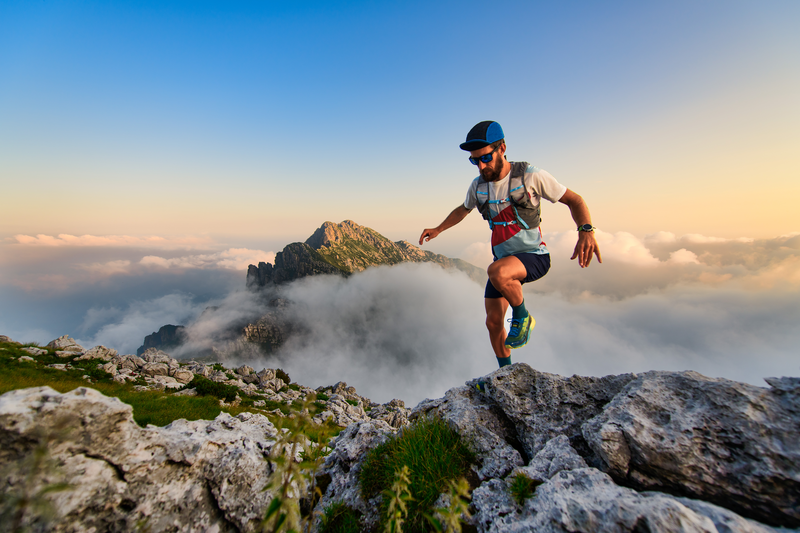
95% of researchers rate our articles as excellent or good
Learn more about the work of our research integrity team to safeguard the quality of each article we publish.
Find out more
ORIGINAL RESEARCH article
Front. Educ. , 19 March 2025
Sec. STEM Education
Volume 10 - 2025 | https://doi.org/10.3389/feduc.2025.1537040
This article is part of the Research Topic Reshaping STEM Education: Strategies for Curriculum Decolonization and Institutional Transformation View all 17 articles
Students’ interest in studying STEM disciplines in Higher Education has decreased over the past decades, especially among women, who have traditionally gravitated toward careers related to social sciences, education, or health—sectors more commonly associated with caregiving. To counteract this trend and prepare students for a society where STEM skills are increasingly valued and in demand, many countries have implemented educational policies that promote these skills through the development of computational thinking. Spain has joined these efforts, and the recent LOMLOE educational law includes computational thinking as a key competence to be developed, encouraging and promoting projects. However, these initiatives do not follow a unified structure but are instead adapted to the technologies and projects most accessible to students and the capacities of each educational center. This study aims to highlight the gender gap in interest toward STEM careers based on a sample of students from the Canary Islands, analyzing the factors contributing to this disparity. Additionally, it explores the role of computational thinking projects in secondary education, evaluating whether they might influence students’ interests and, consequently, help reduce the gender gap in STEM vocations and increase students’ interest in pursuing STEM Higher Education studies.
The integration of women under conditions of equality is a priority both in Spain and across the European Union. This commitment is reflected in multiple strategies and regulations aimed at reducing gender gaps in various areas, especially in education, employment, and political participation. In this regard, the 2030 Agenda for Sustainable Development adopted by the European Union establishes gender equality (Sustainable Development Goal 5) as one of its strategic objectives, recognizing the need to empower all women and girls as a prerequisite for sustainable and inclusive development (European Union, 2019).
In Spain, the commitment to gender equality is also evident in the implementation of specific laws, such as Organic Law 3/2007, of March 22, for the effective equality of women and men, which establishes concrete measures to guarantee equality in the workplace, education, and society. At the European level, the Council of Europe’s 2024–2029 Gender Equality Strategy (Gender Equality Commission (GEC), 2024) has been launched to continue promoting equality policies and to ensure the effective implementation of women’s rights in all member states, with special emphasis on eliminating gender stereotypes and combating violence against women.
Despite multiple efforts to close the gender gap in education and professional fields, significant differences persist in the choice of studies and career paths between men and women. Women continue to be predominantly represented in caregiving-related areas, such as education and health sciences, while men dominate technical and scientific careers. This phenomenon is partly explained by the ongoing influence of gender stereotypes and the differentiated guidance received from an early age. According to a report by the European Commission: Directorate-General for Justice and Consumers (2024), horizontal segregation in education and the labor market remains one of the key barriers to achieving gender equality in Europe, highlighting that women are addressed to traditional feminized professions due to deeply rooted social and cultural expectations (European Commission: Directorate-General for Justice and Consumers, 2024). Similarly, UNESCO’s (2017) report Cracking the Code: Girls’ and Women’s Education in Science, Technology, Engineering, and Mathematics (STEM) emphasizes that, globally, gender stereotypes continue to shape perceptions of ability and competence in STEM disciplines, affecting women’s self-confidence in pursuing technical careers (UNESCO, 2017).
In the educational field, the gender gap in STEM disciplines (Science, Technology, Engineering, and Mathematics) is evident and begins to manifest in the early stages of education, increasing as students’ progress through their academic cycle and significantly affecting the choice of technological careers in higher education (Cobreros et al., 2024). In Spain, the TIMSS 2019 report shows that interest in technology- and science-related subjects is significantly lower among girls compared to boys in primary education (Ministerio de Educación, Formación Profesional y Deportes, 2020). These findings are consistent with the results of the PISA 2022 report, which reveals that girls, despite performing well, show less inclination toward technological and scientific careers compared to their male peers (Ministerio de Educación, Formación Profesional y Deportes, 2023).
This gender disparity in STEM is not unique to Spain; international reports like TIMSS and PISA highlight similar patterns worldwide, pointing to deep-rooted social and cultural factors that shape women’s academic and career choices in these fields (Mullis et al., 2020; OECD, 2023).
However, declining interest in STEM careers is a broader issue, affecting students of all genders. In recent years, enrolment in STEM programs has decreased globally, despite the rising demand for professionals in sectors such as engineering, telecommunications, and computer science (Choi, 2021). This trend has raised alarm in many countries due to its potential implications for technological innovation and economic competitiveness on a global scale (European Commission. Directorate General for Employment, Social Affairs and Inclusion, 2020; OECD, 2020). According to OECD (2020), key factors contributing to this decline include the perception of STEM disciplines as overly challenging and a general lack of interest, particularly among women and underrepresented minorities, which exacerbates existing disparities.
As technology continues to evolve rapidly, the European Commission stresses the urgency of addressing this skills gap to ensure economic growth and stability. Its European Skills Agenda underscores the importance of fostering inclusive and accessible STEM education to cultivate a diverse and skilled workforce capable of meeting labor market demands (European Commission. Directorate General for Employment, Social Affairs and Inclusion, 2020).
In Spain, the decline in student enrolment in STEM disciplines, particularly in Engineering and Architecture, is noticeable and documented in the annual report Datos y Cifras del Sistema Universitario Español by the Ministry of Universities, as illustrated in Figure 1. In the 2011–2012 academic year, the percentage of students in these fields was 22.8%, whereas in the 2021–2022 academic year, this figure decreased by 5.1 percentage points, falling to only 17.7% of total enrolments. This change reflects a downward trend in the choice of these areas, despite the growing demand for professionals in technical and technological sectors (Ministerio de Universidades, 2023).
Figure 1. Evolution in the distribution of students in the Spanish universities. Obtained from Ministerio de Universidades (2023).
To encourage student’s participation and interest in STEM Higher Education, particularly among women, with the aim of also reducing the gender gap, emphasis is placed on developing computational thinking starting in schools.
International concern about the integration of computational thinking into educational systems has led to significant changes in the educational legislation of many European countries, promoting its inclusion in curricula in various ways (European Commission. Joint Research Centre, 2022). In Spain, the Ley Orgánica de Modificación de la LOE (LOMLOE) reinforces this need across several educational stages. Specifically, the development of computational thinking is mentioned 56 times in the different Royal Decrees on minimum education standards, highlighting its incorporation in early childhood education (Real Decreto 95/2022, of February 1, 2022), primary education (Real Decreto 157/2022, of March 1, 2022), and secondary education (Real Decreto 217/2022, of March 29, 2022), with greater emphasis on the latter level, where the concept is mentioned up to 28 times (González Gallego et al., 2022).
The implementation of computational thinking through educational projects is an ongoing process in Spain, driven partly by the provisions of the LOMLOE and partly by the competencies students must acquire to succeed in an increasingly technological labor market. In recent years, numerous case studies have highlighted the benefits and challenges of programming, educational robotics, and, in general, computational thinking in the school context.
Since there is no specific methodology for implementing computational thinking projects in educational institutions, each school adapts activities it considers useful, such as programming, robotics, 3D printing, and electronics. These activities aim to develop logical and computational thinking skills and are typically structured around practical and experimental approaches.
This study aims to examine whether the implementation of computational thinking in secondary education classrooms can influence students’ interest in technology, STEM subjects, and future STEM Higher Education. Furthermore, it seeks to address whether significant gender differences exist in this context and whether certain projects can help reduce the gender gap. To achieve this, various factors identified in the existing literature and data from Hernández-Pérez et al. (2024) have been analyzed to understand the potential causes of this gap.
To this end, an initial state-of-the-art review was conducted to assess the current status of the issue. This is followed by a detailed explanation of the methodology employed in the research, the presentation of the results obtained, and finally, a discussion that addresses the conclusions drawn, the study’s limitations, and potential directions for future work.
This study requires a detailed analysis of the state of the art in two distinct aspects that will be integrated into the final results. First, it is important to understand how Spain, like other countries, is strengthening its policies to implement computational thinking development strategies. The goal is to prepare students and equip them with the necessary skills to meet the demands of an increasingly technological society. Additionally, these policies aim to encourage participation in STEM studies, promoting key skills in science, technology, engineering, and mathematics that are essential for future professional and social contexts.
On the other hand, the existence of the gender gap in STEM studies remains significant despite efforts to reduce it. As highlighted in UNESCO’s Technology on Her Terms report (2024), “Countries are adopting various approaches to address gender divides. However, while 68% of countries globally have policies to support STEM education, only half of these policies specifically support girls and women.” Even more revealing is one of the main headlines of the report: “The share of women among STEM graduates is 35% and has not changed in the past 10 years,” underscoring the persistence of structural barriers to gender equality in this field (GEM Report UNESCO, 2024).
As previously mentioned, the number of Spanish students enrolled in STEM degrees has decreased in recent years. This apparent lack of interest in these careers is partly related to their perceived difficulty and students’ confidence in their abilities, a factor that is particularly influential among girls (Palmer et al., 2017; Vennix et al., 2018).
According to data from Eurostat (the Statistical Office of the European Union), in 2021 and previous years, although the proportion of graduates in STEM fields has grown in absolute terms, students’ relative interest in these disciplines has declined compared to other fields. The number of graduates in science, mathematics, computing, engineering, and construction is approximately 21.9 per 1,000 individuals aged 20–29 in the EU, and a significant gender gap persists in most European countries (Eurostat, 2023).
In Spain, the percentage of students choosing degrees in engineering and computer science has remained practically stable over the past 9 years, with a slight increase in the last 2 years driven mainly by the rise in female enrolments. However, the total variation barely reaches one percentage point (0.67 points). This trend from 2015 to the present year can be seen in Graph (A) of Figure 2.
Figure 2. Distribution of students in technical degrees. Data obtained from Sistema Integrado de Información Universitaria (Ministerio de Ciencia, Innovación y Universidades, 2024). (A) Number of university students enrolled in engineering and computer science in Spain by gender (2015–2024). (B) Percentage of university students enrolled in engineering and computer science in Spain.
When breaking down the data between computer science and engineering, a significant decrease is observed in the number of enrolments in engineering, alongside an increase in computer science. This shift has contributed to the slight overall growth in the STEM area. In Graph (B) of the same figure, these percentages are represented, disaggregated by gender.
These graphs show that engineering and computer science degrees remain highly male-dominated fields, reflecting the persistence of the gender gap in these disciplines.
In the 2022–2023 academic year, the male-to-female ratio in different fields of study is presented in Figure 3.
Figure 3. Proportion of students disaggregated by gender in different university fields. Adapted from the report Datos y Cifras del Sistema Universitario Español 2022–2023 (Ministerio de Universidades, 2023).
According to the same data source, a clear decline is observed in the number of enrolments in STEM fields, specifically in engineering and computer science, in the Canary Islands. This decline has been gradual and continuous over the past nine academic years, reaching a difference of 2.29 percentage points (from 12.44 to 10.15%). This variation has been less pronounced among women, whose enrolment has remained between 2 and 3%, with a slight increase during the last four academic years. This trend is shown in Figure 4, which displays the total percentages and those disaggregated by gender.
Figure 4. Evolution of the percentage of university students enrolled in technological degrees. Data obtained from the Sistema Integrado de Información Universitaria (Ministerio de Ciencia, Innovación y Universidades, 2024).
When analyzing the distribution of enrollments in the Canary Islands in detail during 2023, according to data from the Ministry of Education, Vocational Training, Physical Activity, and Sports of the Government of the Canary Islands, 10.28% of all university students chose a degree related to technology. This calculation has been limited exclusively to studies in engineering and computer science. Other disciplines, such as physics, chemistry, and mathematics, have been classified within the basic sciences group and, therefore, excluded from the technology group, although they are part of the STEM areas. Similarly, architecture, which is often grouped with engineering in many statistics, has been separated in this analysis to more precisely identify specific technological profiles within STEM (Gobierno de Canarias, 2024). Figure 5 illustrates this distribution among university students in the Canary Islands.
Figure 5. Distribution of university studies in the Canary Islands in 2023. Data obtained from the Sistema del Sistema Integrado de Información Universitaria (Ministerio de Ciencia, Innovación y Universidades, 2024).
When analyzing the distribution percentages across different degrees and fields of study, with a final focus on STEM areas and a breakdown of data by gender, a clear difference is observed in the percentage of students choosing a STEM degree oriented toward technology (computer science and engineering) between boys and girls. This difference reaches a noticeable margin of percentage points (19.92% for boys compared to 3.67% for girls), highlighting a significant gap in the choice of these technological disciplines, as shown in Figure 6.
Figure 6. Distribution of university studies in the Canary Islands in 2023 disaggregated by gender. Data obtained from the Consejería de Educación, Formación Profesional, Actividad Física y Deportes del Gobierno de Canarias (Gobierno de Canarias, 2024). (A) Distribution by fields of study by gender. (B) Distribution in science and technology by gender. (C) Distribution in STEM by gender.
The results we have seen stem from the interests and vocations sparked during primary and compulsory secondary education, as well as in high school (baccalaureate). Therefore, it is important to analyze what happens at these levels, since influencing the results at higher levels requires understanding the causes from earlier stages.
According to the Spanish report on the PISA 2022 tests, Spanish 15-year-old students scored below the OECD average in Mathematics (473 points compared to 472). In Science, Spain was at the OECD average with 485 points. The results show a decline compared to previous assessments, partly attributed to the impact of the pandemic on learning. Additionally, gender gaps persist in Mathematics, with boys outperforming girls (Ministerio de Educación, Formación Profesional y Deportes, 2023).
A similar outcome is found in the TIMSS 2019 report, which evaluates fourth- and eighth-grade students in Mathematics and Science. Spain ranked below average in Science and Mathematics compared to other European countries. The TIMSS results highlight the need to strengthen Science and Mathematics learning from early levels, as Spanish students showed stagnation and challenges in scientific competence.
When analyzing the data of high school students (baccalaureate) in the Canary Islands according to different modalities, the distribution for the 2023–2024 academic year, shown in Figure 7, reflects a similar proportion between the Science and Technology track and the Humanities and Social Sciences track. However, there is consistently a higher proportion of boys in the former and girls in the latter.
Figure 7. Distribution of university studies in the Canary Islands in 2023 disaggregated by gender. Data obtained from the Consejería de Educación, Formación Profesional, Actividad Física y Deportes del Gobierno de Canarias (Gobierno de Canarias, 2024).
These data are not particularly significant, as the Science and Technology track is the common pathway chosen to access a wide variety of scientific disciplines. This track encompasses not only engineering and computer science but also health sciences, architecture, basic sciences, and natural sciences, among others.
To foster STEM competencies and promote interest in tertiary studies, computational thinking has emerged as a key tool. Various studies and organizations have highlighted that incorporating programming and robotics into primary and secondary education effectively strengthens problem-solving and critical thinking, which are essential for STEM (Grover and Pea, 2013; OECD, 2023; Bilbao et al., 2024). These practices can also help reduce gender barriers by offering engaging experiences that boost confidence, particularly among girls, who have historically been underrepresented in STEM careers (GEM Report UNESCO, 2024). UNESCO has noted that such initiatives also respond to the need to develop essential digital competencies in today’s society, aligning with the goals of education for sustainable development (UNESCO, 2021).
Computational thinking is a problem-solving process that includes decomposition, abstraction, pattern recognition, and algorithm design. Wing (2006), who popularized the concept, describes computational thinking as “a fundamental skill for everyone,” involving computer science concepts to systematically solve problems.
Selby and Woollard (2013) expand on this definition, stating that computational thinking enables students to “understand complex problems and formulate solutions that can be executed by a machine,” an approach that is valuable in both computer science and other academic disciplines. Complementarily, Huang and Looi (2021) describe computational thinking as a set of widely applicable problem-solving skills, including abstraction, decomposition, pattern recognition, and algorithmic thinking, among others.
The implementation of computational thinking in the educational field offers multiple benefits that contribute to the holistic development of students. Grover and Pea (2013) emphasize that introducing computational thinking in primary and secondary levels not only fosters problem-solving and logical thinking skills but also establishes a solid foundation for tackling complex challenges in a structured manner. By addressing problems through decomposition, pattern recognition, and algorithm design, students acquire skills that can be applied in various disciplines. Meanwhile, Shute et al. (2017) stress that computational thinking is a transferable skill that allows students to tackle problems in diverse contexts using algorithmic methods. This interdisciplinary characteristic not only broadens their competencies in computer science but also strengthens their analytical abilities and adaptability to various fields, making computational thinking an essential tool in today’s education.
In UNESCO’s Technology on Her Terms report, the importance of computational thinking is emphasized as a key tool to improve students’ STEM competencies. The document recommends using educational projects that integrate computational thinking through practical approaches such as programming, robotics, and solving complex problems. These methodologies foster interest and participation in technological and scientific areas, particularly among girls and young women (GEM Report UNESCO, 2024).
The report highlights that the integration of these projects not only enhances students’ technical skills but also promotes essential 21st-century competencies, such as logical reasoning and adaptability, skills increasingly valued in a constantly changing labor environment.
The Reviewing Computational Thinking in Compulsory Education report by the European Commission examines the implementation of computational thinking in compulsory education across 29 European countries, 22 of which are EU member states. The study reveals that, in most of these countries, computational thinking has been integrated into both primary and secondary education, aiming to improve key skills such as problem-solving, coding and programming, and logical thinking through projects that foster creativity and collaboration. Additionally, in 15 of the analyzed countries, one of the main objectives is to promote students’ interest in STEM studies and related careers while in 18 countries, another goal is to enhance employability in the digital sector. Spain is included in both groups (European Commission. Joint Research Centre, 2022).
Computational thinking projects can be implemented in various ways. One option is to address it as a cross-curricular theme, where basic concepts are taught across all subjects, with all teachers sharing the responsibility of developing computational thinking skills in students. Another alternative is to include computational thinking as an independent subject, where projects are worked on in a specific course, usually related to computer science or technology. Finally, projects can be integrated into other subjects, such as Mathematics and Technology, leveraging their interrelation within the curriculum.
The results of this same report, focusing specifically on computer science education, show a varied distribution of project typologies by country. Some countries have opted for a cross-curricular approach, introducing computer science concepts in multiple subjects; others have implemented computational thinking as an independent subject, while some have integrated it into specific subjects. This diversity of approaches reflects the different priorities and capacities of national education systems concerning the teaching of digital competencies and the promotion of STEM skills from early ages (European Commission. Joint Research Centre, 2022).
In Spain, the application of computational thinking still depends on individual schools and autonomous communities (European Commission. Joint Research Centre, 2022). Although the LOMLOE has addressed this field by including computational thinking in its curriculum framework, there are still no clear standards or standardized protocols for its implementation. Moreover, in education, the competencies to complete curricula and educational content based on the minimum requirements established by the State are transferred to the autonomous communities so we can find several differences between schools.
With this new educational law, approved in 2020, computational thinking has been formally integrated into Spain’s educational curriculum, marking a significant change since its inclusion previously depended on the policies of each autonomous community. The Royal Decrees on minimum standards for Early Childhood Education (RD 95/2022), Primary Education (RD 157/2022), and Secondary Education (RD 217/2022) have given computational thinking a central role, in line with European guidelines.
In Secondary Education, RD 217/2022 highlights computational thinking in several key subjects:
1. Biology and Geology: It is proposed as a specific competence for solving problems and explaining processes through logical reasoning and computational thinking.
2. Technology and Digitalization: As a mandatory subject in the first cycle of secondary education, it integrates computational thinking as one of its five pillars, along with problem-solving based on projects and the use of digital technologies. This subject includes a dedicated module titled “Computational Thinking, Programming, and Robotics” and develops competencies in creating algorithms and automating processes.
3. Technology: This subject continues the development of computational thinking from the first cycle, addressing the automation and connectivity of devices. It focuses on “Computational Thinking, Automation, and Robotics,” covering the design and programming of control systems.
4. Mathematics: This subject incorporates computational thinking transversally, including competencies in problem decomposition, algorithmic thinking, and solving everyday problems using algorithms and programs, both in the first cycle and in the fourth-year options, Mathematics A and B.
The LOMLOE introduces computational thinking as a fundamental skill to foster problem-solving and critical thinking abilities essential for today’s society. However, despite its inclusion in the curriculum, the lack of unified criteria or methodologies for implementation leaves schools with the responsibility of integrating computational thinking into their teaching plans, leading to uneven and diverse approaches nationwide. Each institution adapts its projects based on students’ abilities and available resources, including teacher training and materials (Molina-Ayuso et al., 2022).
Schools direct their efforts toward diverse projects, including commercial or custom-built educational robotics, electronics using platforms such as Arduino, visual and textual programming (like Scratch or Python), 3D printing, and “unplugged” or “offline” programming methodologies.
Schools have implemented various initiatives, such as educational robotics (commercial and custom-built), visual and textual programming (e.g., Scratch, Python), electronics platforms like Arduino, 3D printing, and “unplugged” programming methods. For instance, projects like Scratch and robotics have been established as effective tools for developing computational thinking by enhancing problem-solving skills through building physical and virtual models (Valls Pou et al., 2022). The “Creando Robots” initiative further demonstrates the potential of custom-built robotics and programming in developing key competencies aligned with LOMLOE objectives (González Gallego et al., 2021).
Other studies integrate computational thinking into technology content in secondary education, highlighting tools like Scratch combined with MaKey MaKey as effective for fostering interactive and practical knowledge (Connolly et al., 2021). Further studies, such as that of González Gallego et al. (2022), document the planning and implementation of computational thinking projects based on educational robotics and how students positively perceive these activities.
In the European context, a study in Flanders highlights the positive impact of computational thinking on secondary education in terms of students’ digital skills and media literacy. In this case, computational thinking is integrated interdisciplinarily, linking it to socially relevant problems that enhance motivation and learning in skills such as decomposition, pattern recognition, and algorithmic thinking (Gesquiere and Wyffels, 2022). The study suggests that, for this approach to be effective, it is crucial to provide adequate support to teachers and adapt educational materials to students’ needs.
Overall, integrating computational thinking into the curriculum demonstrates clear benefits for both academic and practical competencies. Students develop critical and logical thinking, perseverance, and problem-solving skills essential for addressing complex challenges in the 21st century (Wing, 2006; Grover and Pea, 2013). This approach allows students to break down problems, recognize patterns, abstract information, and formulate algorithms, all of which contribute to a deeper understanding of complex problems and enhance their ability to address them in a structured and efficient manner. These projects help transition students from passive technology consumers to active creators, fostering confidence, creativity, and skills vital for STEM fields and professional contexts (National Academies of Sciences, Engineering, and Medicine, 2021).
A critical factor for the success of these initiatives is teacher training. UNESCO and European Union reports emphasize the urgent need to equip educators with digital competencies, as inadequate preparation can limit the impact of these programs (UNESCO, 2021; European Commission. Joint Research Centre, 2022). In the European context, where policies for educational digitalization are being implemented, teacher training emerges as a key element to ensure that students acquire the skills necessary to face the challenges of a digital society. The PISA 2022 report stresses that countries with robust continuous training programs for teachers in digital and computational domains tend to show better results in the effective integration of these competencies into the school curriculum. This, in turn, helps close skills gaps and improves academic performance in Science, Mathematics, and Technology (OECD, 2023).
In this regard, the book Cultivating Interest and Competencies in Computing: Authentic Experiences and Design Factors (2021) and studies such as that by Molina-Ayuso et al. (2022) emphasize that, to implement successful computational thinking projects, it is essential that teachers not only master technological tools but also have specific pedagogical strategies to motivate and guide students in this field. The experience of using Scratch in teacher training in Spain has demonstrated that initial training in computational thinking can transform teachers’ approaches toward more inclusive and effective teaching, especially benefiting students who traditionally show less interest in STEM areas (Molina-Ayuso et al., 2022). Similarly, the TIMSS 2019 report suggests that the development of these teaching competencies can have a multiplier effect on student performance and motivation, especially when teachers integrate computational thinking into various subjects, promoting interdisciplinary and contextualized learning (Mullis et al., 2020).
The gender gap in STEM remains a major challenge in education and professional fields. UNESCO reports that only 35% of STEM students are women, a figure unchanged over the past decade despite equality-focused policies (GEM Report UNESCO, 2024). This reflects not only structural inequalities but also cultural and social barriers limiting female participation in these disciplines.
Various studies have identified multiple factors contributing to this gender gap. First, self-perception of academic ability plays a crucial role. Hernández-Pérez et al. (2024) point out that young women tend to underestimate their competence in areas such as Mathematics and Engineering, which limits their aspirations in these disciplines. This effect is amplified by gender stereotypes that reinforce the notion that STEM fields are more closely associated with masculine skills and roles (Palmer et al., 2017; Vennix et al., 2018). Furthermore, the ESADE report (2024) highlights that women face multiple barriers, from gender stereotypes to the lack of female role models, which negatively impact their motivation to choose STEM careers. According to this report, the gap in women’s participation in STEM persists not only in education but also in the labor market, limiting diversity and innovation in technologically advanced sectors. It also underscores how the lack of female role models in science and technology perpetuates negative perceptions, creating a vicious cycle where girls and women have fewer examples on which to base their professional aspirations (Cobreros et al., 2024).
Another important factor is the influence of family, school, and social environments. According to Canclini Masserini and Fernández-Darraz (2024), families and educational contexts significantly influence the career choices of young women, who often face pressure to opt for professional paths traditionally associated with female roles (Canclini Masserini and Fernández-Darraz, 2024). This phenomenon is further exacerbated by contemporary social and digital dynamics. The Technology on Her Terms report (2024) examines how social media algorithms amplify gender stereotypes, discouraging girls from taking an interest in technological fields. This has a direct impact on their interest and motivation toward STEM and reinforces cultural norms that limit their participation (GEM Report UNESCO, 2024).
According to this, the relevance of science education has been widely studied in international projects such as RoSE (Sjøberg and Jensen, 2010), which aim to analyze students’ attitudes toward STEM across different countries. This project is expected to provide valuable insights into how gender differences in STEM interest are influenced by cultural and societal factors, highlighting the importance of addressing science identity in educational research.
Additionally, the perception of the difficulty of STEM disciplines is a significant obstacle. As noted in the project “Acércate a la Ingeniería” (2024), when faced with activities related to engineering, students often perceive these areas as too complex, leading to demotivation and a preference for disciplines they consider more accessible (Narganes-Pineda et al., 2024). This finding is consistent with observations by Palmer et al. (2017), who emphasize that the perception of difficulty, combined with a lack of inclusive teaching strategies, discourages women from choosing technological careers (Palmer et al., 2017).
However, not all factors are negative. Some educational initiatives have shown promising results in addressing these issues. Example, for computational thinking projects that promote practical and collaborative activities have proven to be useful tools in reducing the gender gap in certain contexts. According to Molina-Ayuso et al. (2022), the introduction of computational thinking through tools like Scratch has improved female students’ self-perception in STEM areas, fostering active and inclusive learning. This approach not only increases girls’ confidence in their technological skills but also provides them with a positive experience that motivates them to explore these disciplines. These practical activities, being accessible and rewarding, help counteract stereotypes and perceptions of difficulty that traditionally discourage young women from pursuing STEM careers.
In conclusion, the gender gap in STEM has deep and multifaceted roots. Among the main causes are negative self-perception, gender stereotypes, the lack of female role models, social and digital dynamics, and the perception of difficulty in these areas. To address this issue, it is essential to implement inclusive educational policies that actively engage girls and women in STEM projects, as well as to design pedagogical interventions that improve their confidence and motivation. As the reviewed studies indicate, initiatives such as computational thinking projects and the introduction of accessible female role models could be key to transforming this reality.
The present study aims to deepen the understanding of the gender gap in the STEM field and analyze whether the implementation of computational thinking projects in secondary education could bring about changes in this situation, especially in fostering interest in pursuing STEM-related studies in Higher Education. For this purpose, it references a recent study titled The Lack of STEM Vocations and Gender Gap in Secondary Education Students by Hernández-Pérez et al. (2024), which shares the same student survey and much of the data obtained from it, including some more recent findings (Hernández-Pérez et al., 2024).
The mentioned study focuses on identifying the factors contributing to the gender gap in STEM by analyzing both external and internal factors of the students themselves. Using surveys, it examines how influences such as family environment, role models, and perceptions of the difficulty of STEM disciplines affect students’ interest, with a particular focus on women. The results of this study highlight the need for positive STEM role models and for schools and families to stimulate and promote activities related to technology to encourage STEM Higher Education studies and reduce the gender gap.
This subsection describes the sample of students who participated in the study:
• Sample Selection: The sample consisted of 879 secondary and high school students (Educación Secundaria Obligatoria - ESO and Baccalaureate) in the Canary Islands, selected from 10 educational centers across three islands: Gran Canaria, Tenerife, and La Palma. This selection included students from metropolitan and rural areas, ensuring a diverse and representative sample of the different educational and demographic realities present in the archipelago.
• Exclusion Criteria: After an initial analysis of the data, all incomplete or inconsistent responses were excluded from the study. These accounted for 4.2% of the total responses collected, resulting in the previously mentioned sample size of 879 students.
• Diversity of Centers: The selected educational institutions included 8 public institutions, 1 private, and 1 semi-private (charter school) institution, in order to reflect the variety of school types in the Canary Islands and cover levels ranging from the second year of ESO to the second year of Baccalaureate.
• Justification for Selection: The sample was specifically chosen to provide a general view of STEM (Science, Technology, Engineering, and Mathematics) interest in the Canary Islands and to evaluate potential gender gaps. This approach ensures the representativeness and validity of the results by capturing both the geographic diversity between capital and non-capital islands and the variety in socioeconomic contexts.
• Reference Population and Sample Size: The reference population consists of 118,992 students within the analyzed grade levels, distributed across 281 educational institutions in the Canary Islands. To ensure the representativeness of the sample in the study, the minimum sample size was calculated using Cochran’s equation adjusted for finite populations, applying a 95% confidence level and a 5% margin of error.
Sample size calculation
• Cochran’s equation for infinite populations,
Where:
• (95% confidence level)
• (assumed for a conservative approach and maximum variability, given the lack of prior population data (Ahmed, 2024))
• (5% margin of error)
Calculating the initial sample size:
• Adjustment for finite populations (Cochran adjusted):
Where:
• (size of the reference population)
• (minimum sample size for infinite populations)
After adjustment, the minimum required sample size is approximately 383 students. Therefore, the sample of 879 students is sufficient to ensure representativeness in the study.
Surveys are a widely recognized method in social and educational studies, as they allow for the collection of standardized data, the comparison of groups, and the analysis of response patterns (Robson, 2011). In educational research, surveys are especially useful for capturing perceptions and attitudes toward topics such as scientific and technological careers (Anderson and Arsenault, 2002).
The survey used in this study was developed by Merayo and Ayuso (2023a) as part of the research project InGenias: Fostering Technological Vocations and Scientific Outreach.
This questionnaire, specifically designed to study secondary students’ perceptions of STEM careers and disciplines, is available at Merayo and Ayuso (2023b). It was constructed based on a systematic literature review of studies on the perception of STEM disciplines among secondary students (Fouad et al., 2010; Dasgupta and Stout, 2014; Henriksen et al., 2015). Additionally, the questionnaire items were validated by three experts in education and gender, allowing adjustments and refinements to ensure coherence and relevance.
This questionnaire has been applied in previous studies, such as Challenges of STEM Vocations in Secondary Education (Ayuso et al., 2022), Analysis of Barriers, Supports and Gender Gap in the Choice of STEM Studies in Secondary Education (Merayo and Ayuso, 2023a), and The Lack of STEM Vocations and Gender Gap in Secondary Education Students (Hernández-Pérez et al., 2024) supporting its suitability and validity in the context of the present study.
The survey consists of 32 questions distributed across five thematic blocks:
• Context: Basic sociodemographic questions about the educational institution, grade level, gender, age, and location.
• Interests, Hobbies, and Personality: Items aimed at exploring students’ personal preferences, such as games and hobbies during childhood.
• Support and Extracurricular STEM Activities: Questions about the influence of parents, guardians, and teachers on participation in STEM activities outside the classroom.
• Perceptions and Opinions about STEM Studies: Items investigating students’ academic preferences and career aspirations, as well as their perceptions of science and technology careers.
• STEM Role Models: Questions designed to evaluate whether students have role models in the STEM field, both in their immediate environment and in the public domain.
The reliability of the questionnaire was evaluated using Cronbach’s alpha, yielding a value of 0.714, which indicates good internal consistency (Cicchetti and Sparrow, 1981; Cortina, 1993).
This brief and structured questionnaire was specifically designed to collect information about the implementation of computational thinking projects in the educational centers that participated in the original study by Hernández-Pérez et al. (2024) as well as those added after that study. The purpose is to understand how these activities are linked to fostering students’ interest in pursuing STEM Higher Education. The questionnaire is available in an open repository for consultation (González-Gallego, 2025).
The questionnaire addresses various aspects related to computational thinking, its presence, and its approach in the centers, and is organized into the following sections:
• General Information about the Center: This section requests the name of the institution, the educational levels it offers (ESO and/or Bachillerato), and the role of the respondent.
• Current Implementation: Centers must indicate whether they are currently conducting any activities or projects related to computational thinking, covering areas such as programming (analog or digital), electronics, or robotics.
• History of Computational Thinking Projects: This section explores the center’s history in implementing these activities, including whether they have never worked in this area, are starting projects in the current academic year, or have been consolidating these activities for several years.
• Implementation Modality: This section investigates how these projects are carried out, with options such as “independent project in a single subject,” “interdisciplinary project across multiple subjects,” or “extracurricular activity.”
• Educational Levels Involved and Types of Activities: Here, centers are asked to specify, over the past three academic years, which levels (from 1st year ESO to 2nd year Bachillerato) have been involved in activities related to programming, robotics (commercial or custom-built), and electronics, among others.
• Future Plans: If the center considers starting or expanding computational thinking projects in the future, they are asked about potential areas of interest, such as unplugged programming, robotics with commercial kits, robotics built with components, and artificial intelligence projects.
• General Observations: A free-response section is provided for the center to share additional comments about its experience, opinions, and expectations regarding the implementation of computational thinking in its educational community.
This questionnaire provides important context regarding students’ exposure to computational thinking, enabling an evaluation of the potential impact of these projects on the development of interest in STEM Higher Education.
The survey was conducted with students from the participating educational centers during the school year, in a controlled environment at each center to ensure uniform conditions. Participation was voluntary and anonymous.
A questionnaire was sent to each participating educational center to gather information about their level of implementation of computational thinking projects, collecting relevant data for comparative analysis.
• Descriptive Analysis: An initial descriptive analysis was conducted to understand the sociodemographic and educational characteristics of the sample, including gender distribution and students’ academic preferences. This analysis included the creation of frequency tables.
• Content Analysis: A content analysis was specifically applied to the responses to question Q19-R, “What would you like to be when you grow up?” to categorize students’ professional aspirations. The responses were classified into fields of study (e.g., sciences, technology, arts, humanities) and distinguished between trades and university careers, allowing the identification of aspirations related to STEM fields. This approach provided a clearer understanding of students’ vocational preferences in relation to scientific and technological disciplines and STEM Higher Education
• Comparative Analysis: Statistical tests were performed to compare groups (e.g., preference for sciences or humanities, vocational interests) in relation to the gender and the years of participation in computational thinking projects.
Shapiro–Wilk tests conducted cannot be considered indicative of normality as the dataset is too small, including only four ranges of data based on courses using computational thinking projects. Relying on visual methods in the analyzed divisions, we can conclude that the data do not follow a normal distribution. If, to increase the dataset size, we consider all observations available based on the courses worked with computational thinking projects, it can be determined that the p-value in all analyses is below 0.05, indicating a non-normal distribution.
The Kruskal-Wallis test reveals p-values <0.05 when comparing STEM vocation interest by gender. However, analyses based on courses worked with computational thinking projects show higher p-values, suggesting no significant differences between groups, likely due to the small sample size.
• Correlational Analysis: Correlation analyzes (Spearman, a non-parametric test for non-continuous variables or those without a normal distribution, as is the case here) were performed to explore possible relationships between quantitative variables, such as the time spent participating in computational thinking projects and academic preferences or the STEM Higher Education interest.
To perform the statistical analysis, the Jamovi software was used (R Core Team, 2021; The jamovi project, 2022).
Previously, the distribution of the student population across different university majors and their interests in compulsory secondary education was presented, as well as the concept of computational thinking and its advantages for developing competencies in STEM fields. It was also shown that a gender gap exists in this area, which appears difficult to overcome, along with the factors that seem to perpetuate it.
Now, using the data collected for this article, this document analyzes not only the existence of this gap but also whether the implementation of computational thinking projects in schools affects students’ interests and contributes to reducing this gender gap.
The initial descriptive analysis of the sample provides an understanding of the demographic and academic characteristics of the 879 participating students. Below is the distribution of gender, age, and grade level, as represented in the charts in Figure 8:
1. Distribution by Gender (Graph A): The sample consists of 454 female students (51.5%), 412 male students (46.8%), and 15 students who identified in another gender category (1.7%). This distribution ensures gender equity, allowing for the exploration of potential differences regarding STEM vocations and perceptions of these fields.
2. Distribution by Age and Gender (Graph B): Most students fall within the 14–17 age range, with 15-year-olds forming the largest group. The gender distribution is balanced across ages, although slight variations are observed in certain age groups, such as among 15-year-olds, where females slightly predominate. This age range aligns with the expected levels for secondary education (ESO) and high school (Baccalaureate) where the study is conducted.
3. Distribution by Grade Level and Gender (Graph C): Students are distributed from the second year of secondary education (ESO) to the second year of Baccalaureate. The number of students decreases in higher grades, with the second year of Baccalaureate having the lowest representation. Across all grades, a balanced gender representation is maintained, although in 2nd and 4th grade of ESO, the number of female students is higher than that of males.
Figure 8. Distribution of students in the sample by gender, age, and grade level. (A) Distribution by gender. (B) Distribution by age and gender. (C) Distribution by grade and gender.
This analysis provides a clear and detailed context of the sample composition, which is essential for correctly interpreting the study results and ensuring that the conclusions reflect the diversity and equity in terms of gender and educational level.
When comparing the distribution of gender and educational level in the sample of surveyed students with the population data for the Canary Islands, a significant similarity is observed in the proportions of gender and educational levels (ESO and Baccalaureate). This suggests that the sample is representative of the student population in the region.
In the total population, as provided by the Government of the Canary Islands, the data show a distribution of 44,514 male students and 41,667 female students in ESO, and 15,399 males and 17,412 females in Baccalaureate (Gobierno de Canarias, 2024). This proportion is consistent with the gender distribution in the sample, which also reflects a balance between students of both genders in ESO and Baccalaureate. Although the sample includes an additional gender category (“other”) that does not appear in the official data, its proportion is low (1.7%) and therefore does not significantly affect the comparability between the two distributions.
This section examines students’ academic interests and the potential existence of a gender gap in the STEM field, based on the sample responses.
Figure 9: Distribution of Students by Their Intentions for Future Studies presents an analysis of students’ educational aspirations at the end of high school. The first part of the figure (charts A) shows the distribution of students according to their intention to continue studying. According to chart A1, 93% of students express a clear interest in pursuing further education, while only 7% indicate that they do not wish to continue studying.
Figure 9. Distribution of students by their intentions for future studies. (A) Distribution of students by whether they want to continue studying after high school. (A1) Total percentages, (A2) by school type, (A3) by gender. (B) Distribution by type of higher education studies chosen. (B1) Total percentages, (B2) by school type, (B3) by gender.
When analyzing this intention by type of school (A2), we find that the desire to continue studying is widespread: all students from subsidized schools surveyed plan to pursue further education, and the figure remains high for private schools (96.30%). The highest number of cases and the largest percentage of students who do not wish to continue studying after high school are found in public schools, where this rate reaches 7.53%, more than double that of private schools.
When disaggregating by gender the intention to continue studying after high school (A3), a significant difference between boys and girls becomes apparent. Among female students, 95.84% intend to continue their education, compared to 89.6% of male students. This indicates that a higher percentage of male students do not wish to pursue further education, at 10.40%, compared to 4.16% of female students. This difference suggests that female students demonstrate greater motivation to continue their education than their male counterparts.
The second part of the figure (charts B) examines the type of higher education studies students choose, distinguishing between university degrees and vocational training programs. Chart B1 shows that a majority of 74.5% prefer pursuing a university degree, while 25.5% opt for vocational training. This preference appears to be directly related to the type of school (B2), as students from subsidized and private schools show a stronger inclination toward university studies (91.18 and 92.31%, respectively). In contrast, in public schools, vocational training is a more frequently considered option, with 27.66% of students choosing this path.
Regarding gender distribution (B3), a significant difference can be observed. Among female students, 78.59% prefer to pursue a university degree, compared to 65.59% of male students. For vocational training programs, 34.41% of male students opt for this modality, compared to 21.41% of female students. This difference suggests that women tend to prefer university education more than men, while a larger proportion of men show interest in vocational training.
In summary, this figure highlights that most students in the sample intend to continue their studies after high school, particularly female students, and that the choice between university and vocational training varies by school type and gender. Female students show a greater preference for university education, while male students exhibit a slightly higher preference for vocational training.
Regarding the academic interests of the surveyed students, Figure 10 presents two charts: one showing the distribution of students’ preference for Humanities or Science Subjects (Chart A), and the other illustrating their interest in pursuing studies related to Science and Technology (Chart B).
Figure 10. Distribution of students by interest in subjects and studies related to science and technology. (A) Distribution by interest in type of subject. (B) Distribution by interest in continuing studies related to science and technology.
Students were asked to indicate whether they preferred subjects related to Science (such as Mathematics, Physics, Technology, and Natural Sciences) or Humanities and Arts (such as History, Art, and Literature). The distribution reveals a general trend toward science subjects, with 53.5% of male students and 50.2% of female students expressing a preference for these areas. This distribution is balanced across genders, although a stronger inclination toward sciences is observed among male students (66.5% compared to 50.2% among females).
In other question, students were asked whether they intended to pursue studies in disciplines related to science and technology. The results show a direct correlation between the preference for science subjects and the desire to study in these fields. Almost all students who prefer STEM subjects (such as Mathematics, Physics, and Technology) express interest in continuing studies related to science and technology, while those who favor Humanities subjects tend to choose non-STEM studies.
In terms of gender, 65.3% of male students and 50.2% of female students express interest in STEM-related careers, while the proportion of students preferring non-STEM fields is notably higher among females.
These results suggest that, while the initial preference for science and humanities subjects is relatively balanced across genders, the inclination toward STEM studies tends to be stronger among male students, already indicating a potential gender gap in scientific and technological vocations.
After conducting a content analysis of the responses to question Q19-R, “What would you like to be when you grow up?,” students’ professional aspirations were categorized into various areas, with the results presented in Figure 11. At the first level, the responses were classified into major categories: Science and Technology (36% of the total), Social Sciences and Humanities (24%), Security (9%), Primary Sector (6%), Technical Trades (4%), Non-technical Trades (3%), Arts and Design (7%), Sports (5%), and Undefined (13%). The latter category includes general or vague responses that do not specify a concrete professional field.
Within the Science and Technology aspirations (36% of the total), a more detailed analysis was conducted to identify specific interests in STEM fields (Science, Technology, Engineering, and Mathematics) and those corresponding to other scientific and technological areas. This sub-classification revealed that 46% of aspirations in science and technology are directed toward STEM areas, while the rest are distributed among Health Sciences (45%), Natural Sciences (7%), and an Undefined category (2%), which includes responses such as “I want to be a scientist” or “work in a laboratory” without specifying a particular discipline.
Within the STEM aspirations group, an additional classification identified interests directly related to technology. Students interested in STEM demonstrated diverse preferences: 33% focused on Engineering, 44% on Computer Science, 10% on Architecture, and 8% on Basic Sciences (such as Mathematics or Physics). Additionally, 5% expressed interest in teaching within STEM fields, specifying roles like “technology teacher” or “math teacher.” These responses were categorized separately to highlight that, although STEM studies are involved, the focus is on education rather than a technical or scientific role.
Overall, this figure provides a detailed overview of students’ professional aspirations, emphasizing that a significant proportion show interest in fields related to science and technology. The content analysis highlights how these aspirations are distributed across different fields and specifically within STEM areas. It also shows that technology, encompassing engineering and computer science, is the preferred choice for 12.9% of the total student sample.
These findings align with official data on Higher Education in the Canary Islands. In both cases, 36% of students choose the Science and Technology branch as their area of academic interest, reflecting a similar trend between the vocational aspirations in our sample and the actual university study choices in the archipelago. Furthermore, the proportion of students interested in STEM Higher Education is also very similar: 48% in the university enrolment data compared to 46% in our study. Finally, the interest in pursuing STEM-related studies, specifically in engineering and computer science, accounts for 12.9% in our sample—a figure that closely aligns with the proportion observed in official enrolment data, which, while slightly lower (10.3%), remains very similar.
It is worth noting that, unlike the analysis of our sample, the ministry’s data does not break down STEM interest by specific orientations, such as teaching, which highlights the specificity and detail of our analysis. This difference may contribute to slight variations in the results.
The results disaggregated by gender, shown in Figure 12, reveal a significant gender gap in students’ high education aspirations. While the proportion of boys and girls interested in the Science and Technology field is similar, the preference for Social Sciences and Humanities is notably higher among girls. This differentiated interest becomes more pronounced when examining the distribution within the Sciences category: while most boys interested in science lean toward STEM areas (with a strong focus on computer science and engineering), girls show a clear inclination toward Health Sciences. The distribution between these two areas is almost inverse, highlighting a trend where girls prefer careers related to care and health, whereas boys gravitate toward technological careers.
Figure 12. Distribution of students by vocational interest by gender. (A) Complete vocations distribution by gender. (B) Sciences vocations distribution by gender. (C) STEM vocations distributions by gender.
This polarization reflects traditional gender patterns in vocational choices that persist among current students.
The last chart in the figure, which shows the distribution of STEM studies by gender, clearly illustrates these differences. In the Computer Science category, boys overwhelmingly dominate, while the interest from girls is minimal. A similar trend is observed in Engineering, where male representation is significantly higher. Conversely, in STEM subfields like Architecture, Basic Sciences, and Teaching, the gender representation is more balanced, although girls tend to show greater interest in teaching within STEM fields. This indicates that while some areas of STEM exhibit more equitable gender representation, technological careers remain a challenge in terms of gender equity.
Overall, 12.9% of the total student sample expresses interest in technological studies, including both engineering and computer science. However, this proportion is heavily skewed by gender: only 3.7% of girls show interest in these areas, compared to 22.9% of boys. This indicates that although there is a relatively balanced interest in the general Science and Technology field between genders, technological disciplines remain predominantly male, evidencing a persistent gender gap in these areas.
In summary, these results suggest that while girls are interested in science, they primarily lean toward Health Sciences careers or STEM teaching roles, whereas boys are more inclined toward technological and engineering disciplines. This distribution highlights the need to promote interest in technology and engineering among female students to reduce the gender gap in these critical fields for scientific and technological development.
The implementation of computational thinking projects in schools shows considerable variability in terms of duration, integration methods, and types of activities developed. This disparity arises from multiple factors, such as resource availability, the commitment level of teaching teams, and the presence of institutional policies that prioritize or promote computational thinking as a key competency in student education.
Analyzing the time schools have spent working on computational thinking projects reveals a diverse landscape. At the time of the survey, three schools indicated they had only conducted occasional, sporadic activities in this area, without a continuous or systematic approach. One school mentioned that it had formally initiated a computational thinking project this year, while the remaining schools surveyed reported having implemented these activities regularly for several years. This range of experiences reflects the different stages of implementation schools are in, likely corresponding to varying levels of familiarity and expertise in teaching computational thinking among educators.
The survey also explored strategies used by schools to integrate computational thinking projects into the curriculum. The data shows that 80% of schools have implemented these activities in the classroom as standalone projects within a specific subject. This approach reflects an initial, focused method where computational thinking is introduced as a distinct unit of content. However, 50% of schools have opted for a more interdisciplinary model, conducting projects that involve multiple subjects, fostering a holistic view of computational thinking by applying it in different contexts. Additionally, 40% of schools use computational thinking as a complementary resource to enhance other school projects, while 20% have extended their offerings to extracurricular activities related to this competency.
Regarding the types of projects undertaken, schools have adopted a variety of approaches. Programming is the most widespread activity, with 90% of schools engaging in projects in this area. Electronics and the use of commercial robots rank second, with 70% participation in each, reflecting an interest in the practical learning of technology and engineering concepts. Robotics built from components has been employed by 50% of schools, offering an alternative that allows students to delve into creating and assembling devices from scratch.
Additionally, 60% of schools work with unplugged or algorithmic programming, enabling the introduction of basic logic and problem-solving concepts without relying on digital devices. One school reported conducting 3D design projects, adding a dimension of creativity and spatial skills development.
It is worth noting that nearly all schools, whether through occasional activities or established projects, have engaged in several types of projects over the past three school years, across different grade levels.
The lack of clear guidelines for integrating computational thinking into the minimum curricula under the LOMLOE framework has led to uneven implementation across courses and grade levels, resulting in significant disparities among schools. Students in the same grade but attending different schools may have vastly different experiences with computational thinking activities due to variations in schools’ curriculum autonomy and resource availability. This inconsistency affects the uniformity of exposure to computational thinking, potentially influencing students’ interest in technology and their skill development.
These disparities also offer an opportunity to analyze how different levels of exposure to computational thinking impact students’ interest in STEM areas. Schools range from those with sporadic activities to those with extensive experience, enabling comparisons that explore how accumulated experience and the integration of diverse projects influence STEM aspirations. Understanding this variability provides key insights into how education fosters students’ interest and engagement in science and technology.
Based on the data provided by the schools, a variable has been included in the dataset indicating the number of years each student has participated in computational thinking activities at their school. This variable also specifies the type of projects undertaken, considering the student’s current grade and the projects conducted during that grade and in previous years of secondary education. This information will allow us to analyze whether certain types of projects are more effective than others in increasing interest in pursuing STEM-related studies.
However, a certain degree of error or uncertainty must be assumed in this association, as factors such as potential school transfers or grade repetition have not been considered, which could affect the calculation of students’ years of exposure to computational thinking.
First, we analyze the distribution of students based on the number of years they participated in computational thinking (CT) projects. It is important to highlight that group 0 includes students from schools which have carried out occasional activities but not to the extent of being considered a project or those who have had no experience at all.
Chart A in Figure 13 shows the distribution of students by the number of years they have participated in computational thinking (CT) projects. The data indicate that 47% of students (416 students) have engaged in CT activities for three full academic years, representing the group with the most experience. In contrast, 26% (226 students) have had sporadic or no participation, engaging only in occasional activities or none at all. Students with 1 year of CT experience constitute 14% (124 students), and those with 2 years of experience represent 13% (113 students).
Figure 13. Distribution of students by the years working on computational thinking (CT) and by types of projects. (A) Distribution of students by years working on computational thinking (CT). (B) Distribution of students by number of courses working on each type of CT activity.
This distribution highlights a significant difference in students’ exposure to computational thinking, with almost half having a consistent three-year experience, while a quarter have had virtually no involvement in these activities.
Graph B provides details about the types of CT projects in which students have participated, broken down by the number of years they have engaged in each specific type of activity. As observed, most students do not have prolonged experience in any area, and the number of students with 3 years of experience is low across all activities. However, programming stands out as the type of project with the highest continuity, with 11.15% of students having 3 years of experience. This suggests that programming is the most accessible or prioritized activity in schools.
Fabricated robotics shows a high percentage of students with only 1 year of experience (40.16%), which may indicate that this activity is exploratory in many schools but rarely sustained over time, or that it has been recently introduced, meaning there are not yet students with more extended exposure. Regarding commercial robotics and electronics, there is a high percentage of students who have never worked on these types of projects. However, proportionally, there are more students who have engaged in these projects for two or 3 years, suggesting that these activities may require greater initial resources or infrastructure, but once implemented, they can be used continuously.
When analyzing students’ interest in different types of subjects and their intention to pursue future studies related to science and technology, this time broken down by the number of years they have worked on computational thinking projects, a clear trend emerges. The interest in STEM subjects tends to increase in relation to this variable. This trend is illustrated in Figure 14.
Figure 14. Distribution of students by interest in subjects and studies related to science and technology, based on years of exposure to CT projects. (A) Interest in subjects based on the number of courses working on computational thinking (CT). (B) Studies related to sciences based on the number of courses working on computational thinking (CT).
Graph A in Figure 14 illustrates the percentage of students interested in humanities subjects, such as Writing, Language, Literature, History, and Art, compared to those interested in scientific and technological subjects, such as Mathematics, Physics, Chemistry, Biology, and Technology. The general trend shows that as exposure to CT projects increases, interest in STEM subjects grows significantly, rising from 51.77% among students with no CT exposure to 62.98% among those with 3 years of experience in these projects. Conversely, interest in humanities subjects decreases from 48.23 to 37.02% as CT exposure increases. This suggests that participation in computational thinking projects may foster greater interest in scientific and technological disciplines, potentially by engaging students in problem-solving and technology use in educational contexts.
Graph B in the same figure examines the impact of years of exposure to CT projects on students’ inclination to consider studies related to science and technology. The trend mirrors that of Graph A: as years of experience in CT projects increase, so does interest in pursuing STEM studies.
The Spearman correlation analysis applied to the data on interest in STEM subjects and the intention to continue studying science reveals rho values that suggest a consistent trend in the observed relationships. The data indicate that a higher number of computational thinking courses may be related to an increase in interest in these disciplines. However, the p-values do not reach statistically significant levels, reflecting the limited influence of the reduced number of degrees of freedom (2 in this case). Although these statistical limitations do not allow for definitive conclusions, the trends visualized in the graphs support the hypothesis that working with computational thinking has a potentially positive impact.
Figure 15 demonstrates how computational thinking projects influence the development of STEM aspirations among students. While these results should be interpreted cautiously due to the small sample size, there is a clear positive trend indicating an increase in interest in STEM-related studies, such as engineering and computer science. The percentages reflect the proportion of students choosing these fields based on their time spent on CT projects, whether in general or on specific types. These findings offer an initial glimpse into the significance of CT projects in fostering STEM aspirations, underscoring the need for further studies with larger, more representative samples.
Figure 15. Evolution of interest in STEM careers based on courses involving computational thinking projects: breakdown by gender and project type. (A) Evolution of interest in STEM studies based on the number of years engaged in general CT projects. (B) Evolution of interest in STEM studies based on the years engaged in general CT projects, disaggregated by gender. (C) Evolution of interest in STEM studies based on the time spent working on each type of CT projects. (D) Interest in STEM studies based on the time spent working on each type of CT projects, disaggregated by gender.
The first graph (A) shows how interest in STEM studies evolves with years of participation in CT projects. Lines represent different STEM areas (e.g., Computer Science and Technology), with the dashed line indicating overall STEM interest. A gradual increase is observed as students gain more experience in CT projects, particularly in Computer Science, which shows consistent growth. Overall STEM interest rises from 8.65% in students with minimal CT exposure to 15.87% in those with 3 years of participation.
The second graph (B) examines this evolution disaggregated by gender, revealing differences in trends. Boys show a more significant increase in STEM interest, particularly after the third year, while girls exhibit a more moderate trend, with a notable rise in Computer Science and Technology. Due to the small sample size, the “other” gender category is excluded from this breakdown but is included in the overall results.
Graphs C and D analyze the types of CT projects that influence STEM interest. Overall, any project positively impacts interest, but the effect varies by activity type. Programming and commercial robotics have the strongest influence, especially for boys, where interest rises by up to 11 percentage points, doubling initial levels. These projects are engaging and offer quick, tangible results, boosting motivation. In contrast, more complex projects, like electronics or fabricated robotics, demand more time and technical understanding, which can reduce their positive impact. However, fabricated robotics has a moderate positive influence on girls, while for boys, it has little effect and may even reduce interest.
Programming consistently increases STEM interest across genders, while electronics strongly influences boys (8.25-percentage-point increase) but decreases interest among girls (−3.29%). This disparity highlights the need to redesign electronics activities to ensure equitable participation. Commercial robotics, however, appeals to both genders, with a 6.55-percentage-point increase for girls and a substantial 23-point rise for boys. Its creative, practical, and visually engaging nature helps students connect abstract concepts with tangible applications, making it a powerful tool for fostering STEM aspirations.
Analyzing the data statistically through the Spearman correlation analysis reveals valuable trends despite certain limitations. The gender-disaggregated data highlight the impact of computational thinking (CT) projects on fostering interest in STEM fields, but the small sample size presents challenges. Specifically, the limited degrees of freedom (2 in this case) mean that the choices or perceptions of a single individual can significantly influence the observed percentages. Nonetheless, the analysis shows strong rho values, in some cases reaching 1, particularly when examining years of engagement in CT projects or specific activities such as commercial robotics, which exhibit the clearest trends across both genders. While the p-values indicate a lack of statistical significance, these results provide essential initial insights for optimizing the design of CT projects to maximize their positive impact on students of all genders and enhance interest in STEM disciplines.
This study reinforces previous research findings on the low interest in STEM Higher Education, aligning with the observations in the Reviewing Computational Thinking in Compulsory Education report (European Commission. Joint Research Centre, 2022), the TIMSS 2019 (Mullis et al., 2020) and the PISA 2022 (OECD, 2023). The general lack of motivation for these disciplines reflects a global trend that requires sustained attention in educational policies.
Furthermore, the study confirms that girls exhibit less interest in technology and STEM-related fields, consistent with findings from GEM Report UNESCO (2024), Hernández-Pérez et al. (2024) and the study Mujeres en STEM: Desde la educación básica hasta la carrera laboral (Cobreros et al., 2024). This gender disparity is evident in vocational aspirations and perceptions of ability, underscoring the persistence of cultural and structural barriers. In our study, gender differences in the perception of STEM skills reveal more negative self-perceptions among female students. This aligns with González Gallego et al. (2022), who found that 29% of female students considered themselves incapable of performing activities related to programming and electronics before participating in specific projects. This highlights the need to design projects that build confidence in female students and provide positive role models in STEM.
On the other hand, this study highlights the positive impact of computational thinking (CT) projects on students’ interest in technology and STEM Higher Education studies, offering new perspectives on these areas. This aligns with researches like Developing and Assessing Computational Thinking in Secondary Education using a TPACK Guided Scratch Visual Execution Environment (Connolly et al., 2021) or Computational Thinking and Educational Robotics Integrated into Project-Based Learning (Valls Pou et al., 2022) and initiatives such as Creando Robots (González Gallego et al., 2021). Moreover, activities that are dynamic and offer quick results, such as programming and commercial robotics, generate better outcomes, particularly in terms of motivation. This finding aligns with the Acércate a la Ingeniería study (Narganes-Pineda et al., 2024), which highlights how perceptions of complexity can negatively impact motivation, especially in more technical projects like fabricated robotics or electronics.
This study demonstrates that CT projects positively influence students’ interest in STEM subjects and STEM Higher Education studies. However, statistically significant relevance cannot be established due to the limited degrees of freedom in the analyses conducted, highlighting the need for larger samples to draw robust conclusions. Programming and commercial robotics emerge as the most influential activities, especially the latter, which generates a positive response across genders. Conversely, activities like electronics or fabricated robotics, although impactful to some extent, show less consistent results and can even be counterproductive, particularly for girls.
A key limitation of this study is the sample size, which restricts the generalizability of the findings. While the observed trends are consistent with official reports, such as those from the Ministry of Education and the Government of the Canary Islands, future research should work with more representative and diverse samples. Additionally, the lack of uniformly applied projects across schools complicates the identification of which activities have the greatest impact on STEM aspirations.
Another significant limitation is the lack of consideration for contextual variables, such as school transfers or students repeating grades, which could introduce uncertainty into the results. Moreover, it is challenging to isolate the effects of each type of project, as many students have worked with multiple technologies. To obtain clearer results, a larger sample would be necessary to analyze each project type individually, ensuring that each project has a significant sample of students.
The results of the study indicate that the implementation of computational thinking projects can positively influence students’ interest in STEM subjects, such as mathematics, physics and technology.
The increase in interest in these subjects during secondary education after participating in computational thinking projects also extends to interest in pursuing higher education studies related to these fields. This suggests that engaging in such projects could potentially increase the number of students interested in pursuing STEM Higher Education studies, thereby better aligning the workforce with the demands of an increasingly technological society.
However, this influence does not appear to differ significantly between boys and girls, highlighting the need for a more targeted approach to address the persistent gender gap in STEM. Projects such as those using commercial robotics generate interest across genders, helping to combat the general lack of motivation toward these disciplines but failing to directly address gender disparities.
The gender gap remains a significant challenge, particularly in the perception of STEM skills. Female students often exhibit lower confidence in their abilities, which can discourage them from pursuing careers in these fields. To tackle this issue, it is crucial to implement targeted interventions that boost girls’ confidence designed to highlight their skills and provide female role models in STEM, such as mentorship programs with female STEM professionals, hands-on activities in computational thinking and robotics, and workshops designed to counteract gender stereotypes. Showcasing women professionals in these disciplines can transform perceptions, inspire young women, and foster a more inclusive environment. Additionally, initiatives should actively promote diversity and gender equality to create a lasting impact.
Furthermore, it is essential that educational projects in computational thinking are accessible and motivating, particularly during the early stages of their implementation. Engaging girls in structured, hands-on STEM activities, such as collaborative robotics projects or coding workshops, can provide positive reinforcement and build self-efficacy in technical skills. Activities incorporating playful elements, such as visual programming or gamified tasks, can spark initial interest and prevent frustration associated with overly complex projects. These initiatives should balance technical rigor with practical and relevant applications to everyday life, fostering a stronger connection between learning and its real-world utility. Likewise, gender-inclusive workshops addressing societal biases in STEM can help dismantle limiting beliefs and encourage equal participation. Designing inclusive activities that combine challenge and motivation can help sustain long-term interest in technological disciplines.
Although computational thinking is included in the LOMLOE as an essential competence, its uneven implementation in schools limits its impact. The lack of clear and consistent guidelines results in disparities that hinder consistent outcomes. To maximize its impact, it is imperative to develop standardized frameworks that ensure the equitable application of these competencies. Additionally, strengthening teacher training is critical, ensuring educators have the knowledge and resources needed to effectively implement computational thinking in the classroom.
Finally, to accurately assess the real impact of computational thinking, it is necessary to develop more structured projects and apply them systematically across different educational contexts. Uniform implementation will allow for the identification of the most effective practices and the development of evidence-based strategies for the future. Investing in longitudinal studies and pilot programs can provide valuable data to build a robust framework that optimizes the integration of computational thinking into education, ensuring that these initiatives are scalable, inclusive, and effective in diverse environments.
In summary, this study highlights the transformative potential of computational thinking in fostering STEM Higher Education studies, while also emphasizing the pressing need for a more structured and equitable approach to ensure its full impact. By prioritizing coordinated implementation and continuous evaluation, educators and policymakers can address current challenges and better prepare students for the demands of an increasingly technological and inclusive society.
The raw data supporting the conclusions of this article will be made available by the authors without undue reservation.
Ethical approval was not required for the studies involving humans because the data are anonymous and pose no risk to the participants. The studies were conducted in accordance with the local legislation and institutional requirements. Written informed consent for participation was not required from the participants or the participants’ legal guardians/next of kin in accordance with the national legislation and institutional requirements because the voluntariness and anonymity of the participants were ensured. The survey was designed and administered in compliance with applicable ethical standards in the educational context and with the approval of the principals of the participating educational centers.
SG-G: Conceptualization, Data curation, Formal analysis, Investigation, Methodology, Project administration, Resources, Software, Supervision, Validation, Visualization, Writing – original draft, Writing – review & editing. MH-P: Conceptualization, Data curation, Formal analysis, Investigation, Methodology, Project administration, Resources, Supervision, Validation, Visualization, Writing – review & editing. JA-S: Conceptualization, Data curation, Formal analysis, Investigation, Methodology, Project administration, Resources, Supervision, Validation, Visualization, Writing – review & editing. PH-C: Conceptualization, Data curation, Formal analysis, Investigation, Methodology, Project administration, Resources, Supervision, Validation, Visualization, Writing – review & editing. EQ-G: Conceptualization, Data curation, Formal analysis, Investigation, Methodology, Project administration, Resources, Supervision, Validation, Visualization, Writing – review & editing.
The author(s) declare that financial support was received for the research and/or publication of this article. This work was supported by the University of Las Palmas de Gran Canaria through the project PROYECTOS I+D Y TRANSFERENCIA SD-2302 Subconcepto: 6409940, funded by Agencia Canaria De Investigación Innovación Y Sociedad De La Información Gobierno De Canarias.
The authors would like to acknowledge the foundational work presented in the previous article, The lack of STEM vocations and gender gap in secondary education students (Hernández-Pérez et al., 2024), which served as a basis for this research. The prior study, which included significant contributions in data collection and fieldwork, has been instrumental in shaping the current analysis and expanding on aspects not previously addressed. The authors also acknowledge Álvarez and Lanchares for providing the questionnaire used in this study, which has yielded valuable insights and results.
The authors declare that the research was conducted in the absence of any commercial or financial relationships that could be construed as a potential conflict of interest.
The authors declare that Gen AI was used in the creation of this manuscript. To assist with the writing and translation of the text, as well as to locate some of the sources used and find their corresponding references. All information provided by the AI has been corrected, reviewed, and verified to ensure its validity and reliability. ChatGPT, GPT-4 model, version 2024, developed by OpenAI, was used.
All claims expressed in this article are solely those of the authors and do not necessarily represent those of their affiliated organizations, or those of the publisher, the editors and the reviewers. Any product that may be evaluated in this article, or claim that may be made by its manufacturer, is not guaranteed or endorsed by the publisher.
Ahmed, S. K. (2024). How to choose a sampling technique and determine sample size for research: a simplified guide for researchers. Oral Oncol. Rep. 12:100662. doi: 10.1016/j.oor.2024.100662
Anderson, G. J., and Arsenault, N. (2002). Fundamentals of educational research. Second Edn. London: RoutledgeFalmer (Falmer Press Teachers’ Library).
Ayuso, A., Merayo, N., Ruiz, I., and Fernandez, P. (2022). Challenges of STEM vocations in secondary education. IEEE Trans. Educ. 65, 713–724. doi: 10.1109/TE.2022.3172993
Bilbao, J., Bravo, E., García, O., Rebollar, C., Laakso, M. J., Kaarto, H., et al. (2024). Introducing computational thinking and algebraic thinking in the European educational systems. Int. J. Educ. Inf. Technol. 18, 11–19. doi: 10.46300/9109.2024.18.2
Canclini Masserini, M., and Fernández-Darraz, M. C. (2024). Elecciones vocacionales de mujeres jóvenes: factores familiares, sexismo y motivaciones académicas. Calidad en la Educación 60:21–22. doi: 10.31619/caledu.n60.1438
Choi, A. (2021). España ante la Revolución Industrial 4.0: mercado laboral y formación. Araucaria 47, 479–505. doi: 10.12795/araucaria.2021.i47.21
Cicchetti, D. V., and Sparrow, S. A. (1981). Developing criteria for establishing interrater reliability of specific items: applications to assessment of adaptive behavior. Am. J. Ment. Defic. 86, 127–137
Cobreros, L., Galindo, J., and Raigada, T. (2024). Mujeres en STEM: Desde la educación básica hasta la carrera laboral. EsadeEcPol. Available online at: https://www.esade.edu/ecpol/es/publicaciones/mujeres-en-stem/ (Accessed October 27, 2024).
Connolly, C., Hijón Neira, R., and Garcia-Iruela, M. (2021). Developing and assessing computational thinking in secondary education using a TPACK guided scratch visual execution environment. Int. J. Comput. Sci. Educ. Schools 4, 3–23. doi: 10.21585/ijcses.v4i4.98
Cortina, J. M. (1993). What is coefficient alpha? An examination of theory and applications. J. Appl. Psychol. 78, 98–104. doi: 10.1037/0021-9010.78.1.98
Dasgupta, N., and Stout, J. G. (2014). Girls and women in science, technology, engineering, and mathematics: STEMing the tide and broadening participation in STEM careers. Policy Insights Behav. Brain Sci. 1, 21–29. doi: 10.1177/2372732214549471
European Commission. Directorate General for Employment, Social Affairs and Inclusion. (2020). European skills agenda: skills for jobs. LU: Publications Office. Available online at: https://data.europa.eu/doi/10.2767/978543 (Accessed October 27, 2024).
European Commission. Joint Research Centre. (2022). Reviewing computational thinking in compulsory education: state of play and practices from computing education. LU: Publications Office. Available online at: https://data.europa.eu/doi/10.2760/126955 (Accessed October 27, 2024).
European Commission: Directorate-General for Justice and Consumers (2024). 2024 report on gender equality in the EU : Publications Office of the European Union.
European Union (2019). Agenda 2030 for sustainable development goals: a European approach. Brussels: European Commission.
Eurostat (2023). Tertiary education statistics. Available online at: https://ec.europa.eu/eurostat/statistics-explained/index.php?title=Tertiary_education_statistics (Accessed November 2, 2024).
Fouad, N. A., Hackett, G., Smith, P. L., Kantamneni, N., Fitzpatrick, M., Haag, S., et al. (2010). Barriers and supports for continuing in mathematics and science: gender and educational level differences. J. Vocat. Behav. 77, 361–373. doi: 10.1016/j.jvb.2010.06.004
GEM Report UNESCO (2024). Global education monitoring report 2024, gender report: technology on her terms : GEM Report UNESCO.
Gender Equality Commission (GEC) (2024). Gender equality strategy 2024–2029. Estrasburgo: Council of Europe.
Gesquiere, N., and Wyffels, F. (2022) ‘Computational thinking in Flanders’ compulsory education’, CTE-STEM 2022 conference [preprint].
Gobierno de Canarias (2024). Datos de Educación | Consejería de Educación, Formación Profesional, Actividad Física y Deportes | Gobierno de Canarias. Available online at: https://www.gobiernodecanarias.org/eucd/consejeria/datos_consejeria/datos-educacion/index.html (Accessed October 27, 2024).
González Gallego, S., Coll, A. S., Carballo, R. V., Rodríguez, A. A., Rodríguez, O. G., Hernández, H. P., et al. (2022). Lanzamiento de Proyecto de Centro de Pensamiento Computacional en Educación Secundaria. Lecciones Aprendidas y Planificación Futura partiendo del real Decreto de Enseñanzas Mínimas de la LOMLOE. Formación del Profesorado e Investigación en Educación Matemática 14, 137–171.
González Gallego, S., González, R. Q., Quevedo Gutiérrez, E. G., and Callicó, G. M. (2021). “Creando robots: Proyecto de centro para la adquisición de competencias mediante robótica educativa en educación secundaria obligatoria” in Innovación e investigación docente en educación: Experiencias prácticas (Dykinson), 585–614.
González-Gallego, S. (2025). Cuestionario Para los centros educativos artículo a critical examination of the underlying causes of the gender gap in STEM and the influence of computational thinking projects applied in secondary school on STEM Higher Education : Zenodo.
Grover, S., and Pea, R. (2013). Computational thinking in K–12: a review of the state of the field. Educ. Res. 42, 38–43. doi: 10.3102/0013189X12463051
Henriksen, E. K., Jensen, F., and Sjaastad, J. (2015). The role of out-of-school experiences and targeted recruitment efforts in Norwegian science and technology students’ educational choice. Int. J. Sci. Educ. Part B 5, 203–222. doi: 10.1080/21548455.2014.900585
Hernández-Pérez, M., Alonso-Sánchez, J. A., Hernández-Castellano, P. M., and Quevedo-Gutiérrez, E. G. (2024). The lack of STEM vocations and gender gap in secondary education students. Front. Educ. 9:1428952. doi: 10.3389/feduc.2024.1428952
Huang, W., and Looi, C.-K. (2021). “A critical review of literature on unplugged pedagogies in K-12 computer science and computational thinking education”. Computer Science Education, 31, 83–111. doi: 10.1080/08993408.2020.1789411
Merayo, N., and Ayuso, A. (2023a). Analysis of barriers, supports and gender gap in the choice of STEM studies in secondary education. Int. J. Technol. Des. Educ. 33, 1471–1498. doi: 10.1007/s10798-022-09776-9
Merayo, N., and Ayuso, A. (2023b). Encuesta sobre la percepción de barreras y apoyos en la elección de estudios STEM dirigida a estudiantes de secundaria. Available at : https://uvadoc.uva.es/handle/10324/58682
Ministerio de Ciencia, Innovación y Universidades (2024). Workbook: EEU2023 Estadísticas de Estudiantes en las Universidades Españolas. Available online at: https://public.tableau.com/views/EEU2023/InfografiaEEU?%3AshowVizHome=no&%3Aembed=true#3 (Accessed November 12, 2024).
Ministerio de Educación, Formación Profesional y Deportes (2020). TIMSS 2019. Estudio Internacional de Tendencias en Matemáticas y Ciencias. Informe español. Available online at: https://www.libreria.educacion.gob.es/libro/timss-2019-estudio-internacional-de-tendencias-en-matematicas-y-ciencias-informe-espanol_170122/edicion/pdf-184025/ (Accessed: October 27, 2024).
Ministerio de Educación, Formación Profesional y Deportes (2023). PISA 2022. Programa para la Evaluación Internacional de los Estudiantes. Informe español - libreria.educacion.gob.es. Madrid: Instituto Nacional de Evaluación Educación.
Ministerio de Universidades (2023). Datos y Cifras del Sistema Universitario Español. Curso 2022–2023. Ministerio de Universidades. Available online at: https://www.universidades.gob.es/wp-content/uploads/2023/04/DyC_2023_web_v2.pdf (Accessed: October 27, 2024).
Molina-Ayuso, Á., Adamuz-Povedano, N., Bracho-López, R., and Torralbo-Rodríguez, M. (2022). Introduction to computational thinking with scratch for teacher training for Spanish primary school teachers in mathematics. Educ. Sci. 12:899. doi: 10.3390/educsci12120899
Mullis, I. V. S., Martin, M. O., Foy, P., Kelly, D. L., and Fishbein, B. (2020). TIMSS 2019 international results in mathematics and science. Chestnut Hill, MA: TIMSS & PIRLS International Study Center, Boston College.
Narganes-Pineda, A., Araña-Suárez, R. E., Hernández-Pérez, M., González-Suárez, P., Hernández-Castellano, P. M., and Marrero-Alemán, M. D. (2024). “Project “Acércate a la Ingeniería”: promoting scientific and technological vocation of secondary education students” in Advances in design engineering IV. eds. C. Manchado Del Val, et al. (Cham: Springer Nature Switzerland (Lecture Notes in Mechanical Engineering),), 979–988.
National Academies of Sciences, Engineering, and Medicine (2021) in Cultivating interest and competencies in computing: Authentic experiences and design factors. eds. B. M. Means and A. Stephens (Washington, D.C.: National Academies Press), 25912.
OECD (2023). PISA 2022 results (volume I): the state of learning and equity in education : OECD (PISA).
Palmer, T.-A., Burke, P. F., and Aubusson, P. (2017). Why school students choose and reject science: a study of the factors that students consider when selecting subjects. Int. J. Sci. Educ. 39, 645–662. doi: 10.1080/09500693.2017.1299949
R Core Team (2021). A language and environment for statistical computing. Available online at: https://cran.r-project.org (Accessed: November 20, 2024).
Robson, C. (2011). Real world research: A resource for users of social research methods in applied settings. 3rd Edn. Chichester: Wiley.
Selby, C. C., and Woollard, J. (2013). ‘Computational Thinking: The Developing Definition’. Available at : https://eprints.soton.ac.uk/356481/1/Selby_Woollard_bg_soton_eprints.pdf
Shute, V. J., Sun, C., and Asbell-Clarke, J. (2017). Demystifying computational thinking’, Educational Research Review, 22, 142–158. doi: 10.1016/j.edurev.2017.09.003
Sjøberg, Svein, and Jensen, Fredrik (2010). ROSE: The Relevance of Science Education (completed) - Department of Teacher Education and School Research. Available online at: https://www.uv.uio.no/ils/english/research/projects/rose/index.html (Accessed February 21, 2025).
The jamovi project (2022). ‘jamovi’. (jamovi). Available online at: https://www.jamovi.org (Accessed: November 24, 2024).
UNESCO (2017). Cracking the code girls’ and women’s education in science, technology, engineering and mathematics (STEM) : UNESCO.
UNESCO (2021). UNESCO science report: the race against time for smarter development. Paris: United Nations Educational, Scientific and Cultural Organization (UNESCO science report, 2021).
Valls Pou, A., Canaleta, X., and Fonseca, D. (2022). Computational thinking and educational robotics integrated into project-based learning. Sensors 22:3746. doi: 10.3390/s22103746
Vennix, J., Den Brok, P., and Taconis, R. (2018). Do outreach activities in secondary STEM education motivate students and improve their attitudes towards STEM? Int. J. Sci. Educ. 40, 1263–1283. doi: 10.1080/09500693.2018.1473659
Keywords: gender gap, computational thinking, secondary education, STEM vocations, female stereotypes, STEM Higher Education, educational robotics, female references
Citation: González-Gallego S, Hernández-Pérez M, Alonso-Sánchez JA, Hernández-Castellano PM and Quevedo-Gutiérrez EG (2025) A critical examination of the underlying causes of the gender gap in STEM and the influence of computational thinking projects applied in secondary school on STEM Higher Education. Front. Educ. 10:1537040. doi: 10.3389/feduc.2025.1537040
Received: 29 November 2024; Accepted: 03 March 2025;
Published: 19 March 2025.
Edited by:
Zahid Pranjolx2, University of Sussex, United KingdomReviewed by:
Pilar Gema Rodríguez Ortega, University of Cordoba, SpainCopyright © 2025 González-Gallego, Hernández-Pérez, Alonso-Sánchez, Hernández-Castellano and Quevedo-Gutiérrez. This is an open-access article distributed under the terms of the Creative Commons Attribution License (CC BY). The use, distribution or reproduction in other forums is permitted, provided the original author(s) and the copyright owner(s) are credited and that the original publication in this journal is cited, in accordance with accepted academic practice. No use, distribution or reproduction is permitted which does not comply with these terms.
*Correspondence: Sofía González-Gallego, YWx1MDEwMTUzNDY1N0B1bGwuZWR1LmVz; Eduardo G. Quevedo-Gutiérrez, ZWR1YXJkby5xdWV2ZWRvQHVscGdjLmVz
Disclaimer: All claims expressed in this article are solely those of the authors and do not necessarily represent those of their affiliated organizations, or those of the publisher, the editors and the reviewers. Any product that may be evaluated in this article or claim that may be made by its manufacturer is not guaranteed or endorsed by the publisher.
Research integrity at Frontiers
Learn more about the work of our research integrity team to safeguard the quality of each article we publish.