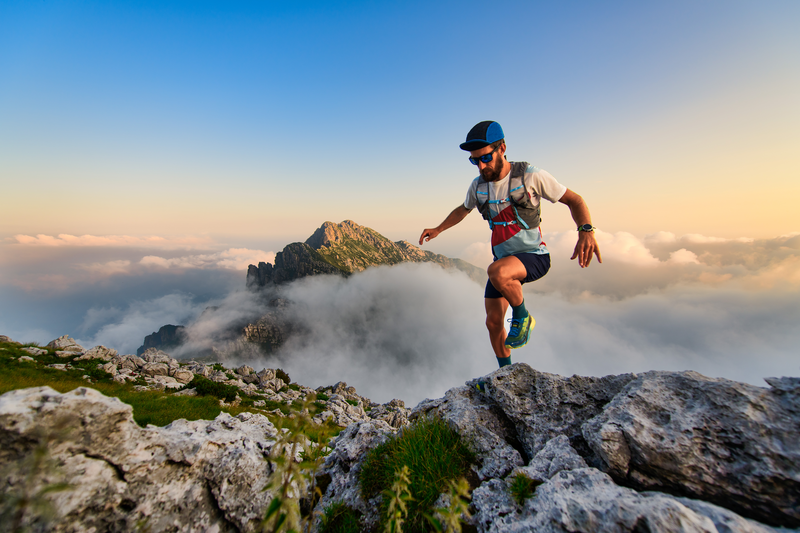
94% of researchers rate our articles as excellent or good
Learn more about the work of our research integrity team to safeguard the quality of each article we publish.
Find out more
REVIEW article
Front. Educ. , 15 August 2024
Sec. STEM Education
Volume 9 - 2024 | https://doi.org/10.3389/feduc.2024.1454788
This article is part of the Research Topic Utilization of Neuroscience Core Concepts to Guide Programs, Curricula, Courses, and Assessment in Diverse Institutional Contexts View all 8 articles
Recently, community-derived core concepts for neuroscience higher education were developed and published. These core concepts can serve as a valuable resource to ensure that a neuroscience-based educational program is not only concept-focused but also addresses the call for reform of higher education, as noted in the vision and change report. The number of undergraduate neuroscience programs is expanding throughout the nation, but unfortunately, the existing blueprints to design and launch such programs do not incorporate these core concepts. Furthermore, unpacking these core concepts in a resource-limited setting is logistically challenging. We reflected on the coverage of these core concepts within our existing neuroscience minor at a medium-sized, primarily residential, high undergraduate, public 4-year institution. In addition to assessing the number of community-derived core concepts addressed in our courses, our reflection discusses strategies for addressing challenges associated with (1) a departmental home for the program, (2) a meaningful student experience with limited resources, and (3) growing and developing the program into a minor, or from a minor into a major. These strategies may provide a roadmap for other institutions to launch or grow their own neuroscience program.
Neuroscience major enrollment has been growing consistently since it was initially assessed compared to the most recent data collection (Ramos et al., 2011; Rochon et al., 2019). However, recommendations from authors and the Society for Neuroscience and the Faculty for Undergraduate Neuroscience (FUN) have largely focused on core competencies for post-secondary neuroscience education and have culminated in revised and re-revised blueprints for getting started (Wiertelak and Ramirez, 2008; Kerchner et al., 2012; Wiertelak et al., 2018), as well as essential principles for primary and secondary education (BrainFacts.org; Ramirez, 2020). Absent from these recommendations was a set of core concepts for post-secondary neuroscience education that can serve as guidelines describing the integral aspects all students should comprehend.
A number of stakeholders collaborated to generate Neuroscience core concepts with input from a variety of educators from diverse institution types, as well as national and working group surveys (Chen et al., 2023). For ease of reference, we have briefly summarized these core concepts below (Table 1). The current article supplements the existing blueprints and illustrates the challenges and successes of incorporating such core concepts into a functional neuroscience program.
Table 1. A brief summary of the community-derived Neuroscience core concepts from Chen et al. (2023).
When examining these core concepts, what becomes clear is that several of them are moored in a foundation of biological principles. This is not particularly surprising given the interdisciplinary nature of neuroscience and neuroscience research. However, this foundation might serve as a barrier to implementation for a notable number of institutions, particularly those where a neuroscience major and/or minor is not offered by a separate neuroscience department or from a biology department.
When initially assessed in 2011, Primarily Undergraduate Institutions (PUIs) comprised 26 of the 111 institutions offering a neuroscience minor (Ramos et al., 2011). By 2019, when a similar assessment was performed, 43 of the 221 institutions were PUIs (Rochon et al., 2019). This demonstrates that the proportion of neuroscience programs offered by PUIs has remained relatively consistent as the total number of programs nearly doubled over a decade. It is important to note that all the analyses done by Ramos et al. (2011) over the years view and analyze neuroscience programs as an offshoot of a life science offering rather than one from psychology. The authors indicate that offerings related to a psychology department (e.g., biopsychology, cognitive science) are viewed as related to neuroscience (Ramos et al., 2011). This decision in and of itself indicates that many within the field view neuroscience as more of a biological discipline than a psychological discipline. We mention this not to debate the specific point but rather to highlight that a number of institutions will have more barriers to implementing these core concepts and will likely require investment from both faculty and administrators.
It is also necessary to place these programmatic challenges in the context of enrollment trends in higher education. Unfortunately, neuroscience majors are not included in national enrollment analysis, but both biology and psychology are consistently represented. Although overall enrollments are trending downward, the slope of that downward direction is decreasing as we exit the pandemic (Berg et al., 2023). However, the number of students who are majoring in biology or biomedical sciences is decreasing, while the number of students who are majoring in psychology is increasing (Berg et al., 2023). If these trends continue, the number of psychology students will overtake the number of biology students in the coming years (Berg et al., 2023). These trends offer an opportunity for growth as both pools represent the majority of students who may enroll in neuroscience courses or become a neuroscience major (or minor or double major). This also suggests that recognizing and addressing the disparity in biological and psychological underpinnings of these core concepts will likely prove beneficial for most institutions, not just smaller and/or undergraduate-focused institutions.
Several core concepts are quite difficult to explore in any meaningful depth in courses typically offered by a psychology department. Please note that this likely excludes a joint psychology and neuroscience department, provided at least some of the neuroscience faculty utilize more biological approaches in their training, research, and teaching. Due to the composition of many undergraduate-focused institutions, some will offer a neuroscience curriculum situated within a psychology department. Given that a significant number of students interested in pursuing neuroscience attend a PUI, be it a small liberal arts college (SLAC) or public institution, it is in the interests of all stakeholders to find a suitable means for all institutions to teach these core concepts to enrolled students.
We will use our own institution as an example, noting that each institution will have its own unique set of challenges and advantages. Our institution, Minnesota State University Moorhead (MSUM), is located in Moorhead, Minnesota, near the North Dakota border and the city of Fargo. According to the Carnegie classification system, MSUM is a medium-sized, primarily residential, high undergraduate, public four-year institution.
Within our institution, there are two primary neuroscientists, one in the psychology department and one in the biology department. These two faculty members teach the foundational curriculum of the neuroscience minor offered. The minor at MSUM includes a three-course core, as well as restricted electives situated in each discipline; see Table 2 for an overview. These two faculty members are the only members of their respective departments with the expertise appropriate to teach the core neuroscience courses. However, other departmental faculty are capable of teaching the restricted elective courses and do so as institutional and departmental needs necessitate.
Table 2. An snapshot of the current neuroscience minor at Minnesota State University Moorhead (MSUM), Moorhead, MN.
Due to the limitations in the number and diversity of courses, a minor would be expected to lack the depth of exploration of each core concept in comparison to a neuroscience major. Although limited, this may be an appropriate offering for a smaller institution with limited resources. However, as the interest in neuroscience grows, these deficiencies must be addressed to potentially transition our offering from a minor to a major. The process of mapping the concepts allowed us to identify which of the core concepts received the least attention. Within the required courses of the minor, the biological neuroscience course addressed all eight concepts to some degree. The introductory neuroscience course and the psychological neuroscience course did not address all of the core concepts, as they each only superficially addressed the core concept of Evolution and did not address the concepts of Gene–Environment Interactions or Structure–Function Relationships.
During further analysis of the restricted electives and other available electives within our own modest neuroscience minor, it became clear that the concepts of Evolution and Gene–Environment Interactions were not addressed in any meaningful depth within offerings from the psychology department. The Structure–Function Relationship concept is well-addressed at the circuit level but not at all at the cellular and molecular levels within psychology department courses. The reality is that none of the faculty currently in the MSUM psychology department have the requisite expertise to address these concepts. Furthermore, it is unreasonable to assume that a faculty member appropriately trained to teach non-neuroscience curriculum within a psychology department would possess the expertise to address these concepts. Quite simply, these are concepts rooted deeply in a different discipline (or disciplines): biology and/or biochemistry.
The analysis of our institution suggests that it is not possible to address all of the core concepts of neuroscience without a collaboration between scientific disciplines. Even if there are multiple qualified individuals to instruct various neuroscience courses from the psychology department, they will still require investment from at least one biology or biochemistry faculty member.
When beginning at a smaller curricular scale with a neuroscience minor, it is easier to gain sufficient coverage of the neuroscience core concepts. For an institution that does not possess a biology faculty member with a neuroscience background and/or interest, developing a neuroscience minor might be the current possible ceiling. Even with a biology faculty member contributing to a neuroscience minor curriculum, it may not be feasible to progress from a minor to a major. Fortunately, post-graduate destinations, even neuroscience doctoral programs, do not require students to obtain an undergraduate degree in neuroscience. Thus, offering a minor in neuroscience provides a means for interested students to gain some relevant exposure at the undergraduate level.
As some programs progress, they may have the opportunity to expand from offering a neuroscience minor to a major. If the minor is housed solely or primarily within a psychology department, biology courses will need to be incorporated to address all of the neuroscience core concepts adequately. Analysis of our own minor yielded some possible solutions in our current composition, with substantial caveats. There are several biology courses, such as developmental biology and cellular physiology, that provide more exploration of the concepts of Evolution, Gene–Environment Interactions, and Structure–Function Relationships. However, at our institution, these are upper-division courses that possess substantial pre-requisite coursework (general biology courses, general chemistry courses, and genetics). The specific pre-requisite coursework will likely differ between institutions, but the aforementioned courses are typically upper-division courses that require completion of other biology courses prior to enrolling. These pre-requisite courses comprise hidden work for students that can add nearly the equivalent of a biology minor for a psychology major pursuing a neuroscience minor. Anecdotally, we have observed that the students majoring in psychology and minoring in neuroscience at MSUM do not enroll in developmental biology or cellular physiology. Informal conversations with these students revealed that the reasons they avoided these courses were the pre-required coursework and the general unease with the subject matter.
MSUM currently does not possess enough faculty with neuroscience expertise to grow our program from a minor to a major. It is possible to increase the coverage of these core concepts within our minor, but we have not implemented any changes as of yet. The inclusion of a required course such as developmental biology (which is currently an elective within the minor) would likely make the minor more appealing to biology majors while simultaneously deterring psychology majors. Given that psychology majors comprise nearly 70% of our current program, this change would likely negatively impact overall enrollment. We are currently determining which courses could be modified to maximize efficiency and help ensure that the student load remains manageable.
Although institutions with graduate programs are not immune to cost concerns, PUIs are more likely to have financial constraints on the experiences they can provide. These financial constraints can limit the quantity, frequency, and/or depth of innovations that can be introduced within a curriculum. For example, if a neuroscience program has only a few associated faculty members, each may choose to implement major changes to a single course at a time, as teaching loads may restrict their ability to enact changes across every course they teach simultaneously. Faculty interested in developing neuroscience courses and those attempting to assemble their current offerings into a minor may benefit from affordable approaches to covering the neuroscience core concepts.
One cost-effective approach to exploring these core concepts is to utilize a series of classic published papers (Harrington et al., 2015). This approach can be molded to fit with a variety of knowledge levels and specific course learning objectives. For a specific example, there is a four-paper series exploring the initial discovery of voltage-gated potassium channel sequence and characterization (Kamb et al., 1987; Tempel et al., 1987; Wei et al., 1990; Zhou et al., 2001) that could serve as a means to explore Structure and Function Relationships, Evolution, Communication Modalities, and Nervous System Functions (Harrington et al., 2015). There are a number of approaches that could be utilized to explore primary literature, and one evidence-based approach that might prove useful is the CREATE method (Consider, Read, Elucidate hypotheses, Analyze and interpret the data, and Think of the next Experiment) (Hoskins et al., 2007). The CREATE method slows the pace at which students are expected to digest the paper and digs into the minutia of the publications through a number of active learning approaches to help the students comprehend all of the individual components, and typically involves exploring four publications from the same laboratory through the duration of the course (Hoskins et al., 2011). This approach has been utilized successfully in both upper-division and introductory offerings (Gottesman and Hoskins, 2013). The approach has also been truncated and modified to be a component of an existing course rather than the sole focus, and success has been found in that format (Lo et al., 2020). The CREATE approach has also been truncated and incorporated into a large enrollment general education course as a successful means of increasing student persistence and completion (Bodnar et al., 2016).
Primary literature has been utilized with case studies to help keep all students interested, as students not on research paths tend to have less engagement with primary literature (O’Keeffe and McCarthy, 2017). Cook-Snyder (2017) effectively utilized case studies to reinforce and deepen student comprehension following more traditional lecture-based content. Willard and Brasier (2014) replaced a traditional textbook with primary literature in an introductory course and found increases in enthusiasm and confidence for their students. Other potential approaches could incorporate some evidence-based practices in conjunction with exploring primary literature. For example, annotating and transforming data was demonstrated to help deepen comprehension (Pugh-Bernard and Kenyon, 2021). There are several other approaches to exploring primary literature, including the jigsaw collaborative learning approach and humanizing literature, that could be incorporated into a course (Hartman et al., 2017). Although the implementation of these approaches varies with respect to the time and effort required from the faculty member, they offer a means to explore difficult concepts at a low financial cost across a variety of educational levels.
A cost-effective approach to exploring Structure and Function Relationships could include 3D printing biomolecules. Given the size of biomolecules, it can sometimes be difficult for students to conceptualize the Structure and Function Relationships between them, and simply providing accurate, tangible models can assist with comprehension and retention (Herman et al., 2006; Jittivadhna et al., 2010). Guides to produce models of surprisingly complex protein structures utilizing 3D printing are readily available and would represent reasonable one-time costs for repeated usage (Da Veiga Beltrame et al., 2017).
These cost-effective approaches offer strategies that could be employed in existing courses to add more comprehensive coverage of one or more core concepts or allow for the creation of a new neuroscience course. Increasing the coverage of core concepts could then potentially facilitate establishing or enhancing a neuroscience minor. We are implementing a truncated CREATE approach to expand the coverage of additional core concepts in the psychological neuroscience course. However, we are still in the process of composing the module and do not have any relevant results to relay on its outcomes.
The inclusion of non-neuroscience courses into a minor might provide a sustainable solution for some programs, but this is likely insufficient for a thriving neuroscience major. As detailed above, our internal analysis revealed that psychology-based neuroscience courses struggle to address the Core Concepts of Evolution, Gene–Environment Interactions, and Structure–Function Relationships. We will now offer some potential solutions to address each of the three core concepts that do not receive sufficient coverage. Some of these approaches are also cost-effective to implement and may provide additional options for instructors with restricted resources.
Evolution is a concept that is well-addressed in a myriad of biological courses but can also be incorporated into a number of psychological courses, at least from a primate evolutionary context. Evolution is arguably the key foundational concept of biology (Wei et al., 2012), and as such, receives appropriate time and attention in many courses, including introductory courses. This provides an avenue for initial exposure to evolutionary concepts within the larger biological framework that could then be explored in more depth within a neuroscience context. Topics such as the origin and expansion of the neocortex could be incorporated into an introductory neuroscience course housed within a psychology department (or biology department), where the course highlights the expansions that have occurred within the primate lineage (Striedter, 2023). In fact, Striedter (2023) identified two publications that provide six different useful illustrations to assist in teaching this specific topic (Buckner and Krienen, 2013; Kaas, 2019). This prefrontal cortical expansion that occurred in primates can be linked to functional benefits and then used to highlight interesting convergent evolution where a number of avian species possess similar functional benefits from morphologically similar but evolutionarily distinct neural structures (Striedter, 2023). Yet again, there are useful figures to help highlight this example of convergent evolution (Brusatte et al., 2015; Puelles et al., 2017).
The Gene–Environment Interaction concept is more difficult to address for several reasons. Generally, numerous concepts in biology and biochemistry are challenging because they conflict with informal ways people think about the world (Coley and Tanner, 2012). More specifically, some students possess misconceptions about biological principles that are derived from either essentialist thinking, teleological thinking, and/or anthropocentric thinking (Coley and Tanner, 2012). Furthermore, the Gene–Environment Interaction concept already receives inadequate exploration within most biological curricula (Gericke and Mc Ewen, 2023). Fully comprehending Gene–Environment Interactions also requires foundational knowledge. Without a background in genetics, molecular biology, and regulatory cellular biology, students may struggle to grasp the depth and import of this concept completely. This presents a challenge for any neuroscience offering housed solely within a psychology department. This would raise the issue of expanding pre-requisite coursework yet again, where students would be required to take several foundational courses before thoroughly addressing this concept.
Part of the challenge with comprehending Gene–Environment Interactions is that students tend to largely attribute only genetic influences to body development (Hammann et al., 2021). Conversely, students largely attribute only environmental influences to the development of mind-related functions (Hammann et al., 2021). However, this also presents an opportunity within neuroscience education to directly address a misconception and expand the knowledge of a core concept. A two-phase model of instruction has been outlined previously (Zang and Hammann, 2022), where students are presented with the topic (e.g., depression) during the first phase and then further explore very specific traits within the topic during the second phase. It is during the second phase, when students are presented with tools to start developing causal relationships, that a single causal relationship (e.g., environment is the sole cause of depression) is compared to another single causal relationship (e.g., genetic inheritance is the sole cause of depression). This exploration of singular explanations aligns with the cognitive preference for reductionist reasoning (Grotzer and Mittlefehldt, 2012). Various prompts throughout the exercise help the students critically analyze the situation and determine that neither single cause is sufficient and understand that both causes are necessary to create the phenomena (Zang and Hammann, 2022).
The Structure–Function Relationship concept is another concept that is difficult to address. Certain aspects of this concept can and should be addressed at the circuit level and above, and psychology faculty should be well-equipped to do just that. Utilizing case studies to explore Structure–Function Relationships in the context of neuroanatomy is one potentially useful approach (Kennedy, 2013). However, a substantial aspect of this concept is the Structure–Function Relationship at the cellular and molecular level. This is rooted in molecular biology and/or biochemistry and, as such, also requires some foundational knowledge to address properly (Yoho et al., 2019). Without an understanding of the central dogma of biology and how that relates to protein structure, it is not possible to reach any substantive depth on this topic. Again, this is an unreasonable expectation for any neuroscience offering housed within a psychology department. Students will need to navigate a number of biology and biochemistry courses to acquire the requisite foundational knowledge.
Although introductory chemistry courses are common fundamental courses for students majoring in biological sciences, psychology departments typically do not require their majors to complete any courses in chemistry. However, these introductory chemistry courses could feature some interdisciplinary topics to not only help neuroscience students adjust but also improve interest and engagement across all students (Sumter and Owens, 2011). Sumter and Owens (2011) utilized a neuroscience module to teach and reinforce general chemistry concepts, as well as to allow students to make connections between chemistry, biology, and psychology. Specifically, the module focused on ion concentrations, charge, and movement in response to various ligands and then other compounds (e.g., toxins and pharmaceutical agents) (Sumter and Owens, 2011). Such a module in and of itself would provide some initial exploration of Structure and Function Relationships, Nervous System Function, and possibly Gene–Environment Interactions.
It is worth noting that this approach requires significant effort from a chemistry faculty member. This may prove challenging or impossible at some institutions and will likely require some additional training and work for the chemistry faculty member. Fortunately, the implementation of these interdisciplinary modules within foundational courses has previously been demonstrated to benefit all students, not just those interested in neuroscience (Sumter and Owens, 2011). However, convincing a faculty member to modify their course is not a trivial endeavor, which is well-documented within the existing literature (Brownell and Tanner, 2012; Borrego and Henderson, 2014; Petersen et al., 2020; Smith and Thoman, 2024). We do not want to understate the challenge associated with recruiting a chemistry colleague receptive to implementing new evidence-based approaches, but we would like to highlight several studies where the authors discuss potential strategies to assist faculty in adopting a new pedagogical approach (Borrego and Henderson, 2014; Froyd et al., 2017; Petersen et al., 2020; Smith and Thoman, 2024).
If a chemistry faculty member or department is interested, there are additional opportunities to expand the coverage of neuroscience core concepts while simultaneously enhancing the educational experiences for all students. Introductory chemistry laboratory curriculum could also incorporate classroom undergraduate research experiences (CUREs) to explore Structure–Function Relationships. A CURE described by Kean et al. (2019) also provides space to incorporate some Gene–Environment Interaction pieces, though the overall focus will be on Structure–Function Relationships. Specifically, this CURE explores protein structure utilizing noncanonical amino acid incorporation to generate the changes (Kean et al., 2019). Previous research found that CURE incorporation increased student comprehension and enthusiasm for the subject, as well as increased participation in faculty-mentored research (Kowalski et al., 2016). The incorporation of CUREs has been demonstrated to increase student retention (Weaver et al., 2008), and involvement in research has also been linked to increased independence and motivation, as well as notable benefits for underrepresented groups (Lopatto, 2007). Established CUREs have communities to provide support for new adopters and opportunities for instructors to build the lab experience as they progress. Furthermore, Kean et al. (2019) have generated and made available a lab manual and instructor guide and will also provide genetic constructs and plasmids upon request.
Another potential alternative for providing exposure to the Structure and Function Relationships, Nervous System Function, and possibly Gene–Environment Interactions core concepts is for a psychology department to develop an introductory course or series of introductory courses on biopsychology, which could introduce biological principles in a psychology/neuroscience context. Such a course, or series of courses, could explore the evolution of the primate brain and convergent evolution of the avian brain, as mentioned above (Striedter, 2023). The course/s could also explore the development of the human brain, with an emphasis on how genes regulate development and/or how specific genes are disrupted in developmental disorders (Eising et al., 2019). This provides an in-road into an exploration of how gene expression can be impacted by environment, with examples across mammalian taxa (Katsioudi and Kostareli, 2020; Venkatesh and Makky, 2020). This also provides an opportunity to explore the function of various genes and how the structure of their resulting proteins can dictate their function (Dorji and Sriwattanarothai, 2015; Howell et al., 2019). Such a course may prove beneficial, even at institutions with a well-developed collaboration between biology and psychology faculty, as it may provide a means for both neuroscience and psychology students to approach and tackle these concepts. It could also provide a human or primate-centered examination of topics typically covered across an expansive range of taxa within biology courses. Although this approach would not provide as much depth in these three core concepts as the introductory chemistry course additions, institutional circumstances may make this alternative more feasible to implement.
In addition to the aforementioned approaches to increase coverage of the core concepts not typically addressed in psychology-based courses, some broader mechanisms may help promote student engagement, comprehension, and/or persistence. Developing a bridge to neuroscience workshop for incoming and/or new students in the program is one approach that can help improve the learning environment. Colón-Rodríguez et al. (2019) implemented a one-day workshop at the University of Puerto Rico for both prospective and current undergraduate students and found that it noticeably improved both understanding and enthusiasm for neuroscience novices. The duration of the workshop is of particular interest, as this is logistically much easier to implement than the original approaches. A bridge to neuroscience workshop could potentially prime new students so that they are more prepared and more persistent when engaged in challenging content. This is particularly relevant given that previous research has also demonstrated that preparation and attitudes are important predictors of success for intimidating classes, as was shown with psychology majors taking a course in biopsychology (Sgoutas-Emch et al., 2007). Furthermore, introducing Utility-Value Intervention at the start of a course has also been demonstrated to help improve student performance and persistence in introductory biomedical courses (Hecht et al., 2019). At our institution, we have also implemented a neuroscience journal club to promote comprehension, persistence, and enthusiasm among current and prospective neuroscience students (Berman et al., 2019; Drumm et al., 2019). It is also important to note that students involved in faculty-mentored research receive many benefits, in particular, fostering more persistence and comprehension, and promoting such opportunities will have many added benefits for the students, faculty, and institution (Russell et al., 2007; Petrella and Jung, 2008; Hernandez et al., 2017; Stanford et al., 2017). All these potential approaches help generate an environment in which students are more likely to succeed in the face of more challenging content.
Currently, the approximate teaching load of the required courses in the neuroscience minor at our institution equates to five-eighths of the teaching load of a single full-time faculty position. The restricted electives in the minor also equate to five-eighths of a teaching load. It is worth noting that these restricted electives are courses that existed prior to the introduction of the neuroscience minor, and these courses, as well as most of the required courses, contribute to multiple degree pathways specific to their respective departments. All the required courses are taught exclusively by the two neuroscience faculty (one in biology and one in psychology), while the restricted electives are taught by the two neuroscience faculty as scheduling allows.
Student demand will necessarily have to increase before any potential expansion of our neuroscience minor could occur. However, if there was sufficient demand such that the administration saw fit to expand the curriculum to offer a major in neuroscience, this could be initially done by devoting the existing two faculty members completely to the neuroscience curriculum (Table 3). This would necessitate a full-time faculty position within the biology department and at least half of a faculty teaching load covered within the psychology department. Ideally, this would be done as a full-time hire to support both the psychology and neuroscience programs. In addition to our existing curricular offerings, we would extend our introductory course across two semesters to provide a more extensive overview of all topics. An existing psychology neuroscience course would be altered to narrow the focus to biopsychology and clinical aspects. We would add a developmental neuroscience course to address Gene–Environment Interactions in a more comprehensive manner, and this course would also have the added benefit of expanding our coverage of Evolution and Structure and Function Relationships. We envision this course also to incorporate experimental approaches utilized within the field, primarily in a laboratory component. We would also alter an existing cellular physiology course to narrow the focus to cellular and molecular neuroscience, which will deepen our coverage of Structure and Function Relationships, as well as Gene–Environment Interactions and Evolution. All other courses included in this hypothetical major (see Table 3) are already offered as part of another degree path. Though not indicated on the table, all the biology, chemistry, and physics courses possess a required laboratory portion. The biology-based neuroscience courses (i.e., biology core) also all possess a required laboratory portion.
Table 3. An overview of a hypothetical major expanded from the existing neuroscience minor offered at our institution.
The hypothetical neuroscience major outlined in Table 3 requires 83–85 credits. Devoting only two full-time faculty members to a single major is quite thin, but the inclusion of foundational courses in biology, psychology, chemistry, and physics, as well as existing upper-division coursework in the biology or psychology major curricula, with some select additions from other departments, makes this feasible. This means that a variety of relevant electives will necessarily be taught by faculty members not associated with the neuroscience major but rather from other departments. This helps to create more breadth and options for students while keeping overhead instructional costs low. The degree offering would only have flexibility with elective courses offered by either the biology or psychology departments and neuroscience majors would have no diversity of neuroscience-specific elective offerings. The hope would be that an additional faculty member could be added as the number of students enrolled continued to grow and that this expansion would allow for more courses to be developed and allow for more flexibility within the neuroscience degree while still adequately addressing all the core concepts. The specialization of an additional faculty member would be carefully considered so as also to represent a foundational branch of neuroscience that is complementary to our institution and resources, such as neurophysiology or computational neuroscience. We also want to reiterate that progression from a minor to a major will not be practical or feasible for every institution. Despite steady growth from 0% at its creation in 2020 to 7.6% of the students enrolled in biology or psychology, the current circumstances dictate that our neuroscience minor should remain as-is until the environment changes sufficiently (e.g., interest, enrollment, funding, available faculty). Obviously, institutions with differing departmental structures and/or composition and different enrollments will necessarily have different concerns. Still, we hope that the analysis of our situation offers some relevant and informative parallels.
AS: Conceptualization, Writing – original draft, Writing – review & editing. CD: Writing – original draft, Writing – review & editing.
The author(s) declare that no financial support was received for the research, authorship, and/or publication of this article.
We thank Sumali Pandey for initiating this process and alerting us to this opportunity.
The authors declare that the research was conducted in the absence of any commercial or financial relationships that could be construed as a potential conflict of interest.
All claims expressed in this article are solely those of the authors and do not necessarily represent those of their affiliated organizations, or those of the publisher, the editors and the reviewers. Any product that may be evaluated in this article, or claim that may be made by its manufacturer, is not guaranteed or endorsed by the publisher.
Berg, B., Lee, S., Randolph, B., Ryu, M., and Shapiro, D. (2023). Current term enrollment estimates: spring 2023. Herndon, VA: National Student Clearinghouse Research Center.
Berman, D., Braig, Z., Simms, B., Anderson, T., Dougherty, K., Marcinkowski, K., et al. (2019). Efficacy of medical student surgery journal club. J. Surg. Educ. 76, 83–88. doi: 10.1016/j.jsurg.2018.06.006
Bodnar, R. J., Rotella, F. M., Loiacono, I., Coke, T., Olsson, K., Barrientos, A., et al. (2016). “C.R.E.A.T.E.”-ing unique primary-source research paper assignments for a pleasure and pain course teaching neuroscientific principles in a large general education undergraduate course. J. Undergrad. Neurosci. Educ. 14, A104–A110.
Borrego, M., and Henderson, C. (2014). Increasing the use of evidence-based teaching in STEM higher education: a comparison of eight change strategies. J. Eng. Edu. 103, 220–252. doi: 10.1002/jee.20040
Brownell, S. E., and Tanner, K. D. (2012). Barriers to faculty pedagogical change: lack of training, time, incentives, and…tensions with professional identity? LSE 11, 339–346. doi: 10.1187/cbe.12-09-0163
Brusatte, S. L., O’Connor, J. K., and Jarvis, E. D. (2015). The origin and diversification of birds. Curr. Biol. 25, R888–R898. doi: 10.1016/j.cub.2015.08.003
Buckner, R. L., and Krienen, F. M. (2013). The evolution of distributed association networks in the human brain. Trends Cogn. Sci. 17, 648–665. doi: 10.1016/j.tics.2013.09.017
Chen, A., Phillips, K. A., Schaefer, J. E., and Sonner, P. M. (2023). Community-derived core concepts for neuroscience higher education. LSE 22:ar18. doi: 10.1187/cbe.22-02-0018
Coley, J. D., and Tanner, K. D. (2012). Common origins of diverse misconceptions: cognitive principles and the development of biology thinking. LSE 11, 209–215. doi: 10.1187/cbe.12-06-0074
Colón-Rodríguez, A., Tiernan, C. T., Rodriguez-Tapia, E. S., and Atchison, W. D. (2019). Bridge to neuroscience workshop: an effective educational tool to introduce principles of neuroscience to Hispanics students. PLoS One 14:e0225116. doi: 10.1371/journal.pone.0225116
Cook-Snyder, D. R. (2017). Using case studies to promote student engagement in primary literature data analysis and evaluation. J. Undergrad. Neurosci. Educ. 16, C1–C6
Da Veiga Beltrame, E., Tyrwhitt-Drake, J., Roy, I., Shalaby, R., Suckale, J., and Pomeranz Krummel, D. (2017). 3D printing of biomolecular models for research and pedagogy. JoVE 121:55427. doi: 10.3791/55427
Dorji, K., and Sriwattanarothai, N. (2015). “Hands-on activities to link gene mutations with three-dimensional protein structures for high school students” in INTED2015 proceedings. Presented at the 9th international technology, education and development conference (Madrid: IATED), 7205–7213.
Drumm, B. T., Rae, M. G., and Ward, S. M. (2019). Active peer-mentored learning can improve student understanding of physiological concepts in an undergraduate journal club. Adv. Physiol. Educ. 43, 359–364. doi: 10.1152/advan.00049.2019
Eising, E., Carrion-Castillo, A., Vino, A., Strand, E. A., Jakielski, K. J., Scerri, T. S., et al. (2019). A set of regulatory genes co-expressed in embryonic human brain is implicated in disrupted speech development. Mol. Psychiatry 24, 1065–1078. doi: 10.1038/s41380-018-0020-x
Froyd, J. E., Henderson, C., Cole, R. S., Friedrichsen, D., Khatri, R., and Stanford, C. (2017). From dissemination to propagation: a new paradigm for education developers. Change Mag. Higher Learn. 49, 35–42. doi: 10.1080/00091383.2017.1357098
Gericke, N., and Mc Ewen, B. (2023). Defining epigenetic literacy: how to integrate epigenetics into the biology curriculum. J. Res. Sci. Teach. 60, 2216–2254. doi: 10.1002/tea.21856
Gottesman, A. J., and Hoskins, S. G. (2013). CREATE cornerstone: introduction to scientific thinking, a new course for STEM-interested freshmen, demystifies scientific thinking through analysis of scientific literature. LSE 12, 59–72. doi: 10.1187/cbe.12-11-0201
Grotzer, T., and Mittlefehldt, S. (2012). “The role of metacognition in students’ understanding and transfer of explanatory structures in science” in Metacognition in science education, contemporary trends and issues in science education. eds. A. Zohar and Y. J. Dori (Dordrecht: Springer), 79–99.
Hammann, M., Heemann, T., and Zang, J. C. S. (2021). “Why does multiple and interactive causation render comprehension of genetics phenomena difficult and what could genetics educators do about it?” in Genetics education, contributions from biology education research. eds. M. Haskel-Ittah and A. Yarden (Cham: Springer International Publishing), 127–144.
Harrington, I. A., Grisham, W., Brasier, D. J., Gallagher, S. P., Gizerian, S. S., Gordon, R. G., et al. (2015). An instructor’s guide to (some of) the most amazing papers in neuroscience. J. Undergrad. Neurosci. Educ. 14, R3–R14
Hartman, A. K., Borchardt, J. N., and Harris Bozer, A. L. (2017). Making primary literature come alive in the classroom. J. Undergrad. Neurosci. Educ. 15, R24–R28
Hecht, C. A., Harackiewicz, J. M., Priniski, S. J., Canning, E. A., Tibbetts, Y., and Hyde, J. S. (2019). Promoting persistence in the biological and medical sciences: an expectancy-value approach to intervention. J. Educ. Psychol. 111, 1462–1477. doi: 10.1037/edu0000356
Herman, T., Morris, J., Colton, S., Batiza, A., Patrick, M., Franzen, M., et al. (2006). Tactile teaching: exploring protein structure/function using physical models. Biochem. Mol. Bio. Educ. 34, 247–254. doi: 10.1002/bmb.2006.494034042649
Hernandez, P. R., Bloodhart, B., Barnes, R. T., Adams, A. S., Clinton, S. M., Pollack, I., et al. (2017). Promoting professional identity, motivation, and persistence: benefits of an informal mentoring program for female undergraduate students. PLoS One 12:e0187531. doi: 10.1371/journal.pone.0187531
Hoskins, S. G., Lopatto, D., and Stevens, L. M. (2011). The C.R.E.A.T.E. Approach to primary literature shifts undergraduates’ self-assessed ability to read and analyze journal articles, attitudes about science, and epistemological beliefs. LSE 10, 368–378. doi: 10.1187/cbe.11-03-0027
Hoskins, S. G., Stevens, L. M., and Nehm, R. H. (2007). Selective use of the primary literature transforms the classroom into a virtual laboratory. Genetics 176, 1381–1389. doi: 10.1534/genetics.107.071183
Howell, M. E., Booth, C. S., Sikich, S. M., Helikar, T., Roston, R. L., Couch, B. A., et al. (2019). Student understanding of DNA structure–function relationships improves from using 3D learning modules with dynamic 3D printed models. Biochem. Mol. Bio. Educ. 47, 303–317. doi: 10.1002/bmb.21234
Jittivadhna, K., Ruenwongsa, P., and Panijpan, B. (2010). Beyond textbook illustrations: hand-held models of ordered DNA and protein structures as 3D supplements to enhance student learning of helical biopolymers. Biochem. Mol. Bio. Educ. 38, 359–364. doi: 10.1002/bmb.20427
Kaas, J. H. (2019). “The origin and evolution of neocortex: From early mammals to modern humans” in Progress in brain research. eds. M. Raab, J. Johnson, and H. Heekeren (Amsterdam: Elsevier), 61–81.
Kamb, A., Iverson, L. E., and Tanouye, M. A. (1987). Molecular characterization of shaker, a Drosophila gene that encodes a potassium channel. Cell 50, 405–413. doi: 10.1016/0092-8674(87)90494-6
Katsioudi, G., and Kostareli, E. (2020). “Personal responses systems and active learning in biology: teaching epigenetics with a Sandwich-based model” in Presented at the 12th International Conference on Education and New Learning Technologies, Online Conference, 7587.
Kean, K. M., Van Zee, K., and Mehl, R. A. (2019). Unnatural chemical biology: research-based laboratory course utilizing genetic code expansion. J. Chem. Educ. 96, 66–74. doi: 10.1021/acs.jchemed.8b00011
Kennedy, S. (2013). Using case studies as a semester-long tool to teach neuroanatomy and structure-function relationships to undergraduates. J. Undergrad. Neurosci. Educ. 12, A18–A22
Kerchner, M., Hardwick, J. C., and Thornton, J. E. (2012). Identifying and using “core competencies” to help design and assess undergraduate neuroscience curricula. J. Undergrad. Neurosci. Educ. 11, A27–A37
Kowalski, J. R., Hoops, G. C., and Johnson, R. J. (2016). Implementation of a collaborative series of classroom-based undergraduate research experiences spanning chemical biology, biochemistry, and neurobiology. LSE 15:ar55. doi: 10.1187/cbe.16-02-0089
Lo, S. M., Luu, T. B., and Tran, J. (2020). A modified CREATE intervention improves student cognitive and affective outcomes in an upper-division genetics course. J. Microbiol. Biol. Educ. 21:70. doi: 10.1128/jmbe.v21i1.1881
Lopatto, D. (2007). Undergraduate research experiences support science career decisions and active learning. LSE 6, 297–306. doi: 10.1187/cbe.07-06-0039
O’Keeffe, G. W., and McCarthy, M. M. (2017). A case study in the use of primary literature in the context of authentic learning pedagogy in the undergraduate neuroscience classroom. J. Undergrad. Neurosci. Educ. 16, A14–A22
Petersen, C. I., Baepler, P., Beitz, A., Ching, P., Gorman, K. S., Neudauer, C. L., et al. (2020). The tyranny of content: “content coverage” as a barrier to evidence-based teaching approaches and ways to overcome it. LSE 19:ar17. doi: 10.1187/cbe.19-04-0079
Petrella, J. K., and Jung, A. P. (2008). Undergraduate research: importance, benefits, and challenges. Int. J. Exerc. Sci. 1, 91–95
Puelles, L., Sandoval, J. E., Ayad, A., Del Corral, R., Alonso, A., Ferran, J. L., et al. (2017). 1.26—The Pallium in reptiles and birds in the light of the updated tetrapartite pallium model. Evol. Nerv. Syst. 12, 519–555. doi: 10.1016/B978-0-12-804042-3.00014-2
Pugh-Bernard, A., and Kenyon, K. L. (2021). Mini-review: CREATE-ive use of primary literature in the science classroom. Neurosci. Lett. 742:135532. doi: 10.1016/j.neulet.2020.135532
Ramirez, J. J. (2020). Undergraduate neuroscience education: meeting the challenges of the 21st century. Neurosci. Lett. 739:135418. doi: 10.1016/j.neulet.2020.135418
Ramos, R. L., Fokas, G. J., Bhambri, A., Smith, P. T., Hallas, B. H., and Brumberg, J. C. (2011). Undergraduate neuroscience education in the U.S.: an analysis using data from the National Center for education statistics. J. Undergrad. Neurosci. Educ. 9, A66–A70
Rochon, C., Otazu, G., Kurtzer, I. L., Stout, R. F., and Ramos, R. L. (2019). Quantitative indicators of continued growth in undergraduate neuroscience education in the US. J. Undergrad. Neurosci. Educ. 18, A51–A56
Russell, S. H., Hancock, M. P., and McCullough, J. (2007). Benefits of undergraduate research experiences. Science 316, 548–549. doi: 10.1126/science.1140384
Sgoutas-Emch, S. A., Nagel, E., and Flynn, S. (2007). Correlates of performance in biological psychology: how can we help? J. Instr. Psychol. 34, 46–53.
Smith, J. L., and Thoman, D. B. (2024). Scaling up: lessons for persuading science faculty to adopt an evidence-based intervention. J. Coll. Sci. Teach. 53, 147–153. doi: 10.1080/0047231X.2024.2316388
Stanford, J. S., Rocheleau, S. E., Smith, K. P. W., and Mohan, J. (2017). Early undergraduate research experiences lead to similar learning gains for STEM and non-STEM undergraduates. Stud. High. Educ. 42, 115–129. doi: 10.1080/03075079.2015.1035248
Striedter, G. F. (2023). Incorporating evolution into neuroscience teaching. Front. Educ. 8:1278279. doi: 10.3389/feduc.2023.1278279
Sumter, T. F., and Owens, P. M. (2011). An approach to teaching general chemistry II that highlights the interdisciplinary nature of science. Biochem. Mol. Bio. Educ. 39, 110–116. doi: 10.1002/bmb.20465
Tempel, B. L., Papazian, D. M., Schwarz, T. L., Jan, Y. N., and Jan, L. Y. (1987). Sequence of a probable potassium channel component encoded at shaker locus of Drosophila. Science 237, 770–775. doi: 10.1126/science.2441471
Venkatesh, I., and Makky, K. (2020). Teaching epigenetic regulation of gene expression is critical in 21st-century science education: key concepts and teaching strategies. Am. Biol. Teach. 82, 372–380. doi: 10.1525/abt.2020.82.6.372
Weaver, G. C., Russell, C. B., and Wink, D. J. (2008). Inquiry-based and research-based laboratory pedagogies in undergraduate science. Nat. Chem. Biol. 4, 577–580. doi: 10.1038/nchembio1008-577
Wei, C. A., Beardsley, P. M., and Labov, J. B. (2012). Evolution education across the life sciences: making biology education make sense. LSE 11, 10–16. doi: 10.1187/cbe.11-12-0111
Wei, A., Covarrubias, M., Butler, A., Baker, K., Pak, M., and Salkoff, L. (1990). K + current diversity is produced by an extended gene family conserved in Drosophila and mouse. Science 248, 599–603. doi: 10.1126/science.2333511
Wiertelak, E. P., Hardwick, J., Kerchner, M., Parfitt, K., and Ramirez, J. J. (2018). The new blueprints: undergraduate neuroscience education in the twenty-first century. J. Undergrad. Neurosci. Educ. 16, A244–A251.
Wiertelak, E. P., and Ramirez, J. J. (2008). Undergraduate neuroscience education: blueprints for the 21(st) century. J. Undergrad. Neurosci. Educ. 6, A34–A39
Willard, A. M., and Brasier, D. J. (2014). Controversies in neuroscience: a literature-based course for first year undergraduates that improves scientific confidence while teaching concepts. J. Undergrad. Neurosci. Educ. 12, A159–A166
Yoho, R., Foster, T., Urban-Lurain, M., Merrill, J., and Haudek, K. C. (2019). Interdisciplinary insights from instructor interviews reconciling “structure and function” in biology, biochemistry, and chemistry through the context of enzyme binding. Discip. Interdscip. Sci. Educ. Res. 1:16. doi: 10.1186/s43031-019-0016-7
Zang, J., and Hammann, M. (2022). “Promoting students’ understanding of gene-environment interaction in genetics education” in Current research in biology education, contributions from biology education research. eds. K. Korfiatis and M. Grace (Cham: Springer International Publishing), 167–180.
Keywords: pedagogy, primarily undergraduate institutions (PUIs), curriculum development, interdisciplinary collaboration, biological principles, post-secondary education, STEM education, curriculum assessment
Citation: Stocker AM and Duncan CS (2024) Incorporating core concepts into an undergraduate neuroscience program in a resource-restricted environment. Front. Educ. 9:1454788. doi: 10.3389/feduc.2024.1454788
Received: 26 June 2024; Accepted: 05 August 2024;
Published: 15 August 2024.
Edited by:
Kimberley A. Phillips, Trinity University, United StatesReviewed by:
Barbara Lom, Davidson College, United StatesCopyright © 2024 Stocker and Duncan. This is an open-access article distributed under the terms of the Creative Commons Attribution License (CC BY). The use, distribution or reproduction in other forums is permitted, provided the original author(s) and the copyright owner(s) are credited and that the original publication in this journal is cited, in accordance with accepted academic practice. No use, distribution or reproduction is permitted which does not comply with these terms.
*Correspondence: Adam M. Stocker, c3RvY2tlckBtbnN0YXRlLmVkdQ==
Disclaimer: All claims expressed in this article are solely those of the authors and do not necessarily represent those of their affiliated organizations, or those of the publisher, the editors and the reviewers. Any product that may be evaluated in this article or claim that may be made by its manufacturer is not guaranteed or endorsed by the publisher.
Research integrity at Frontiers
Learn more about the work of our research integrity team to safeguard the quality of each article we publish.