 into mature forms and causing inflammation.
NLRP3 Inflammasome Mediated IL-1β and IL-18 in OP
Reduced estrogen levels and aging promote low-grade inflammation in the body, and the generated proinflammatory cytokines stimulate OP by affecting the expression and transcription of osteogenic and osteoclastic factors (24, 25). The levels of many inflammatory factors, including IL-1, IL-6, and tumor necrosis factor (TNF)-α, increase during the pathogenesis of OP (26). IL-1β is one of the primary members of the IL-1 family (27) that plays an important role in bone loss following estrogen deficiency (28, 29). A previous study in early postmenopausal women after the discontinuation of estrogen therapy showed that bone resorption is reduced by approximately 50% in subjects randomly receiving anakinra, an IL-1 receptor blocker (30). IL-1β stimulates the expression of Receptor activator of RANKL in osteoblasts or bone marrow mesenchymal stem cells as well as the generation of osteoclasts (25). Besides, IL-1β binds its receptors on T lymphocytes, B lymphocytes, and macrophages, promotes the generation of RANKL, and binds to RANK on osteoclast precursor cells, thus facilitating the differentiation and activation of osteoclasts (31, 32). Therefore, IL-1β is not only an effective bone resorption stimulator but also an effective osteogenic inhibitor. High doses of IL-1β inhibit osteogenic differentiation by activating NF-κB to inhibit the bone morphogenetic protein (BMP)/Smad signal transduction (33). IL-1β decreases Runx2 activation and inhibits the osteoblastic differentiation by activating MAPK pathway (34) (Figure 3).
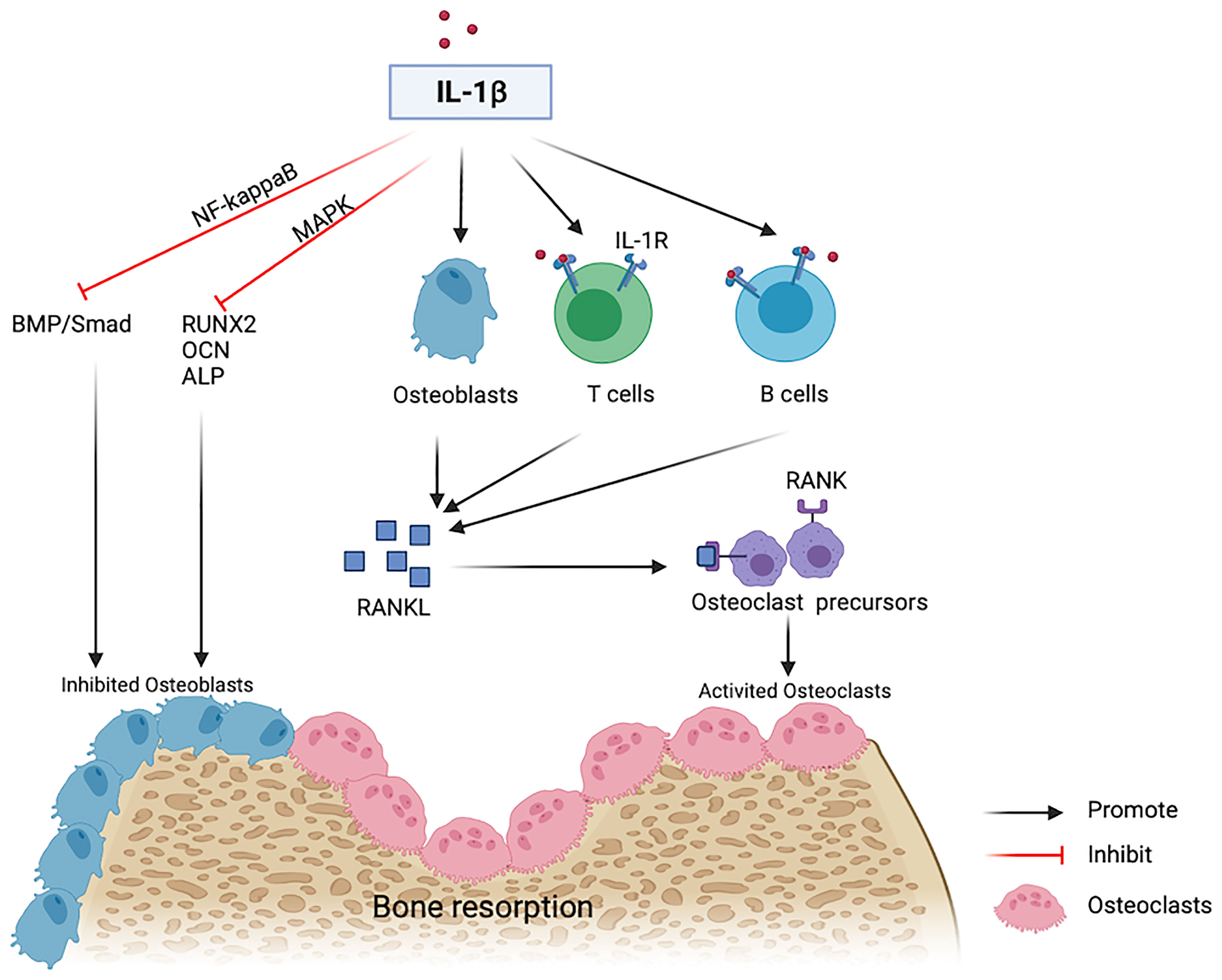
Figure 3 IL-1beta contributes to bone resorption. IL-1β inhibits osteogenic differentiation by inhibiting the BMP/Smad pathway and osteogenic markers including RUNX2, OCN and ALP. IL-1β binds with IL-1R on T cells or B cells and induces the expression of RANKL on osteoblasts and then promotes activated osteoclasts via a RANKL-RANK independent mechanism.
IL-18 and IL-1β are closely related since they belong to the same structural family, have similar 3D structures, and their precursors remain inactive until they are cleaved by intracellular Caspase-1 (35). IL-18 promotes osteoclast differentiation through many pathways. Apart from the bone cells, T helper (Th) cells and various other immunocytes are also major factors participating in bone homeostasis (36). Th17 cells release the marker cytokine IL-17 to upregulate RANKL and promote bone resorption (37). Previous studies in ovariectomized (OVX) mice have suggested that increased IL-18 levels in peripheral monocytes stimulate Th17 cells to secrete IL-17, a process conducive to osteoclast differentiation. In addition, co-culture of osteoblasts with CD4+ T cells and CD11b+ macrophages isolated from OVX mice showed an upregulation of IL-18, activation of NLRP3 inflammasome-related molecules, and inhibition of osteoblasts manifested as reduced expression levels of Wnt-10b, Runt-related transcription factor 2 (Runx-2), and BMP-2 (38). Another in vivo study supported the hypothesis that IL-18 participates in the pathogenesis of OP. In OVX mice, the levels of IL-18 binding protein (IL-18BP, a natural specific IL-18 inhibitor) were declined, whereas IL-18BP supplementation markedly decreased the Th17/Treg ratio and proinflammatory cytokines, restoring the microstructure of bone trabeculae. These findings were confirmed in female patients with OP (38). In serum obtained from patients with Cushing’s syndrome, IL-18 and osteocalcin (OCN) levels were negatively correlated (39). Therefore, IL-1β and IL-18 participate in increased inflammation during OP.
NLRP3 Inflammasome Induces Pyroptosis of Bone Cells in OP
It has been reported that the NLRP3 inflammasome not only aggravates cellular inflammatory response through the Caspase-1/IL-1β/IL-18 activated pathway, but also forms pores on the cell membrane using gasdermin D (GSDMD) as the general substrate, digests the N-terminal domain of GSDMD to bind to the pore of the cell membrane, releases inflammatory mediators, and destructs osmotic pressure, inducing cell swelling, lysis, and death, known as pyroptosis (40). At high glucose concentrations, MC3T3-E1 osteoblasts and Caspase-1 in alveolar bone are activated. Enhanced IL-1β activity increases the expression levels of GSDMD and reduces those of osteogenesis-related proteins, such as p-AKT and β-catenin, whereas Caspase-1 inhibitor can reverse this process (41). In a mouse osteomyelitis model, pyroptosis-related proteins were upregulated, whereas Ac-YVAD-CMK, a specific Caspase-1 inhibitor, not only inhibited the increases of Caspase-1 and GSDMD in mice induced by bacteria, but also helped to restore the osteogenic characteristics (42). Vx765, an inhibitor of Caspase-1, partially reduced bone resorption in apical periodontitis rats, although bone trabecular thickness increased and bone volume did not change significantly. In vitro experiments showed that ROS can induce osteoblast pyroptosis and lead to osteoblast dysfunction (43). Tao et al. (44) summarized the relationship between ROS and NLRP3 in OP, considering that ROS is an important component in the pathogenesis of OP, and speculated that it may be a trigger factor for pyroptosis in this pathological process. Therefore, we hypothesize that the NLRP3 inflammasome not only activates the downstream inflammatory factors to participate in OP pathogenesis, but also induces pyroptosis and maintains or aggravates inflammation (Figure 4).
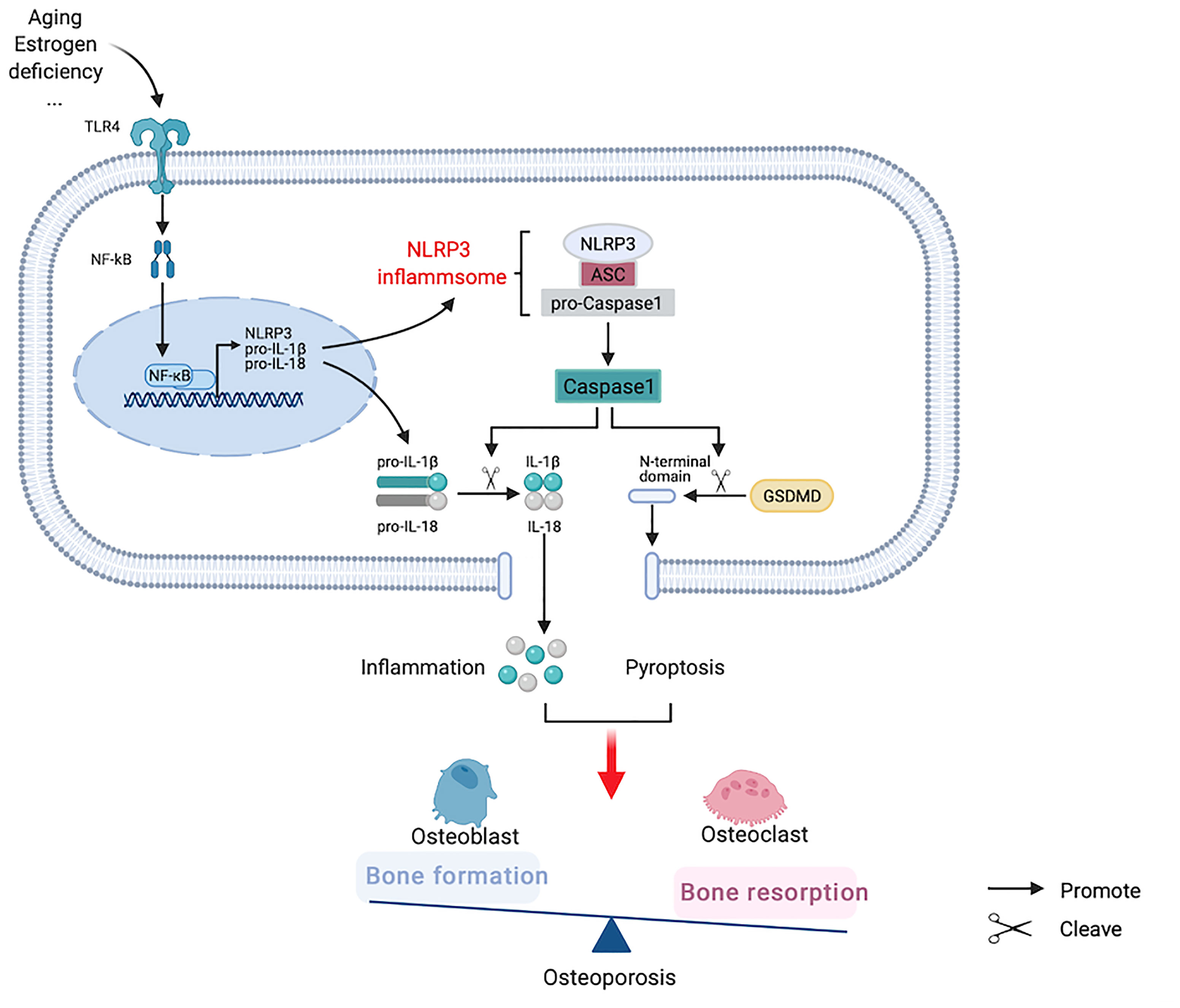
Figure 4 Hypothesized participation of nucleotide-binding oligomerization domain-like-receptor family pyrin domain-containing 3 (NLRP3) inflammasome in the pathogenesis of osteoporosis.
NLRP3 Inflammasome Participates in OP pathogenesis
During the growth and development of bones, the NLRP3 inflammasome and its associated proteins have positive regulatory effects. Compared with NLRP3+/+ mice, NLRP3-/- mice have shorter stature, impaired long bone growth, and defective osteoblast differentiation and mineralization (45). ASC is an essential molecule in the osteoblast phenotype. Compared with osteoblasts obtained from wild-type mice, primary osteoblasts with ASC-source knockout have lower levels of osteogenic properties, and thus, tibial defect healing requires a longer period (46). However, over-activation of the NLRP3 inflammasome is related to osteopenia due to aging. A previous study found that NLRP3 is overexpressed in an aging mouse model, whereas its knockout increased the density of the bone cortex and trabecula (47). A humanized NLRP3 mouse strain created by replacing the mouse NLRP3 locus with the human allele associated with the disease developed progressive arthritis and OP, accompanied by granulocyte infiltration and increased IL-1β levels, after attack by injury-related molecular model molecules (48). Recent studies revealed that in an OVX mouse model, the NLRP3 inflammasome components are upregulated in the femoral bone; however, knockdown of NLRP3 notably enhanced the expression of Runx2 and OCN, which are responsible for osteogenic differentiation (49). Therefore, the NLRP3 inflammasome plays a dual role in bone metabolism, but its abnormal activation produces unfavorable effects in OP development (Table 1). The NLRP3 inflammasome and its components, IL-1β and IL-18, jointly exert effects in OP, whereas the latter may be regulated by NLRP3 inflammasome to result in inflammatory bone injury.
NLRP3 Inflammasome Inhibits Osteogenesis
Bone mesenchymal stem cells (BMSCs) can differentiate into osteoblasts and adipocytes after stimulation by environmental factors (52). During the development of OP, BMSCs exhibit reduced osteogenic capacity and increased fat-forming capacity, resulting in reduced bone formation and increased bone marrow fat accumulation (53, 54). It has been confirmed that the NLRP3 inflammasome is involved in this process and may affect the differentiation of BMSCs via certain active molecules. The group III protein deacetylase, Sirtuin1 (SIRT1), has positive regulatory effects in inhibiting MSC fat formation and promoting bone formation (55). Lipopolysaccharide/palmitic acid (LPS/PA) was previously used to process in vitro MSCs by significantly increasing the expression of NLRP3 inflammasome in MSCs and reducing the expression levels of SIRT1; thus, the NLRP3 inflammasome inhibits osteogenic differentiation by inhibiting SIRT1 and promoting the differentiation of MSCs into adipocytes (51). One study showed that osteogenic differentiation is hampered by the NLRP3 inflammasome in MSCs isolated from the human cord blood and treated with LPS/ATP, probably through the inflammasome assembly, since the siRNA of ASC, the critical component of the targeted inflammasome assembly, can reverse the process (56). However, another study showed that the NLRP3 inflammasome does not promote adipogenic differentiation. Any discrepancies between the studies could be attributed to the different stimulants used.
The activated NLRP3 inflammasome contains multiple exogenous or endogenous substances, but none has been confirmed as the activating substances during bone metabolism. A previous study revealed that NLRP3 is expressed in osteoblasts, mediates cell death induced by bacteria, and participates in bone loss during the inflammatory process (57). In OVX mice, the femur protein and NLRP3 in the osteoblasts are highly expressed even when no direct bacterial infection is observed (49). Since various substances can activate the NLRP3 inflammasome during bone metabolism, it is necessary to investigate the mutual regulation mechanism between the metabolites and the NLRP3 inflammasome in OP due to aging or estrogen deficiency.
NLRP3 Inflammasome Promotes Bone Resorption
Previous in vivo experiments have indicated that the NLRP3 inflammasome accelerates bone resorption under multiple bone turnover states (i.e., estrogen deficiency and persistent parathyroid hormone exposure) and that NLRP3 knockout reduces bone loss in many high bone turnover models (58). Similarly, in osteoclasts exposed to high concentrations of glucose and the rat model of diabetic OP, the expression levels of NLRP3 inflammasome and its related proteins are increased, bone density is reduced, and osteoclast markers are increased. Besides, the overactivated NLRP3 inflammasome can be inhibited by exosomes originating from MSCs (59). It has been reported that the initiation step for NLRP3 inflammasome activation mainly depends on the NF-κB pathway. NF-κB is an inflammation regulation signal downstream of Toll-like receptor (TLR) and the classic inflammatory pathway of the immune response. Several studies have confirmed that the NF-κB pathway has significant effects on the growth and maturation of osteoblasts and osteoclasts (6). At the molecular level, exposure to high glucose concentrations upregulated NLRP3 inflammasome expression and this is regulated by ROS/MAPKs/NF-kB. NF-κB inhibitors significantly reduce the expression level of NLRP3 inflammasome and alleviate bone resorption (50). When bone marrow macrophages (BMMs), which are osteoclast precursors, are exposed to bone matrix particles, the NLRP3 inflammasome is activated, resulting in increased NF-kB and MAPK phosphorylation (58). Therefore, the NLRP3 inflammasome and the classic inflammatory pathway mutually interfere and produce joint effects in osteoclast differentiation, which further confirms the critical role of NF-κB in the initiation of the NLRP3 inflammasome.
Potential Therapeutic Target of NLRP3 Inflammasome in OP
Considering that the NLRP3 inflammasome has dual effects in OP pathogenesis, its regulation may be a novel ideal therapeutic target. Other anti-OP drugs, such as bisphosphonates, parathyroid hormone analogs, and RANKL inhibitors, can only either inhibit bone resorption or promote bone reconstruction to achieve therapeutic effects, and their long-term use has certain safety concerns (1). Previous studies have suggested that bisphosphate, an antiresorptive agent, increases the secretion of NLRP3 dependent IL-1β and induces osteonecrosis in diabetic mice (60). Therefore, combined treatment with an NLRP3 inhibitor might reduce the side effects of bisphosphate, such as jaw necrosis. There are two categories of pharmacological inhibitors for targeting NLRP3 inflammasome: direct inhibitors that directly target NLRP3 protein and some others that are indirect inhibitors, which target constituents of the NLRP3 inflammasome such as Caspase-1, IL-1β and IL-18. Some of the inhibitors that are related to osteoporosis has been reported (Table 2).
Anakinra, a targeted IL-1β inhibitor, has been successfully applied to treat rheumatoid arthritis (68). A clinical study showed that it is also resistant to bone resorption in postmenopausal women (30). Auranofin, another drug used for the treatment of rheumatoid arthritis, significantly reduces bone loss in OVX mice by suppressing osteoclastogenesis induced by RANKL in BMMs and inhibiting IL-1 expression mediated by inflammasomes (67). The drugs, anakinra and auranofin, successfully regulate the cytokine components of the NLRP3 inflammasome to prevent osteoclast-related OP. Although the drugs alone cannot completely block the inflammation, they can be taken orally rather than through injection. Animal experiments have demonstrated that mouse IL-18BP has obvious anti-OP effects, but humanized IL-18BP needs to be further studied (38).
Novel drugs including natural or synthetic molecules that inhibit the maturation or release of IL-1 family cytokines (i.e., NLRP3 inflammasome or Caspase-1 inhibitor) are currently under development and expected to emerge as a new strategy for the treatment of OP, as confirmed by numerous in vivo and in vitro experiments. MCC950, an effective and specific inhibitor of the NLRP3 inflammasome, alleviates the inhibition of MG63 osteoblasts mediated by the NLRP3 inflammasome under oxidative stress (43). In vivo experiments have suggested that NLRP3 knockout and MCC950 supplementation significantly reduces age-related alveolar bone loss in elderly mice. In addition, MCC950 treatment results in higher bone mineral density and bone volume per tissue volume as well as the reduced formation of tartrate-resistant acidic phosphatase (TRAP)-positive cells, delaying osteoclast differentiation (61). Other targeted NLRP3 inflammasome inhibitors, such as OLT1177 and CY-09, also exhibited good therapeutic properties (69). CY-09 reduced bone loss in osteoarthritis modeling. However, the route of administration is achieved by intra-articular injection in the knee (62). At present, the research on OLT1177 in OP has not been reported.
Glyburide, a drug in clinical use, also known as an NLRP3 inflammasome inhibitor, reversed the expression of osteogenic markers such as collagen I and Runx2 and reduced the abnormal activation of osteoclasts (42). However, in this study, the concentration of glyburide far exceeded the concentration of its application in hypoglycemia, which undoubtedly increased other side effects of the drug. In addition, glyburide accelerated the healing of diabetes induced fracture by inhibiting the production of inflammatory factors (63). In a rat model of periodontitis, glyburide suppressed the activation of Caspase-1 and IL-1β, and oral glyburide significantly the inhibited the number of osteoclasts in alveolar bone (64).
It has been found that many natural and endogenous or exogenous molecules exert anti-inflammatory effects by inhibiting NLRP3 inflammasome activation in OP. Arioz ect (70). have summarized the effect of melatonin, a widely used natural and endogenous molecule, on NLRP3 in a variety of different diseases. In vivo experiments have suggested that melatonin inhibits the activation of the NLRP3 inflammasome and improves the inhibition of osteogenic differentiation in OP through the Wnt pathway, which is related to osteogenic differentiation (49). Irisin, another natural molecule, suppressed osteoblast apoptosis and increased the content of ALP in postmenopausal OP rats through the inhibition of NLRP3 inflammasome (65). Dioscin, a plant product, can also inhibit the activation of NLRP3 inflammasome in mouse macrophages and promotes osteogenesis of mouse pre-osteoblasts (57). Therefore, exploring the endogenous and exogenous regulation mechanisms is helpful in improving the understanding of inflammasome activation in the body.
Many studies have focused on the post-transcriptional control of microRNAs (miRNA) based on NLRP3 (71). NLRP3-targeted miRNA is a successful therapeutic method for various diseases, including rheumatoid arthritis and cancer, but little is known about its effectiveness in the treatment of OP (72, 73).
Summary and Outlook
The expression of NLRP3 inflammasome in bone cells affects osteoblast activation and osteoclast differentiation in OP. In addition, it maintains and aggravates the inflammation of osteoblasts or osteoclasts in OP pathogenesis through its downstream inflammatory factors, IL-1β and IL-18, and the induction of Caspase-1-dependent pyroptosis. The NLRP3 inflammasome is involved in bone metabolism by influencing various active molecules and other classic inflammatory pathways. It is obvious that reduced NLRP3 levels delay OP development, but the underlying mechanism involved needs further research. In order to achieve anti-inflammatory functions, the concentration of some drugs is increased, which also increases the possibility of side effects. Therefore, it is necessary to find more suitable drugs, such as MCC950, to inhibit the progress of OP. Basic and clinical studies that provide references for the prevention and treatment of various metabolic diseases are of the utmost necessity.
Author Contributions
NJ, JA, and KY contributed equally to this paper. NJ drafted and prepared manuscript. JA, KY, and CM reviewed and edited the manuscript. JL and CG extracted data and constructed figures with software. XT considered for ideas and overall structure of the article. All authors contributed to the article and approved the submitted version.
Funding
This study was supported by the National Natural Science Foundation of China under grant no. 81370970, and the Science and Technology Support Program of Gansu Province under grants no. 144FKCA075.
Conflict of Interest
The authors declare that the research was conducted in the absence of any commercial or financial relationships that could be construed as a potential conflict of interest.
Publisher’s Note
All claims expressed in this article are solely those of the authors and do not necessarily represent those of their affiliated organizations, or those of the publisher, the editors and the reviewers. Any product that may be evaluated in this article, or claim that may be made by its manufacturer, is not guaranteed or endorsed by the publisher.
Acknowledgments
We would like to thank Editage (www.editage.cn) for English language editing.
References
1. Compston JE, Mcclung MR, Leslie WD. Osteoporosis. Lancet (2019) 393(10169):364–76. doi: 10.1016/S0140-6736(18)32112-3
2. Wright NC, Looker AC, Saag KG, Curtis JR, Delzell ES, Randall S, et al. The Recent Prevalence of Osteoporosis and Low Bone Mass in the United States Based on Bone Mineral Density at the Femoral Neck or Lumbar Spine. J Bone Miner Res (2014) 29(11):2520–6. doi: 10.1002/jbmr.2269
3. Harvey N, Dennison E, Cooper C. Osteoporosis: Impact on Health and Economics. Nat Rev Rheumatol (2010) 6(2):99–105. doi: 10.1038/nrrheum.2009.260
4. Eghbali-Fatourechi G, Khosla S, Sanyal A, Boyle WJ, Lacey DL, Riggs BL. Role of RANK Ligand in Mediating Increased Bone Resorption in Early Postmenopausal Women. J Clin Invest (2003) 111(8):1221–30. doi: 10.1172/JCI200317215
5. Bai XC, Lu D, Bai J, Zheng H, Ke ZY, Li XM, et al. Oxidative Stress Inhibits Osteoblastic Differentiation of Bone Cells by ERK and NF-Kappa B. Biochem Biophys Res Commun (2004) 314(1):197–207. doi: 10.1016/j.bbrc.2003.12.073
6. Novack DV. Role of NF-kappaB in the Skeleton. Cell Res (2011) 21(1):169–82. doi: 10.1038/cr.2010.159
7. Khosla S, Oursler MJ, Monroe DG. Estrogen and the Skeleton. Trends Endocrinol Metab (2012) 23(11):576–81. doi: 10.1016/j.tem.2012.03.008
8. Redlich K, Smolen JS. Inflammatory Bone Loss: Pathogenesis and Therapeutic Intervention. Nat Rev Drug Discovery (2012) 11(3):234–50. doi: 10.1038/nrd3669
9. Rovira-Llopis S, Apostolova N, Banuls C, Muntane J, Rocha M, Victor VM. Mitochondria, the NLRP3 Inflammasome, and Sirtuins in Type 2 Diabetes: New Therapeutic Targets. Antioxid Redox Signal (2018) 29(8):749–91. doi: 10.1089/ars.2017.7313
10. Mcallister MJ, Chemaly M, Eakin AJ, Gibson DS, McGilligan VE. NLRP3 as a Potentially Novel Biomarker for the Management of Osteoarthritis. Osteoarthritis Cartilage (2018) 26(5):612–9. doi: 10.1016/j.joca.2018.02.901
11. Rheinheimer J, De Souza BM, Cardoso NS, Bauer AC, Crispim D. Current Role of the NLRP3 Inflammasome on Obesity and Insulin Resistance: A Systematic Review. Metabol-Clin Exp (2017) 74:1–9. doi: 10.1016/j.metabol.2017.06.002
12. Szekanecz Z, Szamosi S, Kovacs GE, Kocsis E, Benko S. The NLRP3 Inflammasome - Interleukin 1 Pathway as a Therapeutic Target in Gout. Arch Biochem Biophys (2019) 670:82–93. doi: 10.1016/j.abb.2019.01.031
13. Martinon F, Burns K, Tschopp J. The Inflammasome: A Molecular Platform Triggering Activation of Inflammatory Caspases and Processing of ProIL-Beta. Mol Cell (2002) 10(2):417–26. doi: 10.1016/S1097-2765(02)00599-3
14. Agostini L, Martinon F, Burns K, Hawkins PN, Tschopp J. NALP3 Forms an IL-1beta-Processing Inflammasome With Increased Activity in Muckle-Wells Autoinflammatory Disorder. Immunity (2004) 20(3):319–25. doi: 10.1016/S1074-7613(04)00046-9
15. Place DE, Kanneganti TD. Recent Advances in Inflammasome Biology. Curr Opin Immunol (2018) 50:32–8. doi: 10.1016/j.coi.2017.10.011
16. Abderrazak A, Syrovets T, Couchie D, El Hadri K, Friguet B, Simmet T, et al. NLRP3 Inflammasome: From a Danger Signal Sensor to a Regulatory Node of Oxidative Stress and Inflammatory Diseases. Redox Biol (2015) 4:296–307. doi: 10.1016/j.redox.2015.01.008
17. Zheng D, Liwinski T, Elinav E. Inflammasome Activation and Regulation: Toward a Better Understanding of Complex Mechanisms. Cell Discovery (2020) 6:36. doi: 10.1038/s41421-020-0167-x
18. Boucher D, Monteleone M, Coll RC, Chen KW, Ross CM, Teo JL, et al. Caspase-1 Self-Cleavage Is an Intrinsic Mechanism to Terminate Inflammasome Activity. J Exp Med (2018) 215(3):827–40. doi: 10.1084/jem.20172222
19. Jiang D, Chen S, Sun R, Zhang X, Wang D. The NLRP3 Inflammasome: Role in Metabolic Disorders and Regulation by Metabolic Pathways. Cancer Lett (2018) 419:8–19. doi: 10.1016/j.canlet.2018.01.034
20. He Y, Hara H, Nunez G. Mechanism and Regulation of NLRP3 Inflammasome Activation. Trends Biochem Sci (2016) 41(12):1012–21. doi: 10.1016/j.tibs.2016.09.002
21. Song N, Liu ZS, Xue W, Bai ZF, Wang QY, Dai J, et al. NLRP3 Phosphorylation Is an Essential Priming Event for Inflammasome Activation. Mol Cell (2017) 68(1):1–13. doi: 10.1016/j.molcel.2017.08.017
22. Sharif H, Wang L, Wang WL, Magupalli VG, Andreeva L, Qiao Q, et al. Structural Mechanism for NEK7-Licensed Activation of NLRP3 Inflammasome. Nature (2019) 570(7761):338–43. doi: 10.1038/s41586-019-1295-z
23. Tschopp J, Schroder K. NLRP3 Inflammasome Activation: The Convergence of Multiple Signalling Pathways on ROS Production? Nat Rev Immunol (2010) 10(3):210–5. doi: 10.1038/nri2725
24. Cline-Smith A, Axelbaum A, Shashkova E, Chakraborty M, Sanford J, Panesar P, et al. Ovariectomy Activates Chronic Low-Grade Inflammation Mediated by Memory T Cells, Which Promotes Osteoporosis in Mice. J Bone Miner Res (2020) 35(6):1174–87. doi: 10.1002/jbmr.3966
25. Pietschmann P, Mechtcheriakova D, Meshcheryakova A, Foger-Samwald U, Ellinger I. Immunology of Osteoporosis: A Mini-Review. Gerontology (2016) 62(2):128–37. doi: 10.1159/000431091
26. Mclean RR. Proinflammatory Cytokines and Osteoporosis. Curr Osteoporos Rep (2009) 7(4):134–9. doi: 10.1007/s11914-009-0023-2
27. Dinarello C, Arend W, Sims J, Smith D, fcimb.2021.765039 H, O'Neill L, et al. IL-1 Family Nomenclature. Nat Immunol (2010) 11(11):973. doi: 10.1038/ni1110-973
28. Percegoni N, Ferreira AC, Rodrigues CF, Rosenthal D, Castelo Branco MT, Rumjanek VM, et al. Profile of Serum IL-1beta and IL-10 Shortly After Ovariectomy and Estradiol Replacement in Rats. Horm Metab Res (2009) 41(1):50–4. doi: 10.1055/s-0028-1087173
29. Khosla S. Pathogenesis of Age-Related Bone Loss in Humans. J Gerontol A Biol Sci Med Sci (2013) 68(10):1226–35. doi: 10.1093/gerona/gls163
30. Charatcharoenwitthaya N, Khosla S, Atkinson EJ, McCready LK, Riggs BL. Effect of Blockade of TNF-Alpha and Interleukin-1 Action on Bone Resorption in Early Postmenopausal Women. J Bone Miner Res (2007) 22(5):724–9. doi: 10.1359/jbmr.070207
31. Ruscitti P, Cipriani P, Carubbi F, Liakouli V, Zazzeroni F, Di Benedetto P, et al. The Role of IL-1beta in the Bone Loss During Rheumatic Diseases. Mediators Inflamm (2015) 2015:782382. doi: 10.1155/2015/782382
32. Nakamura I, Jimi E. Regulation of Osteoclast Differentiation and Function by Interleukin-1. Vitam Horm (2006) 74:357–70. doi: 10.1016/S0083-6729(06)74015-8
33. Mao CY, Wang YG, Zhang X, Zheng XY, Tang TT, Lu EY. Double-Edged-Sword Effect of IL-1beta on the Osteogenesis of Periodontal Ligament Stem Cells via Crosstalk Between the NF-Kappab, MAPK and BMP/Smad Signaling Pathways. Cell Death Dis (2016) 7:e2296. doi: 10.1038/cddis.2016.204
34. Huang RL, Yuan Y, Tu J, Zou GM, Li Q. Opposing TNF-Alpha/IL-1beta- and BMP-2-Activated MAPK Signaling Pathways Converge on Runx2 to Regulate BMP-2-Induced Osteoblastic Differentiation. Cell Death Dis (2014) 5:e1187. doi: 10.1038/cddis.2014.101
35. Ge Y, Huang M, Yao YM. Recent Advances in the Biology of IL-1 Family Cytokines and Their Potential Roles in Development of Sepsis. Cytokine Growth F R (2019) 45:24–34. doi: 10.1016/j.cytogfr.2018.12.004
36. Srivastava RK, Dar HY, Mishra PK. Immunoporosis: Immunology of Osteoporosis-Role of T Cells. Front Immunol (2018) 9:657. doi: 10.3389/fimmu.2018.00657
37. Ke D, Fu X, Xue Y, Wu H, Zhang Y, Chen X, et al. IL-17A Regulates the Autophagic Activity of Osteoclast Precursors Through RANKL-JNK1 Signaling During Osteoclastogenesis In Vitro. Biochem Biophys Res Commun (2018) 497(3):890–6. doi: 10.1016/j.bbrc.2018.02.164
38. Mansoori MN, Shukla P, Kakaji M, Tyagi AM, Srivastava K, Shukla M, et al. IL-18BP Is Decreased in Osteoporotic Women: Prevents Inflammasome Mediated IL-18 Activation and Reduces Th17 Differentiation. Sci Rep-Uk (2016) 6:33680. doi: 10.1038/srep33680
39. Kristo C, Godang K, Ueland T, Lien E, Aukrust P, Froland S, et al. Raised Serum Levels of Interleukin-8 and Interleukin-18 in Relation to Bone Metabolism in Endogenous Cushing’s Syndrome. Eur J Endocrinol (2002) 146(3):389–95. doi: 10.1530/eje.0.1460389
40. Shi JJ, Zhao Y, Wang K, Shi XY, Wang Y, Huang HW, et al. Cleavage of GSDMD by Inflammatory Caspases Determines Pyroptotic Cell Death. Nature (2015) 526(7575):660–5. doi: 10.1038/nature15514
41. Yang L, Liu J, Shan QS, Geng GN, Shao P. High Glucose Inhibits Proliferation and Differentiation of Osteoblast in Alveolar Bone by Inducing Pyroptosis. Biochem Biophys Res Commun (2020) 522(2):471–8. doi: 10.1016/j.bbrc.2019.11.080
42. Zhu X, Zhang K, Lu K, Shi T, Shen S, Chen X. Staphylococcus Aureusinhibition of Pyroptosis Attenuates -Induced Bone Injury in Traumatic Osteomyelitis. Ann Trans Med (2019) 7(8):170. doi: 10.21037/atm.2019.03.40
43. Liu SS, Du J, Li DF, Yang PP, Kou YY, Li CS, et al. Oxidative Stress Induced Pyroptosis Leads to Osteogenic Dysfunction of MG63 Cells. J Mol Histol (2020) 51(3):221–32. doi: 10.1007/s10735-020-09874-9
44. Tao Z, Wang J, Wen K, Yao R, Da W, Zhou S, et al. Pyroptosis in Osteoblasts: A Novel Hypothesis Underlying the Pathogenesis of Osteoporosis. Front Endocrinol (Lausanne) (2020) 11:548812. doi: 10.3389/fendo.2020.548812
45. Detzen L, Cheat B, Besbes A, Hassan B, Marchi V, Baroukh B, et al. NLRP3 Is Involved in Long Bone Edification and the Maturation of Osteogenic Cells. J Cell Physiol (2021) 236(6):4455–69. doi: 10.1002/jcp.30162
46. Sartoretto S, Gemini-Piperni S, Da Silva R, Calasans M, Rucci N, Pires Dos Santos T, et al. Apoptosis-Associated Speck-Like Protein Containing a Caspase-1 Recruitment Domain (ASC) Contributes to Osteoblast Differentiation and Osteogenesis. J Cell Physiol (2019) 234(4):4140–53. doi: 10.1002/jcp.27226
47. Youm YH, Grant RW, Mccabe LR, Albarado DC, Nguyen LKY, Ravussin A, et al. Canonical Nlrp3 Inflammasome Links Systemic Low-Grade Inflammation to Functional Decline in Aging. Cell Metab (2013) 18(4):519–32. doi: 10.1016/j.cmet.2013.09.010
48. Snouwaert J, Nguyen M, Repenning P, Dye R, Livingston E, Kovarova M, et al. An NLRP3 Mutation Causes Arthropathy and Osteoporosis in Humanized Mice. Cell Rep (2016) 17(11):3077–88. doi: 10.1016/j.celrep.2016.11.052
49. Xu L, Zhang L, Wang Z, Li C, Li S, Li L, et al. Melatonin Suppresses Estrogen Deficiency-Induced Osteoporosis and Promotes Osteoblastogenesis by Inactivating the NLRP3 Inflammasome. Calcif Tissue Int (2018) 103(4):400–10. doi: 10.1007/s00223-018-0428-y
50. An Y, Zhang H, Wang C, Jiao F, Xu H, Wang X, et al. Activation of ROS/MAPKs/NF-Kappab/NLRP3 and Inhibition of Efferocytosis in Osteoclast-Mediated Diabetic Osteoporosis. FASEB J (2019) 33(11):12515–27. doi: 10.1096/fj.201802805RR
51. Wang L, Chen K, Wan X, Wang F, Guo Z, Mo Z. NLRP3 Inflammasome Activation in Mesenchymal Stem Cells Inhibits Osteogenic Differentiation and Enhances Adipogenic Differentiation. Biochem Biophys Res Commun (2017) 484(4):871–7. doi: 10.1016/j.bbrc.2017.02.007
52. Hu L, Yin C, Zhao F, Ali A, Ma J, Qian A. Mesenchymal Stem Cells: Cell Fate Decision to Osteoblast or Adipocyte and Application in Osteoporosis Treatment. Int J Mol Sci (2018) 19(2):360. doi: 10.3390/ijms19020360
53. Choi HK, Yuan H, Fang F, Wei X, Liu L, Li Q, et al. Tsc1 Regulates the Balance Between Osteoblast and Adipocyte Differentiation Through Autophagy/Notch1/beta-Catenin Cascade. J Bone Miner Res (2018) 33(11):2021–34. doi: 10.1002/jbmr.3530
54. Li CJ, Cheng P, Liang MK, Chen YS, Lu Q, Wang JY, et al. MicroRNA-188 Regulates Age-Related Switch Between Osteoblast and Adipocyte Differentiation. J Clin Invest (2015) 125(4):1509–22. doi: 10.1172/JCI77716
55. Sun W, Qiao W, Zhou B, Hu Z, Yan Q, Wu J, et al. Overexpression of Sirt1 in Mesenchymal Stem Cells Protects Against Bone Loss in Mice by FOXO3a Deacetylation and Oxidative Stress Inhibition. Metabol: Clin Exp (2018) 88:61–71. doi: 10.1016/j.metabol.2018.06.006
56. Ahn JS, Seo Y, Oh SJ, Yang JW, Shin YY, Lee BC, et al. The Activation of NLRP3 Inflammasome Potentiates the Immunomodulatory Abilities of Mesenchymal Stem Cells in a Murine Colitis Model. BMB Rep (2020) 53(6):329–34. doi: 10.5483/BMBRep.2020.53.6.065
57. Yin W, Liu S, Dong M, Liu Q, Shi C, Bai H, et al. A New NLRP3 Inflammasome Inhibitor, Dioscin, Promotes Osteogenesis. Small (2020) 16(1):e1905977. doi: 10.1002/smll.201905977
58. Alippe Y, Wang C, Ricci B, Xiao J, Qu C, Zou W, et al. Bone Matrix Components Activate the NLRP3 Inflammasome and Promote Osteoclast Differentiation. Sci Rep (2017) 7(1):6630. doi: 10.1038/s41598-017-07014-0
59. Zhang L, Wang Q, Su H, Cheng J. Exosomes From Adipose Derived Mesenchymal Stem Cells Alleviate Diabetic Osteoporosis in Rats Through Suppressing NLRP3 Inflammasome Activation in Osteoclasts. J Biosci Bioengineering (2021) 131(6):671–8. doi: 10.1016/j.jbiosc.2021.02.007
60. Zhang Q, Yu W, Lee S, Xu Q, Naji A, Le A. Bisphosphonate Induces Osteonecrosis of the Jaw in Diabetic Mice via NLRP3/Caspase-1-Dependent IL-1β Mechanism. J Bone Mineral Res Off J Am Soc Bone Mineral Res (2015) 30(12):2300–12. doi: 10.1002/jbmr.2577
61. Zang Y, Song JH, Oh SH, Kim JW, Lee MN, Piao X, et al. Targeting NLRP3 Inflammasome Reduces Age-Related Experimental Alveolar Bone Loss. J Dent Res (2020) 99(11):1287–95. doi: 10.1177/0022034520933533
62. Li Z, Huang Z, Zhang H, Lu J, Tian Y, Wei Y, et al. P2X7 Receptor Induces Pyroptotic Inflammation and Cartilage Degradation in Osteoarthritis via NF-Kappab/NLRP3 Crosstalk. Oxid Med Cell Longev (2021) 2021:8868361. doi: 10.1155/2021/8868361
63. Yang X, Qu C, Jia J, Zhan Y. NLRP3 Inflammasome Inhibitor Glyburide Expedites Diabetic-Induced Impaired Fracture Healing. Immunobiology (2019) 224(6):786–91. doi: 10.1016/j.imbio.2019.08.008
64. Kawahara Y, Kaneko T, Yoshinaga Y, Arita Y, Nakamura K, Koga C, et al. Effects of Sulfonylureas on Periodontopathic Bacteria-Induced Inflammation. J Dental Res (2020) 99(7):830–8. doi: 10.1177/0022034520913250
65. Xu L, Shen L, Yu X, Li P, Wang Q, Li C. Effects of Irisin on Osteoblast Apoptosis and Osteoporosis in Postmenopausal Osteoporosis Rats Through Upregulating Nrf2 and Inhibiting NLRP3 Inflammasome. Exp Ther Med (2020) 19(2):1084–90. doi: 10.3892/etm.2019.8313
66. Cheng R, Feng Y, Zhang R, Liu W, Lei L, Hu T, et al. The Extent of Pyroptosis Varies in Different Stages of Apical Periodontitis. Biochim Biophys Acta Mol Basis Dis (2018) 1864(1):226–37. doi: 10.1016/j.bbadis.2017.10.025
67. Kim HY, Kim KS, Kim MJ, Kim HS, Lee KY, Kang KW. Auranofin Inhibits RANKL-Induced Osteoclastogenesis by Suppressing Inhibitors of kappaB Kinase and Inflammasome-Mediated Interleukin-1beta Secretion. Oxid Med Cell Longev (2019) 2019:3503912. doi: 10.1155/2019/3503912
68. Kary S, Burmester G. Anakinra: The First Interleukin-1 Inhibitor in the Treatment of Rheumatoid Arthritis. Int J Clin Pract (2003) 57(3):231–4.
69. Yang Y, Wang H, Kouadir M, et al. Recent Advances in the Mechanisms of NLRP3 Inflammasome Activation and its Inhibitors. Cell Death Dis (2019) 10(2):128. doi: 10.1038/s41419-019-1413-8
70. Arioz BI, Tarakcioglu E, Olcum M, Genc S. The Role of Melatonin on NLRP3 Inflammasome Activation in Diseases. Antioxid (Basel) (2021) 10(7):1020. doi: 10.3390/antiox10071020
71. Tezcan G, Martynova EV, Gilazieva ZE, McIntyre A, Rizvanov AA, Khaiboullina SF. MicroRNA Post-Transcriptional Regulation of the NLRP3 Inflammasome in Immunopathologies. Front Pharmacol (2019) 10:451. doi: 10.3389/fphar.2019.00451
72. Xie Q, Wei M, Zhang B, Kang X, Liu D, Zheng W, et al. MicroRNA33 Regulates the NLRP3 Inflammasome Signaling Pathway in Macrophages. Mol Med Rep (2018) 17(2):3318–27. doi: 10.3892/mmr.2017.8224
Keywords: NLRP3, inflammasome, osteoporosis, osteoclasts, osteoblasts
Citation: Jiang N, An J, Yang K, Liu J, Guan C, Ma C and Tang X (2021) NLRP3 Inflammasome: A New Target for Prevention and Control of Osteoporosis? Front. Endocrinol. 12:752546. doi: 10.3389/fendo.2021.752546
Received: 03 August 2021; Accepted: 13 September 2021;
Published: 27 September 2021.
Edited by:
Lucas R Brun, National University of Rosario, ArgentinaReviewed by:
Juan Pablo de Rivero Vaccari, University of Miami, United StatesDivya Singh, Central Drug Research Institute (CSIR), India
Copyright © 2021 Jiang, An, Yang, Liu, Guan, Ma and Tang. This is an open-access article distributed under the terms of the Creative Commons Attribution License (CC BY). The use, distribution or reproduction in other forums is permitted, provided the original author(s) and the copyright owner(s) are credited and that the original publication in this journal is cited, in accordance with accepted academic practice. No use, distribution or reproduction is permitted which does not comply with these terms.
*Correspondence: Xulei Tang, eHVsZWlfdGFuZ0AxMjYuY29t
†These authors have contributed equally to this work