- School of Engineering and Sciences, Tecnológico de Monterrey, Mexico City, Mexico
Introduction: In contemporary educational philosophy, constructivist and constructionist theories emphasize active knowledge construction among learners. These paradigms advocate for learners as active participants, engaging in knowledge creation through interaction and experience. Problem-Based Learning (PBL) exemplifies these principles by placing students at the center of hands-on challenges that foster critical thinking and collaborative problem-solving. This study explores the integration of these educational theories through an innovative pedagogical framework, focusing on the development of bioinspired robotics.
Methods: In a six-stage educational process at Tecnológico de Monterrey, 24 undergraduates were immersed in bioinspired robotics through workshops on animal biomechanics, soft robotics, and neuroscience. Organized into four teams, students designed robots for wildlife observation and search and rescue. The curriculum integrated theoretical lectures, hands-on training, and practical applications, emphasizing interdisciplinary collaboration and real-world problem-solving. This approach blended traditional education with innovative, project-based learning tailored to local and ecological challenges.
Results: The culmination of this initiative was marked by the creation of functional robotic prototypes, underscoring the effectiveness of the interdisciplinary approach. Post-project evaluations revealed marked enhancements in students' proficiency in both sciences and engineering. Furthermore, there was a pronounced strengthening of students' conviction regarding the importance of integrating bioinspired principles into engineering education.
Discussion: The study advocates merging traditional knowledge with modern technological innovation and bioinspiration to enhance learning outcomes. This integrated approach boosts analytical reasoning and scientific skills while also fostering empathy and emotional literacy, preparing students as holistic thinkers and versatile innovators. Combining biology, robotics, and education offers significant benefits, providing insights for educators and policymakers to equip students for future challenges. There is a strong consensus on the value of incorporating biological principles into engineering education, signaling a shift toward innovative, interdisciplinary curricula that enhance technical and broader cognitive skills.
1 Introduction
In the dynamic field of contemporary educational philosophies, the constructivist and constructionist theories stand as significant paradigms, emphasizing active knowledge construction by learners (Alanazi, 2019). Constructivism posits that learners are not passive recipients of information but active participants in their learning, creating knowledge through interaction and experience (Ben-Ari, 1998). Knowledge acquisition, under this paradigm, is a vibrant process where learners actively assemble, refine, and contextualize information. Constructionism, building on constructivist principles, adds a unique dimension to this model. It asserts that learning is most effective when students are involved in creating tangible artifacts (Ackermann, 2001). This philosophy goes beyond intellectual engagement, advocating for the physical manifestation of learning through the creation of objects or projects (Alimisis and Kynigos, 2009). This hands-on approach enriches the learning experience, allowing learners to not only conceptualize but also physically realize their ideas, thereby deepening their understanding and retention of knowledge.
Problem-Based Learning (PBL) exemplifies these principles well, particularly aligning with the experiential, critical thinking, and collaborative tenets of constructivism and constructionism. In PBL, students are at the center of their learning journey, confronted with realistic, hands-on challenges that require active problem-solving (Yew and Goh, 2016). This approach transcends conventional learning by fostering an environment where students dissect, engage with, and often redefine problems, enhancing their cognitive and practical skills. Furthermore, PBL inherently cultivates teamwork and collaboration. As students navigate through complex, real-world problems, they merge diverse perspectives and skills, improving their collective problem-solving abilities (McGrath, 2004). This collaborative aspect of PBL not only enriches individual learning and problem-solving skills but also prepares students for the collaborative dynamics of modern professional settings. Engaging in team-based challenges, students develop crucial skills such as communication, conflict resolution, and joint decision-making, essential for adaptability and efficacy in various occupational realms.
As we navigate the complexities of today's educational ecosystem, a crucial commitment also comes to the forefront: the need to develop resilient, lifelong learners and sharp, critical thinkers. Bloom's Taxonomy, a remarkable framework in educational strategies, offers educators a structured approach to this challenge (Sharunova et al., 2022). It guides learners from basic knowledge absorption to the pinnacle of cognitive abilities: synthesis, evaluation, and creative innovation (Krathwohl, 2002). While the enduring relevance of Bloom's Taxonomy is unquestionable, the rapidly evolving educational landscape and the rising significance of interdisciplinary studies call for more versatile and integrated teaching methodologies. This is where the profound promise of experiential learning comes into play, seamlessly aligning with complex cognitive domains and fostering a learning environment where students and teachers collaboratively explore and link diverse academic fields.
A critical and often overlooked component in the realm of engineering education is the insufficient emphasis on integrating biology into the curriculum. Traditionally, engineering programs have been heavily centered around physics and mathematics, inadvertently marginalizing the profound contributions and insights that can be derived from biological studies. This oversight is significant, as nature, through its intricate evolutionary processes, has engineered highly sophisticated solutions to a wide range of challenges. These natural phenomena and systems exhibit the brilliance of Darwinian natural selection, offering a rich source of inspiration and knowledge that has been honed over millions of years (Whitesides, 2015). The integration of biological principles into engineering education is not just an expansion of academic content, but a paradigm shift toward a more holistic and interdisciplinary approach (Nelson et al., 2017). Such a shift can significantly enrich the educational experience by offering a broader perspective that encompasses both the engineered and the natural world. By drawing lessons from biology, engineering students and professionals can uncover strategies and solutions that are not only innovative but also sustainable, aligning more closely with the principles of ecological harmony and environmental stewardship.
Bioinspired robotics emerges as a shining example in this educational endeavor, bridging traditional engineering principles with the marvels of the natural world. This field encompasses biomechanics, soft robotics, and neuroscience, offering a panoramic view of both engineered and organic systems. Biomechanics explores the natural mechanics underpinning phenomena like bird flight or amphibian locomotion of salamanders, inspiring groundbreaking robotic advancements (Karakasiliotis et al., 2016). Soft robotics, inspired by creatures such as octopuses and starfish, emphasizes the importance of adaptability and compliance in design (El-Atab et al., 2020). Meanwhile, neuroscience investigates neural architecture and animal behavior ranging from simple behavior (Shaikh and Rañó, 2020) up to being able to inform the development of AI systems that emulate complex processes (Bossert and Hagendorff, 2021). In this confluence, students transform from passive observers to active innovators, embodying the core principles of experiential learning. Through bioinspired robotics, they apply PBL methodologies, leveraging their interdisciplinary knowledge to devise solutions that address contemporary challenges (Snell-Rood et al., 2021).
Although educational initiatives in bioinspired robotics have made strides, their focus has often been narrow, primarily targeting K-12 students (Laut et al., 2015). This approach, while commendable, might overlook the significant opportunities bioinspired robotics presents, especially for undergraduate students at a critical stage in their education. These students could greatly benefit from a broader and deeper engagement with the field. Bioinspired robotics, with its inherent potential to bridge various disciplinary gaps and enhance learning experiences, requires a more inclusive and comprehensive approach to unlock its full educational value for these learners.
A detailed examination of bioinspired robotics in education highlights its role in advancing Science, Technology, Engineering and Mathematics (STEM) among K-12 students (Pedersen et al., 2020; Bernstein et al., 2022; Garcia-Langley et al., 2022), acting as a crucial educational tool to acquaint undergraduates with essential concepts and design methodologies (Consi et al., 2019; Garofalo et al., 2020; Kashinath Narvekar et al., 2020), and its effectiveness in promoting gender diversity within engineering (Jackson et al., 2021; Golecki et al., 2022). The positive outcomes, including improved engagement and knowledge gain among participants, call for a more nuanced and effective methodology in deploying bioinspired robotics across educational levels. This refined approach would ensure students across the educational spectrum can explore the interdisciplinary depth of bioinspired robotics, leveraging its full potential to enrich their learning journey.
In this study, we delve into the dynamic educational paradigm, grounded in Bloom's Taxonomy and enhanced by Project-Based Learning (PBL) and experiential learning methodologies. At the heart of our investigation lies the field of bioinspired robotics, which, as illustrated in Figure 1, acts as a pivotal junction for merging disciplinary and interdisciplinary education, technical prowess, and research capabilities, thereby acting as a catalyst for innovative thinking. Building on this foundation, we explore how bioinspired robotics bridges the theoretical principles of constructivism and constructionism with practical, hands-on learning experiences in engineering education. This exploration scrutinizes the ability of interdisciplinary integration to convert abstract educational theories into tangible learning outcomes that profoundly engage students, and investigates the wider implications of fusing biological insights with conventional engineering concepts. We aim to understand the impact of such a multidisciplinary approach on enhancing students' problem-solving skills, teamwork, creativity, adaptability, and innovative capacity, determining how project-based learning can bolster these essential skills to prepare students for the complexities of a rapidly transforming global scenario.
By intertwining these considerations throughout our analysis, we aspire to highlight the collaborative potential of theoretical knowledge and practical application, transcending traditional engineering education. Conducting a bioinspired robotics project in team settings, this paper presents an educational framework that seamlessly integrates core academic principles with the dynamic needs of our global society. The assessment of empirical research is directed toward understanding skill development and the overall learning experience, highlighting the critical importance of adaptability, critical thinking, collaboration, and creative innovation-skills imperative for the contemporary era. Our goal is to demonstrate how a comprehensive approach not only deepens theoretical comprehension but also amplifies practical execution, enabling students to effectively confront the challenges of an increasingly interconnected and constantly evolving world. We invite readers, particularly educators and policymakers, to reflect on the outcomes and evidence-based insights obtained, contributing to the ongoing discussion regarding the significance of interdisciplinary education and experiential learning in equipping students for the challenges of a swiftly changing global society.
2 Materials and methods
In the context of the Final Engineering Project at Tecnológico de Monterrey, which spans a 12-week course, a group of 24 students was introduced to the field of bioinspired robotics through an initial presentation. This presentation highlighted the unique challenges that contemporary robotic systems encounter in various environments, showcasing how this pioneering area skillfully combines nature's time-tested solutions with cutting-edge robotic innovations. Captivated by the intriguing fusion of these two domains, 14 students—13 from mechatronics engineering and one from biomedical engineering-were driven by their burgeoning interest sparked by the presentation to voluntarily come forward. They formed four teams, eager to delve deeper into the possibilities within bioinspired robotics. Before embarking on the journey, students gave their informed consent to participate in the study and for the findings to be published, with the assurance that no private data would be required or disclosed apart from the evidence developed as part of their project work. Moreover, to ensure a focused start, a survey was conducted, gauging students' existing knowledge and their keen interest in bioinspired systems. This survey, comprised of 8 questions and assessed using a 5-point Likert scale ranging from Strongly disagree to Strongly agree, is presented in Table 1. Figure 2 shows the process followed by the students enrolled in this endeavor.
2.1 Stage 1: unraveling the layers of bioinspired robotics
To acquaint the students with the theoretical and technical dimensions of bioinspired robotics, a series of lectures was crafted. These sessions, designed as interactive crash courses, delved into fundamental concepts, providing students with foundational insights to equip them with the knowledge to identify potential areas of focus when addressing engineering challenges. The order and duration of each session were as follows:
• Mobile Robot Locomotion in Complex Terrains (2h): Set against the backdrop of challenging terrains, robots encounter numerous obstacles. This lecture delved into the intricacies of legged locomotion, highlighting the fundamental principles of morphology and biomechanics. It emphasized the importance of compliance and brought these ideas to life with engaging video demonstrations of robots navigating varied terrains.
• Soft Robotics (2h): Venturing into the realm of soft robotics, the session explored a range of artificial muscular structures that echo the adaptability found in nature. From Shape Memory Alloys to Dielectric Elastomer Actuators, this introduction offered students insight into a new generation of robots characterized by flexibility and versatility.
• Neuroscience in Robotics (2h): Bridging the gap between biology and robotics, this presentation unraveled the complexities of neuroscience. It detailed the interplay of neurons, the rhythm of action potentials, and the intrigue of Braitenberg vehicles, along with the pivotal roles of mechanoreceptors, motor neurons, and the synchronization of central pattern generators.
• Digital Signal Processing and Control Systems (4h): Within the digital domain, the lecture guided students through the landscape of digital signal processing and control strategies. This journey revisited time-honored control structures such as the proportional-integral-derivative controller (PID), while introducing the subtleties of digital systems and their implementation in a microcontroller using C++.
2.2 Stage 2: identifying opportunities
Following this intensive phase of knowledge acquisition, attention turned toward hands-on application, with a clear focus on addressing local interests within Mexico. This highlighted a commitment to applying bioinspired robotics in ways that directly benefit the surrounding community and research on endemic species. Leveraging the students' newly acquired insights and ensuring alignment with the course's overarching goals, two distinct project trajectories were chosen:
• Wildlife Observation Robots: Half the teams were entrusted with the task of designing state-of-the-art robots capable of inconspicuously monitoring wildlife. Firstly, they were to obtain invaluable data from the natural habitats, providing deeper insights into the intricate behaviors and interactions of the observed species. Secondly, and of equal importance, was the emphasis on minimizing ecological impact. An added stipulation for this challenge was the selection of a model organism: the chosen species needed to not be only locally found but also face the looming threat of extinction. This inclusion ensured that the technology developed would directly benefit conservation efforts on the ground.
• Search and Rescue Robots: The other half of the participating teams embarked on the design of robots specialized in search and rescue operations, a mission of life-saving significance. These robots, meticulously engineered for resilience and adaptability, are equipped to traverse the most challenging terrains with precision. The primary objective of these robots is to rapidly detect and assist individuals in perilous situations. Two particularly challenging scenarios that Mexico frequently contends with served as the backdrop for this design challenge are navigating the debris aftermath of buildings collapsed by earthquakes, and confronting the unpredictable terrains caused by landslides.
2.3 Stage 3: the quest for knowledge
In their engineering journey, students committed deeply to research, undertaking a structured exploration into academic and industrial knowledge. This process began with a comprehensive understanding of research methodologies, emphasizing that effective research involves both process and content. Students learned to clearly articulate their research problems and intentions in their writings and were introduced to different academic documents like peer-reviewed articles and technical reports, understanding their significance and differences.
Furthermore, they explored the practical implications of their work, delving into tech-based entrepreneurship, technology transfer, and the concept of open innovation, which highlights the importance of collaboration and sharing in the innovation process (Bogers et al., 2018). Their methodology also included dissecting a wealth of patents and scientific literature, including journal articles, and conference papers from databases such as SCOPUS and Web of Science. This literature review provided a solid foundation for their design rationale and highlighted potential innovation areas.
The students also focused on formulating hypotheses and conducting experimental tests using quantitative and qualitative research methods. They honed their ability to communicate findings effectively, crafting compelling narratives around their research, a vital skill for documenting the design process and justifying their choices.
2.4 Stage 4: from conception to blueprint
Drawing from an in-depth grasp of both state-of-the-art and state-of-the-technique and guided by precise specifications, the students initiated their journey of crafting solutions. They set a clear target: achieving a Technology Readiness Level (TRL) of 4. Achieving this milestone, which ensures the technology's components have been tested in laboratory conditions (Mankins, 2009; Olechowski et al., 2015), enables students to assess their design's efficacy and confirm their hypotheses.
The analysis of their proposed system began with a multifaceted approach, incorporating a variety of design methodologies to dissect and understand its nuances. The process is initiated with functional decomposition, allowing the participants to meticulously categorize the primary functions of the system and their sub-functions. This granular approach was instrumental in achieving a deep understanding of the systems embedded within their robots. Concurrently, they employed Quality Function Deployment (QFD), a strategic tool that bridges user and customer expectations with the technical specifications of the design. The integration of QFD was particularly beneficial, as it infused a user-oriented perspective into the design process, ensuring the resulting blueprints were both innovative and closely aligned with user needs. This comprehensive method guaranteed that the solution was not only technically robust but also resonant with the users' anticipated needs.
Subsequently, the focus shifted to the conceptual design phase, where morphological charts played a critical role. These charts are indispensable in exploring various solutions and components that could perform the defined functions (Smith et al., 2006). By comparing and contrasting different options, the students identified the most suitable solutions and design alternatives. This expanded the scope of potential solutions, ensuring that the selected design paths were optimal in terms of functionality and user engagement.
The next stage involved a meticulous breakdown of the project's purpose, objectives, characteristics, and development methods, aligned with a well-planned timeline. The students adopted the SMART framework for setting objectives, which emphasizes Specific, Measurable, Achievable, Relevant, and Time-bound goals (Ogbeiwi, 2017). These SMART objectives encapsulated the project's ambitions, highlighting its unique features. The students established critical milestones, fostering a structured and disciplined approach to project management. Additionally, a SWOT analysis provided insight into potential risks and opportunities, allowing for preemptive strategies and the identification of areas for innovation and improvement.
Finally, the project management process necessitated the creation of both a Gantt chart and a critical path analysis. These tools provided a visual and strategic outline of the steps needed to reach each milestone, assigning appropriate roles for each task, and projecting timelines for completion. This methodical approach was vital in identifying essential resources and developing a comprehensive project timeline. The emphasis on understanding interdependencies was crucial, particularly in a collaborative environment with multiple students, ensuring synchronized progression throughout the project.
2.5 Stage 5: engineering in action
During the phase of transforming their concepts into tangible prototypes, students were dedicated to achieving the standards set by TRL 4. This endeavor necessitated meticulous attention to the integration of both hardware and software elements. Central to this procedure was the utilization of Computer Assisted Design (CAD) for mechanical components, which were subsequently subjected to virtual testing via Computer Assisted Engineering (CAE). Moreover, students also ventured into the design of Printed Circuit Boards (PCBs) that augmented the capabilities of a previously designed and application-tailored board given as a starting point. On the programming front, the adoption of Object-Oriented Programming was pivotal, with an emphasis on achieving modularity, user accessibility, and code maintainability. Upon finalizing the design specifications, students materialized their designs employing advanced fabrication methods, encompassing 3D printing techniques (both Fused deposition modeling and UV-sensitive resin curing), laser cutting, and precision machining. To bolster the robustness and versatility of their innovations, comprehensive documentation was compiled, ensuring both the replicability and scalability of their novel contributions.
To validate their prototypes, students turned to the crucial methodology of “design of experiments.” This approach is instrumental for rigorous testing, accurate result analysis, and precise decision-making, especially when navigating the complex world of engineering developments. It serves as a guidepost, shedding light on uncertainties and providing clarity.
In the initial phase of this approach, the emphasis is on characterizing the process. Here, students discern and pinpoint the essential variables that have a substantial impact on the system's output. This foundational step sets the stage for deeper insights and understanding. Following the characterization, the next phase delves into refining this understanding. It involves a closer examination of the previously identified variables, probing their behavior and interactions to ensure that the design aligns with the desired outcomes.
Throughout this journey, several key considerations remain paramount. Firstly, the very purpose of the experiment must be crystal clear, encompassing both the overarching objective and the detailed expected outcomes. Secondly, the materials and tools for the experiment, ranging from testing instruments to specific devices, need careful selection. Lastly, a robust system for data collection is essential. It's not just about gathering data, but also about where this data will reside and how it will be interpreted to drive informed decisions.
2.6 Stage 6: the culmination
The culmination of the journey was marked by a presentation where students showcased their innovative projects to an expert evaluation panel comprising three specialists. This panel rigorously assessed each project not only for its scientific and technical merits but also for the students' development of soft skills. The evaluation process included a detailed 20-min presentation by the students, followed by a comprehensive 30-min question and answer session, and a thorough review of the accompanying technical documentation. The focus of the evaluation was on practical knowledge application, decision-making skills, and the clarity of explanations.
Following these presentations, a survey was conducted to assess the impact of integrating various knowledge domains into a single cohesive project, particularly in terms of altering the students' perspectives. This survey, consisting of six questions and utilizing the Likert scale for responses, is elaborated in Table 2.
In addition, a comprehensive 20-question test was administered to quantitatively evaluate the students' understanding of design methodologies and fundamental robotics concepts, encompassing mechanical, electronic, programming, and control competencies. The results of this test were compared with those of students involved in non-bioinspired robotics projects, serving as a control group. This comparison was intended to provide a clear measure of the instructional impact of the bioinspired robotics approach on the students' learning outcomes.
Throughout these comprehensive stages, students transitioned from passive learners to active innovators, epitomizing the profound influence of experiential learning and showcasing the limitless horizons within bioinspired robotics.
3 Results
3.1 Initial perceptions on the role of biology in engineering education
The initial survey responses shown in Figure 3 indicated varied perceptions of the role of biology in engineering. A significant 73% of respondents agreed or strongly agreed that biology has a substantial impact on modern engineering solutions (Q1). This sentiment was stronger regarding the competitive edge biology provides in robotics, with 66% agreeing or strongly agreeing (Q2). When it comes to the functional insights from biological organisms for robot design, a majority of 60% agreed or strongly agreed with this statement (Q3).
The analogous nature of challenges in studying biological systems and robotics development was recognized, with 73% concurring (Q4). However, opinions were divided regarding the preference for biological inspiration over traditional engineering methods in robot design, with a combined 53% agreeing or strongly agreeing, and 26% disagreeing or strongly disagreeing (Q5).
The potential for bioinspired robotics to be applied in a wider range of scenarios was acknowledged by 60% of participants (Q6). Furthermore, a compelling 74% believed that bioinspired robotics has more potential for practical real-world applications as opposed to being confined to research (Q7). However, the data revealed a disconnect between this belief and personal experience, as 87% of students indicated they have not been actively involved in bioinspired projects or studies (Q8).
3.2 Project development observations
Upon being presented with their respective challenges, the teams engaged in an intensive ideation phase, where creativity met cutting-edge technology. They dove into brainstorming sessions, applying their fresh insights to the complex task of designing bioinspired robots tailored to their assigned missions-either for the delicate art of wildlife observation or the high stakes of search and rescue operations.
The challenge sparked a surge of innovative concepts amongst the students. For some, this was an initiation into the avant-garde field of soft robotics, drawing inspiration from the pliable forms of nature to conceive robots with the ability to navigate through tight spaces and handle delicate objects without causing harm. A particular fascination was seen in Central Pattern Generators as tools to generate different gaits. Others found themselves intrigued by the concept of whegs, wheel-leg hybrids, that encapsulate the benefits of both wheels and legs for versatile locomotion in uneven terrains, directly reflecting the multifaceted movement strategies found in the animal kingdom. Once defined their approach, they named their robots Axolobot, Turtul, Tuga, and Roller, each reflecting its unique attributes.
The students' study of scientific literature and patent repositories led them to identify knowledge gaps, defining specific problems for their projects and formulating clear, measurable hypotheses. Their exploration into existing materials highlighted the limitations and achievements of current technologies, identifying gaps as opportunities for improvement and refining their designs. This strategic identification not only helped refine their designs but also enhanced their problem-solving skills. Armed with clearer problem definitions, the students formulated hypotheses to guide their design process as shown in Table 3. This empirical approach ensured that their design refinements were data-substantiated.
A notable aspect of the project was the systematic application of various design methodologies and project management tools. These tools were instrumental in refining the students' ideas, assumptions, and proposals through the integration of feedback from potential customers, combined with their own technical expertise and disciplinary knowledge. Furthermore, this process led to an enhanced understanding of not just the terminology but also the practical implications of bioinspired systems. The clear assignment of roles and responsibilities ensured that each student's contributions were in direct alignment with the project's objectives. This cooperative approach was vital in ensuring a smooth and cohesive progression of the project, demonstrating the importance of a well-coordinated team dynamic in achieving consistent progress and success.
The transformation of innovative concepts into functional prototypes at the Technology Readiness Level (TRL) 4 was marked by the student teams' dedication and methodological precision. This section highlights the key results of this transformative phase and presents some of the evidence of the students' work:
• Integration of hardware and software: The integration was marked by seamless interaction between the mechanical and electronic components of the prototypes. CAD and CAE tools were employed extensively as shown in Figure 4, enabling the students to design, iterate, and validate their mechanical components virtually before physical fabrication.
• Fabrication: The use of 3D printing, alongside laser cutting and precision machining, was pivotal in materializing the complex geometries of the prototypes. All teams utilized Fused Deposition Modeling and photopolymer resin curing for fabricating structural components. Furthermore, specifically in Axolobot and Roller (Figure 5), 3D printing technology was also employed in the production of molds to craft soft structures using silicone rubber. The resultant documentation presented a detailed narrative of the fabrication process, offering insights into the students' innovative techniques and setting a replicable and scalable precedent for future work.
• Programming: Embracing Object-Oriented Programming, the students developed modular and maintainable software systems to control each one of the limbs of their robots (Figure 6), as well as to communicate their microcontrollers to a Virtual Instrument implemented in LabVIEW as shown in Figure 7. Moreover, in the case of Turtul, oscillators were implemented using the Van der Pol equation targeted to create CPGs and were initially tested in MATLAB before generating the code for the microcontroller (Figure 8). This modularity ensured that the software could be easily adapted and extended for future enhancements. User accessibility was also a key consideration, indicating that the prototypes were designed with end-user operation in mind.
• Design of experiments: The adoption of the design of experiments methodology allowed for systematic testing and optimization of the prototypes. In the characterization phase, critical variables affecting the system's output were identified, providing a robust foundation for further analysis. An outstanding example was Tuga, where students suggested conducting a virtual experiment to evaluate the vertical displacement of the robot when operating with whegs that exhibited varying phase shifts in their gaits (Figure 9).
• Prototyping success: The culmination of this phase saw each team producing a prototype that met the stringent criteria of TRL 4. These prototypes, such as Tuga presented in Figure 10, demonstrated an advanced stage of development, having been tested in a controlled environment. The prototypes stood as testaments to the students' ability to synthesize complex engineering principles into tangible, innovative solutions. Once with a functional prototype, the students explored how to transition their projects from prototypes to market-ready solutions. They considered commercialization pathways, market needs, feasibility, and intellectual property rights.
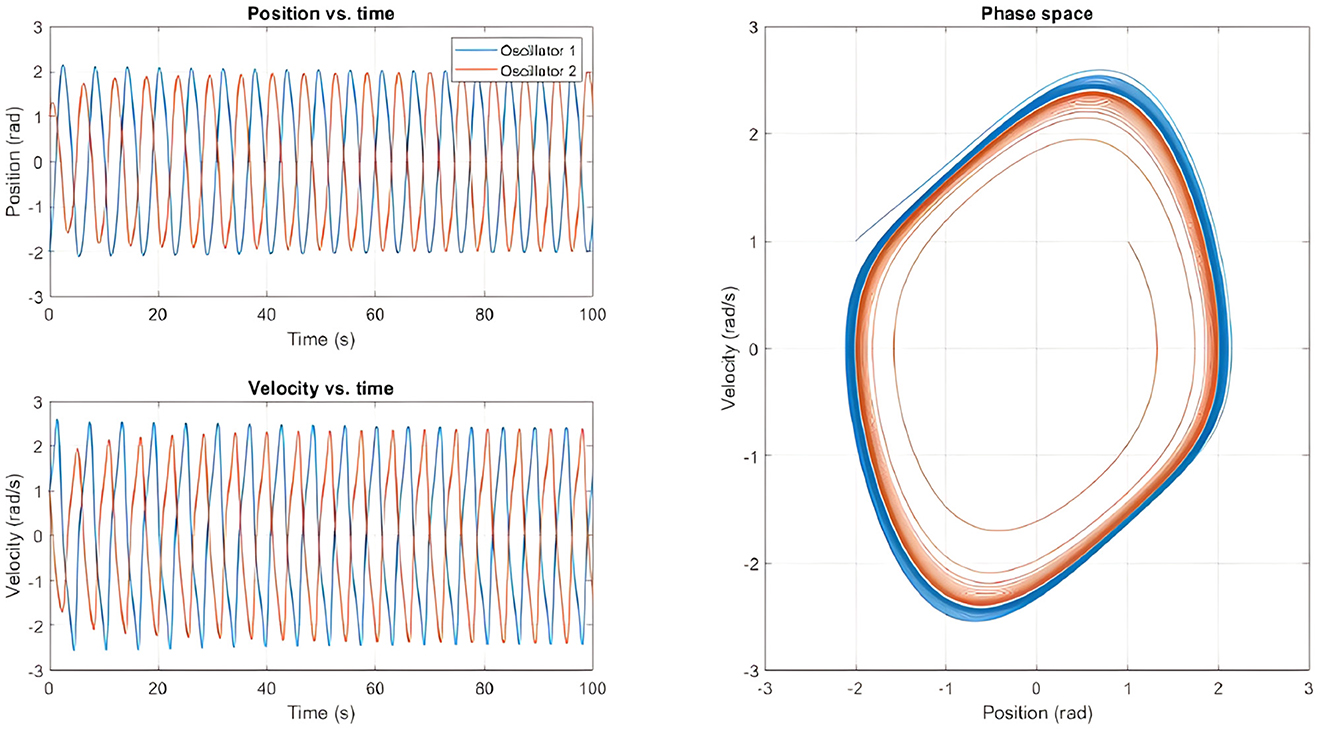
Figure 8. Implementation of Turtul oscillators using Van der Pol equation for central pattern generators (CPGs).
The pivotal presentation drew a myriad of projects, each one manifesting a unique amalgamation of scientific rigor and technical skill.
3.3 Quantitative assessment
The presentation, along with the subsequent 30-min question-and-answer session, garnered commendable feedback from the expert evaluation panel. The panel awarded the four projects an impressive average score of 92%, encompassing both technical proficiency and soft skills. Notably, they emphasized the innovative integration of interdisciplinary elements, the projects' broader impacts, and the students' adeptness in articulating ideas, effectively handling constructive criticism and participating in informed discussions that extended beyond traditional engineering boundaries.
A detailed review of the technical documents further affirmed the panel's initial positive evaluation. The reports exhibited a remarkable level of sophistication in their structure, layout, and the relevance of their content, earning an average score of 91%. The students' dedication to extensive research was especially apparent in their meticulous citations and references.
Finally, the box plot from Figure 11 illustrates the comparative performance outcomes of students on a 20-question test covering fundamental robotics concepts. The students engaged in bioinspired projects achieved an average score of 87%, eclipsing the performance of their peers involved in other forms of robotics projects by a 7% margin. This is not only quantified by the average but is also qualitatively supported by the tight clustering of scores around a higher median, suggesting a strong central tendency toward higher performance levels.
3.4 Final perceptions
The survey results presented in Figure 12 reveal a strong consensus among respondents regarding the influence of bioinspired concepts on future robotics innovations, with 100% agreeing to varying degrees (Q1). This unanimous approval suggests a widespread recognition of the value biology brings to the field of robotics. For the role of engineers with a background in biology driving robotics innovation, 80% agreed or strongly agreed (Q2), indicating a substantial belief in the unique contributions of interdisciplinary knowledge.
The appreciation of biological organisms as inspirations for robotics design is similarly high, with 93% in agreement, highlighting the importance of natural systems as models for aesthetics and functionality (Q3). In confronting challenges where conventional robotics might struggle, 86% agreed or strongly agreed that bioinspired robotics offers viable solutions (Q4), showcasing the adaptability and potential of bioinspired approaches.
The endorsement for bioinspired systems in educational settings is also significant, with 80% recommending such courses to undergraduates (Q5), which points to a belief in the foundational value of bioinspired knowledge for future engineers.Regarding personal engagement with bioinspired robotics through further studies, research, or entrepreneurial ventures, responses were more varied, with 60% considering it, indicating a moderately strong interest in pursuing this innovative field (Q6).
4 Discussion and conclusion
The survey results depicted in Figure 3 offer significant insights into students' initial perceptions of biology's role in engineering. It is clear from the data that there is a strong belief in the relevance of biological principles to engineering innovation, particularly in robotics. This is highlighted by the substantial agreement among respondents, with 73% agreeing or strongly agreeing that biology has a substantial impact on modern engineering solutions (Q1), and 66% recognizing the competitive edge biology provides in robotics (Q2). This recognition might be attributed to the visibility of pioneering companies, such as Boston Dynamics, and breakthroughs in the field that resonate with students' awareness and aspirations.
However, a distinct division exists regarding the practical application of biological inspiration in engineering design, evidenced by the responses to Q5, where 53% agree or strongly agree with the preference for biological inspiration, contrasting with 26% who disagree or strongly disagree. This divide could stem from the complexity and unpredictability of biological systems, which presents a significant challenge to students accustomed to the more predictable and controlled frameworks of traditional engineering. Additionally, there might be a reluctance to deviate from these traditional methods, perceived as more established and reliable. This suggests a need for a more nuanced approach to integrating biological concepts into engineering education.
Despite this, the potential for broader application of bioinspired robotics is recognized by 60% of participants (Q6), and a compelling 74% believe in its relevance for real-world applications beyond academic research (Q7). They indicate a growing acknowledgment of the limitations of conventional robotics and a willingness to explore innovative approaches inspired by the efficiency and adaptability of biological systems. Yet, the contrast between this belief and the minimal engagement in bioinspired projects, with 87% of students indicating no active involvement (Q8), reveals a gap between educational theory and practical experience. This discrepancy underscores the need for educational institutions to offer more practical opportunities in bioinspired projects, emphasizing the importance of bridging theoretical knowledge with hands-on application.
The evolution in participants' views is further clarified in the final survey results shown in Figure 12. Here, a unanimous 100% agreement on the impactful role of biology in future robotics innovations (Q1) signifies a strong consensus on the value of integrating biological principles in engineering education and practice, in contrast to traditional engineering education methods. The survey also indicates that 80% of respondents believe in the unique contributions of interdisciplinary knowledge, suggesting that engineers with a strong foundation in biology are well-positioned to lead innovations in robotics (Q2).
Moreover, the high appreciation for biological organisms as inspirations for robotics design, with 93% agreement (Q3), emphasizes the growing recognition of nature's efficiency, adaptability, and aesthetics. This shift toward valuing biological complexity in design is a notable departure from previous engineering mindsets, potentially paving the way for more innovative and sustainable solutions. Additionally, the belief that bioinspired robotics can offer viable solutions to challenges conventional robotics may not address is strongly supported by 86% of respondents (Q4).
While there is considerable support for integrating bioinspired systems into educational curricula, with 80% recommending such courses (Q5), the actual engagement with bioinspired robotics through further studies, research, or entrepreneurial ventures is more moderate, with 60% considering it (Q6). This moderate level of personal engagement might indicate existing barriers to entry or uncertainties about the field's practicalities, highlighting the importance of addressing these challenges to encourage more active participation in bioinspired robotics. Remarkably, student testimonials shed light on the transformative impact of these activities, with many expressing that their participation unveiled a captivating new domain, igniting a newfound fascination.
The quantitative results presented in Figure 11 demonstrate the effectiveness of bioinspired robotics in enhancing student performance across various learning groups in STEM education. the significant educational advantages of bioinspired robotics projects. With students engaged in these projects scoring an average of 7% higher than their counterparts involved in traditional robotics projects, the benefits extend beyond just an enhanced understanding of robotics concepts. This improvement is not only reflected quantitatively in the average scores but is also qualitatively evident in the tight clustering of scores around a higher median, indicating a robust central tendency toward superior performance. The data suggests that bioinspired robotics projects, through their integration of principles from biological systems, provide a rich, engaging, and effective learning experience.
The consistency in high performance across students involved in bioinspired projects points to a comprehensive enhancement in understanding, driven by the interdisciplinary nature of these projects which blend biology with engineering. Furthermore, the approach of incorporating bioinspired concepts into robotics education ensures not just a boost in acquiring professional skills but also champions inclusivity and accessibility. It cultivates a mindset conducive to creative innovation, offering a pathway to reduce disparities in educational outcomes. This method promotes a more inclusive educational environment, making strides toward equalizing opportunities for all students. By leveraging the inspiration from nature, educators can foster an atmosphere where diverse ideas flourish, thereby contributing significantly to a more equitable educational landscape.
The integration of bioinspired robotics within engineering curricula represents a transformative shift toward embracing constructivist and constructionist learning theories, emphasizing the critical importance of experiential learning in engineering education. This innovative educational model diverges significantly from traditional approaches by facilitating a deep, hands-on engagement with complex, interdisciplinary subjects. It encourages students to venture beyond the academic confines and apply their insights to tackle real-world challenges, fostering a learning environment that cultivates innovation, curiosity, and a proactive problem-solving mindset. Unlike conventional education models that often compartmentalize disciplines, dictate projects, and tailor education predominantly for industrial applications, this approach promotes an active, exploratory learning process that prepares students to navigate and contribute to a rapidly changing technological world.
This method's unique contribution to engineering education lies in its capacity to stimulate curiosity and integrate principles from biology with engineering practices. In sharp contrast to the traditional lecture-based, passive learning models, the curriculum centered around problem-based learning (PBL) and experiential learning fosters an environment where students actively engage in the design and problem-solving process. This educational shift is particularly advantageous in disciplines such as mechanical, mechatronics, robotics, and electronics engineering, where it significantly boosts analytical and creative cognitive skills, positioning these competencies at the pinnacle of Bloom's Taxonomy. For example, by applying biomechanical concepts to vehicle design, mechanical engineering students can explore new efficiencies and innovations. Similarly, mechatronics students can incorporate neurobiological principles into control systems, demonstrating the practical application and interdisciplinary nature of bioinspired robotics. These examples underscore the method's ability to transcend traditional educational boundaries, offering a holistic and integrative learning experience that spans multiple engineering disciplines.
Moreover, the study advocates for a fundamental shift in undergraduate engineering education from the predominant lecture-centric model to one that emphasizes interactive, hands-on learning. By blending theoretical knowledge with practical implementation, the bioinspired robotics curriculum serves as a robust platform for fostering innovative problem-solving skills across various sectors, including healthcare, environmental science, and automation. This approach underscores the crucial role of policy makers in supporting the growth of research and development in these vital areas, advocating for the expansion of experiential learning opportunities across all educational levels, from universities to K-12 schools. Such a comprehensive educational strategy aims to cultivate a more informed, skilled, and versatile future workforce, capable of leading and innovating in an increasingly complex and interconnected global landscape.
The study, though conducted over 12 weeks with a cohort of 14 students, offers a glimpse into the substantial promise of integrating bioinspired robotics into traditional engineering education. Despite its limited scale, the initial results are particularly striking, highlighted by the overwhelmingly positive feedback from participants. Students noted a significant enhancement in their understanding of mechatronics, indicating that this novel educational approach warrants deeper exploration. Nevertheless, the study's scope emphasizes the need for broader research initiatives. Future studies, incorporating more extensive and varied control and experimental groups, are essential to thoroughly evaluate the educational impact of bioinspired robotics. Such comprehensive research would not only validate these preliminary findings but also yield insights to refine and tailor teaching methods more effectively. This endeavor aims to better prepare students for the dynamic requirements of engineering careers. Moreover, the brief duration of the study raises important questions about the long-term retention of knowledge and skills, as well as the extent to which students pursue further research and development in this area. This situation calls for educational leaders to champion and implement innovative teaching strategies, ensuring that the curriculum evolves in tandem with technological progress and equips students to address the engineering challenges of the future with skill and confidence.
Finally, the impressive achievements of specific projects, such as Tuga's first-place victory in a campus-wide engineering contest and Axolobot's recognition at ICMEAE 2023, serve as compelling evidence of the educational model's transformative potential. These achievements validate the approach and demonstrate the potential for leadership and innovation in adopting bioinspired concepts to significantly enhance student engagement and learning outcomes. Moving forward, addressing the study's limitations, and expanding its scope are crucial steps to fully leverage the potential of bioinspired robotics. This comprehensive approach is key not only to preparing future engineers for upcoming technological advancements and challenges, significantly contributing to their fields, but also to positioning educational institutions as leaders in engineering education.
Data availability statement
The original contributions presented in the study are included in the article/supplementary material, further inquiries can be directed to the corresponding author.
Ethics statement
Ethical approval was not required for the studies involving humans because they are not required by the local legislation and institutional requirements. The study, centered on an educational intervention at Tecnológico de Monterrey, involved standard educational practices such as workshops and robot design within the regular academic curriculum, thereby not necessitating additional ethical oversight. The project's focus on the impact of integrating biology with engineering in an educational setting did not engage in sensitive personal data collection or pose any foreseeable risk or harm to the participants. The studies were conducted in accordance with the local legislation and institutional requirements. The participants provided their written informed consent to participate in this study.
Author contributions
IC-J: Writing—original draft, Writing—review & editing, Methodology, Supervision, Validation, Conceptualization, Data curation, Resources, Project administration, Formal analysis, Visualization. RB-B: Writing—review & editing. MR-C: Writing— review & editing.
Funding
The author(s) declare that financial support was received for the research, authorship, and/or publication of this article. The authors acknowledge the financial support of CONACyT grant CVU 682831.
Acknowledgments
We extend our deepest gratitude to the students who participated in this study, for their invaluable contributions, dedication, and enthusiasm throughout the project. Their engagement and innovative thinking were instrumental in bringing this research to fruition. Their willingness to embrace new concepts and interdisciplinary approaches provided vital insights and significantly enriched the study. Their hard work, creativity, and commitment to pushing the boundaries of their knowledge in the fields of engineering and biology have been truly inspiring.
Conflict of interest
The authors declare that the research was conducted in the absence of any commercial or financial relationships that could be construed as a potential conflict of interest.
The reviewer RT declared a shared affiliation with the authors to the handling editor at the time of review.
Publisher's note
All claims expressed in this article are solely those of the authors and do not necessarily represent those of their affiliated organizations, or those of the publisher, the editors and the reviewers. Any product that may be evaluated in this article, or claim that may be made by its manufacturer, is not guaranteed or endorsed by the publisher.
References
Ackermann, E. (2001). Piaget's constructivism, papert's constructionism: what's the difference. Fut. Learn. Group Public. 5:438.
Alanazi, A. (2019). A critical review of constructivist theory and the emergence of constructionism. Am. Res. J. Human. Soc. Sci. 2, 1–8. doi: 10.21694/2378-7031.16018
Alimisis, D., and Kynigos, C. (2009). “Constructionism and robotics in education,” in Teacher Education on Robotic-Enhanced Constructivist Pedagogical Methods, 11–26.
Ben-Ari, M. (1998). Constructivism in computer science education. ACM SIGCSE Bull. 30, 257–261. doi: 10.1145/274790.274308
Bernstein, D., Puttick, G., Wendell, K., Shaw, F., Danahy, E., and Cassidy, M. (2022). Designing biomimetic robots: iterative development of an integrated technology design curriculum. Educ. Technol. Res. Dev. 70, 119–147. doi: 10.1007/s11423-021-10061-0
Bogers, M., Chesbrough, H., and Moedas, C. (2018). Open innovation: research, practices, and policies. Calif. Manag. Rev. 60, 5–16. doi: 10.1177/0008125617745086
Bossert, L., and Hagendorff, T. (2021). Animals and AI. The role of animals in AI research and application – an overview and ethical evaluation. Technol. Soc. 67:101678. doi: 10.1016/j.techsoc.2021.101678
Consi, T. R., Fan, D., and Jodin, G. (2019). “Fishbots: Bio-inspired marine robots give students a hands-on introduction to fluid mechanics,” in OCEANS 2019 MTS/IEEE SEATTLE (IEEE), 1–8. doi: 10.23919/OCEANS40490.2019.8962580
El-Atab, N., Mishra, R. B., Al-Modaf, F., Joharji, L., Alsharif, A. A., Alamoudi, H., et al. (2020). Soft actuators for soft robotic applications: a review. Adv. Intell. Syst. 2:2000128. doi: 10.1002/aisy.202070102
Garcia-Langley, A., Alvarez, I., Chen, A., Li, A., Wang, H., Brancazio, D., et al. (2022). “Development of educational marine soft robotics STEM platform as new iteration of SeaPerch K-12 national outreach program,” in OCEANS 2022 (Hampton Roads, VA, USA: IEEE), 1–8. doi: 10.1109/OCEANS47191.2022.9977015
Garofalo, L., Sandler, S., and Seth, D. (2020). “Evaluation of a snake jaw robot to teach integrated biology, mathematics, and engineering,” in 2020 IEEE Integrated STEM Education Conference (ISEC) (IEEE). doi: 10.1109/ISEC49744.2020.9280695
Golecki, H., Lamer, S., McNeela, E., Tran, T., and Adnan, A. (2022). “Understanding impacts of soft robotics project on female students' perceptions of engineering,” in 2022 ASEE Annual Conference &Exposition (Minneapolis, MN).
Jackson, A., Mentzer, N., and Kramer-Bottiglio, R. (2021). Increasing gender diversity in engineering using soft robotics. J. Eng. Educ. 110, 143–160. doi: 10.1002/jee.20378
Karakasiliotis, K., Thandiackal, R., Melo, K., Horvat, T., Mahabadi, N. K., Tsitkov, S., et al. (2016). From cineradiography to biorobots: an approach for designing robots to emulate and study animal locomotion. J. R. Soc. Interf. 13:20151089. doi: 10.1098/rsif.2015.1089
Kashinath Narvekar, S., Bessariya, R., Sadananda, A., and Arya, K. (2020). “Learn, build and compete: an aquatic robot-fish challenge,” in 2020 3rd International Conference on Education Technology Management, ICETM 2020 (ACM). doi: 10.1145/3446590.3446600
Krathwohl, D. R. (2002). A revision of bloom's taxonomy: an overview. Theory Into Pract. 41, 212–218. doi: 10.1207/s15430421tip4104_2
Laut, J., Bartolini, T., and Porfiri, M. (2015). Bioinspiring an interest in STEM. IEEE Trans. Educ. 58, 48–55. doi: 10.1109/TE.2014.2324533
Mankins, J. C. (2009). Technology readiness assessments: a retrospective. Acta Astron. 65, 1216–1223. doi: 10.1016/j.actaastro.2009.03.058
McGrath, D. (2004). Strengthening collaborative work: go beyond the obvious with tools for technology-enhanced collaboration. Project-based learning. Learn. Lead. Technol. 31, 30–33.
Nelson, K., Husman, J., Cheng, K., and Harackiewicz, J. (2017). “I want to be an engineer, why should i study biology?” Using future time perspective to understand students' beliefs about foundational courses,” in 2017 ASEE Annual Conference & Exposition.
Ogbeiwi, O. (2017). Why written objectives need to be really SMART. Br. J. Healthc. Manage. 23, 324–336. doi: 10.12968/bjhc.2017.23.7.324
Olechowski, A., Eppinger, S. D., and Joglekar, N. (2015). “Technology readiness levels at 40: a study of state-of-the-art use, challenges, and opportunities,” in 2015 Portland International Conference on Management of Engineering and Technology (PICMET), 2084–2094. doi: 10.1109/PICMET.2015.7273196
Pedersen, B. K. M. K., Marchetti, E., Valente, A., and Nielsen, J. (2020). “Fabric robotics-lessons learned introducing soft robotics in a computational thinking course for children,” in International Conference on Human-Computer Interaction (Cham: Springer International Publishing), 499–519. doi: 10.1007/978-3-030-50506-6_34
Shaikh, D., and Rañó, I. (2020). Braitenberg vehicles as computational tools for research in neuroscience. Front. Bioeng. Biotechnol. 8:565963. doi: 10.3389/fbioe.2020.565963
Sharunova, A., Wang, Y., Kowalski, M., and Qureshi, A. J. (2022). Applying Bloom's taxonomy in transdisciplinary engineering design education. Int. J. Technol. Des. Educ. 32, 987–999. doi: 10.1007/s10798-020-09621-x
Smith, G., Troy, T. J., and Summers, J. D. (2006). “Concept exploration through morphological charts: an experimental study,” in Volume 4a: 18th International Conference on Design Theory and Methodology (Philadelphia, Pennsylvania, USA: ASME), 495–504. doi: 10.1115/DETC2006-99659
Snell-Rood, E. C., Smirnoff, D., Cantrell, H., Chapman, K., Kirscht, E., and Stretch, E. (2021). Bioinspiration as a method of problem-based STEM education: a case study with a class structured around the COVID-19 crisis. Ecol. Evolut. 11, 16374–16386. doi: 10.1002/ece3.8044
Whitesides, G. M. (2015). Bioinspiration: something for everyone. Interf. Focus 5:20150031. doi: 10.1098/rsfs.2015.0031
Keywords: bioinspired robotics, education innovation, experiential learning, higher education, interdisciplinary learning
Citation: Cayetano-Jiménez IU, Bustamante-Bello R and Ramírez-Cadena M (2024) Bridging the gap: bioinspired robotics as catalyst for interdisciplinary education. Front. Educ. 9:1375487. doi: 10.3389/feduc.2024.1375487
Received: 23 January 2024; Accepted: 25 April 2024;
Published: 14 May 2024.
Edited by:
Diana Hernández Montoya, Universidad Estatal a Distancia, Costa RicaReviewed by:
Rasikh Tariq, Institute for the Future of Education, Monterrey Institute of Technology and Higher Education (ITESM), MexicoSheila L. Macrine, University of Massachusetts Dartmouth, United States
Copyright © 2024 Cayetano-Jiménez, Bustamante-Bello and Ramírez-Cadena. This is an open-access article distributed under the terms of the Creative Commons Attribution License (CC BY). The use, distribution or reproduction in other forums is permitted, provided the original author(s) and the copyright owner(s) are credited and that the original publication in this journal is cited, in accordance with accepted academic practice. No use, distribution or reproduction is permitted which does not comply with these terms.
*Correspondence: Rogelio Bustamante-Bello, rbustama@tec.mx