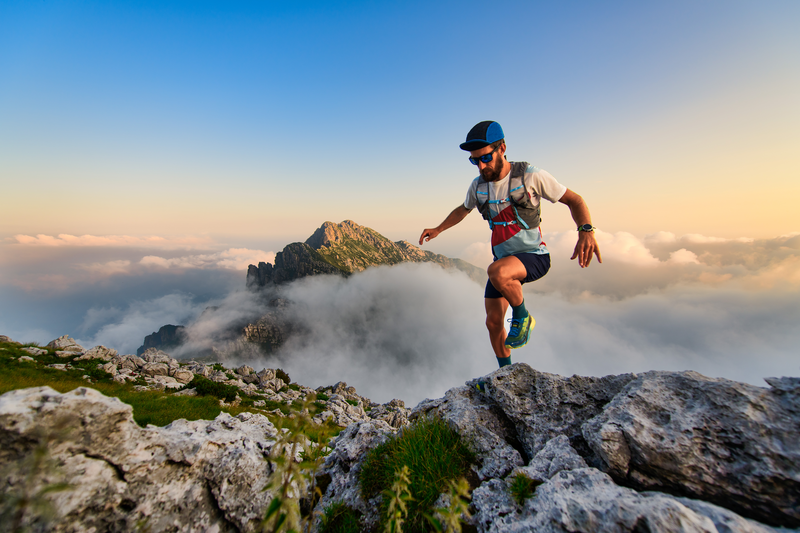
95% of researchers rate our articles as excellent or good
Learn more about the work of our research integrity team to safeguard the quality of each article we publish.
Find out more
ORIGINAL RESEARCH article
Front. Educ. , 12 June 2024
Sec. STEM Education
Volume 9 - 2024 | https://doi.org/10.3389/feduc.2024.1297267
We live in a society in constant scientific and technological change, where engineering education is required to develop the competences needed for future engineers to be able to respond to the complex and interdisciplinary problems that arise from this. It is no longer enough just to provide updated scientific and technological training to students, but it is also required to articulate the needs of society with the design of the curriculum and the expectations of the different stakeholders. The present paper's goal is to present the perceptions about engineering education through the lens of different institutional stakeholders, following a qualitative design study, through semi-structured interviews with seven key-stakeholders representing different scientific and professional institutions. These interviewees play an important role in the definition of guidelines for the engineering profession and in (re)thinking curricula reflecting the interaction with society, training and research. In this sense, it aims to explore the following objectives: (i) to know the perception about the profession, the profile of engineers and the engineering curricula; (ii) to identify the challenges posed to engineers in the job market and in society, today and in the future; and (iii) to reflect on a desirable profile to be attained by engineering students at the end of their Higher Education path. From the interviewees' perspective, competences development effectively involves learning approaches that realize which problem-solving strategies, concepts and capacities for social action should be developed in students, which are not restricted to disciplinary or specific content, but which allow (de)constructing curricula that reflect the different needs of society. In fact, as well as including the point of view of renowned international associations, this article reinforces the idea that the integration of science, technology, engineering and maths (STEM) into curricula is not only a challenge, but also a growing and inevitable phenomenon in response to society's needs. The curriculum, the competences to be privileged, the importance of interdisciplinarity, and the contribution of STEM and STEAM approaches from an integrated engineering perspective are interpreted as difficulties but also the target opportunities.
The United Nations (UN) has established quality education as one of the most important goals of its global sustainable development agenda for the 2020–2030 decade, which translates, in particular, into ensuring university education for all, promoting higher education and equal access for men and women and reducing university dropout, among others (Sá et al., 2022; Burleson et al., 2023; Shephard, 2023). The future engineer must be able to master technical ingenuity and ethically based solutions that are adapted to a constantly evolving environment, while at the same time operate outside their field, solving complex (and unknown) problems of the future in a creative and rapid response manner (Heywood, 2005; Nagel et al., 2019a,b). With the aim of promoting curriculum integration between science, technology, engineering, maths and the arts, encouraging deep and collaborative learning for students, the United States National Research Council proposed, in 2012, STEM (Science, Technology, Engineering and Mathematics) and STEAM (Science, Technology, Engineering, Arts and Mathematics) as a new form of teaching (Videla et al., 2021; Sun et al., 2023). STEAM seems to offer new ways of teaching and learning in the arts and STEM fields, with emancipatory pedagogical approaches, involving the application of mathematics, science, engineering and other subject areas such as social sciences, humanities and the arts to solve real-world problems (Kauffmann et al., 2010; Mejias et al., 2021; Burleson et al., 2023; Miralles-Cardona et al., 2023).
Given that engineering is a field that has direct connection to society, transformation is inevitable when we consider the accelerated changes in society as well as in technology and growing concerns about the environment. In this paper, we present a multi-viewpoint perspective on engineering education according to the perceptions and feedback of seven interviewees. These are representatives from different key-institutions in the field of engineering education and professional engineering development: Public Professional Association representing Engineer Graduates (Ordem dos Engenheiros, Portugal); the Portuguese Society for Engineering Education (SPEE); the European Society for Engineering Education (SEFI), and the Institute of Electrical and Electronics Engineers (IEEE).
The emergence of STEAM methodologies in the field of education has represented ongoing attempts by researchers, teachers and policymakers to make sense of and boost the integration of arts in relation to the learning of science, technology, engineering and mathematics. However, Mejias et al. (2021) draw attention to the fallacy of this claim, which is often masked only by securing funding for the arts and not with the genuine intention of designing and coordinating disciplinary forms of knowledge in the arts and STEM fields. Indeed, STEAM seems to be the answer to society's real problems, requiring integrated and integrative teaching and learning approaches that explore interdisciplinary across different disciplines not so focused on hard or technical skills (Videla et al., 2021; Henze et al., 2022; Meletiou-Mavrotheris et al., 2022; Niu and Cheng, 2022; Buckley et al., 2023). However, the first years of engineering students are commonly spent: “(i) performing mathematical, statistical, chemical, and physical calculations, (ii) studying technical methodologies to solve certain problems, both theoretically and in the laboratory, (iii) developing skills to estimate costs, (iv) understanding the implementation of tools to formulate and manage investment projects, and (v) learning to use software for modeling real phenomena. Although engineering undergraduate programs offer humanistic courses, these are a minor percentage of the number of credits in the curricular plan and do not fully encourage students writing and speaking skills, in comparison with students from Social Sciences, Languages, and Humanities (SSLH) scientific areas” (Cacciuttolo et al., 2023, p. 2). Solving real problems implies thematic knowledge and procedural knowledge of how, why and when to apply the knowledge, in other words, it translates into knowledge that can be transferred to various contexts, true deep learning (Meletiou-Mavrotheris et al., 2022; Niu and Cheng, 2022; Buckley et al., 2023).
Twenty-first-century engineers (here seen in a holistic way) are expected to be proficient in many skills including communications, finance and economics, law and ethics, management, engineering heritage, human behavior, social factors and politics, in parallel with technical aspects such as design skills (Grigg et al., 2004; Heywood, 2005; Chong et al., 2022). These demands have been triggering the scientific community to debate topics related to the required changes needed in Engineering Education (Hadgraft, 2021), the value of engineering, and the skills employers look for in engineers (Trevelyan and Williams, 2019). Regarding the definition of “value”, Trevelyan and Williams for instance stated that this “is a contested term with many different interpretations, though nearly all associate value creation with innovation and entrepreneurship” (Trevelyan and Williams, 2019, p. 461), where innovative and entrepreneurial attitudes by engineering students can be seen as common grounds and requirements for the future.
In the meanwhile, it is worth noting the studies carried out by the Australian Council of Engineering Deans on the definition of the key graduate competencies, having identified several necessary changes in engineering education like (Hadgraft, 2021):
• a re-balancing of the theory-practice requirements of engineering practice;
• the inclusion “real-world” problems that respond to society's needs;
• the need for greater exposure to digital engineering right from initial training;
• a higher practice of e-learning tools and work-integrated learning; and
• the higher need of sharing of good practices, for the effectiveness of processes in a fast-changing world.
Several researchers have pointed to STEM and STEAM approaches as promoting the sensory-motor involvement that underpins the learning experience and contributing to the student's progressive involvement in different situations (e.g. Buhrmann et al., 2013; Hutto and Myin, 2013): help students to apply strategies, knowledge, and skills to new circumstances (e.g. Kelley and Knowles, 2016); enable cooperative learning; stimulates learning through creative problem solving (Wynn and Harris, 2012). However, Videla et al. (2021) list a number of gaps in STEM and STEAM approaches that should be taken into account when applying these methodologies:
(i) “Lack of research on STEAM framed from an enactive-ecological approach;
(ii) Technical foundations on principles of integrated teaching and learning in STEM/STEAM contexts, inside and outside of the classroom;
(iii) Incorporation of mixed reality (XR) as an educational technology approach within the STEAM framework from an enactive-ecological approach;
(iv) Unified enactive-ecological model from dynamic systems theory that allows understanding how students reconfigure their perception of effective action opportunities in digital and/or analog STEAM environments” (p. 2).
On the other hand, STEM and STEAM approaches should involve different competencies such as sustainability competencies like those described at academic report “Framework for education for sustainability: enhancing competences in education”:
(i) Competency in systemic thinking based on a broader, holistic perspective in solving sustainability problems;
(ii) Anticipatory competences that allow to anticipate future scenarios and possible trajectories (sustainability subjects, imagination, creativity, for example);
(iii) Normative competency, which translates into the ability to define, clarify, implement, conciliate and negotiate values and principles, objectives and goals;
(iv) Strategic competency makes it possible to avoid undesirable scenarios (interventions, transitions, transformative governance strategies) by designing and implementing strategic actions aimed at sustainability;
(v) Interpersonal competency interconnects and is indispensable to the application of all the other sustainability competencies because it is related to learning and involves the application of knowledge and social skills such as knowing how to communicate, deliberate, negotiate, collaborate, lead and empathize, among others (Henze et al., 2022; Niu and Cheng, 2022; Sá et al., 2022; Shephard, 2023; Sun et al., 2023).
Traditionally focused on theoretical knowledge, the engineering education claims for innovative teaching methods integrating sustainability into engineering education (Hu et al., 2023). Several Stakeholders are unanimous in the need for an engineering curriculum that focuses on change, the creation and addition of value and the development of transversal skills in engineering training. Below we will summarize these three demands: change, values and skills.
In the United States (US), a change in engineering education has been carried out by Boeing (Najdanovic-Visak, 2017), while in the United Kingdom a recent IET skills survey has provided a stark assessment with a several number of reports that leveling criticism the engineering education process. Some examples of the well-rehearsed arguments include investigations of the “pipeline” of school dropouts on engineering courses (McMasters, 2004), the difficulties faced by underrepresented groups to enter engineering education paths (Perkins, 2013) and reflection on the skills developed during university-level education (MacDonald, 2014). In addition, the Royal Academy of Engineering produced a couple of reports which looked at the process of “educating engineers for the twenty-first century” from both the industry (Morgan and Ion, 2014) and the academic viewpoints (Spinks et al., 2006), highlighting skills shortages and skills gaps in the resulting graduate population.
The call for change seems clear. For Freeman et al. (2014) and Mitchell et al. (2021), to address the curricular objectives, active learning as a curricular strategy was a key strategy, especially problem-based learning and its variants (Rauhut, 2007; Freeman et al., 2014) from what Kolmos (2017) described as a “Mode 1”, higher education institutions where emphasis is placed on theoretical learning, toward “Mode 3”, where a greater focus is posed on social progress (Mitchell et al., 2021). This active learning philosophy (Kolmos, 2017), more than an all-encompassing ideology, it was used as a way to connect the curriculum (Freeman et al., 2014). Being such a crucial factor, curriculum design processes require therefore careful prior considerations in order to properly highlight areas where innovation is really needed, keeping unchanged areas that do not need intervention (Freeman et al., 2014).
According to Simpson et al. (2008) and Biesta (2014), while considered to be the process of learning for life, the curriculum includes a framework that determines the context for transforming information into applicable knowledge by means of a number of activities (Biesta, 2014; Christie and Graaff, 2017). When occurring, the practice of engineering in highly complex environments and the need to prepare students for these scenarios are stimulating the reform and innovation of engineering education (Simpson et al., 2008; Najdanovic-Visak, 2017; Burleson et al., 2023).
Stouffer and Russell (2003) consider civil engineering students, for instance, still have little exposure to the liberal arts or critical professional skills (Bell et al., 2019). Additionally, in most curricula, the underlying value of disciplinary toolsets that are not really engineering tools has been little explored (Stouffer and Russell, 2003). In general, the non-engineering disciplinary toolsets like liberal arts might include political science and public administration, law, economics, history, sociology, behavioral science, finance, and economics.
In this sense, Russell and Stouffer (2005) propose liberal arts education with the aim of challenging students to go beyond their limitations, explore the world, and develop their intellect in reasoning, judgement and communication, in order to become better people, citizens and, consequently, engineers (Masterton and Jeffrey, 2020). So, what are the contributions of STEM and STEAM approaches in curricula to deep learning that corresponds to the needs of society? According to Videla et al. (2021), the design of “STEM/STEAM educational environments is based on interdisciplinary practice that favors enactive and ecological learning, as well as didactic co-design” (p. 5). The idea behind STEM is to connect the sciences with methods that encourage creativity and innovation (STEAM approach) to promote learning in more connected and holistic ways (Henze et al., 2022; Meletiou-Mavrotheris et al., 2022; Niu and Cheng, 2022; Miralles-Cardona et al., 2023; Sun et al., 2023).
In the US, Lafayette College decided, in the Bachelor of Arts in Engineering degree program, to integrate engineering and liberal arts with the aim of bridging the two disciplines, educating “socio-technical engineers”, from 1970 until today (Chong et al., 2022). This engineering approach was initiated as learning communities where several institutions, recognizing the opportunity, have established programs to develop academic and social connections (Gabelnick et al., 1990; Russell and Stouffer, 2005). Authors like Shapiro and Levine (1999) and Kim et al. (2016) claim that engineering curricula needs to be reformed by academics and industry professionals. For example, in civil engineering area, The Royal Academy of Engineering (2007) expressed concern that recent graduates were unable to apply their technical knowledge to real-world problems (Kim et al., 2016).
Industry representatives (The Royal Academy of Engineering, 2007; Najdanovic-Visak, 2017) professional institutions (National Academy of Engineering, 2004; CBI, 2009; MacDonald, 2014; Morgan and Ion, 2014), and government actors (Chong et al., 2022) enforced the need for broadening of curricula. All have emphasized the need for revised curricula encompassing areas such as the engineering's role within society, together with a whole set of transversal skills such as: critical thinking, teamwork, socio-economic considerations, sustainability, ethics, holistic and transdisciplinary teaching and learning approaches, troubleshooting and entrepreneurship (Freeman et al., 2014; Sá et al., 2022; Burleson et al., 2023). In fact, integrated curriculum initiatives, although classified as a learning community, can assume a broader context than a learning community, namely by encompassing several curricular initiatives (Rauhut, 2007). The concept of an “integrated engineering curriculum” can be sustained in the way engineering is defined and how it is practiced.
Froyd and Ohland (2005) and Swartz et al. (2019) censure the misalignment between engineering practice and education, considering that engineering practice highlights the integration between social and technical dimensions (which is not the case in conventional engineering education). That is, while engineering education tends to teaching traditional technical concepts, society is inherently a socio-technical ecosystem (Chong et al., 2022), which requires collaborative and interdisciplinary solutions, driven by professionals with a solid grounding in engineering and the liberal arts (Swartz et al., 2019). In turn, The American Society of Civil Engineers' (ASCE) Body of Knowledge (BOK2) also emphasizes that disciplines such as the humanities and social sciences are fundamental to the technical training of engineers (Bernhardt and Rossmann, 2019).
One of the goals of engineering education in this new era of the global economy, as provided during graduation, is to ensure that graduates succeed in work and life (American Society of Civil Engineers, 2008). According to Jacolbia (2016), communication skills are essential for successful job engineering careers, while Zaharim et al. (2010) and Kaushal (2018) emphasized the importance, in industry, of communication skills, having suggested methods for improving these skills, and also recommended integrating employability skills with engineering education. Zaharim et al. (2010), Kaushal (2018) and Campos et al. (2020) have deliberated different soft skills required for better employment and categorized them into six groups, namely: (i) Problem Solving; (ii) Communication; (iii) Teamwork; (iv) Ethical Perspective; (v) Emotional Intelligence and (vi) Creative Thinking. The same authors also suggested how these skills can be included to make fresh-graduate engineers aligned to the markets' needs, while successful training on these skills is advocated as a need for engineering curricula.
Itani and Srour (2016) have concluded that students can show a significant understanding of the importance of soft skills. In order to strengthen students' non-technical skills in specific areas, such as oral communication skills, for example, the same authors recognize the fundamental role of educational institutions. The contents of different courses, the design of the course curriculum, and teaching and learning processes, are also found as important factors in preparing a graduate to face societal and market challenges (Campos et al., 2020; Gope and Gope, 2022; Sá et al., 2022). Nevertheless, the level of transformational aspects needed can lead to several challenges for institutions (and their faculty) when trying to stability different curriculum demands (Gilleard and Gilleard, 2002; Heywood, 2005; Wu et al., 2023).
For Simpson et al. (2008), the aim of the curriculum is to strike the right balance between underlying science and analysis, and learning from experience. In this sense, there are several methods that may be used to provide a transformative learning experience that allows students to get involved. For example, a problem-based learning (Siller et al., 2009; Davies, 2013), including analysis and learning through failure (Dym et al., 2005); and research-based learning (Cavalline and Delatte, 2015). In this sense, for Sunthonkanokpong (2011) and Gosper and Ilfenthaler (2014), if the curriculum offers students diverse pedagogical approaches in the early stages of their learning experience and exposes them to diverse learning opportunities, it helps them to develop life-long learning skills. In this way, it is enough to ensure an appropriate balance between individual learning, collaborative learning, face-to-face teaching and online teaching (Sunthonkanokpong, 2011), where the “deep learning” must be accounted for in the design of the curriculums. All these redesigning take a team and a concrete aim to be successfully implemented, because for instance Case and Marshall (2004) highlighted “deep learning” requires the teaching team to use teaching methods that promote the effective involvement of students in their own learning, ensuring a balance between problem-solving procedures and conceptual understanding (Paechter and Maier, 2010).
Thus, the curriculum determines the skills, knowledge and professional attributes a graduate will develop and, also, how prepared they are for employment (Case and Marshall, 2004). In a broader sense, and besides the goals stated before, curricula should be able to enable and empower students to engage with risk-taking and create innovative solutions (Knight and Yorke, 2002; Froyd and Ohland, 2005; Trevelyan and Williams, 2019).
Many important institutions have been pointing the focus on creating curriculum, and quality and evaluation practices that recognize and support the holistic student experience. In the UK, the National Student Survey (NSS), the Teaching Excellence Framework and the Higher Education Academy are driving the increasing use and communication of innovative approaches to teaching and student assessment, requiring academics to act as catalysts and promoters of change (Heywood, 2005). The factors that are seen as relevant in a process of curricula design and delivery include the:
(i) understanding that engineering design is an interdisciplinary field of study that involves a range of additional skills and knowledge not related to engineering (Davies, 2013);
(ii) provision of support for individuals in developing personal, presentational, communication and listening skills (Douglas et al., 2006; Itani and Srour, 2016);
(iii) supporting individuals in developing skills, tools and resources such as note-taking or diagramming in order to articulate innovation with content (Moseley et al., 2005);
(iv) exposing individuals to different ways of thinking and solving problems, developing concepts, creating opportunities (Biesta, 2014);
(v) creating opportunities for group and teamwork, with a focus on design and innovation, favoring collaboration (MacDonald, 2014);
(vi) encouraging innovative approaches (Salter and Gann, 2003);
(vii) incorporate an understanding of risk and failure into innovation and provide the necessary tools to analyze failures and determine their causes (Biesta, 2014);
(viii) developing an understanding of the user's perspective, objectives and goals (O'Connor et al., 2008);
(ix) increasingly electronically structured access to and sharing of information (Biesta, 2014);
(x) legislation, on intellectual property for example, and its impact on the design process (Biesta, 2014);
(xi) grading systems for projects involving student collaboration that recognize different skills (Joshi et al., 2012); and
(xii) quality mechanisms and their operation (Gavin, 2011).
Considering the review on alignment and misalignment between engineering courses and societal needs, as described in the previous section, a constructive higher education learning experience has been argued with questions like how students learn, how they learn to work effectively in groups and beyond the limits of a single discipline; how they learn to interpret behaviors and to identify needs; how to develop effective communication skills using the right technologies and keeping up to date with technological developments for secure information sharing (Chong et al., 2022). Currently, the search for a globally relevant academic curriculum has become imperative, where globalization appears as an opportunity to reform engineering education and rethink the role that engineers can play in a world of constant and accelerated change (Heywood, 2005; Chong et al., 2022).
This study intends to further explore the answers that are being given by the current engineering curricula to the demands of society, as well as acknowledge the challenges posed to new engineers in the future.
Therefore, this study following a qualitative research design through semi-structured interviews aims to answer the following research objectives: (i) to know the perception about the profession, the profile of engineers and the engineering curricula; (ii) to identify the challenges posed to engineers in the job market and in society, today and in the future; and (iii) to reflect on a desirable profile to be attained by engineering students at the end of their Higher Education path.
We interviewed seven key-representatives from different institutions and positions (four women and three men were interviewed; their average age was 58; seven were Portuguese and one English): Public Professional Association representing Engineer Graduates (Ordem dos Engenheiros, Portugal); the Portuguese Society for Engineering Education (SPEE); the European Society for Engineering Education (SEFI), and the Institute of Electrical and Electronics Engineers (IEEE).
The different institutions the interviewees represent play an important role in the definition of guidelines for the engineering profession and in (re)thinking curricula considering the interaction with society, training and research. Interviewees were contacted by email, as senior figures in the institutions they represent, and besides detailed information about the aims and the methodological aspects of the interview, a summary of the project was provided. They had no prior access to the questions and all signed an informed consent statement that guaranteed the confidentiality of their data and identity and the possibility to withdraw from the interview whenever they wished. The interviews took place in person, with an average duration of 1 h and 30 min, being recorded, after asking for permission, and transcribed in full.
Based on the literature review provided in the previous sections, with the challenges engineering education is facing in the present societal context, an interview campaign with two dimensions was designed and implemented. Two researchers were involved in the coding process. The dimensions and categories presented result from a closed co-coding process. In the coding process, five dimensions were recognized: “Strengths”; “Challenges”; “Good Practice” cases; “Information” and “Suggestions”. The relevant previous dimensions considered in this study and focus through the interviews focused on Social Representations and Perceptions and Engineer Curriculum, and divided in the following dimensions:
• “Challenges”:
What are the challenges for the engineering professional (nowadays and in the future)?
What is the answer given by current engineering curricula to the challenges imposed for the formation and professionalization of engineers?
• “Strengths”:
What aspects/dimensions would be important to maintain, improve, or change in engineering curricula?
In the “Challenges” dimension, four categories were identified: “Educational Context” (two subcategories: “Curriculum Design” and “Institutional Management”); “Social Representations” (four subcategories: “Expectations of different stakeholders”, “Profile of the Engineering professional”, “Social representations about the course and profession” and “Inclusion”); “Students” (four subcategories: “Engineering student profile upon entry into Higher Education”, “Profile of engineering students leaving Higher Education”, “Profile of the engineering professional” and “Social representations about the course and profession”) and “Resources” (two subcategories: “Human resources” and “Material resources”).
In turn, in the “Strengths” dimension, five categories were identified: “Educational Context” (two subcategories: “Curriculum Design” and “Institutional Management”); “Engineer Profile” (two subcategories: “Engineering Professional Profile” and “Employability Skills”); “Students” (two subcategories: “Curriculum Design” and “Profile of the Engineering student leaving Higher Education”); “Teachers” (two subcategories: “Scientific Skills” and “Professional Skills”); “Teaching and Learning” Process (five subcategories: “Adequacy of activities to students” expectations”, “Adequacy of activities to the challenges of future employers”, “Promotion of diverse skills”, “Articulation between Subjects” and “Teaching Strategies”).
A limitation of the study is the limited time to identify and analyse more dimensions in time to be included in this article. On the other hand, the possibility of interviewing other stakeholders such as, for example, employers.
Qualitative data analysis was performed through thematic analysis, which is a flexible procedure recommended when one has specific research questions to be answered by qualitative data collected through interviews (Boyatziz, 1998; Braun and Clarke, 2006). The main themes were identified previously and according to the main objectives of the interview (also expressed in the questions presented in the interview protocol). The patterns and themes within these main ones were aggregated according to the level of systematicity that the idea or topic was presented.
The analysis was focused on the “Challenges” and “Strengths”, gathering the main positions of the interviewees regarding the different aspects of the engineering curriculum answer to the demands of a society, the (des)alignment between the engineering curricula and these needs, and the challenges of being an engineer nowadays that were approached.
Regarding the Engineer Curriculum, the interviewees pointed to the need to integrate content from other areas such as arts and humanities, which points to STEAM approach, in order to improve communication skills, problem solving and team management skills:
“I think that half of the curriculum should clearly be in engineering and the other half optionally, but indexing to other valences that engineers may have… arts, humanities, why not? (interviewed C);
“(…) but somehow to create in these such complementary contents in the formation of an engineer, to create a chair [curricular unit] of an intervention strategy” (interviewed D).
On the other hand, it was also referred the need to integrate the learning of subjects such as mathematics in the context of fluid mechanics or a specific curricular unit of the course:
“The role of Mathematics, the type of Mathematics that is part of the curriculum needs to be rethought and placed in the context where it will truly be essential” (interviewed B).
It was even discussed the possibility of rethinking what level and type of mathematic problems engineers put into practice in their current daily bases work and readjusting the curriculum according whit their needs. However, this perspective revealed to be controversial even between the interviewers of this study. Effectively, according to one interviewee, one of the great strengths of engineering education is the strong training in mathematics, physics and chemistry and in his opinion, it is important to stress it and keep it:
“It is essential to maintain the strong technical component. What sets engineering courses apart is the focus on mathematics, physics and chemistry.” (interviewed E).
The Social Representations and Perceptions represent a dimension that includes all the interviewees' representations and perceptions of the course and the profession, namely the profile needed to be an engineer. But this dimension also brings challenges. Challenges that materialize in the educational context (curriculum design and institutional management); the students (profile of engineering students upon entering Higher Education; profile of engineering students upon leaving Higher Education; profile of engineering professionals) and the resources (human and material). The requirement for soft skills, hard skills, and social skills is growing very fast to meet the day-to-day challenges (Campos et al., 2020). Therefore, the design of an engineering curriculum must consider the transmission of these skills and the satisfaction of society's needs and demands. Undergraduate education is required to equip students with the skills to solve interdisciplinary engineering challenges across technical and non-technical boundaries, communicate and transfer knowledge. To train engineers in these skills, one of the proposed approaches is to teach design inspired by an engineering curriculum, which offers relevance to professional practice, as well as to frame complex and interdisciplinary problems (Najdanovic-Visak, 2017; Nagel et al., 2019a,b; Trevelyan and Williams, 2019; Hu et al., 2023). Camargo Ribeiro and Mizukami (2005) and Heywood (2005) recognized that an engineering curriculum integrating the liberal arts would be highly relevant for the training of future engineers, as it would provide for the development of professional competences and attitudes that are indispensable to their practice, such as autonomous and lifelong learning, critical thinking, initiative, creativity, teamwork skills, responsibility toward society and their profession, entrepreneurship and ethics. However, according to the same authors, as well as being a necessity, it would also be a challenge.
Another dimension that may help us to understand the interviewees views on these topics was Strengths. According to the interviewees, there are strengths and value that contribute to the enrichment of training, to the training of better professionals and better citizens, and to a better contribution to the challenges of society.
Considering the Social Representation and Perceptions, all interviewees are unanimous in identifying the engineer as a professional who solves problems. For this, he has (or must have) technical and non-technical training and therefore competences. Consequently, the engineering curriculum has a strong scientific component, where multiple experiences as well as differentiated learning strategies should be provided (e.g., PBL, CBL) and its appreciated as an added-value:
”(…) because I think that often what has to be adapted is not the content, but the way of teaching it” (interviewed F);
“(…) what is distinctive about the engineering curriculum is the teaching of the basic sciences” (interviewed E);
“I try to offer other experiences. we have a partnership with another humanities teacher where they carry out projects together. (…) other strategies… PBL, CBL” (interviewed A).
To others, Higher Education Institutions are not the only ones responsible for providing future professionals with all the skills essential to their success. They agree that the curriculum must also be built by the students themselves, either with extracurricular activities or volunteer actions, and when that happens it increases the potential of involvement and competence development of the training:
“But from the moment that the person gives him, in each of the disciplinary areas, the basis for, I'm just talking about the technical skills, I think that later he [student] is able to adjust, right? Because if we are going to give what is now in fashion, artificial intelligence for example, it is not enough because ‘Okay, let's lay the groundwork', but maybe when he goes to work, things, the panorama, photography are already different, but if he knows how to learn, therefore, in skills quickly overcomes” (interviewed A);
“I had a student who went to a job interview and she told me:—I think I was hired more for my extracurricular activities than for the course I took” (interviewed A).
On the other hand, engineer profile (as a professional who solves problems); teachers (the partnerships that are built between different areas of knowledge) and teaching and learning process (multiple experiences and learning strategies) were considered strengths that contributes to thinking and enriching the curriculum. For the International Engineering Alliance (2021), “The fundamental purpose of formative development is to build on the educational base to develop the competences required for independent practice in which the graduate works with engineering practitioners and progresses from an assisting role to taking more individual and team responsibility until competence can be demonstrated at the level required” (p. 3). In this sense, the recognition of graduates of accredited programmes from each signatory by the other signatories has been provided for in various agreements. These Accord programmes define a set of requirements in terms of, for example, the profile of knowledge and learning to be achieved by graduates. Therefore, without reference to the design of programmes that would achieve the requirements: (i) The Washington Accord (WA) provides for the mutual recognition of accredited engineering programmes; (ii) The Sydney Accord (SA) establishes mutual recognition of accredited qualifications for engineering technologist; (iii) The Dublin Accord (DA) provides for mutual recognition of accredited qualifications for engineering technicians. The aim of these agreements is not to match content and results, but to ensure that the principle of substantial equivalence prevails. These documents therefore recognize the attributes of graduates that they consider essential. For example, the Canadian Engineering Accreditation Board (CEAB) identified 12 attributes necessary for graduating engineers include: (1) A knowledge base for engineering; (2) Problem analysis; (3) Investigation; (4) Use of engineering tools; (5) Design; (6) Individual and team work; (7) Communication skills; (8) Professionalism; (9) Impact on society and environment; (10) Ethics and Equity; (11) Economics and (12) Lifelong Learning (Dew et al., 2011).
In fact, we can recognize different perspectives on the curriculum, the competences to be privileged, the responsibility and functions of higher education institutions, the importance of interdisciplinarity, the contribution of Social Sciences, Humanities and Arts from an integrated engineering perspective. Through the program curriculum, engineering educators have continually examined approaches to prepare graduates with the needed skills consider not only the definition of engineering curriculum based on the ‘value' of engineering but also the concept of the engineering approach to problem-solving (Tannock and Burge, 1994; Kauffmann et al., 2010; Hadgraft, 2021). With an exclusive focus on the engineering curriculum, the main ideas to be extracted from the interviews are (i) rethinking the curriculum, with profound restructuring at the level of Mathematics, for example in the selection of content, in identifying content that is really essential in the day-to-day life of an engineer); (ii) it is essential to develop soft skills, transformative skills and spatial skills in students, but they should not be the only objective; (iii) the importance of promoting interdisciplinarity by focusing on the contribution of differentiating approaches from Social Sciences, Humanities and Arts.
Understanding that the profile of the engineering professional is closely associated with problem-solving, the interviewees, while recognizing the need for various changes already listed, emphasize the importance of mathematics in the profession and do not give it up as one of the fundamental pillars of training (Goold, 2014; Jayanthi, 2019):
“You have to understand that maths is essential in the professional life of any engineer. It is an essential pillar in engineering training.” (interviewed E).
The alignment between the importance of maths in engineering education and its impact on science and society seems to be in line with the idea of Jayanthi (2019), who states that mathematical skills and competences are at the basis of a nation's development, as they drive its scientific and technological components:
“Maths is present in everything we do in life. Even more so for an engineer. How will they understand the world around them, find solutions to society's problems, if they don't have a strong maths component in their training?” (interviewed E).
Increasingly indispensable in everyday life and in solving problems, maths today is a diverse subject that goes beyond arithmetic and geometry. Mathematics helps us to understand the world around us, not only with data, measurements and observations from science, with inference, deduction and proof, but especially with mathematical models of natural phenomena, human behavior and social systems (Jayanthi, 2019). However, according to Goold (2014), a weakness in engineering education is the difficulty engineering graduates have in communicating maths, not only because of their own relationship with maths, but also because of sociocultural, discursive and psychoanalytical factors.
The present study pretends to highlight the perspectives of key institutional stakeholders about the curricula of engineering curriculum. However, some limitations should be accounted for, namely, the number of participants is restricted and while they are presenting the institutional perspective it might be embedded in their personal views.
In future works, it would be relevant to extend the study to different groups of stakeholders directly involved in Engineering Education and training, such as teachers, students, Higher Education Institutions representatives, engineers (alumni) and employers. It is also critical to have a robust and balanced sample from the different groups and positions on the topic.
This study aims to expose the challenges and strengths identified by different institutional stakeholders for the development of the engineering curriculum. In this sense, different perspectives were identified. According to Roth (2014), “(…) engineering representations are in the mind because they are integral to the societal relations engineers entertain” (p. 67). The curriculum, the competences to be privileged, the responsibility and functions of Higher Education Institutions, the importance of interdisciplinarity, the contribution of Social Sciences, Humanities and Arts (STEAM approach) from an integrated and sustainability engineering perspective are really changelings and the approaches to accomplish it are not consensual. Engineering, as one may see in all the creative arts, requires professionals with a wide set of skills, knowledge and attributes, e.g., competences. While there is consensus on the need for a solid foundation in science, mathematics and the underlying technical knowledge typically associated with engineering, it is not enough (Freeman et al., 2014) to solve real-world problems. In order to satisfy the requirements of the accreditation body and the needs of the employer, these must be integrated with technical and non-technical skills (Heywood, 2005).
However, they are also the target of challenges and difficulties. The interviewees in this study pointed out the difficulty in provoking more structural and curriculum-based changes; the mobilization of teachers to establish partnerships and for training in engineering education and in more STEAM approaches. While not intended to be representative of all stakeholders, the preliminary findings described above point to areas where perhaps more emphasis should be placed, considering the stakeholders, societal needs and the literature advocating a wide range of engineering competences: such as the ability to work in groups and teams and carefully analyze a problem and its requirements, opportunities to develop understandings and experiences that take into account more interdisciplinary and complex contexts. However, the respondents also seemed to be more likely to emphasize the requirement for soft skills and social skills in the curriculum, but not forgetting the great importance that hard skills have in engineering. Thus, “The fundamental purpose of engineering education is to build a knowledge base and attributes to enable the graduate to continue learning and to proceed to formative development that will develop the competences required for independent practice” (International Engineering Alliance, 2021, p. 3).
In turn, focusing on engineering for sustainable, Burleson et al. (2023) synthesize some emerging trends and proposes recommendations with “the adoption of emerging cultural mindsets, which include: (1) take an interdisciplinary and multi-stakeholder approach, (2) consider dynamic and interconnected systems, (3) increase humility and intercultural competence, (4) prioritize diversity and inclusion, (5) increase localization and center community perspectives, (6) challenge the perception that engineering is neutral, and (7) broaden the goals of engineering” (p. 1).
So, with different perspectives here recognized, how can Higher Education Institutions design a curriculum that responds to all these perspectives and difficulties? What is the attitude of teachers toward the adoption of STEAM and STEAM tools in the formal and non-formal teaching context? Who can contribute to this reflection? How to identify conditions or requirements that could provide more inclusive engineering curricula? In future works, it becomes imperative to listen to other stakeholders such as students, alumni, and employers, in search of perspectives that can contribute to a more integrated approach to the different perspectives regarding answering these questions.
With a focus on the engineering curriculum, the need to include other areas such as arts and humanities, soft skills and, in general, a STEAM approach became clear, with the aim of promoting skills in communication, problem-solving and teamwork for example. But don't forget that one of the great strengths of engineering education is the strong training in mathematics, physics and chemistry. The real challenges for engineering curriculum were seem by the interviewees as present in the course and in the profession; in the engineering professional profile; in the expectations; in the desirable inclusive and sustainability engineering curriculum. Why? Because the stakeholders' social representations of the course and the profession condition the whole process of choosing the course, the curriculum and the teaching and learning process.
The raw data supporting the conclusions of this article will be made available by the authors, without undue reservation.
The studies involving humans were approved by Ethics and Deontology Committee, University of Aveiro. The studies were conducted in accordance with the local legislation and institutional requirements. The participants provided their written informed consent to participate in this study.
CFe: Writing – original draft, Writing – review & editing. BG: Writing – review & editing. RV: Writing – review & editing. CFi: Writing – original draft, Writing – review & editing.
The author(s) declare that financial support was received for the research, authorship, and/or publication of this article. This work was supported by FCT grant EXPL/CED-EDG/1009/2021.
The authors declare that the research was conducted in the absence of any commercial or financial relationships that could be construed as a potential conflict of interest.
All claims expressed in this article are solely those of the authors and do not necessarily represent those of their affiliated organizations, or those of the publisher, the editors and the reviewers. Any product that may be evaluated in this article, or claim that may be made by its manufacturer, is not guaranteed or endorsed by the publisher.
American Society of Civil Engineers (2008). Civil Engineering Body of Knowledge for the 21st Century. Available online at: https://ascelibrary.org/doi/epdf/10.1061/9780784409657
Bell, S., Chilvers, A., Jones, L., and Badstuber, N. (2019). Evaluating engineering thinking in undergraduate engineering and liberal arts students. Eur. J. Eng. Educ. 44, 429–444. doi: 10.1080/03043797.2018.1552663
Bernhardt, K. L. S., and Rossmann, J. S. (2019). “An integrative education in engineering and the liberal arts: an institutional case study,” in 2019 ASEE Annual Conference & Exposition, (Tampa, Florida: ASEE).
Biesta, G. (2014). Pragmatising the curriculum: bringing knowledge back into the curriculum conversation, but via pragmatism. Curricul. J. 25, 29–49. doi: 10.1080/09585176.2013.874954
Boyatziz, R. (1998). Thematic Analysis and Code Development: Transforming Qualitative Information. Thousand Oaks, CA: Sage Publications.
Braun, V., and Clarke, V. (2006). Using thematic analysis in psychology. Qual. Res. Psychol. 3, 77–101. doi: 10.1191/1478088706qp063oa
Buckley, J., Hartell, E., and Blom, N. (2023). Editorial: Current perspectives on the value, teaching, learning, and assessment of design in STEM education. Front. Educ. 8:1247618. doi: 10.3389/feduc.2023.1247618
Buhrmann, T., Di Paolo, E. A., and Barandiaran, X. (2013). A Dynamical Systems Account of Sensorimotor Contingencies. Front. Psychol. 4:285. doi: 10.3389/fpsyg.2013.00285
Burleson, G., Lajoie, J., Mabey, C., Sours, P., Ventrella, J., Peiffer, E., et al. (2023). Advancing sustainable development: emerging factors and futures for the engineering field. Sustainability 15:7869. doi: 10.3390/su15107869
Cacciuttolo, C., Vásquez, Y., Cano, D., and Valenzuela, F. (2023). Research thesis for undergraduate engineering programs in the digitalization era: learning strategies and responsible research conduct road to a university education 4.0 paradigm. Sustainability 15:11206. doi: 10.3390/su151411206
Camargo Ribeiro, L. R., and Mizukami, M. D. G. N. (2005). Student assessment of a problem-based learning experiment in civil engineering education. J. Prof. Issues Eng. Educ. Pract. 131, 13–18. doi: 10.1061/(ASCE)1052-3928(2005)131:1(13)
Campos, D. B., Resende, L. M. M., and Fagundes, A. B. (2020). The importance of soft skills for the engineering. Creat. Educ. 11, 1504–1520. doi: 10.4236/ce.2020.118109
Case, J., and Marshall, D. (2004). Between deep and surface: procedural approaches to learning in engineering education contexts. Stud. Higher Educ. 29, 605–615. doi: 10.1080/0307507042000261571
Cavalline, T., and Delatte, N. (2015). Learning from the World Trade Centre collapse – use of a failure case study in a structures and materials laboratory course. J. Eng. Technol. 32, 2–17. Available online at: https://www.researchgate.net/publication/303753676_ETD_BEST_PAPER_2015_Learning_from_the_World_Trade_Center_Collapse-Use_of_a_failure_Case_Study_in_a_Structures_and_Materials_Laboratory_Course
Chong, R., Belayutham, S., Ibrahim, C., and Adhikari, S. A. (2022). Theoretical exploration on the standing of liberal arts in the civil engineering curriculum. Environm.-Behav. Proceed. J. 7, 77–83. doi: 10.21834/ebpj.v7i19.3261
Christie, M., and Graaff, E. (2017). The philosophical and pedagogical underpinnings of active learning in engineering education. Eur. J. Eng. Educ. 42, 5–16. doi: 10.1080/03043797.2016.1254160
Davies, H. C. (2013). Integrating a multi-university design competition into a mechanical engineering design curriculum using modern design pedagogy. Journal of Engineering Design, Vol. 24, No. 5, pp. 383–396. doi: 10.1080/09544828.2012.761679
Dew, S., Lavoie, M., and Snelgrove, A. (2011). “An engineering accreditation management system,” in Proceedings of the Canadian Engineering Education Association. doi: 10.24908/pceea.v0i0.3577
Douglas, J., Douglas, A., and Barnes, B. (2006). Measuring student satisfaction at a UK university. Qual. Assur. Educ. 14, 251–267. doi: 10.1108/09684880610678568
Dym, C. L., Agogino, A. M., Eris, O., Frey, D. D., and Leifer, L. J. (2005). Engineering design thinking, teaching, and learning. J. Eng. Educ. 94, 103–120. doi: 10.1002/j.2168-9830.2005.tb00832.x
Freeman, S. S. L, Eddy, M., McDonough, M. K., Smith, N., Okoroafor, H. J., et al. (2014). Active learning increases student performance in science, engineering, and mathematics. Proc. Nat. Acad. Sci. 111, 8410–8415. doi: 10.1073/pnas.1319030111
Froyd, J. E., and Ohland, M. W. (2005). Integrated engineering curricula. J. Eng. Educ. 94, 147–164. doi: 10.1002/j.2168-9830.2005.tb00835.x
Gabelnick, F., MacGregor, J., Matthews, R., and Smith, B. L. (1990). Learning Communities: Creating Connections Among Students, Faculty and Disciplines. San Francisco: John Wiley & Sons.
Gavin, K. (2011). Case study of a project-based learning course in civil engineering design. Eur. J. Eng. Educ. 36, 547–558. doi: 10.1080/03043797.2011.624173
Gilleard, J., and Gilleard, J. D. (2002). Developing cross-cultural communication skills. J. Prof. Issues Eng. Educ. Pract. 128, 187–200. doi: 10.1061/(ASCE)1052-3928(2002)128:4(187)
Goold, E. (2014). “Mathematics: creating value for engineering students,” in 17th SEFI Mathematics Working Group seminar. Available online at: http://sefi.htw-aalen.de/Seminars/Dublin2014/17th%20SEFIMWG%20Seminar/Monday%20Session%201/MWG2014_Goold.pdf (accessed April 12, 2024).
Gope, D., and Gope, A. (2022). Students and academicians views on the engineering curriculum and industrial skills requirement for a successful job career. Open Educ. Stud. 4, 173–186. doi: 10.1515/edu-2022-0011
Grigg, N. S., Criswell, M. E., Siller, T. J., Fontane, D. G., Sunada, D. K., and Saito, L. (2004). Integrated civil engineering curriculum: Five-year review. J. Prof. Issues Eng. Educ. Pract. 130, 160–165. doi: 10.1061/(ASCE)1052-3928(2004)130:3(160)
Hadgraft, R. (2021). “The Engineer of 2035 – What changes in Engineering Education are required?,” in Paper presented at SEFI 2021 Annual Conference, Berlin, Germany. Available online at: https://www.sefi.be/2021/09/27/sefi-2021-annual-conference-keynote-speaker-rodger-hadgraft/ (accessed April 12, 2024).
Henze, J., Schatz, C., Malik, S., and Bresges, A. (2022). How might we raise interest in robotics, coding, artificial intelligence, STEAM and sustainable development in university and on-the-job teacher training? Front. Educ. 7:872637. doi: 10.3389/feduc.2022.872637
Heywood, J. (2005). Engineering Education: Research and Development in Curriculum and Instruction. Hoboken, New Jersey: John Wiley & Sons, Inc.
Hu, A., Mao, X., Fu, C., Wu, M., and Zhou, S. (2023). Engineering curriculum reform based on outcome-based education and five-color psychology theory. Sustainability 15:8915. doi: 10.3390/su15118915
Hutto, D. D., and Myin, E. (2013). Radicalizing Enactivism: Basic Minds without Content. Cambridge, MA: MIT Press.
International Engineering Alliance (2021). Graduate Attributes and Professional Competencies (GAPC). Paris: UNESCO.
Itani, M., and Srour, I. (2016). Engineering students' perceptions of soft skills, industry expectations, and career aspirations. J. Prof. Issues Eng. Educ. Pract. 142, 1–10. doi: 10.1061/(ASCE)EI.1943-5541.0000247
Jacolbia, R. B. (2016). Future educators' perceptions on technology and livelihood education status and development of work skills. J. Adv. Humani Social Sci. 2, 85–97. doi: 10.20474/jahss-2.2.3
Jayanthi, R. (2019). Mathematics in society development - a study. IRE J. 3, 59–64. Available online at: https://www.irejournals.com/formatedpaper/1701630.pdf
Joshi, S., Morkos, B., Shankar, P., Summers, J. D., and Mocko, G. M. (2012). Requirements in engineering design: what are we teaching? Tools Methods Comp. Eng. 1, 1319–1326. Available online at: https://www.academia.edu/2812900/REQUIREMENTS_IN_ENGINEERING_DESIGN_WHAT_ARE_WE_TEACHING
Kauffmann, P., Sullivan, S., Dixon, G., and Kim, B. (2010). “Integration of engineering economics, statistics, and project management: reinforcing key concepts,” in 2010 Annual Conference & Exposition. Louisville, Kentucky. doi: 10.18260/1-2–16551
Kaushal, U. (2018). Integrating employability skills in engineering education. J. Adv. Humani Social Sci. 3, 179–185. doi: 10.26500/JARSSH-03-2018-0503
Kelley, T. R., and Knowles, J. G. (2016). A conceptual framework for integrated STEM education. Inter. J STEM Edu. 3:11. doi: 10.1186/s40594-016-0046-z
Kim, J., Schmöcker, J. D., and Fujii, S. (2016). Exploring the relationship between undergraduate education and sustainable transport attitudes. Int. J. Sustain. Transp. 10, 385–392. doi: 10.1080/15568318.2014.961108
Knight, P. T., and Yorke, M. (2002). Employability through the curriculum. Tert. Educ. Manage. 8, 261–276. doi: 10.1080/13583883.2002.9967084
Kolmos, A. (2017). “PBL curriculum strategies,” in PBL in Engineering Education – International Perspectives on Curriculum Change, eds. A. Guerra, R. Ulseth, and A. Kolmos (Rotterdam: Sense Publishers), 1–12.
MacDonald, A. (2014). “Not For People Like Me?” Under-represented Groups in Science, Technology and Engineering: A Summary of the Evidence: The Facts, the Fiction and What We Should Do Next. Bradford: Wise Campaign.
Masterton, G. G. T., and Jeffrey, P. (2020). Integrating the liberal arts into the body of knowledge for civil engineering systems engineers. Civil Eng. Environm. Syst. 37, 234–243. doi: 10.1080/10286608.2020.1832086
McMasters, J. H. (2004). Influencing engineering education: one (aerospace) industry perspective. Int. J. Eng. Educ. 20, 353–371. Available online at: https://api.semanticscholar.org/CorpusID:59389148
Mejias, S., Thompson, N., Sedas, R. M., Rosin, M., Soep, E., Peppler, K., et al. (2021). The trouble with STEAM and why we use it anyway. Sci. Educ. 105:209–231. doi: 10.1002/sce.21605
Meletiou-Mavrotheris, M., Paparistodemou, E., Dick, L., Leavy, A., and Stylianou, E. (2022). Editorial: New and emerging technologies for STEAM teaching and learning. Front. Educ. 7:971287. doi: 10.3389/feduc.2022.971287
Miralles-Cardona, C., Kitta, I., and Cardona-Moltó, M.-C. (2023). Exploring pre-service STEM teachers' capacity to teach using a gender-responsive approach. Sustainability 15:11127. doi: 10.3390/su151411127
Mitchell, J. E., Nyamapfene, A., Roach, K., and Tilley, E. (2021). Faculty wide curriculum reform: the integrated engineering programme. Eur. J. Eng. Educ. 46, 48–66. doi: 10.1080/03043797.2019.1593324
Morgan, R., and Ion, S. (2014). The Universe of Engineering: A Call to Action. London: Royal Academy of Engineering.
Moseley, D., Elliott, J., Gregson, M., and Higgins, S. (2005). Thinking skills frameworks for use in education and training. J. Br. Educ. Res. 31, 367–390. doi: 10.1080/01411920500082219
Nagel, J. K., Pidaparti, R., Rose, C. S., Tafoya, E. M., Ponnusamy, P. G., Wahl, T. J., et al. (2019a). “Board 113: Evidence-based Resources that Scaffold Students in Performing Bio-inspired Design,” in Paper presented at 2019 ASEE Annual Conference & Exposition, Tampa, Florida.
Nagel, J. K., Rose, C. S., Pidaparti, R. M., Tafoya, E. M., Pittman, P. L., and Knaster, W. (2019b). “Preliminary findings from a comparative study of two bio-inspired design methods in a second-year engineering curriculum,” in Paper presented at 2019 ASEE Annual Conference & Exposition, Tampa, Florida.
Najdanovic-Visak, V. (2017). Team-based learning for first year engineering students. Educ. Chem. Eng. 18, 26–34. doi: 10.1016/j.ece.2016.09.001
National Academy of Engineering (2004). The Engineer of 2020: Visions of Engineering in the New Century. Washington, DC: National Academy of Engineering.
Niu, W., and Cheng, L. (2022). Editorial: Creativity and innovation in STEAM education. Front. Educ. 7:1045407. doi: 10.3389/feduc.2022.1045407
O'Connor, G. C., Ravichandran, T., and Robeson, D. (2008). Risk management through learning: management practices for radical innovation success. J. High Technol. Manage. Res. 19, 70–82. doi: 10.1016/j.hitech.2008.06.003
Paechter, M., and Maier, B. (2010). Online or face-to-face? Students' experiences and preferences in e-learning. Int. Higher Educ. 13, 292–297. doi: 10.1016/j.iheduc.2010.09.004
Perkins, J. (2013). Professor John Perkins' Review of Engineering Skills. London: UK Department of Business Innovation and Skills.
Roth, W.-M. (2014). “The social nature of representational engineering knowledge,” in The Cambridge Handbook of Engineering Education Research, A. Johri and B. M. Olds (New York: Cambridge University Press), 67–82.
Russell, J. S., and Stouffer, W. B. (2005). Survey of the national civil engineering curriculum. J. Prof. Issues Eng. Educ. Pract. 131, 118–128. doi: 10.1061/(ASCE)1052-3928(2005)131:2(118)
Sá, P., Lourenço, M., and Carlos, V. (2022). Sustainability competencies in higher education research: an analysis of doctoral theses in Portugal. Eur. J. Investig. Health Psychol. Educ. 12, 387–399. doi: 10.3390/ejihpe12040028
Salter, A., and Gann, D. (2003). Sources of ideas for innovation in engineering design. Research Policy. 32, 1309–1324. doi: 10.1016/S0048-7333(02)00119-1
Shapiro, N. S., and Levine, J. H. (1999). Creating Learning Communities: A Practical Guide to Winning Support, Organizing for Change, and Implementing Programs. San Francisco: John Wiley & Sons.
Shephard, K. (2023). Academic identity and “education for sustainable development”: a grounded theory. Front. Educ. 8:1257119. doi: 10.3389/feduc.2023.1257119
Siller, T., Rosales, A., Haines, J., and Benally, A. (2009). Development of undergraduate students' professional skills. J. Prof. Issues Eng. Educ. Pract. doi: 10.1061/(ASCE)1052-3928(2009)135:3(102)
Simpson, E., Bradley, D., and O'Keeffe, J. (2008). Failure is an option: an innovative engineering curriculum. Int. J. Build. Pathol. Adapt. 36, 268–282. doi: 10.1108/IJBPA-10-2017-0046
Spinks, N., Silburn, N., and Birchall, D. (2006). Educating Engineers for the 21st Century: The Industry View. London: The Royal Academy of Engineering.
Stouffer, W., and Russell, J. (2003). “Too liberal or not liberal enough: liberal arts, electives, and professional skills,” in Paper presented at 2003 Annual Conference. Nashville, Tennessee. doi: 10.18260/1-2–11814
Sun, Y., Ni, C.-C., and Lin, R. (2023). From stereotype to reality: A pilot study on the use of science, technology, engineering, and mathematics and STEAM in design education in Taiwan. Front. Educ. 8:1098584. doi: 10.3389/feduc.2023.1098584
Sunthonkanokpong, W. (2011). Future global visions of engineering education. Procedia Eng. 8, 160–164. doi: 10.1016/j.proeng.2011.03.029
Swartz, M., Leydens, J. A., Walter, J. D., and Johnson, K. (2019). “Is socio-technical thinking important in engineering education? Survey perceptions of male and female undergraduates,” in 2019 ASEE Annual Conference & Exposition. Tampa, Florida. Available online at: https://api.semanticscholar.org/CorpusID:208190167
Tannock, J. D. T., and Burge, S. E. (1994). The EPC model for quality assurance in higher education. Eur. J. Eng. Educ. 19, 263–274. doi: 10.1080/03043799408923293
The Royal Academy of Engineering (2007). Educating Engineers for the 21st Century. Available online at: https://www.raeng.org.uk/publications/reports/engineering-graduates-for-industryreport (accessed April 12, 2024).
Trevelyan, J., and Williams, B. (2019). Value creation in the engineering enterprise: an educational perspective. Eur. J. Eng. Educ. 44, 461–483. doi: 10.1080/03043797.2017.1421905
Videla, R., Aguayo, C., and Veloz, T. (2021). From STEM to STEAM: an enactive and ecological continuum. Front. Educ. 6:709560. doi: 10.3389/feduc.2021.709560
Wu, Y., Xu, L., and Philbin, S. P. (2023). Evaluating the role of the communication skills of engineering students on employability according to the outcome-based education (OBE) theory. Sustainability 15:9711. doi: 10.3390/su15129711
Wynn, T., and Harris, J. (2012). Toward a Stem 2b+ arts curriculum: creating the teacher team. Art Edu. 65, 42–47. doi: 10.1080/00043125.2012.11519191
Zaharim, A., Omar, M. Z., Yusoff, Y. M., Muhamad, N., Mohamed, A., and Mustapha, R. (2010). “Practical framework of employability skills for engineering graduate in Malaysia,” in Proc Education Engineering (EDUCON) 2010 IEEE (Piscataway, NJ), 921–927. Available online at: https://ieeexplore.ieee.org/stamp/stamp.jsp?tp=&arnumber=5492478
Keywords: engineering education, curriculum design, competences development, learning approaches, STEM and STEAM
Citation: Ferreira C, Gabriel B, Valente R and Figueiredo C (2024) Engineering education challenges and strengths: reflecting on key-stakeholder's perspectives. Front. Educ. 9:1297267. doi: 10.3389/feduc.2024.1297267
Received: 10 January 2024; Accepted: 29 May 2024;
Published: 12 June 2024.
Edited by:
Jian Zhang, University of Wisconsin Green Bay, United StatesReviewed by:
Cucuk Wawan Budiyanto, Sebelas Maret University, IndonesiaCopyright © 2024 Ferreira, Gabriel, Valente and Figueiredo. This is an open-access article distributed under the terms of the Creative Commons Attribution License (CC BY). The use, distribution or reproduction in other forums is permitted, provided the original author(s) and the copyright owner(s) are credited and that the original publication in this journal is cited, in accordance with accepted academic practice. No use, distribution or reproduction is permitted which does not comply with these terms.
*Correspondence: Cláudia Figueiredo, Y2xhdWRpYWZpZ3VlaXJlZG9AdWEucHQ=
Disclaimer: All claims expressed in this article are solely those of the authors and do not necessarily represent those of their affiliated organizations, or those of the publisher, the editors and the reviewers. Any product that may be evaluated in this article or claim that may be made by its manufacturer is not guaranteed or endorsed by the publisher.
Research integrity at Frontiers
Learn more about the work of our research integrity team to safeguard the quality of each article we publish.