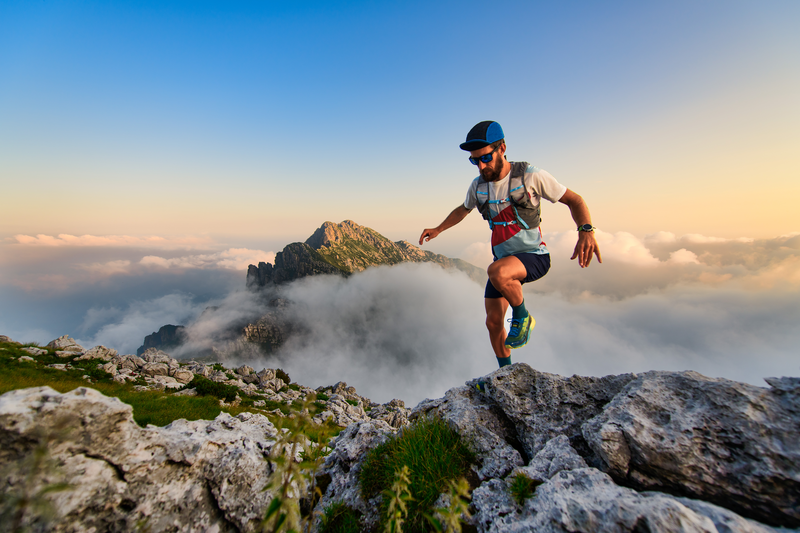
95% of researchers rate our articles as excellent or good
Learn more about the work of our research integrity team to safeguard the quality of each article we publish.
Find out more
REVIEW article
Front. Endocrinol. , 09 June 2022
Sec. Bone Research
Volume 13 - 2022 | https://doi.org/10.3389/fendo.2022.910602
This article is part of the Research Topic New Progress in Understanding and Treatment of Osteoporosis View all 28 articles
In aging society, osteoporotic fractures have become one major social problem threatening the health of the elderly population in China. Compared with conventional fractures, low bone mass, bone defect and retarded healing issues of osteoporotic fractures lead to great difficulties in treatment and rehabilitation. Addressing major concerns in clinical settings, we proposed the “three in one” bone repair strategy focusing on anti-osteoporosis therapies, appropriate bone grafting and fracture healing accelerating. We summarize misconceptions and repair strategies for osteoporotic fracture management, expecting improvement of prognosis and clinical outcomes for osteoporotic fractures, to further improve therapeutic effect and living quality of patients.
Osteoporosis is a systemic metabolic bone disease characterized by decreased bone strength and increased risk of fracture. Osteoporotic fractures refer to fractures caused by force that does not usually cause fractures (1). Data from the first epidemiological survey of osteoporosis among residents in China show that the prevalence of osteoporosis among people over 50 years old is 19.2%, and the prevalence rate over 65 years old is 32%. Among them, the prevalence of females is 5 times that of males of the same age (2). The epidemiological survey data provided by the US Preventive Medicine Working Group show that in 2020, the number of patients with osteoporosis in the population over 50 years old in the United States is expected to reach 12 million (3).
Osteoporotic fractures are the inevitable outcome of long-term and sustained bone loss, which is a major cause of death and disability among the elderly, and brings huge social and economic burdens. In aging society, one osteoporotic fracture occurs every 3 seconds in the world, and about 50% of women and 20% of men have their first osteoporotic fracture after the age of 50 (1). Due to abnormal bone metabolism, compromised bone healing, and relatively long-time immobilization for osteoporotic fractures patients, secondary bone loss and complications including pneumonia, pressure ulcers and thrombosis have brought great difficulties to clinical diagnosis and treatment. According to statistics, 21%~30% of elderly hip fracture patients die within 1 year after injury (3).
Osteoporotic fractures are often dealt with surgeries. But there are three major misconceptions currently popular among orthopedic surgeons in China: insufficient understanding of the primary disease osteoporosis, lacking concept of abnormal bone metabolism environment in osteoporosis, and underestimating bone grafting in surgery. Thus, the clinical outcome is often unsatisfactory.
The author summarized over 20 years of experience in diagnosis and treatment of osteoporotic fractures, and proposed the “Three-in-one” bone repair strategy, emphasizing the concept of vigorous anti-osteoporosis, proper bone grafting and promoting bone healing, and expects to further improve the diagnosis and treatment efficacy and the quality of life of patients.
Compared with fractures due to high-every trauma, osteoporotic fractures show following features: (1) It is more common in the elderly, has more complications, and is difficult to manage and recover; (2) During the fracture, immobilization or reduced exercise results in rapid secondary bone loss; (3) Common bone defects due to compression lead to difficulty in surgical reduction and stability; (4) Improper internal fixation leads to loss of internal fixation failure; (5) The risk of delayed fracture union or nonunion is higher; (6) The risk of re-fracture is significantly increased. However, there are three major misconceptions in the current clinical treatment of osteoporotic fractures.
Osteoporotic fractures result from chronic bone loss due to osteoporosis. Abnormal bone metabolism and weakening of bone formation ability in the body substantially delay the healing of fractures. In the process of diagnosis and treatment of elderly osteoporotic fractures, orthopedic surgeons should fully pay attention to the negative effects of osteogenesis/osteoclastogenesis imbalance on fracture healing. At present, the Chinese people’s awareness of osteoporosis is generally insufficient. Among those >50 years old, the proportion of people who have undergone bone density testing is 3.7%, and the proportion of people with low bone mass is 57.4% (2). In addition, orthopedic surgeons’ attention to the bone mass and quality needs to be improved. More than 50% of patients with osteoporotic fractures have not received systematic anti-osteoporosis treatment, and only <10% of orthopedic doctors choose bone mineral density examinations for patients with first fractures.
Bone maintains dynamic balance coordinated by osteoclast-mediated bone resorption and osteoblast-mediated bone formation (4). Fluctuations in estrogen levels in postmenopausal women lead to excessive activation of osteoclasts, and the bone formation ability by mesenchymal stem cells (MSCs) and osteoblasts gradually decreases with aging (5). The second stage of fracture healing includes the recruitment of MSCs, cartilage callus formation, hard callus formation, and bone remodeling (6). In the case of osteoporosis, the key steps such as blood vessel ingrowth and bone remodeling are compromised, which needs to be regulated by local physical or biochemical factors (7–9). When dealing with osteoporotic fractures, orthopedic surgeons often focus on the alignment and internal fixation of the fracture, while ignore the local abnormal bone metabolism environment.
In patients with osteoporosis, the weakening of bone density and the binding force of the bone-implant interface directly leads to the decrease of screw holding, which increases the risk of internal fixation failure. Abnormal bone metabolism around the screw increases the incidence of internal implant cutting. In addition, low-strength cortical bone and high-porosity cancellous bone structures tend to form large-area bone defects when fractures occur, which also poses challenges to the initial stability of internal fixation. At present, orthopedic surgeons have not paid enough attention to the concept of bone grafting in reduction and fixation, and the stability by internal fixation is insufficient, which is likely to cause the later internal fixation failure and even a second fracture (10).
In the context of chronic bone loss and decreased bone formation ability, the healing rate of osteoporotic fracture patients decreases. Immobilization-related complications by delayed union also inhibit fracture healing. In order to break this vicious circle, orthopedic surgeons should focus on maintaining bone quality, repairing bone defect and promoting bone healing, which will systematically optimize the diagnosis and treatment of osteoporotic fractures.
Osteoporotic fracture is the most severe complication of osteoporosis. The treatment is to promote and accelerate fracture healing. In the past decade, 915 cases of hip fracture aged over 80 years old have been treated in the department of orthopedic trauma of the First Affiliated Hospital of PLA Naval Medical University. All patients achieved a satisfactory prognosis with the guidance of systematic and personalized treatment, including general status adjustment, appropriate implant and enhanced fracture union during the perioperative period (11).
To solve this, the authors summarized the “Three in one” bone repair strategy based on clinical experiences and basic researches, focusing on the baseline bone mass, bone deficiency management and fracture union, and correcting misconceptions in cognition, concept and technique. It is believed that patients with osteoporotic fracture could obtain a better quality of life and a better prognosis through vigorous anti-osteoporosis, appropriate bone grafting and accelerated fracture healing, which are the three core matters of “Three in one” bone repair strategy (Figure 1).
Figure 1 “Three in One” bone repair strategy for osteoporotic fractures. The “Three in one” strategy consists of anti-osteoporosis, bone implantation and fracture healing acceleration.
Intervention of perioperative period bone loss has been well recognized by surgeons all around the world (12, 13). Bone senses mechanical signals during metabolism and converts them into biological signals to adapt biomechanical requirements. During bone remodeling, bone resorption occurs at the site of less stress and bone formation occur at the site of more stress (14). Proper mechanical stimulation regulates bone metabolism through complex signaling pathways such as nerve growth factor-tyrosine kinase receptor 1 (NGF-TrkA), transforming growth factor-beta (TGF-beta). Lacking load results in rapid bone loss at rates of up to 1% per week, compared to physiological bone loss of only 1% per year in normal adults (15, 16). Rapid bone loss exacerbates osteoporosis, prolongs the duration of bed rest and restricts functional exercise, which aggravates the bone loss process in a vicious circle. The assessment of clinical osteoporosis status mainly includes symptoms, laboratory tests, imaging tests and bone density tests. Among the laboratory tests for bone metabolic indicators are mainly the bone formation marker N-terminal propeptide of type I procollagen (P1NP) and the bone resorption marker collagen type-I crosslinked C-peptide (S-CTX). Dual-energy X-ray assay (DXA) is used for bone density test. The difference of T value is used as a standard. It is usually considered that T value ≥ -1.0 SD represents normal bone mass, -1.0 SD > T value > -2.5 SD represents reduced bone mass, and T value ≤ -2.5 SD is osteoporosis, and those with fragility fracture on this basis are severe osteoporosis (1). In addition, muscle impairment and pain that significantly affect disability status in osteoporotic patients and that might be improved by anti-osteoporotic drugs, particularly those with fragility fractures (17, 18).
Factors affecting the stability of internal fixation in osteoporotic fractures mainly include screw holding force and bone healing rate (19, 20). Studies have shown that the level of T-value in DXA examination or the level of bone density in quantitative CT testing is directly related to the risk of internal fixation failure, with a significant increase in the rate of internal fixation failure in patients with local osteoporotic bone density values <95 mg/cm3 (19). In a study of elderly osteoporotic proximal femur fractures, the incidence of screw cut-out was found to be significantly higher in the femoral bone density value <250 mg/cm3 group (21). Interestingly, cortical bone thickness is a key factor affecting screw holding, and a 1 mm decrease in cortical bone thickness is associated with a subsequent decrease in screw holding force of 1000 N (or 50%) (22).
Perioperative anti-osteoporosis treatment has become a consensus worldwide (Figure 2) (25). Perioperative anti-osteoporosis treatment mainly consists of both basic supplements and anti-osteoporotic drug therapy. The average daily intake of elemental calcium in the elderly population is approximately 400 mg, and still need an additional supplementation of about 600 mg calcium carbonate and calcium citrate (26). Active vitamin D is routinely used with calcium, guidelines and expert consensus recommend an osteoporosis treatment dose of approximately 800 to 1200 IU daily (1). As for anti-osteoporosis drugs, the first-line drugs commonly used in clinical practice are bisphosphonates (27). Bisphosphonates inhibit the bone resorption effect of osteoclasts after binding to hydroxyapatite and are more effective in the treatment of postmenopausal osteoporosis characterized by excessive osteoclast activation (28). Currently, a novel osteoclastic activity inhibitor, Denosumab, has been used clinically as a monoclonal antibody to nuclear factor-κB (NF-κB) receptor activator ligand (RANKL) that specifically inhibits osteoclasts differentiation, thereby reducing bone resorption and improving bone quality (29, 30). It is worth noting that, recent advances have shown that the nuclear factor-κB receptor-ligand (RANK-RANKL) signaling pathway promotes osteoclast maturation while inhibiting the osteogenic differentiation process of mesenchymal cells (31–33). In response to age-related osteoporosis, in which decreased osteogenic capacity is the main pathological factor, the efficacy of drugs that modulate MSC osteogenic differentiation is relatively favorable (34–36). Teriparatide, a parathyroid hormone analog, can promote new bone formation and enhance bone healing efficiency when administered intermittently at low doses (37).
Figure 2 Treat osteoporosis. (A) Bedridden leads to accelerated bone loss (23). (B) Current drug treatment. 1. Estrogen & SERM, 2. Calcitonin, 3. Bisphosphonate, 4. Sclerostin antibody, 5. Denosumab (24).
Appropriate compressive stress should be applied to promote fracture healing in accordance according to the classic AO theory (38, 39). Sufficient bone grafting is vital for anatomical reduction, stability and microenvironment improvement. Reliable initial stability and cross-sectional compressive stress are difficult to obtain because the local cancellous bone is sparse and the strength of cortical bone is low in the fracture site of patients with osteoporosis and thus bone grafting is commonly used. The intraoperative bone grafting is helpful to restore and maintain the normal anatomy and alignment of the limb and articular surface, and increase the holding force of the internal fixation. Autologous fibular segment transplantation is helpful in internal fixation of comminuted proximal humeral fractures with insufficient medial support (40). The application of artificial bone and allograft bone filling in internal fixation of tibial plateau fractures has been recognized (41, 42).
Compared with conventional fractures by high energy trauma, osteoporotic fractures have larger bone defects and are more difficult to maintain stability after internal fixation, which laid higher requirements for the volume and technology of bone grafting materials (43). Autologous bone is the best bone grafting material with low immunogenicity, good bone induction and mechanical conduction. Nevertheless, a large number of autologous bone increase risks of infection, pain and even iatrogenic fracture (44). To improve the utilization rate of autologous bone, clinicians are supposed to take some measures to promote the success of bone grafting which requires reliable fixation and sufficient blood supply. The cushion method enhances the holding force of the screw end and the stability of internal fixation by inserting the screw into the contralateral cortical bone. In order to fully repair bone defects and achieve early postoperative functional exercise and rehabilitation, various bone grafting skills can be used when dealing with huge bone defects (Figure 3).
Figure 3 Emphasize proper internal fixation and bone implantation. (A) Improvement in bone grafting method. a cushion method; b splint method; c seeding method (45). (B) A 74-year-old patient with proximal humerus fractures treated with open reduction and internal fixation with allograft bone graftings. a preoperative image; b immediate postoperative image; c 12 months after surgery (46). (C)a. A 55-year-old male patient with humeral shaft fracture, nonunion occurred 6 months after internal fixation using a dynamic compression plate due to screw breakage; (b) Four months after implantation of shape memory Ni-Ti alloy swan-like bone connector fixation and autologous bone grafting (45).
Currently, a large number of available allogeneic bone and artificial bone materials in clinical application can be selected by orthopedic surgeons based on specific demands (47, 48). Biomaterials could promote fracture healing by stimulating the bone formation ability of skeletal stem cells as well as providing tissue growing scaffolds. The new biological mesoporous materials are absorbed and degraded by osteoclasts, followed by the formation of hydroxyapatite and bone matrix mineralization (49). Bone cement reinforcement technology can reduce the occurrence of internal fixation loosening and reduction loss in the decompression, reduction and pedicle screw internal fixation of osteoporotic vertebral compression fracture (50). Non-self-heating phosphate bone cement materials is worthy of attention (51). The new inorganic mesoporous microsphere material can promote the growth and effective integration of bone tissue locally, which promote fracture healing while realizing mechanical filling (52).
The stability of the traditional internal fixation depends on the friction between the internal fixation and the bones (53). However, the stability is significantly compromised in osteoporosis, and complications such as screw cutting and loosening are often. The principle of choice of internal fixation for osteoporotic fractures is to increase stability and preserve bone mass. In terms of plates selection, the locking plate is preferred due to less dependence on the holding force of the screw. The multi-angle fixation based on the anatomical shape further increases the stability. When dealing with fractures such as proximal humeral fractures, the cement intensifying technology of the screw channel can effectively increase the screw holding force, and relatively reduce the risk of humeral head collapse and screw cutting (54, 55). When dealing with non-load-bearing diaphyseal fractures, the new shape memory alloy bone connector provides continuous and effective axial stress stimulation for the fracture end while resisting shear, bending and rotation, which is conducive to fracture healing (56).
Apart from the nature of the biomaterial itself, the biologically active factors loaded also play an important role in the process of fracture healing (57, 58). Biomaterials loaded with bone morphogenetic protein-2 (BMP-2) can promote osteogenic differentiation and bone repair (59). In addition, the problem that the drug concentration continues to decrease when the carrier releases biological factors locally cannot be avoided, and new bone-targeted drug carriers such as exosomes show considerable therapeutic potential, and the local biological microenvironment regulation of fractures can be achieved through targeted drug delivery. The effect of metal ions on local stimulation of fracture healing has been confirmed. The novel magnesium-based bone cement material can activate the osteoblast signal pathway while filling the defect to improve the bone repair effect (60, 61).
The functional recovery of patients with osteoporotic fractures is restricted by multiple factors, and the reduced stability of internal fixation renders higher risks to functional exercise. Appropriate rehabilitation aids allow the fracture site to withstand less shear stress and improve safety (62, 63). Under the concept of accelerating bone healing, perioperative functional exercises protected by rehabilitation aids are essential to break the vicious circle and accelerate bone healing. The rigid support provided by the spinal orthosis can assist patients with vertebral compression fractures to walk after surgery, reducing the load and pain of the spine. Foot rehabilitation aids have good results in postoperative applications of calcaneal fractures and ankle fractures (64). Some elderly patients with osteoporotic fractures have more complications and relatively high surgical risks. When choosing non-surgical treatment, attention should be paid to weight-free exercise under the protection of rehabilitation aids to speed up the process of bone healing.
The pathogenic mechanism is the basis of developing novel drugs or interventions. The understanding of osteoclastogenesis has led to the development and application of bisphosphonates and RANKL inhibitors. Although the osteoclasts inhibiting strategies have been used in clinical experience for decades, there are still side effects unsolved. For example, untypical fractures and osteonecrosis brought by bisphosphonates might be well handled by latest candidate of anti-osteoclastogenesis drug (65). So far, interesting progresses have been reported by surgeons and researchers.
The actin-bundling protein L-plastin (LPL) mediates the resorption activity of osteoclasts, but its therapeutic potential in pathological bone loss remains unexplored. Here, we report that LPL regulates osteoclasts fusion, and targeting LPL serves as a novel anabolic therapy for pathological bone loss. (Targeting actin-bundling protein L-plastin as an anabolic therapy for bone loss (Figure 4A) (35).
Figure 4 Explore pathogenic mechanism of osteoporosis. (A) Targeting L-plastin prevents pre-osteoclasts fusion and promotes PDGF-BB secretion (35). (B) RANKL signaling inhibits osteoblastic differentiation of BMSCs (31). (C) Bone marrow adipocyte-derived RANKL controls trabecular osteoclastogenesis (66). (D) PGC-1α controls skeletal stem cell fate and bone-fat balance in osteoporosis and skeletal aging (67).
RANKL signaling is essential for osteoclastogenesis (68). Its role in osteoblastic differentiation and bone formation is unknown. Our results reveal that RANKL signaling regulates both osteoclasts and osteoblasts by inhibition of osteogenic differentiation of BMSCs and promotion of osteoclastogenesis. This study interprets the underlying mechanisms of unexpected bone formation by RANKL blocking and explains the sustained bone volume increase among patients taking RANKL antibodies (Figure 4B) (31).
Receptor activator of NF-κB ligand (RANKL) is essential for osteoclast formation and bone remodeling (69, 70). Nevertheless, the cellular source of RANKL for osteoclastogenesis has not been fully uncovered. BM adipose lineage cells therefore represent an essential source of RANKL for the formation of trabecula osteoclasts and resorption of cancellous bone during remodeling under physiological and pathological conditions. Targeting bone marrow adiposity is a promising way of preventing pathological bone loss (Figure 4C, D) (66, 67).
Various mesoporous biomaterials are exploited and applied as efficient nanocarriers to loading drugs by virtue of their large surface area, high porosity, and prominent biocompatibility. Herein, recent progress and assembly mechanisms on mesoporous inorganic biomaterials are summarized systematically, and typical functionalization methods for nanocarriers are also discussed in depth. Particularly, structure-activity relationship and the effect of physicochemical parameters of mesoporous biomaterials in DDS application are overviewed. As one of the important development directions, advanced stimuli-responsive DDSs are highlighted. Finally, the prospect of mesoporous biomaterials in disease therapeutics is stated, and it will open a new stage for the development of mesoporous nanocarriers (Figure 5A) (71).
Figure 5 Develop bone grafting biomaterials. (A) The advantages of tailored mesoporous nanocarriers (71). (B) Endothelial cell-secreted exosomes could be internalized by bone marrow-derived macrophages and inhibit osteoclastogenesis (61). (C) The bone-targeted hybrid nanoparticle that promotes osteoclastogenesis and inhibits adipogenesis of BMSCs (72). (D) The potential applications of bone organoid (73).
Though the relationship between vascular and bone homeostasis has been recognized recently, the role of vascular endothelial cell (EC)-secreted exosomes (EC-Exos) in bone homeostasis is not well understood. Herein, we found that EC-Exos inhibit osteoclast activity in vitro and inhibit osteoporosis in an ovariectomized mouse model. Our findings suggest that EC-Exos may be utilized as a bone targeting and nontoxic nanomedicine for the treatment of bone resorption disorders (Figure 5B) (61).
The differentiation shift from osteogenesis to adipogenesis of bone marrow mesenchymal stem cells (BMSCs) characterizes many pathological bone loss conditions (74, 75). Stromal cell-derived factor-1 (SDF1) is highly enriched in the bone marrow for C-X-C motif chemokine receptor 4 (CXCR4)-positive hematopoietic stem cell (HSC) homing and tumor bone metastasis. In this study, we fused CXCR4+ exosomes with liposomes carrying antagomir-188 to produce hybrid nanoparticles (NPs). The hybrid NPs promoted osteogenesis and inhibited adipogenesis of BMSCs and thereby reversed age-related trabecular bone loss. Taken together, this study presents a novel way to obtain bone-targeted exosomes via surface display of CXCR4 and a promising anabolic therapeutic approach for age-related bone loss (Figure 5C) (72). Based on the above study, we summarized strategies of engineered extracellular vesicles for bone therapy (76, 77).
Bone regeneration is a key issue in the clinics. Bone tissue engineering technology provides various types of functional scaffold materials and seed cells for bone repair (78, 79). However, the bone metabolism mechanism is complicated. Organoids as a new concept, which is built in vitro with the help of tissue engineering technology, can simulate the complex biological functions of organs in vivo. Once proposed, it shows broad application prospects in the research of organ development, drug screening, mechanism study, and so on. As a complex and special organ, bone organoid construction itself is quite challenging. In this study, we introduced the characteristics of bone microenvironment, the concept of organoids, focused on the research progress of bone organoids, and proposed the strategies for bone organoid construction, study direction, and application prospects (Figure 5D) (73).
“Three in one” bone repair strategy reflects and summarizes the wisdom of dealing with osteoporotic fractures. Wang et al. reported that bisphosphonate and non-bisphosphonate medications for osteoporosis were significantly associated with decreased mortality after fragility hip fracture (80). Tai et al. showed that anti-osteoporosis treatment after hip fracture is associated with lower all-cause mortality (81). A longer duration of treatment was also associated with lower mortality. Besides, studies reported that muscle impairment and pain significantly affecting disability in osteoporotic patients might be improved by anti-osteoporotic drugs, particularly those with fragility fractures (17, 18). Although bone morphogenetic proteins (BMPs) show their potential roles in promoting fracture healing by increasing osteoblastic formation in osteoporotic patients, there has been no current published clinical data (82). Limited evidence of animal studies suggests that BMPs could stimulate fracture healing in ovariectomized rats (83). Therefore, the therapeutic effects of BMPs in osteoporotic fractures should be verified with further clinical trials. As for rehabilitation, although currently there has been no conclusion on the intensity, frequency and duration of postoperative rehabilitation, many studies reported positive effects of early activity and rehabilitation on functional recovery (84). These studies are somewhat consistent with the “Three in one” strategy stressing on anti-osteoporosis therapy, intraoperative bone grafting and rehabilitation, although reported independently.
Based on the “Three in one” bone repair strategy, we drafted three experts on important clinical problems in the treatment of osteoporotic fractures: Expert consensus on bone repair strategies for osteoporotic fractures in China (2019) (Consensus 1) (85), Chinese expert consensus on perioperative management of osteoporotic fractures (2018) (Consensus 2) (86), Expert consensus on lower limp periarticular osteoporotic fractures (Consensus 3 and 4) (2020) (87, 88).
We believe that the perioperative period is critical for orthopedic surgeons to manage osteoporosis and osteoporotic fractures. In view of the current misconceptions in osteoporotic fracture treatment, our team published Consensus 2, which elaborates the surgical protocols, rehabilitation time points and complication management of osteoporotic fracture treatment, and emphasizes the necessity and strategies of perioperative anti-osteoporosis treatment widely recognized in clinical practices (86). With regards to technical misconceptions, the team further established Consensus 1, which focused on the features of bone defects, clinical bone repair materials and repair strategies for common fractures based on Consensus 2, to promote the clinical application of proper bone grafting for osteoporotic fractures (85). As for the treatment of the most prevalent fractures, especially hip and vertebral fracture, the team developed Consensus 3, aiming to summarize the experience of clinical treatment of osteoporotic fracture to provide a systemic rehabilitation treatment plan, and promote the individualized fracture management (2020; 2020).
Osteoporotic fracture is not just a simple local fracture, but a manifestation of abnormal systemic bone metabolism. In the clinical diagnosis and treatment of osteoporotic fractures, the lack of understanding of the primary disease, bone metabolism environment, and the technical underestimation of bone grafting leads to unsatisfactory prognosis.
Osteoporotic fractures heal slowly, and long-term bed rest accelerates bone loss, forming a vicious circle. The key to breaking the vicious circle is to accelerate bone healing. China has entered an aging society. With the aging of the population and the improvement of quality of life, elderly patients with osteoporotic fractures urgently need faster and better treatment. Orthopedic surgeons need to understand the abnormal state of bone metabolism, choose the appropriate implants for fracture fixation, and appropriately use bone healing materials and rehabilitation aids to accelerate fracture healing under the concept of “Three in One” bone repair strategy. The purpose is to improve the clinical prognosis of elderly patients with osteoporotic fractures.
XC, YH, and ZG contributed equally to this work. JS conceived the manuscript. XC, YH and ZG wrote the manuscript. JS and XC reviewed and edited the manuscript. All authors listed have made a substantial, direct and intellectual contribution to work, and approved it for publication.
This project was supported by the National Key R&D Program of China (2018YFC2001500); National Natural Science Foundation of China (82172098, 81871099, 91749204, 81771491).
The authors declare that the research was conducted in the absence of any commercial or financial relationships that could be construed as a potential conflict of interest.
All claims expressed in this article are solely those of the authors and do not necessarily represent those of their affiliated organizations, or those of the publisher, the editors and the reviewers. Any product that may be evaluated in this article, or claim that may be made by its manufacturer, is not guaranteed or endorsed by the publisher.
1. Reid IR. A Broader Strategy for Osteoporosis Interventions. Nat Rev Endocrinol (2020) 16(6):333–9. doi: 10.1038/s41574-020-0339-7
2. Zhang L. Status Quo and Challenge of Osteoporotic Fracture in the Elderly. Chin J Mult Organ Dis Elderly (2020) 19(7):4. doi: 10.11915/j.issn.1671-5403.2020.07.114
3. Curry SJ, Krist AH, Owens DK, Barry MJ, Caughey AB, Davidson KW, et al. Screening for Osteoporosis to Prevent Fractures: US Preventive Services Task Force Recommendation Statement. Jama (2018) 319(24):2521–31. doi: 10.1001/jama.2018.7498
4. Hu Y, Chen X, Wang S, Jing Y, Su J. Subchondral Bone Microenvironment in Osteoarthritis and Pain. Bone Research (2021) 9(1):1–13. doi: 10.1038/s41413-021-00147-z
5. Zhu J, Liu Y, Chen C, Chen H, Huang J, Luo Y, et al. Cyasterone Accelerates Fracture Healing by Promoting MSCs Migration and Osteogenesis. J Orthop Translat (2021) 28:28–38. doi: 10.1016/j.jot.2020.11.004
6. Sun X, Li X, Qi H, Hou X, Zhao J, Yuan X, et al. MiR-21 Nanocapsules Promote Early Bone Repair of Osteoporotic Fractures by Stimulating the Osteogenic Differentiation of Bone Marrow Mesenchymal Stem Cells. J Orthop Translat (2020) 24:76–87. doi: 10.1016/j.jot.2020.04.007
7. Einhorn TA, Gerstenfeld LC. Fracture Healing: Mechanisms and Interventions. Nat Rev Rheumatol (2015) 11(1):45–54. doi: 10.1038/nrrheum.2014.164
8. Dou C, Li J, He J, Luo F, Yu T, Dai Q, et al. Bone-Targeted pH-Responsive Cerium Nanoparticles for Anabolic Therapy in Osteoporosis. Bioact Mater (2021) 6(12):4697–706. doi: 10.1016/j.bioactmat.2021.04.038
9. Liu J, Zhang J, Lin X, Boyce BF, Zhang H, Xing L. Age-Associated Callus Senescent Cells Produce TGF-β1 That Inhibits Fracture Healing in Aged Mice. J Clin Invest (2022) 132(8):e148073. doi: 10.1172/jci148073
10. Guo J, Pan S, Su J. Focal Issues and Strategies for Treatment of Periarticular Osteoporotic Fractures. Chin J Traumatol (2020) 36(8):5. doi: 10.3760/cma.j.issn.1001-8050.2020.08.001
11. Cao L, Chen X, Weng W, Cui J, Wang L, Zhang J, et al. Surgical Treatment Options for Hip Fractures in Elderly Patients Aged 80 Years Old and Over: A Report of 915 Cases. Acad J Second Mil Med Univ (2017) 38(4):6. doi: 10.16781/j.0258-879x.2017.04.0409
12. Sievänen H. Immobilization and Bone Structure in Humans. Arch Biochem Biophys (2010) 503(1):146–52. doi: 10.1016/j.abb.2010.07.008
13. Rolvien T, Milovanovic P, Schmidt FN, von Kroge S, Wölfel EM, Krause M, et al. Long-Term Immobilization in Elderly Females Causes a Specific Pattern of Cortical Bone and Osteocyte Deterioration Different From Postmenopausal Osteoporosis. J Bone Miner Res (2020) 35(7):1343–51. doi: 10.1002/jbmr.3970
14. Seeman E. Bone Modeling and Remodeling. Crit Rev Eukaryot Gene Expr (2009) 19(3):219–33. doi: 10.1615/critreveukargeneexpr.v19.i3.40
15. Tsakalakos N, Magiasis B, Tsekoura M, Lyritis G. The Effect of Short-Term Calcitonin Administration on Biochemical Bone Markers in Patients With Acute Immobilization Following Hip Fracture. Osteoporos Int (1993) 3(6):337–40. doi: 10.1007/bf01637320
16. Tomlinson RE, Li Z, Li Z, Minichiello L, Riddle RC, Venkatesan A, et al. NGF-TrkA Signaling in Sensory Nerves is Required for Skeletal Adaptation to Mechanical Loads in Mice. Proc Natl Acad Sci USA (2017) 114(18):E3632–e3641. doi: 10.1073/pnas.1701054114
17. Iolascon G, Moretti A, Giamattei MT, Migliaccio S, Gimigliano F. Prevalent Fragility Fractures as Risk Factor for Skeletal Muscle Function Deficit and Dysmobility Syndrome in Post-Menopausal Women. Aging Clin Exp Res (2015) 27(Suppl 1):S11–16. doi: 10.1007/s40520-015-0417-1
18. Moretti A, de Sire A, Curci C, Toro G, Gimigliano F, Iolascon G. Effectiveness of Denosumab on Back Pain-Related Disability and Quality-of-Life in Patients With Vertebral Fragility Fractures. Curr Med Res Opin (2019) 35(1):151–5. doi: 10.1080/03007995.2018.1545636
19. Konstantinidis L, Helwig P, Hirschmüller A, Langenmair E, Südkamp NP, Augat P. When is the Stability of a Fracture Fixation Limited by Osteoporotic Bone? Injury (2016) 47 Suppl 2:S27–32. doi: 10.1016/s0020-1383(16)47005-1
20. Tian L, Tang N, Ngai T, Wu C, Ruan Y, Huang L, et al. Hybrid Fracture Fixation Systems Developed for Orthopaedic Applications: A General Review. J Orthop Translat (2019) 16:1–13. doi: 10.1016/j.jot.2018.06.006
21. Bonnaire F, Weber A, Bösl O, Eckhardt C, Schwieger K, Linke B. “Cutting Out” in Pertrochanteric Fractures–Problem of Osteoporosis? Unfallchirurg (2007) 110(5):425–32. doi: 10.1007/s00113-007-1248-0
22. von Rüden C, Augat P. Failure of Fracture Fixation in Osteoporotic Bone. Injury (2016) 47(Suppl 2):S3–s10. doi: 10.1016/s0020-1383(16)47002-6
23. Rudner L, Chai D. A Mars Mission Simulation to Determine the Efficacy of 0.38 G as a Countermeasure to Microgravity Induced Bone Demineralization. SAE Tech Papers (2000). doi: 10.4271/2000-01-2245
24. Kim B, Cho YJ, Lim W. Osteoporosis Therapies and Their Mechanisms of Action (Review). Exp Ther Med (2021) 22(6):1379. doi: 10.3892/etm.2021.10815
25. Qaseem A, Forciea MA, McLean RM, Denberg TD, Barry MJ, Cooke M, et al. Treatment of Low Bone Density or Osteoporosis to Prevent Fractures in Men and Women: A Clinical Practice Guideline Update From the American College of Physicians. Ann Intern Med (2017) 166(11):818–39. doi: 10.7326/m15-1361
26. Cosman F, de Beur SJ, LeBoff MS, Lewiecki EM, Tanner B, Randall S, et al. Clinician's Guide to Prevention and Treatment of Osteoporosis. Osteoporos Int (2014) 25(10):2359–81. doi: 10.1007/s00198-014-2794-2
27. Wang Y, Chen X, Zhi X, Su J. Research Progress in Effect of Alendronate on Healing of Osteoporotic Fracture. Chin J Clin Med (2020) 36(1):5. doi: 10.3760/cma.j.issn.1001-8050.2020.01.013
28. Black DM, Rosen CJ. Clinical Practice. Postmenopausal Osteoporosis. N Engl J Med (2016) 374(3):254–62. doi: 10.1056/NEJMcp1513724
29. Cummings SR, San Martin J, McClung MR, Siris ES, Eastell R, Reid IR, et al. Denosumab for Prevention of Fractures in Postmenopausal Women With Osteoporosis. N Engl J Med (2009) 361(8):756–65. doi: 10.1056/NEJMoa0809493
30. Zhu Y, Huang Z, Wang Y, Xu W, Chen H, Xu J, et al. The Efficacy and Safety of Denosumab in Postmenopausal Women With Osteoporosis Previously Treated With Bisphosphonates: A Review. J Orthop Translat (2020) 22:7–13. doi: 10.1016/j.jot.2019.08.004
31. Chen X, Zhi X, Wang J, Su J. RANKL Signaling in Bone Marrow Mesenchymal Stem Cells Negatively Regulates Osteoblastic Bone Formation. Bone Res (2018) 6:34. doi: 10.1038/s41413-018-0035-6
32. Ikebuchi Y, Aoki S, Honma M, Hayashi M, Sugamori Y, Khan M, et al. Coupling of Bone Resorption and Formation by RANKL Reverse Signalling. Nature (2018) 561(7722):195–200. doi: 10.1038/s41586-018-0482-7
33. Wang L, Huang B, Chen X, Su J. New Insight Into Unexpected Bone Formation by Denosumab. Drug Discovery Today (2020) 21(11). doi: 10.1016/j.drudis.2020.09.001
34. Kendler DL, Marin F, Zerbini CAF, Russo LA, Greenspan SL, Zikan V, et al. Effects of Teriparatide and Risedronate on New Fractures in Post-Menopausal Women With Severe Osteoporosis (VERO): A Multicentre, Double-Blind, Double-Dummy, Randomised Controlled Trial. Lancet (2018) 391(10117):230–40. doi: 10.1016/s0140-6736(17)32137-2
35. Li X, Wang L, Huang B, Gu Y, Luo Y, Zhi X, et al. Targeting Actin-Bundling Protein L-Plastin as an Anabolic Therapy for Bone Loss. Sci Adv (2020) 6(47):eabb7135. doi: 10.1126/sciadv.abb7135
36. Sun P, He L, Jia K, Yue Z, Li S, Jin Y, et al. Regulation of Body Length and Bone Mass by Gpr126/Adgrg6. Sci Adv (2020) 6(12):eaaz0368. doi: 10.1126/sciadv.aaz0368
37. Fan Y, Hanai JI, Le PT, Bi R, Maridas D, DeMambro V, et al. Parathyroid Hormone Directs Bone Marrow Mesenchymal Cell Fate. Cell Metab (2017) 25(3):661–72. doi: 10.1016/j.cmet.2017.01.001
38. Wagner M. General Principles for the Clinical Use of the LCP. Injury (2003) 34(Suppl 2):B31–42. doi: 10.1016/j.injury.2003.09.023
39. Nauth A, Miclau T 3rd, Bhandari M, Schemitsch EH. Use of Osteobiologics in the Management of Osteoporotic Fractures. J Orthop Trauma (2011) 25 Suppl 2:S51–55. doi: 10.1097/BOT.0b013e31821b8c52
40. Dasari SP, Kerzner B, Fortier LM, Rea PM, Bodendorfer BM, Chahla J, et al. Improved Outcomes for Proximal Humerus Fracture Open Reduction Internal Fixation Augmented With a Fibular Allograft in Elderly Patients: A Systematic Review and Meta-Analysis. J Shoulder Elbow Surg (2022) 31(4):884–94. doi: 10.1016/j.jse.2021.11.004
41. Bigoni M, Turati M, Arnoldi M, Omeljaniuk RJ, Peri Di Caprio A, Del Bene M, et al. Distal Humeral Septic Non-Union Treated With Debridement and Vascularized Fibular Transfer: Case Report and Review of the Literature. Eur Rev Med Pharmacol Sci (2019) 23(2 Suppl):12–8. doi: 10.26355/eurrev_201904_17469
42. Zhang H, Li X, Su J. Controversies and Countermeasures in Treatment of Osteoporotic Distal Humerus Fracture in the Eldly. Chin J Traumatol (2020) 36(9):5. doi: 10.3760/cma.j.issn.1001-8050.2020.09.002
43. Jones CB. Augmentation of Implant Fixation in Osteoporotic Bone. Curr Osteoporos Rep (2012) 10(4):328–36. doi: 10.1007/s11914-012-0124-1
44. Khouri RK, Koudsi B, Reddi H. Tissue Transformation Into Bone In Vivo. A Potential Practical Application. Jama (1991) 266(14):1953–5. doi: 10.1001/jama.1991.03470140065025
45. Su JC, Liu XW, Yu BQ, Li ZD, Li M, Zhang CC. Shape Memory Ni-Ti Alloy Swan-Like Bone Connector for Treatment of Humeral Shaft Nonunion. Int Orthop (2010) 34(3):369–75. doi: 10.1007/s00264-009-0726-0
46. Cao L, Weng W, Chen X, Zhou Q, Zhang J, Wang L, et al. Clinical Research on Allograft Bone Transplantation in the Treatment of Osteoporotic Proximal Humeral Fracture. Chin J Shoulder Elbow (2018) 6(1):11–8. doi: 10.3877/cma.j.issn.2095-5790.2018.01.003
47. Yu W, Li R, Long J, Chen P, Hou A, Li L, et al. Use of a Three-Dimensional Printed Polylactide-Coglycolide/Tricalcium Phosphate Composite Scaffold Incorporating Magnesium Powder to Enhance Bone Defect Repair in Rabbits. J Orthop Translat (2019) 16:62–70. doi: 10.1016/j.jot.2018.07.007
48. Cui L, Xiang S, Chen D, Fu R, Zhang X, Chen J, et al. A Novel Tissue-Engineered Bone Graft Composed of Silicon-Substituted Calcium Phosphate, Autogenous Fine Particulate Bone Powder and BMSCs Promotes Posterolateral Spinal Fusion in Rabbits. J Orthop Translat (2021) 26:151–61. doi: 10.1016/j.jot.2020.06.003
49. Wan L, Song H, Chen X, Zhang Y, Yue Q, Pan P, et al. A Magnetic-Field Guided Interface Coassembly Approach to Magnetic Mesoporous Silica Nanochains for Osteoclast-Targeted Inhibition and Heterogeneous Nanocatalysis. Adv Mater (2018) 30(25):e1707515. doi: 10.1002/adma.201707515
50. Rong Z, Zhang F, Xiao J, Wang Z, Luo F, Zhang Z, et al. Application of Cement-Injectable Cannulated Pedicle Screw in Treatment of Osteoporotic Thoracolumbar Vertebral Compression Fracture (AO Type A): A Retrospective Study of 28 Cases. World Neurosurg (2018) 120:e247–58. doi: 10.1016/j.wneu.2018.08.045
51. Kim JK, Koh YD, Kook SH. Effect of Calcium Phosphate Bone Cement Augmentation on Volar Plate Fixation of Unstable Distal Radial Fractures in the Elderly. J Bone Joint Surg Am (2011) 93(7):609–14. doi: 10.2106/jbjs.J.00613
52. Yue Q, Li J, Zhang Y, Cheng X, Chen X, Pan P, et al. Plasmolysis-Inspired Nanoengineering of Functional Yolk-Shell Microspheres With Magnetic Core and Mesoporous Silica Shell. J Am Chem Soc (2017) 139(43):15486–93. doi: 10.1021/jacs.7b09055
53. Grant KD, Busse EC, Park DK, Baker KC. Internal Fixation of Osteoporotic Bone. J Am Acad Orthop Surg (2018) 26(5):166–74. doi: 10.5435/jaaos-d-16-00142
54. Kennedy J, Molony D, Burke NG, FitzPatrick D, Mullett H. Effect of Calcium Triphosphate Cement on Proximal Humeral Fracture Osteosynthesis: A Cadaveric Biomechanical Study. J Orthop Surg (Hong Kong) (2013) 21(2):173–7. doi: 10.1177/230949901302100211
55. Katthagen JC, Lutz O, Voigt C, Lill H, Ellwein A. Cement Augmentation of Humeral Head Screws Reduces Early Implant-Related Complications After Locked Plating of Proximal Humeral Fractures. Obere Extrem (2018) 13(2):123–9. doi: 10.1007/s11678-018-0440-x
56. Su J, Zhang C, Yu B, Wang J, Xu S, Ji F, et al. Stress Loaded Selection of Humerus Fracture Fixated With Swan-Like Shape Memory Connector. Chin J Clin Rehabil (2003) 7(026):3558–9. doi: 10.3321/j.issn:1673-8225.2003.26.008
57. Cecchi S, Bennet SJ, Arora M. Bone Morphogenetic Protein-7: Review of Signalling and Efficacy in Fracture Healing. J Orthop Translat (2016) 4:28–34. doi: 10.1016/j.jot.2015.08.001
58. Chen F, Liang Q, Mao L, Yin Y, Zhang L, Li C, et al. Synergy Effects of Asperosaponin VI and Bioactive Factor BMP-2 on Osteogenesis and Anti-Osteoclastogenesis. Bioact Mater (2022) 10:335–44. doi: 10.1016/j.bioactmat.2021.09.001
59. Metavarayuth K, Maturavongsadit P, Chen X, Sitasuwan P, Lu L, Su J, et al. Nanotopographical Cues Mediate Osteogenesis of Stem Cells on Virus Substrates Through BMP-2 Intermediate. Nano Lett (2019) 19(12):8372–80. doi: 10.1021/acs.nanolett.9b02001
60. Cai L, Zhang J, Qian J, Li Q, Li H, Yan Y, et al. The Effects of Surface Bioactivity and Sustained-Release of Genistein From a Mesoporous Magnesium-Calcium-Silicate/PK Composite Stimulating Cell Responses In Vitro, and Promoting Osteogenesis and Enhancing Osseointegration In Vivo. Biomater Sci (2018) 6(4):842–53. doi: 10.1039/c7bm01017f
61. Song H, Li X, Zhao Z, Qian J, Wang Y, Cui J, et al. Reversal of Osteoporotic Activity by Endothelial Cell-Secreted Bone Targeting and Biocompatible Exosomes. Nano Lett (2019) 19(5):3040–8. doi: 10.1021/acs.nanolett.9b00287
62. Gibbs JC, MacIntyre NJ, Ponzano M, Templeton JA, Thabane L, Papaioannou A, et al. Exercise for Improving Outcomes After Osteoporotic Vertebral Fracture. Cochrane Database Syst Rev (2019) 7(7):Cd008618. doi: 10.1002/14651858.CD008618.pub3
63. Pinto D, Alshahrani M, Chapurlat R, Chevalley T, Dennison E, Camargos BM, et al. The Global Approach to Rehabilitation Following an Osteoporotic Fragility Fracture: A Review of the Rehabilitation Working Group of the International Osteoporosis Foundation (IOF) Committee of Scientific Advisors. Osteoporos Int (2022) 33(3):527–40. doi: 10.1007/s00198-021-06240-7
64. Quacinella M, Bernstein E, Mazzone B, Wyatt M, Kuhn KM. Do Spatiotemporal Gait Parameters Improve After Pilon Fracture in Patients Who Use the Intrepid Dynamic Exoskeletal Orthosis? Clin Orthop Relat Res (2019) 477(4):838–47. doi: 10.1097/corr.0000000000000487
65. Black DM, Geiger EJ, Eastell R, Vittinghoff E, Li BH, Ryan DS, et al. Atypical Femur Fracture Risk Versus Fragility Fracture Prevention With Bisphosphonates. N Engl J Med (2020) 383(8):743–53. doi: 10.1056/NEJMoa1916525
66. Hu Y, Li X, Zhi X, Cong W, Huang B, Chen H, et al. RANKL From Bone Marrow Adipose Lineage Cells Promotes Osteoclast Formation and Bone Loss. EMBO Rep (2021) 22(7):e52481. doi: 10.15252/embr.202152481
67. Yu B, Huo L, Liu Y, Deng P, Szymanski J, Li J, et al. PGC-1α Controls Skeletal Stem Cell Fate and Bone-Fat Balance in Osteoporosis and Skeletal Aging by Inducing TAZ. Cell Stem Cell (2018) 23(2):193–209.e195. doi: 10.1016/j.stem.2018.06.009
68. Guo W, Li H, Lou Y, Zhang Y, Wang J, Qian M, et al. Tyloxapol Inhibits RANKL-Stimulated Osteoclastogenesis and Ovariectomized-Induced Bone Loss by Restraining NF-κb and MAPK Activation. J Orthop Translat (2021) 28:148–58. doi: 10.1016/j.jot.2021.01.005
69. Hong G, Chen Z, Han X, Zhou L, Pang F, Wu R, et al. A Novel RANKL-Targeted Flavonoid Glycoside Prevents Osteoporosis Through Inhibiting NFATc1 and Reactive Oxygen Species. Clin Transl Med (2021) 11(5):e392. doi: 10.1002/ctm2.392
70. Ko YJ, Sohn HM, Jang Y, Park M, Kim B, Kim B, et al. A Novel Modified RANKL Variant can Prevent Osteoporosis by Acting as a Vaccine and an Inhibitor. Clin Transl Med (2021) 11(3):e368. doi: 10.1002/ctm2.368
71. Zou Y, Huang B, Cao L, Deng Y, Su J. Tailored Mesoporous Inorganic Biomaterials: Assembly, Functionalization, and Drug Delivery Engineering. Adv Mater (2021) 33(2):e2005215. doi: 10.1002/adma.202005215
72. Hu Y, Li X, Zhang Q, Gu Z, Luo Y, Guo J, et al. Exosome-Guided Bone Targeted Delivery of Antagomir-188 as an Anabolic Therapy for Bone Loss. Bioact Mater (2021) 6(9):2905–13. doi: 10.1016/j.bioactmat.2021.02.014
73. Chen S, Chen X, Geng Z, Su J. The Horizon of Bone Organoid: A Perspective on Construction and Application. Bioact Mater (2022) 18:15–25. doi: 10.1016/j.bioactmat.2022.01.048
74. Ye G, Wang P, Xie Z, Li J, Zheng G, Liu W, et al. IRF2-Mediated Upregulation of lncRNA HHAS1 Facilitates the Osteogenic Differentiation of Bone Marrow-Derived Mesenchymal Stem Cells by Acting as a Competing Endogenous RNA. Clin Transl Med (2021) 11(6):e429. doi: 10.1002/ctm2.429
75. Xie Y, Han N, Li F, Wang L, Liu G, Hu M, et al. Melatonin Enhances Osteoblastogenesis of Senescent Bone Marrow Stromal Cells Through NSD2-Mediated Chromatin Remodelling. Clin Transl Med (2022) 12(2):e746. doi: 10.1002/ctm2.746
76. Jiang Y, Li J, Xue X, Yin Z, Xu K, Su J. Engineered Extracellular Vesicles for Bone Therapy. Nano Today (2022) 44:101487. doi: 10.1016/j.nantod.2022.101487
77. Liu H, Geng Z, Su J, Acids CN. Engineered Mammalian and Bacterial Extracellular Vesicles as Promising Nanocarriers for Targeted Therapy. Extracell Vesicles Circ Nucleic Acids (2022) 3(2):63–86. doi: 10.1016/j.bioactmat.2021.12.006
78. Xue X, Hu Y, Deng Y, Su J. Recent Advances in Design of Functional Biocompatible Hydrogels for Bone Tissue Engineering. Advanced Functional Materials (2021) 31(19):2009432. doi: 10.1002/adfm.202009432
79. Xue X, Hu Y, Wang S, Chen X, Jiang Y, Su J. Fabrication of Physical and Chemical Crosslinked Hydrogels for Bone Tissue Engineering. Bioact Mater (2022) 12:327–39. doi: 10.1016/j.bioactmat.2021.10.029
80. Wang PW, Li YZ, Zhuang HF, Yu HM, Cai SQ, Xu H, et al. Anti-Osteoporosis Medications Associated With Decreased Mortality After Hip Fracture. Orthop Surg (2019) 11(5):777–83. doi: 10.1111/os.12517
81. Tai TW, Li CC, Huang CF, Chan WP, Wu CH. Treatment of Osteoporosis After Hip Fracture is Associated With Lower All-Cause Mortality: A Nationwide Population Study. Bone (2022) 154:116216. doi: 10.1016/j.bone.2021.116216
82. Van Lieshout EM, Alt V. Bone Graft Substitutes and Bone Morphogenetic Proteins for Osteoporotic Fractures: What Is the Evidence? Injury (2016) 47 Suppl 1:S43–46. doi: 10.1016/s0020-1383(16)30011-0
83. Huang K, Wu G, Zou J, Peng S. Combination Therapy With BMP-2 and Psoralen Enhances Fracture Healing in Ovariectomized Mice. Exp Ther Med (2018) 16(3):1655–62. doi: 10.3892/etm.2018.6353
84. Bruder AM, Shields N, Dodd KJ, Taylor NF. Prescribed Exercise Programs may Not be Effective in Reducing Impairments and Improving Activity During Upper Limb Fracture Rehabilitation: A Systematic Review. J Physiother (2017) 63(4):205–20. doi: 10.1016/j.jphys.2017.08.009
85. Youth C, Orthopedic E, Multitrauma O, Committee S. Expert Consensus on Bone Repair Strategies for Osteoporotic Fractures in China. Chin J Traumatol (2019) 35(9):769–75. doi: 10.3760/cma.j.issn.1001-8050.2019.09.001
86. Youth C, Multitrauma O, Osteoporosis C. Chinese Expert Consensus on Perioperative Management of Osteoporotic Fracture. Chin J Clin Med (2018) 25(5):860–6. doi: 10.12025/j.issn.1008-6358.2018.20181053
87. Cao L, Niu F, Zhang W, Yang Q, Chen S, Yang G, et al. Expert Consensus on Rehabilitation Strategies for Traumatic Spinal Cord Injury. Chin J Traumatol (2020) 36(5):8. doi: 10.3760/cma.j.issn.1001-8050.2020.05.001
Keywords: Three in one, osteoporotic fractures, repair, treatment, strategy
Citation: Chen X, Hu Y, Geng Z and Su J (2022) The “Three in One” Bone Repair Strategy for Osteoporotic Fractures. Front. Endocrinol. 13:910602. doi: 10.3389/fendo.2022.910602
Received: 01 April 2022; Accepted: 09 May 2022;
Published: 09 June 2022.
Edited by:
Zhiyong Hou, Third Hospital of Hebei Medical University, ChinaReviewed by:
Yong-Can Huang, Peking University, ChinaCopyright © 2022 Chen, Hu, Geng and Su. This is an open-access article distributed under the terms of the Creative Commons Attribution License (CC BY). The use, distribution or reproduction in other forums is permitted, provided the original author(s) and the copyright owner(s) are credited and that the original publication in this journal is cited, in accordance with accepted academic practice. No use, distribution or reproduction is permitted which does not comply with these terms.
*Correspondence: Jiacan Su, ZHJzdWppYWNhbkAxNjMuY29t
Disclaimer: All claims expressed in this article are solely those of the authors and do not necessarily represent those of their affiliated organizations, or those of the publisher, the editors and the reviewers. Any product that may be evaluated in this article or claim that may be made by its manufacturer is not guaranteed or endorsed by the publisher.
Research integrity at Frontiers
Learn more about the work of our research integrity team to safeguard the quality of each article we publish.