- 1Department of Chemistry, Universidad Técnica Particular de Loja, Loja, Ecuador
- 2Facultad de Ingeniería, Universidad Nacional de Chimborazo, Riobamba, Ecuador
- 3Facultad de Ciencias Escuela Superior Politécnica de Chimborazo (ESPOCH), Riobamba, Ecuador
- 4Facultad de Ciencias - Grupo de Investigación CIDED de la Escuela Superior Politécnica de Chimborazo (ESPOCH), Riobamba, Ecuador
- 5INFN-Laboratori Nazionali di Frascati, Frascati, Italy
Objective: This systematic review aims to synthesize current knowledge on integrating immersive technologies, namely Virtual Reality (VR) and Augmented Reality (AR), in Science, Technology, Engineering, Mathematics (STEM) education, and to lighten their impact on student performance and engagement.
Methods: Adhering to PRISMA guidelines, a detailed search strategy across Scopus and Web of Science databases identified relevant peer-reviewed journal articles published from 2002 to 2023. The PICOS approach informed our inclusion criteria, focusing on empirical studies that assessed the application of VR and AR technologies in Science, Technology, Engineering, Mathematics (STEM) educational settings. Studies were evaluated for methodological quality, and data extraction was meticulous, with variables categorized as engagement, performance, or a combination of both.
Analysis: A descriptive and statistical analysis using chi-square tests was employed to examine the relationship between the type of technology (AR, VR, XR, MR) and the educational outcomes. This quantitative assessment provided a nuanced understanding of the interplay between various immersive technologies and their pedagogical effectiveness.
Results: Out of 143 initially identified articles, 22 met the inclusion criteria for detailed analysis. Findings revealed that AR was the most studied technology, followed by VR. Most studies reported positive effects on student engagement and performance, with increased effectiveness being less frequently observed. A notable portion of the studies specifically investigated the combination of performance and engagement, underscoring the multifaceted benefits of immersive technologies in education.
Conclusions: Immersive technologies are reshaping STEM education by enhancing engagement and performance. Integrating VR and AR offers promising educational benefits, including improved comprehension of complex concepts, increased student motivation, and enriched collaborative learning experiences. However, the field requires a globally inclusive and adaptable framework for rapid technological evolution and diverse educational contexts. Future research should broaden its scope to include multilingual literature and non-traditional academic channels, ensure ethical standards are upheld, and focus on personalization and adaptability to maximize the educational potential of these technologies.
1 Introduction
Integrating immersive technologies such as Augmented Reality (AR), Virtual Reality (VR), and Mixed Reality (MR) into STEM education heralds a transformative era for teaching and learning processes. In this systematic review, we examine several works that explore various dimensions and outcomes of leveraging immersive technologies in STEM education, aiming to encapsulate their pedagogical potential, challenges, and future directions.
In this context, Shirazi and Behzadan (2018), through their work in 2015, initiated the debate by demonstrating how AR could enhance student performance in construction and civil engineering projects, suggesting that AR's interactive nature significantly improves collaboration and idea exchange. Following this trajectory, Rogers et al. (2018) explored the efficacy of VR environments in e-learning platforms for computerized numerical control (CNC) milling operations, emphasizing VR's capability to provide realistic, accessible learning experiences without the need for physical lab facilities.
In the same way, Lin et al. (2018) investigated the impact of AR on learning motivation and imaginative capability through picture e-books, revealing that AR not only bolsters imaginative skills but also significantly enhances learning motivation across various dimensions. These findings underscore the role of AR in creating engaging and motivating learning experiences that are crucial for fostering creativity and innovation in STEM education. Expanding the scope to MR, Barrett et al. (2018) showed how MR could enrich chemistry education by facilitating student-driven investigative learning, thereby enhancing engagement and understanding through tactile feedback and simulation-based teaching. Similarly, Sharma and Mantri (2019) developed an AR Learning System (ARLS) combined with an Electronics Kit, illustrating that AR can significantly reduce cognitive load and make science fundamentals more accessible and enjoyable for young learners.
Kassim and Zubir (2019) introduced the Augmented Reality on Engineering Equipment for Education (AREEE) platform, highlighting the potential of AR to provide a hands-on feel in a virtual setting, thus enhancing the learning experience for engineering students. This work, along with the outcomes by Kazanidis and Pellas (2019), explored the applications of immersive technologies in mathematics education, emphasizing the importance of integrating AR into classroom contexts to support effective teaching and learning processes.
The advent of 2021 brought further insights from Mahanan et al. (2021) and Marques and Pombo (2021), who focused on the impact of teacher training with mobile AR games on professional development, illustrating the critical role of teacher readiness and confidence in adopting innovative technologies for STEM learning enhancement. These findings are complemented by Iqbal et al. (2022) based on a critical perspective of AR in education, which not only delineates current challenges but also outlines future research directions for more interactive, personalized, and collaborative learning experiences facilitated by AR technologies.
Subsequent studies, such as those by Çoban et al. (2022), Li et al. (2022), and Sumardani et al. (2023), continue to explore the capacity of AR/VR to enhance understanding and engagement in subjects like mathematics, physics, and material science, stressing the role of these technologies in fostering spatial skills and representational fluency. The evaluation of teacher competencies for using AR in physics lessons by Freese et al. (2023) and the development of VR-based educational tools for projectile motion by Cruz et al. (2023) further elucidate the educational value of immersive technologies in enhancing STEM learning.
Very recently, Meccawy (2023) examined teacher attitudes toward adopting extended reality (XR) technologies in classrooms, highlighting the potential benefits and challenges of XR integration into teaching and learning processes. In particular, this work underlines the emerging consensus on the transformative potential of immersive technologies in STEM education, while also acknowledging the hurdles to their widespread adoption, such as infrastructural limitations, financial constraints, and the need for comprehensive teacher training.
The preceding studies collectively present a huge potential for integrating immersive technologies within STEM educational frameworks. Hence, this systematic review is poised to shed light on future research directions and practical implementations in this swiftly progressing domain. By amalgamating the insights from these varied applications, our review contributes a critical understanding of how STEM methodologies are intricately woven into the constitution of immersive technology evolution. This inquiry not only traces the development of immersive technologies in STEM education across nearly a decade but also delves deep into the influence of VR and AR in this area.
Adhering to the PRISMA guidelines, this systematic review endeavors to consolidate existing literature, offering a nuanced synthesis of knowledge on the deployment of virtual technologies across diverse contexts, with a particular focus on their effects on the engagement and performance of students and professionals. As well, guided by the PICOS framework, our research inquiry is articulated as follows:
• “How do immersive technologies enhance the performance and engagement of students and professionals within STEM-based educational settings, and what impact do these technologies have on learning outcomes?”
This refined focus aims to elucidate the transformative potential of immersive technologies in enriching STEM education, fostering a deeper action, and elevating the learning experience for all participants.
2 Methodology
In alignment with the focus of our systematic review on integrating immersive technologies within STEM education, we adopt the PICOS approach—a widely recognized research framework for formulating precise and focused research inquiries for systematic reviews (Amir-Behghadami and Janati, 2020; Deng et al., 2024; Franco et al., 2024). In particular, we follow the inclusion and eligibility criteria exposed by Amir-Behghadami and Janati (2020). The PICOS framework methodically dissects the research question into five essential components (Population, Intervention, Comparison, Outcome, and Study Design), as outlined in Table 1. Particularly, this framework not only guides the formulation of precise research questions but also aids in the strategic design of study inclusion criteria, ensuring the collection of relevant and impactful evidence tailored to the goal of our systematic review work.
Figure 1 presents the methodological framework adopted in the present review, conforming to the Preferred Reporting Items for Systematic Reviews and Meta-Analyses (PRISMA) guidelines (Page et al., 2021). The delineated time frame spans from 2002 to 2023, a period strategically selected to encompass the burgeoning stages of immersive technologies. This period marks the initial integration of these technologies into a breadth of domains, capturing the progressive intertwining with STEM education—most notably, the enhancement of teaching and learning in foundational STEM concepts. Additionally, this time frame allows us to analyze the impact of immersive technologies on STEM models, observing not only their developmental targets but also the parallel evolution in pedagogical strategies.
2.1 Identification
In January 2024, our literature search was executed, targeting studies pertinent to our systematic review. We opted for Scopus, Web of Science, and PubMed as our primary databases due to their extensive access and multidisciplinary nature, which is crucial for our investigation into “Exploring Immersive Technologies in STEM-oriented Education.”
• Particularly, Scopus is recognized for its expansive collection of peer-reviewed content, excelling in the fields of science, technology, and education. It offers a comprehensive array of journals and conference proceedings, ensuring a rich amalgamation of research pertinent to the academic and practical dimensions of STEM education. Its advanced indexing capabilities facilitate an exhaustive retrieval of contemporary and archival studies.
• Similarly, Web of Science complements Scopus with its rigorous selection of quality research articles and robust citation indexing, which is invaluable for tracing the influence and evolution of key research within the field. It provides a structured and academically robust platform for accessing influential studies that have shaped the current landscape of immersive technology in education.
• We also included PubMed due to its strong emphasis on biomedical and life sciences, which are integral to the broader STEM education framework. PubMed provides access to a vast repository of biomedical literature, including studies on the educational applications of immersive technologies in health and medical training. Its comprehensive indexing and advanced search functionalities ensure the inclusion of high-quality, relevant studies that contribute to our understanding of immersive technologies in various STEM disciplines.
Hence, these databases collectively provide a more exhaustive scope than alternatives such as IEEE Xplore or MathSciNet, which, while valuable, offer narrower insights that may not capture the interdisciplinary breadth required for this review. The superior search functionalities and filtering options available through Scopus, Web of Science, and PubMed enable precise and comprehensive literature retrieval, ensuring that our review is built upon a foundation of the most relevant and significant studies. Keeping this in mind, the outcomes of our systematic search are displayed in Table 2, detailing the volume of articles retrieved, which form the basis for our subsequent analysis and synthesis.
2.2 Screening
Upon completion of a database search, we identified a collection of 152 pertinent articles: 80 sourced from Scopus, 66 from Web of Science, and 6 from PubMed (Table 2). In the initial filtering phase, we purposefully excluded conference proceedings, books, and book chapters to ensure a focus on peer-reviewed journal articles. We excluded conference proceedings, books, and book chapters to maintain a high standard of empirical rigor and consistency in our review. Peer-reviewed journal articles undergo a rigorous evaluation process by experts in the field, ensuring the validity, reliability, and scientific merit of the research. In contrast, conference proceedings, books, and book chapters often vary widely in their review processes and may not consistently provide the same level of methodological rigor and detailed empirical data required for a systematic review. By focusing on peer-reviewed journal articles, we ensure that our review is built on a foundation of high-quality, reliable, and reproducible research. At this point, this process yielded 99 unique articles after the elimination of duplicates.
Subsequently, these articles were subjected to a rigorous title and abstract screening process based on inclusion criteria meticulously aligned with our research question and the PRISMA guidelines:
• Full-length original research articles: We excluded editorials, brief communications, reviews, and commentaries to maintain the integrity of empirical evidence provided by detailed research articles.
• Focus on STEM education and training: Articles must explicitly address STEM education and training, ensuring relevance to our research question on immersive technologies in these disciplines.
• Evaluation of VR, AR, or IVEs: Only studies evaluating the use of virtual reality (VR), augmented reality (AR), or immersive virtual environments (IVEs) as educational tools were included.
• Implementation of simulators or learning outcomes: Studies must investigate the use of simulators or assess learning outcomes related to STEM integration through immersive technologies, aligning with our PICOS framework.
Employing these criteria, 33 articles were omitted during the selection phase. The remaining 66 articles advanced to a comprehensive full-text review to ascertain their suitability for inclusion in this review.
2.3 Eligibility
During the Eligibility phase, we conducted a methodical assessment of the full-text articles. To promote objective and comprehensive evaluation, the remaining works were randomized among research team members. The specific eligibility criteria were crafted based on the core concepts of our research question and study design:
• Application of AR and VR technologies: articles needed to delineate the application of augmented reality (AR) and virtual reality (VR) technologies within STEM-focused educational contexts.
• Emphasis on immersive technologies for learning and teaching: the focus was on using immersive virtual or augmented reality as tools for learning and teaching within STEM education.
• Educational objectives within STEM disciplines: the utilization of virtual environments had to predominantly serve educational objectives within STEM disciplines.
Following the full-text review, 50 articles were found to meet all the set eligibility criteria and were selected for in-depth data extraction and analysis. During this phase, we excluded 16 articles that did not demonstrate the use of virtual environments for educational purposes with a central focus on STEM education. Additionally, 20 articles were removed as they lacked clear educational interventions related to teaching or STEM.
It is noteworthy that the excluded 16 articles contributed significantly to the conceptualization of our introduction, offering valuable insights that helped delineate the current research landscape surrounding the use of virtual or augmented reality in a broad context. At this stage, then, 30 articles satisfied the inclusion prerequisites and were advanced for comprehensive analysis.
2.4 Included
In the final stage of selection, systematic data extraction was conducted for articles satisfying all our inclusion criteria. This procedure aimed to gather detailed information on the following aspects:
• Influence of immersive technology interventions: impact on student performance and engagement.
• Type of immersive technology employed: distinguishing between VR and AR interventions.
• Observed effects: results from the implementation of these technologies.
• Participant demographics and roles: including sample size and participant roles.
From our search, 30 articles closely aligned with our research objectives and were selected for exhaustive analysis. The data extraction protocol focused on:
• Architecture and instructional design: design of the VR or AR tools used in the studies.
• Learning materials: nature and creation of materials within the virtual environment.
• Cognitive demands and time management: cognitive load imposed by the technologies and implications for time management.
• Feedback mechanisms and interactivity: presence of feedback systems and level of interactivity afforded by the technologies.
• Stratification of participants: sorting participants by their roles and degrees of involvement.
During this process, we identified eight review articles. In adherence to PRISMA guidelines, these were omitted from the principal analysis due to their lack of original research data. However, these reviews provided valuable information for comparative purposes and were instrumental in crafting the nuanced discussions in Section 4.3 of our systematic review. They highlighted our study contributions against the backdrop of existing literature. Thus, a subset of 22 articles was brought forward to the next phase for a thorough analysis of the interventions. These articles are discussed in detail in the subsequent sections of our review, emphasizing significant findings and their implications for the use of immersive technologies in STEM education.
3 Results
3.1 Summary of search results
Following the PRISMA guidelines and employing the PICOS framework, in this section, we summarize the outcomes of our systematic review on the utilization of AR/VR within STEM education. An initial search across Scopus and Web of Science yielded 152 articles–80 from Scopus, 66 from Web of Science, and six from PubMed. Post-removal of duplicates, 99 articles were screened based on titles and abstracts, leading to the exclusion of 33 articles that did not align with our selection criteria. This resulted in 66 articles being considered for further analysis. Upon a detailed full-text assessment, 36 articles were further excluded due to either their lack of direct relevance to educational applications of virtual environments in STEM, the absence of specific educational interventions, or because they were review articles rather than original research.
Consequently, 30 articles were earmarked for in-depth data extraction. These studies covered a spectrum of AR and VR interventions within STEM education, scrutinizing their impact on aspects such as learner engagement and performance. At this point, eight review articles, while excluded from the primary intervention analysis, were recognized for their contribution to contextualizing the landscape of AR/VR applications in STEM education. The focal analysis then proceeded with 22 articles that undertook a complete examination for intervention analysis. This phase entailed a closer look into the nature of VR/AR interventions and their immediate effects on STEM education outcomes.
The 22 studies have been carefully selected to assess the influence of immersive VR/AR interventions within STEM education. These studies were specifically chosen for their alignment with our research question, examining:
• Performance: the effect of these interventions on performance is analyzed, focusing on the enhancement of skill acquisition and knowledge retention. This analysis spans a broad range of outcomes, from increased proficiency in educational practices to improved accuracy in applying STEM methodologies. Performance, in this context, is crucial as it directly correlates with the essential competencies that professionals in science, technology, engineering, and mathematics need to develop and refine throughout their careers.
• Engagement: this aspect evaluates the diverse ways in which students engage with and embrace VR/AR technologies within their STEM education. Indicators of engagement include a heightened readiness to incorporate these technologies into the learning environment, active participation in tech-driven educational tasks, and a persistent interest in the evolution of immersive tools. Engagement is a vital measure, reflecting both the integration of these technologies in STEM fields and their capacity to foster transformative educational approaches.
Keeping this in mind, Table 3 delineates the outcomes of these technologies across the analyzed studies. Among the 22 studies scrutinized, nine are dedicated to exploring the performance enhancements enabled by immersive technologies, eight concentrate on evaluating learner engagement, and the remaining five ambitiously address both engagement and performance. Furthermore, our study categorizes the observed effects into three distinct types (Positive, Increased, and Effectiveness) defined as follows:
• Positive Effects underscore the beneficial outcomes of employing immersive technologies in STEM education, encompassing enhanced academic performance, elevated motivation among learners, and a more engaging learning environment.
• Increased Proficiency signifies the marked enhancement in students' STEM capabilities and understanding, facilitated by the interactive and hands-on learning experiences that VR and AR offer.
• Effectiveness gauges the achievement of educational objectives through VR and AR interventions, emphasizing their role in bolstering engagement, improving performance metrics, and aiding the retention of knowledge in STEM disciplines.
Among the nine studies focusing on performance, seven reported positive effects, while two observed increased proficiency. Of the eight studies centered on engagement, five indicated positive effects, two documented increased engagement, and one highlighted effectiveness. Of the five studies evaluating both performance and engagement, four revealed positive outcomes, and one noted increased proficiency.
In terms of technological preferences across the 22 studies evaluated, AR emerged as the most frequently studied, with 11 studies. VR was the focus of seven studies. Extended Reality (XR) was explored in one study, two studies investigated the use of all three technologies (VR, AR, XR), and one study focused on Mixed Reality (MR), encompassing elements of both VR and AR.
3.2 Summary of interventions and impact
Here, we contextualize the significant interventions and their impacts:
• Nadan et al. (2011) utilized VR technologies like CAVE and PowerWall in educational settings, observing a positive impact on student engagement.
• Nagata et al. (2017) employed AR for mobile learning to enhance comprehension in STEM, noting positive effects on both performance and engagement.
• Hsu et al. (2017) explored the role of AR in medical surgery lessons, finding an increase in STEM interest and comprehension.
• Abd Majid and Abd Majid (2018) leveraged AR for interactive content on atomic structures, enhancing comprehension and engagement positively.
• Cooper et al. (2019) investigated the perceptions of VR in pre-service teachers, marking an increased engagement in educational settings.
• Jesionkowska et al. (2020) used AR in extracurricular STEAM workshops, observing positive outcomes in both performance and engagement.
• Dayarathna et al. (2020) introduced a VR module on queuing theory, showing increased performance in learning manufacturing systems.
• Lasica et al. (2020) and Rowe et al. (2020) assessed the AR and XR pedagogical value, respectively, each noting positive impacts on performance.
• Mohammadi et al. (2019) and Holly et al. (2021) demonstrated the positive influence of VR on engagement in online experiential learning and physics education.
• Montalbo (2021) and Shu and Huang (2021) utilized AR for chemistry education and VR in Maker education, respectively, with positive effects on performance and effectiveness in engagement.
• Miller et al. (2021), Wu et al. (2021), and Hobbs and Holley (2022) each reported positive impacts of MR and VR on performance and engagement through immersive learning designs and curriculum integration.
• Kao and Ruan (2022), Lindner et al. (2022), and Campos et al. (2022) found increased engagement and performance through interactive AR modes and applications in programming and environmental science.
• McNerney et al. (2023) and See et al. (2023) explored the role of XR and AR in simulation learning and remote sensing education, noting positive outcomes.
• Chiang and Liu (2023) examined XR technologies in STEM activities, observing positive impacts on learning response and performance.
3.3 Data analysis
Figure 2 categorizes the research focus within STEM education studies on immersive technologies, showing “Engagement” as the most investigated variable at 40.91%. “Performance” accounts for 36.36%, highlighting its significant role, while the combined “Performance and Engagement” is considered in 22.73% of the studies. This breakdown indicates that while student engagement with immersive technologies is the primary research interest, the performance outcomes, both separately and in combination with engagement, are also critical to understanding the full impact of these technologies in STEM education.
Figure 3 provides an analysis of the types of effects observed from the integration of immersive technologies in STEM education. The most substantial portion, 72.73%, signifies the “Positive” effects, illustrating that the majority of the studies reported beneficial outcomes from these interventions. The “Increase” in metrics such as engagement or performance is denoted by a 22.73% slice, reflecting significant growth attributable to the technology's use. Meanwhile, “Effectiveness,” representing the smallest segment at 4.55%, indicates a more place yet impactful outcome on educational objectives. The chart conveys that positive impacts and measurable improvements are the predominant effects observed, with effectiveness forming a smaller but vital aspect of the results.
Figure 4 explains the distribution of immersive virtual technologies (IVT) utilized in the analyzed studies within the context of STEM education. The pie chart is segmented to reflect the percentage of studies that applied each type of technology. Half of the studies (50%) utilized AR, indicating its dominant use in educational interventions. VR was the focus of 31.82% of the studies, showcasing its substantial role. MR and XR, while less represented, each accounted for 4.55% of the studies, highlighting their emerging but less prevalent application. Additionally, 9.09% of the studies integrated a combination of AR, VR, and XR, suggesting a multidisciplinary approach to immersive technology use in education. The chart indicates a strong preference for AR, with VR also being a significant tool, while MR and XR technologies are currently less common but important aspects of immersive technology integration in STEM education.
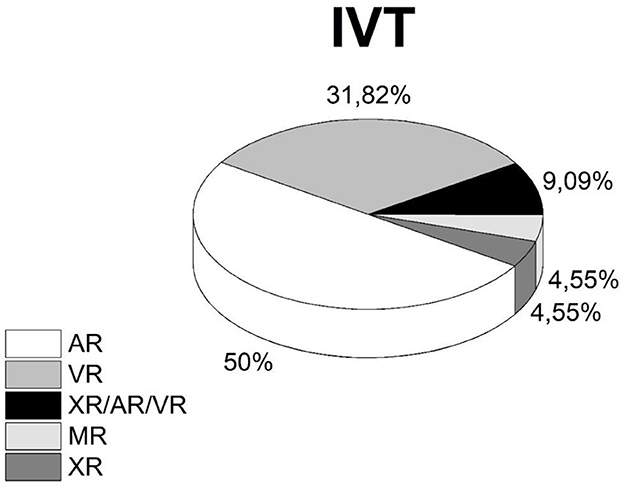
Figure 4. The types of immersive virtual technology (IVT) analyzed in percentage: augmented reality (AR), virtual reality (VR), extended reality (XR), and mixed reality (MR).
3.4 Descriptive analysis
The incorporation of chi-square tests within our systematic review marks an innovative approach that enhances the traditional narrative synthesis by allowing a quantitative assessment of categorical data. Systematic reviews often synthesize findings primarily through qualitative methods, but by applying chi-square tests, we can statistically examine relationships among categorical variables, such as stages of work, the variables examined, and the effects observed in studies involving immersive virtual technologies.
Hence, we employed descriptive statistics to quantify the frequencies of these categories, as shown in Table 4. The chi-square test is particularly suited for determining whether there is a statistically significant association between two categorical variables. In our case, these variables are the stages of work, the specific aspects of engagement and performance scrutinized, and the effects associated with the use of VR and AR technologies in STEM education. Where the expected frequencies are too low, thus not meeting the chi-square test assumptions, we resort to Fisher's exact test to attain a more accurate evaluation, thereby maintaining the integrity of our statistical conclusions even with limited sample sizes.
We have set our p-value threshold at below 0.05, aligning with standard statistical conventions, to determine the significance of the relationships under study. At this level, we reject the null hypothesis—which posits that there is no association between the examined variables—if the p-value is < 0.05. This threshold implies that we can assert, with 95% confidence, that the associations observed are not due to chance and may represent true underlying relationships within the data.
When analyzing the cross-Table Stage*IVT, we observe the following distribution of IVT types (AR, VR, XR/AR/VR, and MR) across different stages of research (Evaluation, Development, Development and Evaluation, Development and Testing):
• Evaluation Stage: AR was used in five studies, VR in 3, and XR in 1, but no MR was utilized. This indicates that AR is the preferred technology during the evaluation phase of research.
• Development Stage: Both AR and VR were used in three studies each, with XR and MR each being used in one study. This demonstrates an equal preference for AR and VR during the development phase, with some consideration given to mixed or extended realities.
• Development and Evaluation Stage: Only AR was used in one study. No instances of VR, XR/AR/VR, or MR were recorded at this stage.
• Development and Testing Stage: AR was used in two studies, and VR in 1, with no use of XR/AR/VR or MR.
From the chi-square test, the following key values are observed:
• Chi-squared Value: 4.754.
• Degrees of Freedom: 12.
• p-value: 0.966.
The high p-value (0.966) suggests that there is no statistically significant association between the stages of research and the types of IVT used. The high degrees of freedom (12) could be attributed to the number of categories within the stages of research and types of IVT. In general, while there is a diverse utilization of AR, VR, XR, and MR across the various stages of research, the chi-square test indicates that the choice of technology is not statistically dependent on the research stage. This might suggest that the selection of a particular IVT for research in STEM education is based on factors other than the research stage, such as the specific requirements of the study, the availability of technology, or the personal preference of the researchers. The lack of a significant association also suggests that researchers are open to employing a range of technologies at any stage of their research.
From Variables vs. ITV, the distribution of IVT usage across the research variables is as follows:
• Performance: AR was used in 36.4% of the studies, and VR in 28.6%.
• Engagement: AR featured heavily at 71.4%, confirming a strong preference for AR in studies examining engagement.
• Performance and Engagement: the combination of AR and VR was used in 50% of studies each, suggesting an equal distribution for studies that looked at both variables simultaneously.
The chi-square statistical test highlights the following findings:
• Chi-squared Value: 9.752.
• Degrees of Freedom: 8.
• p-value: 0.283.
With a p-value of 0.283, this chi-square test does not reveal a statistically significant association between the research variables and the types of IVT used. The p-value is above the 0.05 threshold, which means we fail to reject the null hypothesis, suggesting that any association observed in the data could be due to random chance rather than a systematic relationship. In the context of your systematic review, these findings imply that while AR is a popular choice for research focusing on engagement, the decision to use a particular IVT may depend on factors beyond the scope of this analysis, such as the specific needs of the study, the objectives of the research, or even resource availability. The results also emphasize the importance of considering individual technological strengths when designing studies to investigate performance, engagement, or the combination of both in STEM education.
Finally, for Effect vs. IVT, the Cross-Tabulation Analysis shows the relationship between the effects of IVT and the types of technologies used with the following key findings:
• Increase: the effect classified as “Increase” was observed in 27.3% of studies using AR and 28.6% of studies using VR, with no occurrences in XR/AR/VR or MR.
• Positive: most effects classified as “Positive” were observed in studies using AR (72.7%), followed by VR (57.1%), XR/AR/VR (100%), and MR (100%). This indicates that studies using AR and VR frequently report positive outcomes, and those that include a mix of technologies or MR always report positive outcomes.
• Effectiveness: “Effectiveness” was observed in 14.3% of VR studies, with no recorded effectiveness in AR or combined technologies, and no studies were reporting on MR under this category.
The Chi-Square Test Results evidence the following values:
• Chi-squared value: 3.900.
• Degrees of freedom: 8.
• p-value: 0.866.
The p-value of 0.866, well above the conventional 0.05 threshold for statistical significance, suggests that there is no significant association between the observed effects and the types of IVT used in the studies. Indeed, these results point to the need for more nuanced research that may focus on differentiating between various outcomes associated with each technology.
3.5 Data generated
In the interest of encouraging transparency and facilitating future research, we have documented our data generation process at each stage of this systematic review, see Figure 5. This documentation offers a comprehensive view of our methodology, ensuring that our approach is both replicable and open to scrutiny. For researchers seeking to delve deeper into our findings or to utilize our dataset for subsequent studies, we have made this information accessible. The complete dataset, along with a detailed account of the systematic review process, can be accessed via the following link: https://doi.org/10.17605/OSF.IO/YR2FG.
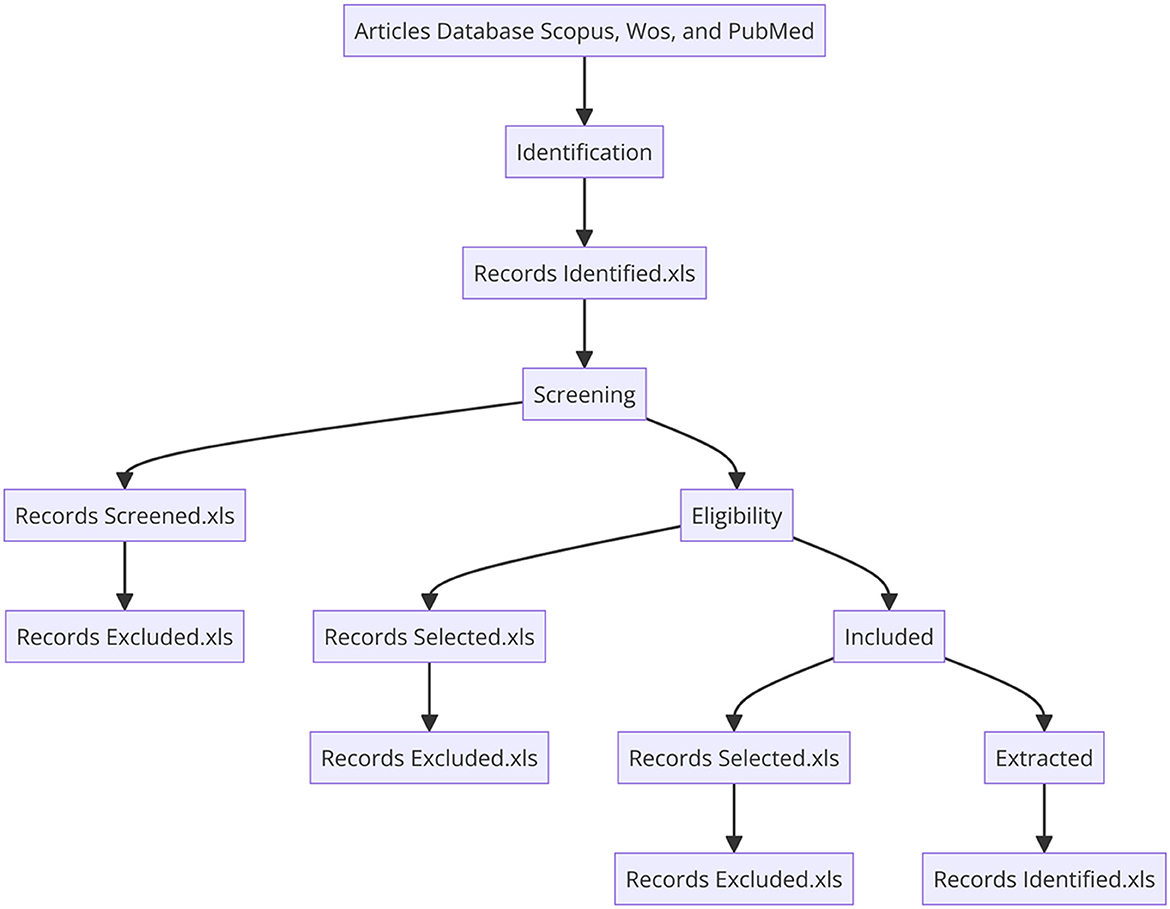
Figure 5. Flowchart of generated data available at: https://doi.org/10.17605/OSF.IO/YR2FG.
3.6 Theoretical considerations for the analysis
To provide a solid foundation for interpreting the study results and their implications, the current systematic review is based on well-established theories on learning, technology integration, and self-regulated learning (see Figure 6), summarized as follows:
• Constructivist learning theory: constructivist learning theory posits that learners construct knowledge through experiences and interactions with their environment (Marougkas et al., 2023). Immersive technologies such as VR and AR align well with this theory by providing interactive and experiential learning environments. These technologies enable learners to actively engage with content, facilitating deeper understanding and retention of complex STEM concepts.
• Technology Acceptance Model (TAM): the Technology Acceptance Model explains how users come to accept and use a technology (Rejali et al., 2023). It suggests that perceived ease of use and perceived usefulness significantly influence users' attitudes toward technology. By integrating VR and AR into STEM education, this model helps explain how these technologies can be effectively adopted by both educators and students, enhancing engagement and performance.
• Self-Regulated Learning Theory: self-regulated learning (SRL) refers to the process where learners proactively manage their learning by setting goals, monitoring progress, and reflecting on outcomes (Chocarro et al., 2023). Immersive technologies support SRL by providing personalized and adaptive learning experiences. Features such as immediate feedback and interactive simulations empower students to take control of their learning process, promoting autonomy and motivation.
• SAMR Model: the Substitution, Augmentation, Modification, Redefinition (SAMR) model provides a framework for integrating technology into education (Hamilton et al., 2016). It categorizes the use of technology into four levels, from the basic substitution of traditional methods to the transformative redefinition of learning tasks. This model helps in evaluating how VR and AR can be used to not only enhance but also transform STEM education practices.
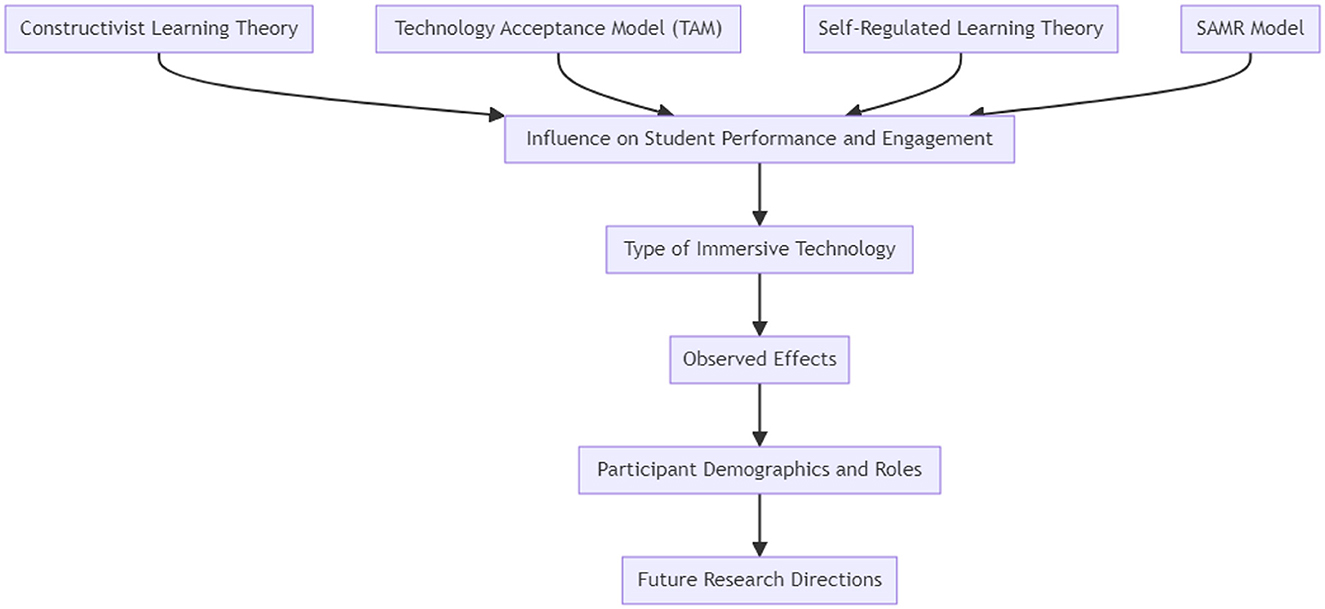
Figure 6. Illustration of how various theoretical frameworks are integrated into the systematic review. These frameworks influence key aspects of the study, such as student performance and engagement, type of immersive technology, observed effects, and future research directions.
By grounding the current systematic review in these theories, one can better interpret how immersive technologies impact student engagement and performance in STEM education. This theoretical framework provides a robust foundation for our systematic review, ensuring that our findings are contextualized within established educational paradigms.
4 Discussions
4.1 Main contributions to the knowledge
The 22 works analyzed provide a multifaceted perspective on the adoption and impact of AR, VR, XR, and MR in different educational contexts, as follows:
• Nadan et al. (2011) contributed to the understanding of VR's memorability, laying foundational work for its potential to enhance learning experiences in STEM.
• Nagata et al. (2017) offered a pioneering application of AR in mobile learning, enhancing the comprehension of STEM disciplines in real-world contexts.
• Hsu et al. (2017) examined AR's impact on stimulating interest in STEM fields through medical surgery simulations, addressing the need for engagement in high school students.
• Abd Majid and Abd Majid (2018) contributed an AR application designed to support guided discovery learning, providing an innovative approach to complex STEM subjects.
• Cooper et al. (2019) explored VR's transformative potential in classrooms, capturing pre-service teachers' perceptions and apprehensions.
• Jesionkowska et al. (2020) showed the effectiveness of AR in active STEAM learning, allowing students to create their own AR applications.
• Dayarathna et al. (2020) provided insight into the gender-based effects of VR teaching modules on learning manufacturing systems, contributing to the inclusivity in STEM education.
• Lasica et al. (2020) developed a TPD program to examine AR's impact on teaching, adding value to teachers' professional development in STEM education.
• Rowe et al. (2020) ventured into K-12 perceptions of XR and smart building sensors, pioneering the use of such technologies in early education.
• Mohammadi et al. (2019) studied the benefits of online experiential learning, particularly for workforce development and inclusion in STEM fields.
• Holly et al. (2021) focused on VR for teaching physics, addressing both the design challenges and the pedagogical benefits of immersive experiences.
• Montalbo (2021) developed an AR-enhanced TLM, providing evidence for the effectiveness of AR in enhancing spatial skills in chemistry education.
• Shu and Huang (2021) combined VR with Maker education to address COVID-19 challenges, demonstrating its positive impact on learning effectiveness and self-efficacy.
• Wu et al. (2021) integrated SSM with mixed reality to optimize RFID education, paving the way for better alignment of technology education with stakeholder needs.
• Miller et al. (2021) revealed the benefits of IVR activities on organic chemistry performance, particularly for first-generation college students.
• Hobbs and Holley (2022) discussed AR's role in curriculum redesign, emphasizing its effectiveness in engaging students and developing project-based learning skills.
• Kao and Ruan (2022) introduced an interactive AR system for programming learning, showing that high interactivity can improve learning outcomes.
• Lindner et al. (2022) leveraged AR in teaching remote sensing through the UN SDGs, illustrating how technology can make complex subjects accessible and engaging.
• Campos et al. (2022) investigated the use of VR for teaching vectors in physics, showing its potential for enhancing understanding of three-dimensional concepts.
• See et al. (2023) described a transdisciplinary approach to teaching XR solution creation, adding to the repertoire of technology education models.
• McNerney et al. (2023) piloted the SatelliteSkill5 AR app in schools, exemplifying the successful integration of AR into geography and remote sensing lessons.
• Chiang and Liu (2023) analyzed the effects of XR on students' learning responses in STEM education, providing evidence for the efficacy of XR in improving performance and engagement.
Collectively, these studies evidence the significant strides made in integrating IVT into STEM education. They highlight the diverse applications and positive outcomes associated with the use of AR, VR, XR, and MR, from enhancing spatial skills and improving knowledge retention to boosting engagement and interest in STEM subjects.
4.2 Advantages
The integration of IVT with STEM education offers a range of advantages that enhance teaching and learning experiences. These benefits can be summarized as follows:
• AR and VR have been consistently shown to increase student engagement. The immersive nature of these technologies captivates students' attention and increases their willingness to participate in learning activities. For instance, Nadan et al. (2011) and Cooper et al. (2019) documented how VR created memorable learning experiences that actively engaged students.
• The use of IVT supports complex concept visualization, making abstract STEM concepts more concrete. Abd Majid and Abd Majid (2018) demonstrated how AR can facilitate the understanding of atomic structures, enhancing students' grasp of intricate subjects.
• The novel and interactive experiences provided by IVT can inspire students to pursue STEM majors and careers. Hsu et al. (2017) found that AR increased students' interest in STEM fields, which is crucial for cultivating future scientists and engineers.
• Technologies like AR help in developing spatial reasoning, a vital skill in STEM education. Montalbo (2021) showcased that AR could significantly improve students' spatial skills in chemistry.
• IVT can accommodate various learning preferences, including auditory, visual, and kinesthetic, thus catering to a broader range of learning styles and needs. This is especially beneficial in STEM fields, where learners often face challenges with traditional teaching methods.
• VR and AR can simulate real-world environments, allowing students to practice and refine their skills in a safe and controlled setting. For example, the work by Dayarathna et al. (2020) used VR for teaching manufacturing systems concepts, providing hands-on experience without the associated risks or resource constraints.
• IVT offers flexible learning opportunities, enabling students to learn from anywhere. This was particularly evident in studies by Mohammadi et al. (2019) and Shu and Huang (2021), where VR and online experiential learning platforms facilitated education during the COVID-19 pandemic.
• IVT not only benefits students but also supports teacher education, as seen in Lasica et al. (2020), where AR was used for teacher professional development programs, enhancing their confidence and ability to teach STEM subjects.
• By simulating real-world scenarios, IVT like XR provides experiential learning opportunities that might otherwise be inaccessible. Rowe et al. (2020) explored this through the application of sensor data in smart buildings, expanding the horizons of what can be taught and experienced in a classroom.
• The integration of arts with STEM (STEAM) through IVT encourages a holistic educational approach, promoting creativity and critical thinking. Jesionkowska et al. (2020) highlighted this by having students develop their own AR applications, bridging technology with artistic expression.
• By providing scalable and potentially cost-effective solutions, IVT can democratize STEM education, making it accessible to larger and more diverse student populations.
• Integrating real-world data, like remote sensing, into classroom lessons using AR, as in the work by Lindner et al. (2022), connects students with global issues, promoting problem-solving skills related to actual environmental challenges.
4.3 Comparison with previous reviews
Our current systematic review paper builds upon and extends the foundations laid by prior works in the integration of IVT with STEM education (see Table 5), presenting a novel and comprehensive analysis of the field. Compared to previous reviews, our work stands out in several significant aspects:
• Potkonjak et al. (2016) focused on virtual laboratories, while our review encompasses a wider range of IVT applications, providing a broader understanding of immersive technologies in STEM education.
• Unlike Barakabitze et al. (2019), which concentrated on African education systems, our review has a global perspective, considering studies from a variety of international contexts and acknowledging the universal relevance of IVT in STEM education.
• While Pellas et al. (2020), Del Cerro Velázquez and Morales Méndez (2021), and Yegorina et al. (2021) reviewed specific technologies like VR and AR, our paper integrates a wider array of technologies including XR and MR, showcasing a comprehensive landscape of immersive technologies' applications.
• Yegorina et al. (2021) and McDonald et al. (2022) focused on the undergraduate and primary school levels, respectively. In contrast, our review spans all levels of education, recognizing the importance of IVT across the entire educational spectrum.
• Our systematic review paper employs an extensive, methodologically rigorous approach, enhancing the findings of Marrero-Galván and Hernández-Padrón (2022) by addressing some of the methodological deficiencies identified in their review.
• We involved an interdisciplinary approach that exceeds the work of Palacios et al. (2022) by not only discussing the importance of new technologies but also critically analyzing their pedagogical implications across various STEM disciplines.
• We delve deeper into the effects of IVT on both performance and engagement in STEM education, whereas Pellas et al. (2020) and Del Cerro Velázquez and Morales Méndez (2021) primarily focused on spatial skills and learning motivation. This allows for a more nuanced understanding of how these technologies impact educational outcomes.
• As noted by Marrero-Galván and Hernández-Padrón (2022), VR and AR are becoming increasingly popular in educational settings. Our review situates this trend within the broader context of emerging technologies, thereby underscoring the timeliness and relevance of our analysis.
In addition, four review articles have been identified that were excluded at the bigging due to the screening source algorithm. As noted, these articles highlight the importance of various approaches to learning with immersive virtual reality (Won et al., 2023). As well as Mystakidis et al. (2022) also provide a systematic mapping review of AR applications in higher education, identifying research gaps in Technology and Mathematics, a lack of location-based and markerless AR studies, and presenting a taxonomy of instructional models and strategies. Ibáñez and Delgado-Kloos (2018) conducted a systematic review of AR in STEM education, discussing its general characteristics, instructional strategies, evaluation approaches, and highlighting the predominance of exploration and simulation activities while emphasizing the need for more instructional support. Sirakaya and Alsancak Sirakaya (2022) review the use of AR in STEM education, assessing its advantages and challenges, and focusing on the role of AR in enhancing learning outcomes by integrating complex STEM concepts with practical applications.
Then, the novelty of our present work lies in its expansive and integrative review methodology that provides a synthesized understanding of the cumulative evidence on the application and efficacy of IVT in STEM education. It contextualizes the rapid evolution of these technologies and their pedagogical implications, offering new insights into their effectiveness and providing a forward-looking perspective on future research and practice.
4.4 Strategies to enhance student engagement and motivation
Enhancing student engagement and motivation is crucial for the effective integration of immersive technologies such as AR, VR, and MR in STEM education. Based on the database of our systematic review, we have identified several strategies that leverage gamification, interactive simulations, and personalized learning experiences to improve engagement and learning outcomes. Here, we provide a synthesis of suggested strategies:
• Develop powerful models for understanding scientific and mathematical concepts in K-12 education. Utilize scaffolding, visualizations, animations, and computer-based tools to help students construct, revise, and utilize mental models (Seel, 2017).
• Implement mixed reality (MR) systems in lab activities to provide an intuitive understanding of complex scientific concepts, enhance accessibility, and foster collaboration among students (Barrett et al., 2018).
• Use virtual reality (VR) simulations to create immersive and interactive learning experiences that enhance student engagement, learning attitude, and enjoyment, especially in non-STEM subjects (Sung et al., 2020).
• Integrate augmented reality (AR) applications in primary school curricula to foster collaborative and gamified learning experiences, making subjects like geometry and geography more engaging and interactive (Yegorina et al., 2021).
• Incorporate computational and simulation tools in undergraduate curricula to develop algorithmic thinking and problem-solving skills, transforming students from users to developers of innovative computational solutions (McDonald et al., 2022).
• Use gamification and ICT tools in geometry teaching to create an engaging and interactive learning environment, utilizing AR and VR to visualize concepts and motivate students through gamified elements like points and badges (Moral-Sánchez et al., 2022).
• Design and develop interactive learning environments using multimedia elements, such as 2D and 3D graphics, videos, and AR, to enhance understanding and retention of complex biological processes in subjects like animal developmental biology (Ramli et al., 2022).
• Develop AR serious games to introduce and motivate young children toward subjects like chemistry, using tangible interfaces and storytelling to make learning fun and informative (Olim et al., 2023).
• Utilize advanced technologies such as AR, CAD software, 3D printing, and GPS in mathematics education to make abstract concepts more tangible and applicable to real-world situations, encouraging creativity and problem-solving (Haas et al., 2023).
• Implement gamification and extended reality technologies in courses to enhance student engagement and motivation, using VR and AR to create immersive and interactive learning experiences (Iacono, 2023).
• Integrate advanced technologies such as robotics and augmented reality into educational video games to enhance learning outcomes and engagement for preschool children in STEM subjects (Méndez-Porras et al., 2021).
• To boost engagement and motivation in STEM education with immersive technologies, design activities that let students actively interact with content, like using VR controllers for virtual experiments. Create immersive, high-quality experiences that make students feel present and in control. Use VR for tasks that benefit from 3D perspectives, such as visualizing molecules or exploring engineering designs (Johnson-Glenberg et al., 2021).
4.5 Implications and suggestions for educators, developers, and policymakers
The integration of immersive technologies such as AR, VR, and MR into STEM education has profound implications for various participants. To maximize the benefits of these technologies, we propose the following points (see Figure 7):
• For educators:
∘ Curriculum integration:
• Integrate AR and VR tools into the curriculum to create interactive and engaging learning experiences. For example, AR can be used to visualize complex scientific concepts, enhancing students' understanding and retention.
• Develop lesson plans that incorporate immersive simulations and virtual labs, allowing students to experiment and explore concepts in a safe, controlled environment.
∘ Professional development:
• Participate in professional development programs focused on the effective use of immersive technologies in the classroom. Training should cover both the technical aspects and pedagogical strategies to leverage these tools for maximum impact.
• Collaborate with other educators to share best practices and successful case studies of AR and VR integration in STEM education.
∘ Student engagement and motivation:
• Use VR environments to create immersive and realistic scenarios that can motivate students and increase their engagement in STEM subjects. For instance, VR can be used for virtual field trips or complex laboratory experiments that are otherwise inaccessible.
• Encourage students to create their own AR and VR content as part of project-based learning, fostering creativity and deeper understanding.
• For technology developers:
∘ User-centric design:
∗ Develop AR and VR educational tools that are user-friendly and accessible to both educators and students. The interface should be intuitive, requiring minimal training for effective use.
∗ Incorporate feedback from educators and students into the design and development process to ensure the tools meet the practical needs of the classroom.
∘ Content relevance and quality:
• Ensure that the educational content delivered through immersive technologies is aligned with curriculum standards and learning objectives. The content should be accurate, up-to-date, and pedagogically sound.
• Provide comprehensive support materials, including user guides, lesson plans, and troubleshooting resources to assist educators in integrating these technologies into their teaching.
∘ Scalability and adaptability:
• Design scalable solutions that can be easily integrated into various educational settings, from primary schools to universities. The technology should be adaptable to different subjects and educational levels.
• Develop modular content that allows educators to customize and adapt the technology to their specific teaching needs and objectives.
• For policymakers:
∘ Infrastructure and funding:
• Allocate funding for the acquisition and maintenance of AR and VR technologies in schools. Ensure that educational institutions have the necessary infrastructure, including high-speed internet and compatible hardware, to support these technologies.
• Provide grants and subsidies to support schools and educators in adopting and integrating immersive technologies into their teaching practices.
∘ Policy development:
• Develop policies that encourage the adoption of immersive technologies in education. This includes setting standards for the quality and use of AR and VR tools in the classroom.
• Ensure that policies address equity and access, making sure that all involved students, regardless of socio-economic background, can benefit from immersive technologies.
∘ Collaboration and research:
• Promote collaboration between educational institutions, technology developers, and research organizations to explore the potential of immersive technologies in education. Support research initiatives that investigate the long-term impacts of these technologies on learning outcomes and student engagement?.
• Encourage the publication and dissemination of research findings to inform policy and practice, ensuring that the latest evidence-based insights are available to educators and policymakers.
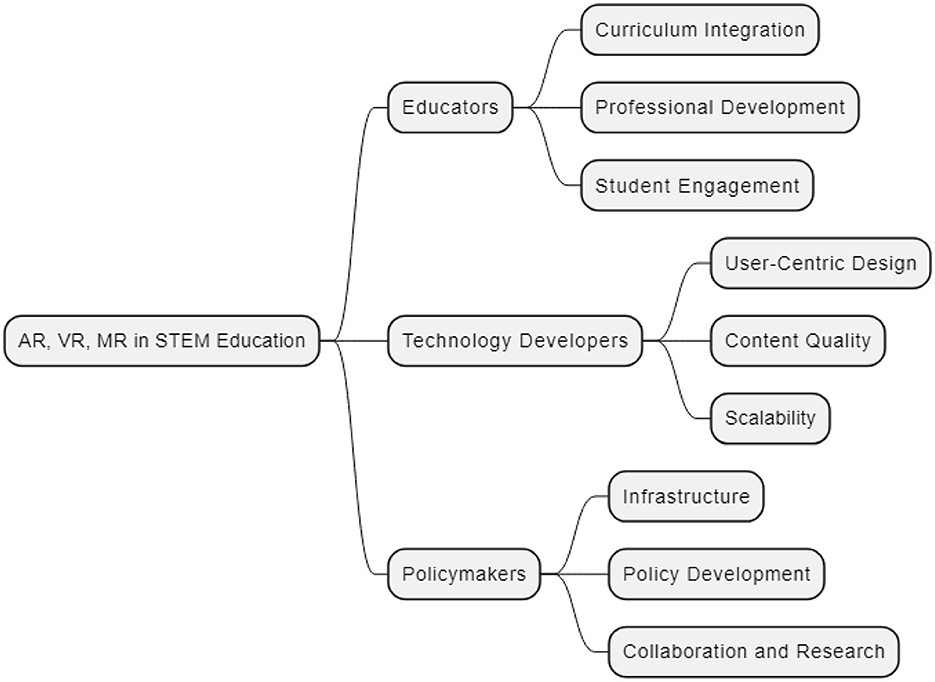
Figure 7. This illustration highlights key implications and suggestions for integrating AR, VR, and MR into STEM education. It outlines roles for educators, technology developers, and policymakers.
By addressing these proposals, educators, technology developers, and policymakers can work together to effectively integrate immersive technologies into STEM education, enhancing learning experiences and outcomes for students.
4.6 Limitations
In our systematic review focused on the integration of IVT within STEM education, we navigated a dense landscape of scholarly work. Despite our comprehensive search strategy, there remains the possibility that essential studies could have been inadvertently overlooked. The inherent complexities of defining precise search terms and selecting relevant keywords mean that some significant contributions might not have been captured due to the limitations of our methodology and the constraints of our review timeline. For instance, the recent work by Christopoulos et al. (2024) examined the impact of 3D virtual game immersion on mathematical skills in a game-based learning context. This study, involving 59 Greek high school students, compared traditional methods with a four-week 3D virtual learning environment.
Our inquiry was also bound by predetermined selection criteria, specifically limiting the review to peer-reviewed journal articles. This decision resulted in the exclusion of pertinent research published in conference proceedings or presented in alternative academic forums, thereby potentially narrowing the scope of international insights into the field. For example, the works by Nersesian et al. (2019) and Villanueva et al. (2020) where the authors highlight the importance of immersive technologies in the STEM learning process.
4.7 Recommendation for future research
To fully harness the potential of immersive technologies in STEM education, it is imperative to develop a universally recognized framework. This framework should outline the critical components of VR and AR as they pertain to learner-centered methodologies within STEM disciplines, establishing clear principles and standardized definitions for their application across diverse educational contexts. Additionally, this framework must be adaptable, evolving with technological advancements and insights from educational psychology:
• Long-term effects on learning outcomes: future research should focus on the long-term effects of immersive technologies on learning outcomes. While current studies provide valuable insights into immediate benefits, understanding how these technologies impact students' knowledge retention, skills development, and academic progression over extended periods is crucial. Longitudinal studies that track student performance and engagement with immersive technologies over several years will be particularly valuable in this regard.
• Scalability in different educational contexts: investigating the scalability of immersive technologies across various educational contexts is another vital area for future research. Studies should explore how VR and AR can be effectively implemented in diverse settings, including primary and secondary schools, higher education institutions, and vocational training programs. Research should also address the challenges and opportunities associated with scaling these technologies, such as infrastructure requirements, cost-effectiveness, and teacher training.
• Integration of pedagogical strategies: building on the findings of researchers like Nadan et al. (2011) and Nagata et al. (2017), future systematic reviews should conduct exhaustive examinations of how pedagogical strategies in VR and AR can be integrated effectively. Particular attention should be paid to enhancing student engagement and accommodating individual learning preferences. Identifying best practices for incorporating immersive technologies into STEM curricula will provide educators with practical guidance on maximizing their educational impact.
• Adaptive and personalized learning models: developing and assessing adaptive educational models that utilize VR and AR as more than just interactive tools is essential. These models should cater to the unique learning styles and needs of students, offering personalized learning experiences that adapt to individual progress and preferences. Future research should explore how adaptive technologies can support differentiated instruction and personalized learning pathways in STEM education.
• Ethical implications and privacy concerns: as VR and AR technologies continue to permeate STEM education, addressing their ethical implications and privacy concerns becomes increasingly important. Future research must thoroughly investigate data management practices within simulations and ensure the ethical deployment of these technologies for student assessments and educational experiences. Maintaining ethical integrity and privacy protections will be paramount as immersive technologies become more integrated into educational environments.
• Evaluation of VR and AR platforms: a critical evaluation of current VR and AR platforms and systems used in STEM contexts is essential. Research should aim to discern which systems align best with diverse educational goals and user needs. Identifying the most effective educational practices and systems will provide invaluable guidance for educators and institutions when selecting and integrating VR and AR technologies into STEM curricula. Comparative studies that evaluate the effectiveness of different platforms and their impact on student outcomes will be particularly beneficial.
By addressing these areas, future research can provide insights into the effective integration of immersive technologies in STEM education, ensuring their long-term success and sustainability.
5 Conclusions
This systematic review comprehensively examined the integration of immersive technologies into STEM education. The main findings indicate that these technologies are not only increasingly being adopted in educational settings but are also making a significant impact on both student engagement and performance. Specifically, AR emerged as the most prevalent technology in the studies examined, featured in half of the analyzed articles, suggesting its more accessible implementation within educational frameworks.
Quantitative analyses revealed that 72.73% of the studies reported positive outcomes on learning experiences, with a further 22.73% noting increased proficiency in STEM-related skills among students. These results underscore the efficacy of immersive technologies in enriching STEM education by making abstract concepts more tangible and adopting a deeper understanding through experiential learning.
Looking ahead, the field of immersive technology in education is ripe with potential and evolving rapidly. However, to harness this potential fully, the review highlights the need for a universally recognized framework for the application of VR and AR in education. Such a framework would not only consolidate the best practices observed but also guide future application and development. Additionally, it would need to remain adaptable to accommodate technological advances and emerging pedagogical insights.
Given the intrinsic limitations of the review, which included a focus on English-language peer-reviewed journal articles, future research should aim for a more inclusive approach. By expanding the scope to incorporate multilingual studies and diverse academic channels, subsequent reviews could offer a more holistic and global perspective. Moreover, there is a critical need for future research to address the adaptability and personalization of VR and AR technologies, ensuring that they meet the individual needs of learners and uphold ethical standards in data management and privacy.
Lastly, immersive technologies like VR and AR hold the promise of transforming STEM education, as evidenced by the positive impacts on student engagement and performance reported in the literature. By continuing to build on these technologies with a mindful approach toward inclusivity, adaptability, and ethical considerations, educators and researchers can propel STEM education toward a more interactive, personalized, and effective future.
Author contributions
TT: Conceptualization, Data curation, Formal analysis, Funding acquisition, Investigation, Methodology, Project administration, Resources, Software, Supervision, Validation, Visualization, Writing – original draft, Writing – review & editing. JM: Data curation, Formal analysis, Methodology, Writing – original draft, Writing – review & editing. MP: Data curation, Formal analysis, Methodology, Writing – original draft, Writing – review & editing. MM: Data curation, Formal analysis, Methodology, Writing – original draft, Writing – review & editing. CV: Conceptualization, Data curation, Formal analysis, Funding acquisition, Investigation, Methodology, Project administration, Resources, Software, Supervision, Validation, Visualization, Writing – original draft, Writing – review & editing. SB: Conceptualization, Data curation, Formal analysis, Funding acquisition, Investigation, Methodology, Project administration, Resources, Software, Supervision, Validation, Visualization, Writing – original draft, Writing – review & editing.
Funding
The author(s) declare financial support was received for the research, authorship, and/or publication of this article. This work has been supported by the Universidad Técnica Particular de Loja (Grant no. POA_VIN-56).
Acknowledgments
The authors would like to thank Corporación Ecuatoriana para el Desarrollo de la Investigación y Academia—CEDIA for the support given to the present research, development, and innovation work through its CEPRA program, especially for the “Proyecto 18–Tecnologias Inmersivas” fund.
Conflict of interest
The authors declare that the research was conducted in the absence of any commercial or financial relationships that could be construed as a potential conflict of interest.
Publisher's note
All claims expressed in this article are solely those of the authors and do not necessarily represent those of their affiliated organizations, or those of the publisher, the editors and the reviewers. Any product that may be evaluated in this article, or claim that may be made by its manufacturer, is not guaranteed or endorsed by the publisher.
References
Abd Majid, N. A., and Abd Majid, N. (2018). Augmented reality to promote guided discovery learning for STEM learning. J. ICT Res. Appl. 8, 1494–1500. doi: 10.18517/ijaseit.8.4-2.6801
Amir-Behghadami, M., and Janati, A. (2020). Population, intervention, comparison, outcomes and study (PICOS) design as a framework to formulate eligibility criteria in systematic reviews. Emerg. Med. J. 37:387. doi: 10.1136/emermed-2020-209567
Barakabitze, A. A., Lazaro, A. W.-A., Ainea, N., Mkwizu, M. H., Maziku, H., Matofali, A. X., et al. (2019). Transforming African education systems in science, technology, engineering, and mathematics (STEM) using ICTs: challenges and opportunities. Educ. Res. Int. 2019:6946809. doi: 10.1155/2019/6946809
Barrett, R., Gandhi, H. A., Naganathan, A., Daniels, D., Zhang, Y., Onwunaka, C., et al. (2018). Social and tactile mixed reality increases student engagement in undergraduate lab activities. J. Chem. Educ. 95, 1755–1762. doi: 10.1021/acs.jchemed.8b00212
Campos, E. H., Hidrogo, I., and Zavala, G. (2022). Impact of virtual reality use on the teaching and learning of vectors. Front. Educ. 7:965640. doi: 10.3389/feduc.2022.965640
Chiang, Y.-C., and Liu, S.-C. (2023). The effects of extended reality technologies in STEM education on students' learning response and performance. J. Balt. Sci. Educ. 22, 568–578. doi: 10.33225/jbse/23.22.568
Chocarro, R., Cortinas, M., and Marcos-Matás, G. (2023). Teachers' attitudes towards chatbots in education: a technology acceptance model approach considering the effect of social language, bot proactiveness, and users' characteristics. Educ. Stud. 49, 295–313. doi: 10.1080/03055698.2020.1850426
Christopoulos, A., Mystakidis, S., Kurczaba, J., Laakso, M. J., and Stylios, C. (2024). Is immersion in 3D virtual games associated with mathematical ability improvement in game-based learning? Int. J. Sci. Math. Educ. 1–21. doi: 10.1007/s10763-023-10440-4
Çoban, M., Akçay, N. O., and Çelik, I. (2022). Using virtual reality technologies in STEM education: ICT pre-service teachers' perceptions. Knowl. Manag. E-Learn. 14, 269–285. doi: 10.34105/j.kmel.2022.14.015
Cooper, G., Park, H., Nasr, Z., Thong, L. P., and Johnson, R. (2019). Using virtual reality in the classroom: pre-service teachers' perceptions of its use as a teaching and learning tool. Educ. Media Int. 56, 1–13. doi: 10.1080/09523987.2019.1583461
Cruz, J., Coluci, V. R., and Moraes, R. (2023). ORUN-VR2: a VR serious game on the projectile kinematics: design, evaluation, and learning outcomes. Virtual Real. 27, 2583–2604. doi: 10.1007/s10055-023-00824-w
Dayarathna, V. L., Karam, S., Jaradat, R., Hamilton, M. A., Nagahi, M., Joshi, S., et al. (2020). Assessment of the efficacy and effectiveness of virtual reality teaching module: a gender-based comparison. Int. J. Eng. Educ. 36, 1938–1955.
Del Cerro Velázquez, F., and Morales Méndez, G. (2021). Systematic review of the development of spatial intelligence through augmented reality in STEM knowledge areas. Mathematics 9:3067. doi: 10.3390/math9233067
Deng, N., Soh, K. G., Abdullah, B. B., Huang, D., Xu, F., Bashir, M., et al. (2024). Effects of plyometric training on health-related physical fitness in untrained participants: a systematic review and meta-analysis. Sci. Rep. 14:11272. doi: 10.1038/s41598-024-61905-7
Franco, A., Ditonno, F., Manfredi, C., Pellegrino, A. A., Licari, L. C., Bologna, E., et al. (2024). Single port robot-assisted radical and simple prostatectomy: a systematic review and meta-analysis. Prostate Cancer Prostatic Dis. 1–12. doi: 10.1038/s41391-024-00787-2
Freese, M., Teichrew, A., Winkelmann, J., Erb, R., Ullrich, M., Tremmel, M., et al. (2023). Measuring teachers' competencies for a purposeful use of augmented reality experiments in physics lessons. Front. Educ. 8:1180266. doi: 10.3389/feduc.2023.1180266
Haas, B., Lavicza, Z., Houghton, T., and Kreis, Y. (2023). Can you create? Visualising and modelling real-world mathematics with technologies in STEAM educational settings. Curr. Opin. Behav. Sci. 52:101297. doi: 10.1016/j.cobeha.2023.101297
Hamilton, E. R., Rosenberg, J. M., and Akcaoglu, M. (2016). The substitution augmentation modification redefinition (SAMR) model: a critical review and suggestions for its use. TechTrends 60, 433–441. doi: 10.1007/s11528-016-0091-y
Hobbs, M. H., and Holley, D. A. (2022). Radical approach to curriculum design: engaging students through augmented reality. Int. J. Mob. Blended Learn. 14:17. doi: 10.4018/IJMBL.313595
Holly, M., Pirker, J., Resch, S., Brettschuh, S., Gütl, C., and Designing, V. R. (2021). Experiences – expectations for teaching and learning in VR. Educ. Technol. Soc. 24, 107–119. Available online at: https://www.jstor.org/stable/27004935
Hsu, Y.-S., Lin, Y.-H., and Yang, B. (2017). Impact of augmented reality lessons on students' STEM interest. Res. Pract. Technol. Enhanc. Learn. 12:2. doi: 10.1186/s41039-016-0039-z
Iacono, S. (2023). Augmented learning: case study of gamified and extended reality courses. Eng. Proc. 38:13. doi: 10.3390/engproc2023038013
Ibáñez, M. B., and Delgado-Kloos, C. (2018). Augmented reality for STEM learning: a systematic review. Comput. Educ. 123, 109–123. doi: 10.1016/j.compedu.2018.05.002
Iqbal, M. Z., Mangina, E., and Campbell, A. G. (2022). Current challenges and future research directions in augmented reality for education. Multimodal Technol. Interact. 6:75. doi: 10.3390/mti6090075
Jesionkowska, J., Wild, F., and Deval, Y. (2020). Active learning augmented reality for STEAM education—a case study. Educ. Sci. 10:198. doi: 10.3390/educsci10080198
Johnson-Glenberg, M. C., Bartolomea, H., and Kalina, E. (2021). Platform is not destiny: embodied learning effects comparing 2D desktop to 3D virtual reality STEM experiences. J. Comput. Assist. Learn. 37, 1263–1284. doi: 10.1111/jcal.12567
Kao, G. Y.-M., and Ruan, C.-A. (2022). Designing and evaluating a high interactive augmented reality system for programming learning. Comput. Hum. Behav. 132:107245. doi: 10.1016/j.chb.2022.107245
Kassim, M., and Zubir, M. (2019). Design of augmented reality for engineering equipment in education. Int. J. Adv. Trends Comput. Sci. Eng. 8, 2773–2781. doi: 10.30534/ijatcse/2019/15862019
Kazanidis, I., and Pellas, N. (2019). Developing and assessing augmented reality applications for mathematics with trainee instructional media designers: an exploratory study on user experience. J. Univers. Comput. Sci. 25, 489–514.
Lasica, I.-E., Meletiou-Mavrotheris, M., and Katzis, K. (2020). Augmented reality in lower secondary education: a teacher professional development program in Cyprus and Greece. Educ. Sci. 10:121. doi: 10.3390/educsci10040121
Li, S., Shen, Y., Jiao, X., and Cai, S. (2022). Using augmented reality to enhance students' representational fluency: the case of linear functions. Mathematics 10:1718. doi: 10.3390/math10101718
Lin, P. H., Huang, Y. M., and Chen, C. C. (2018). Exploring imaginative capability and learning motivation difference through picture E-book. IEEE Access 6, 63416–63425. doi: 10.1109/ACCESS.2018.2875675
Lindner, C., Rienow, A., Otto, K.-H., and Juergens, C. (2022). Development of an app and teaching concept for implementation of hyperspectral remote sensing data into school lessons using augmented reality. Remote Sens. 14:791. doi: 10.3390/rs14030791
Mahanan, M. S., Ibrahim, N. H., Surif, J., and Nee, C. K. (2021). AR module for learning changes of matter in chemistry. Int. J. Interact. Mob. Technol. 25, 489–514. doi: 10.3991/ijim.v15i23.27343
Marougkas, A., Troussas, C., Krouska, A., and Sgouropoulou, C. (2023). Virtual reality in education: a review of learning theories, approaches and methodologies for the last decade. Electronics 12:2832. doi: 10.3390/electronics12132832
Marques, M. M., and Pombo, L. (2021). The impact of teacher training using mobile augmented reality games on their professional development. Educ. Sci. 11:404. doi: 10.3390/educsci11080404
Marrero-Galván, J. J., and Hernández-Padrón, M. (2022). The importance of virtual reality in STEM education: a systematic review from the point of view of experimentation in the classroom. Bordón 74, 45–63. doi: 10.13042/Bordon.2022.94179
McDonald, A. R., Roberts, R., Koeppe, J. R., and Hall, B. L. (2022). Undergraduate structural biology education: a shift from users to developers of computation and simulation tools. Curr. Opin. Struct. Biol. 72, 39–45. doi: 10.1016/j.sbi.2021.07.012
McNerney, E., Faull, J., Brown, S., McNerney, L., Foley, R., Lonergan, J., et al. (2023). SatelliteSkill5—an augmented reality educational experience teaching remote sensing through the UN sustainable development goals. Remote Sens. 15:5480. doi: 10.3390/rs15235480
Meccawy, M. (2023). Teachers' prospective attitudes towards the adoption of extended reality technologies in the classroom: interests and concerns. Smart Learn. Environ. 10:36. doi: 10.1186/s40561-023-00256-8
Méndez-Porras, A., Alfaro-Velasco, J., and Rojas-Guzmán, R. (2021). Educational video games for girls and boys in preschool education using robotics and augmented reality. Aula Abierta 50, 482–495. doi: 10.17811/rifie.50.1.2021.482-495
Miller, M. D., Castillo, G., Medoff, N., and Hardy, A. (2021). Immersive VR for organic chemistry: impacts on performance and grades for first-generation and continuing-generation university students. Innov. High. Educ. 46, 565–589. doi: 10.1007/s10755-021-09551-z
Mohammadi, A., Grosskopf, K., and Killingsworth, J. (2019). Workforce development through online experiential learning for STEM education. Adult Learn. 31, 27–35. doi: 10.1177/1045159519854547
Montalbo, S. M. (2021). eS2MART teaching and learning material in chemistry: enhancing spatial skills thru augmented reality technology. Palawan Sci. 13, 14–30.
Moral-Sánchez, S. N., Sánchez-Compaña, M. T., and Romero, I. (2022). Geometry with a STEM and gamification approach: a didactic experience in secondary education. Mathematics 10:3252. doi: 10.3390/math10183252
Mystakidis, S., Christopoulos, A., and Pellas, N. (2022). A systematic mapping review of augmented reality applications to support STEM learning in higher education. Educ. Inf. Technol. 27, 1883–1927. doi: 10.1007/s10639-021-10682-1
Nadan, T., Alexandrov, V. N., Jamieson, R., and Watson, K. A. (2011). Is virtual reality a memorable experience in an educational context? Int. J. Emerg. Technol. Learn. 6, 53–57. doi: 10.3991/ijet.v6i1.1433
Nagata, J., García-Bermejo Giner, J., and Martínez Abad, F. (2017). Augmented reality in pedestrian navigation applied in a context of mobile learning: resources for enhanced comprehension of science, technology, engineering and mathematics. Int. J. Eng. Educ. 33, 768–780.
Nersesian, E., Spryszynski, A., and Lee, M. J. (2019). “Integration of virtual reality in secondary STEM education,” in 2019 IEEE Integrated STEM Education Conference (ISEC) (Princeton, NJ: IEEE), 83–90. doi: 10.1109/ISECon.2019.8882070
Olim, S. C., Nisi, V., and Romão, T. (2023). Periodic fable discovery: an augmented reality serious game to introduce and motivate young children towards chemistry. Multimed. Tools Appl. 83, 52593–52619. doi: 10.1007/s11042-023-17526-9
Page, M., McKenzie, J., Bossuyt, P., Boutron, I., Hoffmann, T., Mulrow, C., et al. (2021). The PRISMA 2020 statement: an updated guideline for reporting systematic reviews. Int. J. Surg. 88:105906. doi: 10.1016/j.ijsu.2021.105906
Palacios, A., Pascual, V., and Moreno-Mediavilla, D. (2022). The role of new technologies in STEM education. Bordón 74, 11–21. doi: 10.13042/Bordon.2022.96550
Pellas, N., Dengel, A., and Christopoulos, A. A. (2020). Scoping review of immersive virtual reality in STEM education. IEEE Trans. Learn. Technol. 13, 748–761. doi: 10.1109/TLT.2020.3019405
Potkonjak, V., Petrović, V. M., Gardner, M., Jovanović, K., Callaghan, V., Mattila, P., et al. (2016). Virtual laboratories for education in science, technology, and engineering: a review. Comput. Educ. 95, 309–327. doi: 10.1016/j.compedu.2016.02.002
Ramli, R. Z., Sahari Ashaari, N., Mat Noor, S. F., Mat Noor, M., Abd Majid, N. A., Dahlan, H. A., et al. (2022). Assessing usability of learning experience prototype. Int. J. Emerg. Technol. Learn. 17:29955. doi: 10.3991/ijet.v17i09.29955
Rejali, S., Aghabayk, K., Esmaeli, S., and Shiwakoti, N. (2023). Comparison of technology acceptance model, theory of planned behavior, and unified theory of acceptance and use of technology to assess a priori acceptance of fully automated vehicles. Transp. Res. A Policy Pract. 168:103565. doi: 10.1016/j.tra.2022.103565
Rogers, C. B., El-Mounayri, H., Wasfy, T., and Satterwhite, J. (2018). Assessment of STEM e-learning in an immersive virtual reality (VR) environment. ASEE. 8:4.
Rowe, S., Riggio, M., De Amicis, R., and Rowe, S. R. (2020). Teacher perceptions, of training and pedagogical value of cross-reality and sensor data from smart buildings. Educ. Sci. 10:234. doi: 10.3390/educsci10090234
See, Z. S., Ledger, S., Goodman, L., Matthews, B., Jones, D., Fealy, S., et al. (2023). Playable experiences through technologies: opportunities and challenges for teaching simulation learning and extended reality solution creation. J. Inf. Technol. Educ. Innov. Pract. 22, 67–90. doi: 10.28945/5121
Seel, N. M. (2017). Model-based learning: a synthesis of theory and research. Educ. Tech. Res. Dev. 65, 931–966. doi: 10.1007/s11423-016-9507-9
Sharma, B., and Mantri, A. (2019). Augmented reality underpinned instructional design (ARUIDs) for cogno-orchestrative load. J. Comput. Theor. Nanosci. 16, 4379–4388. doi: 10.1166/jctn.2019.8529
Shirazi, A., and Behzadan, A. H. (2018). Content delivery using augmented reality to enhance students' performance in a building design and assembly project. Adv. Eng. Educ. 4:3.
Shu, Y., and Huang, T.-C. (2021). Identifying the potential roles of virtual reality and STEM in maker education. J. Educ. Res. 114, 108–118. doi: 10.1080/00220671.2021.1887067
Sirakaya, M., and Alsancak Sirakaya, D. (2022). Augmented reality in STEM education: a systematic review. Interact. Learn. Environ. 30, 1556–1569. doi: 10.1080/10494820.2020.1722713
Sumardani, D., Sipayung, E. R., and Chiu, P.-S. (2023). Enabling spatial thinking through an augmented reality for teaching crystal structure. Innov. Educ. Teach. Int. 60, 555–566. doi: 10.1080/14703297.2022.2076716
Sung, B., Mergelsberg, E., Teah, M., D'Silva, B., and Phau, I. (2020). The effectiveness of a marketing virtual reality learning simulation: a quantitative survey with psychophysiological measures. Br. J. Educ. Technol. 1–18. doi: 10.1111/bjet.13003
Villanueva, A., Zhu, Z., Liu, Z., Peppler, K., Redick, T., Ramani, K., et al. (2020). “Meta-AR-app: an authoring platform for collaborative augmented reality in STEM classrooms,” in Proceedings of the 2020 CHI conference on human factors in computing systems (New York, NY: ACM), 1–14. doi: 10.1145/3313831.3376146
Won, M., Ungu, D. A. K., Matovu, H., Treagust, D. F., Tsai, C. C., Park, J., et al. (2023). Diverse approaches to learning with immersive virtual reality identified from a systematic review. Comput. Educ. 195:104701. doi: 10.1016/j.compedu.2022.104701
Wu, C. H., Tang, Y. M., Tsang, Y. P., and Chau, K. Y. (2021). Immersive learning design for technology education: a soft systems methodology. Front. Psychol. 12:745295. doi: 10.3389/fpsyg.2021.745295
Keywords: immersive technologies, virtual reality, augmented reality, stem education, PICOS approach, PRISMA guidelines, teaching and learning
Citation: Tene T, Marcatoma Tixi JA, Palacios Robalino MdL, Mendoza Salazar MJ, Vacacela Gomez C and Bellucci S (2024) Integrating immersive technologies with STEM education: a systematic review. Front. Educ. 9:1410163. doi: 10.3389/feduc.2024.1410163
Received: 31 March 2024; Accepted: 27 May 2024;
Published: 20 June 2024.
Edited by:
Stylianos Mystakidis, Hellenic Open University, GreeceReviewed by:
Yunus Doğan, Firat University, TürkiyeCristian Merino, Pontificia Universidad Católica de Valparaíso, Chile
Copyright © 2024 Tene, Marcatoma Tixi, Palacios Robalino, Mendoza Salazar, Vacacela Gomez and Bellucci. This is an open-access article distributed under the terms of the Creative Commons Attribution License (CC BY). The use, distribution or reproduction in other forums is permitted, provided the original author(s) and the copyright owner(s) are credited and that the original publication in this journal is cited, in accordance with accepted academic practice. No use, distribution or reproduction is permitted which does not comply with these terms.
*Correspondence: Talia Tene, dGJ0ZW5lQHV0cGwuZWR1LmVj; Cristian Vacacela Gomez, dmFjYWNlbGFAbG5mLmluZm4uaXQ=; Stefano Bellucci, YmVsbHVjY2lAbG5mLmluZm4uaXQ=