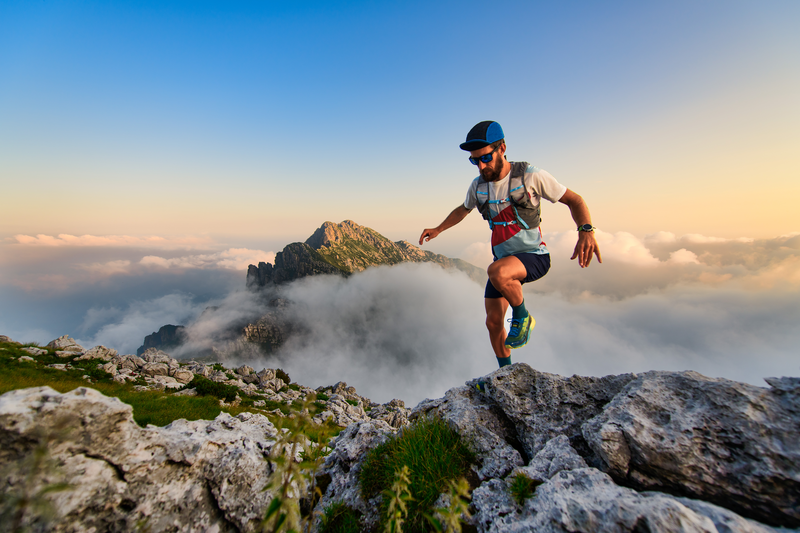
95% of researchers rate our articles as excellent or good
Learn more about the work of our research integrity team to safeguard the quality of each article we publish.
Find out more
ORIGINAL RESEARCH article
Front. Educ. , 09 April 2024
Sec. STEM Education
Volume 9 - 2024 | https://doi.org/10.3389/feduc.2024.1343492
This article is part of the Research Topic Global Lesson Study Policy, Practice, and Research for Advancing Teacher and Student Learning in STEM View all 9 articles
Maker Education (ME) focuses on fostering students’ Maker Mindsets (MM). However, prevailing ME practices often lack a structured approach, that impedes the effective development of students’ MM. To address this challenge, a Design Thinking-making pedagogy (DTMP) is used to design ME lessons through a three-month Lesson Study (LS) cycle. Employing a qualitative multiple case study methodology, we studied the development and transformation of MM in three elementary school students. Diverse data were collected, including student artifacts, video recordings of teacher discussions, and audio recordings of the interviews. The findings revealed common cognitive, self-efficacy, and collaboration improvements among the three students. Unique changes in individual cognitive skills, motivational patterns, and obstacles to collaboration were also observed. Furthermore, key factors influencing MM development were identified across individual, social, and pedagogical dimensions. Individual factors included prior knowledge, maker skills, and motivation, whereas social factors included teachers’ attitudes, recognition, feedback, and peer collaboration. Pedagogical practices, such as project difficulty, structure, and authenticity, play a crucial role in MM development. This study not only contributes valuable insights into MM development, but also underscores the pivotal role of tailored, personal real-life experiences in ME pedagogy. Furthermore, our study suggests that teachers can enhance ME pedagogy by intentionally designing it to facilitate MM in students, using the LS approach.
Numerous studies in Maker Education (ME) underscore the significance of fostering Maker Mindsets (MM) for students’ cognitive, affective, and behavioral development (Iversen et al., 2015; Smith et al., 2015; Regalla, 2016; Flores, 2018). However, concerns have been raised by researchers, such as Blikstein and Worsley (2016), and Kim and Zimmerman (2017) indicated that current ME practices often lack structure and standardization, impeding the internalization of students’ MM.
In response to these concerns and aligning with other researchers (Christensen et al., 2016; Gözen, 2016; Jeng et al., 2020), we propose the integration of Design Thinking (DT) into ME, creating what we term DT-making pedagogy (DTMP). DT provides a structured project management process that emphasizes student ideas from project inception to completion (Veldhuis et al., 2021), potentially facilitating the transformation of student MM.
Despite the growing body of research on MM’s learning activities and outcomes (Chu et al., 2015; Soltis and Wells, 2019; Jeng et al., 2020), understanding of how students’ MM develops and the factors influencing this transformation remains limited. Addressing the call for more research in this area by Kim and Zimmerman (2017), our study aimed to build upon our previous work (Li et al., 2023) by investigating students’ MM formation and transformation under the implementation of DTMP. To achieve this, we conducted a comprehensive within- and cross-case analysis, tracking three case students’ MM changes over a three-month Lesson Study (LS) cycle.
Maker education (ME) is an innovative pedagogical approach rooted in constructivism and inspired by Papert’s constructionism theory (Papert and Harel, 1993). It is characterized by interactive student-centered learning that integrates digital and hands-on craftsmanship. This approach emphasizes the development of knowledge, skills, and attitudes, empowering students to confidently address real-world challenges with an open mindset (Blikstein and Worsley, 2016).
Within ME, Maker mindsets (MM) have become central components of educational research (e.g., Martin, 2015; Bevan, 2017; Chu et al., 2017). Although a unified definition of MM is lacking in literature, research has evolved from theory to practice. Dougherty (2013) introduced the term “Maker Mindsets” as a “can-do” attitude, including perseverance, curiosity, risk-taking, and embracing failure—all crucial for effective learning through making. Building on this foundation, Martin (2015) extended the MM construct to include playful, growth-oriented, failure-embracing, and collaborative values, which are particularly relevant in STEM (Science Technology Engineering Mathematics) education. Kuznetsov and Paulos (2010) identified open sharing, learning, and creativity as central values among Makers. Subsequently, empirical research predominantly explored MM within motivational domains, investigating elements like self-efficacy, curiosity, situated interest, self-concept, and agency (e.g., Regalla, 2016; Kim and Zimmerman, 2017; Jeng et al., 2020; Martínez Moreno et al., 2021). A smaller subset examined MM in cognitive and behavioral domains, exploring dimensions such as creativity, problem-solving, STEM knowledge acquisition, and self-regulation and collaboration (e.g., Iversen et al., 2015; Smith et al., 2015; Flores, 2018).
Our study adopts a holistic definition of MM, encompassing cognitive, affective, and behavioral domains. These domains include:
• The cognitive domain, which encompasses STEM knowledge and relevant thinking skills and dispositions (e.g., Kuznetsov and Paulos, 2010; Peppler and Hall, 2016; Davis et al., 2017; Jeng et al., 2020).
• The affective domain, which relates to students’ self-efficacy, self-identity, self-concepts, and intrinsic motivation (e.g., Chu et al., 2015, 2017; Vongkulluksn et al., 2018; Fadhli Kamaruzaman et al., 2020).
• The behavioral domain, which involves self-regulated learning and collaborative knowledge sharing (Iversen et al., 2015; Flores, 2018; Kim et al., 2022). This framework guides our study in understanding how students’ MM evolve during the making process.
Design thinking (DT) is a creative process that encompasses methods and mindsets aimed at fostering innovation, creativity, and development of new artifacts. It encourages practices such as experimentation, prototyping, gathering feedback, and redesign (Dorst, 2011; Noweski et al., 2012; Razzouk and Shute, 2012). The Hasso Plattner Institute of Design at Stanford, known as the d.school, has introduced a well-established DT framework consisting of five essential phases: Empathize involves observing user behaviors, engaging with them, and immersing oneself in their environments to build empathy; Define is the phase in which users identify a meaningful challenge after empathizing; Ideate focuses on organized brainstorming to generate solutions for addressing the identified challenge; Prototype phase entails creating physical models to substantiate solutions for testing and visualization; Test is the step where prototypes are placed in the user’s real-life environment to gather feedback (d.school, 2010).
The structured DT process model is widely acknowledged as an effective remedy to address the limitations associated with conventional ME pedagogical methods, researchers including Iversen et al. (2015), Blikstein and Worsley (2016), and Kim et al. (2020) have recognized its potential in this regard. Furthermore, scholars, such as Bethke Wendell and Rogers (2013), Alden and Tramonti (2020), Boakes (2020), and Martínez Moreno et al. (2021) have adeptly integrated DT into ME learning activities, yielding notable improvements in various domains.
These integrations not only augment students’ cognitive, motivational, and behavioral engagement, as substantiated in Veldhuis et al.’s comprehensive review (Veldhuis et al., 2021), but also foster the development of indispensable skills. These skills encompass creativity, problem-solving abilities, collaborative competence, and heightened self-efficacy. Notably, this transformation transcends the mere acquisition of specific STEM knowledge and skills (Bethke Wendell and Rogers, 2013; Alden and Tramonti, 2020; Martínez Moreno et al., 2021). This harmonizes with the components we pre-defined for MM, creating a synergistic and comprehensive learning environment.
While MM undergoes ongoing theoretical and empirical exploration, there has been a limited discourse on the factors influencing its development. Nevertheless, a consistent trend emerges in current practices, where efforts are made to leverage the self-determination theory (Ryan and Deci, 2000) to elucidate the factors driving changes in MM. This theory underscores the significance of autonomy, competence, and relatedness in fostering intrinsic motivation.
These influential factors can be systematically categorized from the perspectives of pedagogy, teacher, and students. In terms of pedagogy, considerations involve the difficulty and variety of learning tasks within a project (Kim et al., 2020). Students’ perceptions of their own abilities and prior knowledge constitute a crucial dimension from the student perspective (Vongkulluksn et al., 2018). Meanwhile, from the teacher’s standpoint, it encompasses the strategies employed in providing scaffoldings to assist students in overcoming challenges (Iversen et al., 2015).
Lesson Study (LS) is a structured and effective professional development model renowned for its capacity to enhance teaching practices, facilitate teacher development, and endorse student-centered pedagogy (Huang and Bao, 2006; Dudley, 2013; Ni Shuilleabhain and Seery, 2018; Schipper et al., 2018). At its foundation, LS is an inquiry cycle led by a team of teachers that revolves around a “research lesson.” This research lesson is an actual classroom session that has been intentionally designed to investigate and improve the teaching of a certain topic (Lewis et al., 2009). The LS team explore the complexities of selected students, known as “case pupils” (Dudley, 2013), to examine how their understanding, reaction, and learning experience changes and discover components of the course design that either enhance or hinder learning (Dudley, 2013). These case students, chosen specifically for their unique abilities, skills, and learning requirements, play a critical role in increasing instructors’ professional development and overall classroom learning (Goei et al., 2021). They represent the educational variability seen by teachers as a microcosm of the larger student population (Dudley, 2013).
According to Dudley’s LS model (Dudley, 2013), teachers systematically observe and collected data on case students’ engagement, response, and interactions during the research lesson. The observations include both verbal and non-verbal cues, providing a holistic view of the students’ learning experience.
Subsequently, post-lesson discussions revolve around the progress and challenges faced by case students, using the observed data to inform these conversations. The insights garnered from these discussions lead to adjustments in teaching practices, fostering continuous improvement through iterative cycles of re-teaching, re-observing, and re-analysis.
This steadfast attention to case pupils ensures that teaching practices and lessons developed within the LS framework are finely tailored to meet diverse learning needs in the classroom. It was evident that these targeted approaches yield significant growth among case pupils, influencing not only their academic progress but also bolstering their confidence, engagement, and problem-solving abilities (Norwich et al., 2014; Dudley et al., 2019).
In this case, LS model stands out is suitable to development DTMP targeted at MM because of its emphasis on student-centered pedagogy and its particular attention to the learning experiences of case students. In other words, using the LS model, teachers gain a more nuanced knowledge of students’ behaviors, emotions, and cognitive processes while they engage in hands-on making activities. This knowledge is crucial for developing DTMP that effectively contribute to the larger goal of cultivating students’ MM.
LS is recognized as a practice-based methodology that enhances students’ learning by fostering the professional development of teachers, including aspects such as knowledge, beliefs, and curriculum design. Guided by the theoretical model illustrating the impact pathways of LS (refer to Figure 1; Lewis et al., 2006), our study is designed to investigate the transformation of students’ MM throughout the LS process.
Figure 1. The theoretical model of lesson study cycle and pathways of impact adopted from Lewis’ study (Lewis et al., 2006).
In alignment with the studies of scholars like Christensen et al. (2016), Gözen (2016), and Jeng et al. (2020), who integrated the DT process model into ME, and responding to the call by Kim and Zimmerman (2017), this study builds upon our earlier investigation (see Li et al., 2023) that primarily focused on the professional development of teachers within the framework of LS.
Thus, the aim of this study was to gain a deeper understanding of the specific mechanisms and factors influencing changes in students’ MM during the implementation of the DT-making pedagogy (DTMP). To guide our investigation, we formulated two research questions (RQs):
RQ1: How do case students' MM evolve during the implementation of the DTMP?
RQ2: What are the major factors that influence changes in students’ MM?
To explore the transformation and evolution of students’ MM comprehensively, we implemented a two-phase multiple-case study research design, as advocated by Stake (2013). In this design, we delved into the data concerning three specific case students who were intentionally selected by the teachers for observation during the LS process.
The first phase entailed analysis of the data for each of the three case students, treating each as an individual case study (Phase 1). Subsequently, in the second phase, we conducted a cross-case comparison to scrutinize the commonalities and distinctions that emerged across all three students. This allowed us to identify shared patterns of MM changes that extended beyond the individual participants (Phase 2).
As Stake (2013) noted, qualitative case study research is ideally suited for investigating real-life experiences in authentic settings. The case study research design offers valuable insights into each student’s learning process within a genuine educational context. Moreover, the multiple case study research design (Stake, 2013) facilitated the construction of contextualized experiences and systematic analysis procedures, contributing to a comprehensive and holistic understanding of the trajectory of MM transformation.
The study was conducted at a Chinese elementary school from April to July 2022. The participants consisted of four STEM teachers, 20 grade-4 students, and the primary author of this study. All participants engaged in a structured process involving four cycles of LS following the framework defined by Dudley (2013). In these LS cycles, the teachers collectively planned, taught, observed, analyzed, and refined lessons around three selected case students. These case students were chosen with deliberate intent, following teacher interviews, and an assessment of their academic performance. The aim was to ensure a diverse representation of students with varying skill levels, including low, middle, and high ability students. Table 1 presents an overview of these three students.
Additionally, the primary author of this study assumed a dual role, acting as a participant researcher who actively recorded and participated in all LS-related activities, encompassing planning, research lessons, and reflection meetings (Bogdan and Biklen, 1997). Moreover, the primary author also took on the role of the “knowledgeable other” (Corcoran et al., 2011), providing guidance and training to the participating teachers while overseeing the entire LS process.
After an initial training phase, teachers engaged in four cycles of LS, as shown in Figure 2. These cycles involved several key stages:
• Pre-meeting: Teachers grouped 20 students into five teams, selected three case students, and defined the central problem for the Maker project while aligning it with the curriculum standards.
• Planning meeting: Teachers collaboratively crafted lesson plans, detailing learning tasks, Maker toolkits, instructional scaffolds (including prompts, handouts, and hints), and designed observation sheets.
• Research lesson: Teachers executed the developed lesson plans, closely observing the case students during these lessons.
• Interviewing case students: Teachers assessed content knowledge by surveying all 20 students and conducting interviews with the case students.
• Post-lesson discussion: After the research lessons, the teachers collectively analyzed and refined them based on observations of the case students and insights from the interviews. This process was concluded with discussion meetings aimed at enhancing the quality of teaching and learning.
The LS cycles led to the creation of four research lessons (RLs), teachers utilized the DTMP to guide students in completing four different Maker projects, each spanning approximately 4 h. Throughout each project, teachers provided scaffolding to support students in both individual and collaborative tasks. Table 2 provides a brief introduction to these projects to help readers better understand them. Students worked on these four projects following the DT process, which involved understanding users during the Empathize stage, identifying root causes in the Define stage, brainstorming solutions in the Ideate stage, and then progressing to tasks such as connecting, programming, testing electronic components, and designing the appearance in the Prototype stage. Finally, they shared, upgraded, and reflected on their products in the Share and Reflect stages.
This multiple case study research design incorporated a range of rich cases to provide diverse data sources (Stake, 2013). Each case student in this study was constructed using three data sources: student portfolios, teachers’ observations, and interviews.
The student portfolios included background information, academic grades, and RL materials such as worksheets and reflection logs. Teachers’ observations were derived from video recordings of four post-discussion meetings related to RL activities. It’s important to note that these discussions exclusively involved teachers, and students did not actively participate. During these meetings, the teachers shared their observations regarding the case students’ behaviors, with a specific focus on elements related to MM in the cognitive, affective, and behavioral domains. Sample questions posed during these observations included queries about the development of students’ thinking skills, changes in their confidence and self-efficacy, and the progression of their teamwork and communication skills within the context of MM.
In terms of the interviews, we collected audio recordings of post-lesson interviews conducted by teachers with the case students after each RL, with each interview lasting approximately 5 min. These interviews aimed to gain insight into students’ learning experiences, any misconceptions they may have had, and suggestions for improving future classes (Dudley et al., 2019). Sample questions during these interviews encompassed the process undertaken for projects, challenges faced and overcome, lessons learned, areas for improvement in subsequent projects, and the students’ willingness to tackle more challenging projects.
Furthermore, individual teachers participated in semi-structured interviews, each lasting approximately 30 min. These interviews explored teachers’ perspectives on the cognitive, affective, and behavioral development of the case students. Some sample questions from these teacher interviews encompassed topics such as the observations of changes or developments in students’ attitudes and behaviors related to making and creating, the challenges and obstacles students face in cultivating and sustaining a growth-oriented MM, unexpected or surprising instances of students’ MM changes, and the strategies or practices employed to foster a positive MM within the classroom.
This multi-faceted approach allowed for a comprehensive examination of students’ MM and the factors contributing to their development. It encompassed a variety of data sources, including portfolios, observations, and interviews, to offer a robust foundation for understanding the cases.
The data analysis for this study was conducted in two phases to provide a comprehensive understanding of students’ MM formation and development.
In Phase 1, we focused on constructing individual case studies using a within-case analysis approach. This method allowed us to gain an in-depth understanding of how individual students’ MM evolved. To guide our analysis, we employed a predefined MM definition incorporating specific features and behaviors (see Supplementary Appendix).
Our analysis of Phase 1 involved an examination of the records of the three case students. We concentrated on the knowledge, behaviors, and attitudes that reflected their MM as well as the factors influencing their MM changes. Consistent with Stake (2013) guidelines, we conducted multiple reviews for each case record during a single session. Subsequently, we coded the data for each student within each RL, using our established MM definition.
To facilitate our analysis, we utilized a coding matrix adapted from Terzis et al. (2022). This matrix was visually depicted in Figure 3, where the MM attributes of each case student intersect with six columns, corresponding to distinct stages of DT experienced in the RLs. The analysis of change patterns involved the use of specific symbols: “+,” denoting an increase; “−” representing a decrease; “=,” indicating no change; and “+/−” indicating fluctuations. These symbols effectively convey the variations observed in each DT cycle. Leveraging this matrix, we conducted a comprehensive comparative analysis. This allowed us to discern shared characteristics and individual variations in MM attributes across the three case students was instrumental in visually representing MM’s changes in a single student and across students throughout the RLs.
Figure 3. Coding matrix representing the change patterns of three case students’ MM across four RLs.
In Phase 2, we adopted an inductive thematic analysis approach following the framework proposed by Braun and Clarke (2006). This method facilitated the exploration and identification of recurring themes and patterns within our qualitative data. Through inductive thematic analysis, we systematically categorized and comprehend the factors that influence students’ MM changes. This method served as a robust foundation for uncovering nuanced insights and connections embedded in the dataset.
It is essential to emphasize that Phases 1 and 2 are interlinked in our analysis approach. Phase 1 serves as the foundation, offering key insights that guided our thematic analysis in Phase 2. This two-phase approach ensured a comprehensive and holistic understanding of students’ MM changes, thereby contributing to the rigor and validity of our research findings.
To enhance the validity of our qualitative study, we employed data triangulation, utilizing multiple data sources. Moreover, member checks were adopted in this qualitative research, where the teachers were invited to review and validate the interpretation of the results.
Ethical approval was granted for the study, participation was voluntary, and the participants were free to withdraw from the study without prejudice. Participants’ identities were assigned pseudonyms to protect their privacy.
This section presents the evolution of each of the three case students’ MM and the major factors influencing these changes through a comparison of the three cases.
This research question focuses on understanding the transformations in students’ MM across four RLs, where DTMP was employed by teachers to guide students in creating Maker projects. To address this question, we adopted a comprehensive approach that combined teacher observations and student interviews. The following sections present the findings for each case student’s MM development.
Emma was initially characterized as a low-ability, unmotivated female student with limited self-efficacy. However, significant positive changes were observed for across various aspects over the four lessons. Supporting data are presented in Table 3.
Emma’s progression was evident as she evolved from an initial hesitancy in responding to teachers’ questions and frequently skipping classes to progressively engage in cognitive thinking processes during the second and third lessons. During these sessions, she began to answer questions with the guidance from the teachers. By the final lesson, her thinking skills had further developed. She was willing to participate in discussions and adapted her thinking based on teacher feedback, demonstrating a newfound willingness to tackle complex questions by actively participating in and adjusting her thought processes.
Initially, Emma exhibited low self-efficacy, passively following the teacher’s instructions, and readily giving up when confronted with challenges. During the first two lessons, she relied heavily on external guidance and sought recognition. Her frustration emerged when her peers disregarded ideas during the collaborative activities. Nevertheless, as the course progressed to the third and fourth lessons, a notable transformation occurred. Emma began to demonstrate heightened resilience and persistence in the face of programming failures, and she increasingly felt a sense of accomplishment after answering the teacher’s questions correctly.
In the first lesson, Emma displayed passive engagement without enthusiasm, and her motivation further decreased further in the second lesson because her contributions were overlooked. However, a positive shift in her motivation emerged from the third lesson onwards. She showcased determination and resilience in the face of challenges and displayed a newfound willingness to learn from failures.
Emma initially hesitated to participate in group activities and had minimal interaction with her peers in the first lesson. Frustration emerged in the second lesson when her ideas were dismissed by group members. However, a positive shift was observed in her collaborative learning abilities from the third lesson onwards. She took a more active role within group activities and displayed a growing openness to seeking input and actively contributing to the group.
In summary, Emma’s transformation from low ability, motivation, and self-efficacy to increased engagement, resilience, and collaborative involvement highlights the transformative potential of the learning experience.
John was initially identified as a high-ability male student with robust STEM knowledge and motivation but exhibited lower self-efficacy. Supporting data are available in Table 4.
An analysis of John’s development revealed an evolution in the variety and depth of his thinking skills across the four lessons. He initially displayed creativity and idea generation skills during brainstorming in the first lesson. In the second lesson, despite not recalling the sensor’s name, he provided a reasonable solution using a sensor, showcasing his problem-solving abilities and adaptability. By the third and fourth lessons, John consistently contributed unique and innovative suggestions, reflecting deeper thinking and the ability to generate novel solutions.
Changes in John’s self-efficacy were evident, particularly in terms of increased confidence, resilience, and his ability to influence others. In the initial two lessons, he faced frustration and low confidence when his ideas were rejected by group members. However, in subsequent lessons, John grew his self-efficacy by offering feedback, pointing out others’ disadvantages, and persuading others to accept his ideas. He further demonstrated increased self-efficacy by justifying his ideas and challenging them with examples.
His self-efficacy continued to strengthen, as he provided examples when his ideas faced doubt, reflecting increased resilience and confidence in persuading others based on his belief in his ideas.
John’s motivation experienced a transformation over the four lessons, with increased determination, resilience, and enthusiasm for learning and problem-solving. His motivation was initially at its lowest in the first lesson, where he was ready to give up upon encountering programming failures. However, from the second lesson onwards, John displayed progressive determination and resilience when facing setbacks. He persisted in debugging and testing, not giving up until seeking help. In the final lesson, he acknowledged the importance of learning from failure with the expression, “failure is the mother of success.”
John’s collaborative learning ability improved in terms of communication and teamwork. He initially struggled to express his ideas clearly during group work, which negatively impacted collaboration. However, in the second lesson, he began encouraging other group members, particularly when they faced multiple failures, highlighting improved teamwork and motivation. After the second lesson, he further developed his communication skills by providing suggestions and persuading others to adopt his ideas, although challenges in clearly explaining his ideas remained.
In summary, John’s journey demonstrates a transformation from initial challenges in self-efficacy and motivation to increased confidence, resilience, and the ability to influence and persuade others. His thinking skills expanded significantly, and his collaboration abilities improved, highlighting the dynamic nature of the educational experiences.
Initially identified as a medium-ability female student with basic STEM knowledge, high self-efficacy, and limited collaborative ability, Mary’s MM underwent notable changes. Supporting data are available in Table 5.
Mary exhibited a variety of thinking skills, including problem-solving, creativity, and innovation. In the first lesson, she displayed logical and analytical thinking, focusing on calculating dimensions and shapes during sketching. The second and third lessons revealed her flexibility in problem-solving, suggesting alternative solutions when her group faced disagreements and setbacks. In the last lesson, her creativity and innovation became evident, as she incorporated her favorite colors and figures into the appearance design, demonstrating her ability to consider alternative solutions.
Fluctuations were observed in Mary’s belief in her own abilities and her willingness to accept and act upon others’ feedback and suggestions. Initially, she was reluctant to embrace others’ ideas and feedback, express uncertainty about their validity, and indicate a lack of self-efficacy in evaluating and incorporating external inputs. In the second lesson, her self-efficacy seemed to decrease as she grew tired and delegated a task to others. However, she displayed a desire to explore new ideas, hinting at a subsequent boost in her self-efficacy and willingness to experiment. In the fourth lesson, she exhibited a willingness to incorporate others’ suggestions while adding her personal touch, indicating a more balanced approach.
Mary’s motivation underwent fluctuations. In the first two lessons, she remained highly motivated, actively engaging in the design process, offering suggestions during brainstorming, and taking the initiative to test prototypes. However, passive behaviors emerged in the third lesson, where she repeatedly assumed the role of designer in group work. Her motivation resurged in the fourth lesson, as she made changes and iterations during the design of a physical prototype. Overall, her motivation showed fluctuations, transitioning from proactive to passive engagement and then soaring again.
Mary improved her collaborative ability over time, particularly in terms of active listening, appreciating others’ opinions, and engaging in supportive and constructive participation in group activities. In the first two lessons, she exhibited assertiveness and consistently disregarded others’ opinions, presenting a challenge in collaboration. In the third lesson, despite delegating a task to others and remaining disengaged, there was reduced involvement in collaboration. However, in the fourth lesson, Mary not only became willing to listen to others’ opinions during group collaboration but also actively sought feedback, reflecting a more collaborative and open approach.
In summary, Mary’s journey showcases her transition from initial hesitance to accepting others’ input to moments of increased self-efficacy, as she actively participates, experiments with new ideas, and integrates suggestions from peers. Her thinking skills and motivation also evolved significantly, with collaborative abilities showing positive changes over time. This highlights the dynamic nature of educational experiences and the potential for growth in various aspects of learning and development.
This research question delves into the core factors driving changes in students’ MM throughout the implementation of the DTMP by analyzing students’ reflection logs, post-lesson discussion meetings, and the post-interviews with teachers and students. The findings and supporting evidence are presented below.
The analysis results revealed that three personal-related factors significantly impacted students’ MM transformation. These factors include students’ prior knowledge base, Maker skills, and motivation engaged in Maker projects.
It pertains to students’ mastery of STEM subjects before engaging in Maker projects. In the case of Emma, it was evident that compared to other students, she refrained from answering teachers’ questions and passively participated in group discussions during the first two lessons. Her reluctance stemmed from feelings of inadequacy and anxiety related to her limited knowledge of programming and electronic components. As she candidly expressed to the teacher after the first lesson:
“I have very limited knowledge of programming and electronic components, [so] I’m afraid of answering the teacher’s questions incorrectly… I get nervous in class, [because] my knowledge is quite limited, and during group discussions, I also can’t understand, so I don’t know how to participate.”
Emma’s explanation illustrates how the depth of subject knowledge can significantly impact students’ cognitive, motivational, and behavioral engagement in project making, ultimately influencing MM transformation.
Maker skills encompass practical abilities, such as coding, 3D printing, and electronic connections, that are essential for hands-on making. Insufficient proficiency in Maker skills can impede students from successfully completing projects, making it challenging for them to bring their ideas to fruition. Consequently, these difficulties can lead to feelings of demotivation. Mary’s experience with the glue gun highlights this issue:
“When our group tried to use a glue gun to attach the control board to the backpack, it just wouldn’t stick. As a result, our group could not complete the task as much as the other groups.’ This made me feel very frustrated, and I think we should improve our crafting skills.”
Additionally, when students lack essential making skills, they may become overly reliant on others to complete their tasks, which may hinder their independence and problem-solving abilities. John’s reflection in the first lesson exemplifies this: “I cannot connect electronic components to the appropriate pins on the Arduino Nano using jumper wires; I have to rely on other students’ help.” These stories illustrate that the lack of making skills negatively impacts students’ hands-on making experiences, ultimately hindering their MM transformation.
We discovered that students’ motivation played a pivotal role in shaping students’ classroom performance and their transformation of MM. We found that intrinsic and extrinsic motivation exerted different influences on student performance.
Intrinsic motivation encompasses the students’ active engagement in an activity driven by their inherent enjoyment, personal interests, and aspirations for self-improvement (Ryan and Deci, 2000). On the other hand, extrinsic motivation is rooted in external factors, such as rewards or external pressures, that lead students to participate (Ryan and Deci, 2000).
From Table 1, it can be observed that Emma’s motivation primarily stems from extrinsic sources, potentially due to the pressure of parental expectations, which consequently results in a relatively passive classroom performance. In contrast, John and Mary are predominantly fueled by intrinsic motivation, ignited by their profound interest and positive past experiences in Maker projects.
This divergence in motivational sources illuminates the reason for Emma’s comparatively lower participation in the initial two lessons, whereas John and Mary exhibited heightened motivation and enthusiasm for the projects. It becomes evident that motivation not only serves as a critical determinant of students’ involvement in Maker projects but also plays an indispensable role in their MM transformation.
The results revealed that three social-related factors significantly impacted students’ MM transformation. These factors include teacher attitude, recognition and feedback, and peer collaboration.
Teachers’ attitudes encompass the beliefs, behaviors, and approaches demonstrated by teachers during the facilitation of Maker projects and classroom activities. These attitudes can be broadly classified as either supportive or unsupportive, and their impact on students’ engagement and creative thinking in Maker projects is quite significant.
This impact of teacher attitudes becomes evident when we examine Mary’s response to the question, “Why aren’t you participating in the project-making as enthusiastically as you did in the past two projects?” Her elaboration sheds light on this matter:
“During the first two projects, Mr. Liu and Mr. Yin gave us ample time to work on our projects, allowing for continuous experimentation. Today, Mr. Han primarily focused on explaining the program and didn’t allocate enough time for hands-on work, leaving me somewhat bored.”
Mary’s additional insights underscore the contrasting approaches of Mr. Liu and Mr. Yin, who encouraged students to experiment and learn from their mistakes, thus cultivating a supportive environment that fostered creativity. In contrast, Mr. Han’s emphasis on precision and the limited opportunities for hands-on activities adversely affected the students’ enthusiasm and creative thinking. This underscores the pivotal role that teacher attitudes play, either in nurturing a positive transformation or hindering the development of the MM.
Recognition and feedback encompass the acknowledgment and input provided in response to a student’s work, efforts, or achievements. It was observed that teachers’ recognition and feedback played distinct roles in the MM transformation of the three students.
In Emma’s case, a student with low self-efficacy, teacher recognition had an impact by instilling a belief in her abilities. She began to perceive that she had “possessed the capability to succeed in tasks,” thus boosting her confidence.
For John, he viewed teachers’ feedback as “a valuable source for improvement.” This feedback served as a guide in his project-making process, enabling him to make necessary adjustments and refinements to his work. The students’ artifacts serve as evidence of their improvement following teachers’ feedback and recognition. As illustrated in Figure 4, the changes in students’ Sketch worksheets during the LS cycle demonstrate their responsiveness to feedback. This indicates that students incorporate more constraints into their designs based on teachers’ feedback.
In Mary’s experience, regular recognition and feedback contributed to “a deep sense of achievement.” As she completed various aspects of her projects, this sense of accomplishment reinforced her commitment to the project and served as a motivating factor, encouraging her to strive for further success.
These findings highlight the importance of recognition and feedback in shaping students’ MM and their commitment to project-based learning.
Peer collaboration is a fundamental aspect of students working together to complete projects, enabling the exchange of ideas, skills, tools, and learning resources. This collaborative approach significantly influenced the development of the MM among these three students, resulting in both negative and positive effects.
Initially, the drawbacks of peer collaboration became evident during the first two projects. First, John experienced frustration and reduced engagement as his ideas were often disregarded or overlooked by his peers. This led to a decline in his enthusiasm for subsequent project participation, a point emphasized by Mr. Liu, who remarked, “A notable example is John, who eagerly sought to share his ideas but often found them rejected, leaving him frustrated and significantly diminishing his involvement in subsequent classes.”
Second, peer collaboration resulted in resistance to feedback and suggestions from peers, which in turn gave rise to conflicts and challenges in reaching a group consensus. As Mr. Li highlighted, “Mary’s inclination to adhere to her own opinions and dismiss suggestions from others led to significant disagreements and conflicts within the group.”
Third, peer collaboration led Emma to assume a passive role in group discussions, causing her to miss opportunities for active participation and skill development. Mr. Han observed, “Emma appeared disengaged during group discussions, which represented a missed opportunity for her growth.” These observations shared by participant teachers suggest that peer collaboration may negatively impact students’ cognitive, emotional, and behavioral engagement, potentially hindering their MM development.
However, in the subsequent lessons, peer collaboration had a positive impact on the three students’ MM development. This was evident in their reflections on what they learned the most from these four projects. They acknowledged that when they faced challenges or lacked certain skills, their team members stepped in to assist and provide guidance. This collaborative approach enabled them to overcome obstacles and make progress in their projects. For example, Emma mentioned, “When I did not know how to program electronic components, other team members helped me.” Mary further shared, “When I drew sketches, other team members provided feedback from different angles,” and “when we built prototypes, all team members contributed, such as gluing the cardboard and cutting papers.” The students’ words reflect the importance of collaborative learning and peer support in their MM development.
The findings highlight how teachers’ pedagogical practices shaped their students’ MM transformation. The subsequent sections address factors such as project difficulty, structure, and authenticity.
This pertains to the level of knowledge, skills, and competencies required for students to successfully complete a project. The analysis revealed that when the students took on challenging projects in the first lesson, they encountered frustration and a sense of being overwhelmed by to the initial difficulty of these projects. Mary expressed this frustration, stating, “I got frustrated when I tried many times, but still failed… and finally, I gave up… because the project was too difficult to complete.”
Both John and Mary shared similar feelings, which discouraged them from opting for more complex projects in the following lessons. John articulated:
“I know that I have some strengths, but I also have areas where I still need to improve. Choosing a project that aligns with my current knowledge and skills doesn't mean I'm not creative or ambitious. It just means that I want to build on what I already know and feel more confident in my abilities.”
John’s statement emphasizes the importance of aligning project choices with their current knowledge and skills. This illustrates that the students place value on achieving a balance between challenge and competence. They prioritize projects that align with their existing abilities, with the goal of building confidence through successful experiences.
Project structure encompasses the structured process that leads students from the initiation of a project to its successful completion, facilitating the transfer of knowledge (Scarbrough et al., 2004). According to the findings of the analysis, the students felt that the systematic approach provided by the DTMP was helpful in effectively navigating the entire project. This pedagogical practice also increased students’ knowledge and grasp of the project’s procedures, as well as the importance of each phase in the process. This was evident in the three students’ reflection journals, where they provided detailed descriptions of how they finished their tasks. Emma, for example, describes their project completion method as follows, noting:
“Our group completed the project by following the procedure: interviewing others to collect information, analyzing obtained information, brainstorming possible ideas, programming and designing, and sharing products with others.”
Furthermore, the teachers’ practice of summarizing each procedure was identified as a critical feature in enabling the students to understand the goal and significance of each stage in the method. John stated: “Teachers outlining each procedure made me grasp why I needed to engage in this procedure.”
These findings imply that the organized methods provided by the DTMP increased students’ understanding of the project’s organization and the reason for their activities.
In this context, authenticity refers to the relevance of projects to students’ daily lives. An examination of the students’ project preferences revealed that their assessment of project authenticity and its connection to their daily experiences significantly influenced their choices.
For example, Emma preferred a project addressing parent–child communication conflicts (as referred in RL4). She found it meaningful, believing that it could improve her daily life by facilitating information exchange and reducing conflicts. As she expressed, “Creating a parent-and-child communicator is especially meaningful to me, as it can enhance information sharing and reduce conflicts.”
On the other hand, John was drawn to a novel and challenging project aimed at improving canteen staff efficiency using electronic components. He was intrigued by the untapped potential of electronic components in food delivery from the very first lesson. As he remarked, “I never imagined that electronic components could enhance the efficiency of food delivery for canteen staff.”
Mary was interested in a smart backpack solution, as it could address several issues in her daily life, including missing books and homework, improving her posture, and resolving zipper problems (as referenced in RL2). She explained, “I particularly like the backpack project because it is highly practical. I can use electronics to solve a long-standing issue - always forgetting to zip up my backpack, poor posture and leaving homework at home.”
These preferences underscore the unique perspectives of the students and the significance of project authenticity and relevance to their daily lives.
In summary, the findings revealed the interplay between personal, social, and pedagogical factors in shaping students’ MM transformation. A combination of a solid foundation of prior knowledge, maker skills, intrinsic motivation, supportive teacher attitudes, recognition, feedback, and effective peer collaboration contributes to a positive MM transformation. These insights offer valuable guidance for educators seeking to nurture MM in students.
It is known that the utilization of the LS approach can have a considerable influence on the professional advancement of teachers, resulting in the improvement of instructional techniques and the academic progress of pupils (Lewis et al., 2006). Under this theoretical framework, we extended our prior work (Li et al., 2023) to focus on the transformation of students’ MM. Utilizing the Dutch-LS model (Goei et al., 2021), we selected case students of varying abilities to investigate MM formation and development within ME context. Our findings, detailed below, shed light on the dynamic MM changes in cognitive, affective, and behavioral domains of three case students engaged in an LS cycle.
To explore the intricacies of MM development in the three case students, we conducted an in-depth analysis, combining teacher observations and student interviews over four lessons. Our examination revealed three key aspects that either align with or differ from existing studies in the domains of cognition, affect, and behavior.
Our study found a significant increase in STEM knowledge and thinking skills across all students, regardless of their varying baseline abilities. These thinking skills encompass creativity, problem-solving, and adaptability. This result, consistent with many ME studies, affirms that hands-on project-based learning enhances cognitive development. Notably, our study identified nuanced changes in cognitive development patterns. Specifically, Emma excelled in content knowledge but showed slower improvement in thinking skills. John demonstrated discernible improvement in thinking depth and diversity. Mary exhibited early flexibility and versatility in her STEM approach due to her unique combination of thinking abilities. These nuanced changes can be attributed to two characteristics of the LS approach. LS centers around specific learning objectives, enabling teachers to observe individual responses to different strategies. Moreover, LS involves multiple observations over an extended period, facilitating a comprehensive understanding of students’ growth.
Our study result shows that, despite all three students transforming their self-efficacy beliefs, their motivation and self-efficacy fluctuated throughout these four RLs. Specifically, Emma and John’s motivation levels fluctuated, with bursts of enthusiasm interspersed by decrease. Mary, on the other hand, displayed an initial rush of enthusiasm followed by periods of inactivity. These variations underscore the intricate nature of MM transformation, aligning with similar findings in Vongkulluksn et al.’s longitudinal studies (Vongkulluksn et al., 2018) and Kim and Zimmerman’s case studies (Kim and Zimmerman, 2017).
Our results demonstrate that all three students improved their collaboration abilities, but during the process of shaping these abilities, each student faced different collaboration challenges. Emma was first hesitant to fully participate, John battled with effectively conveying his thoughts, and Mary struggled to notice and assimilate the perspectives of others. These challenges were also discussed by Moriwaki et al. (2012) and Buchholz et al. (2014). This highlights the need to consider the context-specific aspects of collaboration within the MM trajectory.
In summary, our analysis provides a comprehensive understanding of MM development among the three case students. It highlights the shared growth in cognitive abilities, self-efficacy, and collaborative skills, while acknowledging the individualized nature of motivation and the context-specific challenges associated with collaboration. In this context, we propose that personalized educational support is a viable approach to nurture students’ MM. This approach recognizes the uniqueness of each student, empowering them to assume ownership of their learning journey and fostering the development of MM.
Our analysis of students’ reflections, teacher and student interviews, and post-lesson discussions revealed several factors that influenced the formation of students’ MM, categorized into individual, social, and pedagogical perspectives.
Individual factors that affect the development of MM include prior knowledge, maker skills, and motivation. Students’ depth of prior knowledge in STEM subjects, exemplified by Emma’s limited programming skills, can lead to feelings of inadequacy and hinder engagement in Maker projects. Proficiency in practical maker skills, like coding and 3D printing, is essential for project success, however a lack of these skills, as seen in Mary’s experience with a glue gun, can result in frustration and demotivation. Students’ motivation varies, with Emma being externally driven and others internally motivated. These differences significantly impacted classroom engagement and MM development. Studies by Flores (2018) and Hansen et al. (2019) also highlight the importance of prior knowledge and maker abilities in enabling engagement, building confidence, and improving problem-solving skills.
From a social perspective, teachers’ attitudes, recognition, feedback, and peer collaboration are significant for MM development. Supportive teachers foster a positive environment that encourages creative thinking and engagement through experimentation. Conversely, teachers who overly focus on correctness may hinder their creativity. Teacher recognition and feedback play an instrumental role in MM transformation, instilling belief in students’ abilities, guiding improvement, and contributing to a sense of achievement, influenced by individual self-efficacy and mindset. Peer collaboration can enhance engagement, skill development, and collaboration, but it can also lead to frustration and conflicts when not effectively managed. Situated in existing studies, Hughes et al. (2019), Hansen et al. (2019), and Weng et al. (2023) underscore the significance of instructor and peer support in creating a supportive ecosystem for project completion, learning, and personal growth.
In pedagogical practices, our research highlights the pivotal role of project design, which encompasses project difficulty, structure, and authenticity in influencing students’ MM development. Students value a balance between challenge and competence, prioritizing projects aligned with their abilities to build confidence through successful experiences. This approach resonates with concepts like optimal challenge and self-determination in learning (Ryan and Deci, 2000). A systematic project structure, guided by DTMP pedagogy, enhances students’ understanding of the project’s organization and the significance of each phase. Teachers’ summarizing each procedure is vital for students to comprehend the purpose of each stage within the project. The authenticity of the project context significantly influenced students’ project preferences and engagement, emphasizing the importance of connecting classroom projects with real-life scenarios for a more engaging learning experience. Gözen (2016), McCurdy et al. (2020), and Zhang et al. (2020), who researched using DT to reframe ME, their study results also acknowledged that DT gave students a sense of direction during project making, leading to developing various thinking skills such as creativity, problem framing, problem-solving.
Our review identified two underexplored areas in the existing literature: students’ initial motivation before engaging in maker projects and their familiarity with real-life situations. These gaps in research can be attributed to our adoption of the LS approach, which offers a context-specific, in-depth exploration of the educational process, uncovering insights unattainable through other methods.
Through LS, we collaborated with four teachers to closely examine the students’ initial motivation and track their evolution throughout the project. This longitudinal approach provided detailed insights which are often missing in cross-sectional studies. This allowed us to illuminate the factors shaping students’ participation and performance, a previously overlooked aspect.
Furthermore, our focus on individual, real-life situations within the LS approach enabled the integration of authentic, real-world problems into the learning process. Our observations on how students’ familiarity with these situations affected their engagement, learning, and problem-solving abilities provided valuable insights. Specifically, we noticed that when confronted with an unfamiliar problem setting, both high-ability and average-ability students face difficulties in properly defining the problems to be addressed in the projects, which has an impact on their enthusiasm to participate in subsequent projects. When we emphasize students’ personal lives in following projects, we notice a significant transformation - all three children adeptly outline challenges and actively participate in project design process. This emphasis on students’ real-life familiarity addresses a notable gap in previous research, which frequently centered on abstract social and global issues.
In conclusion, our study adds to the understanding of MM development in elementary students. This was achieved by employing within-case and cross-case analyses of three students with different abilities across four RLs within the LS context. The findings revealed shared commonalities in cognitive development, self-efficacy shifts, and collaboration enhancement among these three students. Additionally, unique transformations in individual cognitive skills, motivational patterns, and collaboration hurdles were identified. The analysis underscores the importance of personalized education support for students with different abilities. Further, we identified potential factors that may influence MM development across individual, social, and pedagogical dimensions. Individual factors encompass prior knowledge, maker skills, and motivation, while social factors include teacher attitudes, recognition, feedback, and peer collaboration. Pedagogical practices, such as project difficulty, structure, and authenticity, play a crucial role in MM development. Therefore, this study enhances the knowledge of MM development and formation. As a result, this work advances our understanding of the creation and development of MM. Additionally, we propose that LS may be a useful strategy for establishing tailored ME education that meets the needs of different students.
The limitations of this study can be outlined in three main aspects. Firstly, even though we deliberately selected three students with diverse abilities to monitor their MM transformation across four RLs, the small sample size may restrict the extent to which our findings can be generalized to a broader student population. Secondly, the study is firmly situated within a specific educational context, which could limit its relevance and applicability to other educational settings. Thirdly, our data collection heavily relied on subjective qualitative methods, including teacher observations and student interviews, introducing the potential for bias in the interpretation of the data.
To enhance the robustness of future research in this area, it is recommended to consider diversifying the sample size, incorporating more objective quantitative measures, and addressing potential researcher bias. Additionally, expanding the study’s timeframe and adopting a longitudinal research approach could offer valuable insights into the persistence and evolution of MM over an extended period. These considerations aim to strengthen the generalizability, reliability, and validity of the study’s findings and contribute to a more comprehensive understanding of MM transformation.
The raw data supporting the conclusions of this article will be made available by the authors, without undue reservation.
Written informed consent was obtained from the individual(s) for the publication of any potentially identifiable images or data included in this article.
JL: Conceptualization, Data curation, Formal analysis, Writing – original draft. SG: Funding acquisition, Supervision, Writing – review & editing. RH: Supervision, Writing – review & editing.
The author(s) declare that financial support was received for the research, authorship, and/or publication of this article. This study was funded by the Chinese Government Scholarship (No. 202008300032).
We express our gratitude to Li Zhuang, Yin Xiaohui, Liu Ziang, and Han Chengdong, the dedicated teachers from Livon Elementary School in Changchun, for their valuable participation in this research.
The authors declare that the research was conducted in the absence of any commercial or financial relationships that could be construed as a potential conflict of interest.
All claims expressed in this article are solely those of the authors and do not necessarily represent those of their affiliated organizations, or those of the publisher, the editors and the reviewers. Any product that may be evaluated in this article, or claim that may be made by its manufacturer, is not guaranteed or endorsed by the publisher.
The Supplementary material for this article can be found online at: https://www.frontiersin.org/articles/10.3389/feduc.2024.1343492/full#supplementary-material
Alden, D., and Tramonti, M. (2020). Computational design thinking and physical computing: preliminary observations of a pilot study. Robotics 9:71. doi: 10.3390/robotics9030071
Bethke Wendell, K., and Rogers, C. (2013). Engineering design-based science, science content performance, and science attitudes in elementary school. J. Eng. Educ. 102, 513–540. doi: 10.1002/jee.20026
Bevan, B. (2017). The promise and the promises of making in science education. Stud. Sci. Educ. 53, 75–103. doi: 10.1080/03057267.2016.1275380
Blikstein, P., and Worsley, M. (2016). “Children are not hackers” in Makeology. eds. K. Peppler, E. R. Halverson, and Y. B. Kafai. 1st ed (New York: Routledge), 64–79.
Boakes, N. J. (2020). Cultivating design thinking of middle school girls through an origami STEAM project. J. STEM Educ. Res. 3, 259–278. doi: 10.1007/s41979-019-00025-8
Bogdan, R., and Biklen, S. K. (1997). Qualitative research for education. Boston, MA: Allyn & Bacon.
Braun, V., and Clarke, V. (2006). Using thematic analysis in psychology. Qual. Res. Psychol. 3, 77–101. doi: 10.1191/1478088706qp063oa
Buchholz, B., Shively, K., Peppler, K., and Wohlwend, K. (2014). Hands on, hands off: Gendered access in crafting and electronics practices. Mind Cult. Act. 21, 278–297. doi: 10.1080/10749039.2014.939762
Christensen, K. S., Hjorth, M., Iversen, O. S., and Blikstein, P. (2016). Towards a formal assessment of design literacy: analyzing K-12 students’ stance towards inquiry. Des. Stud. 46, 125–151. doi: 10.1016/j.destud.2016.05.002
Chu, S. L., Angello, G., Saenz, M., and Quek, F. (2017). Fun in making: understanding the experience of fun and learning through curriculum-based making in the elementary school classroom. Entertain. Comput. 18, 31–40. doi: 10.1016/j.entcom.2016.08.007
Chu, S. L., Quek, F., Bhangaonkar, S., Ging, A. B., and Sridharamurthy, K. (2015). Making the maker: a means-to-an-ends approach to nurturing the maker mindset in elementary-aged children. Int. J. Child Comput. Interact. 5, 11–19. doi: 10.1016/j.ijcci.2015.08.002
Corcoran, D., O’Reilly, M., Breen, S., Dooley, T., and Ryan, M. (2011). The application of lesson study across mathematics and mathematics education departments in an Irish third-level institution.
d.school. (2010). An Introduction to Design Thinking. Process Guide. https://web.stanford.edu/~mshanks/MichaelShanks/files/509554.pdf
Davis, R. L., Schneider, B., and Blikstein, P. (2017). Making the invisible visible: a new method for capturing student development in makerspaces.
Dorst, K. (2011). The core of ‘design thinking’ and its application. Des. Stud. 32, 521–532. doi: 10.1016/j.destud.2011.07.006
Dougherty, D. (2013). “The maker mindset” in Design, make, play: growing the next generation of STEM innovators. eds. M. Honey and D. E. Kanter (New York: Routledge), 7–11.
Dudley, P. (2013). Teacher learning in lesson study: what interaction-level discourse analysis revealed about how teachers utilised imagination, tacit knowledge of teaching and fresh evidence of pupils learning, to develop practice knowledge and so enhance their pupils’ learning. Teach. Teach. Educ. 34, 107–121. doi: 10.1016/j.tate.2013.04.006
Dudley, P., Xu, H., Vermunt, J. D., and Lang, J. (2019). Empirical evidence of the impact of lesson study on students’ achievement, teachers’ professional learning and on institutional and system evolution. Eur. J. Educ. 54, 202–217. doi: 10.1111/ejed.12337
Fadhli Kamaruzaman, A., Rasdan Ismail, A., Azhar Mat Daud, K., and Jusoh, N. (2020). Makerspace as an environment to cultivate the maker mindset among industrial design student. IOP Conf. Ser. Earth Environ. Sci. 616:012031. doi: 10.1088/1755-1315/616/1/012031
Flores, C. (2018). Problem-based science, a constructionist approach to science literacy in middle school. Int. J. Child Comp. Interact. 16, 25–30. doi: 10.1016/j.ijcci.2017.11.001
Goei, S. L., Norwich, B., and Dudley, P. (Eds.). (2021). Lesson study in inclusive educational settings. Routledge: Singapore.
Gözen, G. (2016). Influence of design thinking performance on children’s creative problem-solving skills: An estimation through regression analysis. Br. J. Educ. Soc. Behav. Sci. 12, 1–13. doi: 10.9734/bjesbs/2016/22153
Hansen, A. K., McBeath, J. K., and Harlow, D. B. (2019). No bones about it: how digital fabrication changes student perceptions of their role in the classroom. J. Pre-Coll. Eng. Educ. Res. 9:6. doi: 10.7771/2157-9288.1155
Huang, R., and Bao, J. (2006). Towards a model for teacher professional development in China: Introducing Keli. J. Math. Teach. Educ. 9, 279–298.
Hughes, J., Morrison, L., Kajamaa, A., and Kumpulainen, K. (2019). “Makerspaces promoting students’ design thinking and collective knowledge creation: examples from Canada and Finland” in Interactivity, game creation, design, learning, and innovation. eds. A. L. Brooks, E. Brooks, and C. Sylla, vol. 265 (Springer International Publishing), 343–352.
Iversen, O. S., Smith, R. C., Blikstein, P., Katterfeldt, E. S., and Read, J. C. (2015). Digital fabrication in education: expanding the research towards design and reflective practices. Int. J. Child Comput. Interact. 5, 1–2. doi: 10.1016/j.ijcci.2016.01.001
Jeng, Y. L., Lai, C. F., Huang, S. B., Chiu, P. S., and Zhong, H. X. (2020). To cultivate creativity and a maker mindset through an internet-of-things programming course. Front. psychol. 11:1572.
Kim, J. Y., Chung, H., Jung, E. Y., Kim, J. O., and Lee, T. W. (2020). Development and application of a novel engineering-based maker education course for pre-service teachers. Educ. Sci. 10:126.
Kim, J. Y., Seo, J. S., and Kim, K. (2022). Development of novel-engineering-based maker education instructional model. Educ. Inf. Technol. 27, 7327–7371. doi: 10.1007/s10639-021-10841-4
Kim, S. H., and Zimmerman, H. T. (2017). Towards a stronger conceptualization of the maker mindset: a case study of an after-school program with squishy circuits. Proceedings of the 7th Annual Conference on Creativity and Fabrication in Education, 1–4.
Kuznetsov, S., and Paulos, E. (2010). Rise of the expert amateur: DIY projects, communities, and cultures. Proceedings of the 6th Nordic conference on human-computer interaction: extending boundaries (pp. 295–304). ACM.
Lewis, C. C., Perry, R. R., and Hurd, J. (2009). Improving mathematics instruction through lesson study: A theoretical model and North American case. J. Math. Teach. Educ. 12, 285–304
Lewis, C., Perry, R., and Murata, A. (2006). How should research contribute to instructional improvement? The case of lesson study. Educ. Res. 35, 3–14. doi: 10.3102/0013189X035003003
Li, J., Goei, S. L., and Van Joolingen, W. R. (2023). A case study of teacher learning in enacting maker pedagogy through lesson study. Int. J. Less. Learn. Stud. 12, 240–256. doi: 10.1108/IJLLS-04-2023-0042
Martin, L. (2015). The promise of the maker movement for education. J. Pre-Coll. Eng. Educ. Res. 5, 30–39. doi: 10.7771/2157-9288.1099
Martínez Moreno, J., Santos, P., and Hernandez-Leo, D. (2021). Maker education in primary education: changes in students’ maker-mindset and gender differences. Ninth International Conference on Technological Ecosystems for Enhancing Multiculturality (TEEM’21), 120–125.
McCurdy, R. P., Nickels, M. L., and Bush, S. B. (2020). Problem-based design thinking tasks: engaging student empathy in STEM. Electr. J. Res. Sci. Math. Educ. 24, 22–55.
Moriwaki, K., Brucker-Cohen, J., Campbell, L., Saavedra, J., Stark, L., and Taylor, L. (2012). Scrapyard Challenge Jr., Adapting an art and design workshop to support STEM to STEAM learning experiences. Paper presented at the IEEE 2nd Integrated STEM Education Conference.
Ni Shuilleabhain, A., and Seery, A. (2018). Enacting curriculum reform through lesson study: a case study of mathematics teacher learning. Prof. Dev. Educ. 44, 222–236. doi: 10.1080/19415257.2017.1280521
Norwich, B., Dudley, P., and Ylonen, A. (2014). Using lesson study to assess pupils’ learning difficulties. International journal for Lesson and Learning studies, 3, 192–207.
Noweski, C., Scheer, A., Büttner, N., von Thienen, J., Erdmann, J., and Meinel, C. (2012). “Towards a paradigm shift in education practice: developing twenty-first century skills with design thinking” in Design thinking research. eds. H. Plattner, C. Meinel, and L. Leifer (Berlin Heidelberg: Springer), 71–94.
Peppler, K., and Hall, T. (2016). The make-to-learn youth contest: Gaining youth perspectives on learning through making. In Makeology: Makerspaces as learning environments Vol. 1. eds., K. Peppler, E. R. Halverson, and Y. B. Kafai (Routledge), pp. 141–157.
Razzouk, R., and Shute, V. (2012). What is design thinking and why is it important? Rev. Educ. Res. 82, 330–348. doi: 10.3102/0034654312457429
Regalla, L. (2016). “Developing a maker mindset” in Makeology: makerspaces as learning environments. eds. K. Peppler, E. R. Halverson, and Y. B. Kafai, vol. 1 (New York: Routledge), 257–272.
Ryan, R. M., and Deci, E. L. (2000). Intrinsic and extrinsic motivations: classic definitions and new directions. Contemp. Educ. Psychol. 25, 54–67. doi: 10.1006/ceps.1999.1020
Scarbrough, H., Bresnen, M., Edelman, L. F., Laurent, S., Newell, S., and Swan, J. (2004). The processes of project-based learning: an exploratory study. Manag. Learn. 35, 491–506. doi: 10.1177/1350507604048275
Schipper, T., Goei, S. L., de Vries, S., and van Veen, K. (2018). Developing teachers’ self-efficacy and adaptive teaching behaviour through lesson study. Int. J. Educ. Res. 88, 109–120. doi: 10.1016/j.ijer.2018.01.011
Smith, R. C., Iversen, O. S., and Hjorth, M. (2015). Design thinking for digital fabrication in education. Int. J. Child Comput. Interact. 5, 20–28. doi: 10.1016/j.ijcci.2015.10.002
Soltis, J., and Wells, T. (2019). The Impact of Developing a Maker Mindset in an Interdisciplinary Fifth Grade Classroom.
Terzis, L. D., Saltzman, L. Y., Logan, D. A., Blakey, J. M., and Hansel, T. C. (2022). Utilizing a matrix approach to analyze qualitative longitudinal research: a case example during the COVID-19 pandemic. Int. J. Qual. Methods 21:160940692211237. doi: 10.1177/16094069221123723
Veldhuis, A., d’Anjou, B., Bekker, T., Garefi, I., Digkoglou, P., Safouri, G., et al. (2021). The connected qualities of design thinking and maker education practices in early education: a narrative review. Fablearn Europe/MAKEED 2021-an International Conference on Computing, Design and Making in Education, 1–10.
Vongkulluksn, V. W., Matewos, A. M., Sinatra, G. M., and Marsh, J. A. (2018). Motivational factors in makerspaces: a mixed methods study of elementary school students’ situational interest, self-efficacy, and achievement emotions. Int. J. STEM Educ. 5:43. doi: 10.1186/s40594-018-0129-0
Weng, C., Chen, C., and Ai, X. (2023). A pedagogical study on promoting students’ deep learning through design-based learning. Int. J. Technol. Des. Educ. 33, 1653–1674. doi: 10.1007/s10798-022-09789-4
Keywords: lesson study, maker mindsets, stem education, elementary students, design thinking
Citation: Li J, Goei S and Huang R (2024) Unveiling maker mindsets: a journey of formation and transformation through design thinking-making pedagogy within a lesson study context. Front. Educ. 9:1343492. doi: 10.3389/feduc.2024.1343492
Received: 23 November 2023; Accepted: 20 February 2024;
Published: 09 April 2024.
Edited by:
Carola Manolino, Università della Valle d'Aosta, ItalyReviewed by:
Federica Mennuni, University of Bari Aldo Moro, ItalyCopyright © 2024 Li, Goei and Huang. This is an open-access article distributed under the terms of the Creative Commons Attribution License (CC BY). The use, distribution or reproduction in other forums is permitted, provided the original author(s) and the copyright owner(s) are credited and that the original publication in this journal is cited, in accordance with accepted academic practice. No use, distribution or reproduction is permitted which does not comply with these terms.
*Correspondence: Jiajia Li, amlhamlhLmxpQHZ1Lm5s
Disclaimer: All claims expressed in this article are solely those of the authors and do not necessarily represent those of their affiliated organizations, or those of the publisher, the editors and the reviewers. Any product that may be evaluated in this article or claim that may be made by its manufacturer is not guaranteed or endorsed by the publisher.
Research integrity at Frontiers
Learn more about the work of our research integrity team to safeguard the quality of each article we publish.