- 1Department of Mathematics, Computer Science, and Physics, Roanoke College, Salem, VA, United States
- 2Chance to Change Lives (CCL-US), Pittsburgh, PA, United States
- 3Department of Psychology, Roanoke College, Salem, VA, United States
- 4Department of Chemistry and Physics, University of Mary Washington, Fredericksburg, VA, United States
Gender representation in the physical sciences remains inequitable and continues to lag behind other fields. Even though there exists adequate documentation regarding programmatic postures, difficulties persist within the physics discipline. In this paper, we present innovative, programmatic elements over an 8-year period at an undergraduate, liberal arts, physics program. These elements were added in response to the following two questions: “What practices cultivate an increase of physics major numbers in an undergraduate, liberal arts setting?” and “What practices facilitate a depth of experience for individual physics graduates?” Some of these innovations aligned with published, “best practices” for undergraduate physics programs, while others were novel to the program's context. Within this 8-year period, alterations were separated into curricular and co-curricular elements. Innovations are described, and data are presented in 3-year timeframes before, during, and after their implementation. The number of total majors and graduates increased, including a 200% increase of women degree recipients compared to the previous 10 years. This boosted average graduation rates for women above the national average (30% > 20%). Moreover, women were retained within the undergraduate physics major at a higher percentage during this time period when compared to men in the program. Lastly, these women physics majors maintained careers in science advancement fields at a rate of 80±% after ≤ 5 years post-graduation. While this paper presents a singular case study, the purpose is two-fold: (a) to validate quantitatively the work of national physics organizations within the context of a liberal arts institution, and (b) to suggest that a multi-level approach is most efficacious when considering programmatic innovations.
1. Introduction
1.1. Global landscape
One of 17 United Nations Sustainable Development Goals reads, “achieve gender equality and empower all women and girls” (United Nations, 2023). Within purportedly more rational and logical disciplines in Science, Technology, Engineering, and Mathematics (STEM), one might expect a better fulfillment of this goal. Yet, STEM disciplines reveal a glaring “gender gap” at the professional level, despite the increase of women undertaking higher-education STEM studies. Gender gaps express themselves as differences “between women and men in terms of their levels of participation, access, rights, remuneration, or benefits” (World Economic Forum, 2019). Education is one of the four key areas of identification and measurement. Within the sciences, fewer than 30% of the world's researchers are women, which reflects a clear gender gap (UNESCO Institute for Statistics, 2019). Through a conglomeration of global scientific organizations, individual survey results from global scientists, and publication pattern information, a deep synthesis by Roy et al. (2020) reveals a persistent and significant gender gap within the sciences globally. Sampling equally over 30,000 men and women from 159 countries, this gap remains regardless of STEM discipline, geographic location, or economic development. While what follows is a focused examination of one physics program at an undergraduate institution in the United States (US), the local environment of the study and the national context of the institution are representative of the global landscape.
1.2. National landscape
Over the past 30 years in the US, gender representation in the physical sciences continues to be disproportionately skewed against women (Ivie and Tesfaye, 2012), despite a three-fold increase in the percentage of undergraduate physics degrees awarded to women (6% in 1970 vs. 22% in 2018, Porter and Ivie, 2019). According to a 2021 report by the American Physical Society (APS) on building America's workforce, the importance of developing an inclusive and diverse workforce is crucial to boost the innovation and productivity of science and technology and to maintain America as a global leader in these areas (Johnson, 2021). Problems persist in gender equity issues within most STEM fields (e.g., Hill et al., 2010), though physics remains one of the most inequitable. At approximately 20% women recipients of undergraduate degrees, physics continues to lag behind mathematics (40%), chemistry (~50%), and biology (60%). This considerable difference is displayed clearly in Porter and Ivie (2019), where the percentage of women physics-degree recipients remains approximately constant since 2,000. Within this same climate, the number of undergraduate-degree recipients is more balanced. For example, the National Center for Education Statistics reports that women receive 58% of all undergraduate degrees, while only 36% of those are awarded to STEM majors (US Department of Education, National Center for Education Statistics, 2019).
There are various potential reasons for this continued gender disparity in the physical sciences, including stereotypes relating the practice of physical science to hyper-masculinity (Good et al., 2008; Smyth and Nosek, 2015; Francis et al., 2017), or a lack of perceived representation of women or women role models in the field, in turn leading to issues of belonging and fit (Nelson and Brammer, 2010; Good et al., 2012; Gisler et al., 2018). There are also individual-level factors to consider, including women's lower self-efficacy for physics (not coupled with lower objective skills, Kalender et al., 2020), their experienced science identity (Trujillo and Tanner, 2014; Eren, 2021), or a perceived lack of opportunity to fulfill communal career goals, which tend to be valued more by women (Diekman et al., 2017).
Simultaneous to persistent gender inequity for physics undergraduate recipients, US organizations like the Statistical Research Center of the American Institute of Physics (AIP) address and publicize these known inequities through detailed study, survey, and site visitation (AIP, 2021). The AIP continues to produce valuable statistics on historically underrepresented populations in physics specifically, and STEM fields as a whole. This important work presents the results of labor within the field, as well as the persistent gaps and needed future focus. In addition, commissions of specialists within the discipline have produced and published manuals containing guidelines on undergraduate program efficacy. This is particularly true for undergraduate programs as a whole (SPIN-UP, Hilborn et al., 2003), career preparation (J-TUPP, Heron and McNeil, 2016), and African-American undergraduates within physics (TEAM-UP, James and Bertschinger, 2020). Resources related to programmatic guidance, change, and growth are available through the interactive AAPT-EP3 website, supported by the American Association of Physics Teachers (AAPT/APS, 2021). It is essential that physics programs consult and digest the results of these resources and apply the general guidelines to their specific institutional contexts.
Because the current study focuses on an undergraduate physics program in the US, some differences between traditional American and non-US undergraduate universities are made clear. Most popularly, it is well-known that an undergraduate degree in the US takes approximately 1-year longer than systems outside of the US, particularly European-based systems. Part of this prolonging by the US system accords with an emphasis on general education coursework, which usually delays the declaration of an intended undergraduate degree program, usually referred to as a “major.” Moreover, student choice of an undergraduate major is a novelty within a US-based, post-secondary education, and it is a source of intense study. For example, how students choose an undergraduate major is a recent, scholarly focus for economics (Wiswall, 2021), race and gender studies (Rainey et al., 2018), and social psychology (Denice, 2021). A major declaration is further complicated in a liberal arts environment, which is discussed below in Section 1.3. In contrast, choosing a major is not a feature of many/most non-US systems. Whether in favor of an Dual System, like Germany (e.g., Nash, 2012), or a traditional 3-year framework, undergraduate degree pursuits outside the US are more streamlined.
Within the context of total undergraduate degrees awarded in the US, physics programs maintain a meager percentage of STEM bachelor's recipients. Approximately 20% of all undergraduate degrees are awarded to STEM fields, while approximately 2.2% of those degrees were awarded to physics over the past 20 years (APS, 2020). Even though Ph.D. granting institutions comprise only one-quarter of all institutions offering a physics bachelor's degree, they award approximately one-half of all physics bachelor's degrees. When these national percentages are applied to the reduced numbers of undergraduate populations at liberal arts colleges, where a STEM culture does not often exist, the corresponding enrollments for STEM courses and physics majors are drastically reduced.
While demand for STEM-degree recipients increases, it may appear to the public that the numbers of physics graduates are insignificant compared to the whole. Even though a small percentage of bachelor's degree recipients will pursue graduate studies in physics, the importance of a physics degree persists through the application of the discipline's transferable skills in other fields (Hunt, 2013). “Hidden physicists” (that is, “those who are trained in physics but actually work in a job more widely,” Heron and McNeil, 2016) populate not only other STEM fields and education landscapes, but also peripheral fields like law and management. Physics degree recipients historically score among the highest on standardized, pre-professional entrance exams like the Medical College and Law School Admission Tests (MCAT and LSAT, Tesfaye and Mulvey, 2013; Tyler and Mulvey, 2022). Furthermore, physics degree recipients maintain a privileged position in terms of earning opportunities and employment satisfaction (cf., Figure 3 in Heron and McNeil, 2016). For these reasons, along with the continued need for STEM innovation across various career paths, it is vital that physics programs continue to produce degree recipients for careers as “hidden physicists.” This is especially true at institutions where typically less than half of the bachelor's recipients matriculate to graduate physics programs.
1.3. Local landscape
The current investigation is situated within a context of small number statistics, both nationally within the discipline of physics and locally within an undergraduate, liberal arts college. The physics program discussed here (referred to as the Physics Group) is part of a shared department with mathematics and computer science. Bachelor's degree-granting, physics programs within shared departments comprise less than 10% of all US programs (Mulvey, 2021). Moreover, while offering approximately one-half of the bachelor's degrees, non-Ph.D. granting programs comprise three-quarters of the undergraduate physics landscape. Therefore, a fertile opportunity exists for these programs to affect STEM students with physics content and potential future trajectories.
Beyond the broad differences of matriculation length and focus of study outlined in Section 1.2, a liberal arts setting adds another layer of nuance to the US undergraduate system. Liberal arts colleges in the US typically provide an undergraduate-focused education emphasizing a general curriculum balanced with humanities and social sciences content, which is required for all students. Both public, state-funded and selective, private-funded institutions are labeled “liberal arts colleges.” In addition, most liberal arts institutions have enrollments of less than 5,000 students. Regardless of the differences, the selection of a major is not rushed for undergraduates at these institutions. It is not uncommon to declare a major in the second year of undergraduate studies at a liberal arts institution, where becoming a (physics) major is a celebrated event.
Many non-Ph.D. granting physics programs contain less than five full-time faculty and produce less than 10 graduates per year. For instance, Tyler et al. (2020) present statistics demonstrating that a majority of undergraduate-only programs have five or less graduates per year and five or less full-time equivalent faculty. The Physics Group also finds itself in a similar situation, where it has three tenure-track (TT) members, a non-permanent visiting position (VAP), and a permanent, non-tenure track (NTT) position. This was also the case 10–15 years ago when the Physics Group contained four TT and one NTT faculty, while it awarded 3.6 degrees per year. The number of women Physics Group faculty during the time-period of the study was either two (1 TT, 1 NTT), or three (1 TT, 1 NTT, 1 VAP) of five. While the NTT and visiting faculty members primarily taught physics courses for non-majors, they actively contributed to additional learning experiences for women students such as training teaching assistants, troubleshooting lab equipment, facilitating travel to CUWiP conferences. It is not the focus of the current study to evaluate the effects of faculty gender distribution. The average percentage of degrees awarded to women during the same time period (2003–2012) was approximately 20%, with the national average. When considering published national statistics, the program in this study could be classified as a typical, undergraduate physics program in the US, prior to the study focus (2013–2021).
The current study maintains the following trajectory: innovative, programmatic elements are introduced and described in the next section. Next, we provide representation and retention of physics degree recipients before, during, and after the implementation of the programmatic elements. While causality is not the purpose of the presentation, it will be clear that the increases for the program accord with the implementation period. A discussion of the impact on the Physics Group in light of these increases follows with some suggestions for other similar programs. While each institutional context is unique, and the synergistic effect of innovative elements cannot be deduced a priori, the experience of the Physics Group substantiates two demonstrative realities: published guidelines and statistics support programmatic growth goals, and multi-compartment implementation provides an effective programmatic impact.
2. Materials and methods
2.1. Data acquisition
The Office of Institutional Research and the Office of the Registrar were queried for the numbers of majors and graduates for the previous 20 years so that a baseline of physics participation was determined. For the 8 years of interest within the study (graduates from 2013 to 2021), more detailed information was acquired and collated, including course rosters, extra-curricular participation, and post-graduation employment. This information was accessed through interpersonal communication, social media, and programmatic assessment. The figures within the study were compiled from the results of these data.
The timetable for programmatic alteration was carried out with the following provisos: only one element was implemented in a given year, innovative elements were connected directly to items within the annual programmatic assessment plan, and these elements were carried out for a minimum of 3 years before conducting evaluation. This 3-year baseline for introduction and implementation of programmatic elements forms a justification for the presentation of data in subsequent sections. Innovative elements were implemented locally based on consultation of the Physics Education Research (PER) literature as well as familiarity with the program, as detailed in the following sections. The addition of innovative elements for programmatic growth was driven equally by the following two questions: “What practices cultivate an increase of physics major numbers in an undergraduate, liberal arts setting?”; and, “What practices facilitate a depth of experience for individual physics graduates?”
Responsibilities for implementing innovative elements were shared among the Physics Group faculty. Within courses, the instructor of record maintained primary leadership over the specific implementation and data collection. For intra-programmatic or extra-curricular elements, a particular physics faculty member oversaw implementation and collection. While literature-based elements were implemented as accurately as possible, concessions for context and student populations were judiciously applied. In what follows, physics program alterations are categorized and discussed within the contexts of undergraduate education literature and the particular institutional environment.
2.2. Introduction of innovative elements
Programmatic transformation within STEM undergraduate programs is not clear-cut and often dependent on localized factors. Despite public usage of the STEM acronym, it is clear that even the “S” (science) is not monolithic in its practice (Marder, 2013). Moreover, when considering programmatic change initiatives, points of emphasis vary based on discipline (Reinholz et al., 2019). These differences stand outside the unique departmental and institutional cultures on each campus (Henderson et al., 2015). Detailed case studies of undergraduate programmatic growth exist in the literature for large, US institutions (Stewart et al., 2013) and diverse “thriving” programs (Hilborn et al., 2003). The “best practices” documentation contains some, but not all, of the innovations that are introduced here.
Consideration of significant programmatic change began in 2011 while program faculty were preparing for an external review. This physics program review occurred in 2012, during which some of the proposed elements were discussed with evaluators. Accordingly, a focus on growth pivoted from recruitment to retention, because program faculty overlooked that retention could double the number of physics graduates. While some changes were contextual and on-going, major elements were implemented over a 4-year period, 2013–2017, along two distinct compartments of the program (curricular and co-curricular). With a 4-year undergraduate matriculation period, eight academic years within the Physics Group provide the basis for the present study (i.e., 2013–2021).
2.2.1. Curricular
While faculty may have significant autonomy within the classroom or individual course offerings, instituting change within a curriculum is not an individual escapade. The process requires input and collaboration from all the physics faculty, where consensus is the aspirational goal. Moreover, the students themselves must also display a receptivity to any modifications and justifications that are offered. Here, we present details regarding two major curricular changes.
(A) First-year colloquium. Belonging and science identity are interrelated, drive retention in STEM majors, and may be especially important for women and minority students (Good et al., 2012; Rainey et al., 2018). Outside of a traditional curriculum, these qualities can be facilitated by affording students early and ample opportunities to connect with one another and with faculty members. The first-year, Physics and Engineering Colloquium meets weekly as an exploratory course emphasizing overarching themes in the physical sciences. While maintaining a high relational component for cohort-building, the half-credit course is graded on a “pass-fail” basis and is based on participation, completion of assignments, and written reflection quality. Throughout the semester, the first-year students are also introduced to several different cohorts within the physics major (upper-level students and faculty), while also engaging with a breadth of generalized content (order-of-magnitude estimates, physical modeling, and “how things work”). Hands-on investigations supplement classroom sessions in order to emphasize the experimental aspects of the discipline, as well as increase self-efficacy for tasks needed in subsequent physics courses. In summary, a successful colloquium experience cultivates the following: social capital (cohort-building and inclusion, Abbott and Sapsford, 2005), content engagement in the discipline (identity), and active learning in the discipline (self-efficacy). By instituting a first-semester course where students of similar interests gather, a like-minded cohort of learners is formed within an inclusive environment, which is supported as a means of establishing a STEM identity and sense of belonging (Lewis et al., 2017).
(B) Upper-level laboratories. The Physics Group also made alterations to increase active and applied learning opportunities, as well as facilitate essential experimental skill development. Due to students' interests in applied physics and engineering, two laboratory augmentations were made, while adhering to the college-wide constraints for number of major-only credits. First, the previous, junior-level, one-semester “advanced laboratory” course, which consisted of verifying physical constants, was converted into an intermediate laboratory (The Advanced Laboratory Physics Association, ALPhA, 2021). This laboratory accompanied a third-semester Modern Physics course that introduced the following novel facets: more developed experimentation and report writing, deepened uncertainty quantification, and emphasized historical and philosophical aspects of science.
With space created at the junior-level, a second laboratory course was added to effectively and efficiently address interdisciplinary topics within the physics major. Following guidance from the literature on Course-based Undergraduate Research Experiences (CUREs, Auchincloss et al., 2014; Wooten et al., 2018), authentic perspective was provided on the specific techniques, while also instructing the students on content that they would not otherwise receive (Mordacq et al., 2017). Students collaboratively completed four mini-research projects in the following areas: Astrophysics, Biophysics, Materials, and Optics/Spectroscopy. These four areas coincided with four, rotating, upper-level electives. As a result, students were introduced to all interdisciplinary electives through the CURE at a cursory level, even though they will not take all the courses in their entirety.
2.2.2. Co-curricular
Guidance from US-based organizations on undergraduate physics education emphasizes several co-curricular strategies to facilitate student and program success (Hilborn et al., 2003; AAPT/APS, 2021). All published studies mention a vibrant Society of Physics Students (SPS) chapter, or similar institution-based student cohort. With very little previous involvement, the Physics Group became more active with SPS in 2013, though its first year-end Chapter Report was not submitted until 2015. Here, five co-curricular, programmatic innovations are introduced that extend beyond a thriving SPS chapter.
(A) Public science outreach (informal programming). Cohort-building is a significant component of the program already mentioned (e.g., the first-year physics colloquium). Cohort-building implies an individual belonging and inclusion that traditional usage of “community” does not (Gowar, 2013). Another way to build interest-based inclusion is through student groups and science outreach to the public (Hinko et al., 2016). Science outreach opportunities (or “informal programs”) not only serve public scientific literacy by raising awareness at an early age, but informal programs also empower undergraduates (Rethman et al., 2021). When the Physics Group began a concerted informal program effort 10 years ago, most of the events were faculty-organized and led. Within responsible and eager undergraduate leaders, informal programs transformed into a student-led effort. One such example was the total solar eclipse of 2017, where students served as Eclipse Ambassadors, which resulted in a nationally recognized award (Blake Lilly Prize). After conducting a well-organized event that served over 500 citizens, physics majors presented their experiences to their peers after their return. This further resulted in local news articles about their ambassadorship. Experiences such as these provide demonstrable opportunities for increased inclusion, efficacy, and identity within physics.
(B) Junior review. A second, related co-curricular addition to the program is Junior Review, an informal interview involving at least two faculty members and the individual physics major. This addition to the program is beneficial for multiple reasons. First, having multiple faculty members in attendance allows students to participate in collegiality and camaraderie first-hand. This approach also fosters belonging within an inclusive learning environment, which seems particularly meaningful for women (Lewis et al., 2017). Secondly, informal questions encourage each student to verbalize the ways and directions in which their interests may have changed (e.g., “In what ways has your interest in physics increased and/or decreased?”). Such self-reflection contributes positively to learning and achievement, and may help develop students' sense of meaning or purpose within physics (Fleenor, 2018). Instances of “hidden physicist” trajectories often arose within Junior Review conversations, which encourage new avenues of exploration are not hindered by presumptive assumptions (Alon, 2009). Third, the review is also an opportunity to facilitate participation in “high-impact practices” tied to deep learning, including research mentored by faculty, supportive minors and/or concentrations, and off-campus internships (Heron and McNeil, 2016).
(C) Conference attendance. Prior to 2012, student conference attendance within the Physics group was primarily synchronized with the mentoring faculty researcher. This was sparse, totaling less than five instances in 10 years. There were many contributing factors, including a lack of faculty attendance, lack of results, and lack of funding. Beginning in 2012, students attended conferences where undergraduate participation was encouraged regardless of faculty presence (e.g., regional opportunities). The institution developed on-campus poster sessions where students could present their work in less-threatening environments. With the establishment of a campus-wide Director of Undergraduate Research, monetary funding opportunities for students increased. These college-wide initiatives led to increased numbers of physics majors attending conferences.
(D) Definitions of physics excellence. Another co-curricular initiative related to the number and definition of year-end physics recognition, for (not-yet) majors. Traditionally, the institution sponsored one “Senior Scholar” award for the highest academic grades. To incorporate a holistic picture of excellence reflecting more than academic achievement, and to facilitate identity and belonging within the discipline, several new awards were added. For example, year-end recognition for majors was given for research within and service to the Physics Group. First-year awards were given for early achievement in the discipline to those considering a major in physics. These emphases properly reminded students that grades (marks) do not solely determine their undergraduate success, their inclusion within the discipline, nor their future trajectory as a physicist.
(E) Experiential learning. Traditional extracurricular research and internship opportunities are widely recognized as best-practices for cultivating STEM identity and belonging, particularly within traditionally underrepresented STEM populations (Estrada et al., 2018). Due to the limited number of research projects within the Physics Group, faculty pursued creative avenues for physics-related extracurricular experiential learning (EEL). Beyond more common, widely-publicized Research Experiences for Undergraduates (REUs), EEL opportunities for majors within the Physics Group were initiated with regional industry corporations, regional and on-campus collaborators, and Physics Group alumni. An introductory independent study course was also created to better prepare students for their (predominantly) summer EEL participation. The pre-emptive courses gauged student interest, facilitated research prowess, and built resilience. By not making a research requirement within the curriculum, the Physics Group invites a student to discover for themselves how best to uniquely experience physics. These opportunities not only increase the total number of students who participate in EEL, but they also broaden and diversify how a physicist is defined in society.
3. Results
Since specific changes and their effects are not isolated, program data are presented before, during, and after implementation. This precedent follows best-practices by recognizing departmental culture and multiple change agents (Dunne and Zandstra, 2011; Reinholz et al., 2019). Where results pertain to primarily one element, it is recognized that other elements are also “running in the background.” Entanglement between innovative elements is discussed after the presentation of the increases in programmatic markers.
Two features of the results deserve clarification. There is a preference to average (or sum) over 3-year increments in the data presented. There are three justifications for this approach. One, the innovations were staggered and repeated for a 3-year timescale before evaluating their effectiveness. Curricular implementations did not initiate in the same academic year, so the 3-year average provides an opportunity to see the partial development of one innovation and its integration within the program more holistically. Second, a 3-year timescale defines the active trajectory for an undergraduate physics major. Upper-level core courses are taught on every-other-year basis, which also serves to compress the third and fourth year of the program. Moreover, by a student's fourth year, much of what they do serves as preparation for post-graduate decisions. While a physics major may undergo some significant intellectual transformation within their fourth year, this is an exception not the rule. Third, a 3-year timescale provides an opportunity to discuss numbers of physics majors (and graduates) in more meaningful quantities. Since the issue of small-number statistics forms the basis for a second clarification, we now transition to that discussion.
Historically, the number of people pursuing physics degrees has always been small in comparison to the whole of undergraduate degrees awarded. Compounded with that fact is the total enrollment at the liberal arts college where the study was conducted (~2, 000). Since Poisson distribution statistics characterize samples that are collected at random but with a definite average rate, we believe that they accurately describe the occasion of a woman choosing and matriculating through an undergraduate physics program. The definite average rate is set by the typical 4-year matriculation period through the US undergraduate system, where the randomness is based on the gender of a student and their choice of a major. The uncertainties for Poisson samples are provided by , where N is the number of events. Such uncertainties are valid whether cumulative or average populations are examined, since the standard deviation for a Poisson distribution is also given as , where μ is the mean count (Taylor, 1997). Based on the programmatic justification for 3-year increments given above, Poisson uncertainties measure the benefit of innovation implementation above a typical statistical noise.
The use of 3-year increments and Poissonian uncertainties are utilized throughout the results of the study. Therefore, a description is provided for the general presentation of the results section. In all histograms outlining the numbers of students for time-periods before and after the study, the results are binned according to the same years. A marker denotes the initiation of the study. When error bars are provided, they are calculated by the square-root of the number, whether cumulative or average numbers are presented. When other presentations of data are utilized, they are explained in context.
3.1. Increased number of physics majors and graduates
During the time period of implementation (2012–2021), the Physics Group did not have any external changes regarding number of faculty, departmental situation, or physical location. Over the 20-year period presented here, there was some faculty turnover though the number of positions remained constant. With respect to national standards, the Physics Group is considered “normal” regarding the number of physics faculty (Tyler et al., 2020). However, the number of women faculty could be considered important since it was higher than the national average (40% compared to 20%). The Physics Group's building did not change during the period of implementation and remained the oldest academic building without renovation.
Figure 1 displays the 3-year averages for the number of physics majors officially declared and bachelor's degree recipients. The data covers a 20-year time period, which extends significantly before the implementations were added. As a reminder, the data column “2013–2015” covers the initiation period for several innovative elements, including the first-year colloquium. The following averages are more revealing when the innovative elements permeate a physics major's full matriculation. Specifically, from 2016 to 2021, the average number of graduates was 9.8, while previously it was 3.9 (2001–2015). Not only did the number of graduates increase by more than two-fold, but the yearly fluctuation stabilized considerably.
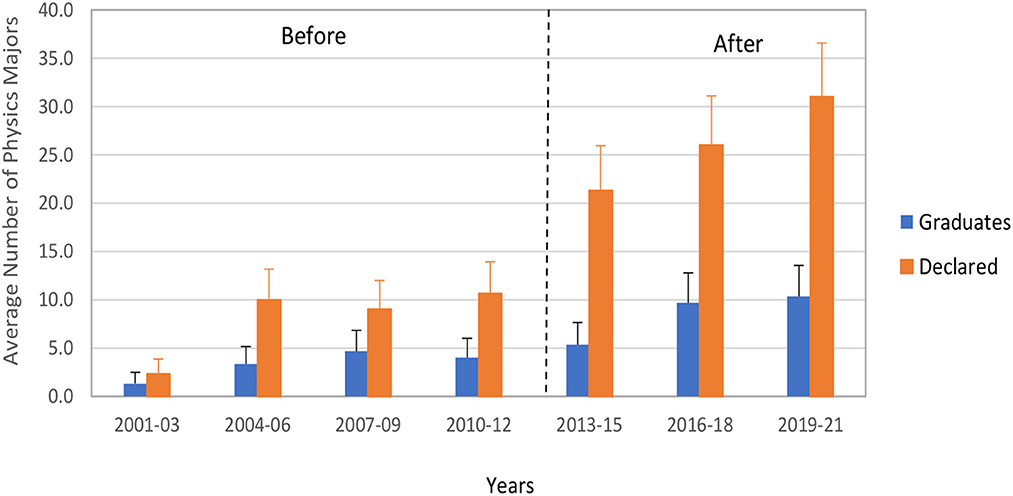
Figure 1. Three-year averages for the numbers of declared physics majors (“declared”) and the number of undergraduate degree recipients (“graduates”). Differences between the number of declared and the number of graduates are based on the definition of physics majors as discussed in the text (Section 1.2). Vertical dashed line demarcates the beginning of the innovation initiation.
One immediate result of adopting an inclusive, cohort-building mindset was the admittance of Sophomores (second-year students) as declared majors. This practice began in 2013 and helped explain the significant increase in “declared” column between 2012 and 2013. Therefore, declared majors include second-, third-, and fourth-year students intentionally pursuing a physics degree. Prior to 2013, a “gate-keeping” mindset was more prevalent in the Physics Group, which required majors to show proficiency in upper-level coursework. This change in mindset and practice afforded students earlier access as members of the cohort, including a greater sense of connection to the discipline. This decision does not fully account for all the increases observed, since the number of graduates also increased significantly after 2016.
Lastly, the noticeable increases of physics majors and graduates cannot be due to a weakening of the program or a loosening of accountability for its majors. During the time period covered in Figure 1, the number of credit units for the major remained roughly the same. In fact, with the addition of the first-year colloquium and lab restructuring (c.f., Section 2.2.1), one could make the argument that the amount of coursework increased by at least one unit during the time period. Student expectations and engagement heightened due to programmatic augmentation, which was evidenced by recognition for both individuals (Goldwater Scholar) and the program (SPS Chapter Award).
3.2. Increased number of women degree recipients
While the numbers of physics degree recipients have increased considerably over the last 40 years, the percentages for women recipients remain approximately constant at 20±5% since 2,000 (Porter and Ivie, 2019). Figure 2 displays the number of women bachelor's recipients in the Physics Group over a 20-year period. Specifically, the yearly average of women graduating in physics from 2001 to 2012 is 0.75, while the same average from 2013 to 2021 is 2.4. As a 200% increase is weighed, a few considerations are addressed.
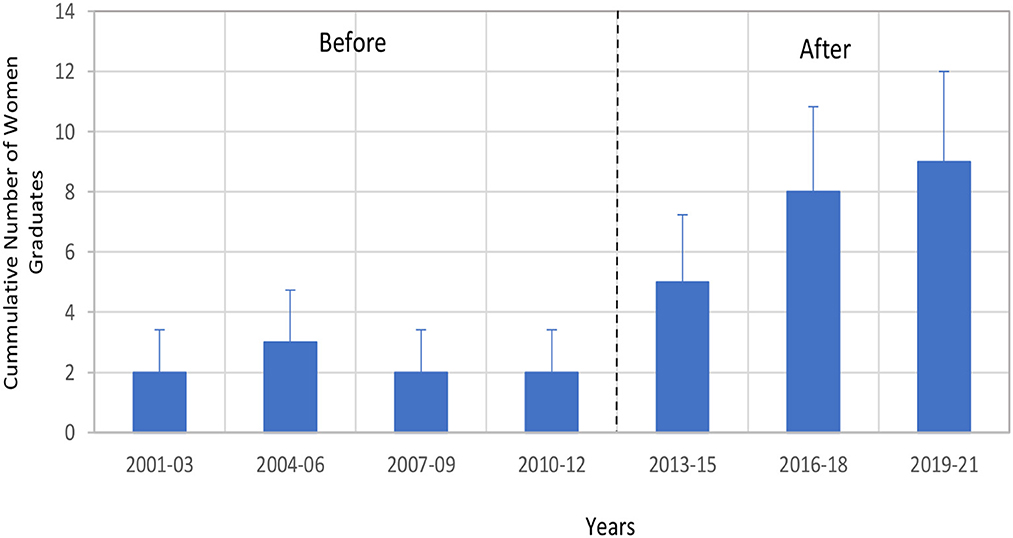
Figure 2. Three-year, cumulative numbers of women who received an undergraduate physics degree in the Physics Group over a 20-year period. Vertical dashed line demarcates the beginning of the innovation initiation.
The undergraduate institution where the Physics Group is located enrolls a higher percentage of women undergraduate students, usually around 60%, which is also the case for the period of the study. However, prior to the study (2001–2012), the average percentage of women was higher (closer to 65%). No particular alterations were made that would easily explain the significant rise in women physics graduates. For the years of focused implementation (2013–2021), the percentage of women graduates held steady around 30%, which is about 10% above the US average. The retention of women in physics during the implementation period is now examined as a function of two innovative elements.
3.3. Increased participation in experiential learning
Over the last 10 years, there has been a steady increase in the number of EEL opportunities from which our students have benefited. Figure 3 displays data to support evidence of growth in the number of physics majors participating in one or more EELs. This includes all research performed by students on campus supervised by physics faculty, collaborative and/or inter-disciplinary research projects with other departments (chemistry, computer-science, mathematics), off campus research experiences such as NSF sponsored REUs and collaborative projects supervised by off campus mentors, and internships. Several of our physics graduates often have more than one such EEL. Between 2013 and 2018, the 5-year average number of on-campus EEL for our students increased to 90% compared to 69% for the previous 5 years 2007–2011. More starkly, the numbers of off-campus EEL for our majors has increased by a factor of six (7–45%) over a similar period. Figure 3 reflects the growth in each area over the time-period considered.
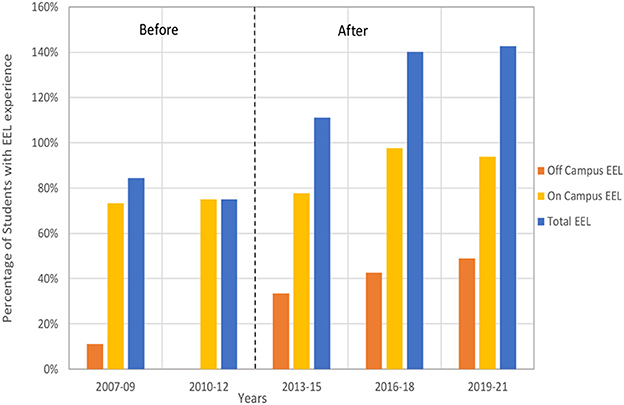
Figure 3. Percent participation for all Physics Group majors in one (or more) EEL opportunities averaged over 3-year periods. Multiple experiences by the same student were tallied, therefore 100% participation should not be interpreted as every major had a single experience. “On Campus” EEL include inter-disciplinary opportunities with programs like biology, chemistry, computer science, or mathematics. “Off Campus” EEL include internships, REUs, and other collaborative partnerships.
3.4. Retention of women physics majors
Prior to 2013, there was no consistent means of accounting for matriculation in the physics major as a function of original interest. For example, there was no direct measure of incoming interest compared with the number of students who enrolled in the first semester of calculus-based physics (in Spring). Therefore, the retention rates presented since the implementation period (2013) have no prior comparative data. That said, it is still clear that the number of women retained within the physics major accord with the innovative elements introduced in the program (cf., Section 2.2).
3.4.1. With respect to first-year colloquium enrollment
Since 2013, a first-year colloquium was required for the physics degree, and it was strongly recommended by pre-admission advising to all incoming students who were interested in physics and/or engineering. The enrollment for the course increased steadily for every pre-pandemic year, from 19 in 2013 to 36 in 2019. Figure 4 presents the retention percentages by gender for original enrollers who persisted until graduation. Due to the frequency of course offering and higher enrollments, retention percentages are provided for each year during the study. The asterisk for 2019 indicates those who had not officially graduated before the completion of the study, so their retention was measured prematurely (at the end of the third year). Since it is in the third year that upper-level coursework begins, it is held with more certainty that a student would be retained. With the exception of a single year (2015), women were retained within the physics major at a higher percentage than men. Therefore, during the time period of the study, women were retained in the physics major at a higher average percentage than men.
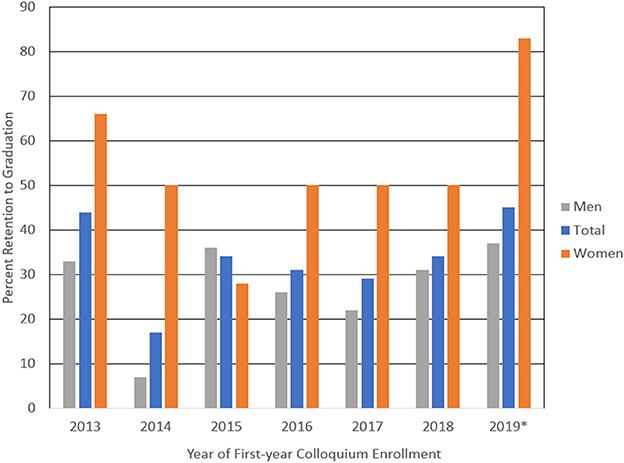
Figure 4. Retention-to-graduation within the Physics Group for participants in the first-year colloquium by gender. The percentages are based on final enrollments in colloquium and final numbers of degree recipients. The asterisk for 2019 indicates those who had not officially graduated before the completion of the study. Their retention was calculated after the third-year of completion.
Even though the first-year colloquium continues, more recent numbers are not given here for two reasons. In Figure 4, 2019 is the last year that majors can confidently be reported as matriculating through the major, since a student's first- and second-year remain less certain. Second, since the course maintains a high relational component, pandemic effects are unclear for both institutional and colloquium enrollments.
3.4.2. With respect to conference participation
In particular, the Conference for Undergraduate Women in Physics (CUWiP) provided a timely and specific opportunity for women physics majors in the Physics Group. These regional conferences are supported by the APS through funding from the National Science Foundation (NSF) and the Department of Energy (DOE). Typically, associated costs for conference participants are subsidized. Figure 5 shows the increase in numbers of women participants at CUWiP conferences, where each participant is counted only once. These increases are independent and irrespective of the increases in total numbers of majors.
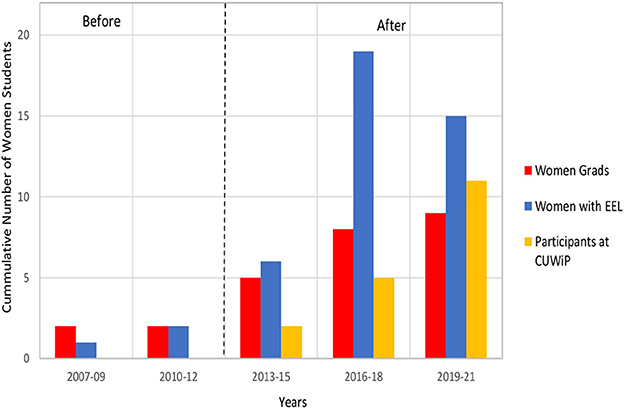
Figure 5. Retention-to-Graduation for women in the Physics Group in comparison with EEL and CUWiP participation. Cumulative number of participants at the APS Conference for Undergraduate Women in Physics (CUWiP) by 3-year grouping. Prior to 2013, there was no CUWiP attendance in the Physics Group. If a participant attended more than once, it is only tallied at the first instance and suppressed for subsequent years. Multiple EEL for the same student were included in the tallies.
3.4.3. With respect to EEL participation
As a reminder, the following types of opportunities are included as EEL participation: REU experiences, on-campus research with STEM faculty, STEM-related educational experiences, and physics-related internships. Students who participate in multiple EEL, particularly within historically underrepresented groups, are shown to positively correlate with self-evaluated STEM identity (Estrada et al., 2018). Figure 5 shows the relationship between women physics graduates and their participation in EEL opportunities. The number of EEL accords with the increases in women physics degree recipients. For Figure 5, multiple EEL for the same woman, physics major are included in the total, so only for the years “2016–2018” are there an average of >2 EEL per woman graduate. During the years of innovative element implementation (2013–2021), there was greater than one EEL opportunity per woman graduate. For years prior to 2007, there was no EEL information cataloged.
3.5. Retention of women within STEM careers
As displayed in Figure 2, noticeable and consistent increases in women degree recipients begin during the “2013–2015” segment, which coincides with the initiation of most innovative elements. This same 3-year segment also marks the first time where the average number of physics majors eclipses 20.0, and total graduate average above 5.0 (c.f. Figure 1). Since immediately after graduation presents another pressure point for attrition from STEM fields, we maintain an interest in what these retained physics graduates do after they receive their degree.
Figure 6 presents the decisions for 22 women degree recipients from 2013 to 2021, 1-year after graduation. Decisions are separated loosely along the following categories: Physics-related graduate school (PHYS GS), non-Physics, STEM-related graduate school (STEM GS), STEM-related employment professions (STEM Employ), STEM-related education professions (STEM Ed), and non-STEM employment (non-STEM). Of the 22 women, < 10% (2/22) persist in a career not directly related to STEM advancement. Here, advancement is defined as involvement in STEM research and support (STEM Employ), learning (GS), or education (STEM Ed). Therefore, women graduates from the Physics Group matriculate into STEM-related trajectories at ~90% during the 2013–2021 time period.
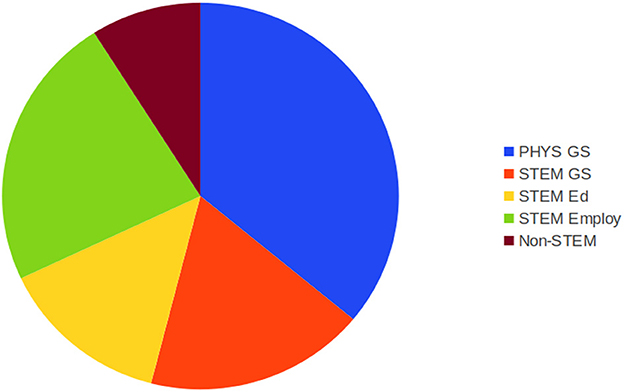
Figure 6. Post-graduate career trajectory decisions for 22 women degree recipients from the Physics Group in the years 2013–2021. Decisions were categorized 1-year after graduation. All but two of the graduates were retained within STEM advancement positions. Legend categories are discussed in the text (Section 3.5).
Graduate training, whether physics-related or STEM broadly, comprised over half of the post-graduate decisions (12/22) for these women. Physics-related training (PHYS GS) included physics subdisciplines, engineering, and materials science. More broadly, STEM-related, graduate training (STEM GS) included fields like computer science, applied math, and veterinary medicine. A few of the women graduates (3) completed their graduate training since leaving the Physics Group, and all three matriculated into STEM-related employment. Moreover, at the time of publishing, all of these women graduate students remained in their programs, ranging from 1 to 6 years later, or graduated from them.
When considering the entire group of women bachelor recipients from 2013 to 2021, a more broad statement emerges about their longevity within STEM advancement careers. Through continuing to follow the post-graduate trajectories of the 20 women already involved in STEM advancement, we find that all of them continue to find meaningful, STEM-related employment, even up to 8 years after graduation, beginning in 2013. Even though some of them have transitioned into parenthood, parallel fields, and/or promotion, these women continue to thrive within STEM-advancement careers.
4. Discussion
To summarize, several innovative elements augmented the undergraduate program in the Physics Group over a period of 8 years. With a staggered initiation, these additions were categorized as curricular, co-curricular, or experiential (Section 2.2). Some of these elements were introduced as a result of national organization documentation, some from STEM literature, and some were novel within the Physics Group. Changes and growth in the program were noted over the same 8-year period, from 2013 to 2021. There were three primary results that accorded with the addition of these innovative elements. First, the total number of majors and graduates increased by approximately 200% compared with the previous 13 years (Figure 1). Second, the number of women in the major were retained at a higher rate than men (Figure 4). Third, these women graduates were employed in STEM-advancement positions at ~90%, from 1-year after graduation and extending out to 8 years post-graduation (Figure 6). Since the implementation and evaluation period of the elements overlapped, it was impossible to determine which elements contributed specifically to each result. That said, we provide a guiding analogy to frame an interpretative discussion of these results within the context of programmatic change.
4.1. Leaky pipelines and delta distributaries
Historically, “leaky pipelines” refer to losses in STEM representation, ranging from aggregate (National Institute of Food and Agriculture, 2014) to minoritized populations (Liu et al., 2019), and even both (Metcalf, 2010). More specifically, the analogy pertains to a decrease in STEM participation for women, initially beginning in transitions from secondary to undergraduate (Archer et al., 2016), then extending to Ph.D. representation (Miller and Wai, 2015) and careers in academia (Sheltzer and Smith, 2014). Systemic problems are prevalent with historically underrepresented groups that are more clearly recognized at formal transitions, though other alternatives are suggested (Rainey et al., 2018; Witteveen and Attewell, 2020). Logically, since the numbers of men and women in K-12 are roughly equal, including the numbers of students taking physical science coursework in high school, then there should be roughly equal numbers of women and men in STEM careers. For women in physics and engineering, there are greatly reduced numbers of bachelor's recipients, which is interpreted as a “leak.” Sometimes the cause of a leak is focused on gender discrimination (Grogan, 2018) and sometimes more broadly on the nature of science (Blickenstaff, 2005). However, questions about the analogy have been raised, either as to its efficacy toward improvements (Cannady et al., 2014) or its over-simplicity (Hinton et al., 2020).
The innovative changes implemented in the Physics Group offer a more inclusive and branched approach than a pipeline analogy. By cultivating a “hidden physicist” model, the program encourages students to view physics as a pathway to diverse STEM careers. Perhaps, a more flexible image is more helpful. Specifically, the Physics Group considers a river delta analogy where distributaries (post-graduation decisions) are initially kept broad within a primary tributary (major program) but allowed to spread and disseminate at the delta by the third- and fourth-years. Figure 7 shows a schematic of a typical river delta. While not completely accurate in every detail, we note that river delta plains become fertile areas for new growth. By leaving unanswered the question, “What can a physics major do?,” any number of post-graduation options are encouraged (Fleenor, 2018). We examine a river delta analogy within two important concepts, STEM identity and programmatic structure.
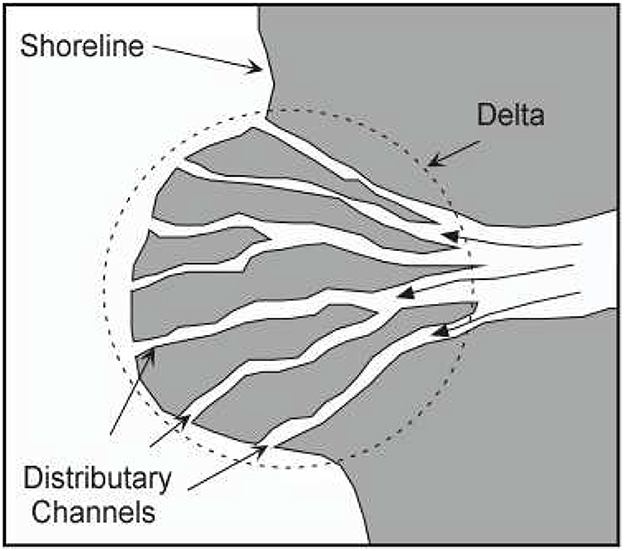
Figure 7. A typical schematic of a river delta. The primary tributary (right side) branches into several distributaries as it nears the much larger body of water. The Physics Group image of a typical undergraduate's matriculation through the program loosely follows this image (https://www2.tulane.edu/~sanelson/Natural_Disasters/riversystems.htm).
4.2. Programmatic structure, building the banks of the tributary
Within a river delta analogy, the number and strength of the distributaries depends on the tributary. In our analogy, the augmented physics program serves as the tributary and includes student majors with faculty. The innovative elements discussed in Section 2.2 provide fortified banks for the increased number of majors. To be clear, “fortifying” does not mean constraining individual choices, whereby students may choose to leave the program and/or switch majors. In the establishment of STEM identity, freedom and encouragement must be given as students persist through difficult circumstances (Fleenor, 2021). By drawing on best-practices from PER literature, in combination with unique innovations specific to the Physics Group (Henderson et al., 2015), a well-fortified tributary is kept. Two implemented examples from the physics program confirm the imagery of building strong banks for women in undergraduate trajectories.
The first-year colloquium serves as the tributary entry point. Keeping the course in an exploratory state, while also introducing a broad diversity of those who are in the Physics Group, seems to keep undecided participants in an interested state. The connection seems clear between the increase of majors and graduates overall (Figure 1) and the introduction of the first-year colloquium (Figure 4). An open award structure for physics excellence rewards the unique contributions and accomplishments of many who persevere in one of the most rigorous disciplines (Section 2.2), while early participation within many informal programs sponsored by the Physics Group helps to establish identity (Rethman et al., 2021). By continuing to keep the tributary entry broad, a student comes to the Junior Review event with a greater opportunity of successful STEM experiences.
Synergizing CURE results from the literature with the curriculum structure in the Physics Group was an innovation beyond documentation (Corwin et al., 2015; Wooten et al., 2018; Reichart, 2019). With the implementation of a CURE-based laboratory, students were able to receive some partial instruction in all upper-level electives, but it also facilitated further EEL persistence. By implementing a half-credit research/independent study experience that usually precedes a larger, full-credit summer experience, the Physics Group better connects research experiences between the coursework and beyond. These pre-experiences parlay well and create inertia for a student's formative summer EEL opportunities (particularly after their third-year). Such opportunities allow scaffolding of skills and strengthening a knowledge-base through a research experience, which promote a heightened persistence in undergraduates (Estrada et al., 2011). All of these EEL experiences serve to fortify the banks of the program tributary facilitating students to successful STEM trajectories post-graduation.
4.3. STEM identity, flooding the delta
Many of the innovative elements reinforce the development of interpersonal and intrapersonal factors known to affect retention in the STEM disciplines, such as science (STEM) identity and sense of belonging. As science identity is predictive of longer-term persistence in STEM-related fields (Eren, 2021), and sense of non-belonging is cited as a reason for “leaving” the sciences (especially for women, Lewis et al., 2017), these aims are essential to the presented study and well-supported in the literature. Specifically, Estrada et al. (2018) note that completing multiple semesters of research and/or internship within STEM correlates strongly with establishing identity within STEM. Related, Findley-Van Nostrand and Pollenz (2017) demonstrate that participation in an intensive week-long co-curricular program just as students enter college, including engaging with peers with similar interests in addition to several elements related to reflecting on goals and career aims and getting connected to faculty, is related to increased science identity and sense of belonging. In addition, increases in science identity seem to be driven by increases in belonging (to STEM and institutional community, Kuchynka et al., 2019), suggesting that efforts such as providing greater connection to faculty and peers from early on in the program facilitate such development. As research suggests, formation of identity is iterative as students grow in confidence through repeated interaction with content, problem-solving, and experimental techniques (Keagan, 2018). It would be unsurprising if women were better established as shareholders and valued members, where the numbers of women graduates increased.
To substantiate a distributaries analogy within the Physics Group, measures in Figure 4 seem to reduce attrition at a high school to undergraduate transition, since women are retained in the major at higher rates than men. Specifically, if a high school student shows high aptitude and interest in physics (so-called, “Exceptional Physics Girls,” Archer et al., 2016), then she is retained at rates above national averages in the Physics Group. The result in Figure 4 accord with an attempt to keep the primary tributary as broad as possible, early within the undergraduate experience.
Similarly, if a woman receives her bachelor's degree in the Physics Group, then her STEM identity seems more solidified as she moves into a STEM advancement position (Figure 6). When examining the approximate percentages shown in Figure 6, it is clear that a greater number of women in STEM advancement occupy the non-PHYS GS categories. That is, fewer women are retained to become (traditional) physicists than not. However, the Physics Group still considers this successful, since the goal is to flood the delta. A “flooded delta” represents scenarios where there is a greater likelihood that young women (K-12 students) will see someone like them in STEM-advancement positions. Without becoming elitist, the Physics Group believes that “hidden physicists” critically participate in other non-physics, STEM fields, because physics provides a unique way of knowing (Marder, 2013). These unique pathways serve to diversify the face of science by increasing the number of distributaries for physics bachelor recipients.
Data availability statement
Publicly available datasets were analyzed in this study. This data can be found here: Roanoke College Institutional Research Office.
Author contributions
RB and MF contributed to conception, implementation of programmatic innovation, constructed the histograms, organized, and collected the pertinent data. MF wrote most of the manuscript's first draft, where DF-VN provided significant contribution. DF-VN contributed to the construction of STEM identity and belonging framework. All authors contributed to the article and approved the submitted version.
Funding
MF thanks the Roanoke College sabbatical program for its generosity in reassign-time. MF also thanks the Margaret Duke Endowment at the University of Mary Washington from which reassign-time was provided.
Acknowledgments
All authors appreciate the Office of Institutional Research and the Registrar at Roanoke College for their gracious cooperation.
Conflict of interest
The authors declare that the research was conducted in the absence of any commercial or financial relationships that could be construed as a potential conflict of interest.
Publisher's note
All claims expressed in this article are solely those of the authors and do not necessarily represent those of their affiliated organizations, or those of the publisher, the editors and the reviewers. Any product that may be evaluated in this article, or claim that may be made by its manufacturer, is not guaranteed or endorsed by the publisher.
References
AAPT/APS. (2021). Effective Practices for Physics Programs (EP3). Available onliine at: https://ep3guide.org/ (accessed November 17, 2021).
Abbott, P., and Sapsford, R. (2005). Living on the margins: older people, place and social exclusion. Policy Stud. 26, 29–46. doi: 10.1080/01442870500041660
AIP. (2021). Statistical Research Center (SRC). Available online at: https://www.aip.org/statistics (accessed November 17, 2021).
Alon, U. (2009). How to choose a good scientific problem. Mol. Cell 35, 726–728. doi: 10.1016/j.molcel.2009.09.013
APS. (2020). Bachelor's Degrees in Physics and STEM. Available online at: https://www.aps.org/programs/education/statistics/bachelors.cfm(accessed November 18, 2021).
Archer, L., Moote, J., Francis, B., DeWitt, J., and Yeomans, L. (2016). The “exceptional” physics girl: a sociological analysis of multimethod data from young women aged 10–16 to explore gendered patterns of post-16 participation. Am. Educ. Res. J. 54, 88–126. doi: 10.3102/0002831216678379
Auchincloss, L., Laursen, S., Branchaw, J., Eagan, K., Graham, M., Hanauer, D., et al. (2014). Assessment of course-based undergraduate research experi- ences: a meeting report. CBE Life Sci. Educ. 13, 29–40. doi: 10.1187/cbe.14-01-0004
Blickenstaff, J. C. (2005). Women and science careers: leaky pipeline or gender filter? Gend. Educ. 17, 369–386. doi: 10.1080/09540250500145072
Cannady, M. A., Greenwald, E., and Harris, K. N. (2014). Problematizing the STEM pipelinemetaphor: is the STEM pipeline metaphor serving our studentsand the STEM workforce? Sci. Educ. 98, 443–460. doi: 10.1002/sce.21108
Corwin, L. A., Graham, M. J., and Dolan, E. L. (2015). Modeling course-based undergraduate research experiences: an agenda for future research and evaluation. CBE Life Sci. Educ. 14, es1. doi: 10.1187/cbe.14-10-0167
Denice, P. (2021). Choosing and changing course: postsecondary students and the process of selecting a major field of study. Sociol. Perspect. 64, 82–108. doi: 10.1177/073112142091903
Diekman, A., Steinberg, M., Brown, E., Belanger, A., and Clark, E. (2017). A goal congruity model of role entry, engagement, and exit: understanding communal goal processes in stem gender gaps. Pers. Soc. Psychol. Rev. 21, 142–175. doi: 10.1177/1088868316642141
Dunne, E., and Zandstra, R. (2011). Students as Change Agents – New Ways of Engaging with Learning and Teaching in Higher Education, 1 Edn. Bristol, UK: University of Exeter/ESCalate/HE Academy.
Eren, E. (2021). Exploring science identity development of women in physics and physical sciences in higher education. Sci. Educ. 30, 1131–1158. doi: 10.1007/s11191-021-00220-3
Estrada, M., Hernandez, P. R., and Schultz, P. W. (2018). A longitudinal study of how quality mentorship and research experience integrate underrepresented minorities into stem careers. CBE Life Sci. Educ. 17, ar9. doi: 10.1187/cbe.17-04-0066
Estrada, M., Woodcock, A., Hernandez, P. R., and Schultz, P. W. (2011). Toward a model of social influence that explains minority student integration into the scientific community. J. Educ. Psychol. 103, 206–222. doi: 10.1037/a0020743
Findley-Van Nostrand, D., and Pollenz, R. (2017). Evaluating psychosocial mechanisms underlying stem persistence in undergraduates: evidence of impact from a six-day pre–college engagement stem academy program. CBE Life Sci. Educ. 16, ar36. doi: 10.1187/cbe.16-10-0294
Fleenor, M. C. (2018). Cultivating experimental innovation within undergraduate physics majors. Glob. Educ. Rev. 5, 73–87. Available online at: https://ger.mercy.edu/index.php/ger/article/view/419
Fleenor, M. C. (2021). Grounded mentoring as a pathway for program-building. Chron. Mentor. Coach. 5, 421–428. Available online at: https://www.mentor-cmc.com/cmc/cmc2021/MobilePagedReplica.action?pm=2&folio=420#pg420
Francis, B., Archer, L., Moote, J., DeWitt, J., MacLeod, E., and Yeomans, L. (2017). The construction of physics as a quintessentially masculine subject: young people's perceptions of gender issues in access to physics. Sex Roles 76, 156–174. doi: 10.1007/s11199-016-0669-z
Gisler, S., Kato, A. E., Lee, S., and Leung, D. (2018). One size does not fit all: gender inequity in STEM varies between subfields. Indust. Organ. Psychol. 11, 314–318. doi: 10.1017/iop.2018.21
Good, C., Aronson, J., and Harder, J. (2008). Problems in the pipeline: stereotype threat and women's achievement in high-level math courses. J. Appl. Dev. Psychol. 29, 17–28. doi: 10.1016/J.APPDEV.2007.10.004
Good, C., Rattan, A., and Dweck, C. (2012). Why do women opt out? Sense of belonging and women's representation in mathematics. J. Pers. Soc. Psychol. 102, 700–717. doi: 10.1037/a0026659
Gowar, C. (2013). Inclusive Communities: A Research Report. Technical report, Disability Rights UK. Available online at: https://www.disabilityrightsuk.org/sites/default/files/pdf/3.%20InclusiveCommunitiesResearch.pdf (accessed November 22, 2021).
Grogan, K. E. (2018). How the entire scientific community can confront gender bias in the workplace. Nat. Ecol. Evol. 3, 3-6. doi: 10.1038/s41559-018-0747-4
Henderson, C., Cole, R., Froyd, J., Friedrichsen, D., Khatri, R., and Stanford, C. (2015). Designing Educational Innovations for Sustained Adoption: A How-to-Guide for Education Developers Who Ant to Increase the Impact of Their Work, 1st Edn. Kalamazoo, MI: Increase the Impact.
Heron, P., and McNeil, L. (2016). Phys21: Preparing Physics Students for 21st-Century Careers (J-TUPP), 1st Edn. College Park, MD: The American Physical Society (APS).
Hilborn, R. C., Howes, R. H., and Krane, K. S. (2003). Strategic Programs for Innovations in Undergraduate Physics: Project Report (SPIN-UP), 1st Edn. College Park, MD: The American Association of Physics Teachers (AAPT).
Hill, C., Corbett, C., and St. Rose, A. (2010). Why So Few? Women in Science, Technology, Engineering, and Mathematics. Washington, DC: American Association of University Women.
Hinko, K. A., Madigan, P., Miller, E., and Finkelstein, N. D. (2016). Characterizing pedagogical practices of university physics students in informal learning environments. Phys. Rev. Phys. Educ. Res. 12, 010111. doi: 10.1103/PhysRevPhysEducRes.12.010111
Hinton, A. O. Jr., Termini, C. M., Spencer, E. C., Rutanganira, F. U. N., Cherry, D., Roby, R., et al. (2020). Patching the leaks: revitalizing and reimagining the STEM pipeline. Cell 183, 568–575. doi: 10.1016/j.cell.2020.09.029
Hunt, J. (2013). An alternate view: is industry really a “non-traditional” career? APS Forum on Industrial and Applied Physics Newsletter.
Ivie, R., and Tesfaye, C. L. (2012). Women in physics: a tale of limits. Phys. Today 65, 47–50. doi: 10.1063/PT.3.1439
James, M., and Bertschinger, E. (2020). The Time Is Now: Systemic Changes to Increase African Americans wiht Bachelor's Degrees in Physics and Astronomy (TEAM-UP), 1st Edn. College Park, MD: The American Institute of Physics (AIP).
Johnson, T. A. (2021). APS releases new report: building America's STEM workforce. APS News 30, 1–4. Available online at: https://www.aps.org/publications/apsnews/202111/congressional.cfm
Kalender, Z. Y., Marshman, E., Schunn, C. D., Nokes-Malach, T. J., and Singh, C. (2020). Damage caused by women's lower self-efficacy on physics learning. Phys. Rev. Phys. Educ. Res. 16, 010118. doi: 10.1103/PhysRevPhysEducRes.16.010118
Keagan, R. (2018). “What “form” transforms? a constructive-developmental approach to transformative learning,” in Contemporary Theories of Learning: Learning Theorists …In Their Own Words, 2nd Edn., ed K. Illeris (New York, NY: Routledge), 35–52.
Kuchynka, S., Findley-Van Nostrand, D., and Pollenz, R. (2019). Evaluating psychosocial mechanisms underlying stem persistence in undergraduates: scalability and longitudinal analysis of three cohorts from a six-day pre–college engagement stem academy program. CBE Life Sci. Educ. 18, 1–13. doi: 10.1187/cbe.19-01-0028
Lewis, K. L., Stout, J. G., Finkelstein, N. D., Pollock, S. J., Miyake, A., Cohen, G. L., et al. (2017). Fitting in to move forward: belonging, gender, and persistence in the physical sciences, technology, engineering, and mathematics (pSTEM). Psychol. Women Quart. 41, 420–436. doi: 10.1177/0361684317720186
Liu, S.-N. C., Brown, S. E. V., and Sabat, I. E. (2019). Patching the “leaky pipeline”: interventions for women of color faculty in STEM academia. Arch. Sci. Psychol. 7, 32–39. doi: 10.1037/arc0000062
Marder, M. (2013). A problem with STEM. CBE Life Sci. Educ. 12, 148–150. doi: 10.1187/cbe.12-12-0209
Metcalf, H. (2010). Stuck in the pipeline: a critical review of STEM workforce literature. Interact. UCLA J. Educ. Inform. Stud. 6, 1–21. doi: 10.5070/D462000681
Miller, D. I. and Wai, J. (2015). The bachelor's to Ph.D. stem pipeline no longer leaks more women than men: a 30-year analysis. Front. Psychol. 6:37. doi: 10.3389/fpsyg.2015.00037
Mordacq, J. C., Drane, D. L., Swarat, S. L., and Lo, S. M. (2017). Development of course-based undergraduate research experiences using a design-based approach. J. Coll. Sci. Teach. 46, 64–75. Available online at: http://www.jstor.org/stable/44579916
Nash, B. J. (2012). Journey to work: European model combines education with vocation. Fourth Quart. 16, 17–19, Available online at: https://fraser.stlouisfed.org/title/3941/item/476957
National Institute of Food Agriculture. (2014). Addressing Challenges in Education. Technical report, National Institute of Food Agriculture. Available online at: https://nifa.usda.gov/sites/default/files/resource/ed_facts_8_8_fnl.pdf
Nelson, D. J., and Brammer, C. N. (2010). A National Analysis of Minorities in Science and Engineering Faculties at Research Universities. Technical report. Available online at: http://drdonnajnelson.oucreate.com//diversity/Faculty_Tables_FY07/07Report.pdf (November 25, 2021).
Rainey, K., Dancy, M., Mickelson, R., Stearns, E., and Moller, S. (2018). Race and gender differences in how sense of belonging influences decisions to major in STEM. Int. J. STEM Educ. 5, 14. doi: 10.1186/s40594-018-0115-6
Reichart, D. (2019). Exploring the Impact of Robotic Telescope-Based Observing Experiences on Students' Learning and Engagement in STEM. Technical Report, National Science Foundation, USA. https://www.nsf.gov/awardsearch/showAward?AWD_ID=2013300&HistoricalAwards=false
Reinholz, D. L., Matz, R. L., Cole, R., and Apkarian, N. (2019). STEM is not a monolith: a preliminary analysis of variations in STEM disciplinary cultures and implications for change. CBE Life Sci. Educ. 18, mr4. doi: 10.1187/cbe.19-02-0038
Rethman, C., Perry, J., Donaldson, J. P., Choi, D., and Erukhimova, T. (2021). Impact of informal physics programs on university student development: creating a physicist. Phys. Rev. Phys. Educ. Res. 17, 020110. doi: 10.1103/PhysRevPhysEducRes.17.020110
Roy, M.-F., Guillope, C., Cesa, M., Ivie, R., White, S., Mihaljevic, H., et al. (2020). A Global Approach to the Gender Gap in Mathematical, Computing, and Natural Sciences: How to Measure It, How to Reduce It?, 1st Edn. Geneva: Zenodo.
Sheltzer, J. M., and Smith, J. C. (2014). Elite male faculty in the life sciences employfewer women. Proc. Natl. Acad. Sci. U.S.A. 111, 10107–10112. doi: 10.1073/pnas.1403334111
Smyth, F., and Nosek, B. (2015). On the gender–science stereotypes held by scientists: explicit accord with gender-ratios, implicit accord with scientific identity. Front. Psychol. 6:415. doi: 10.3389/fpsyg.2015.00415
Stewart, J., Oliver III, W., and Stewart, G. (2013). Revitalizing an undergraduate physics program: a case study of the University of Arkansas. Am. J. Phys. 81, 943–950. doi: 10.1119/1.4825039
Taylor, J. (1997). An Introduction to Error Analysis, 2nd Edn. Sausalito, CA: University Science Books.
Tesfaye, C. L., and Mulvey, P. (2013). MCAT, LSAT and Physics Bachelor's. Technical report, AIP. Available online at: https://www.aip.org/statistics/reports/mcat-lsat-and-physics-bachelors
The Advanced Laboratory Physics Association, ALPhA. (2021). Alpha Homepage. Available online at: https://advlab.org/ (November 22, 2021).
Trujillo, G., and Tanner, K. (2014). Considering the role of affect in learning: monitoring students' self-efficacy, sense of belonging, and science identity. CBE Life Sci. Educ. 13, 6–15. doi: 10.1187/cbe.13-12-0241
Tyler, J., and Mulvey, P. (2022). How Well do Physics Bachelor's Degree Recipients Perform on the MCAT and LSAT Exams? Technical Report, AIP. Available online at: https://www.aip.org/statistics/reports/how-well-do-physics-bachelor%E2%80%99s-degree-recipients-perform-mcat-and-lsat-2022
Tyler, J., Mulvey, P., and Nicholson, S. (2020). Size of Undergraduate Physics and Astronomy Programs: Results from the Enrollments and Degrees and Academic Workforce Surveys. Technical report, AIP. Available online at: https://files.eric.ed.gov/fulltext/ED607301.pdf (November 15, 2021).
UNESCO Institute for Statistics. (2019). Women in Science. Available online at: http://uis.unesco.org/en/topic/women-science (accessed January 26, 2023).
United Nations. (2023). United Nations Sustainable Development Goals. Available online at: http://www.un.org/sustainabledevelopment (accessed January 26, 2023).
US Department of Education. National Center for Education Statistics. (2019). Indicator 26: STEM Degrees. Available online at: https://nces.ed.gov/programs/raceindicators/indicator/reg.asp (accessed November 16 2021).
Wiswall, M.J., and Zafar, B. (2021). New approaches to understanding choice of major. NBER Report. 2, 18–21. Available online at: https://www.nber.org/reporter/2021number2/new-approaches-understanding-choice-major
Witteveen, D. and Attewell, P. (2020). The STEM grading penalty: an alternative to the “leaky pipeline” hypothesis. Sci. Educ. 104, 714–735. doi: 10.1002/sce.21580
Wooten, M., Coble, K., Puckett, A., and Rector, T. (2018). Investigating introductory astronomy students' perceived impacts from participation in course-based undergraduate research experiences. PRPER 14, 010151. doi: 10.1103/PhysRevPhysEducRes.14.010151
World Economic Forum. (2019). The Global Gender Gap Index 2020. Technical report, World Economic Forum. Available online at: http://reports.weforum.org/global-gender-gap-report-2020/the-global-gender-gap-index-2020/
Keywords: physics undergraduate education, experiential learning, women retention, STEM identity, STEM belonging
Citation: Balasubramanian R, Findley-Van Nostrand D and Fleenor MC (2023) Programmatic innovations that accord with the retention of women in STEM careers. Front. Educ. 8:1018241. doi: 10.3389/feduc.2023.1018241
Received: 12 August 2022; Accepted: 23 February 2023;
Published: 13 March 2023.
Edited by:
Lisbet Rønningsbakk, UiT The Arctic University of Norway, NorwayReviewed by:
Silvina Ponce Dawson, University of Buenos Aires, ArgentinaSusan Howitt, Australian National University, Australia
Copyright © 2023 Balasubramanian, Findley-Van Nostrand and Fleenor. This is an open-access article distributed under the terms of the Creative Commons Attribution License (CC BY). The use, distribution or reproduction in other forums is permitted, provided the original author(s) and the copyright owner(s) are credited and that the original publication in this journal is cited, in accordance with accepted academic practice. No use, distribution or reproduction is permitted which does not comply with these terms.
*Correspondence: Matthew C. Fleenor, bWZsZWVub3JAdW13LmVkdQ==