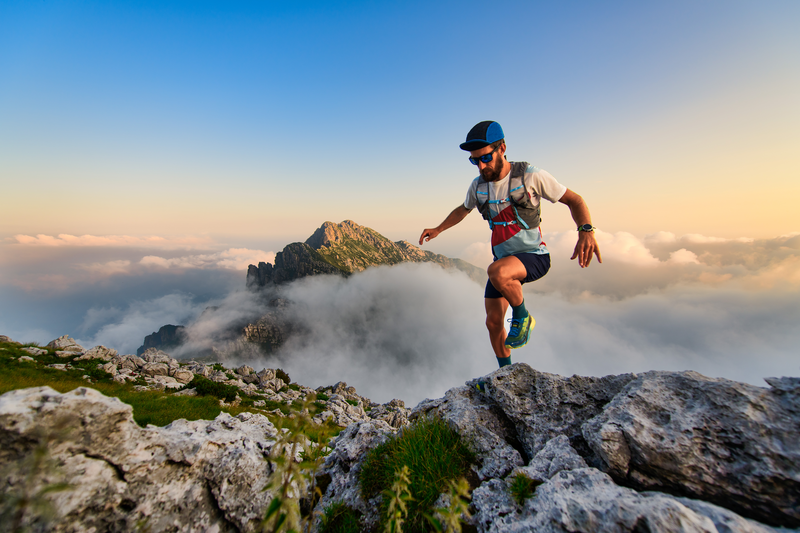
94% of researchers rate our articles as excellent or good
Learn more about the work of our research integrity team to safeguard the quality of each article we publish.
Find out more
ORIGINAL RESEARCH article
Front. Educ. , 12 December 2022
Sec. STEM Education
Volume 7 - 2022 | https://doi.org/10.3389/feduc.2022.982035
This article is part of the Research Topic Current Perspectives on the Value, Teaching, Learning, and Assessment of Design in STEM Education View all 12 articles
Introduction: This study explored the impact of a community of inquiry on Science, Design and Technology and Mathematics curriculum competencies of 159 Year six students as they carried out a design task.
Methods: A quasi-experimental design was employed with both qualitative and quantitative analyses. A community of inquiry approach was adopted by the teachers (n = 3) in the experimental group but not by teachers (n = 4) in the comparison group. Both groups participated in a learning sequence on electricity culminating in a design challenge in small collaborative groups.
Results: The results showed that the experimental group (n = 65) demonstrated significantly greater instances and a broader range of Science, Design and Technology and Mathematics competencies across the design task as well as significantly higher learning gains than their comparison group (n = 94) peers.
Discussion: The cognitive shifts towards higher competency development in the experimental group is stronger as a result of the reflection and reasoning required to engage in a community of inquiry.
The introduction of design and technology to the Australian curriculum for primary and secondary schools signals the recent focus on new pedagogies to address this age of technological advancement (Kimbell and Perry, 2001) and to address calls for a stronger focus on science, technology, engineering and mathematics or STEM education (The Australian Industry Group, 2015). The addition of this subject area is also mindful of the argument put forward by several scholars (Bereiter and Scardmalia, 2006; Collins and Halverson, 2010; Brown et al., 2011) that greater emphasis in education on design is required in order to emphasize creating as a way of knowing given “design epistemology is concerned with generating useful, practical ideas to resolve existing real-world problems” (Koh et al., 2015, p. 9).
With increasing complexity of global issues and the world of work, education systems are trying to equip students with 21st century skills that include thinking critically and creatively, solving complex problems, making evidence-based decisions, and working collaboratively (Weldon; 2017). We are currently experiencing what has been termed “the skills movement” (Care et al., 2016). Since the beginning of the 21st century, curriculum documents have increasingly focused on the changing skills required for employment. There is rising interest amongst education authorities in these skills, with UNESCO reporting that “almost 90 countries… refer to generic competencies in their general education curricula” (Tedesco et al., 2013, p. 11). As recently as 2017 it was shown that specific skills are advocated within national policy documents in 117 countries (Roth et al., 2017).
Given this period of focus of the school curriculum on skills and competencies, it is important to understand the distinction. Skills are “doing or acting in practice, involving motor skills as well as cognitive skills” (Baartman and de Bruijn, 2011, p. 127). Competence or capability is generally understood to comprise sets of knowledge, skills and attitudes and the capacity to use them (Baartman and de Bruijn, 2011, p. 126). While a disposition is having knowledge and skills and a sense of when to use them.
Curriculum subject areas are often represented as competency frameworks (Yates, 2017). And as is the case for the Australian Curriculum, competency frameworks often include 21st century skills as a subset of the competencies students are expected to achieve. In a review conducted for the New South Wales Department of Education, Lamb et al. (2017) question whether these skills and competencies have found their way into teaching and learning in Australian schools and whether they can be taught or assessed. This study addresses these questions with a focus on the competency frameworks specified in Science, Design and Technology and Mathematics (STEM) subject areas of the Australian Curriculum as shown in Figure 1. The design and technology curriculum competency framework is considered to describe technology and engineering competencies. A glance across these competency frameworks shows an emphasis on 21st century and inquiry skills including identifying questions and problems, analyzing, comparing and contrasting information, conducting investigations, interpreting evidence, assessing arguments and drawing conclusions.
Given the emphasis on inquiry skills in these competency frameworks, we hypothesized that an inquiry pedagogy like community of inquiry that engages students in active and dialogic inquiry would improve STEM competencies. There is a need for more intentional and explicit research around the instructional environment and how it contributes to supporting students’ engagement with these competencies through inquiry work. This study also proposed that STEM competencies can be assessed using discourse analysis when students are actively involved in dialogic open-ended design tasks. Tasks such as these make visible student thinking, subject matter knowledge, intellectual skills and dispositions. This analytical approach will be described following a discussion of community of inquiry pedagogy and its impacts on teaching and learning in the classroom.
Community of inquiry (CoI) is a dialogic inquiry pedagogy for the implementation of philosophy in the primary and secondary school classroom. It engages students in deep thinking through collaborative dialogue about critical concepts with the main objective of improving reasoning abilities (Reznitskya and Gregory, 2013). The pedagogical practice known as community of inquiry has distinctive philosophical underpinnings. It was framed by Charles Sanders Peirce and based on his conception of communities of discipline-based inquiry involved in knowledge construction. The community of inquiry (CoI) pedagogy was developed by Matthew Lipman as part of his Philosophy for Children program to guide classroom discussion through the introduction of philosophy into the school curriculum (Lipman, 1991). It is espoused to “develop students’ ability to think for themselves through the internalization of social practices which in turn develops their social and intellectual dispositions and capacities for active citizenship” (Burgh and Nichols, 2011). The CoI method has the potential to foster capacities to become acquainted with the conventions of disciplines through active participation in the practices of discipline-based communities of inquiry where not only disciplinary competence can be developed but also habits of self-correction. These habits then allow the reconstruction of those conventions when confronted with new problematic situations. These theoretical suppositions are empirically explored in this paper.
Matthew Lipman believed that children were capable of critical, creative and caring thinking (Lipman, 2002) and that these forms of thinking were mutually reinforcing. This is an important point of distinction to other dialogic inquiry pedagogical approaches discussed in the literature such as Collaborative Reasoning (Waggoner et al., 1995), Accountable Talk (Wolf et al., 2006) and Thinking Together (Dawes et al., 2003). CoI is the only approach that espouses the essential need to focus on caring thinking in order to solicit, stimulate and engage critical and creative thinking.
Lipman developed this dialogic inquiry educational approach to allow students to philosophize, problematize, and psychologize the curriculum. He coined the term educational philosophy to mean
“a discipline that promotes thinking in other disciplines. It is philosophy that has been developed for practical use in the classroom to get students to think in other subject areas by employing concepts, ideas and reasoning skills; skills borrowed from philosophy in order to facilitate thinking about the subject matter under examination” (Lipman, 2008, p 152).
Lipman’s program is founded on the tenets of pragmatism espoused by John Dewey and Charles Sanders Peirce. Pragmatism holds to the notion that “knowledge is the product of inquiry, that knowing is not merely the acquisition of facts external to the knower, but comes through a problem-solving exercise that moves from doubt to belief on the basis of evidence and inference” (Millett and Tapper, 2012, p. 3).
CoI has three critical elements. It is collaborative with a built-in social dimension involving communal dialogue with the requirement that students listen carefully and respond respectfully to the ideas of others. It is philosophical in that students clarify concepts, explore meanings and work towards a shared understanding approaching knowledge with an understanding that it is fallible. The third element is inquiry where students engage with open-ended or divergent questions and intelligent agreement and disagreement through a community of inquiry process. CoI is a practice that brings together collaboration and inquiry using a philosophical approach. It permits students to engage in a disciplinary inquiry where they are able to “problematize or transform commonly accepted facts into problems to be explored, thereby opening knowledge to thinking” (Lefstein, 2010, p. 176).
The CoI process is initiated by presenting students with a problematic situation within the context of the subject under study that engages them in thinking about what is unclear or in conceptualizing the problem. Based on what they find problematic, students generate a list of questions – that guide the conceptualization of the problem. A central question is chosen and students then offer their opinions, explore ideas, state conjectures and generate hypotheses in order to seek solutions or explanations. Finally, students engage in analysis, reasoning and argumentation to achieve a deeper understanding or conceptualization of the problem into which they are inquiring.
Making thinking an explicit focus in science lessons requires pedagogical strategies that provide students with opportunities to engage in communal dialogue about how science and technology impacts society. Sprod (2014) argues that pedagogies that make thinking more visible, like CoI, are not widely implemented at a system level. However, there is good evidence for such an approach impacting on students’ learning and cognitive abilities. Research on CoI has shown that teachers use significantly more open-ended questions when trained (Trickey and Topping, 2004, 2006; Baumfield, 2016; Nichols et al., 2017). Students that engage in CoI show improved cognitive abilities to conceptualize problems, sustained for several years, increased student–student dialogue characterized by prolonged length of student utterances/elaborations (rather than increased total student utterances), enhanced student reasoning and justification skills (Topping and Trickey, 2014) and significantly higher student substantive questioning and other inquiry behaviors (Nichols et al., 2017).
Given these impacts of CoI and the problem conceptualization students’ encounter through CoI, we asked the following research question. What is the effect of embedding the CoI process in inquiry science curriculum on the development of the Australian Curriculum’s identified STEM competencies demonstrated during a design problem solving task? Although studies have shown improvements on psychometric measures of cognition, reasoning and argumentation as a result of participating in dialogic inquiry or CoI (Reznitskya et al., 2012; Topping and Trickey, 2014), these measurements have limitations. They were not designed for exploring subject-specific skills and competencies and so lack the appropriate subject-specific context. Performance-based subject-specific dialogic assessment in the form of an authentic open-ended task is more appropriate for exploring gains in STEM-specific competencies. As Chinn et al. (2011) argue, to gain a more contextual understanding of diverse aspects of students’ epistemological development, studies need to utilise more situated and refined measures. De Liddo et al. (2011) have shown that the quality of student discourse in the classroom provides key insights into learning and skill development. Drawing on Niel Mercer’s socio-cultural discourse analysis and argumentation theory they identified patterns of activity in students’ discourse that corresponded to learning processes and knowledge construction. They also refer to the use of discourse to relate learning outcomes to learning processes as discourse-centric learning analytics. This study employed sociocultural discourse analysis and discourse learning analytics to explore the relationship of CoI and/or a 5E’s inquiry learning sequence, learning outcomes and visible STEM competencies.
This study, that was part of a larger project (Nichols et al., 2022), employed an intervention approach. Teachers attended a two-day workshop on implementation of a researcher-designed Year 6 Science inquiry learning sequence on energy that aligned with the Australian science curriculum. The learning sequence culminated with assessment in the form of an inquiry design problem solving task that required students to engage with Science, Design and Technology and Mathematics subject area competencies. We modelled how to open the learning seqeunce through a CoI with an approach to problematizing the topic under study that would be revisited by teachers and students throughout the learning seqeunce.
Participants included 159 Year six primary school students and seven teachers across five schools with similar socio-demographic profiles (full range of school size was from 600 to 800 students, with an age range of 10 to 12 years, 45–60% of students were female and 40–55% were male) in municipal and regional areas of Brisbane, Australia. Teacher participants included six females and one male that ranged in years of teaching experience from less than 1 year to 12 years with two first year graduates, three who had worked for six to 8 years and two that worked anywhere from eight to 12 years. There were 87 female students and 72 male students. Ethical clearance was acquired for this study and participants were recruited through several inclusion criteria such as.
1. The teachers were intending to teach Year six in the year of the study.
2. The teachers consented to participate in professional learning around implementation of a Year six curriculum learning sequence on electrical energy.
3. Both teachers and students were fully informed about and consented to participating in classroom observations, tests and interviews.
A before and after convergent mixed methods approach (Cresswell, 2012) was utilized with all teacher participants participating in 2 days of professional learning around CoI and the implementation of an inquiry science learning sequence (Figure 2) designed with the 5E’s (Figure 3; Bybee, 2014). However, while implementing the intervention in their classrooms, four of the teachers in the study chose not to engage their students in CoI as they perceived there was a sufficient inquiry emphasis with the learning sequence design. All other aspects of the intervention were conducted in their classrooms, the only difference was that they did not start the learning sequence off with a CoI, they started with where electricity comes from activity (see Figure 2 for the intervention activities). As a result, the study design became a two-by-two pre-post intervention and comparison approach. This inherent shift in study design due to participant decisions around implementation has been validated in the literature (Topping and Trickey, 2014). The CoI group consisted of three teachers and 65 students from two schools and the Non-CoI group comprised four teachers and 94 students from three schools.
The intervention activities are outlined in Figure 2. During the professional learning all teachers were introduced to embedding a CoI approach in a learning sequence of work on electricity and energy that culminated in a design task. As part of the professional development around the CoI pedagogical process, a collaborative community of inquiry on human rights for access to electricity was a way to problematize the learning sequence content. The CoI was modelled for the teachers by showing two stimuli (a creative commons image of a classroom in a developing country without electricity and a short video that provided some detail about where electricity comes from and what happens in a blackout). Dialogue was facilitated by exploring the question “Is electricity a basic human right?” Teachers were encouraged to justify their perspectives. An additional question based on the opening question being a plausible truth was posed; “If electricity is a basic human right, then why are so many people disadvantaged?” In order to gauge depth of understanding, foster deeper thinking about and problematize the learning sequence topic, additional substantive questions were explored around considering if electricity is a need or a want, thinking about the implications of having no electricity, the ethical implications of the prohibitive expense of electricity, accessibility of renewable energy, where the responsibility lies in using energy more efficiently and what would happen if no one took responsibility.
The process focused on building a culture of respect through a collaborative shared dialogue concerned with providing examples and counterexamples, seeking clarification, reason giving, making distinctions and intellectual progress through thinking, reasoning and conceptual analysis. A deliberate goal of the discussion was to test generalizations and uncover assumptions. The process was concluded with reflection considering how the teachers perceived their contributions and engagement in the community, respect and the value of thinking about others.
This collaborative inquiry process encourages the development of ethical perception by fostering the ability to discern any situation’s critical features and relationships and then utilize these to make judgements (Sharp, 2017). Students develop concern for the implications of motives, judgements, actions for others and for themselves. In identifying these implications, students must imagine the varied permutations that are feasible and in so doing position themselves critically and creatively within a context consciously relating to its diverse aspects. Students learn to make judgements within a set of circumstances and from particular positions. In this way, the concern or caring thinking that is activated within a CoI is inextricably linked to, and fuels critical and creative thinking.
The learning sequence: The intervention aimed to endow teachers with physical resources and skills to deliver a Year six learning sequence of work around electricity and energy. The learning sequence activities were designed to employ cooperative learning approaches and the 5E’s inquiry model (Bybee, 2014). This instructional model is underpinned by a constructivist learning theory with the notion that conceptual understanding of science concepts and their meaning are constructed through experiences. These experiences occur in a learning cycle over a number of phases known as Engage, Explore, Explain, Elaborate and Evaluate. Figure 3 provides a description of the 5E’s phases that were used to plan the learning sequence. The learning sequence included an emphasis on design tasks including the building of switches, exploring lemon batteries and constructing circuits to identify energy transformations. This prepared students to complete the final assessment task where cooperative groups were challenged to design and construct a device that would provide electricity to a third world community using recycled materials provided to them.
This study focuses exclusively on the design phase where students worked in small groups to brainstorm the main features of their device, exploring what their generator would require. Groups comprised four students provided with specific roles. The roles included a project manager to oversee the group work, a drafts person to draw sketches, a photographer digitally capture the final design sketch, and a materials manager to encourage the group to consider and discuss the materials that would be used for construction of the device. While students each had a role to perform they were encouraged to also provide ideas for the design.
Figure 4 lists and describes the data corpus for the study and the associated analyses that were conducted. A test was provided to students prior to and immediately after the learning sequence. The test included questions that related to the content of the learning sequence (Figure 5). The total test score was 26. Overall learning gain was calculated for the CoI and Non-CoI groups (see Figure 4; McDaniel et al., 2007). Mann–Whitney U test was used to compare pre-test scores, post-test scores and learning gain across the CoI and Non-CoI groups. A non-parametric statistical test was carried out given the CoI group scores were not normally distributed.
Two small student groups per classroom conducting their power-generating device design were video-recorded for up to 40 min (group task recordings ranged from 15 to 40 min). Video recordings, totaling approximately 240 min, were transcribed and parsed into turns (Johnstone, 2007). A turn was initiated by a student talking and completed by another student talking or when talking ceased. We then identified episodes with verbal demonstrations of STEM competencies shown in Figure 1. Two or more turns of talk initiated by a student with demonstrations of STEM competencies was identified as an episode. When a question had been addressed or a design issue had been resolved or the topic shifted this was identified as the end of an episode. Episodes provided the units of analysis to which the codes for the competencies applied. We applied discourse analytics (De Liddo et al., 2011) where we coded episodes for the competencies. We also applied sociocultural discourse analysis (Mercer, 2004) to summarise the nature of the talk.
A method for analyzing student talk in the classroom that incorporates qualitative and quantitative methods is sociocultural discourse analysis (Mercer, 2004). Neil Mercer describes this analytical approach as analyzing collective thinking in the classroom given that when students work together they not only interact, they interthink. This type of discourse analysis takes a sociocultural perspective on learning where the classroom dialogue (teacher-student and/or student–student interactions) can provide insights into educational success and failure. It is a contextual approach to analyzing the relationship between dialogue processes and outcomes. Mercer’s analysis of student’s talk in groups over time resulted in the development of a typology. He describes three types of student talk based on the degree to which students are showing cooperative or competitive behaviors and whether or not they display reciprocal acceptance of ideas or critical reflection. Disputational talk is characterized by quick exchanges such as assertions and counter assertions or challenges. Decision making tends to be on an individual basis and there is obvious disagreement but there is little attempt to reconcile ideas, offer suggestions or engage in constructive criticism. Cumulative talk is characterized by the construction of common ideas that are built in an uncritical way. This talk is evident when there are repetitions, positive confirmations and elaborations. Exploratory talk is evident when group members are all contributing their ideas, and opinions are clearly considered before the group builds to a particular decision. This form of talk will consist of critical and constructive engagement with shared ideas and suggestions put forward for everyone to consider. Challenges and counter challenges may be evident but they are justified and alternative ideas or hypotheses are provided. Reasoning is more evident in this form of talk and forced accountability of claims is visible. Sociocultural discourse analysis was used in this study to explore and describe the nature of the talk demonstrated by student groups as they worked on their design challenge.
Learning analytics using discourse as a source of the analysis (De Liddo et al., 2011) was used to apply a coding scheme approach for a systematic observation in which utterances were assigned to defined categories and relative frequencies of occurrence of these categories were calculated. The categories used for this study were the STEM competencies defined by the Australian Curriculum competency frameworks for Science, Design and Technology and Mathematics subject areas shown in Table 1. These two approaches to discourse analysis were used to compare learning outcomes and demonstrations of competencies in a group of students that engaged in CoI and their peers that did not engage in CoI.
Table 1. Mann–Whitney U test comparison of median scores and interquartile ranges (in parentheses) for CoI and Non-CoI groups.
In order to standardize across the different timeframes for the CoI and Non-CoI group discussions, percentage of coded lines of transcript were calculated. The proportion of coded lines in the entire transcript of lines was compared between CoI and Non-CoI groups using a Mann–Whitney U test. Inter-rater reliability scores were determined for each of the coded competencies using two researchers across 25 percent of the episodes. Cohen’s kappa calculation (Cohen, 1960) was utilized to compare scores. Where disagreements arose, they were resolved through discussion until agreement reached 81 to 90 percent.
Following completion of the learning sequence, teachers were asked to select a group of 6 to 8 students to participate in a student focus group semi-structured interview. During the focus group discussion, students were asked to reflect on their learning and compare how the completed learning sequence was different from previous learning sequences in science earlier in the year in their school. Audio recordings of focus group discussions totaled approximately 210 min. The responses from groups that had participated in CoI were separated from responses of those groups that did not participate in CoI. Student focus group discussions were used as qualitative evidence to support overall findings.
Table 1 shows that there was no significant difference between the CoI and Non-CoI groups in their pre-test scores indicating that the groups were similar at the start of the learning sequence. However, there was a significant difference in post-test scores and learning gain across the two groups.
Table 2 shows overall the CoI group has a significantly higher proportion of their discussion coded for STEM competencies suggesting that CoI does promote engagement with these competencies and to a greater extent than in the Non-CoI group. This comparison is based on the proportion of coded STEM competencies relative to the total lines of transcript and so normalizes for any differences in the length of the student small group discussions. A finer grained discourse analysis shows how students in the CoI and Non-CoI groups demonstrated and utilized STEM competencies.
Table 2. Comparison of CoI and Non-CoI group proportion of total lines of transcript coded for STEM competencies.
The stacked bar graphs in Figure 6 show coded demonstrations of competencies in the discourse for a CoI and Non-CoI group in three-minute intervals as they worked in their small group on the design of their generator. This data representation reveals that the CoI group engages a broader range of coded STEM competencies then the Non-CoI group. Note also that the CoI group spend 33 min designing their generator while the Non-CoI group discussion is 15 min long. These two groups are representative of other group discussions because these findings were consistent across all CoI and Non-CoI groups in the study. Teachers confirmed that there was nothing procedural they did to influence the time students spent in group discussions conceptualizing their design.
Figure 6. Student discourse STEM competencies in a CoI and Non-CoI group task. T1 = investigating and defining, T2 = generating and designing, T3 = producing and implementing, T4 = evaluating, S1 = science understanding, S2 = science social/ethical, S3 = science inquiry skills, M1 = mathematical understanding.
Figure 6 also shows that the CoI group engages with the Mathematical competency understanding while the Non-CoI group does not engage with this competency. Other notable contrasts between the two groups is the higher relative engagement with the science inquiry and social/ethical competencies as well as the Design and Technology competency evaluation by the CoI group.
If we express the same data from Figure 6 as time trend graphical representations we see more clearly in Figure 7 different patterns of competency recruitment over time and across the discussion between the two groups. The trend line for the Design and Technology competency generating/designing across the discussions of the two groups shows a different approach to the task. The Non-CoI group launch into generating/designing their generator in the first 3-min interval (2 min into the discussion) and engagement with this competency steeply declines across the short discussion. The same trend line in the CoI group shows a different approach to generating/designing that begins in the third 3-min interval (7 min into the discussion), generally declines up to the 15–18 min interval and then steeply increases over the next five 3-min intervals. There is a biphasic nature to the discussion within the CoI group evident through this competency trend line with an overall positive slope compared to a negative slope evident in the Non-CoI group discussion.
Figure 7. Time trends showing STEM competencies in discourse across a CoI and Non-CoI group. T1 = investigating and defining, T2 = generating and designing, T3 = producing and implementing, T4 = evaluating, S1 = science understanding, S2 = science social/ethical, S3 = science inquiry skills, M1 = mathematical understanding.
Engagement with the other competencies across the discussion provides a richer picture of the different approaches to the problem-solving task across the two groups. The Non-CoI group draws on the Design and Technology defining and Science inquiry competencies in the first two 3-min intervals and then in the last three intervals draw on the Design and Technology evaluating and Science inquiry competencies. The shorter discussion time and the lack of engagement with the Science social/ethical, Design and Technology implementation and Mathematical understanding competencies in this group suggests a more rigid approach with procedural tactics applied to the task. The CoI group open their discussion by engaging with Design and Technology defining and evaluating competencies as well as Mathematical understanding competency before they launch consistently into designing. The second surge of designing from 18 to 22 min happens after 7 min of evaluating their initial design phase. The initial period (from 17 to 23 min) of the second design phase shows engagement with many competencies including Mathematical understanding, Science understanding, Science inquiry competencies as well as Design and Technology defining and evaluating competencies. The CoI group finish their discussion by further engaging with Design and Technology defining and implementing as well as Science social/ethical competencies. In other words, the CoI group enter into two designing cycles recruiting a wider range of competencies. This group shows a more flexible and engaged approach to the task.
Vignettes of the CoI and Non-CoI discussions showing how they conceptualize the design of their generators elucidate the contrasts of competencies across the two groups and how they are employed in the discourse. Student names are pseudonyms.
(Example showing instances of mathematical understanding (6–9 min interval)
Josh: That’s a cylinder (M1: Thinking and interpreting information mathematically).
Suraya: Teacher said it’s a semi-circle (M1: Thinking an interpreting information mathematically).
Ali: I call it a long semi-circle because this is what it is meant to be, like that (showing the shape with a curved sheet of paper) (M1: Thinking and interpreting information mathematically, Connecting concepts to develop new ideas).
Suraya: It is long (T2: Developing and communicating ideas).
Ali: Long semi-circle. It is a cylinder fully solid inside of it. (M1: Thinking and interpreting information mathematically, Connecting concepts to develop new ideas).
(18–21 min interval)
Ali: Sticky tape it! Would the sticky tape hold that much sand and pressure? (S1. Applying science knowledge to the situation; M1. Identifying commonalities and differences).
Ellie: To move with paddle pop stick we need something else. Will think about it later, finish the design (T2. Developing and communicating ideas).
Josh: I’ll pour sand here. if I keep pouring over (T2. Considering alternatives).
Ellie: This is the cup. It goes all the way around and tips out and it will stay there (T2. Focusing on high quality design solutions).
Ali: It will keep the force, this will do the same but the cups will do it more efficiently. (M1. Identifying commonalities and differences mathematically, S1. Applies knowledge to new situations).
Suraya: Where is it attached? (S3. Identifying and posing questions).
Ali: This bit is attached from the center. This is the pin. The mill is attached to the pin. And the pin is attached to that. And this is inside the cup, this becomes taped to the wall. So these two will be the only ones spinning. (T2. Communicating design ideas, S3. Exercising scientific thinking).
Suraya: Where does the sand come from? (S3. Identifying and posing questions).
Ali: I do not like this idea about sand. (T1 Critiquing needs and information).
Ellie: I agree, I feel it is confusing.
Ali: Like the sand pours down on the mill. Mill spins and while the mill spins there’s also like a pin that’s behind the mill. This was the mill [showing a ruler], there’s a pin coming behind it. While the mill spins the pin spins with this [turning the ruler]. And because the pin spins this turns the turbine (T2. Developing and communicating ideas, S1 Applying knowledge to new situations).
Suraya: So what if there are no pins? (S3. Identifying and posing questions).
Ali: Then we can use something else and tape it (T2. Documenting design ideas and possibilities, focusing on high-quality designed solutions).
Ellie: How is the sand supposed to run in here go into that? (S3. Identifying and posing questions).
Ali: There’s a pathway where the sand comes, here [S. demonstrates]. (T2. Developing and communicating design ideas).
Ellie: So just say a milk bottle right here (T2. Developing and communicating ideas; Figure 8).
Ali: There’s a pathway, pathway between the mill, because of the force of the sand from the potential energy this will turn (S1. Applying knowledge to new situations, Integrates apt science knowledge).
Ellie: And it becomes kinetic? (S1. Applying knowledge to new situations).
These transcripts provide evidence in the CoI group of Exploratory talk. It begins with Josh, Ali and Suraya disputing the 3D shape of their design and coming to a joint decision with inclusion of all ideas. They build on each other’s ideas throughout the conceptualization of the design features with differing opinions being offered and the ideas are supported by reasoning (for example: There’s a pathway, pathway between the mill, because of the force of the sand from the potential energy this will turn.)
Example showing instances of investigating and defining (0–3 min interval):
Kai Wei: We have to create this. We can paste two plates together (T2: Developing and communicating ideas).
Katie: We can use cups (T2: Making design choices).
Kai Wei: What cups? We have paper plates.
Magda: OK.
Katie: Garbage bags? (T2: Making design choices)
St.2: Egg cartons (T2: Making design choices; Figure 9).
Example showing instances of evaluating (6–9 min interval):
Katie: What about plastic cups cut in half? (T2: Making design choices).
Magda: Use that as a paddle? (T2: Consider design alternatives).
Brad: Yes and use two different paddles. You want to try that? (T2: Developing and communicating design ideas).
Katie: And one is a bit bigger? I do not know how to draw it (T2: Documenting design ideas).
Brad: We will still use a plate though. (T2: Making design choices).
Katie: Yes we will use plates. (T2: Making design choices).
Kai Wei: We will put little slits in a paper plate and use them (T2: Making design choices).
These coded episodes reveal that students in the Non-CoI group tended to show Cumulative talk. Participants contribute ideas and these ideas are accepted without dispute. There are repetitions, confirmations and elaborations and there is evidence of cooperative interaction. But unlike the CoI group, a critical consideration of ideas is lacking. The discourse featured predominantly design and technology competencies with little integration of science or mathematics competencies, while students in the CoI group utilized their science and mathematical competencies to extend their design. In addition, students in the CoI group had longer individual utterances than the Non-CoI group and tended to provide justifications or explanations of their ideas.
Student focus group discussions provided opportunities to reflect on and share their perceptions of their learning experience.
St1: We got to think and design first, like turn the turbine to create electricity because we need some energy or sand or water to power and turn the turbine to generate electricity or to turn on the LED light.
St1: I agree with St2. My knowledge has improved in science because I used to not understand much. But when we started to learn about electricity I started to understand more science … We were not really told much before.
St3: As a group we got to play and look at the generator – we never had one before.
St4: I also agree with these two (St1&St3) that my knowledge has improved because our teacher made us go step by step, using a process. She asked us what it meant and if we did not she went through it again, putting it into a scenario. She told us how the generator moved, and obviously we did not know. She said there are magnets inside the generator and wires wrapped around it, and she explained that. Some of us kind of got a little confused about how it is moving. So she explained it again. So the wind energy, moves the turbine, and it makes the generator turn.
CoI students tended to build on each other’s ideas and to explain the insights they gained using justifications and providing content examples. They were able to reflect and articulate how and what they learned. The FGD confirmed the Exploratory nature of the talk that we observed in the group work. This finding suggests that the skills learned in the CoI are transferred to other situations.
St1: You got to build stuff, in the other ones (science learning sequences) we did not. In this one we got to work with a lemon. We got 2.8 something.
St3: Volts.
St2: We got up to 3 point something. We learned the very first person to make electricity, and it was based on his last name. Volta or something.
St4: We enjoyed the circuits and, using the electro-lab, enjoyed them flying off.
When asked the same questions about the science learning sequence compared to previous ones, Non-CoI group students tended to provide simple short answers that lacked detail. The use of ‘stuff’ and ‘something’ indicates that this group was not able to clearly articulate their learning. The answers are very short compared to the substantial and deeper reflections of the CoI group.
This study explored the notion that science students who engage in a collaborative community of inquiry around the topic they are learning better develop curriculum-defined STEM competencies. The findings of this study where student development of STEM competencies was a focus, show that engaging in CoI across a science learning sequence promotes a disposition or habit or desire to engage in thinking and reasoning through Exploratory talk which more strongly fosters STEM competencies. The CoI group demonstrated a full range of STEM competencies. The Non-CoI group that did not engage in CoI, only tended to show Cumulative talk that unlike the CoI group, lacked a critical consideration of ideas. This group also did not demonstrate a full range of STEM competencies and applied more procedural tactics to complete the task.
Inquiry approaches not only elicit reasoning and help in making judgements (Reznitskya et al., 2012) but also develop creativity. A study by Hathcock et al. (2015) investigated the use of inquiry-based questioning as a means of supporting creativity within an engineering/design STEM activity. The aim was to determine the impact of inquiry question-based scaffolding on the observed product creativity in a design activity. The activity was to build a buoy that would not rest at the bottom or the sides of a tank using provided materials. Findings suggested that student groups facilitated by inquiry-based questioning strategies were better able to solve an ill-structured problem and achieved a more linear progression toward creative products than student groups who were not facilitated by inquiry-based questions. The questions were designed to encourage students to think and talk with their partners and cue them to issues related to their design. The authors concluded using inquiry-based questioning strategies with ill-structured tasks may assist teachers in scaffolding student success in STEM learning, both in terms of solving the task as well as cueing students toward translating their creative ideas into creative products. Our study adds to these findings and showed that engaging in a community of inquiry supports students to engage in deeper and more reasoned discussions about their design and exhibit a broader range of STEM competencies.
In addition to these findings our study showed that students that engaged in CoI demonstrated significantly higher instances of STEM competencies during their design task alongside of significantly higher learning gains. These findings are consistent with a recent study conducted by English et al. (2017). They examined Year six students’ approaches to solving an engineering-based problem on earthquakes which drew on the Australian curriculum in Mathematics, Science and Design and Technology. Six teachers and 136 students from two independent schools and one government school from Queensland, Australia participated in the study. In small groups, students applied their preliminary learning about earthquakes to the design and construction of a building that could withstand earthquake damage. The problem involved the design of three-dimensional models that were constructed, tested, redesigned and further tested in generating final products that met given criteria and constraints. Analyses of group work for Year six students’ engineering process competencies that comprised problem-scoping, idea creation, designing and constructing, assessing design, and redesigning and reconstructing revealed that students showed evidence of STEM disciplinary knowledge through development of these skills. In our study all students showed learning gains but the students that engaged in community of inquiry dialog, demonstrated significantly higher learning gains and a broader range of STEM competencies. This indicates that the thinking skills acquired through community of inquiry enhances both the STEM competency development and disciplinary knowledge. English and colleagues argue that the STEM inquiry, design task supported students to develop engineering habits of mind. We agree with English and colleagues and would argue that while these design tasks promote student engagement with STEM competencies, embedding CoI into the learning sequence significantly enhances these skills through development of habits of mind and dispositions to know when and how to apply reasoning alongside the competencies to create a design solution.
This good thinking that students in the CoI group demonstrated in this study and previous studies (Sprod, 2017) evolves over time and through collaborative, challenging and stimulating learning experiences (Adey et al., 2007). Sprod (2014) argues that “if we have the aim to teach good thinking in science, we need to be aware that the sort of thinking we are seeking to encourage and inculcate is quite a complex set of capabilities and dispositions, backed by sound judgment” (p. 1534). Engaging students in a community of philosophical inquiry that problematizes science content under study, encourages and models rigorous thinking in the discussions and through explicit consideration, the skills and dispositions of scientific thinking are addressed.
In a CoI, as students communally devise, defend, and analyze each other’s perspectives, they adopt capabilities, dispositions and reasoned argumentation linguistic skills, which they can apply to solve complex problems. The dialogic discussions about open-ended questions that relate to and problematize the disciplinary content provides students with content-appropriate experiences, where the tenets of rigorous inquiry, enacted with their peers, become part of their overall cognitive performance. Through dialogic interactions with peers, students hone their skills to engage in reasoned argumentation, as they grapple with new language and thinking practices (Reznitskya and Gregory, 2013).
Thinking within science, design and technology and mathematics draws on both the general thinking capacities and dispositions that apply across all domains as well as more specialized subject thought processes or competencies. In CoI, students are able to engage in deep thinking about science topic-related issues. The cognitive shifts towards higher competency development is stronger as a result of the reflection and reasoning required to engage in CoI. CoI enhances students’ competencies by positioning them in a community that utilizes, reflects on and cares about the epistemic criteria of thinking, problem solving and inquiry.
Students in the CoI group engaged more substantively in thinking and problem solving than their peers in the Non-CoI group. They were better able to articulate what they learned and how they learned. A fundamental part of problem-solving is the cognitive processes involved in conceiving problems. The problem conceptualization phase of problem solving is the period of cognitive processing occurring before the externalization of ideas using sketches, verbal communication or mathematical expression. In this dynamic process there is an active construction of thoughts, ideas and memories that are influenced by thinking, attitudes, emotions, and experiences (Delahunty et al., 2020).
Delahunty et al. (2018, 2020) have shown that providing experiences to support students to understand how to conceptualize a problem has profound benefits in developing problem solving skills in STEM education. Without support, students lack flexibility in problem solving evident in a rigid approach that often results in procedural and surface tactics (McCormick and Davidson, 1996). CoI provides students with content-specific rich experiences that engage them in problem conceptualization that they can recall from memory to apply in new situations. The students in the CoI group engaged deeply and flexibly with conceptualizing the design problem drawing on their CoI experience. The Non-CoI group did not have this prior experience and showed a shallow, procedural engagement with the task.
Finally, the CoI focus group discussions in this study revealed a capacity to reflect on, clearly describe and consider the epistemic benefits of their learning. The Non-CoI group were less able to reflect on their learning and were not able to articulate well what they learned or how they learned the content. The CoI method involves reflection on the process, learning and group interactions and contributions. This promotes the ability of students in the CoI group to reflect on learning and so students can call on their experience to more clearly articulate the content they learned and how they learned it.
It is noted that the findings of the study could be attributed to test effects, maturation or other confounders. The sample size could also impose limitations for making the findings generalizable beyond the contexts, students and participants presented.
The raw data supporting the conclusions of this article will be made available by the authors, without undue reservation.
The study involving human participants was reviewed and approved by The University of Queensland’s Human Research Ethics Committee. Written informed consent to participate in this study was provided by the participants’ legal guardian/next of kin.
KN contributed to conception, design of study, performed statistical analysis, wrote first draft of the manuscript, and approved the submitted version. RM contributed to conception, wrote sections of the manuscript, contributed to manuscript revision, and read and approved submitted version. MH organized the database, contributed to conception and presentation of data. All authors contributed to the article and approved the submitted version.
This research was funded by the Australian Research Council (LP160101374).
The authors declare that the research was conducted in the absence of any commercial or financial relationships that could be construed as a potential conflict of interest.
All claims expressed in this article are solely those of the authors and do not necessarily represent those of their affiliated organizations, or those of the publisher, the editors and the reviewers. Any product that may be evaluated in this article, or claim that may be made by its manufacturer, is not guaranteed or endorsed by the publisher.
Adey, P., Csapó, B., Demetriou, A., Hautamäki, J., and Shayer, M. (2007). Can we be intelligent about intelligence?: Why education needs the concept of plastic general ability. Educ. Res. Rev. 2, 75–97. doi: 10.1016/j.edurev.2007.05.001
Australian Industry Group (ATN) (2015). Innovate and prosper: ensuring Australia’s future competitiveness through university-industry collaboration. Avialable at: https://www.atn.edu.au/siteassets/publications/atninnovateprosper.pdf
Baartman, L. K. J., and de Bruijn, E. (2011). Integrating knowledge, skills and attitudes: Conceptualising learning processes towards vocational competence. Educ. Res. Rev. 6, 125–134. doi: 10.1016/j.edurev.2011.03.001
Baumfield, V. (2016). “Changing minds: the professional learning of teachers in a classroom community of inquiry” in The Routledge international handbook of philosophy for children. eds. M. Gregory, J. Haynes, and K. Murris (Routledge: New York), 119–127.
Bereiter, C., and Scardamalia, M. (2006). Education for the knowledge age: design-centered models of teaching and instruction. Handbook of Educational Psychology 2, 695–713.
Brown, R., Brown, J., Reardon, K., and Merrill, C. (2011). Understanding STEM: current perceptions. Technology and Engineering Teacher 70:5.
Burgh, G., and Nichols, K. (2011). The parallels between philosophical inquiry and scientific inquiry: implications for science education. Educational Philosophy and Theory 44, 1045–1059.
Bybee, R. W. (2014). The BSCS 5E instructional model: personal reflections and contemporary implications. Sci. Child. 51, 10–13. doi: 10.2505/4/sc14_051_08_10
Care, E., Anderson, K., and Kim, H. (2016). Visualising the breadth of skills movement across education systems. Available at: https://www.brookings.edu/research/visualizing-the-breadth-of-skills-movement-across-education-systems/ (Accessed November 25, 2019).
Chinn, C. A., Buckland, L. A., and Samarapungavan, A. L. A. (2011). Expanding the dimensions of epistemic cognition: arguments from philosophy and psychology. Educ. Pscychol. 46, 141–167. doi: 10.1080/00461520.2011.587722
Collins, A., and Halverson, R. (2010). The second educational revolution: rethinking education in the age of technology. Journal of Computer Assisted Learning 26, 18–27.
Cresswell, J. (2012). Educational research: Planning, conducting, and evaluating quantitative and qualitative research. 4th ed. Boston: Pearson.
Dawes, L., Mercer, N., and Wegerif, R. (2003). Thinking together: A programme of activities for developing speaking, listening and thinking skills for children aged 8–11. Birmingham, UK: Imaginative Minds.
Delahunty, T., Seery, N., and Lynch, R. (2018). Exploring the use of electroencephalography to gather objective evidence of cognitive processing during problem solving. J. Sci. Educ. Technol. 27, 114–130. doi: 10.1007/s10956-017-9712-2
Delahunty, T., Seery, N., and Lynch, R. (2020). Exploring problem conceptualization and performance in STEM problem solving contexts. Instr. Sci. 48, 395–425. doi: 10.1007/s11251-020-09515-4
De Liddo, A., Buckingham Shum, S., Quinto, I., Bachler, M., and Cannavacciuolo, L. (2011). “Discourse-centric learning analytics” in Proceedings of the 1st international conference on learning analytics and knowledge. New York, NY: Association for Computing Machinery. 23–33.
English, L. D., King, D., and Smeed, J. (2017). Advancing integrated STEM learning through engineering design: sixth-grade students’ design and construction of earthquake resistant buildings. J. Educ. Res. 110, 255–271. doi: 10.1080/00220671.2016.1264053
Hathcock, S. J., Dickerson, D. L., Eckhoff, A., and Katsioloudis, P. (2015). Scaffolding for creative product possibilities in a design-based STEM activity. Res. Sci. Educ. 45, 727–748. doi: 10.1007/s11165-014-9437-7
Kimbell, R., and Perry, D. (2001). Design and technology in a knowledge economy. London: Engineering Council.
Koh, J. H. L., Chai, C. S., Wong, B., and Hong, H. Y. (2015). Design thinking for education: conceptions and applications in teaching and learning. Springer.
Lamb, S., Maire, Q., and Doecke, E. (2017). Key skills for the 21st century: An evidence-based review. Sydney: NSW Department of Education. Available at: https://education.nsw.gov.au/our-priorities/innovate-for-the-future/education-for-a-changing-world/research-findings/future-frontiers-analytical-report-key-skills-for-the-21st-century/Key-Skills-for-the-21st-Century-Analytical-Report.pdf (Accessed November 25, 2019).
Lefstein, A. (2010). “More helpful as problem than solution: some implications of situated dialogue in classrooms” in Educational dialogues: Understanding and promoting productive interaction. eds. C. Littleton and C. Howe (London, UK: Routledge), 170–191.
Lipman, M. (2008). A life teaching thinking. Montclair, N.J.: The Institute for Advancement of Philosophy for Children.
McCormick, R., and Davidson, M. (1996). Problem solving and the tyranny of product outcomes. J. Des. Technol. Educ. 1, 230–241.
McDaniel, C. N., Lister, B. C., Hanna, M. H., and Roy, H. (2007). Increased learning observed in redesigned introductory biology course that employed web-enhanced, interactive pedagogy. CBE-Life Sci. Educ. 6, 243–249. doi: 10.1187/cbe.07-01-0003
Mercer, N. (2004). Sociocultural discourse analysis. J. Appl. Linguist. 1, 137–168. doi: 10.1558/japl.2004.1.2.137
Millett, S., and Tapper, A. (2012). Benefits of collaborative philosophical inquiry in schools. Educ. Philos. Theory 44, 546–567. doi: 10.1111/j.1469-5812.2010.00727.x
Nichols, K., Kennedy, C., and Burgh, G. (2017). Comparing two inquiry professional development interventions in science on primary students’ questioning and other inquiry behaviours. Research in Science Education 47, 1–24.
Nichols, K., Musofer, R., Fynes-Clinton, L., and Blundell, R. (2022). Design thinking and inquiry behaviours are co-constituted in a community of inquiry middle years’ science classroom context: empirical evidence for design thinking and pragmatist inquiry interconnections. International Journal of Technology and Design Education 32, 2527–2551.
Reznitskya, A., Glina, M., Carolan, B., Michaud, O., Rogers, J., and Sequeira, L. (2012). Examining transfer effects from dialogic discussions to new tasks and contexts. Contemp. Educ. Psychol. 37, 288–306. doi: 10.1016/j.cedpsych.2012.02.003
Reznitskya, A., and Gregory, M. (2013). Student thought and classroom language: examining the mechanisms of change in dialogic teaching. Educ. Psychol. 48, 114–133. doi: 10.1080/00461520.2013.775898
Roth, A., Kim, H., and Care, E. (2017). New data on the breadth of skills movement: Over 150 countries identified. Available at: https://www.brookings.edu/blog/education-plus-development/2017/08/31/new-data-on-the-breadth-of-skills-movement-over-150-countries-included/ (Accessed November 25, 2019).
Sharp, A. M. (2017). “The other dimension of caring thinking” in Community of inquiry with Ann Margaret Sharp: Childhood, philosophy, and education. eds. M. R. Gregory and M. J. Laverty (NY, Routledge: New York), 209–214.
Sprod, T. (2014). “Philosophical inquiry and critical thinking in primary and secondary science education” in International handbook of research in history. ed. M. R. Mathews (Dordrecht: Springer), 1531–1564.
Sprod, T. (2017). “Teaching science and morality via P4C” in History, Theory and Practice of Philosophy for Children. Routledge. 67–71.
Tedesco, J. C., Opertti, R., and Amadio, M. (2013). The curriculum debate: Why it is important today. Geneva: UNESCO International Bureau of Education.
Topping, K. J., and Trickey, S. (2014). The role of dialog in philosophy for children. Int. J. Educ. Res. 63, 69–78. doi: 10.1016/j.ijer.2013.01.002
Trickey, S., and Topping, K. J. (2004). Philosophy for children: a systematic review. Res. Pap. Educ. 19, 365–380. doi: 10.1080/0267152042000248016
Trickey, S., and Topping, K. J. (2006). Collaborative philosophical enquiry for school children. Socio-emotional effects at 11 to 12 years. Sch. Psychol. Int. 27, 599–614. doi: 10.1177/0143034306073417
Waggoner, M., Chinn, C. A., Yi, H., and Anderson, R. C. (1995). Collaborative reasoning about stories. Lang. Arts 72, 582–589.
Weldon, P. (2017). Changing priorities? The role of general capabilities in the curriculum. Camberwell, Australia: Australian Council for Educational Research.
Wolf, M., Crosson, A., and Resnick, L. (2006). Accountable talk in reading comprehension instruction (CSE Tech. Rep. No. 670). Pittsburgh, PA: Learning and Research Development Center, University of Pittsburgh.
Keywords: community of inquiry, design, discourse analysis, STEM competencies, dialogic inquiry
Citation: Nichols K, Musofer R and Haynes M (2022) How to promote STEM competencies through design. Front. Educ. 7:982035. doi: 10.3389/feduc.2022.982035
Received: 30 June 2022; Accepted: 11 November 2022;
Published: 12 December 2022.
Edited by:
Jeffrey Buckley, Athlone Institute of Technology, IrelandReviewed by:
Zuzana Haláková, Comenius University, SlovakiaCopyright © 2022 Nichols, Musofer and Haynes. This is an open-access article distributed under the terms of the Creative Commons Attribution License (CC BY). The use, distribution or reproduction in other forums is permitted, provided the original author(s) and the copyright owner(s) are credited and that the original publication in this journal is cited, in accordance with accepted academic practice. No use, distribution or reproduction is permitted which does not comply with these terms.
*Correspondence: Kim Nichols, ay5uaWNob2xzQHVxLmVkdS5hdQ==
Disclaimer: All claims expressed in this article are solely those of the authors and do not necessarily represent those of their affiliated organizations, or those of the publisher, the editors and the reviewers. Any product that may be evaluated in this article or claim that may be made by its manufacturer is not guaranteed or endorsed by the publisher.
Research integrity at Frontiers
Learn more about the work of our research integrity team to safeguard the quality of each article we publish.