- 1Department of Behavioural Sciences and Learning, Linköping University, Linköping, Sweden
- 2Faculty of Education, University of Johannesburg, Johannesburg, South Africa
STEM—science, technology, engineering, and mathematics—has become important as an educational construct and phenomenon in recent years. However, it is only just recently that STEM education has begun to be examined from a philosophical point of view. There is therefore a need for further investigation of its philosophical basis, particularly in relation to integrated STEM education (iSTEM). Recent conceptual and empirical studies emphasize the crucial role of design in achieving successful STEM integration, and design thinking has also lately gained traction in such integration. The aim of this study is to investigate an integrated philosophy of STEM education, based on the methodological backbone of design. The research methodology consisted of a critical review of the literature regarding the philosophy of STEM (education), science, technology, engineering, mathematics, and STEM education research, related to the current issues of integrating the various STEM subjects. We thus base the philosophical framework on philosophy and studies from/on the STEM subjects in education. It is concluded that from a methodological point of view, design holds promising affordances for unifying the STEM subjects through “pure STEM problems”. Design as part of, for instance, particular engineering design projects may consequently require the “design” of applicable scientific experiments as well as mathematics expressions and formulae specifically when engaging in technological modeling.
Introduction
Integrated STEM education is increasingly viewed as a viable way of preparing students for real-world problem solving in a global society that faces complex social and environmental challenges (Kelley and Knowles, 2016). Integrated approaches to STEM education could promote authenticity and improve learning, prepare students for future STEM careers and higher education paths, as well as develop so-called twenty-first century skills, for example, creativity, innovation, collaboration, and critical thinking capabilities (Hallström and Schönborn, 2019; Banks and Barlex, 2020). Recent conceptual studies emphasize the crucial role of engineering design in achieving successful STEM integration (e.g., Margot and Kettler, 2019; Roehrig et al., 2021). The centrality of design is also largely confirmed by recent empirical studies on integrated STEM education. For example, it is suggested that design connects STEM education to real-world practices and makes students better at applying disciplinary knowledge in the individual STEM subjects (e.g., English and King, 2015; Lin et al., 2021; Hallström et al., 2022; Sung and Kelley, 2022).
However, there is a philosophical vagueness surrounding the concept of integrated STEM education, for example, when it comes to what knowledge components of the individual subjects should be integrated and how (e.g., Erduran, 2020; Ortiz-Revilla et al., 2020, p. 857). The need for a foundational philosophy of integrated STEM education is thus urgent, in order to make educational initiatives rest on a solid philosophical foundation, especially when centered around a particular methodology such as design. It is only just recently that integrated STEM education was first probed from a philosophical point of view, with the aim of investigating what it is and what underpins it theoretically. Most such philosophical work, however good, was done from the point of view of one or two of the individual subjects such as science or mathematics (e.g., Chesky and Wolfmeyer, 2015; Akerson et al., 2018), so there is a need for developing a philosophical framework for integration of more or all of the STEM subjects.
Tang and Williams (2019) tested the concept of integrated STEM literacy empirically, and concluded that:
Based on the similarities found in several language and thought processes of the disciplines, we conclude that there is presently a research basis for postulating a unitary STEM literacy that reflects the shared general capabilities required in all the STEM disciplines. At the same time, there are also substantial differences that support the retention of the existing literacy constructs (i.e., S.T.E.M. literacies) to reflect the specific linguistic, cognitive and epistemic requirements found in each disciplinary area. This distinction from the singular STEM literacy is necessary to highlight the skills and practices that are unique to each particular discipline, and therefore not applicable in all the other disciplines (p. 675).
If integrated STEM education is to remain philosophically solid and powerful as an educational endeavor it is clear that it should revolve around some kind of integration of two or more of the subjects, at the same time as the core content and methods of each subject have to be respected. Hallström and Schönborn (2019) began developing such a framework based on design as a core capability or method common to all STEM subjects, and this study will expand that framework. Design lends itself particularly well to philosophical analysis because it is not only a making activity but also a pattern of planning and thinking, described succinctly by Mitcham (2020): “Engineering design […] constitutes a distinctive way of turning making into thinking, engendering not only a special kind of making but also a unique way of thinking (p. 78–79).” Design thinking could, however, also be seen as a central pattern of thought even in science and mathematics (Bishop, 1988; Doppelt, 2009; De Vries, 2021), which makes it broader than engineering and technological design and thus potentially more novel for STEM integration than the previous studies mentioned above. The aim of this study is to investigate an integrated philosophy of STEM education, based on the methodological backbone of design. The research question that underpinned the study is: What are the affordances of Mitcham's (1994) 4-fold philosophical framework of technology and engineering for unifying the STEM subjects, with particular consideration of the methodology of design?
The research methodology for this conceptual article consisted of philosophical analysis of a selection of literature in the philosophy of STEM (education), science, technology, engineering, mathematics, and STEM education research, related to the current issues of integrating the various STEM subjects (Hospers, 1997; Dusek, 2006). The selection of literature was carried out by, first of all, searching for journal articles, books and book chapters related to the research question and the just mentioned scholarly fields in pertinent search engines (ERIC, Google Scholar, Unisearch), from roughly 2000 to 2022. Furthermore, we included relevant literature that was found in reference lists in previously known philosophical and STEM educational literature, or the literature found through the searches. This literature could in some cases be older than the year 2000, which is natural in philosophical analysis because philosophy relies at least partly on a cumulative acquisition of knowledge. Our review is not a systematic one but rather a critical review to support a philosophical analysis and, as such, it is the degree of sustainability of the philosophical argumentation that decides and ultimately confirms the thoroughness of the review (see Grant and Booth, 2009, p. 93–97).
As such, a philosophical framework is theoretical and cannot, ultimately, be “proven.” However, a philosophical framework, at least in the social sciences and humanities, is used heuristically to advance knowledge of a phenomenon or understand empirical data related to that phenomenon. Thus, to be able to fulfill that function the framework constructed in this study cannot just be made up, but it must be related to the philosophy and science of that phenomenon, in this case integrated STEM education. We consequently base the philosophical framework on (1) Philosophy of STEM and/or science, technology, engineering, and mathematics (education), and (2) Scientific studies from/on the STEM subjects and education. The more sources that can underpin this philosophical framework the better it can be used as a heuristic tool in future studies and in STEM education practice.
A philosophical framework for integrated STEM education
According to the philosopher Mitcham's (1994) 4-fold philosophical framework, technology is manifested as knowledge, volition, activity, and object. Thus, technological knowledge and volition, with their origin within human beings, give rise to technological activities resulting in concrete technological objects. The framework bears many similarities with other frameworks explaining human volition, knowledge and behavioral interaction with an environment, see, for example, Fishbein and Ajzen (2010) and Ankiewicz (2019); the Mitcham framework therefore has a more general and broad application than merely in technology and engineering. These four modes of manifestation of technology have also been linked to the four components of general philosophy, as well as to the analytical tradition within the philosophy of technology, namely, epistemology, axiology, methodology and ontology respectively (Ankiewicz et al., 2006; Ankiewicz, 2016, 2019; De Vries, 2017), as illustrated in Figure 1. There is also a natural affiliation with a general philosophical outlook on the STEM subjects. The subjects/domain-specific knowledge have strong commonalities in terms of knowledge (conceptual knowledge—knowing that), skills/activities (procedural knowledge—knowing how) and axiology, as they form the core of the respective knowledge domain.
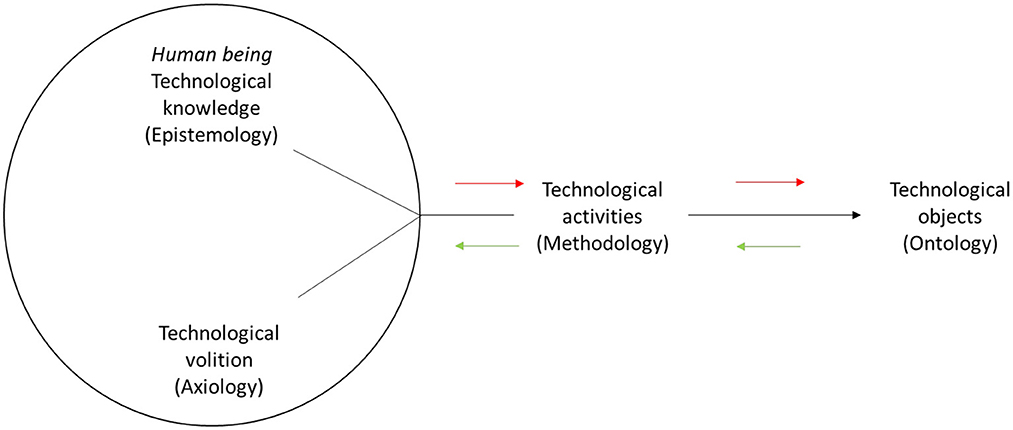
Figure 1. Modes in which technology is manifested (Mitcham, 1994, p. 160 and 209, as adapted by Ankiewicz et al., 2006 and Ankiewicz, 2019).
By superimposing the components of general philosophy onto Mitcham's (1994) framework, a “philosophy modulation” of the latter is obtained. This process is metaphorically similar to the concept of frequency modulation in physics, where the characteristics of one system (here, Mitcham's framework) are changed by impressing another system (here, general philosophy) onto it. Consequently, philosophy modulation in the form of superimposing general philosophy onto Mitcham's four modes of a technology-specific philosophical framework shows how the former can be used to analyze/interpret the latter. On the one hand, technological knowledge and volition give rise to technological activities expressed as concrete technological objects (indicated by the red arrows). On the other hand, objects can also influence peoples' activities, knowledge and their will (indicated by the green arrows).
It follows that all of these four components of philosophy, superimposed on Mitcham's framework, could be applied to the “S,” “T,” “E,” and “M” in a STEM education philosophy, although it remains to be seen from the analysis below whether they can be cogently unified philosophically. Evidence from other studies suggests that the STEM subjects may be too dissimilar concerning ontology and epistemology for a successful philosophical integration on these grounds (e.g., Tang and Williams, 2019). Furthermore, ontology and epistemology are also the most researched philosophical aspects (e.g., Mitcham, 1994, p. 209; Ortiz-Revilla et al., 2020). Thus, methodology and axiology are especially pertinent components of a philosophy of STEM education to research. In order to achieve order and focus to our study, we here deal only with methodology as an area to explore philosophically across the STEM subjects, with special regard to design as a methodology equally important across all the subjects. Mitcham's (1994) analysis includes also other forms of activities such as crafting, inventing, and operating, but designing holds the most promising affordances for STEM integration. We thus need to find common ground for a transdisciplinary STEM philosophy built on authentic interaction and cooperation (English, 2016)—“looking sideways” (Banks and Barlex, 2020), whilst respecting the integrity of each subject—in precisely the methodological dimension.
The centrality of design in all STEM subjects can thus be reinforced by looking specifically at inquiry knowledge (Quinn et al., 2020), that is, procedural knowledge, which translates to Mitcham's (1994) activity and methodology in general philosophy, after the philosophy modulation. Design, not as an exclusive feature of engineering (Ortiz-Revilla et al., 2020), is a common activity/methodology in “S,” “T,” “E,” and “M” when integrated STEM is achieved by means of complex, pure STEM problems and inquiry (Pleasants, 2020). We justify philosophically why the STEM subjects can be integrated, and we have through Mitcham (1994) identified design in broad terms as a means to do this: experimental design in “S;” design of mathematical algorithms and models in “M;” technological design in “T;” and engineering design in “E.” Thus, design—not engineering design as such, although it plays a major role as a form of problem solving (see Kelley and Knowles, 2016)—integrates the STEM subjects philosophically. According to Pleasants (2020), examining the natures of the individual STEM fields is not a sufficient approach. For Pleasants the more productive approach is to examine “pure STEM problems,” which points to the methodological domain, rather than seek overlap among the STEM fields epistemologically (Pleasants, 2020).
It would be possible to view design methodology as a broader societal undertaking (cf. axiology), bringing in even ethical, cultural, political and economic influences as a context in the above model (see Hallström, 2022; cf. “STEM-relevant problems,” Pleasants, 2020). However, in this study design is mainly defined in correspondence with what Feng and Feenberg (2009) designate as “proximate design,” that is, conceptualized as a technical task at a micro level in workshops, design studios or STEM classrooms. In other words, the focus is on the design process in itself and how it can be conceptualized and modeled as a methodology to promote integrated STEM education with pure STEM problems, in real-world practices as well as in classrooms.
Design methodology as a way of philosophically unifying the STEM subjects
Design processes are studied in the discipline of design methodology (De Vries, 2001). Design by its nature is adapting reality—in fact reality as a whole—by changing situations, objects, systems, and natural environments to optimally serve the needs of people (Mitcham, 2020; De Vries, 2021). Two different paradigms form the basis of the discipline of design methodology, i.e., the rational problem-solving and the reflective-practice paradigm. The rational problem-solving paradigm is a more structured approach generally associated with engineers while the reflective practice paradigm is a less structured approach usually associated with architects (Dorst, 1997; Ankiewicz et al., 2006; Ammon, 2017). A combination of the two approaches into the conceptual, information and embodiment stages of design activity results in a dual model of design methodology proposed by Dorst (1997). In the following paragraph we will argue that Dorst's (1997) dual model of design methodology—the combination of the rational problem-solving and the reflective-practice paradigms—is actually applicable in design processes, which not only consist of rational problem solving but also reflective practices with many intuitive elements that make them relevant across the STEM subjects (cf. Kroes et al., 2009).
Design is the primary methodology of technology which is more intuitive than engineering design (De Vries, 2018; Seery et al., 2022) as it has an element of trial and error to it (Williams, 2011) and is in itself “an independent epistemic praxis” (Ammon, 2017, p. 495); thus, it is largely associated with the reflective practice paradigm. However, this type of design also features in engineering (De Vries, 2018; Sung and Kelley, 2022), and engineering is actually a sub-set of the broad area of technology (Williams, 2011). Based on Dorst's (1997) dual model we suggest a definition of design as the combination of the two approaches to design; rational problem solving which is largely associated with conceptual knowledge production, and reflective practice which is to a great extent connected with procedural knowledge production. Design conceived in this way could provide a clue for the problem of how to exploit the affordances of STEM further regarding science and mathematics (De Vries, 2018). Thus, for example, in engineering design problems the “E” particularly brings together the knowing that (or conceptual knowledge) which also characterizes the “S” and “M,” and the knowing how of the “T” (or procedural knowledge; De Vries, 2021).
The roles of design for integrating the STEM subjects
From the literature on STEM education there seems to be mainly two ways of interaction between STEM subjects; the application of existing knowledge from the STEM subjects (e.g., Barlex, 2007) and an approach where there is knowledge development in all STEM subjects at the same time, as well as the application of knowledge from various STEM subjects (De Vries, 2018, 2021). Focusing on design as a methodological characteristic of all STEM subjects as presented above, may be conducive to the application of existing knowledge from the STEM subjects as well as knowledge development in all STEM subjects simultaneously.
In design methodology, therefore, the central methods of the STEM subjects could be integrated. For example, modeling is an integral method of both science, technology, engineering, and mathematics authentic practices and educational endeavors (Hallström and Schönborn, 2019; Tang and Williams, 2019). Models are simplified representations of phenomena. Modeling is therefore used in all STEM subjects, either to represent reality as in science, to represent algorithms as in mathematics, or to represent something that does not exist, for instance, an object or system being innovated, as in engineering and technology (Gilbert et al., 2000; Norström, 2014; Sung and Kelley, 2022). In engineering design, not only engineering and technological modeling are included, but also scientific and mathematical modeling. In many engineering projects, it is common to model scientific phenomena such as when designing structures and materials and making mechanical calculations, in which scientific modeling could be in the form of models of material properties. Mathematical modeling is also frequently applied in engineering contexts and design processes (Brady et al., 2015); in fact, many engineering models are mathematical by nature (Zawojewski et al., 2008; Ryberg et al., 2015).
Barlex (2007) mentions a more incidental type of interaction between the STEM subjects which occurs in design projects when technology students apply knowledge from the other STEM subjects (cf. Williams, 2011). As opposed to incidental interaction between the STEM subjects—and in order to establish a real connection between them—De Vries (2018, 2021) suggests the use of particular technological design challenges around authentic problems in which engineering principles, scientific concepts and mathematical ways of thinking are essential for finding solutions to the challenge. Thus, he advocates an approach where there is knowledge development in all STEM subjects simultaneously, and not merely the application of existing knowledge related to them—similar to how it happens in practice in engineering design projects (Sung and Kelley, 2022). When students' intuitive ideas are challenged in such design projects, they will inquire and perform experiments to understand scientific phenomena that relate to the given problem—scientific design. They will also undertake mathematical calculations to optimize their designs, and do modeling to test this. This activity will result in an integrated STEM activity; a rich technology and engineering design experience, a better understanding of science concepts (that relate to the design) and new experiences and applications of using mathematics (De Vries, 2018, 2021). Such an activity does justice to the nature of design as a process in which both new knowledge is developed (about designing itself, but also science and engineering), and existing knowledge (previously learnt in science, technology, and/or mathematics) is applied (Pleasants, 2020).
De Vries (2018) presents another example from real-world engineering. Scientific knowledge of aerodynamics is developed in the context of designing. Although there are exceptions such as the wholly computer-modeled Boeing 777 (Mitcham, 2020), the design of airplanes is still today also based on tinkering with prototypes and investigating the effects of systematically changing the design of the flying behavior of the airplane by putting models in wind tunnels (cf., Ferguson, 1992). The process of simultaneously developing knowledge about natural phenomena and improving the design can easily be simulated in class by using paper airplane designing and testing. Design in science may also be more experimentally oriented—such as, for example, in synthetic chemistry—whereas design in technology and engineering are not really experiments in the scientific sense but rather epistemic practices of their own that are open ended, iterative and produce their own knowledge (Kroes et al., 2009; Ammon, 2017).
Design in technology and engineering—and even sometimes in mathematics, according to Bishop (1988)—will therefore typically lead to the making of an object, or system (Vermaas et al., 2011). The students will also design mathematical expressions or functions and do calculations to optimize their design by modeling to test their optimisations. The steps of mathematical modeling thus resemble, to a large extent, the stages of engineering design in that they systematically address real-world problems (Wei et al., 2022). These kinds of engineering challenges will fit the nature of design as a process encompassing both rational problem solving and reflective practice, and in the philosophical sense a methodology in which both new knowledge is constructed and existing knowledge is employed (De Vries, 2018; Hallström and Ankiewicz, 2019; Vossen et al., 2020).
Kelley and Knowles (2016) view engineering design as an important component in the integration with science, mathematics, and technology in STEM education, although, for them, it is the community of practice that is the actual integrator (cf. Hacker, 2018; Han et al., 2022; Sung and Kelley, 2022). When considering integrating STEM education components, however, design can become the situated platform and methodology for STEM learning (Hallström et al., 2022). Using design as a catalyst to STEM learning is vital to bring all STEM subjects on an equal platform. The very nature of design thus provides students with a both systematic and reflective approach to solving pure problems that often occur naturally in all the STEM fields. Engineering problem solving could address such problems and build connections among the STEM subjects, which has been identified as key to subject integration (Barnett and Hodson, 2001; Frykholm and Glasson, 2005; Kelley and Knowles, 2016; Daugherty and Carter, 2018; Han et al., 2022; Sung and Kelley, 2022).
Engineers' inquiry is also comparable with scientific inquiry (Sung and Kelley, 2022), something which becomes apparent when designing. Science education can therefore be enhanced by infusing an engineering design approach because it creates opportunity to apply science knowledge and inquiry as well as provide an authentic context for learning mathematical reasoning and modeling for informed decisions during the design process (English, 2022; Wei et al., 2022). The analytical element of the engineering design process allows students to use mathematics and science inquiry to create and conduct experiments that will inform the student about the function and performance of potential design solutions before a final prototype is constructed. This approach to engineering design allows students to build upon their own experiences and provides opportunities to construct new science and mathematics knowledge through engineering design analysis and scientific investigation around a pure STEM problem (Kelley and Knowles, 2016; Han et al., 2022; Sung and Kelley, 2022).
A reinterpretation of Mitcham's framework for STEM education
As we have shown above, Mitcham's (1994) 4-fold philosophical framework holds affordances for cogently unifying the STEM subjects. Bishop (1988) argues that mathematics is basically to be seen as a technology, a human-made “artifact,” and specifically expounds on a conception of design similar to in technology and engineering (cf. Kertil and Gurel, 2016), which further underlines the potential fruitfulness of expanding Mitcham's framework for technology to include all of STEM. Consequently, from a methodological point of view the design in technology and engineering holds promising affordances for unifying the STEM subjects, especially when considering that design in mathematics in certain conceptions also aligns with this (Bishop, 1988). Design as part of pure STEM projects therefore may require the “design” of applicable scientific experiments as well as the design of pertinent mathematics expressions and formulae specifically when modeling in engineering and technology. Based on the findings of this article, we thus expand the “philosophy modulation” and reinterpret Mitcham's 4-fold philosophical framework as follows (see Figure 2): On the one hand, transdisciplinary “S,” “T,” “E,” and “M” knowledge and volition give rise to design activities expressed as concrete STEM objects or projects (indicated by the red arrows). On the other hand, specific STEM objects or projects require design activities by students which develop students' “S,” “T,” “E,” and “M” knowledge and influence their will/STEM volition (indicated by the green arrows). Exactly how STEM design activities “re-affect” human learning and volition (left green arrow) is beyond the scope of this study. Suffice it to say here that for pure STEM problems, design as a methodology is crucial for STEM integration.
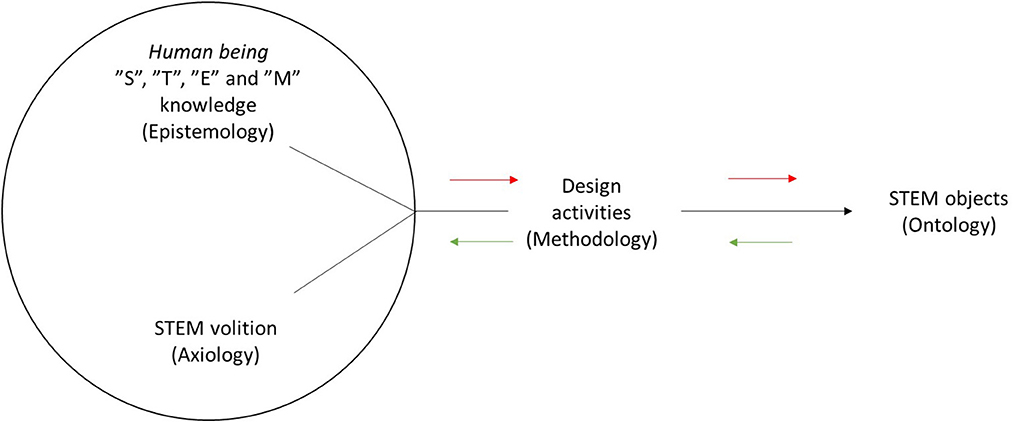
Figure 2. A reinterpretation of Mitcham's (1994) philosophical framework for STEM education.
STEM volition is the will to solve a problem using a transdisciplinary STEM approach. When doing this, knowledge from some or all the STEM subjects is required by “looking sideways” in collaboration without “diluting” the individual subject knowledge/domain-specific knowledge components, as proposed by Banks and Barlex (2020). The specific STEM design activities are embodied in pure STEM problems (Pleasants, 2020), as outlined above representing both a rational problem solving and reflective practice paradigm (Dorst, 1997; Kroes et al., 2009) that would include knowledge from the “S,” “T,” “E,” and/or the “M.” The specific STEM objects that are the outcome of the design activities could be technological or engineering objects or systems, mathematical solutions or models, or scientific results, in response to the problem to be solved.
Conclusion
In this article we underpin a new philosophical framework of integrated STEM education through design, based on Mitcham's (1994) conception of technology. Such a philosophical framework is much needed because previous frameworks were primarily based on integration of one or two of the subjects (e.g., Chesky and Wolfmeyer, 2015). Furthermore, recent research on the engineering design process, authentic STEM projects and the actual execution of collaborative engineering and/or architectural design projects in education support the basic ideas of the framework, in terms of the merger of reflective practice and rational problem solving (e.g., Davidsen et al., 2020). Design in the form of engineering design is also often at the core of integrated STEM projects, either explicitly or implicitly (Hallström et al., 2022), which is also supported by, for example, the American Next Generation Science Standards (2013) and the Standards for Technology and Engineering Literacy (International Technology and Engineering Educators Association, 2020).
Finally, we call for more research about design in STEM classrooms; in particular, studies could test the applicability of the philosophical framework presented in this paper. It is imperative that interventions are carried out which integrate the STEM subjects in, for example, engineering design projects in a similar manner to those described by De Vries (2018, 2021), in which technological and engineering principles, scientific concepts and mathematical ways of thinking are essential for finding solutions to the challenges (cf. pure STEM problems, Pleasants, 2020). Such research should also consider the ways the separate STEM subjects interact around a design challenge, and how students could benefit by engaging in design as a methodology in all STEM subjects. Models and modeling could, for example, be one way of methodologically creating bridges between the STEM subjects in such problems (cf. Hallström and Schönborn, 2019). Furthermore, future studies could consider engineering design in different societal and educational contexts, so as to investigate how both design and integrated STEM education relate to intentionality, contextual and spatial restraints (Feng and Feenberg, 2009; Nazar et al., 2019).
Educational implications
In their recent systematic literature review of classroom iSTEM projects, McLure et al. (2022) argue that “projects that do not allow students to design their own solutions to problems, evaluate those designs and then re-design do not meet criteria for best practice in integrating STEM domains” (p. 10). While this statement certainly validates the philosophical framework put forward in this article, McLure et al. (2022) also express concerns about the lack of relevance to the students' contexts or interests, as well as the lack of actual STEM integration, in the studied projects (p. 10–12). Consequently, we argue that to obtain successful STEM integration and student engagement in classrooms one should import pedagogical approaches to teaching STEM which include problem-, project-, design-, or inquiry-based teaching approaches or strategies (e.g., Wei et al., 2022). We further argue that technology and engineering design projects may be very suitable to promote all the design types which relate to the separate STEM subjects or domain-specific knowledge. Not all science or mathematics projects will necessarily include or promote technology and engineering activities and knowledge. Thus, engineering design could be seen primarily as an authentic instructional problem-solving approach, in which both scientific, technological, and mathematical design will appear. Computational design involving computational modeling or simulations may support such an approach (Tucker-Raymond et al., 2019; Papadakis et al., 2022). Students are thus tasked with an engineering problem, often such a one in which students will need to engage in designing an object or system that will require them to learn and/or use relevant science and mathematics concepts (Ortiz-Revilla et al., 2020, 2022; Pleasants, 2020; English, 2022; Hallström et al., 2022).
Author contributions
Both authors listed have made a substantial, direct, and intellectual contribution to the work and approved it for publication.
Acknowledgments
The authors acknowledge funding for open access publication fees from Linköping University.
Conflict of interest
The authors declare that the research was conducted in the absence of any commercial or financial relationships that could be construed as a potential conflict of interest.
Publisher's note
All claims expressed in this article are solely those of the authors and do not necessarily represent those of their affiliated organizations, or those of the publisher, the editors and the reviewers. Any product that may be evaluated in this article, or claim that may be made by its manufacturer, is not guaranteed or endorsed by the publisher.
References
Akerson, V. L., Burgess, A., Gerber, A., Guo, M., Khan, T. A., and Newman, S. (2018). Disentangling the meaning of STEM: Implications for science education and science teacher education. J. Sci. Teacher Educ. 29, 1–8. doi: 10.1080/1046560X.2018.1435063
Ammon, S. (2017). Why designing is not experimenting: Design methods, epistemic praxis and strategies of knowledge acquisition in architecture. Philos. Technol. 30, 495–520. doi: 10.1007/s13347-017-0256-4
Ankiewicz, P. (2016). “The relevance of indigenous technology knowledge systems (ITKS) for the 21st century classroom,” in PATT-32 Proceedings: Technology Education for 21st Century Skills, eds M. J. de Vries, A. Bekker-Holtland, and G. van Dijk (Utrecht: University of Applied Sciences), 22–34.
Ankiewicz, P. (2019). Alignment of the traditional approach to perceptions and attitudes with Mitcham's philosophical framework of technology. Int. J. Technol. Design Educ. 29, 329–340. doi: 10.1007/s10798-018-9443-6
Ankiewicz, P., De Swardt, E., and De Vries, M. (2006). Some implications of the philosophy of technology for science, technology and society (STS) studies. Int. J. Technol. Design Educ. 16, 117–141 doi: 10.1007/s10798-005-3595-x
Banks, F., and Barlex, D. (2020). Teaching STEM in the Secondary School: Helping Teachers Meet the Challenge. 2nd Edn. London: Routledge. doi: 10.4324/9780429317736
Barlex, D. (2007). “Capitalising on the utility embedded in design and technology activity: An exploration of cross-curricular links,” in Linking Learning, The Design and Technology Association Education and International Research Conference 2007, eds Dr. E. W. L. Norman and D. Spendlove (Wellesbourne: Design & Technology Association), 5–10.
Barnett, J., and Hodson, D. (2001). Pedagogical context knowledge: Toward a fuller understanding of what good science teachers know. Sci. Educ. 85, 426–453. doi: 10.1002/sce.1017
Bishop, A. J. (1988). Mathematics education in its cultural context. Education. Stud. Math. 19, 179–192. doi: 10.1007/BF00751231
Brady, C., Lesh, R., and Sevis, S. (2015). “Extending the reach of the models and modelling perspective: A course-sized research site,” in Mathematical Modelling in Education Research and Practice, eds G. A. Stillman, W. Blum, and M. Salett Biembengut (Dordrecht: Springer International Publishing), 55–66. doi: 10.1007/978-3-319-18272-8_4
Chesky, N. Z., and Wolfmeyer, M. R. (2015). Philosophy of STEM Education: A Critical Investigation. New York, NY: Palgrave MacMillan. doi: 10.1057/9781137535467
Daugherty, M. K., and Carter, V. (2018). “The nature of interdisciplinary STEM education,” in Handbook of Technology Education, ed M.J. de Vries (Cham: Springer; Springer International Handbooks of Education), 159–171. doi: 10.1007/978-3-319-44687-5_12
Davidsen, J., Ryberg, T., and Bernhard, J. (2020). “Everything comes together”: Students' collaborative development of a professional dialogic practice in architecture and design education. Think. Skills Creativity 37, 100678. doi: 10.1016/j.tsc.2020.100678
De Vries, M. J. (2001). The history of industrial research laboratories as a resource for teaching about science-technology relationships. Res. Sci. Educ. 31, 15–28. doi: 10.1023/A:1012610328351
De Vries, M. J. (2017). “Philosophy as critique,” in Critique in Design and Technology Education, eds P. J. Williams and K. Stables (Dordrecht, NL: Springer), 2. doi: 10.1007/978-981-10-3106-9_2
De Vries, M. J. (2018). “The T and E in STEM: From promise to practice,” in Research in Technology Education, eds M. J. de Vries, S. Fletcher, S. Kruse, P. Labude, M. Lang, I. Mammes, et al. (Münster; New York, NY: Waxmann), 11–19.
De Vries, M. J. (2021). “Design-based learning in science and technology as integrated STEM,” in Design-Based Concept Learning in Science and Technology Education, eds M. J. de Vries and I. Henze (Boston, MA: Brill), 14–24. doi: 10.1163/9789004450004_002
Doppelt, Y. (2009). Assessing creative thinking in design-based learning. Int J Technol Design Educ. 19, 55–65. doi: 10.1007/s10798-006-9008-y
English, L. D. (2016). STEM education K-12: Perspectives on integration. Int. J. STEM Educ. 3, 1–8. doi: 10.1186/s40594-016-0036-1
English, L. D. (2022). Multidisciplinary modelling in a sixth-grade tsunami investigation. Int. J. Sci. Math. Educ. 2022, 4. doi: 10.1007/s10763-022-10303-4
English, L. D., and King, D. (2015). STEM learning through engineering design: Fourth-grade students' investigations in aerospace. Int. J. STEM Educ. 2, 14. doi: 10.1186/s40594-015-0027-7
Erduran, S. (2020). Nature of “STEM”?: Epistemic underpinnings of integrated science, technology, engineering, and mathematics in education. Sci. Educ. 29, 781–784. doi: 10.1007/s11191-020-00150-6
Feng, P., and Feenberg, A. (2009). “Thinking about design: Critical theory of technology and the design process,” in Philosophy and Design: From Engineering to Architecture, eds P. E. Vermaas, P. Kroes, A. Light, and S. A. Moore (Dordrecht: Springer), 105–118. doi: 10.1007/978-1-4020-6591-0_8
Fishbein, M., and Ajzen, I. (2010). Predicting and Changing Behavior: The Reasoned Action Approach. London: Taylor & Francis. doi: 10.4324/9780203838020
Frykholm, J., and Glasson, G. (2005). Connecting science and mathematics instruction: Pedagogical context knowledge for teachers. School Sci. Math. 105, 127–141. doi: 10.1111/j.1949-8594.2005.tb18047.x
Gilbert, J. K., Boulter, C. J., and Elmer, R. (2000). “Positioning models in science education and in design and technology education,” in Developing Models in Science Education, eds J. K. Gilbert and C. J. Boulter (Dordrecht: Kluwer Academic Publishers), 1. doi: 10.1007/978-94-010-0876-1_1
Grant, M. J., and Booth, A. (2009). A typology of reviews: An analysis of 14 review types and associated methodologies. Health Inform. Librar. J. 26, 91–108. doi: 10.1111/j.1471-1842.2009.00848.x
Hacker, M. (2018). “Engineering and technology concepts: Key ideas that students should understand,” in Handbook of Technology Education, Springer International Handbooks of Education, ed M. J. de Vries (Cham: Springer), 173–191. doi: 10.1007/978-3-319-44687-5_15
Hallström, J. (2022). Embodying the past, designing the future: Technological determinism reconsidered in technology education. Int. J. Technol. Design Educ. 32, 17–31. doi: 10.1007/s10798-020-09600-2
Hallström, J., and Ankiewicz, P. (2019). “Laying down the ‘T' and ‘E' in STEM education: Design as the basis of an integrated STEM philosophy,” in Proceedings PATT 37: Developing a Knowledge Economy Through Technology and Engineering Education, eds S. Pulé and M. J. de Vries (Msida: University of Malta), 187–194.
Hallström, J., Norstróm, P., and Schönborn, K. J. (2022). “International views of authenticity in integrated STEM education,” in PATT 39: PATT on the Edge Technology, Innovation and Education, eds D. Gill, J. Tuff, T. Kennedy, S. Pendergast, and S. Jamil (St. John's, Newfoundland and Labrador).
Hallström, J., and Schönborn, K. J. (2019). Models and modelling for authentic STEM education: Reinforcing the argument. Int. J. STEM Educ. 6, 1–10. doi: 10.1186/s40594-019-0178-z
Han, J., Kelley, T., and Knowles, J. G. (2022). Building a sustainable model of integrated STEM education: Investigating secondary school STEM classes after an integrated STEM project. Int. J. Technol. Design Educ. 8, 1–25. doi: 10.1007/s10798-022-09777-8
Hospers, J. (1997). An Introduction to Philosophical Analysis, 4th Edn. New York, NY; London: Routledge.
International Technology Engineering Educators Association (2020). Standards for Technological and Engineering Literacy: The Role of Technology and Engineering in STEM Education. Available online at: https://www.iteea.org/STEL.aspx (accessed February 12, 2023).
Kelley, T. R., and Knowles, J. G. (2016). A conceptual framework for integrated STEM education. Int. J. STEM Educ. 3, 11. doi: 10.1186/s40594-016-0046-z
Kertil, M., and Gurel, C. (2016). Mathematical modeling: A bridge to STEM education. Int. J. Educ. Math. Sci. Technol. 4, 44–55. doi: 10.18404/ijemst.95761
Kroes, P., Light, A., Moore, S. A., and Vermaas, P. E. (2009). “Design in engineering and architecture: Towards an integrated philosophical understanding,” in Philosophy and Design: From Engineering to Architecture, eds P. E. Vermaas, P. Kroes, A. Light, and S. A. Moore (Dordrecht: Springer), 1–17. doi: 10.1007/978-1-4020-6591-0_1
Lin, K. Y., Wu, Y. T., Hsu, Y. T., and Williams, P. J. (2021). Effects of infusing the engineering design process into STEM project-based learning to develop preservice technology teachers' engineering design thinking. Int. J. STEM Educ. 8, 1. doi: 10.1186/s40594-020-00258-9
Margot, K. C., and Kettler, T. (2019). Teachers' perception of STEM integration and education: A systematic literature review. Int. J. STEM Educ. 6, 1–16. doi: 10.1186/s40594-018-0151-2
McLure, F. I., Tang, K. S., and Williams, P. J. (2022). What do integrated STEM projects look like in middle school and high school classrooms? A systematic literature review of empirical studies of iSTEM projects. Int. J. STEM Educ. 9, 1–15. doi: 10.1186/s40594-022-00390-8
Mitcham, C. (1994). Thinking Through Technology. Chicago, IL: The University of Chicago Press. doi: 10.7208/chicago/9780226825397.001.0001
Mitcham, C. (2020). Steps toward a Philosophy of Engineering: Historico-Philosophical and Critical Essays. London, New York, NY: Rowman & Littlefield Publishers.
Nazar, C. R., Barton, A. C., Morris, C., and Tan, E. (2019). Critically engaging engineering in place by localizing counternarratives in engineering design. Sci. Educ. 103, 638–664. doi: 10.1002/sce.21500
Next Generation Science Standards (2013). Next Generation Science Standards. Available online at: https://www.nextgenscience.org/sites/default/files/AllDCI.pdf (accessed February 12, 2023).
Norström, P. (2014). Technological Knowledge and Technology Education. (Dissertion), KTH Royal Institute of Technology, Stockholm, Sweden.
Ortiz-Revilla, J., Adúriz-Bravo, A., and Greca, I. M. (2020). A framework for epistemological discussion on integrated STEM education. Sci. Educ. 29, 857–880. doi: 10.1007/s11191-020-00131-9
Ortiz-Revilla, J., Greca, I. M., and Arriassecq, I. (2022). A theoretical framework for integrated STEM education. Sci. Educ. 31, 383–404. doi: 10.1007/s11191-021-00242-x
Papadakis, S., Kalogiannakis, M., and Gözüm, A. I. C. (2022). STEM, STEAM, computational thinking, and coding: Evidence-based research and practice in children's development. Front. Psychol. 13, 1110476. doi: 10.3389/fpsyg.2022.1110476
Pleasants, J. (2020). Inquiring into the nature of STEM problems: Implications for pre-college education. Sci. Educ. 29, 831–855. doi: 10.1007/s11191-020-00135-5
Quinn, C. M., Reid, J. W., and Gardner, G. E. (2020). S+ T+ M= E as a convergent model for the nature of STEM. Sci. Educ. 29, 881–898. doi: 10.1007/s11191-020-00130-w
Roehrig, G. H., Dare, E. A., Ellis, J. A., and Ring-Whalen, E. (2021). Beyond the basics: A detailed conceptual framework of integrated STEM. Discipl. Interdiscipl. Sci. Educ. Res. 3, 11. doi: 10.1186/s43031-021-00041-y
Ryberg, A. B., Bäckryd, R. D., and Nilsson, L. (2015). A metamodel-based multidisciplinary design optimization process for automotive structures. Eng. Comput. 31, 711–728. doi: 10.1007/s00366-014-0381-y
Seery, N., Phelan, J., Buckley, J., and Canty, D. (2022). Epistemological treatment of design in technology education. Int. J. Technol. Design Educ. 2022, 1–15. doi: 10.1007/s10798-022-09781-y
Sung, E., and Kelley, T. R. (2022). “Using engineering design in technology education,” in Applications of Research in Technology Education. Contemporary Issues in Technology Education, eds P. J. Williams and B. Von Mengersen (Singapore: Springer), 9.
Tang, K.-S., and Williams, P. J. (2019). STEM literacy or literacies? Examining the empirical basis of these constructs. Rev. Educ. 7, 675–697. doi: 10.1002/rev3.3162
Tucker-Raymond, E., Puttick, G., Cassidy, M., Harteveld, C., and Troiano, G. M. (2019). “I Broke Your Game!”: critique among middle schoolers designing computer games about climate change. Int. J. STEM Educ. 6, 1–16. doi: 10.1186/s40594-019-0194-z
Vermaas, P., Kroes, P., van de Poel, I., Franssen, M., and Houkes, W. (2011). A Philosophy of Technology: From Technical Artefacts to Sociotechnical Systems. San Rafael, CA: Morgan & Claypool Publishers.
Vossen, T. E., Henze, I., De Vries, M. J., and Van Driel, J. H. (2020). Finding the connection between research and design: The knowledge development of STEM teachers in a professional learning community. Int. J. Technol. Design Educ. 30, 295–320. doi: 10.1007/s10798-019-09507-7
Wei, Y., Zhang, Q., and Guo, J. (2022). Can mathematical modelling be taught and learned in primary mathematics classrooms: A systematic review of empirical studies. Educ. Sci. 12, 923. doi: 10.3390/educsci12120923
Williams, P. J. (2011). STEM education: Proceed with caution. Design Technol. Educ. 16, 26–35. Available online at: https://ariadnestaging.lboro.ac.uk/DATE/article/view/1590
Zawojewski, J. S., Hjalmarson, M. A., Bowman, K. J., and Lesh, R. (2008). “A modeling perspective on learning and teaching in engineering education,” in Models and Modeling in Engineering Education: Designing Experiences for All Students, eds J. S. Zawojewski, H. Diefes-Dux, and K. Bowman (Rotterdam: Sense Publishers), 43.
Keywords: STEM education, iSTEM, design, philosophy of technology, Science, Technology, Engineering, Mathematics (STEM)
Citation: Hallström J and Ankiewicz P (2023) Design as the basis for integrated STEM education: A philosophical framework. Front. Educ. 8:1078313. doi: 10.3389/feduc.2023.1078313
Received: 24 October 2022; Accepted: 06 February 2023;
Published: 23 February 2023.
Edited by:
Jeffrey Buckley, Athlone Institute of Technology, IrelandReviewed by:
Daniela Pedrosa, University of Aveiro, PortugalNicolaas Blom, University of Limerick, Ireland
Copyright © 2023 Hallström and Ankiewicz. This is an open-access article distributed under the terms of the Creative Commons Attribution License (CC BY). The use, distribution or reproduction in other forums is permitted, provided the original author(s) and the copyright owner(s) are credited and that the original publication in this journal is cited, in accordance with accepted academic practice. No use, distribution or reproduction is permitted which does not comply with these terms.
*Correspondence: Jonas Hallström, am9uYXMuaGFsbHN0cm9tQGxpdS5zZQ==
†These authors have contributed equally to this work and share first authorship