- 1Discipline of Physiology, School of Medicine, Trinity College Dublin, Dublin, Ireland
- 2Science & Society Research Group, School of Education, Trinity College Dublin, Dublin, Ireland
Introduction: The rapid pace of technological advancement, globalisation, and complex socio-economic challenges facing 21st-century society necessitates a rethinking of undergraduate science education. Undergraduate science curriculum reform is essential to prepare students for the demands of the modern workforce in an ever-changing world. Accordingly, in Trinity College Dublin (the University of Dublin), the oldest science degree course in Ireland was intensively reviewed and redeveloped between 2014 and 2021. This study aims to collate and disseminate the knowledge acquired by university staff through the experience of undertaking a major science curriculum redevelopment.
Methods: Nine senior staff members closely involved with the redevelopment were interviewed about why the curriculum reform was necessary, what it had achieved, and how the process could have been improved.
Results: The reasons behind the curriculum changes are described and placed in the context of contemporary pedagogical research. Reflections from the academic and administrative staff involved in the redevelopment process are presented, emphasising the challenges and opportunities that emerged from that process.
Discussion: Recommendations for other universities undertaking similar reforms are included. Aligning undergraduate science curriculum reform with the needs of 21st-century society is vital for ensuring that science graduates are well-prepared to make positive contributions to a sustainable future.
1 Introduction
Curriculum reform in higher education is not only an inherently challenging process, but is considered a “contested space” across global contexts (Shay, 2015, p. 431). “Profound reform” is difficult to undertake and it is “seldom investigated in empirical research” (Chen et al., 2009, p. 161). Consequently, while the reform of undergraduate curricula is central to “defining research-based higher education for future generations” (Annala et al., 2021, p. 1), it remains a challenging process to implement (Keesing-Styles et al., 2014; Velthuis et al., 2018; Simon et al., 2019) and adequate post-reform evaluation is rare (McEwen et al., 2009; Hicks, 2018; Kandiko Howson and Kingsbury, 2021). The reasons for undergraduate curricula reform are varied, such as external pressure to align courses with advances in evidence-based pedagogical research (Patel et al., 2009; Wood, 2009; Singer and Smith, 2013), or due to internal pressure within the university, such as changing student numbers (McKay et al., 2005), new facilities (Campbell et al., 2022), or feedback from course reviewers (Simon et al., 2019).
When curriculum reform does happen in undergraduate degree programmes, it is generally as a reaction to meet the changing needs of society and as an attempt to develop key skills for the workplace. Recent examples include developing project-based learning skills to improve collaboration, communication, and problem-solving in biology graduates (Hart, 2019; St. Louis et al., 2021); embedding open science (Jekel et al., 2020) and active learning skills (Weir et al., 2019); investigating laboratory interactions (Wei et al., 2019) and primary literature (Rawlings, 2019); and decolonising curricula (McGregor and Park, 2019; Uleanya et al., 2023). There have long been calls for undergraduate science curriculum reform to integrate teaching on the nature of science, a topic not commonly considered in undergraduate science curricula, due to a perceived discomfort in asking philosophical questions about science, especially when they lack consensus answers (Hand, 1999a,b). Hand argues that “All those involved in science, particularly those involved in university science education,” should “adopt a more critical approach to science education that includes an articulation of hidden assumptions and consideration of the philosophical and ethical issues raised by science” (Hand, 1999b, p. 501). This aligned with curriculum reform that had occurred in the Irish school system (Varley et al., 2013; van Kampen, 2022) which has seen the introduction of a more specific focus on inquiry-based learning at primary level (Murphy et al., 2012; Dunne et al., 2013), and the nature of science at post-primary level (Erduran and Dagher, 2014).
This paper describes the most significant curriculum reform of the undergraduate science degree programme in Trinity College Dublin, the longest-running science degree programme in Ireland’s oldest university.
1.1 The need for 21st century undergraduate science curricula
The need for the undergraduate science degree at Trinity College Dublin to undergo curriculum reform in 2018 mirrored common needs for change in higher education institutions, such as changing graduate employability and skills development, evolving teaching–research relationships, changes to teaching and learning due to educational technologies (Roberts, 2015), and the need for a 21st-century university to adapt to current trends and demands of interdisciplinarity (Millar, 2016).
A degree course in natural sciences has been offered at Trinity College Dublin since 1851 (Trinity College Dublin, 2023), and the modern format of the undergraduate science course, established in the 1970s, experienced no major changes to its overall structure for decades. Prior to the curriculum reform in 2018, Trinity’s undergraduate science degree, TR071, was a popular common-entry degree programme with an annual intake of more than 300 students. All TR071 students spent 2 years studying a choice of fundamental science modules from across several disciplines, before specialising into one of sixteen moderatorship options, which they would study for a further 2 years. After a total of 4 years, the students graduated with a B.A. (Mod.) in Natural Sciences. Five direct-entry science moderatorships existed alongside TR071, each with a small (5–30 students) but highly competitive intake. These students shared many modules with TR071 students but did not have an option to change their predetermined moderatorship.
Feedback from staff and students in the years leading up to the curriculum reform highlighted some issues that necessitated change. Staff observed that the course lacked transparency regarding the flexibility of moderatorship choice. The students’ choice of modules upon commencing the course restricted the moderatorship options that were available to them in their third and fourth years. Thus, although the course was supposed to be a common-entry general science course, students were effectively streamed from the outset and could not study all branches of science. This unofficial, unacknowledged streaming meant that the course did not offer as much freedom of choice as it seemed to. It was considered difficult to teach classes when different cohorts had significantly different prior experiences and different intentions (e.g., students with a solid grounding in advanced maths vs. those without; students who got their first moderatorship preference vs. those who did not). Similarly, it was difficult to have coherent programme-led design when the students in a given module had not all done the same preparatory modules. Other perceived issues with the science degree included that some staff felt that the direct-entry courses had been arbitrarily created for historical reasons pertaining to available funding at the time, rather than having a grounded academic rationale for them to be separate entities, while some students felt that there was some duplication of content across modules and they sometimes had to undertake intensive modules in subjects they felt they did not require to such depth. Even without these issues, the evolving nature of science education alone would have eventually required the undergraduate science programme to be updated and streamlined.
The undergraduate science curriculum reform began at Trinity College in 2014, when a routine external review of the TR071 science course praised aspects of the programme but highlighted areas that needed to be updated. Notably, the reviewers recommended introducing streaming to improve alignment between student intake and moderatorship choices; to review teaching methods and rationalise course content; and to restructure the course management. This coincided with the implementation of the Trinity Education Project – a university-wide endeavour to renew all undergraduate curricula to suit the changing needs of the 21st century (Prendergast and Morash, 2017). The Trinity Education Project followed the calls for undergraduate curricula to be informed by advances in evidence-based research (Wood, 2009; Hicks, 2018) and for discipline-based teaching to feature an emphasis on active, problem-based learning (Patel et al., 2009; Singer and Smith, 2013). Such shifts in higher education teaching have been implemented across different fields (McEwen et al., 2009; Simon et al., 2019) and are especially relevant in undergraduate science education where more “soft” or transferable skills (Prendergast and Morash, 2017; Gladys et al., 2022) are needed, given that many science students pursue careers outside of science (Fischer, 2011).
The Trinity Education Project reviewed and updated the undergraduate curriculum throughout the university around four graduate attributes: the abilities to think independently, to communicate effectively, to act responsibly, and to develop continuously (Trinity College Dublin, 2016; Prendergast and Morash, 2017). It then designed curriculum principles to support the development of those attributes and simplified and streamlined the structures of the programmes offered. Accordingly, a comprehensive redevelopment of the undergraduate science programme was initiated both to implement the recommendations of the external review and to align the programme with the new university-wide curriculum strategy. The key aims of the reform were to streamline entry routes, to make course management more effective, and to increase emphasis on transferable skills, critical thinking, and active learning (Mitchell, 2017).
2 Methods
To understand the changes that were implemented during the curriculum reform and the rationale behind those changes, relevant policy texts and institutional documents were analysed. The TR071 Programme Review self-assessment report (2014) and external reviewers’ report (Busby et al., 2014) were important primary sources for the observations and decisions that led to the programme redevelopment. Minutes from the meetings of the Undergraduate Science Education Working Group (the committee that managed the redevelopment) established the timeline and dynamics of the decision-making process. Prospectuses and course handbooks described the old and new programme structures.
The main data collection method for this study was a series of interviews with academic and administrative staff, following the approach of previous studies of higher education curriculum reform, such as Roberts (2015). The aim of the interviews was to collate the experiential wisdom accumulated by university staff during a major programme redevelopment and to better understand the “academic agency involved in curriculum change in higher education” (Annala et al., 2021, p. 1).
Given the small number of individuals involved in the process, piloting the interview questions with a subgroup of participants was not possible and instead the questions were tested and validated within the research team by constructing an inquiry-based conversation and gathering feedback on alignment with the research aims (Castillo-Montoya, 2016). Purposeful sampling (Suri, 2011) was used to select nine staff members who played important roles in the reform process. By capturing their views on the new programme and the reform process, a qualitative evaluation of the programme redevelopment from the instructors’ perspective can be presented, similarly to Velthuis et al. (2018). Of the nine staff members interviewed, seven were academic staff members and two were administrative staff. All were closely involved with the programme review and/or curriculum reform and included the Faculty Dean, Science Course Director, Associate Dean of Undergraduate Science Education, and the Science Course Administrator.
Interviewees were invited to participate on a voluntary basis and all those who were invited agreed to participate. The interviews took place between April and August 2022. Each interview was conducted separately and privately and lasted between 40 and 90 min. Interviews were conducted on Microsoft Teams unless the participant expressed a preference for a face-to-face interview, in which case the participant chose the venue. Each interview was recorded (audio and/or video), unless the participant expressed a preference not to be recorded. The main interview questions were sent to the participants approximately a week in advance to give them time to think about their answers, and each interviewee received a copy of their transcript afterwards and had the opportunity to amend the transcript if desired. Confidentiality and anonymity were assured for all participants. Ethical approval was received from the Faculty of STEM and the School of Education in Trinity College Dublin.
Semi-structured interviews (Husband, 2020) were carried out based around the following questions:
1. Briefly explain your role in the review of the science programme in 2014, the decision to redevelop the programme, and/or in the design and implementation of the new programme.
2. What were the aspects of the old programme that you thought most needed to be updated?
3. What aspects of the new programme do you think will be most beneficial (to students or staff)?
4. What aspects of the science degree programme in Trinity still need to be improved, in your opinion?
5. How could the review process have been made better?
6. What advice would you give to your former self if you had to do it all over again?
These broad questions were chosen to give the participants the chance to mention whatever points mattered most to them. The interviewer also posed follow-up questions as topics arose, to encourage interviewees to elaborate on their answers. In some instances, the interviewer prompted the participant to give their opinion on specific matters such as the new modules, the changes to course management and the impact of the pandemic, while avoiding asking leading questions. The interviewer was not known to any of the participants and had not been involved in the redevelopment, to ensure the interviewees could speak freely.
3 Results
The principal outcomes of the curriculum reform were the merging of the small direct-entry courses with the common-entry science course, the division of the latter into four distinct entry routes or “streams” based on the major scientific disciplines, and the introduction of new modules in the ethics and philosophy of science, science communication, and statistics and computation for biologists and geoscientists.
3.1 Structural changes
The two most significant structural changes made to the science programme involved redesigning the entry routes. Figure 1 compares the structure of the science programme before and after the redesign. The first major change was to “fold in” the five direct-entry courses (Human Genetics; Chemistry with Molecular Modelling; Medicinal Chemistry; Nanoscience, Physics and Chemistry of Advanced Materials; Earth Sciences), converting them to moderatorships within the common-entry science programme. Before the redevelopment, students in the direct-entry courses had no option to change their mind and switch to a different science moderatorship, even though in the first and second year almost all their modules were shared with the common-entry science course, TR071. Likewise, students in TR071 had no option to switch to one of the direct-entry courses. The second major change was to divide the common-entry science course into four entry “streams” (Physical Sciences; Chemical Sciences; Biological and Biomedical Sciences; Geography and Geoscience), each of which is essentially a separate course because there is no possibility of transfer between streams under normal circumstances. Each entry route leads to several moderatorship options. Students choose their preferred moderatorship at the end of second year. When the number of applications to a given moderatorship exceeds the quota, places are allocated based on performance in the second-year examinations. Most moderatorships may only be accessed through a specific stream, but Nanoscience may be accessed through either the Physical Sciences or Chemical Sciences stream. One consequence of streaming is that every science student is now guaranteed to be able to pursue a moderatorship within their broad field of interest. For instance, each student in the Biological and Biomedical Sciences stream is guaranteed a place in a biology moderatorship. This guarantee was formerly impossible because there was no way to control student intake to match moderatorship capacity. Another consequence of streaming is that modules for first-and second-year students can be customised for specific streams.
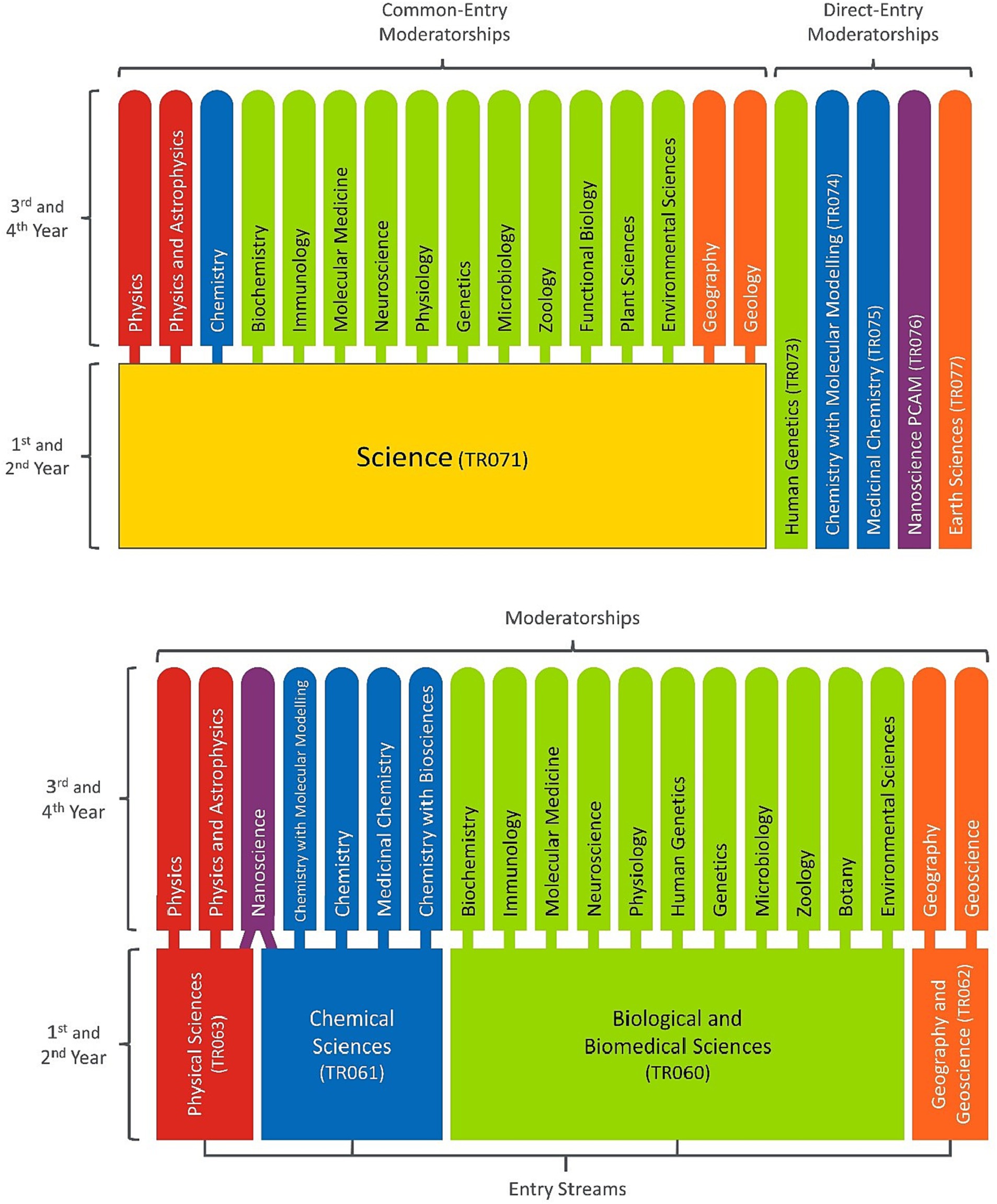
Figure 1. Trinity College undergraduate science: programme structure before (top) and after (bottom) redevelopment.
3.2 Curriculum changes
The entire science curriculum was reviewed and, where appropriate, refreshed to match the university’s five new curriculum principles: to provide structured but flexible pathways to support the development of the graduate attributes, to be programme-focused, to be research-centred, to employ a range of teaching, learning and assessment strategies, and to use appropriate technology-enhanced approaches (Trinity College Dublin, 2016). Consequently, the new curriculum places greater emphasis on stimulating active learning through use of blended learning resources and more formative assessment. Critical thinking and transferable skills are likewise emphasised, in the understanding that “the goal of science education in Trinity is not just to train the next generation of scientists, but also those who will work in other careers enabled by and impacted by advances in scientific knowledge [..] not just in existing industries, but in new ones that we cannot even yet imagine” (Mitchell, 2017).
Concomitantly with the undergraduate science curriculum reform, a flexible programme architecture was introduced across the university, encompassing a balance of mandatory, discipline-specific (“Core”) modules, optional (“Open”) modules from adjacent disciplines, and extradisciplinary (“Elective”) modules. This framework aimed to promote breadth of learning without sacrificing depth. It governed the shape of the revised science curriculum, placing added emphasis on interdisciplinarity. This addressed the need for greater interdisciplinarity in science education, both because disciplines increasingly overlap and because interdisciplinarity stimulates reasoning and conceptual understanding (Gouvea et al., 2013). The course content was rationalised so that it fitted together more logically, and the progression of topics from year to year, in terms of vertical integration (Oliver et al., 2008), was refined. Duplication of content across modules was eliminated and it was ensured that the different modules within each year of study complemented one another (horizontal integration). The existing focus on research-led teaching was maintained, as was the inclusion of a mandatory final-year research project, which was deemed an essential feature of the programme.
The most substantial curriculum change was the introduction of new modules. One such module, The History, Philosophy and Ethics of Science (5 ECTS), was made mandatory for every second-year science student. This module gives an overview of the philosophy of science (induction, scientific realism and anti-realism, perspectivism, etc.) and teaches the student how to critically assess scientific theories. It also develops the skills needed to navigate the ethical issues inherent to scientific research. This directly addresses the call by Hand (1999a) for undergraduate science curricula to include philosophy, and the subsequent benefits that can provide (Laplane et al., 2019; Fjelland, 2022; Lusk, 2022).
Another new module, Science Communication (10 ECTS), was introduced as an optional module for first-year students in the Biological and Biomedical Sciences stream. This module explores science communication as a field of research and practice and how it affects the relationship between science and society. It develops skills in communication, presentation, and critical thinking. Students gain an understanding of how science is taught and communicated, an awareness of how research policy and public perception are crafted, and a familiarity with the challenges arising at the interface of science and society. It draws together national developments, such as the growth of citizen science (Roche et al., 2021b), with international developments in the field of science communication (Roche et al., 2021a). While once considered an “emerging discipline” (Trench and Bucchi, 2010, p. 1), science communication is increasingly important in science education (Mercer-Mapstone and Kuchel, 2017) and guidance can now be found on how best to teach science communication across disciplines (Lewenstein and Baram-Tsabari, 2022). The module content was designed around the idea that science education that responsibly incorporates citizen science and science communication can help tackle grand societal challenges and contribute to the Sustainable Development Goals (SDGs) of the United Nations (Dillon et al., 2016; Fritz et al., 2019; Fraisl et al., 2020). The value of interdisciplinary knowledge and understanding is also core to modern science education as it underpins sustainable societal progress and the need to make scientific research more inclusive and equitable (Massarani and Merzagora, 2014; Medin and Bang, 2014; Roche et al., 2023).
A new mandatory mathematics module, Mathematics, Statistics and Computation (10 ECTS), was created for first-year students in the Biological and Biomedical Sciences and Geography and Geoscience streams. In addition to components in calculus and discrete mathematics, this module includes a statistics component that prepares students for handling data, a crucial skill for a modern biologist or geoscientist. Students learn how to extract information from a data set and make inferences about a population using ideas of sampling distributions, confidence intervals and hypothesis testing, and how to perform basic tasks using the statistical software, R. Modern biology and geoscience are highly quantitative disciplines (Manduca et al., 2008; Cummings and Temple, 2010; Hoffman et al., 2016). Nonetheless, students often perceive them as purely qualitative subjects (Manduca et al., 2008; Hester et al., 2014), and the development of numeracy often lags behind other core skills (Cummings and Temple, 2010; Hoffman et al., 2016). Studies have shown that even when instructed in pure mathematics, students generally lack the ability to apply quantitative reasoning to their own discipline, but infusing applied numerical problems to biology and geoscience curricula improves students’ contextual quantitative literacy (Manduca et al., 2008; Speth et al., 2010; Hester et al., 2014).
Finally, for the Biological and Biomedical Sciences stream, two Chemistry for Biologists modules were introduced: 10 ECTS (mandatory) in first year and 5 ECTS (optional) in second year. These foundational modules addressed the issue that biology students in the former science course had studied chemistry to a much greater depth than was necessary for their moderatorships, which had been a significant source of stress for many students. The new curriculum was designed to focus on the aspects of chemistry that have practical relevance to the life sciences.
3.3 Management changes
The governance structure of the science programme was changed to make it more efficient. Previously, a Science Course Management Committee managed the academic structure, curriculum, and regulations of the science programme. The committee membership included the Directors of Teaching and Learning of each of the science schools. The external reviewers observed that curriculum design tended to prioritise the needs of the individual schools as research institutes, rather than the needs of the course as a whole, and that decision-making was “commendably democratic, but tortuous in practice” (Busby et al., 2014, p. 12) because each school had power of veto. Now, each stream has a Course Committee that is independent of the schools. Teaching remains research-led, but pedagogical concerns can be dealt with more decisively and purposefully. A Science Programme Management Committee manages all issues of common interest across the four streams. Furthermore, the role of Associate Dean of Undergraduate Science Education was created to replace the previous role of Science Course Director. The new role has considerably more authority to implement changes to the science programme. Figure 2 summarises the changes made by the programme redevelopment.
4 Discussion
4.1 Benefits of the new science curriculum
The staff members interviewed were largely satisfied with the changes that were made to the science programme. When the interviewees were asked what aspects of the new science programme were most beneficial, the most prevalent response was that the curriculum design is now more rational. There is a more integrative framework: modules within a stream are more clearly connected from year to year (vertical integration), and they fit together better within a given year (horizontal integration). Students are better encouraged to consider underlying concepts and principles.
Other popular responses were that the introduction of streaming enabled the creation of foundation modules for specific streams, notably foundational chemistry for the biology stream, and that there is better transparency regarding progression through the course because students understand how their module choices impact their moderatorship options. When asked about the new History, Philosophy and Ethics of Science module, most of the interviewees were strongly in favour of it, describing it as an important tool for stimulating critical thinking and teaching students to be responsible scientists, although some of the interviewees had no strong opinion on the module and suggested that it should have been made optional. However, the supporters of the module pointed out that students do not always appreciate the value of the module at the time but will realise the benefits of it later in their careers. Some mentioned that it is vital for such a module to focus on the philosophy of science, rather than being a general philosophy course (c.f., de Regt and Koster, 2021); they said that some work was still required to optimise the curriculum. Staff members closely associated with the Biological and Biomedical Sciences stream were very pleased by the new Maths/Statistics/Computation module because, unlike the previous mathematics modules, it teaches students how to handle large data, which is an increasingly important skill both in the academy and in industry.
Other benefits of the new programme mentioned by interviewees included that streamed entry means that student cohorts (at least in the physics, chemistry, and geoscience streams, which have smaller intakes than the biology stream) have a class identity from the outset. Some first-year classes are much smaller, facilitating more interaction between students and lecturers, while levels of interest among students seem higher because they are focused on their area of interest. Students have more opportunities to study subjects outside their core discipline and outside of science. The more logical structure facilitates adding new elements such as the Chemistry with Biosciences moderatorship and the Science Communication module which, in turn, ensure that transferable skills are better reflected in the programme. Interviewees also felt that the line of responsibility for administrative decision-making is clearer.
4.2 Streaming and moderatorships
All those interviewed were in favour of the introduction of streaming as a way to ensure that every incoming student is guaranteed to be able to do a moderatorship within their broad discipline of choice. However, one interviewee argued that each module in first and second year should be identical for any participant. For example, the first-year introductory chemistry module should be shared by students from all streams, rather than there being a separate, less intensive chemistry module for students in the Biological Sciences stream. Thus, students would be able to transfer to a different stream if they wished to, because they would have the required prerequisites. This system would retain all the flexibility of the old TR071 programme. However, it would preclude the possibility of tailoring modules to a specific stream or streams – which, as stated above, most interviewees considered to be a major benefit of the streaming that was introduced. As several interviewees pointed out, it is impossible to have both maximal choice and optimal specialisation; one has to find a balance between the two, a trade-off between breadth and depth. Most interviewees thought that a good compromise had been found. One said, “If you ask someone, “do you want more choice or less choice?,” human beings are always going to say, “more choice,” but too much choice is a bad thing. There’s an element of abdicating your responsibility as an educator, if you just say to a student, “pick what you want,” because it makes them happy. It would be the same as me letting my children eat as many sweets as they want. It’s my job as an educator to design a programme that they might not love all bits of.” The consensus was that the benefit of having improved programme-led design within each stream outweighed the reduced ability to transfer between subject areas.
Two interviewees proposed that the Nanoscience moderatorship should revert to being a direct-entry course. They said that the former direct-entry Nanoscience course attracted an exceptionally high calibre of student because there was intense competition for entry places, while, under the new structures, the Nanoscience moderatorship is no longer guaranteed to fill its quota. However, other interviewees argued that Nanoscience should remain within the common-entry structure because there is no pedagogical reason for it to be a separate entity. Furthermore, one interviewee said that it was important that any new science moderatorships developed in the future should likewise be accessed through the common-entry science streams, to ensure that the benefits of the newly streamlined governance structure are not lost. Another argued that the former popularity of the Nanoscience course could be an artefact of the entry mechanism: there were a small number of places in the course, which meant that the threshold for entry was very high. Therefore, the course was perceived as prestigious, which led to high demand for entry.
4.3 Opportunities for improvement
Interviewees had varied opinions on what further improvements the science programme requires. When the interviewees were asked what aspects of the science programme still need to be improved, their responses varied greatly. No specific major issue was outstanding with regards to the content or structure of the programme.
Several interviewees mentioned the “flipped classroom” model of teaching (Al-Samarraie et al., 2020) as a possibility that should be explored. In this approach, content that would previously have been delivered in large lectures is delivered online, allowing time for other forms of engagement with students in person, such as question-and-answer sessions, problem-solving workshops, and nuanced discussions. The interviewees said that their experience of teaching during the COVID-19 pandemic had shown them that the flipped classroom model can improve the students’ learning experience.
There was consensus among all interviewees that the different schools in the faculty that contribute to the science course should not view themselves as competing for students, because this perception can lead to small-minded decision making. However, they said that due to the funding model within the university, whereby schools are allocated resources based on their FTSEs (the amount of course credits or “full-time student equivalents” earned by each student in their school), such competition is perhaps inevitable. They stressed that the students’ best interests were always the highest priority, regardless of this problem. They accepted that the funding model was an issue that is beyond the scope of science curriculum reform.
While the interviewees praised the new governance structure of the science programme, including the increased independence of course management from the contributing science schools, which are research bodies, they mentioned that communication pathways within the university need to be adjusted to reflect this new structure. Some interviewees saw this specific internal communication issue as symptomatic of a wider problem wherein pedagogical concerns in universities can often be undervalued or overlooked in favour of research concerns.
Other possible improvements to the new science programme that were suggested included establishing a system whereby students could study abroad for a semester without incurring any risk to their ultimate graduation in the moderatorship of their choice, having more support for teaching assistants, and ensuring financial support is available so that all students are guaranteed to have adequate access to IT infrastructure.
4.4 Curriculum reform timeline
Sharing curriculum reform processes can be of clear value to higher education institutions, yet it is often the case that “no aggregated data is available […] on the structures, frequency, durations, processes, participants, and outcomes of deliberations on curriculum at the programme level” (Hicks, 2018, p. 17). To help other institutions considering curriculum reform, the reform process at Trinity College Dublin is outlined below.
In 2015, the Dean of the Faculty of STEM formed a Working Group to discuss and manage the redevelopment of the science programme. The group members were chosen by the Dean for their openness to change and their interest in revitalising the course, rather than purely according to seniority. All had significant experience in delivery of the programme. In addition to academic staff, the Working Group included the Science Course Administrator, the manager of the Trinity Education Project, a faculty administrator, and a Student Union representative. The Working Group aimed to redesign the course to promote the best student outcomes and student experience, purposely without considering constraints of available resources or facilities. Thus, the academic imperative was the overriding principle of the committee’s deliberations. The group met regularly (typically fortnightly) from January 2016 until the implementation of the revised programme in 2018. Having first discussed the new curriculum principles, the Working Group established the broad structure of the new programme, such as streamed entries and the implementation of the programme architecture described above. Sub-committees were established for each of the four new “streams” to liaise with the moderatorship stakeholders, schools, and disciplines. The Associate Dean of Undergraduate Science Education liaised regularly with the existing management structures and heads of schools and met with students to get feedback.
By the end of 2016, the new programme structure was finalised, and in early 2017, the stream sub-committees began reviewing their respective curricula. Further sub-committees were established to design modules that needed input from multiple schools. The national university admissions office was informed of the changes and marketing began for the restructured programme with a launch event in November 2017 featuring Nobel laureate and alumnus of the Trinity science degree William Campbell. Intake of first-year students to the new science programme commenced in September 2018. Meanwhile, second-, third-and fourth-year students continued in the old science programme. The old and new programmes were run in parallel until the final cohort of students from the old programme graduated in 2021. Throughout the academic year 2018/19, the curriculum and timetabling for the following year’s second-year students was finalised; in 2019/20, the curriculum and timetabling for the rising third-year students was finalised, and so on. Thus, the new science programme remained a work in progress until 2021. The first cohort of students graduated in 2022.
4.5 Challenges for curriculum reform
Most of the interviewees thought that the review and redevelopment of the science programme had been a successful process: “there were too many moving parts for [reorganisation of the programme] to be done from the bottom up. Some top-down governance decisions needed to be taken. […] The gridlock of shared overlapping modules [had] made it impossible to change anything because everything was interlocking. We needed a global overhaul.”
Varied suggestions were put forward as to how the reform process could have been improved. Firstly, all those interviewed who had been involved in the preparation for the external review of the science course in 2014 agreed that they had received insufficient guidance. Over a period of 7 months, they had to compile a comprehensive self-assessment report on the science programme, while overseeing the day-to-day running of the programme, without any clear indication of the expected structure or content of the report or a clear sense of the purpose of the review. Furthermore, the staff members responsible for creating the report were not given access to pertinent historical student data, e.g., pass/fail ratios, percentage of incoming students with higher-level mathematics. The task was therefore unduly stressful, even though the self-assessment report was subsequently praised as exemplary by the external reviewers and university authorities. These interviewees stated that when a university commissions a course review, it should issue a clear plan that sets out what data need to be presented and ensures that the relevant staff members can access those data.
Secondly, some interviewees thought that the timeline of the curriculum reform was too compressed, and that many decisions seemed to be made at the last minute. When asked whether it would have been helpful if the implementation of the redeveloped science programme had been deferred by a year, responses were mixed. One said that that might have eased some of the pressure but acknowledged that work tends to expand to fill the time available, regardless of how much time is allotted to it. Another said that a longer process would have lost momentum. One interviewee explained that extending the process was not the solution, but rather, “anticipating the policy decisions, […] working the timelines backwards, identifying when the critical [college applications] decisions are made and the critical marketing [for prospective students] and saying, OK, by this deadline, we need to have made a call about this.” The records of the reform Working Group meetings make it clear that the redevelopment timeline was, in fact, structured around essential dates such as college application deadlines. Nonetheless, there was a perception among some staff members that decisions were cut too fine: “I felt when we got to that first Open Day, we were really cutting it too close, and we were not in a position to project a lot of confidence in the answers that that we provided” … “it was a miracle that [the new programme] was ultimately launched on time.”
Thirdly, interviewees discussed the difficulties involved in getting staff members to engage with the redevelopment process. Academic staff were typically resistant to the idea of making changes to the science programme. The interviewees attributed this reluctance partly to the fact that widespread changes (to exam regulations, the academic year structure etc.) were being made across the university under the Trinity Education Project initiative at the same time as the curriculum reform of the science programme. Staff became exhausted by the continual upheaval of well-worn paths and disconnected from the process. It must be noted that any future assessment of the success of the implementation of the changes to the science programme must be considered in light of the changes mandated via the broader Trinity Education Project. At University level, there has yet to be a review of the outcomes, successes, and limitations of the Trinity Education Project, as only 3 years have passed since its completion in October 2023. The experience of the science courses outlined here should form a key input into any such review.
Finally, according to the interviewees, many research-focused scientists had little interest in the teaching and management of the undergraduate course and there was a general inertia among more senior academic staff who were set in their ways. The extremely busy schedules of all staff members made it difficult to prioritise extra meetings.
4.6 Lessons learned for undergraduate science curriculum reform
When asked how to solve the problem of generating enthusiasm for the reform process, two interviewees said that, in hindsight, more should have been done to establish a common vision for the new programme at the beginning of the process. They acknowledged that building a shared ethos is time-consuming and difficult. Town-hall meetings were held during the programme redevelopment but were poorly attended by academic staff. One interviewee gave the opinion that Heads of Schools and Heads of Disciplines should have been involved much earlier in the process, as they would have been able to communicate directly with the academic staff, alerting them to the forthcoming changes, getting their input, and building support for the changes.
Insufficient communication with academic staff sometimes caused opposition to what was perceived as change for change’s sake rather than change designed to produce real improvements for students, academic staff, and administrative staff. Further suggestions for more specific improvements to the curriculum reform process included: having the timetable for the new science course blocked out earlier in the process, rather than developing the new course first and struggling to make it fit a timetable; having new module code numbers that adhere to international conventions, to avoid confusion when marketing to international students; and placing more importance on the marketing of the new programme to secondary-school teachers and prospective students, a critical step for the success of a new programme.
Finally, interviewees were asked what they would do differently with the benefit of hindsight. One said that, at the programme review stage, they would insist on deadlines being more realistic and would try to find a way for experienced people on the ground, e.g., lab technicians, to have more input. Another said that while managing the implementation of the new programme, they would avoid the pitfall of trying to solve every problem by themselves, so as not to have to bother other people. One interviewee said that they would not stress about the redevelopment so much if they had to do it again; they would remind themselves that everything settles into place eventually, even though the obstacles may seem insurmountable at times. Several interviewees remarked that they would not do anything differently because they were very pleased with what they had achieved. In particular, one said, “It was important to get the right people involved from the very beginning… We had people who had a good understanding of what was happening in other universities – and, crucially, who were open to the idea that change was necessary.”
5 Conclusion
Overall, reforming the oldest undergraduate science curriculum in Ireland to better meet the needs of the 21st century for both the student and the university followed patterns seen in other large-scale curriculum reform. Already the reform has allowed for a more efficient deployment of the course, as is often the case (McKay et al., 2005). While having a top-down university strategy for curriculum reform is often necessary (McEwen et al., 2009), bottom-up engagement with the staff and students themselves was found to be valuable and vital in avoiding mere “linguistic mimicry” of the university’s strategy (Kandiko Howson and Kingsbury, 2021).
The curriculum reform allowed more pedagogical innovation, as has long been a desire in undergraduate science teaching (Bodner, 1992), and the reform process gave special attention to the nuances of the different subject areas (Barnett et al., 2001). Although undergraduate teaching can sometimes be undervalued compared to research (Anderson et al., 2011; Fischer, 2011; Singer and Smith, 2013), engagement by the academic and administrative staff in the process was comprehensive, albeit with lessons learned throughout, and with the COVID-19 pandemic making it more difficult to immediately gauge the impact of the reform process (Noone et al., 2021).
Future work from this study will share the analysis of the students’ perceptions of the reformed science curriculum and will compare student performance data from before and after the reform, following the examples of Mitsis (2015), Noone et al. (2021), and Simon et al. (2019). This will build toward a comprehensive evaluation of the curriculum reform process in the hope that other higher education institutions may likewise choose to evaluate and share the benefits and challenges of reforming undergraduate science curricula.
Data availability statement
The raw data supporting the conclusions of this article will be made available by the authors, without undue reservation.
Ethics statement
The studies involving humans were approved by the Faculty of Science, Technology, Education and Mathematics Research Ethics Committee, and the School of Education Research Ethics Committee, Trinity College Dublin. The studies were conducted in accordance with the local legislation and institutional requirements. The participants provided their written informed consent to participate in this study.
Author contributions
LM: Conceptualization, Formal analysis, Methodology, Writing – original draft, Writing – review & editing, Data curation, Investigation, Project administration, Validation, Visualization. JR: Conceptualization, Formal analysis, Methodology, Writing – original draft, Writing – review & editing. ÁK: Conceptualization, Formal analysis, Methodology, Project administration, Resources, Supervision, Validation, Writing – original draft, Writing – review & editing.
Funding
The author(s) declare that no financial support was received for the research, authorship, and/or publication of this article.
Acknowledgments
The authors wish to extend their thanks to all the academic and administrative staff and the student representatives involved in the reform of the undergraduate science curriculum at Trinity College Dublin.
Conflict of interest
The authors declare that the research was conducted in the absence of any commercial or financial relationships that could be construed as a potential conflict of interest.
The author(s) declared that they were an editorial board member of Frontiers, at the time of submission. This had no impact on the peer review process and the final decision.
Publisher’s note
All claims expressed in this article are solely those of the authors and do not necessarily represent those of their affiliated organizations, or those of the publisher, the editors and the reviewers. Any product that may be evaluated in this article, or claim that may be made by its manufacturer, is not guaranteed or endorsed by the publisher.
References
Al-Samarraie, H., Shamsuddin, A., and Alzahrani, A. I. (2020). A flipped classroom model in higher education: a review of the evidence across disciplines. Educ. Technol. Res. Dev. 68, 1017–1051. doi: 10.1007/s11423-019-09718-8
Anderson, W. A., Banerjee, U., Drennan, C. L., Elgin, S. C., Epstein, I. R., Handelsman, J., et al. (2011). Science education. Changing the culture of science education at research universities. Science 331, 152–153. doi: 10.1126/science.1198280
Annala, J., Lindén, J., Mäkinen, M., and Henriksson, J. (2021). Understanding academic agency in curriculum change in higher education. Teach. High. Educ. 28, 1310–1327. doi: 10.1080/13562517.2021.1881772
Barnett, R., Parry, G., and Coate, K. (2001). Conceptualising curriculum change. Teach. High. Educ. 6, 435–449. doi: 10.1080/13562510120078009
Bodner, G. M. (1992). Why changing the curriculum may not be enough. J. Chem. Educ. 69:186. doi: 10.1021/ed069p186
Busby, S., Cloonan, S., Jordan, G. B., O’Murchu, P., Robinson, D., and Williams, I.. (2014). “External reviewers’ report to council on the review of the TR071 science Programme.” Trinity College Dublin. Available at: https://www.tcd.ie/teaching-learning/quality/assets/pdf/Report%20to%20Council%20on%20review%20of%20TR071.pdf. Accessed April 13 2023
Campbell, C. D., Midson, M. O., Bergstrom Mann, P. E., Cahill, S. T., Green, N. J. B., Harris, M. T., et al. (2022). Developing a skills-based practical chemistry programme: an integrated, spiral curriculum approach. J. Chem. Teach. Int. 4, 243–257. doi: 10.1515/cti-2022-0003
Castillo-Montoya, M. (2016). Preparing for interview research: the interview protocol refinement framework. Qual. Rep. 21, 811–831. doi: 10.46743/2160-3715/2016.2337
Chen, S., Hsu, I. C., and Wu, C. M. (2009). Evaluation of undergraduate curriculum reform for interdisciplinary learning. Teach. High. Educ. 14, 161–173. doi: 10.1080/13562510902757203
Cummings, M. P., and Temple, G. G. (2010). Broader incorporation of bioinformatics in education: opportunities and challenges. Brief. Bioinform. 11, 537–543. doi: 10.1093/bib/bbq058
de Regt, H. W., and Koster, E. (2021). The Hows and whys of philosophy of science teaching: a comparative analysis. Eur. J. Philos. Sci. 11:106. doi: 10.1007/s13194-021-00421-1
Dillon, J., Stevenson, R. B., and Wals, A. E. J. (2016). Moving from citizen to civic science to address wicked conservation problems. Conserv. Biol. 30, 450–455. doi: 10.1111/cobi.12689
Dunne, J., Mahdi, A. E., and O'Reilly, J. (2013). Investigating the potential of Irish primary school textbooks in supporting inquiry-based science education (IBSE). Int. J. Sci. Educ. 35, 1513–1532. doi: 10.1080/09500693.2013.779047
Erduran, S., and Dagher, Z. R. (2014). Regaining focus in Irish junior cycle science: potential new directions for curriculum and assessment on nature of science. Ir. Educ.Stud. 33, 335–350. doi: 10.1080/03323315.2014.984386
Fischer, C. N. (2011). Changing the science education paradigm: from teaching facts to engaging the intellect: science education colloquia series, spring 2011. Yale J. Biol. Med. 84, 247–251.
Fjelland, R. (2022). Teaching philosophy of science to science students: an alternative approach. Stud. Philos. Educ. 41, 243–258. doi: 10.1007/s11217-021-09802-8
Fraisl, D., Campbell, J., See, L., Wehn, U., Wardlaw, J., Gold, M., et al. (2020). Mapping citizen science contributions to the UN sustainable development goals. Sustain. Sci. 15, 1735–1751. doi: 10.1007/s11625-020-00833-7
Fritz, S., See, L., Carlson, T., Haklay, M. M., Oliver, J. L., Fraisl, D., et al. (2019). Citizen science and the United Nations sustainable development goals. Nat. Sustain. 2, 922–930. doi: 10.1038/s41893-019-0390-3
Gladys, M., Rogers, L., Sharafutdinova, G., Barnham, N., Nichols, P., and Dastoor, P. (2022). Driving course engagement through multimodal strategic technologies. Int. J. Innov. Sci. Math. Educ. 30, 19–32 doi: 10.30722/IJISME.30.03.002
Gouvea, J. S., Sawtelle, V., Geller, B. D., and Turpen, C. (2013). A framework for analyzing interdisciplinary tasks: implications for student learning and curricular design. CBE Life Sci. Educ. 12, 187–205. doi: 10.1187/cbe.12-08-0135
Hand, R. J. (1999a). Hard questions—easy science? Or why do scientists not teach philosophy? Teach. High. Educ. 4, 121–124. doi: 10.1080/1356251990040108
Hand, R. J. (1999b). Science education: Consensus versus critique. Teach. High. Educ. 4, 501–510. doi: 10.1080/1356251990040406
Hart, J. (2019). Interdisciplinary project-based learning as a means of developing employability skills in undergraduate science degree programs. J. Teach. Learn. Grad. Emp. 10, 50–66. doi: 10.21153/jtlge2019vol10no2art827
Hester, S., Buxner, S., Elfring, L., and Nagy, L. (2014). Integrating quantitative thinking into an introductory biology course improves students' mathematical reasoning in biological contexts. CBE Life Sci. Educ. 13, 54–64. doi: 10.1187/cbe.13-07-0129
Hicks, O. (2018). Curriculum in higher education: confusion, complexity and currency, vol. 5 Australia: HERDSA Review of Higher Education, 5–30.
Hoffman, K., Leupen, S., Dowell, K., Kephart, K., and Leips, J. (2016). Development and assessment of modules to integrate quantitative skills in introductory biology courses. CBE Life Sci. Educ. 15:ar14. doi: 10.1187/cbe.15-09-0186
Husband, G. (2020). Ethical data collection and recognizing the impact of semi-structured interviews on research respondents. Educ. Sci. 10:206. doi: 10.3390/educsci10080206
Jekel, M., Fiedler, S., Allstadt Torras, R., Mischkowski, D., Dorrough, A. R., and Glöckner, A. (2020). How to teach open science principles in the undergraduate curriculum—the Hagen cumulative science project. Psychol. Learn. Teach. 19, 91–106. doi: 10.1177/1475725719868149
Kandiko Howson, C., and Kingsbury, M. (2021). Curriculum change as transformational learning. Teach. High. Educ. 1-20, 1–20. doi: 10.1080/13562517.2021.1940923
Keesing-Styles, L., Nash, S., and Ayres, R. (2014). Managing curriculum change and ‘ontological uncertainty’ in tertiary education. High. Educ. Res. Dev. 33, 496–509. doi: 10.1080/07294360.2013.841655
Laplane, L., Mantovani, P., Adolphs, R., Chang, H., Mantovani, A., McFall-Ngai, M., et al. (2019). Why science needs philosophy. Proc. Natl. Acad. Sci. 116, 3948–3952. doi: 10.1073/pnas.1900357116
Lewenstein, B. V., and Baram-Tsabari, A. (2022). How should we organize science communication trainings to achieve competencies? Int. J. Sci. Educ., Part B 12, 289–308. doi: 10.1080/21548455.2022.2136985
Louis, A. T. S., Thompson, P., Sulak, T. N., Harvill, M. L., and Moore, M. E. (2021). Infusing 21st century skill development into the undergraduate curriculum: the formation of the iBEARS network. J. Microbiol. Biol. Educ. 22, 10–1128. doi: 10.1128/jmbe.00180-21
Lusk, G. (2022). Is HPS a valuable component of a STEM education? An empirical study of student interest in HPS courses within an undergraduate science curriculum. Eur. J. Philos. Sci. 12:19. doi: 10.1007/s13194-021-00433-x
Manduca, C. A., Baer, E., Hancock, G., Macdonald, R. H., Patterson, S., Savina, M., et al. (2008). Making undergraduate geoscience quantitative. EOS Trans. Am. Geophys. Union 89, 149–150. doi: 10.1029/2008EO160001
Massarani, L., and Merzagora, M. (2014). Socially inclusive science communication. J. Sci. Commun. 13:C01. doi: 10.22323/2.13020301
McEwen, L. A., Harris, D., Schmid, R. F., Vogel, J., Western, T., and Harrison, P. (2009). Evaluation of the redesign of an undergraduate cell biology course. CBE - Life Sci. Educ. 8, 72–78. doi: 10.1187/cbe.07-09-0088
McGregor, R., and Park, M. S. A. (2019). Towards a deconstructed curriculum: rethinking higher education in the global north. Teach. High. Educ. 24, 332–345. doi: 10.1080/13562517.2019.1566221
McKay, J., Yau-ming, L., Fuk-hay, T., White, P., and Wu, V. (2005). Establishment of a model to guide the redevelopment of the radiography undergraduate degree. The Hong Kong Radiograp. J. 9, 51–57.
Medin, D. L., and Bang, M. (2014). Who's asking?: Native science, western science, and science education. MIT Press, Cambridge, MA, USA,
Mercer-Mapstone, L., and Kuchel, L. (2017). Core skills for effective science communication: A teaching resource for undergraduate science education. Int. J. Sci. Educ. B, Part B 7, 181–201. doi: 10.1080/21548455.2015.1113573
Millar, V. (2016). Interdisciplinary curriculum reform in the changing university. Teach. High. Educ. 21, 471–483. doi: 10.1080/13562517.2016.1155549
Mitchell, K. J. (2017). “Reimagining science education at Trinity College Dublin: preparing graduates for a scientific future” in Ireland's yearbook of education 2017–2018. ed. B. Mooney (Dublin: Education Matters), 314–317.
Mitsis, A. (2015). Redeveloping a business undergraduate honours research degree to improve educational outcomes: implications for PhD supervision. Teach. High. Educ. 20, 807–820. doi: 10.1080/13562517.2015.1085853
Murphy, C., Varley, J., and Veale, Ó. (2012). I’d rather they did experiments with us…. Than just talking: Irish children’s views of primary school science. Res. Sci. Educ. 42, 415–438. doi: 10.1007/s11165-010-9204-3
Noone, M., Thompson, A., and Mooney, A. (2021). An overview of the redevelopment of a computer science support Centre and the associated pedagogy impacts. Ire. J. Teach. Learn. High. Educ. 13, 1–24
Oliver, R., Kersten, H., Vinkka-Puhakka, H., Alpasan, G., Bearn, D., Cema, I., et al. (2008). Curriculum structure: principles and strategy. Eur. J. Dent. Educ. 12, 74–84. doi: 10.1111/j.1600-0579.2007.00482.x
Patel, V. L., Yoskowitz, N. A., and Arocha, J. F. (2009). Towards effective evaluation and reform in medical education: a cognitive and learning sciences perspective. Adv. Health Sci. Educ. 14, 791–812. doi: 10.1007/s10459-007-9091-1
Prendergast, P., and Morash, C. (2017). “The trinity education project: university learning in the 21st century” in Ireland's yearbook of education 2017–2018. ed. B. Mooney (Dublin: Education Matters), 301–304.
Rawlings, J. S. (2019). Primary literature in the undergraduate immunology curriculum: strategies, challenges, and opportunities. Front. Immunol. 10, 1–5. doi: 10.3389/fimmu.2019.01857
Roberts, P. (2015). Higher education curriculum orientations and the implications for institutional curriculum change. Teach. High. Educ. 20, 542–555. doi: 10.1080/13562517.2015.1036731
Roche, J., Arias, R., Bell, L., Boscolo, M., Fornetti, A., Knutas, A., et al. (2021a). Taking stock and re-examining the role of science communication. Front. Environ. Sci. 9, 1–5. doi: 10.3389/fenvs.2021.734081
Roche, J., Barber, G. L., Bell, L., Brown, A., Crean, S., Darling, O., et al. (2023). Interrogating illusions of progress: citizen science, science communication, and a call for inclusive reform. Front. Environ. Sci. 11, 1–6. doi: 10.3389/fenvs.2023.1269816
Roche, J., Ni Shuilleabhain, A., Mooney, P., Barber, G. L., Bell, L., and Ryan, C. (2021b). Citizen science in Ireland. Front. Commun. 6, 1–8. doi: 10.3389/fcomm.2021.629065
Shay, S. (2015). Curriculum reform in higher education: a contested space. Teach. High. Educ. 20, 431–441. doi: 10.1080/13562517.2015.1023287
Simon, M., Martens, A., Finsterer, S., Sudmann, S., and Arias, J. (2019). The Aachen model study course in medicine - development and implementation. Fifteen years of a reformed medical curriculum at RWTH Aachen University. GMS J. Med. Educ. 36:Doc60. doi: 10.3205/zma001268
Singer, S., and Smith, K. A. (2013). Discipline-based education research: understanding and improving learning in undergraduate science and engineering. J. Eng. Educ. 102, 468–471. doi: 10.1002/jee.20030
Speth, E. B., Momsen, J. L., Moyerbrailean, G. A., Ebert-May, D., Long, T. M., Wyse, S., et al. (2010). 1, 2, 3, 4: infusing quantitative literacy into introductory biology. CBE - Life Sci. Educ. 9, 323–332. doi: 10.1187/cbe.10-03-0033
Suri, H. (2011). Purposeful sampling in qualitative research synthesis. Qual. Res. J. 11, 63–75. doi: 10.3316/QRJ1102063
Trench, B., and Bucchi, M. (2010). Science communication, an emerging discipline. J. Sci. Commun. 9:C03. doi: 10.22323/2.09030303
Trinity College Dublin. (2016). “Trinity education project interim report.” Trinity College Dublin. Available at: https://www.tcd.ie/academic-services/assets/final_tep_interimreport_council_september2016.pdf. Accessed April 13 2023
Trinity College Dublin. (2023). “About trinity: History.” Trinity College Dublin. Available at: https://www.tcd.ie/about/history. Accessed April 13 2023. Accessed April 13 2023.
Uleanya, K. O., Furfari, S. K., Jones, L. C., Selwe, K. P., Milner, A. B., and Dessent, C. E. (2023). A resource to support decolonization of the undergraduate chemistry curriculum. J. Chem. Educ. 100, 2583–2590. doi: 10.1021/acs.jchemed.2c01003
van Kampen, P. (2022). “Irish school science curricula 1831–2020” in Education policy in Ireland since 1922 (Cham: Springer International Publishing), 401–440.
Varley, J. P., Murphy, C., and Veale, Ó. (2013). At the crossroads: the impact of new Irish science curricula on first year post-primary students. Res. Sci. Educ. 43, 275–298. doi: 10.1007/s11165-011-9252-3
Velthuis, F., Varpio, L., Helmich, E., Dekker, H., and Jaarsma, A. D. C. (2018). Navigating the complexities of undergraduate medical curriculum change: change Leaders' perspectives. Acad. Med. 93, 1503–1510. doi: 10.1097/acm.0000000000002165
Wei, J., Treagust, D. F., Mocerino, M., Lucey, A. D., Zadnik, M. G., and Lindsay, E. D. (2019). Understanding interactions in face-to-face and remote undergraduate science laboratories: a literature review. Discip. Interdiscip. Sci. Educ. Res. 1, 1–16. doi: 10.1186/s43031-019-0015-8
Weir, L. K., Barker, M. K., McDonnell, L. M., Schimpf, N. G., Rodela, T. M., and Schulte, P. M. (2019). Small changes, big gains: A curriculum-wide study of teaching practices and student learning in undergraduate biology. PLoS One 14:e0220900. doi: 10.1371/journal.pone.0220900
Keywords: curriculum reform, higher education science, undergraduate teaching and learning, course development, university, 21st century teaching and learning, higher education in Ireland, academic and administrative reflections
Citation: McAteer L, Roche J and Kelly ÁM (2023) Renewing an undergraduate science curriculum for the 21st century. Front. Educ. 8:1270941. doi: 10.3389/feduc.2023.1270941
Edited by:
Tom Crick, Swansea University, United KingdomReviewed by:
Aloysius H. Sequeira, National Institute of Technology, Karnataka, IndiaZuzana Haláková, Comenius University, Slovakia
Copyright © 2023 McAteer, Roche and Kelly. This is an open-access article distributed under the terms of the Creative Commons Attribution License (CC BY). The use, distribution or reproduction in other forums is permitted, provided the original author(s) and the copyright owner(s) are credited and that the original publication in this journal is cited, in accordance with accepted academic practice. No use, distribution or reproduction is permitted which does not comply with these terms.
*Correspondence: Joseph Roche, Joseph.Roche@tcd.ie