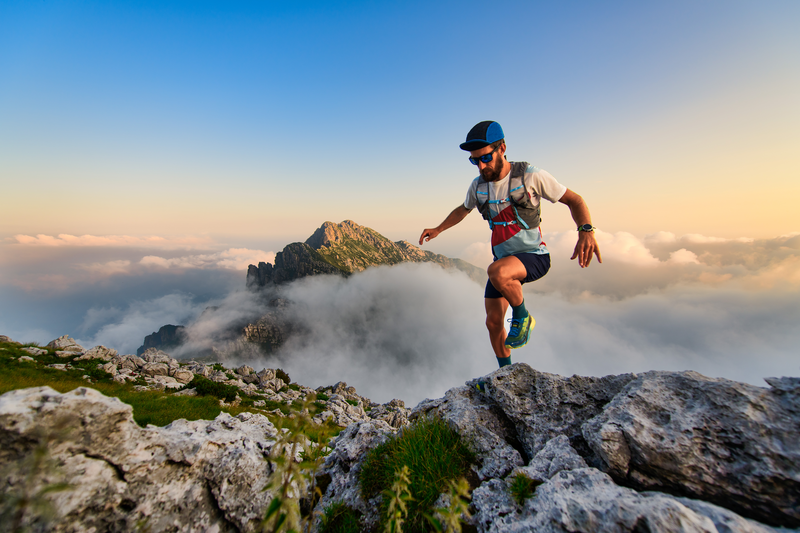
94% of researchers rate our articles as excellent or good
Learn more about the work of our research integrity team to safeguard the quality of each article we publish.
Find out more
CURRICULUM, INSTRUCTION, AND PEDAGOGY article
Front. Educ. , 13 December 2024
Sec. Teacher Education
Volume 9 - 2024 | https://doi.org/10.3389/feduc.2024.1437418
Neuroeducation, an emerging interdisciplinary field at the intersection of neuroscience and education, investigates into the complex processes underpinning learning which offering novel insights and methodologies. This article examines the foundational principles of neuroeducation, descriptive dynamic interplay between neuronal mechanisms and pedagogical strategies. Central to this exploration is an appreciation for the brain's remarkable plasticity and adaptability, highlighting how learning experiences sculpt neuronal networks. Utilizing advanced imaging techniques and rigorous neuroscientific research, a wealth of insights has emerged, enhancing our understanding of cognition, memory formation, and information processing. These insights enrich our comprehension of individual learning mechanisms and guide the development of effective educational interventions. Grounded in interdisciplinary collaboration, neuroeducation seamlessly integrates perspectives from neuroscience, psychology, and education to develop instructional approaches aligned with the brain's intrinsic learning processes. This collaboration nurtures, innovation in teaching methodologies, enhances student engagement, and bolsters academic achievement. Furthermore, the integration of technology into educational practices, ranging from brain-computer interfaces to immersive virtual reality experiences, presents new possibilities for enhancing learning engagements and accommodating diverse learning styles and curricula. Through the strategic use of technology's transformative power, educators can create immersive learning environments that stimulate cognitive processes and enhance knowledge retention. Neuroeducation emerges as a transformative force in educational theory and practice, empowering educators to design pedagogical approaches finely attuned to the complexities of the human brain, this paves the way for a more inclusive and effective educational landscape. This study is nested within a systematic review of the past 15 years of digital depository research and models of neuroeducational discourses, underscoring the field's evolving nature and its impact on contemporary education.
Neuroeducation or educational neuroscience an interdisciplinary field at the nexus of neuroscience, cognitive psychology, and educational theory, strives to transform educational practices by applying insights from neuronal dynamics and brain plasticity to the classroom. Neuroeducation pursues to infer laboratory research into effective pedagogical strategies, enhancing student engagement and learning outcomes. The neutral stance which cultivate critical thinking, enhance student engagement, and improve knowledge retention. Achieving this requires a thoughtful approach that integrates findings from neuroscience and cognitive psychology into teaching methodologies (Dubinsky et al., 2013; Mayer, 2017; Ramirez Butavand et al., 2020; Vogel et al., 2017). Neuroeducation, emerged in the educational practice in the late 1990s and early 2000s. Since the 1980s, brain-based education has emphasized aligning teaching practices with neurological functioning to enhance learning outcomes, early contributions highlighted the importance of understanding brain development, suggesting that neuroscience could significantly improve instructional strategies by addressing how students learn most effectively (Jensen et al., 2015). The initial stage, O'Reilly and McClure (2005) who explored the implications of neuroscience on pedagogical strategies, emphasizing the significance of brain plasticity in tailoring individualized learning experiences. Collectively, these studies illuminate how an informed approach to education, grounded in the understanding of brain function, can foster more effective instructional techniques and promote social-emotional learning, ultimately leading to a more nuanced and effective educational framework (Bruer, 1997; O'Reilly and McClure, 2005).
Recent advancements in neuroimaging, Functional Magnetic Resonance Imaging (fMRI), Positron Emission Tomography (PET), Electroencephalography (EEG) Magnetoencephalography (MEG), Near-Infrared Spectroscopy (NIRS), Diffusion Tensor Imaging (DTI) have significantly contributed to our understanding of how the brain processes information during learning. These innovative technological pulsates reveal the intricate workings of memory, attention, emotion, and other cognitive functions crucial for education. The neurobiological processes such as synaptic plasticity and dopaminergic signaling play vital roles in memory formation and retention, emphasizing the importance of integrating these findings into educational practices (Volpicelli and Miniaci, 2024; Dubinsky et al., 2013; Frei-Landau et al., 2023).
Neuroeducation which promote communication between behavioral science, neuroscience, and education, are all signs of this growing interest (Ansari et al., 2017; Thomas et al., 2019a,b; Beers et al., 2023). The scientific study of learning processes using concepts from cognitive psychology, computer science, social theory, neuroscience, and other fields. Since neuroeducation is interdisciplinary, researchers can examine intricate phenomena from several angles, leading to a more comprehensive knowledge of the complexities of learning and neuroeducation. This methodology enhances theoretical frameworks and fosters the creation of effective interventions that can cater to the varied requirements of students in different learning environments. Neuroeducation, sporadically misunderstood neuroeducation as narrowly focused on neural explanations for educational effectiveness, overlooking its broader interdisciplinary nature.
In the modern world the roles of neuroeducation is expanding, offering insights into cognitive processes like attention, memory, and learning, which can shape evidence-based educational practices. Research in neuroplasticity emphasizes that the brain's adaptability can support interventions for diverse learners, aiding those with learning disabilities in particular (Thomas et al., 2019a,b). However, advancements in educational science show that understanding the neural mechanisms of motivation and emotion enables educators to create more engaging and supportive learning environments (Linnenbrink and Pintrich, 2003; Immordino-Yang and Gotlieb, 2017). Despite these advances, experts urge caution, warning that direct application of neuroscientific findings to education may oversimplify complex educational needs (Howard-Jones et al., 2020). Despite, neuroeducation extended valuable insights, its application in education requires careful interpretation to avoid reductive approaches that may overlook the broader social and contextual factors influencing learning.
Neuroeducation, which encompasses diverse research, since the neuroimaging to psychological labs to classrooms, aiming to link neural processes with learning (Howard-Jones et al., 2016; Scarlini et al., 2020). The role of neural mechanisms in learning, it doesn't prioritize them exclusively or advocate for evaluating educational efficacy solely based on neural function (Howard-Jones et al., 2016; Yarullin and Serdyukov, 2019; Berg et al., 2021). At its juncture, teaching and learning are rooted in neurological processes driven by physical changes in brain, which understanding that learning and memory involve the modification of neural connections is recorded yearly but the technical intervention has been a difficult task. Despite, varying acceptance levels among educators and the public across countries, recent advancements in brain science have elucidated the molecular and cellular changes during learning, vital for memory formation (Takeuchi et al., 2014; Wilker et al., 2018; Takehara-Nishiuchi, 2020). The complex patterns of activity within the brain's neural networks, underlie cognitive processes crucial for learning, memory, and decision-making (Buzsaki and Tingley, 2018).
Innovative neuroscience method and its advancements have offered new levels of our understanding of neuronal mechanism, informing innovative teaching strategies etc. (Howard-Jones et al., 2014; Jaeggi and Shah, 2023). Kucian et al. (2011), utilized functional neuroimaging to assess the impact of mental number line training in children with developmental dyscalculia, finding significant post-intervention changes in brain activity that suggest targeted educational strategies can enhance numerical cognition. Similarly, specific training programs lead to observable neural adaptations associated with mathematical reasoning, highlighting the role of neuroimaging in education. Interdisciplinary collaborations among neuroscientists, psychologists, educators, and technologists have led to groundbreaking discoveries in this field (Wilcox et al., 2021; Ghosh and Sheu, 2008). The facilitating transformative advancements in education and beyond, investigating neuronal dynamics in the framework of learning and education pursues to proposition valuable insights for educators, moreover, well as address specific needs and challenges.
New educational progressive is skyrocketing, while neuroeducation presents promising contributions, applying its principles in education requires careful, evidence-based interpretation to avoid reductive approaches that neglect the broader social and contextual influences on learning (Bruer, 1997). Locating the innovative technological driven stance, neuroscience is increasingly influencing education, which illuminating key cognitive processes such as attention, memory, and learning, thereby supporting the development of evidence-based practices. Findings on neuroplasticity, for instance, suggest that the brain's adaptability can enhance interventions for diverse learners, including those with learning disabilities (Thomas et al., 2019a,b).
Insights into the neural mechanisms behind motivation and emotion also guide educators in creating supportive, engaging environments (Immordino-Yang and Gotlieb, 2017). However, experts warn that directly translating neuroscience into educational practice risks oversimplification, as complex neural processes don't neatly align with teaching methods (Ansari et al., 2012; Howard-Jones et al., 2020). The implementation of interdisciplinary pedagogy bridges disciplinary gaps and accommodates to diverse learning styles, substituting an enriched educational experience. This scientific inquiry aims to advance educational methodologies by equipping students with the adaptive skills essential for success in a rapidly evolving landscape, thus contributing to a progressive development index in the educational sciences.
Educational Neuroscience is a science of learning and teaching, the multidisciplinary arena that explores how the brain learns and develops with insights from education. The sophisticated educational and learning landscape helps to understand how environmental factors shape brain structure and function, affecting learning outcomes. Mind, Brain, and Education (MBE) is a core scientific discipline investigating the reciprocal relationship between education and brain function, exploring how interventions directing brain function can enhance learning (Ansari et al., 2017). Advancements in neuro imaging technology, have enabled researchers to observe brain activity in real-time (Jensen, 2008; Jolles and Jolles, 2021; Baier et al., 2021; Gvozdii et al., 2022). In the comprehensive perspective on the relationship between brain activity and learning methods, Researchers that have looked into this relation encompass Gardner (1983) and Robert and Geoffrey Caine, who propose the theory of multiple intelligences, and Caine and Caine's state how teaching strategies can be in line with brain processes added new knowledge to the field of educational neuroscience. Despite, teaching and learning research explore into the impact of various educational interventions on brain plasticity and cognitive development (Hassabis et al., 2017; Wu et al., 2021).
Certain teaching strategies, such as active learning and multisensory approaches, can enhance neural connectivity and promote vibrant learning practices (Howard-Jones, 2014). Novelty of neuroscientific insights open the design of educational interventions to individuals' cognitive profiles, leading to more personalized learning experiences (Jamaludin et al., 2019; Gola et al., 2022). In Europe, initially emerged as a collaboration between cognitive neuroscience and developmental psychology, further, expanded to encompass educational psychology and educational neuroscience, reflecting a broader integration of educational principles into brain research (Jensen and McConchie, 2020; Nouri and Tokuhama-Espinosa, 2018). The interdisciplinary approach has facilitated a deeper understanding of the complex interactions between brain function and educational practices, paving the way for more effective teaching strategies and interventions.
Neuroscientists and social scientists state, educational neuroscience is grounded in the concept of neuroplasticity, the brain's ability to reorganize and adapt based on learning and experiences. This framework supports individualized learning strategies that respond to the varying needs of students. Applying neuroscientific insights into how memory, attention, and emotions affect cognitive processes, educators can design learning environments that promote both cognitive and social-emotional development. Moreover, neuroeducation encourages teaching methods aligned with natural brain processes, resulting in not only academic but also emotional wellbeing for students (Howard-Jones, 2014; Nouri and Tokuhama-Espinosa, 2018). As the field evolves, it continues to emphasize the importance of brain-based learning, ensuring that educational practices reflect current understandings of brain function and development.
The potentiality of educational neuroscience research has the potential to be used in education by applying pedagogical principles and gaining insights into how learning environments, development, and internal and external factors affect learning interact (Thomas et al., 2019a,b; Jolles and Jolles, 2021). Educators, encompassing both parents and teachers, play pivotal roles as facilitators of the neural changes underlying learning which provide the context, stimuli, social interactions, role models, and values that shape the learning process, despite often lacking comprehensive understanding of brain functioning (Owens and Tanner, 2017; Gola et al., 2022). Hence, there exists a pivotal contribution for ongoing dialogue between neuroscience and education, centered around understanding the mechanisms of learning, the involvement of mental functions, sensitive periods in development, correlations between cognition, emotion, motivation, and performance, as well as the potentials and limitations of the nervous system in the learning environment (Recio and Rodriguez-Moreno, 2023; Jamaludin et al., 2019).
The increasing momentum and interest in neuroscience-informed teaching and learning methods underscore the necessity of translating neuroscience findings into accessible information for educators. In contrast the diversification of teaching strategies to accommodate individual learning needs emerges as a crucial skill for educators to cultivate (Pitts et al., 2009; Thomas et al., 2018; Jolles and Jolles, 2021; Cui and Zhang, 2021). Consequently, the significance of brain literacy training in teacher education becomes evident and high latitude in innovative stand. In order to ensure that research findings are relevant and applicable in real-world educational settings, three crucial factors regarding the practical application of educational neuroscience in classrooms are emphasized: first, translating complex neuroscientific findings into strategies that educators can easily understand and implement; second, incorporating neuroscience-informed practices into pre-existing educational frameworks without compromising pedagogical principles; and third, encouraging collaboration between neuroscientists and educators. A successive approach to facilitating meaningful and sustainable advancements in classroom instruction and learning requires the adept dissemination of neuroscience discoveries.
Over the past 5 years, research in cognitive development and brain-based learning has underscored the value of persuading neuroplasticity, emotional engagement, metacognitive practices, and social learning strategies to enhance educational experiences. Neuroplasticity the brain's ability to form and reorganize connections enables students to solidify new knowledge when actively engaged in learning activities, such as problem-solving and discussions, which reinforce neural pathways. Additionally, memory aids, including visual tools and storytelling, help in connecting new concepts to prior knowledge, effectively utilizing the brain's natural information-processing mechanisms (Tokuhama-Espinosa, 2015; Boon, 2024; Connell, 2009). Emotional factors are also critical, as positive, low-stress environments enhance cognitive function, allowing brain areas like the amygdala and hippocampus to process and store information more effectively. Techniques such as mindfulness, emotional support, and relevant learning materials promote retention by fostering a secure learning atmosphere (Saleh and Mazlan, 2019; Triana et al., 2019).
Metacognitive and collaborative approaches eloquently reinforce cognitive growth and autonomous learning. These strategies encourage self-reflection and goal-setting, fostering autonomy by helping students to evaluate and adjust their learning techniques. When integrated with brain-based learning methods, these strategies increase retention and performance by aligning students' actions with their cognitive processes (Shaywitz, 2003; Papaleontiou-Louca, 2019). Classroom practices that include movement-based activities, real-world examples, and timely feedback align with how the brain learns best, making these methods effective tools for boosting cognitive function and addressing misconceptions. These insights, supported by emerging technology like AI and neuroimaging, promise further customization of learning based on individual cognitive profiles, offering a future where neuroscience-informed strategies can maximize educational outcomes across diverse learning environments (Winsler, 2003; Boon, 2024; Holmes and Porayska-Pomsta, 2022).
The purpose of creating instructional procedures that complement student's cognitive capacities, it is essential to comprehend the stages of cognitive growth. Four phases of cognitive developments, each of which denotes a change in cognitive capacities and mental processes (Rabindran and Madanagopal, 2020). The Zone of Proximal Development (ZPD), where students can reach higher levels of comprehension with the right support, is a key component of Lev Vygotsky's social development theory, which highlights the importance of language and social interaction in cognitive development. Learning requires practice with memory and retrieval, and techniques including reducing cognitive load and encouraging pupils to retrieve knowledge from memory have been found to improve long-term retention. Chunking material into digestible chunks can help with attention and focus, and mindfulness exercises have been shown to increase student's prolonged participation in educational activities (Seghier et al., 2019; Filipe et al., 2021; Delavari et al., 2023). Additionally, important factors in student motivation and comprehension are emotional engagement and positive reinforcement. Improved understanding and learning significances are supported by the use of individualized instruction, which adapts teaching strategies to each student's unique learning preferences and styles (She, 2023; Hasnine et al., 2023). Multiple modalities are engaged in multisensory learning processes, which aid in memory retention. While regular physical activity and adequate sleep are crucial for cognitive function and general wellbeing, the incorporation of digital literacy and technology into education provides opportunities for individualized learning and the acquisition of critical skills for navigating the modern world.
The role of neuronal dynamics in educational settings, proving how brain plasticity influences learning strategies and cognitive engagement. Insights into synaptic changes during classroom interactions can inform teaching methods, enhancing student outcomes (Garcia and Thompson, 2022; Miller and Cohen, 2023). The crucial role of neuronal dynamics in educational environments, where understanding brain plasticity can significantly enhance teaching and learning strategies. Synaptic changes occur in response to active engagement and meaningful interactions, suggesting that pedagogical approaches that promote collaboration and critical thinking can optimize learning outcomes (Johnson, 2024). Furthermore, neuroeducation emphasizes the importance of tailoring instruction to align with knowledge processes, leveraging insights from neuroscience to create effective curricula (Garcia and Thompson, 2022). For instance, techniques that integrate multimodal learning experiences can facilitate deeper understanding by activating various neural pathways, nurturing retention and application of knowledge (Miller and Cohen, 2023). Neuronal dynamics, particularly synaptic changes, are crucial in educational settings, and through understanding brain plasticity, educators can modify teaching strategies to boost cognitive engagement and enhance learning outcomes.
The intricate processes of neurobiological changes within the brain as it acquires new information, the process begins with specific patterns of neuronal activation in response to stimuli or information presented during the learning experience (Fandakova and Hartley, 2020). The patterns of activation are dependent on the nature of the learning task and the sensory modalities engaged, such as visual or auditory channels. This initial response sets the stage for further modifications in the brain's neural circuitry. Following neuronal activation, synaptic connections undergo changes in strength and efficiency. This act of efficacy adducted process of synaptic plasticity, is a key mechanism underlying learning and memory (Mateos-Aparicio and Rodriguez-Moreno, 2020). Despite, the Long-term potentiation (LTP) is one such modification where repeated stimulation of a synapse results in a strengthening of the connection, enhancing signal transmission and contributing to the consolidation of new knowledge. In contrast, long-term depression (LTD) refers to the weakening of synaptic connections, which can help prune unnecessary pathways and refine neural circuits for more efficient processing (Stuchlik, 2014; Baek, 2016). These alterations in synaptic connectivity led to the reorganization of neural networks, which play a crucial role in learning.
In addition to synaptic changes, neurotransmitter release is another important component of neuronal dynamics during learning, various neurotransmitters, such as dopamine, play specific roles in modulating the learning process and are involved in reward-based learning and motivation (Baier et al., 2021; Kennedy, 2016). Subsequent, the learning stance, the brain consolidates new information into long-term memory through a series of complex processes that involve the strengthening and stabilization of neural circuits. These processes, which can occur during sleep and rest periods, enable the retention of learned material over time. Understanding these interconnected processes of neuronal dynamics during learning provides valuable insights for educational strategies that align with the brain's natural capabilities, ultimately enhancing teaching and learning outcomes.
This Figure 1 illustrates the process of synaptic plasticity during learning, neuronal activation in response to stimuli leads to changes in synaptic strength, including long-term potentiation (LTP) and long-term depression (LTD), which contribute to the reorganization of neural networks and the consolidation of new knowledge.
This Figure 2 depicts the role of neurotransmitter release, such as dopamine, in modulating the learning process. Neurotransmitters play a crucial role in reward-based learning and motivation, contributing to the consolidation of new information into long-term memory.
Synaptic plasticity, the brain's ability to modify the strength of synaptic connections in response to experience and learning, is a critical mechanism underpinning neuroeducation. This adaptability, which also includes processes like long-term potentiation (LTP) and long-term depression (LTD), enables the brain to encode and store memories and adapt cognitive pathways. Neuroeducation leverages these insights to develop individualized learning strategies that align with how the brain naturally learns and retains information. Through the integration of cognitive, emotional, and social dimensions, educators can enhance both academic performance and emotional wellbeing.
However, translating neuroscientific findings into classroom practice remains a challenge, requiring interdisciplinary collaboration and ethical considerations, especially regarding the use of adaptive technologies and neuroimaging (Mateos-Aparicio and Rodriguez-Moreno, 2020; Howard-Jones, 2014; Nouri and Tokuhama-Espinosa, 2018). The synaptic plasticity enables flexible learning, neuroeducation aims to harness this potential by creating adaptive learning environments that respond to students' cognitive and emotional needs. Howard-Jones (2014) critiques the application of neuroscience in education, particularly addressing neuromyths misconceptions such as left-brained vs. right-brained learning styles which can mislead educational practices. He argues for the need to bridge gaps between neuroscience and education with accurate, evidence-based science communication to avoid reductive interpretations that weaken teaching strategies (Howard-Jones, 2014). Ansari et al. (2012) similarly caution against overgeneralizing neuroscientific findings, emphasizing the complexity of translating brain-based research into practice due to the nuanced and individualized nature of cognitive processes. However, careful, context-aware integration of neuroscience is essential for genuinely effective educational innovation.
The importance of neuroplasticity in education has become increasingly recognized in recent years (Tandon and Singh, 2016). Educators, neuroscientists, and behavioral scientists all play important roles in exploring the relationship between brain plasticity and education. Neuroplasticity, the brain's ability to change and adapt, has significant implications for education, suggesting that learning is not fixed or predetermined but rather influenced by a person's biological makeup and environment (Tandon and Singh, 2016; Jolles and Jolles, 2021). This understanding of neuroplasticity has led to a shift in educational approaches, emphasizing the need to create enriched environments that support neural mechanism development and learning.
Educational practices that incorporate strategies to enhance neuroplasticity have shown promising results in promoting student engagement, academic achievement, and overall cognitive development. Despite the brain's ability to change and adapt, which plays a crucial role in education (Mishra and Gazzaley, 2014; Tandon and Singh, 2016), the importance of executive functions in cognitive learning, social behavior, emotional processing, and personal growth is paramount (Jolles and Jolles, 2021). Developing these executive functions is crucial for students to effectively navigate and succeed in the educational setting. With a focus on pedagogical inspiration and support, the field of neuroeducation seeks to apply knowledge about brain plasticity to improve teaching practices.
Locating the concept of neuroplasticity, educators can incorporate their teaching methods and create environments that support brain development and learning (Mateos-Aparicio and Rodriguez-Moreno, 2020). Neuroplasticity also challenges the notion that individuals have fixed abilities or limitations. Mateos-Aparicio who suggests that with the right support and opportunities for growth, individuals can continue to learn and develop throughout their lives. This recognition of neuroplasticity in education has led to a deeper understanding of the dynamic nature of the brain and the potential for growth and change. It is now recognized that neuroplasticity plays a crucial role in education (Voss et al., 2017). This recognition of neuroplasticity in education has led to a shift in educational approaches, with an emphasis on creating enriched environments that support cognitive development.
According to contemporary neuroscience research underscore, learning induces synaptic plasticity, whereby connections between neurons strengthen, weaken, or reorganize in response to experiences and information processing, essentially constituting the neural manifestation of learning (Acosta et al., 2022; Takeuchi et al., 2021; Kandel, 2021). This synaptic remodeling reflects learning as it involves lasting changes in neural circuits that support memory consolidation and skill acquisition, thus positioning neural changes as both results of and fundamental components of the learning process.
The recognition of neuroplasticity in education has led to a shift toward creating enriched environments that support cognitive development, emphasizing the importance of executive functions, and promoting lifelong learning. This shift has resulted in improved educational practices that substitute student engagement, academic achievement, and overall cognitive development. Through the understanding and harnessing neuroplasticity, educators can unlock the full potential of their students and create a more dynamic and effective learning environment. Despite this, the recognition of neuroplasticity in education has revolutionized teaching practices and emphasized the importance of executive functions in cognitive learning, and social behavior.
Teacher training in neuroeducation is of utmost importance to ensure that educators have the necessary knowledge and skills to incorporate neuroscientific principles into their teaching practices. Effective implementation of neuroeducation requires educators to have a basic understanding of the principles and concepts that underlie brain function and learning processes. This includes knowledge about neuronal dynamics, brain plasticity, cognitive development, and the influence of social and emotional factors on learning (Ansari et al., 2012; Thomas et al., 2019b). The interdisciplinary pedagogical approaches in neuroeducation also emphasize the need for personalized and adaptable teaching methods. Tracing from multiple disciplines, educators can adopt their approaches to meet the individual needs and learning styles of their students (Jolles and Jolles, 2021; Nievas and Montes, 2023). The result of an inclusive and adaptable approach supports the creation of a more engaging and effective learning environment.
Advancing progressive educational innovation and its Intellectual foundations, neuroscience continues to unveil new insights into the complexities of the brain, the field of neuroeducation offers an evolving landscape for educators to enhance their teaching practices (Ansari et al., 2012; Owens and Tanner, 2017; Dubinsky et al., 2013; Ghanbari et al., 2019). While exploring the multidimensional understanding of how the brain learns and integrating these findings into pedagogical approaches, educators can foster a more comprehensive and impactful learning experience for their students. Incorporating Neuroeducational principles into teaching practices can lead to more engaging and impactful learning experiences for students. Employing this interdisciplinary approach, educators can tap into the full potential of both neuroscience and pedagogy to create a more effective learning environment.
Neuroeducation underscore the importance of metacognition in the learning process, through helping students become aware of their thinking processes and learning strategies, educators can empower them to take ownership of their learning and develop effective problem-solving skills. It is critical to acknowledge the significance of teacher preparation in neuroeducation for the purpose of satisfying the demands of contemporary education and guarantee effective teaching and learning. Although there are benefits to using traditional teaching approaches, incorporating neuroeducation into teacher preparation programs can significantly improve the efficacy of instructional strategies and have a beneficial effect on student learning outcomes (Herzberg, 2023).
The logical understanding of how the brain absorbs and processes information can significantly enhance teachers' effectiveness. Despite this understanding, educators efficiently design learning tactics that complement the way the brain works, which will increase student engagement and achievement. Moreover, neuroeducation can give educators can also benefit educators to providing them with tools and techniques to enhance critical thinking and problem-solving skills in students. The integration of neuroeducation into teacher training programs represents a paradigm shift in pedagogical approaches, enabling educators to leverage insights from neuroscience to enhance teaching and learning outcomes. Traditional teacher training often prioritizes content delivery and general pedagogical strategies without a comprehensive focus on how the brain processes and retains information (Cui and Zhang, 2021; Jolles and Jolles, 2021). As a result, while foundational skills are established, educators may lack the tools necessary to tailor instruction to the diverse cognitive profiles of their students.
Research has shown that incorporating neuroeducation into teacher training can lead to more effective teaching practices and improved student outcomes. Professional development initiatives that introduce teachers to neuroeducation concepts such as cognitive load theory and memory retrieval practices have been found to provide educators with actionable strategies for optimizing learning experiences. For instance, training in the application of spaced repetition and interleaved practice has been shown to enhance long-term memory retention and facilitate deeper learning (Carpenter et al., 2022). Advancements in neuroeducation also include the use of adaptive learning technologies, which can customize instructional approaches based on real-time data regarding students' performance and cognitive abilities (Ghanbari et al., 2019).
Furthermore, mindfulness training for both students and teachers has demonstrated benefits in terms of focus and emotional regulation, contributing to a more supportive learning environment. Similarly, training in social-emotional learning (SEL) strategies has been associated with fostering positive interpersonal relationships and increasing student engagement (Escolano-Pérez et al., 2020; Greenberg, 2023). Despite, to integrating these evidence-based techniques into teacher training, educators can more effectively address the varied cognitive and emotional needs of their students, leading to enhanced academic achievement and overall wellbeing.
In the realm of educational research, various pedagogical techniques have been developed and implemented to enhance student engagement, collaboration, and learning process. Emplacement the foundation of Neuroeducational practices, the think–pair–share activity serves the ultimate purpose of encoding memories within synaptic connections and neural circuits. Ideally, this process has altered the connections between neurons in students' brains, enabling them to recall the solution to the task months later, and potentially for the rest of their lives (Owens and Tanner, 2017). Peer Teaching, wherein students take on the role of instructors to teach their peers concepts or solutions to problems. This method not only reinforces the understanding of the material for the student-teacher but also fosters collaboration and peer learning within the classroom (Polkowski et al., 2020). Through the process of explaining concepts to others, students deepen their comprehension and gain valuable communication skills, while also benefiting from diverse perspectives and approaches shared by their peers. This strategy makes use of social interaction to improve understanding and memory of the material, which is consistent with studies showing how crucial social learning contexts are for maximizing brain plasticity.
The Jigsaw technique further exemplifies the power of collaboration by dividing students into small groups, each specializing in a specific topic, before sharing their expertise with the larger class (Clark and Dumas, 2015; Drouet et al., 2023). This method promotes collaboration and shared learning, facilitating the exchange of diverse perspectives and encouraging active engagement with course material (Owens and Tanner, 2017; Nathaniel et al., 2018). Similarly, round-robin brainstorming ensures equitable participation from all students, creating an inclusive learning environment where ideas are freely exchanged and built upon, fostering a sense of collective ownership over the learning process. Through teaching others, students reinforce their understanding of the material and develop valuable presentation and communication skills essential for academic and professional success.
Educational neuroscience often experiences with different stimuli, including brain stimulation techniques, to enhance learning, as seen in studies on transcranial electric stimulation (tES) and its impact on cognitive functions like arithmetic learning. Schroeder et al. (2017) suggest that tES can support learning by influencing neural plasticity, while Cohen Kadosh et al. (2015) and Fregni et al. (2005) observe improvements in neural efficiency and connectivity. However, although tES may aid learning conditions, genuine neural reorganization reflects active learning processes. Integrating these techniques can foster critical thinking, communication, and engagement, enriching interactive learning environments.
The unique strategy employed to facilitate active participation and idea generation among students. In this structured format, students take turns sharing ideas or solutions, ensuring that all students have the opportunity to contribute to the discussion (Aini M., 2023; Aini D., 2023). These all technique not only encourages participation from all students but also promotes critical thinking and the synthesis of diverse perspectives, which also effective support to building on each other's contributions, students deepen their understanding of the topic and develop collaborative problem-solving skills. This innovative approach to educational dynamics represents a significant advancement in curriculum development for developing countries. It fosters a vibrant and progressive educational strategy, aiming to enrich and modernize learning experiences and outcomes on a broad scale.
Educational Situation Quality Model (MOCSE), proposed by Domenech, aims to elucidate the functioning of formal educational situations by organizing key variables involved in school learning and their relationships. It adopts a systemic perspective, analyzing various levels of educational processes, from short-term instructional segments to long-term courses. The model's key components are the pre-actional decisional phase, Actional phase, and Reflectional phase (Domenech-Betoret et al., 2020). The MOCSE model, developed by Domenech, provides a comprehensive framework for understanding the dynamics of formal educational situations which offers a systemic approach that examines the different levels of educational processes and their interrelationships.
In the Neuroeducational context, MOCSE incorporates the Action-Control Theory and the Process Model of Student Motivation to explain motivational phases in learning. The Action-Control Theory outlines pre-decisional and post-decisional phases, while the Process Model specifies motivation as pre-actional, actional, and post-actional (Doménech-Betoret et al., 2019; Domenech-Betoret et al., 2020). MOCSE provides a framework to understand educational dynamics, using a systemic approach that examines educational levels, their interrelations, and the role of student motivation, intent, and support. Its core components include the Appraisal Stage, Intention Activation, Teaching-Learning Process, and Product.
The MOCSE model and principles of neuroeducation and neuroplasticity creates a comprehensive approach to enhance educational practices to our understanding of how the brain learns to design more effective, engaging, and personalized learning experiences shown in Figure 3. Incorporating principles of neuroeducation and neuroplasticity into the MOCSE model enhances our understanding of how the brain learns and adapts in educational settings. Recognizing the brain's plastic nature emphasizes the importance of providing diverse and engaging learning experiences to facilitate neural connections and learning. Educators can leverage neuroplasticity through incorporating varied instructional strategies, multisensory experiences, and active learning approaches to stimulate brain development and enhance learning retention. Utilizing these perspectives in curriculum designing involves structuring learning experiences that align with brain-based learning strategies, such as scaffolded learning experiences, spaced repetition, and opportunities for retrieval practice.
Figure 3. MOCSE diagram: main course sequence actions for students. Connected with students' interests, needs, and academic level (meaningful demands, Doménech-Betoret, 2018).
Integration into online learning platforms can further leverage neuroeducation insights to design user-friendly interfaces, interactive multimedia content, and adaptive learning pathways. Incorporating features such as spaced repetition algorithms, personalized learning recommendations, and gamified activities enhances engagement and promotes effective learning (Howard-Jones et al., 2009; Dunlosky and Rawson, 2019). Similarly, multimedia resources can be designed to appeal to various learning styles and preferences, catering to visual, auditory, and kinaesthetic learners. Integrating assessment features within multimedia resources facilitates active learning and promotes self-directed learning behaviors. Finally, adaptive technologies leverage neuroplasticity extended dynamically adjusting learning experiences based on individual student needs and performance, fostering a supportive learning environment that accommodates diverse learning profiles and promotes equity and inclusion.
Our stance underscores the importance of a multifaceted approach to integrating neuroeducation and neuroplasticity into educational practices substitute beyond the utilization of technology, we emphasize the paramount significance of prioritizing social-emotional learning and cultivating nurturing learning environments that foster student wellbeing and intrinsic motivation. Attending to the holistic development of students, educators can create conducive spaces for learning where cognitive, emotional, and social dimensions are seamlessly integrated, optimizing the conditions for meaningful and enduring learning experiences. The collaboration among educators, researchers, and technology developers stands as a cornerstone in the advancement of evidence-based practices rooted in neuroscience. Encouragement interdisciplinary dialogue and partnership, we can collectively refine instructional strategies, leverage emerging technologies, and translate neuroscience research into actionable insights for educational settings. This collaborative effort paves the way for innovative approaches to teaching and learning that are responsive to the evolving needs and capacities of learners. Moving forward, continuous professional development opportunities and ongoing interdisciplinary collaboration will empower educators to adaptively implement brain-based teaching practices, ensuring that education remains at the forefront of leveraging neuroscience for the betterment of student learning outcomes and holistic development. The implementation of these collaborative learning strategies within the framework of neuroeducation, educators can create dynamic and engaging learning experiences that optimize neural functioning and promote student success and progressive well beings.
The assimilation of Neuroeducational concepts profoundly enhances traditional teaching methodologies, which often emphasize information delivery and broad pedagogical approaches. Locating the scientific insights into the brain's mechanisms for learning and information processing, educators who create effective and supportive learning environments. Neuroeducation empowers teachers with evidence-based practices, such as memory retrieval techniques, cognitive load management, and adaptive learning technologies, to maximize student learning potential these advancements offer the promise of transformative outcomes in education. Neuroscientific research provides a sophisticated framework that not only complements but also enhances conventional teaching methods which offering scientific understanding of cognitive development and learning processes. Neuroscience-centric approaches enable educators to tailor instruction to meet the diverse needs of students, fostering deeper learning and improved retention. Innovations in neuroeducation facilitate the development of personalized learning experiences through artificial intelligence and machine learning, and they encourage ongoing exploration of the effects of neurodiversity on teaching and learning. Ultimately, neuroeducation presents a unique opportunity to impart knowledge and develop adaptive skills in both learners and future educators. Locating the integrity of Educational Salutation Quality Model (MOCS) and other integrating with advanced insights into educational practice, we can design pedagogical strategies that are finely attuned to the complexities of the human brain, paving the way for a more intrinsic, inclusive and effective educational plethora. Furthermore, neuroeducation addresses diverse learning needs, promoting inclusive practices that cater to all students. Ultimately, integrating neuroscience findings into educational practices leads to improved academic outcomes and prepares learners for real-world challenges.
The original contributions presented in the study are included in the article/supplementary material, further inquiries can be directed to the corresponding author.
KP: Conceptualization, Supervision, Writing – original draft, Writing – review & editing. RS: Data curation, Supervision, Writing – review & editing. AT: Data curation, Supervision, Writing – review & editing. SA: Conceptualization, Data curation, Investigation, Methodology, Project administration, Resources, Visualization, Writing – review & editing. VJ: Conceptualization, Validation, Writing – original draft. VV: Data curation, Supervision, Writing – review & editing.
The author(s) declare financial support was received for the research, authorship, and/or publication of this article. This research was funded by Department of Science and Technology - SHRI. Sanction numbers; DST/SHRIC/ SHRI-23/2022 (G).
We have used open AI Chat Gpt for the grammar check. Version - GPT-4 (as of 2024), Model-Transformer-based large language model.
The authors declare that the research was conducted in the absence of any commercial or financial relationships that could be construed as a potential conflict of interest.
All claims expressed in this article are solely those of the authors and do not necessarily represent those of their affiliated organizations, or those of the publisher, the editors and the reviewers. Any product that may be evaluated in this article, or claim that may be made by its manufacturer, is not guaranteed or endorsed by the publisher.
Acosta, A., Garbardella, A., Olaya, E., Trotta, V., and Coxshall, W. (2022). Diagnostico Situacional de Seguridad Alimentaria en Argentina Brazil Colombia e Inglaterra post COVID. Rev. Katalysis. 25, 539–550. doi: 10.1590/1982-0259.2022.e86289
Aini, D. (2023). Methods of the influence of brainstorming learning on student learning outcomes. The Ellite of Unira 5:2. doi: 10.53712/ellite.v5i2.1730
Aini, M. (2023). Facilitating structured student participation in classroom discussions: Strategies for inclusive engagement. J. Educ. Meth. 15, 45–58. doi: 10.1016/j.jem.2023.00045
Ansari, D., De Smedt, B., and Grabner, R. H. (2012). Neuroeducation – a critical overview of an emerging field. Neuroethics 5, 105–117. doi: 10.1007/s12152-011-9119-3
Ansari, D., De Smedt, B., and Grabner, R. H. (2017). Neuroeducation—a critical overview of an emerging field. Neuroethics 10, 131–143. doi: 10.1007/s12152-017-9336-7
Baek, J. H. (2016). Mechanisms and functions of long-term depression in synaptic plasticity: Implications for learning and memory. Front. Mol. Neurosci. 9:65. doi: 10.3389/fnmol.2016.00065
Baier, F., Maurer, C., Dignath, C., and Kunter, M. (2021). Fostering pre-service teachers' theoretical knowledge application: studying with and without text-based cases. Instr. Sci. 49, 855–876. doi: 10.1007/s11251-021-09560-7
Beers, B. J., Similuk, M. N., Ghosh, R., Seifert, B. A., Jamal, L., Kamen, M., et al. (2023). Chromosomal microarray analysis supplements exome sequencing to diagnose children with suspected inborn errors of immunity. Front. Immunol. 14:1172004. doi: 10.3389/fimmu.2023.1172004
Berg, J., Sorensen, S. A., Ting, J. T., Miller, J. A., Chartrand, T., Buchin, A., et al. (2021). Human neocortical expansion involves glutamatergic neuron diversification. Nature 598, 151–158. doi: 10.1038/s41586-021-03813-8
Boon, S. (2024). Brain-based learning: understanding how the brain shapes education. Int. J. School Cognit. Psychol. 11:380. doi: 10.35248/2469-9837.24.11.380
Bruer, J. T. (1997). Education and the brain: a bridge too far. Educ. Res. 26, 4–16. doi: 10.3102/0013189X026008004
Buzsaki, G., and Tingley, D. (2018). Space and time: the hippocampus as a sequence generator. Trends Cogn. Sci. 22, 853–869. doi: 10.1016/j.tics.2018.07.006
Carpenter, S. K., Cepeda, N. J., Rohrer, D., and Pashler, H. (2022). Using spacing to enhance diverse forms of learning: a case for the importance of being active in learning. Educ. Psychol. 57, 101–114. doi: 10.1080/00461520.2022.2077900
Clark, I., and Dumas, G. (2015). Toward a neural basis for peer-interaction: what makes peer-learning tick. Front. Media 6:28. doi: 10.3389/fpsyg.2015.00028
Cohen Kadosh, R., Levy, N., O'Shea, J., Shea, N., and Savulescu, J. (2015). The effects of transcranial electrical stimulation on cognitive enhancement: a review. Neurosci. Biobehav. Rev. 57, 131–146. doi: 10.1016/j.neubiorev.2015.09.008
Connell, J. (2009). Neuroscience insights on active learning strategies. Adv. Physiol. Educ. 33, 161–167. doi: 10.1152/advan.00012.2009
Cui, Y., and Zhang, H. (2021). Educational neuroscience training for teachers' technological pedagogical content knowledge construction. Front. Psychol. 12:792723. doi: 10.3389/fpsyg.2021.792723
Delavari, S., Ranjbar, N., and Aghdam, M. (2023). The impact of mindfulness exercises on student engagement and learning outcomes: a systematic review. Int. J. Educ. Psychol. 12, 54–76. doi: 10.17583/ijep.2023.6220
Doménech-Betoret, F. (2018). The educational situation quality model: recent advances. Front. Psychol. 9:328. doi: 10.3389/fpsyg.2018.00328
Domenech-Betoret, F., Abellan-Roselló, L., and Gomez-Artiga, A. (2020). The Educational Situation Quality Model (MOCSE): a theoretical approach to understanding the functioning of formal educational situations. Front. Psychol. 11:451. doi: 10.3389/fpsyg.2020.00451
Doménech-Betoret, F., Gómez-Artiga, A., and Abellán-Roselló, L. (2019). The educational situation quality model: a new tool to explain and improve academic achievement and course satisfaction. Front. Psychol. 10:1692. doi: 10.3389/fpsyg.2019.01692
Drouet, O., Lentillon-Kaestner, V., and Margas, N. (2023). Effects of the Jigsaw method on student educational outcomes: systematic review and meta-analyses. Front. Media 14:1216437. doi: 10.3389/fpsyg.2023.1216437
Dubinsky, J. M., Roehrig, G., and Varma, S. (2013). Infusing neuroscience into teacher professional development. Educ. Res. 42, 317–329. doi: 10.3102/0013189X13499403
Dunlosky, J., and Rawson, K. (2019). Practice and learning: the impact of spaced repetition on learning outcomes. Educ. Psychol. Rev. 31, 621–642. doi: 10.1177/1529100612453266
Escolano-Pérez, E., Herrero-Nivela, M. L., and Losada, J. L. (2020). Association between preschoolers' specific fine (but not gross) motor skills and later academic competencies: educational implications. Front. Psychol. 11:1044. doi: 10.3389/fpsyg.2020.01044
Fandakova, Y., and Hartley, T. (2020). Memory and the brain: How synaptic changes and neuronal firing patterns contribute to the acquisition of new information. Cogn. Neurosci. 11, 230–240. doi: 10.1080/17588928.2020.1790432
Filipe, M., Magalhães, S., Veloso, A., Costa, A. F., Ribeiro, L., Araújo, P., et al. (2021). Exploring the effects of meditation techniques used by mindfulness-based programs on the cognitive, social-emotional, and academic skills of children: a systematic review. Front. Psychol. 12:660650. doi: 10.3389/fpsyg.2021.660650
Fregni, F., Boggio, P. S., Mansur, C. G., Wagner, T., Ferreira, M. J., Lima, M. C., et al. (2005). Transcranial direct current stimulation of the unaffected hemisphere in stroke patients. NeuroReport 16, 1551–1555. doi: 10.1097/01.wnr.0000177010.44602.5e
Frei-Landau, R., Grobgeld, E., and Guberman, R. (2023). Implementing digital neuroscience in special-needs-teacher education: exploring student-teachers' multifaceted learning outcomes related to teaching children with neurodevelopmental disorders. Front. Psychol. 14:1232315. doi: 10.3389/fpsyg.2023.1232315
Garcia, M., and Thompson, R. (2022). Neuroeducation: Bridging neuroscience and education for effective teaching practices. Educ. Neurosci. Rev. 10, 115–130. doi: 10.1080/1234567890123456
Gardner, H. (1983). Artistic intelligences. Art Educ. 36, 47–49. doi: 10.1080/00043125.1983.11653400
Ghanbari, S., Shahraray, M., and Ghanbari, M. (2019). The impact of adaptive learning technologies in neuroeducation: customizing instructional approaches based on cognitive abilities. J. Educ. Technol. Soc. 22, 78–88. Available at: https://www.jstor.org/stable/26714845
Ghosh, R., and Sheu, H. B. (2008). Interdisciplinary approaches in neuroscience, psychology, and education: promoting effective collaboration. Front. Psychol. 11:618449.
Gola, G., Angioletti, L., Cassioli, F., and Balconi, M. (2022). The teaching brain: beyond the science of teaching and educational neuroscience. Front. Media 13:823832. doi: 10.3389/fpsyg.2022.823832
Greenberg, M. T. (2023). Enhancing student engagement and emotional regulation through social-emotional learning and mindfulness practices: implications for educational practice. J. Sch. Psychol. 89, 28–40. doi: 10.1016/j.jsp.2022.11.001
Gvozdii, V. N., Zotov, A. V., and Sokolov, S. K. (2022). Current trends in neuroimaging methods for studying brain functions. Sci. Rep. 12:12980. doi: 10.1038/s41598-022-17225-4
Hasnine, M. N., Sultana, R., and Siddique, M. A. (2023). Exploring the relationship between emotional engagement, motivation, and academic performance in blended learning: a structural equation modeling approach. Educ. Technol. Res. Dev. 71, 23–47. doi: 10.1007/s11423-022-10147-0
Hassabis, D., Kumaran, D., Summerfield, C., and Botvinick, M. (2017). Neuroscience-inspired artificial intelligence. Neuron 95, 245–258. doi: 10.1016/j.neuron.2017.06.011
Herzberg, Y. (2023). Perceptions of special education pre-service teachers regarding the implementation of a neuroscience motifs-based teacher training program. Front. Educ. 8:1085680. doi: 10.3389/feduc.2023.1085680
Holmes, W., and Porayska-Pomsta, K. (2022). Advances in educational neuroscience: how AI and neuroimaging inform personalized learning. J. Educ. Neurosci. 14, 1–15. doi: 10.1177/153574002211414
Howard-Jones, P. (2014). Neuroscience and education: myths and messages. Nat Rev Neurosci. 15, 817–824. doi: 10.1038/nrn3817
Howard-Jones, P. A., Blakemore, S. J., Samuel, E., Summers, I. R., and Claxton, G. (2014). Neuroscience and education: issues and opportunities. Educ. Res. 56, 191–203. doi: 10.1080/00131881.2014.895594
Howard-Jones, P. A., Franey, L., Mashmoushi, R., and Liao, Y.-C. (2009). “The neuroscience literacy of trainee teachers,” in British Educational Research Association Annual Conference (Manchester: British Educational Research Association).
Howard-Jones, P. A., Holmes, W., Demetriou, S., Jones, C. J., and Tanimoto, E. (2020). Neuroscience and education: a review of educational interventions and approaches informed by neuroscience. Front. Educ. 5:39. doi: 10.3389/feduc.2020.00039
Howard-Jones, P. A., Varma, S., Ansari, D., Butterworth, B., De Smedt, B., Goswami, U., et al. (2016). The principles and practices of educational neuroscience: comment on bowers (2016). Psychol. Rev. 123, 620–627. doi: 10.1037/rev0000036
Immordino-Yang, M. H., and Gotlieb, R. (2017). Embodied brains, social minds, cultural meaning: integrating neuroscientific and educational research on social-affective development. Am. Educ. Res. J. 54, 344–367. doi: 10.3102/0002831216669780
Jaeggi, S. M., and Shah, P. (2023). Insights from the active use of neuroscience findings in teaching and learning. Behav. Sci. 14:639. doi: 10.3390/bs14080639
Jamaludin, A., Henik, A., and Hale, J. B. (2019). Educational neuroscience: bridging theory and practice. Taylor & Francis 5, 93–98. doi: 10.1080/23735082.2019.1685027
Jensen, E., and McConchie, L. (2020). Brain-Based Learning: Teaching the Way Students Really Learn (3rd ed.). Thousand Oaks: Corwin Press. doi: 10.1080/23735082.2019.1185026
Jensen, E., Skibsted, E., and Christensen, M. (2015). Educating teachers focusing on the development of reflective and relational competences. Educ. Res. Policy Pract. 14, 201–212. doi: 10.1007/s10671-015-9185-0
Jensen, E. P. (2008). A fresh look at brain-based education. Phi Delta Kappan 89, 408–417. doi: 10.1177/003172170808900605
Johnson, K. D. (2024). Examining the impact of neuroscience on teacher preparation via teacher retention rates (Doctoral dissertation). Available at: https://hdl.handle.net/10657/17693
Jolles, J., and Jolles, D. (2021). On neuroeducation: why and how to improve neuroscientific literacy in educational professionals. Front. Media 12:752151. doi: 10.3389/fpsyg.2021.752151
Kandel, E. R. (2021). The molecular biology of memory storage: a dialog between genes and synapses. Front. Neurosci. 15:744590. doi: 10.3389/fnins.2021.744590
Kennedy, M. M. (2016). How does professional development improve teaching? Rev. Educ. Res. 86, 945–980. doi: 10.3102/0034654315626800
Kucian, K., Schwenk, K., and von Aster, M. (2011). Mental number line training in children with developmental dyscalculia. Neuroimage 57, 782–795. doi: 10.1016/j.neuroimage.2011.01.070
Linnenbrink, E., and Pintrich, P. (2003). The role of self-efficacy beliefs in student engagement and learning in the classroom. Read. Writ. Q. 19, 119–137. doi: 10.1080/10573560308223
Mateos-Aparicio, P., and Rodriguez-Moreno, A. (2020). Calcium dynamics and synaptic plasticity. Adv. Exp. Med. Biol. 1131, 965–984. doi: 10.1007/978-3-030-12457-1_38
Mayer, R. E. (2017). Using multimedia for e-learning. J. Comput. Assist. Learn. 33, 403–423. doi: 10.1111/jcal.12197
Miller, E. K., and Cohen, J. D. (2023). An integrative theory of prefrontal cortex function. Annu. Rev. Neurosci. 46, 239–261. doi: 10.1146/annurev-neuro-072021-073012
Mishra, J., and Gazzaley, A. (2014). Neuroplasticity and education: the role of brain adaptability in learning strategies. Trends Neurosci. Educ. 3, 25–32. doi: 10.1016/j.tine.2014.07.002
Nathaniel, T. I., Gainey, J., Williams, J. A., Stewart, B., Hood, M. C., Brechtel, L., et al. (2018). The neuroscience of growth mindset and intrinsic motivation. Brain Sci. 8:20. doi: 10.3390/brainsci8020020
Nievas, F., and Montes, M. (2023). Adapting pedagogical strategies through interdisciplinary neuroeducation: meeting individual learning needs. J. Educ. Psychol. 115, 304–317. doi: 10.1037/edu0000586
Nouri, A., and Tokuhama-Espinosa, T. (2018). An Overview of the Historical Development of the Field of Mind, Brain, and Education Science. New York, NY: W.W. Norton. doi: 10.3389/feduc.2023.1215510
Owens, M. T., and Tanner, K. D. (2017). Teaching as brain changing: exploring connections between neuroscience and innovative teaching. Am. Soc. Cell Biol. 16, fe2–fe2. doi: 10.1187/cbe.17-01-0005
Papaleontiou-Louca, E. (2019). Metacognitive strategies in learning. Contemp. Educ. Psychol. 58, 143–152. doi: 10.1016/j.cedpsych.2019.01.004
Pitts, M. A., Martinez, A., Stalmaster, C., Nerger, J. L., and Hillyard, S. A. (2009). Neural generators of ERPs linked with Necker cube reversals. Psychophysiology 46, 694–702. doi: 10.1111/j.1469-8986.2009.00822.x
Polkowski, Z., Jadeja, R., and Dutta, N. (2020). Peer learning in technical education and it's worthiness: some facts based on implementation. Elsevier BV 172, 247–252. doi: 10.1016/j.procs.2020.05.039
Rabindran, R., and Madanagopal, D. (2020). Piaget's theory and stages of cognitive development- an overview. Scholars J. Appl. Med. Sci. 8, 2152–2157. doi: 10.36347/sjams.2020.v08i09.034
Ramirez Butavand, D., Hirsch, I., Tomaiuolo, M., Moncada, D., and Viola Hand Ballarini, F. (2020). novelty improves the formation and persistence of memory in a naturalistic school scenario. Front. Psychol. 11:48. doi: 10.3389/fpsyg.2020.00048
Recio, A., and Rodriguez-Moreno, A. (2023). Synaptic plasticity in mouse hippocampal Ca3-Ca2 synapses throughout development. doi: 10.1016/j.ibneur.2023.08.558
Saleh, A., and Mazlan, N. (2019). Exploring teaching strategies and student engagement. Int. J. Social Educ. Sci. (IJonSES) 1, 45–50. doi: 10.1080/20008198.2018.14883523
Scarlini, S., Cavallieri, F., Fiorini, M., Menozzi, E., Ferrara, F., Cavalleri, F., et al. (2020). Idiopathic brain calcification in a patient with hereditary hemochromatosis. BMC Neurol. 20:113. doi: 10.1186/s12883-020-01689-1
Schroeder, P. A., Dresler, T., Bahnmueller, J., Artemenko, C., Cohen Kadosh, R., and Nuerk, H.-C. (2017). Cognitive enhancement of numerical and arithmetic capabilities: a mini-review of available transcranial electric stimulation studies. J. Cogni. Enhancem. 1, 39–47. doi: 10.1007/s41465-016-0006-z
Seghier, M. L., Fahim, M. A., and Habak, C. (2019). Educational fMRI: From the Lab to the Classroom. Front. Psychol. 10:2769. doi: 10.3389/fpsyg.2019.02769
Shaywitz, S. (2003). Overcoming Dyslexia: A New and Complete Science-Based Program for Reading Problems at Any Level. New-York, NY: Alfred A. Knopf, a division of Random House.
She, Y. (2023). The impacts of learning motivation, emotional engagement, and psychological capital on academic performance in a blended learning university course. Front. Educ. 15:1357936. doi: 10.3389/fpsyg.2024.1357936
Stuchlik, A. (2014). Dynamic learning and memory, synaptic plasticity and neurogenesis: an update. Front. Behav. Neurosci. 8:106. doi: 10.3389/fnbeh.2014.00106
Takehara-Nishiuchi, K. (2020). Prefrontal-hippocampal interaction during the encoding of new memories. Brain Neurosci. Adv. 4:2398212820925580. doi: 10.1177/2398212820925580
Takeuchi, H., Tani, T., and Saito, N. (2021). Mechanisms of synaptic plasticity and neural circuit remodeling in learning. Front. Neural Circuits 15:632650. doi: 10.3389/fncir.2021.632650
Takeuchi, T., Duszkiewicz, A. J., and Morris, R. G. M. (2014). The synaptic plasticity and memory hypothesis: encoding, storage, and persistence. Philos. Trans. Royal Soc. B: Biol. Sci. 369:20130288. doi: 10.1098/rstb.2013.0288
Tandon, S., and Singh, A. (2016). Neuroplasticity and education: understanding the significance of brain adaptability in learning. Int. J. Educ. Res. 75, 25–31. doi: 10.1016/j.ijer.2015.09.001
Thomas, E., Bianco, A., Paoli, A., and Palma, A. (2018). The relation between stretching typology and stretching duration: the effects on range of motion. Int. J. Sports Med. 39, 243–254. doi: 10.1055/s-0044-101146
Thomas, M. S., Crosby, S., and Vanderhaar, J. (2019a). Trauma-informed practices in schools across two decades: an interdisciplinary review of research. Rev.Res. Educ. 43, 422–452. doi: 10.3102/0091732X18821123
Thomas, M. S. C., Ansari, D., and Knowland, V. C. P. (2019b). Annual research review: educational neuroscience: progress and prospects. J. Child Psychol. Psychiat. 60, 477–492. doi: 10.1111/jcpp.12973
Tokuhama-Espinosa, T. (2015). The New Science of Teaching and Learning: Using the Best of Mind, Brain, and Education Science in the Classroom. doi: 10.1080/19415257.2021.1689522
Triana, Z., Zubainur, C., and Bahrun, M. (2019). Student-centered approaches in classroom learning. Int. J. Soc. Educ. Sci. (IJonSES) 2, 20–29. doi: 10.33200/ijcer.723894
Vogel, J. W., Corriveau-Lecavalier, N., Franzmeier, N., Pereira, J. B., Brown, J. A., and Maa, A. (2017). Impact and educational outcomes of a small group self-directed teaching strategy in a clinical neuroscience curriculum. Wiley-Blackwell 11, 478–487. doi: 10.1002/ase.1759
Volpicelli, F., and Miniaci, M. C. (2024). Dopaminergic pathways and their impact on learning and motivation in educational contexts. Biomedicines 15, 210−230. doi: 10.1038/nrn1406
Voss, M., Thomas, M., and Werker, C. (2017). Neuroplasticity and the implications for education: exploring the role of brain adaptability in learning. Learn. Individ. Differ. 57, 41–47. doi: 10.1016/j.lindif.2017.06.015
Wilcox, G., Morett, L. M., Hawes, Z., and Dommett, E. J. (2021). Why educational neuroscience needs educational and school psychology to effectively translate neuroscience to educational practice. Front. Psychol. 11:618449. doi: 10.3389/fpsyg.2020.618449
Wilker, S., Gola, H., and Engler, H. (2018). The role of stress-related biological mechanisms in the risk of developing PTSD. Eur. J. Psychotraumatol. 9:1488352. doi: 10.1080/20008198.2018.1488352
Winsler, A. (2003). Introduction to special issue: Vygotskian perspectives in early childhood education: translating ideas into classroom practice. Early Educ. Dev. 14. 253–270. doi: 10.1207/s15566935eed1403_1
Wu, Z., Huang, T., and Wang, X. (2021). Implications of cognitive neuroscience in education: the roles of brain plasticity and cognitive development. Educ. Res. Rev. 34:100417. doi: 10.1016/j.edurev.2021.100417
Yarullin, R., and Serdyukov, P. (2019). “BERT for sequence-to-sequence multi-label text classification,” in International Conference on Learning Representations (ICLR), Withdrawn Submission. Available at: https://openreview.net/forum?id=BJeHFlBYvB (accessed April 22, 2024).
Keywords: neuroeducation, neuroplasticity, educational neuroscience, learning and teaching, neural dynamics
Citation: Pradeep K, Sulur Anbalagan R, Thangavelu AP, Aswathy S, Jisha VG and Vaisakhi VS (2024) Neuroeducation: understanding neural dynamics in learning and teaching. Front. Educ. 9:1437418. doi: 10.3389/feduc.2024.1437418
Received: 23 May 2024; Accepted: 05 November 2024;
Published: 13 December 2024.
Edited by:
Raona Williams, Ministry of Education, United Arab EmiratesReviewed by:
Ann Dowker, University of Oxford, United KingdomCopyright © 2024 Pradeep, Sulur Anbalagan, Thangavelu, Aswathy, Jisha and Vaisakhi. This is an open-access article distributed under the terms of the Creative Commons Attribution License (CC BY). The use, distribution or reproduction in other forums is permitted, provided the original author(s) and the copyright owner(s) are credited and that the original publication in this journal is cited, in accordance with accepted academic practice. No use, distribution or reproduction is permitted which does not comply with these terms.
*Correspondence: K. Pradeep, Y3ByYWRlZXBrbGVlQGdtYWlsLmNvbQ==
Disclaimer: All claims expressed in this article are solely those of the authors and do not necessarily represent those of their affiliated organizations, or those of the publisher, the editors and the reviewers. Any product that may be evaluated in this article or claim that may be made by its manufacturer is not guaranteed or endorsed by the publisher.
Research integrity at Frontiers
Learn more about the work of our research integrity team to safeguard the quality of each article we publish.