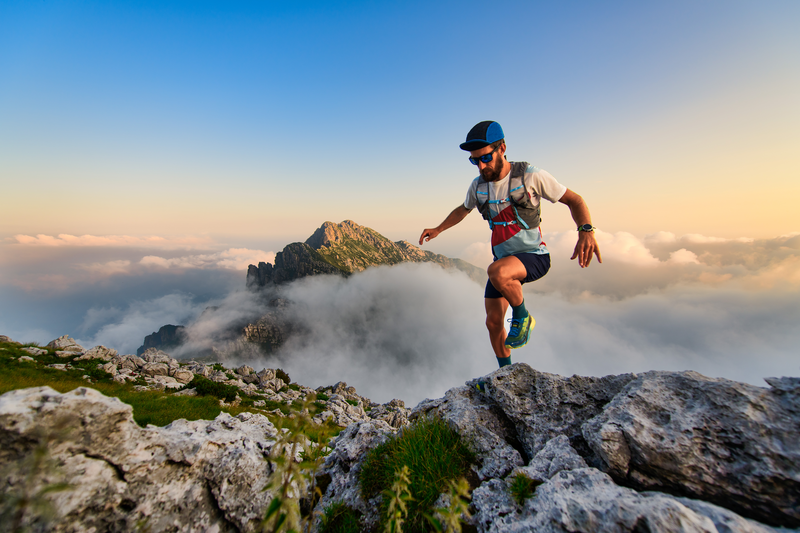
94% of researchers rate our articles as excellent or good
Learn more about the work of our research integrity team to safeguard the quality of each article we publish.
Find out more
ORIGINAL RESEARCH article
Front. Educ. , 05 December 2024
Sec. Higher Education
Volume 9 - 2024 | https://doi.org/10.3389/feduc.2024.1444810
Computational thinking represents an innovative pedagogical paradigm embraced by numerous academic institutions to cultivate the problem-solving acumen of students. Despite its widespread acceptance across diverse disciplines, the integration of computational thinking within medical education remains incomplete. This study seeks to ascertain the attitudes of medical university students in Saudi Arabia regarding computational thinking. Employing an exploratory research design, the investigation encompassed 394 health sciences students, transcending educational backgrounds and gender, with the objective of gaging their attitudes and awareness concerning the value, learning, and perceived difficulty associated with computational thinking within the context of medical curricula. The findings of the study indicate a notably positive disposition among students enrolled in medicine and health informatics programs toward computational thinking, surpassing the attitudes observed in other health disciplines. This discernible preference underscores the potential for integrating computational learning into medical curricula. The results further reveal a pronounced enthusiasm among students for computational thinking, suggesting a genuine interest in leveraging this approach for clinical problem-based learning. Consequently, it can be posited that the incorporation of computational thinking within medical education and clinical problem-solving scenarios warrants active encouragement in forthcoming educational initiatives.
Computational thinking (CT) has emerged as a contemporary educational paradigm, encompassing a set of skills imperative for effective problem-solving across diverse academic domains. Coined by Wing (2006), CT represents the amalgamation of critical thinking and computer science, evolving into a fundamental cognitive process amid the transformative influence of computers on various industries. This innovative approach entails the formulation of problems and their solutions in a manner conducive to execution by information processing agents (Cuny et al., 2010). Students’ cognizance of CT, its theoretical underpinnings, and their proficiency in its application bear significance, as it fosters systematic thinking and the utilization of CT tools as a framework for an enhanced repertoire of problem-solving skills.
This paper seeks to investigate the attitudes among medical university students regarding the importance and value of CT in their lives. Pioneering an exploratory research design, this study represents the first of its kind in the Saudi context, endeavoring to comprehensively collect and analyze students’ CT knowledge irrespective of their educational background and gender.
In the contemporary era characterized by data-driven paradigms and computational advancements, the extent of awareness among medical students regarding CT assumes particular significance. Notably, the absence of available Saudi data delineating the proficiency of students in computing and CT skills underscores the importance of this study. Proficiency in such skills empowers students to engage in broad and creative problem-solving endeavors, utilizing computational tools effectively. Consequently, this research serves as an initiative to introduce CT to the Saudi educational community, emphasizing its inherent value and elucidating the actual extent of students’ knowledge. In an epoch dominated by data-driven imperatives, the acquisition of computational skills, especially in problem-solving, becomes indispensable across academic fields.
The conceptualization of Computational Thinking (CT) was expanded by Wing (2006), marking a vital moment in educational discourse as her advocacy for the integration of CT into K-12 curricula garnered significant attention and prompted diverse initiatives across fields. The higher education domain has, in turn, exhibited a burgeoning interest in exploring the implications of CT for students’ learning. Wing (2006) posits CT as an indispensable skill applicable to individuals engaging in mental processes for problem-solving and seeking computational solutions. Contrary to a common assumption associating CT primarily with computer scientists, its relevance extends across diverse disciplines, including the sciences, humanities, and education. Positioned as a component of logical thinking repertoires, CT is perceived to exert a pronounced impact on students’ analytical capabilities (Agbo et al., 2023; Aryan and Shettar, 2023; Shin et al., 2021). Wing (2006) clarifies that the intent behind applying CT to various academic domains, particularly in education, is not to mold human thought in emulation of computers but rather to provide an innovative framework facilitating adept problem-solving.
The constituents of CT encompass algorithmic thinking, decomposition, abstraction, parallel thinking, and pattern-matching. Of these, abstraction assumes paramount significance, as emphasized by Wing (2011). Abstraction, in the context of CT, involves the strategic removal of extraneous details or complexities, directing attention to the essential features of an object, concept, or system. This foundational concept holds particular relevance in computer science and programming, facilitating the development of more efficient and maintainable code. The application of abstraction is instrumental in creating intricate systems and synthesizing information by generalizing from elementary components.
Various strategies exist for the integration of CT into educational frameworks. One approach involves infusing CT concepts into existing subjects and courses, such as incorporating them into math and science curricula to cultivate students’ adeptness in problem-solving. Alternatively, dedicated CT courses can be instituted to impart the requisite skills and competencies associated with CT. Such courses, offered across diverse academic fields, aim to equip students with the proficiencies essential for success in the digital age (Su and Yang, 2023; Triantafyllou et al., 2024; Chen et al., 2023).
The integration of CT into education holds the potential to exert a profound impact on students’ learning outcomes. A body of research has delved into the repercussions of CT instruction on learning outcomes, revealing enhancements in students’ creativity, problem-solving acumen, and collaborative skills. Furthermore, CT instruction has demonstrated the capacity to elevate student achievement in science and technology subjects, fostering improvements in critical thinking, creativity, and communication skills. These empirical investigations collectively underscore the substantial promise of CT in augmenting students’ learning outcomes across a spectrum of academic disciplines (Liu et al., 2023; Ye et al., 2023; Richardo et al., 2023).
CT holds the potential to enhance creativity among students by instilling a structured and systematic approach to problem-solving. CT instructs students to deconstruct intricate problems into more manageable components and to discern patterns and relationships within the elements of the problem. This methodological approach fosters the generation of novel and innovative solutions, enabling students to perceive connections and relationships that may elude them otherwise. Furthermore, CT cultivates critical thinking skills as students engage in a meticulous analysis of each step in the problem-solving process, discerning potential areas for improvement. The development of these skills equips students with the capability to confront complex problems adeptly, culminating in the formulation of creative and inventive solutions (Richardo et al., 2023; del Olmo-Muñoz et al., 2023; Zhang et al., 2024; Agbo et al., 2023).
Empirical evidence from several studies corroborates the affirmative impact of CT instruction on diverse learning outcomes, encompassing creativity, problem-solving acumen, collaboration skills, critical thinking, communication proficiency, and digital competencies. Key contributors to this body of research include Brennan and Resnick (2012), Van Der Linde et al. (2021), and Angeli (2021).
Brennan and Resnick's (2012) study, for instance, investigated the effects of CT instruction on high school students’ creativity, problem-solving, and collaboration skills. The study, conducted during a four-week summer program in the United States, encompassed 105 participants and involved comprehensive instruction on CT concepts such as algorithmic thinking, decomposition, abstraction, and pattern-matching. Additionally, hands-on activities were integrated to afford students practical experience in applying these skills. Pre-and post-program assessments were administered to gage students’ creativity, problem-solving, and collaboration skills.
Results from the study unveiled a significant augmentation in scores related to creativity, problem-solving, and collaboration among students who underwent the CT program compared to their non-participating counterparts. Notably, the CT program exerted a positive influence on students’ attitudes toward computer science, as evidenced by a substantial increase in the number of students expressing interest in pursuing computer science post-program. This empirical investigation thus contributes substantive evidence attesting to the efficacy of CT instruction in fostering enhanced creativity and other crucial learning outcomes among students.
Beyond student outcomes, scholarly inquiries have extended to the impact of CT on teacher education. For instance, the investigation conducted by Angeli (2021) demonstrated that CT instruction contributes to the enrichment of teachers’ pedagogical content knowledge and fosters the adoption of innovative teaching practices. These collective findings underscore the imperative of incorporating CT into teacher education programs, ensuring that educators acquire the requisite skills and competencies to effectively prepare students for the challenges posed by the digital age.
The integration of CT into health science education programs assumes critical significance, given the increasing data-centric nature of professions within the health sciences. These fields necessitate a heightened computational aptitude and a sophisticated level of thinking, aligning with the evolving landscape of healthcare systems reliant on data-driven decision-making processes. Such integration is essential for preparing future medical professionals to navigate and contribute meaningfully to the complexities of contemporary healthcare.
Musaeus et al. (2017) advocate for the integration of “Medical Computational Thinking” into medical education, emphasizing its potential to enhance problem-solving skills and deepen understanding of complex medical concepts as it is asserted in other research studies (Si et al., 2023; Si et al., 2021; Torregiani and Torregiani, 2023). The study, as a theoretical discussion, underscores the growing importance of computational thinking in healthcare, where data-driven approaches and technology are increasingly pivotal. Integrating computational thinking into medical curricula equips students to navigate expanding medical information landscapes and use technology effectively. Musaeus et al. (2017) propose dedicated courses where students can learn to analyze medical data, apply algorithms in decision-making, and devise innovative solutions to medical challenges. This inclusion empowers future healthcare professionals to make informed decisions, improve patient outcomes, and contribute to medical research and innovation. The study also highlights the broader benefits, including fostering critical thinking and multidimensional problem-solving, leading to more accurate diagnoses, personalized treatment plans, and enhanced healthcare delivery.
Expanding this line of inquiry, Law et al. (2019) conducted a comprehensive study exploring the role of computing in medical education and the imperative need to prepare medical students for the future. They assert that the transformative potential of technology in healthcare necessitates the adept incorporation of computer literacy skills among future physicians to ensure optimal patient care. Recognizing the inadequacies in the preparedness of aspiring doctors in computer literacy, the authors devised a 14-month Computing for Medicine certificate course (C4M), initiated at the University of Toronto. This course incorporates hands-on programming workshops, homework exercises, seminars by computer science experts contextualized within the medical domain, and coding projects. A qualitative study, utilizing purposive and maximal variation sampling, was conducted, involving interviews with 17 students who completed the course from April to May 2017. Thematic analysis, employing an iterative constant comparison approach, revealed that participants lauded the C4M as an avenue for achieving computer literacy, encompassing language, syntax, and fundamental computational concepts, with direct applicability to medicine.
Additionally, participants emphasized the enhancement of algorithmic and logical thinking skills vital for problem-solving. The course was commended for elucidating the intricate linkages between computer science and medicine, although participants acknowledged the potential divide between technology producers and users in the medical domain. Consequently, a recommendation emerged for fostering two-way communication between these disciplines during the development of medical technology. The study advocates for medical schools to recognize computer literacy as an indispensable skill, fostering collaborative computing partnerships for enhanced technology utilization by physicians and, consequently, optimal patient care. The authors advocate for ongoing evaluation of future iterations of the C4M and similar courses to continually refine and improve their effectiveness.
Collectively, these studies create compelling evidence of the transformative potential of CT in elevating medical students’ problem-solving abilities, diagnostic accuracy, and decision-making skills. The incorporation of CT into medical education emerges as a strategic imperative to equip students with the requisite skills and competencies essential for success in the contemporary digital age (Si et al., 2023; Torregiani and Torregiani, 2023; Si et al., 2021; Law et al., 2019; Musaeus et al., 2017).
The primary objective of this research study is to address several key inquiries pertaining to Saudi medical students’ attitudes toward CT. Specifically, the study endeavors to elucidate the following questions:
1. What are the prevailing attitudes of Saudi medical students toward CT, and what are the emergent categories characterizing these attitudes?
2. To what extent do these attitude categories rank among the students, and is there a discernible inclination toward one category over another?
3. Are there significant variations in the attitudes toward CT among students based on their health science major?
The research enlists the participation of 394 medical students enrolled in a Saudi university, where the curriculum predominantly comprises science and medical courses. Notably, these students undergo a computer science course during their sophomore year, emphasizing foundational skills for computer literacy. The curriculum of this course encompasses essential topics in computer science, including computer architecture, basics of computer networks, fundamentals of algorithms, basics of database management, and their applications in electronic health records. The course is designed to equip students with computational thinking principles derived from the domain of computer science, facilitating their application in solving medical problems through computer-based agents.
The study employs an exploratory survey crafted by Yadav et al. (2011) to gage the attitudes of medical students toward CT. This survey is administered to participants concurrently with their enrollment in the computer science course, with their written informed consent.
Following the submission of surveys by the students, the researchers conduct an exploratory factor analysis to distill the results into coherent categories, thereby eliminating extraneous survey items. To examine potential variations in attitudes toward CT based on students’ health science majors, statistical analyses including ANOVA tests and Tukey post-hoc tests are employed. These tests are crucial for elucidating any significant differences that may exist among students with varying health science majors.
To investigate the underlying structure of a computational thinking questionnaire, data collected from 394 participants were subjected to principal axis factoring with Promax rotation (kappa = 4; suppressing small coefficients of <0.4; Pituch et al., 2013). Please note that 354 participants remained after list-wise exclusion procedure.
Prior to running the principal axis factoring, we examined all the variables and found that the variables were reasonably normally distributed, although not every variable passed the Shapiro–Wilk test. Note that these deviations were not considered problematic, given the robust nature of factor analysis. Negatively worded items were reverse coded. An exploratory factor analysis was conducted on the 19 questionnaire items (3 questions assessing academic achievements and 16 questions to assess different aspects of computational thinking).
We found a high Kaiser-Meyer-Olkin (KMO) value of 0.87 suggested that the factor analysis could identify distinct and reliable factors. Bartlett’s Test of Sphericity was significant x2 (df = 171, N = 354) = 2099.89, p < 0.001, confirming the presence of significant correlations between some variables (Zygmont and Smith, 2014). The correlation matrix showed that every item had a correlation with at least one other above 0.3 (Tabachnick et al., 2007). Overall, the analysis revealed no problems with multicollinearity or singularity (i.e., all r’s < 0.85; Allen and Bennett, 2012) as examined in the correlation matrix. Multiple correlations within the anti-image matrices ranged between 0.619 and 0.942, exceeding the recommended threshold of 0.5 and confirming adequate sampling adequacy (Field, 2013).
Four factors (i.e., categories) were initially identified with eigenvalues greater than 1, accounting for 54.2% of the total variance, which was confirmed by the corresponding scree plot. However, three items were found to be problematic, failing to load to any of the factors. These items were removed, and the analysis was then rerun on the remaining 16 items. As results, we again observed four factors with eigenvalues greater than 1, consistent with the scree plot which also suggested a four-factor solution accounting for 58.35% of the total variance would be retained. Please refer to Table 1 for the full pattern matrix following Promax rotation.
Table 1. Pattern matrixa.
As can be seen from Table 1, category 1 comprised 5 items, with the highest factor loading item: “Think Computational Science (CS) is interesting.” This category was labeled “CT value” (i.e., the level of importance and enjoyment students have while solving problems using computers, desire to employ computational thinking approached in the future etc.). Category 2 included four items with the highest loading item: “Can achieve good grades in computational courses.” The second factor was labeled “CT learning,” as it was clearly associated with expectations of learning the use of computer science.
Category 3 consisted of three items with the highest factor loading: “SAAT,” followed by “GPA” and “GAT.” This category was labeled “Academic measurements” as all three items reflect academic performance and overall learning abilities. Finally, category 4 encompassed two items with the highest factor loading: “No requirement for computational skills” and “Not comfortable with computing concepts.” This category was labeled: “CT difficulty” since this category described to what extent students sense the difficulty of computer science (i.e., not comfortable with computer science).
The factor correlation matrix showed correlations up to r = 0.57, suggesting that the oblique [Promax] rotation was justified (Schmitt, 2011; see Table 2). Finally, note also that additional Chronbach’s alpha analyses across the four Factors revealed moderate to very good values (Factor 1 = 0.83, Factor 2 = 0.72, Factor 3 used Chronbach’s alpha based on standardized items due to scale differences in GPA vs. SAAT/GAT = 0.72, Factor 4 = 0.63). This implies acceptable internal consistency of items in each of the four observed factors.
In subsequent steps, we have directly contrasted participants’ responses in the three categories (CT value, CT learning and CT difficulty). For this purpose, we have averaged response scores separately for each of category so that each participant would have 1 value that represents his specific category score. Since each participant answered questions that were related to each of the categories, we used a repeated measures ANOVA design with within-group items with three levels. Note that Greenhouse–Geisser corrected values are reported whenever the sphericity assumptions were violated.
As a result, we found a significant main effect of categories [F(2, 754) = 337.68, p < 0.001, pEta2 = 0.47]. Subsequent pairwise comparisons revealed that there was a significantly higher expectations of CT learning (mean score = 3.36) relative to CT value [mean score = 2.96; t(377) = −16.35, p < 0.001]. Interestingly, there were higher levels of CT value (mean score = 2.25) relative to CT difficulty [t(377) = 13.98, p < 0.001] and higher levels of CT learning expectations relative to CT difficulty [t(380) = 22.42, p < 0.001].
Due to the fact that each category average consistent of different number of questionnaire items across all participants (i.e., CT value contained 7 questions, CT learning had 4 questions and CT difficulty only 2 questions) and due to violations of normality (K-S, all p’s < 0.001) we have additionally performed a non-parametric version of one-way ANOVA, the Friedman’s test. The test revealed a significant main effect of categories (X2 = 373.9, p < 0.001). Follow-up Wilcoxon signed-ranks test revealed a significant difference between expectations of CT learning and CT value (Z = −13, p < 0.001), CT value and CT difficulty (Z = −11.7, p < 0.001) and CT learning expectations vs. CT difficulty (Z = −14.73, p < 0.001). To conclude, it seems that participants could clearly see the learning of computational science in the near future and although there was some degree of negative attitude toward CT in the student population, there was even higher degree of value expressed toward CT.
Finally, we found a number of positive and negative correlations between the three categories (see Table 3).
Now, for analyzing the difference among students according to their health science major, we run ANOVA analysis and Tukey post-hoc test. We look at the three categories: “CT value,” “CT learning,” and “CT difficulty.” For the first category, CT value, there is 99% significant difference between respondents from the considered colleges. To find out which college students really differ on that category, we need to run multiple comparison table based on Tukey post-hoc test. It reveals that College of Public Health and Health informatics has a statistically higher value for CT value than for College of Medicine (p = 0.003) and for College of Dentistry (p = 0.010).
For the second category, “CT learning,” there is a significant difference among the colleges with 99% level of confidence. The College of Medicine (COM) has a more loaded positive attitude for CT learning than the other colleges.
For the third category, “CT difficulty,” even though it proves that with p < 0.05 the college type has a significant effect on this category, there are no significant differences between every possible pair of colleges.
The application of CT in the medical domain involves the exploration of computer-aided solutions to clinical challenges, requiring an evaluative framework to discern the value of such solutions in facilitating medical decision-making. This study delves into a comprehensive analysis of medical students’ attitudes regarding CT within the context of a Saudi University. Employing an exploratory survey as the primary instrument, the research aims to assess students’ awareness and attitudes toward computational thinking. Subsequently, an exploratory factor analysis is conducted to distill key insights into students’ perceptions, and an investigation is undertaken to identify potential variations in attitudes based on students’ health science majors.
The exploration focuses on three distinct categories, namely CT value, CT learning, and CT difficulty, each pivotal in understanding the nuanced dimensions of Saudi medical students’ awareness and attitudes toward CT. The study positions itself as an exploration into the attitudes of health-oriented students within the Saudi Arabian context, aspiring to influence educational decision-makers by advocating for the incorporation of principles from the Computer Science community into the curriculum for non-Computer Science students.
The outcomes of the study unveil a pronounced admiration and valuation of computational thinking principles among students, signaling a collective endorsement for their integration into educational curricula. In consonance with several studies (Agbo et al., 2023; Aryan and Shettar, 2023; Shin et al., 2021; Wing, 2011), the findings underscore students’ recognition that medical challenges can be effectively addressed through the application of CT principles, particularly in the generation of computer-based agents. The identified categories encapsulating students’ attitudes—CT value, CT learning, and CT difficulty—provide a nuanced understanding of the appeal, confidence, and perceived challenges associated with computational thinking.
Notably, students enrolled in the College of Medicine exhibit a significantly positive attitude toward computational thinking and learning in comparison to their counterparts in other colleges. This discernible trend underscores the potential applicability and relevance of computational thinking within a medical curriculum. The study further highlights a palpable interest among medical students in computer sciences, manifested through a voluntary willingness to engage in computing courses, as it is aligned with the other studies within the medical field (Si et al., 2023; Si et al., 2021; Law et al., 2019; Musaeus et al., 2017).
The exploratory survey encompasses diverse categories that collectively underscore the central role of computational thinking in medical education. The findings advocate for the inclusion of computational thinking and learning content within medical curricula, with a specific focus on cultivating competence in utilizing information technology to enhance patient care. The envisaged computational thinking and learning curricula in medical education, as proposed by this study, advocate for an initial focus on population health, emphasizing its potential impact on disease prediction, risk stratification, and management.
Pearson correlation results clearly indicated that students believed in more involvement of CT as a part of their medical education in their near future. Courses related to computational thinking and learning with a particular focus on public health and evidence-based medicine could initially be introduced for the students. Students’ awareness toward the computer sciences indicates that the CT will become an additional tool for the clinician to provide care.
Health informatics majors, engaged in the realms of data manipulation, analysis, and reporting, notably expressed a heightened affinity for CT, endorsing its integration into educational curricula. Conversely, medical majors exhibited the strong confidence in learning CT principles and their application to address medical challenges.
Remarkably, only two Saudi studies have delved into the role of CT in education so far but not yet in medical education, consistently converging on the conclusion that there is a significant interest among Saudi educators in utilizing CT for problem-based learning and improving students cognitive skills.
Alfayez and Lambert (2019) have raised concerns regarding the adequacy of computational thinking knowledge among computer science educators for effective subject instruction. To explore this matter, a quantitative research study involving 55 male computer science teachers in Riyadh, Saudi Arabia, was conducted. The outcomes of the investigation disclosed that a considerable proportion of the computer science teachers exhibited a diminished conceptual proficiency in various aspects of computational thinking, including problem-solving, algorithmic thinking, abstraction, and automation.
Additionally, a subset of these educators harbored misconceptions concerning the precise conceptualization of computational thinking. The findings underscored a discernible need for enhanced training among computer science teachers to augment their comprehension of computational thinking and to refine their pedagogical strategies for the effective dissemination of this subject matter.
Within the context of Saudi teachers’ low awareness of CT, Alyahya and Alotaibi (2019) focused in their study on how CT would help students to enhance their proficiency in mathematics. This research seeks to explore the interrelationships between CT and their corresponding outcomes in the context of mathematics tests conducted as part of the Trends in International Mathematics and Science Study (TIMSS). The study considers five dimensions of CT: creativity, algorithmic thinking, cooperativity, critical thinking, and problem-solving.
Recognizing the vital role of these cognitive skills and their impact on students’ academic outcomes would furnish educators with valuable insights for refining instructional methodologies and enhancing TIMSS achievement. The study, conducted with a cohort of 46 participants, exclusively comprising female students, revealed that heightened levels of CT significantly predicted superior mathematics performance in TIMSS. Specifically, problem-solving skills emerged as the most influential factor in test results, whereas creativity skills demonstrated the least impact.
The findings suggest that students would derive greater benefits from honing their problem-solving abilities rather than emphasizing critical thinking skills to excel in TIMSS assessments. This study’s positive impact is paramount in the realm of CT and its influence on mathematics education. By highlighting the crucial role of CT skills, particularly problem-solving, algorithmic thinking, and critical thinking, the findings urge educators to strategically integrate these skills into instructional practices. The identification of problem-solving skills as the most impactful contributor to TIMSS performance underscores CT as a catalyst for academic success. Moreover, the study’s focus on female students promotes gender-inclusive CT education, encouraging educators to foster these skills across diverse student populations. Overall, the research provides a solid foundation for integrating CT into curricula, ensuring that education aligns with the demands of a technologically advanced and problem-solving-oriented future.
Collectively Alfayez and Lambert (2019), Alyahya and Alotaibi (2019), and our study provide compelling evidence of the transformative potential of CT in fortifying students’ problem-solving competencies. However, as in our study supported by the other two studies, the incorporation of CT into medical education emerges as a strategic imperative, affording students the opportunity to cultivate the skills and competencies indispensable for success in the contemporary digital age and, consequently, effecting improvements in patient care.
The incorporation of medical computational thinking within the medical curriculum emerges as a potential avenue to educate medical students and trainee physicians in the intricacies of analyzing and designing complex healthcare organizations heavily reliant on computer technology, as highlighted by Musaeus et al. (2017). This educational initiative is envisioned to immerse students in information practices, fostering an understanding of computer technology’s direct involvement in patient care provision. Despite the established presence of computational thinking in bioinformatics and its impact on teaching and research, its penetration into the medical curriculum remains limited (Musaeus et al., 2017). The paucity of literature underscores the need for further research to delineate the fundamental principles that medical students should assimilate to develop a profound comprehension of computational thinking and its application in problem-solving within systemic healthcare and individual patient care contexts. The identified categories in this study serve as clear indicators for advocating the introduction of computer science courses into pre-medical and professional health programs in Saudi Arabia. While these categories provide valuable insights, future studies should elucidate the correlations between them.
Medical students dedicate a substantial portion of their education to understanding principles of clinical practice, problem-based learning, and patient care. Integrating computational thinking into their educational framework can synergize traditional healthcare approaches with innovative computer-aided thinking tools. The study’s exploratory survey, conducted among Saudi medical college students, irrespective of their educational background and gender, reveals a noteworthy attitude and enthusiasm toward computational thinking. The positive attitude observed among students suggests a receptiveness to the potential integration of computational thinking courses into future medical curricula. This shift toward a computing-based paradigm is indicative of a broader understanding of the principles inherent in computational thinking, thereby endowing healthcare practitioners with enhanced problem-solving skills aligned with contemporary technology (Saqr and Tedre, 2019). In summation, Saudi university medical students exhibit enthusiasm and a positive disposition toward the integration of computer sciences into their medical education, acknowledging the imperative need for computational thinking and advocating for its inclusion in forthcoming medical curricula.
The raw data supporting the conclusions of this article will be made available by the authors, without undue reservation.
The studies involving humans were approved by King Abdullah International Medical Research Center (KAIMRC). The studies were conducted in accordance with the local legislation and institutional requirements. The participants provided their written informed consent to participate in this study. Written informed consent was obtained from the individual(s) for the publication of any potentially identifiable images or data included in this article.
AA: Writing – original draft, Writing – review & editing. OA: Writing – original draft, Writing – review & editing.
The author(s) declare that financial support was received for the research, authorship, and/or publication of this article. This study was funded by KAIMRC for publication.
The authors declare that the research was conducted in the absence of any commercial or financial relationships that could be construed as a potential conflict of interest.
All claims expressed in this article are solely those of the authors and do not necessarily represent those of their affiliated organizations, or those of the publisher, the editors and the reviewers. Any product that may be evaluated in this article, or claim that may be made by its manufacturer, is not guaranteed or endorsed by the publisher.
Agbo, F. J., Olaleye, S. A., Bower, M., and Oyelere, S. S. (2023). Examining the relationships between students’ perceptions of technology, pedagogy, and cognition: the case of immersive virtual reality mini games to foster computational thinking in higher education. Smart Learn. Environ. 10:16. doi: 10.1186/s40561-023-00233-1
Alfayez, A. A., and Lambert, J. (2019). Exploring Saudi computer science teachers’ conceptual mastery level of computational thinking skills. Comput. Sch. 36, 143–166. doi: 10.1080/07380569.2019.1639593
Allen, P., and Bennett, K. (2012). SPSS statistics: A practical guide : Cengage Learning Australia Pty Ltd.
Alyahya, D. M., and Alotaibi, A. M. (2019). Computational thinking skills and its impact on TIMSS achievement: an instructional design approach. Issues Trends Learn. Technol. 7. doi: 10.2458/azu_itet_v7i1_alyahya
Angeli, C. (2021). “Examining the effects of scaffolded programming activities with robots on pre-service teachers’ computational thinking.” In Society for Information Technology & teacher education international conference (pp. 7–16). Association for the Advancement of Computing in Education (AACE).
Aryan, P. H., and Shettar, A. (2023). Effectiveness of computational thinking in problem-based learning. Journal of Engineering Education Transformations. Available at: https://journaleet.in/articles/effectiveness-of-computational-thinking-in-problem-based-learning
Brennan, K., and Resnick, M. (2012). “New frameworks for studying and assessing the development of computational thinking.” Paper presented at the annual meeting of the American Educational Research Association, Vancouver, Canada.
Chen, P., Yang, D., Metwally, A. H. S., Lavonen, J., and Wang, X. (2023). Fostering computational thinking through unplugged activities: a systematic literature review and meta-analysis. Int. J. STEM Educ. 10:47. doi: 10.1186/s40594-023-00434-7
Cuny, J., Snyder, L., and Wing, J. M. (2010). Demystifying computational thinking for non-computer scientists. Retrieved from http://www.cs.cmu.edu/CompThink/Resources/TheLinkWing.pdf
del Olmo-Muñoz, J., Bueno-Baquero, A., Cózar-Gutiérrez, R., and González-Calero, J. A. (2023). Exploring gamification approaches for enhancing computational thinking in young learners. Educ. Sci. 13:487. doi: 10.3390/educsci13050487
Law, M., Veinot, P., Campbell, J., Craig, M., and Mylopoulos, M. (2019). Computing for medicine: Can we prepare medical students for the future? Academic Medicine, 94, 353–357. Available at: https://journals.lww.com/academicmedicine/fulltext/2019/03000/computing_for_medicine__can_we_prepare_medical.26.aspx
Liu, S., Peng, C., and Srivastava, G. (2023). What influences computational thinking? A theoretical and empirical study based on the influence of learning engagement on computational thinking in higher education. Comput. Appl. Eng. Educ. 31, 1690–1704. doi: 10.1002/cae.22669
Musaeus, P., Tatar, D., and Rosen, M. (2017). “Medical computational thinking: computer scientific reasoning in the medical curriculum” in Emerging research, practice, and policy on computational thinking. eds. S. Rich, C. Hodges, and M. M. Cooper (Springer) 85–98.
Pituch, K. A., Whittaker, T. A., and Stevens, J. P. (2013). Intermediate statistics: A modern approach. (3rd ed.). Routledge.
Richardo, R., Dwiningrum, S. I. A., Wijaya, A., Retnawati, H., Wahyudi, A., Sholihah, D. A., et al. (2023). The impact of STEM attitudes and computational thinking on 21st-century via structural equation modelling. Int. J. Evaluation Res. Educ. 12, 571–578. doi: 10.11591/ijere.v12i2.24232
Saqr, M., and Tedre, M. (2019). Should we teach computational thinking and big data principles to medical students? Int. J. Health Sci. 13, 1–2. Available at: https://pmc.ncbi.nlm.nih.gov/articles/PMC6619459/
Schmitt, T. A. (2011). Current methodological considerations in exploratory and confirmatory factor analysis. J. Psychoeduc. Assess. 29, 304–321. doi: 10.1177/0734282911406653
Shin, N., Bowers, J., Krajcik, J., and Damelin, D. (2021). Promoting computational thinking through project-based learning. Discip. Interdiscip. Sci. Educ. Res. 3, 1–15. doi: 10.1186/s43031-021-00033-y
Si, J., Feng, H., Niu, Z., Bian, Y., and Fu, Y. (2021). “Training methods of computational thinking for medical students in big data age.” In 2021 16th international conference on Computer Science & Education (ICCSE) (pp. 13–20). IEEE.
Si, J., Huang, Y., Yang, L., Liu, Y., and Liu, J. (2023). Research of computational thinking empowering medical students, vol. 10133. EasyChair.
Su, J., and Yang, W. (2023). A systematic review of integrating computational thinking in early childhood education. Computers and Education Open, 4:100122. doi: 10.1016/j.caeo.2023.100122
Tabachnick, B. G., Fidell, L. S., and Ullman, J. B. (2007). Using multivariate statistics, vol. 5. Pearson Boston, MA.
Torregiani, E., and Torregiani, W. (2023). AI and computational thinking in medicine. Ir. Med. J. 116:784. Available at: https://imj.ie/ai-and-computational-thinking-in-medicine/
Triantafyllou, S. A., Sapounidis, T., and Farhaoui, Y. (2024). Gamification and computational thinking in education: a systematic literature review. Salud, Ciencia y Tecnologia-Serie de Conferencias 3:659. doi: 10.56294/sctconf2024659
Van Der Linde, D., Voogt, J., and van Aar, N. (2021). Computational thinking skills of young children working on a programming task. J. Comput. Math. Sci. Teach. 40, 357–376. Available at: https://www.researchgate.net/publication/356917169_Computational_Thinking_Skills_of_Young_Children_Working_on_a_Programming_Task
Yadav, A., Zhou, N., Mayfield, C., Hambrusch, S., and Korb, J. T. (2011). Introducing computational thinking in education courses. Proceed. 42nd ACM Technical Symposium on Computer Sci. Educ., 465–470. doi: 10.1145/1953163.1953297
Ye, H., Liang, B., Ng, O.-L., and Chai, C. S. (2023). Integration of computational thinking in K-12 mathematics education: A systematic review on CT-based mathematics instruction and student learning. Int. J. STEM Educ. 10. doi: 10.1186/s40594-023-00396-w
Zhang, W., Guan, Y., and Hu, Z. (2024). The efficacy of project-based learning in enhancing computational thinking among students: a meta-analysis of 31 experiments and quasi-experiments. Educ. Inf. Technol. 29, 14513–14545. doi: 10.1007/s10639-023-12392-2
Keywords: computational thinking, college education, medical education, computing literacy, college students
Citation: Althewini A and Alobud O (2024) Exploring medical students’ attitudes on computational thinking in a Saudi university: insights from a factor analysis study. Front. Educ. 9:1444810. doi: 10.3389/feduc.2024.1444810
Received: 06 June 2024; Accepted: 20 November 2024;
Published: 05 December 2024.
Edited by:
Natanael Karjanto, Sungkyunkwan University, Republic of KoreaReviewed by:
Ramadoni Ramadoni, National Research and Innovation Agency (BRIN), IndonesiaCopyright © 2024 Althewini and Alobud. This is an open-access article distributed under the terms of the Creative Commons Attribution License (CC BY). The use, distribution or reproduction in other forums is permitted, provided the original author(s) and the copyright owner(s) are credited and that the original publication in this journal is cited, in accordance with accepted academic practice. No use, distribution or reproduction is permitted which does not comply with these terms.
*Correspondence: Abdulaziz Althewini, YS5hbHRoZXdpbmlAZ21haWwuY29t
Disclaimer: All claims expressed in this article are solely those of the authors and do not necessarily represent those of their affiliated organizations, or those of the publisher, the editors and the reviewers. Any product that may be evaluated in this article or claim that may be made by its manufacturer is not guaranteed or endorsed by the publisher.
Research integrity at Frontiers
Learn more about the work of our research integrity team to safeguard the quality of each article we publish.