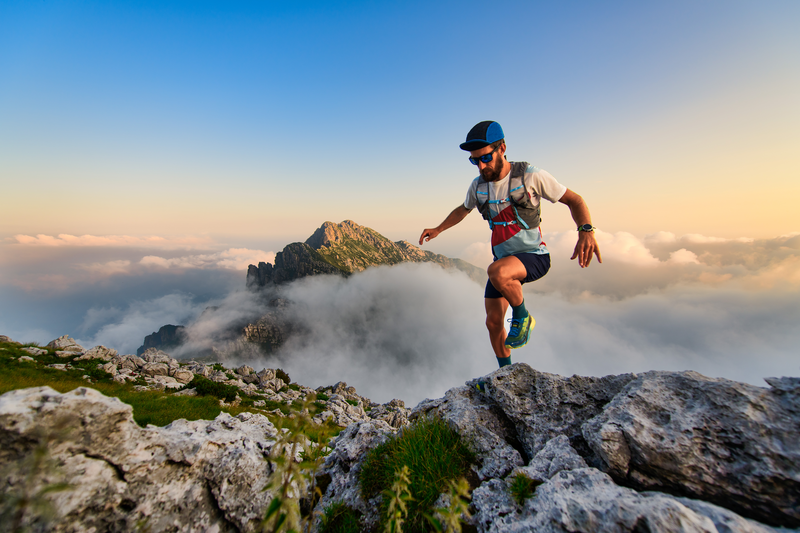
95% of researchers rate our articles as excellent or good
Learn more about the work of our research integrity team to safeguard the quality of each article we publish.
Find out more
ORIGINAL RESEARCH article
Front. Educ. , 13 February 2024
Sec. Digital Education
Volume 9 - 2024 | https://doi.org/10.3389/feduc.2024.1287615
This article is part of the Research Topic Impact and implications of AI methods and tools for the future of education View all 15 articles
We conducted an immersive virtual reality (IVR) intervention in an analytical biotechnology course to evaluate its effectiveness in promoting student learning and engagement. The objective was to assess the impact of an IVR tool on learning infrared (IR) spectroscopy and academic engagement. The IVR experience was integrated into the course sessions, which included theoretical framework, IR spectra exercises, an individual quiz, and competition games. Students were immersed in a first-person IVR experience simulating an analytical room, where they performed sample analysis and operated a virtual IR spectrometer. The quiz and survey assessed their understanding and perception of the IVR experience. When compared to control groups, IVR intervention group achieved higher scores on midterm exam questions related to IR spectroscopy and reported greater academic engagement according to the satisfaction survey. The findings highlighted the effectiveness of IVR in enhancing learning outcomes, curiosity, motivation, and engagement among students. Moreover, our research provides empirical evidence of IVR’s positive impact on student learning and engagement in the context of biotechnology engineering. Based on the study’s implications, educators in engineering fields should consider integrating IVR as an instructional tool, particularly for laboratory-related topics with limited equipment accessibility and overcrowded courses. Future research should explore the generalizability of these findings across different subjects and educational levels. Additionally, investigating the underlying factors influencing the effectiveness of IVR in promoting academic engagement would further enhance the understanding and implementation of this technology in educational settings.
Nowadays, the context of higher education demands educational strategies based on technological tools that facilitate the understanding of complex concepts, developing problem-solving skills and at the same time increasing the interest and motivation of students. Immersive learning is an emerging technology in the field of education, which allows the student to participate in the learning process by achieving meaningful and engaging interactions.
Virtual reality (VR) is implemented through two modalities based on the user experience. From one side, there is the modality based on the use of a desktop computer, in which the user has access to the content through a screen and interacts with it through the keyboard and mouse (Lee, 2014). The second modality, as described by Freina and Ott (2015), utilizes virtual reality headsets (VRH) as a form of immersive virtual reality (IVR) experience. This mode is characterized using a device that shows the user different views of a visual content as he turns his head. In addition, the user can complement his experience with handheld hearing aids and control devices.
Commonly, it is recognized that the use of VR in education has the advantages that it stimulates motivation to learn, improves attention to the object of study and allows experiential learning (Lei et al., 2018). In the case of education in the engineering field, the use of VR has allowed to simulate in real time the use of laboratory equipment that is difficult to access, it prevents students from causing damage to laboratory equipment, it solves the problem of lack of direct contact with laboratory equipment in overcrowded courses, reduces occupational risks and allows students to interact with complete processes (Vergara et al., 2017).
Structural chemistry is an important branch of chemistry focused on the study of the spatial arrangements of atoms and molecules, as well as the relationship between structure and properties (Zhou, 1993). Various IVR tools have been developed for learning chemistry, ranging from the visualization and manipulation of molecules in an immersive virtual environment to the simulation of practical experiences laboratories, either as organic chemistry lab (Tee et al., 2018; Camel et al., 2020; Williams et al., 2022), lab safety (Smith, 2016; Zhu et al., 2018; Unimersiv, 2019), molecular dynamics in enzyme catalysis (Bennie et al., 2019), and analytical instrumentation design (Naese et al., 2019). Regarding structural chemistry teaching, reports stand out because they test the visualization and manipulation of molecules in the immersive virtual environment, such as the construction of hydrocarbon molecules (Edwards et al., 2019), drug design (Norrby et al., 2015), the movement of molecules through carbon nanotubes (Ferrell et al., 2019), and the obtaining and interpretation of IR spectra to elucidate molecular structure (Dunnagan et al., 2020). In these studies, evidence is reported that the use of IVR generates interest, motivation, engagement, and greater learning.
Particularly, Dunnagan et al. (2020) developed and evaluated how an IVR tool could replace the use of equipment in an organic chemistry laboratory course. The authors designed a virtual immersive experience with a first-person perspective, comprised by the following stages:
1. An instructor greets the student, provides safety instructions, and offers an overview of the laboratory.
2. The student is taken to an analytical room where the IR spectrometer, a computer screen, four samples of unknown compounds and cleaning material are located. Here, the student receives the necessary explanations for obtaining the IR spectrum of one of the samples. Finally, the student carries out the routine of analyzing the sample and cleaning the virtual machine.
3. The student leaves the analytical room, and the IR spectrum of the unknown compound is presented on a blackboard. During the analysis, the student must select sections of the spectrum and identify the frequencies to determine the corresponding functional groups and finally identify the unknown compound.
To assess the experience, the authors divided the students into “treatment” and “control” groups. At the end of the practical activity, students from both groups completed identical questionnaires, and they took the same exam at the end of the semester. The comparison of responses indicated that, although the use of IVR is more memorable, there is no statistically significant difference between the two learning methods. However, the results also suggested that the students who had the IVR experience retained learning better than those who had the conventional experience.
In recent decades, researchers and educators have shown a growing interest in the concept of engagement as a way to avoid student boredom, improve student motivation, and participate in school-related activities (Baron and Corbin, 2012), and increase the levels of student performance (Phan and Ngu, 2014), where the intrinsic motivation for studies called “academic engagement” is an important element to take into account.
Engagement has been recognized as a complex term that encompasses various patterns of motivation, cognition, and behavior. It occurs when students invest themselves psychologically in their learning, striving to understand the materials presented to them and internalize them into their lives (Appleton et al., 2008). This characteristic has been widely recognized as an important influence on achievement and learning in higher education (Kahu, 2013) and can be employed as a significant predictor of academic performance (Upadyaya and Salmela-Aro, 2013; Lee, 2014). Furthermore, engagement has been described that it improves the participation and motivation of students in STEM courses (science, technology, engineering and mathematics) in general, and specifically in organic and structural chemistry (Rossi, 2015).
The course “Analytical Aspects in Bioprocesses” is a subject in the Biotechnology Engineering (IBT) major that provides students with a fundamental understanding of instrumental analysis. One of the topics covered in this course is infrared (IR) spectroscopy, which incorporates concepts of molecular structure, chemical bonding, functional groups, and electromagnetic radiation. Traditionally, learning content related to analytical chemistry is supported by practical activities in a laboratory. However, “Analytical Aspects in Bioprocesses” is a theoretical course that does not involve laboratory activities, making the subject more abstract for most students. To address this challenge, we proposed the use of IVR in the study of IR spectra interpretation to improve the learning experience and increase academic engagement, while solving the lack of costly instrumentation and delicate handling by students.
This study aims to evaluate the use of an IVR tool as part of an integral didactic sequence for learning IR spectra interpretation in the course “Analytical Aspects in Bioprocesses.” We assessed the students’ learning by means of an individual midterm exam and compared the scores with those obtained by students that did not use the IVR tool. Also, we evaluated students’ attitudes and opinions regarding the use of IVR to complement the learning of IR spectra interpretation.
The didactic sequence proposed in this study was carried out in the “Analytical Aspects in Bioprocesses” course during the February–June 2020 semester (FJ20), preceding the COVID-19 pandemic quarantine. This course is typically undertaken by IBT students in the eighth semester.
The didactic sequence, as depicted in Figure 1, unfolded through various steps. The initial session focused on the theoretical framework of IR, coupled with practical exercises using IR spectra in identifying organic compounds. In a second session, the students had the IVR experience proposed by Dunnagan et al. After the IVR experience, the students took an individual quiz, and the lecturer conducted a survey to collect the students’ attitudes and opinions about the IVR experience. In the third and last session, students were asked to make randomized teams and participate in a competitive team game activity. It is noteworthy that while the IVR activity was a central component, the didactic sequence was designed to be integrative, complementing Dunnagan’s IVR tool with additional pedagogical elements.
Figure 1. The didactic sequence carried out in this study included: explanation of the theoretical foundations of IR, resolution of IR spectra exercises (session 1), IVR experience based on the work of Dunnagan et al. (2020), individual quiz, satisfaction survey (session 2), and competitive team games (session 3). Created with BioRender.
The assessment tools used during the didactic sequence are described next. The individual quiz required students to associate an IR spectrum with five compounds identified by their chemical structure. The survey, administered anonymously via Google Forms, encompassed two sections. The first one collected general demographic data, such as gender, age, semester, minor, and origin of VR lenses. The second section comprised 12 questions assessing the student’s perception of the IVR experience, measured using a Likert scale ranging from 1 (strongly disagree) to 5 (strongly agree). This scale is a widely used measurement tool in educational research and is designed to gather information about individuals’ attitudes, opinions, and behaviors by presenting a series of statements and asking respondents to indicate their level of agreement or disagreement (Kusmaryono and Wijayanti, 2022). To maintain the validity of the questionnaire, we ensured that the statements were clear, unbiased and cover the entire range of possible responses. Lastly, during the competitive team activity, students were tasked with associating 10 IR spectra with their respective compounds, identifying them by both their chemical structure and generic name.
The students also took a midterm individual exam including a section with IR questions. The scores from these questions were retrieved to be compared with the corresponding ones in historical results from the January–May 2017 (JM17), 2018 (JM18), and 2019 (JM19) groups. It is important to clarify that the didactic sequence for JM17, JM18, and JM19 groups included only the activities described for sessions 1 and 3.
Quantitative data from the individual quiz, survey, team game, and the midterm individual exam were drawn and analyzed. Statistical analysis employed the use of difference in proportions for survey results and analysis of variance for comparing group and team performance in the game and the midterm individual exam, respectively. A significance level of p-value < 0.05 was considered, and data processing was carried out with Minitab (v19, Minitab, Inc., United States) and RStudio (v1.3.1056, RStudio, United States). In addition to collecting quantitative data, the lecturer conducted a qualitative observation of students’ mood during the IVR experience. This qualitative aspect, solely for descriptive purposes, was not quantified or subjected to formal analysis.
The FJ20 group, in which the IVR experience was implemented, consisted of 39 students, with 21 women (55.3%) and 17 men (44.7%) (Figure 2A). The students had a mean age of 22 years old (±0.9 years). In terms of minors (specialization area), 31 students (79.5%) were from Bioprocesses (BPR), and 8 students (20.5%) were from Molecular Biology (MOL). As depicted in Figure 2B, for the IVR experience, approximately half of the students borrowed VR headsets (54%), one-third of the group purchased them (31%), some students had prior experience with VR (10%), and a few students had the creativity to make their own headsets (5%).
The IVR experience took place in the classroom and lasted approximately 30 min. The students were relaxed and actively engaged during the session. At the end of the IVR experience, the students answered an individual quiz, obtaining an average score of 98.4% (±5.5%). This suggests a consistent understanding of the theoretical aspects covered both in class and with the IVR experience. Following the quiz, the students were asked to participate in a survey, and all students responded.
The students had a highly positive perception of the IVR experience, as indicated by the percentage of students who agreed or strongly agreed on the Likert scale (4 and 5, respectively). As shown in Figure 3, the students considered the experience to be immersive (84.6%), with learning activities that related to real-life situations (92.3%). They also reported enjoying the activities (89.7%) and expressed interest in repeating the experience to learn other analytical techniques (84.6%). The IVR experience was deemed helpful in understanding the theory effectively (79.5%), facilitating the completion of the individual quiz (76.9%), and most students (92.3%) would recommend IVR technology as a learning tool. Notably, in response to the question regarding recommendation of IVR technology, a higher proportion of women indicated agreement compared to men (p = 0.045). These results suggest that the IVR experience was very appealing and satisfying to the students, in addition to facilitating the understanding of the content.
The students of FJ20 and historic groups JM17, JM18, and JM19 played in teams in the association game of 10 IR spectra with 10 molecules. This occurred in their respective courses. As shown in Figure 4A, a significant difference was not found between the score of the group that experienced IVR (FJ20) and the results from previous academic periods. Regarding the FJ20 game performance, there was also no significant difference between the teams, and only 2 teams had less than 6 points (teams D4 and D9, Figure 4B). When comparing the IR questions score included in the midterm exam of the FJ20 group, by the teams organized during the game, we did not find any difference either.
Figure 4. (A) Score obtained in IR game of groups JM17, JM18, JM19, and FJ20. (B) Performance comparative by teams in IR game (blue bars) and questions scores (boxplots) included in midterm exam of group FJ20.
It is important to mention that teams with the highest scores in the game also demonstrated the highest scores in the IR questions of the midterm exam (teams D3 and D5, Figure 4B). Out of the 39 students, only 2 displayed poor performance in the IR questions of the midterm exam. Similar data could not be retrieved from previous periods. In this sense, our findings align with those reported by the developers of the IVR tool used (Dunnagan et al., 2020), who found no statistically significant difference between the use of IVR and conventional learning. However, we believe that by complementing the theoretical contents with the IVR experience, an otherwise arid topic became interesting and attractive to students. 4,175.
There are still a limited number of IVR resources available for teaching topics such as instrumental analysis. Particularly, Naese et al. (2019) used augmented reality technology to teach the design and operation of four analytical instruments: flame atomic absorption spectrometer, gas chromatograph−mass spectrometer, liquid chromatograph, and double-beam UV–vis spectrophotometer. It was noted that, although the participating students liked using augmented reality to learn more about the instruments used in class, they had mixed views when prompted to further comment on the activity. This was mainly because of difficulties with the app when accessing the displayed information when not in front of the instrument. Moreover, the main limitation of this specific augmented reality (AR) tool is that it cannot be deployed in other universities unless the exact equipment is available. However, it is possible that the same or a similar AR platform could be used to visualize the components and operation of other types of equipment, but this would likely require the creation of new content specific to the equipment in question.
Conversely, Dunnagan et al. tool was based on recorded sessions. The VR experience was designed to be an immersive experience that would be as effective as the being in the lab. It comprised both teaching students how to operate an IR spectrometer, and how to elucidate simple features of an IR spectrum. This was the experience we needed for the infrared spectroscopy lecture, particularly because the course was a theoretical one, and the lack of this equipment in our labs.
In a second study, Dunnagan reported that the undergraduate students who tried the IVR experience during the pandemic reported a high degree of satisfaction with the tool and no significant usability barrier (Dunnagan and Gallardo-Williams, 2020). Nonetheless, some of the students were not comfortable with the experience, given that they found their plans for a face-to-face lab experience disrupted by the unexpected quarantine situation. In contrast, we deployed the IVR tool weeks before the quarantine, and our results shows a highly appreciated learning experience, related to real-life situations. Also, our students showed a keen interest in repeating the experience to learn other analytical techniques, and most of them would recommend IVR technology as a learning tool. It is noteworthy that the students found creative ways to overcome the lack of VR headsets, which demonstrates their keen interest in participating in the IVR experience. The student’s positive perception to augmented and/or immersive virtual reality tools have been reported in numerous other topics. Students have benefited from the VR technology to aid the learning experience. Bennie et al. (2019) found that while learning about molecular dynamics in enzyme catalysis, undergraduate students found the VR platform enjoyable and easy to use. The authors did not attempt to quantify the direct learning gains of the participants, but students that experienced VR tool in enzyme catalysis obtained a higher median score than the ones who did not used it.
In light of our objectives, this study demonstrated significant positive outcomes. We evaluated the effectiveness of using an IVR experience as a support tool for learning an analytical technique. Although we did not observe a significant improvement in student learning compared to other courses, the results of the satisfaction survey indicated that the IVR experience was highly valued by the students, and it successfully enhanced their engagement. The findings also underscore the broader potential of IVR in reducing difficulties related to abstract course content, limited access to specialized equipment, and large class sizes.
While this study’s results highlight how IVR might improve student learning and engagement, certain limitations merit consideration. First off, the study’s applicability was restricted to a particular course, a specific analytical technique, and a particular instrument, so it’s unclear whether the findings can be applied in other educational settings or to another analytical methods. Second, the evaluation focused on the short term results of the IVR intervention, mostly by means of a satisfaction survey and midterm exam that were conducted soon after the IVR experience. Third, it would be interesting how individual learning styles, backgrounds, or past experiences with virtual reality technology can affect students’ reactions and affect how successful is the IVR tool.
Addressing the identified limitations opens possibilities for future research to enrich our understanding of IVR tools in educational settings. For example, exploring the tool’s efficacy in a variety of academic fields and classes would offer a more comprehensive view of its versatility and impact. Also, a few weeks or months following the IVR intervention follow-up evaluations may be carried out to see how long the advantages last and to provide guidance on how to incorporate IVR into curriculum. A study comprising more students in remote and in-person courses, from different campus, different majors, and institutions, would shed light on this matter. Also, the transferability of IVR strategy to the use of real equipment will solve training concerns for costly equipment.
In conclusion, the use of IVR proved to be an exceptional supportive feature for a course that lacked hands-on lab activities. The high level of engagement generated through the IVR experience suggests that this tool has the potential to be considered not only in traditional classroom settings but also in remote learning environments.
The raw data supporting the conclusions of this article will be made available by the authors, without undue reservation.
AS-L: Investigation, Methodology, Writing – original draft, Writing – review & editing. JJ-J: Investigation, Writing – original draft, Methodology, Writing – review & editing. NG-C: Writing – original draft. YP-A: Conceptualization, Data curation, Formal analysis, Funding acquisition, Investigation, Methodology, Resources, Supervision, Visualization, Writing – original draft, Writing – review & editing.
The author(s) declare that no financial support was received for the research, authorship, and/or publication of this article.
The authors would like to express their gratitude to the Department of Bioengineering at the Guadalajara campus for providing the necessary facilities to implement this project. In addition, the authors acknowledge the Emerging Food Technologies and Nutraceuticals group. The authors would like to acknowledge the financial support of Writing Lab, Institute for the Future of Education, Tecnologico de Monterrey, Mexico, in the production of this work.
The authors declare that the research was conducted in the absence of any commercial or financial relationships that could be construed as a potential conflict of interest.
All claims expressed in this article are solely those of the authors and do not necessarily represent those of their affiliated organizations, or those of the publisher, the editors and the reviewers. Any product that may be evaluated in this article, or claim that may be made by its manufacturer, is not guaranteed or endorsed by the publisher.
Appleton, J. J., Christenson, S. L., and Furlong, M. J. (2008). Student engagement with school: critical conceptual and methodological issues of the construct. Psychol. Sch. 45, 369–386. doi: 10.1002/pits.20303
Baron, P., and Corbin, L. (2012). Student engagement: rhetoric and reality. High. Educ. Res. Dev. 31, 759–772. doi: 10.1080/07294360.2012.655711
Bennie, S. J., Ranaghan, K. E., Deeks, H., Goldsmith, H. E., O’Connor, M. B., Mulholland, A. J., et al. (2019). Teaching enzyme catalysis using interactive molecular dynamics in virtual reality. J. Chem. Educ. 96, 2488–2496. doi: 10.1021/acs.jchemed.9b00181
Camel, V., Maillard, M.-N., Piard, J., Dumas, C., Cladière, M., Fitoussi, G., et al. (2020). CHIMACTIV: an open-access website for student-centered learning in analytical chemistry. J. Chem. Educ. 97, 2319–2326. doi: 10.1021/acs.jchemed.0c00023
Dunnagan, C. L., Dannenberg, D. A., Cuales, M. P., Earnest, A. D., Gurnsey, R. M., and Gallardo-Williams, M. T. (2020). Production and evaluation of a realistic immersive virtual reality organic chemistry laboratory experience: infrared spectroscopy. J. Chem. Educ. 97, 258–262. doi: 10.1021/acs.jchemed.9b00705
Dunnagan, C. L., and Gallardo-Williams, M. T. (2020). Overcoming physical separation during COVID-19 using virtual reality in organic chemistry laboratories. J. Chem. Educ. 97, 3060–3063. doi: 10.1021/acs.jchemed.0c00548
Edwards, B. I., Bielawski, K. S., Prada, R., and Cheok, A. D. (2019). Haptic virtual reality and immersive learning for enhanced organic chemistry instruction. Virtual Real. 23, 363–373. doi: 10.1007/s10055-018-0345-4
Ferrell, J. B., Campbell, J. P., McCarthy, D. R., McKay, K. T., Hensinger, M., Srinivasan, R., et al. (2019). Chemical exploration with virtual reality in organic teaching laboratories. J. Chem. Educ. 96, 1961–1966. doi: 10.1021/acs.jchemed.9b00036
Freina, L., and Ott, M. (2015). A literature review on immersive virtual reality in education: state of the art and perspectives. In Rethinking education by leveraging the eLearning pillar of the digital agenda for Europe (Carol I National Defence University Publishing House), 133–141.
Kahu, E. R. (2013). Framing student engagement in higher education. Stud. High. Educ. 38, 758–773. doi: 10.1080/03075079.2011.598505
Kusmaryono, I., and Wijayanti, D. (2022). Number of response options, reliability, validity, and potential Bias in the use of the Likert scale education and social science research: a literature review. Int. J. Educ. Methodol. 8, 625–637. doi: 10.12973/ijem.8.4.625
Lee, J.-S. (2014). The relationship between student engagement and academic performance: is it a myth or reality? J. Educ. Res. 107, 177–185. doi: 10.1080/00220671.2013.807491
Lei, X., Zhang, A., Wang, B., and Rau, P.-L. P. (2018). “Can virtual reality help children learn mathematics better? The application of VR headset in Children’s discipline education” in Cross-cultural design. Applications in cultural heritage, creativity and social development, Lecture notes in computer science. ed. P.-L. P. Rau (Cham: Springer International Publishing), 60–69.
Naese, J. A., McAteer, D., Hughes, K. D., Kelbon, C., Mugweru, A., and Grinias, J. P. (2019). Use of augmented reality in the instruction of analytical instrumentation design. J. Chem. Educ. 96, 593–596. doi: 10.1021/acs.jchemed.8b00794
Norrby, M., Grebner, C., Eriksson, J., and Boström, J. (2015). Molecular rift: virtual reality for drug designers. J. Chem. Inf. Model. 55, 2475–2484. doi: 10.1021/acs.jcim.5b00544
Phan, H. P., and Ngu, B. H. (2014). An empirical analysis of students’ learning and achievements: a motivational approach. Educ. J. 3:203. doi: 10.11648/j.edu.20140304.11
Rossi, R. D. (2015). ConfChem conference on flipped classroom: improving student engagement in organic chemistry using the inverted classroom model. J. Chem. Educ. 92, 1577–1579. doi: 10.1021/ed500899e
Smith, C. (2016). Chemistry lab VR. Dev. Dent. Available at: https://devpost.com/software/chemistry-lab-vr (Accessed June 24, 2023).
Tee, N. Y. K., Gan, H. S., Li, J., Cheong, B. H.-P., Tan, H. Y., Liew, O. W., et al. (2018). Developing and demonstrating an augmented reality colorimetric titration tool. J. Chem. Educ. 95, 393–399. doi: 10.1021/acs.jchemed.7b00618
Unimersiv (2019). Chemistry VR. Unimersiv. Available at: https://unimersiv.com/review/chemistry-vr/ (Accessed June 25, 2023).
Upadyaya, K., and Salmela-Aro, K. (2013). Development of school engagement in association with academic success and well-being in varying social contexts. Eur. Psychol. 18, 136–147. doi: 10.1027/1016-9040/a000143
Vergara, D., Rubio, M. P., and Lorenzo, M. (2017). On the design of virtual reality learning environments in engineering. Multimodal Technol. Interact. 1:11. doi: 10.3390/mti1020011
Williams, N. D., Gallardo-Williams, M. T., Griffith, E. H., and Bretz, S. L. (2022). Investigating meaningful learning in virtual reality organic chemistry laboratories. J. Chem. Educ. 99, 1100–1105. doi: 10.1021/acs.jchemed.1c00476
Keywords: immersive virtual reality, academic engagement, experiential learning, infrared spectroscopy, biotechnology engineering, educational innovation, higher education, professional education
Citation: Sánchez-López AL, Jáuregui-Jáuregui JA, García-Carrera NA and Perfecto-Avalos Y (2024) Evaluating effectiveness of immersive virtual reality in promoting students’ learning and engagement: a case study of analytical biotechnology engineering course. Front. Educ. 9:1287615. doi: 10.3389/feduc.2024.1287615
Received: 02 September 2023; Accepted: 25 January 2024;
Published: 13 February 2024.
Edited by:
Julius Nganji, University of Toronto, CanadaReviewed by:
Mauro Mocerino, Curtin University, AustraliaCopyright © 2024 Sánchez-López, Jáuregui-Jáuregui, García-Carrera and Perfecto-Avalos. This is an open-access article distributed under the terms of the Creative Commons Attribution License (CC BY). The use, distribution or reproduction in other forums is permitted, provided the original author(s) and the copyright owner(s) are credited and that the original publication in this journal is cited, in accordance with accepted academic practice. No use, distribution or reproduction is permitted which does not comply with these terms.
*Correspondence: Yocanxóchitl Perfecto-Avalos, eW9jYW5AdGVjLm14
Disclaimer: All claims expressed in this article are solely those of the authors and do not necessarily represent those of their affiliated organizations, or those of the publisher, the editors and the reviewers. Any product that may be evaluated in this article or claim that may be made by its manufacturer is not guaranteed or endorsed by the publisher.
Research integrity at Frontiers
Learn more about the work of our research integrity team to safeguard the quality of each article we publish.