- Research Center Robotics and E-Health: A Challenge for Education (RECE), University of Modena and Reggio Emilia, Reggio Emilia, Italy
In recent years, due to technological advancement, research has been directed to the development and analysis of resources and tools related to educational robotics with particular attention to the field of special needs and training actions aimed at learners, teachers, professionals, and families. The use of robotics in all levels of education can support the development of logical and computational thinking, interaction, communication, and socialization, and the acquisition of particularly complex work practices, for example, in the medical field. The adoption of successful educational robotics training practices can be a potential tool to support rehabilitation interventions for disabilities and comprehensive training for students or future professionals in healthcare. A scoping review was conducted on the main topics “education” AND “robotics” with three specific focuses on complementary themes in educational research about ER: (1) teaching and computational thinking, (2) training in the health sector, and (3) education and special needs. The authors systematically searched two online databases, Scopus and Web of Science, up to April 2022. A total of 164 articles were evaluated, and 59 articles were analyzed, in a particular way N = 33 related to computational thinking, N = 15 related to e-health, and N = 11 related to special needs. The following four questions guided our research: (1) What are the educational and experimental experiences conducted through robotics in transdisciplinary fields? (2) What tools and resources are most used in such experiments (educational robotics kit, humanoid robots, telepresence robots etc.)? (3) What are the constitutive elements of the experiments and studies involving robotics and health in educational contexts? and (4) What are those explicitly related to students with special needs? In this study, part of the research project “Robotics and E-health: new Challenges for Education” (RECE) activated at the University of Modena and Reggio Emilia. RECE aims to investigate the training, educational, cognitive, and legal processes induced by the increasing diffusion of educational robotics and telemedicine in clinical and surgical contexts.
Introduction—research background
In a recent study by Bettencourt et al. (2021), starting from the definition of educational robotics (ER), referring to “robots specifically designed to interact with children in their educational activities”, two approaches to ER are distinguished: “learning about robots, learning with robots; in other words, between robotics education and robotics for education. The first approach concerns robotics-oriented technical education, while the second involves teaching different subjects (technical and non-technical) through robotics” (p. 2, our translation). Therefore, robots can be tools for learning or products of the learning processes. Their use can be a valuable instrument for acquiring digital skills and creating innovative, creative, and collaborative learning environments.
However, despite the growing research interest and the increase in studies, the potential of robotics, particularly in the educational context (Trigo et al., 2019; Negrini, 2020; Papadakis et al., 2021; Neophytou and Eteokleous, 2022) or in the training paths (e.g., for teacher training or for use in apparently different contexts and disciplines) remains in many cases not fully developed.
The educational activities and the research on robotics are not limited to children, but these tools are diffused in institutes of all educational levels till higher education and professional training. A specific field is represented by medicine, where robotics has become essential in diagnosis, surgery, long-term treatment and checking, and health professionals (Puliatti et al., 2022a) have to deal with the more advanced technologies. We are in areas of research that have seen the academic community's interest grow in recent years, focusing on interdisciplinary studies. However, at the same time, there are still few transdisciplinary studies that value and mix pedagogical, psychological, technological, legal, and health principles on the use of robotics in education and training processes, also in relation to the spread of e-health. As the recent report of the European Agency for Special Needs and Inclusive Education (2021) showed, also in response to the consequences of the COVID-19 health emergency, it will be necessary to foster “a wider range of services (e.g., health and social sectors) to work more closely with the education sector” (ivi, p. 9).
This paper is one of the starting actions of the research project RECE, “Robotics and E-health: new Challenges for Education”, activated at the University of Modena and Reggio Emilia (Italy) at the end of 2021. The project led to the creation of a research center which puts together experts of numerous scientific sectors around the theme of robotics: researchers in medicine, statistics, labor law, psychology, education. Among the actions planned by the center, there is the establishment of a laboratory of educational robotics with specific programmable robotics kits where research, experimentation, and training activities will involve four categories of users: teachers, students at high school and university, and health and education professionals. RECE proposes to study and analyze the educational, cognitive, and legal processes and needs generated by both the advent of robotics and telemedicine in clinical and surgical settings and the adoption of educational robotics as a potential support tool for assistance, rehabilitation, or development of logical and computational thinking in educational and training settings. It intends to study the use of robotics in educational and training processes from a transdisciplinary point of view, working on pedagogical, psychological, technological, and legal issues and practices, in qualitative and quantitative terms, with attention to the perception of the effectiveness of interactions and learnings, ease-of-use, added value compared to traditional techniques, and sustainability.
The paper aims to investigate and describe the educational features of interventions in ER and define what educational actions can contribute to the organization of helpful training at all educational levels and what effective research practices can be developed in an interdisciplinary group such as RECE. In order to reach these goals, we decided to analyze three areas that are included in education studies: the teaching practices on ER with a focus on those directed to the acquisition of computational thinking; the experiences of ER in health training; and the role of ER in the education of students with special needs.
The three areas can seem distant from each other: contexts, training, robots, and learners have different features in each one. However, we think that the common thread that links them is the relation between computational skills, disciplinary skills, and teaching practices. We aim to investigate how teaching practices use the development of computational thinking as a tool to set disciplinary skills and make the transition from one to the other effective. The three areas in our search offer a different perspective on this issue because they allow seeing how the three elements (computational skills, disciplinary skills, and teaching practices) coexist in each sector: at school, robots use (and as a consequence, the acquisition of computational thinking) is predominantly a tool for developing knowledge, creativity and transversal skills in basic training; they are used as assistive, re-educational or support tools in the education for SEN (Special Education Needs); in the professional training, the endpoint of teaching activities is learning how medical robots work: can the prior acquisition of computational skills facilitate this task? Of course, the investigation on professional skills could be extended to other professions, but we chose the health sector because it is a rapidly growing sector on these themes, it is closer to the social area and less related to industrial one, and, as a last, it is interesting for us in RECE center. Whatever the learning goals in the three fields, our focus is on the development of computational and algorithmic thinking, the acquisition of basic or professional skills, and the teaching practices that link the two processes. This choice gives us the opportunity: (1) to describe the whole scenario of robotics by considering the activities more related to schools with younger students (in the first two queries) and those more dedicated to professional training (in the third one); (2) to look at robots, as mentioned at the beginning of the paragraph, as a tool for learning and as a product of learning processes.
The three analysis trajectories represent three complementary aspects of educational research within the project RECE, which aims to realize a transdisciplinary approach to robotics issues and to find common solutions between practices adopted in various fields. As UNESCO stated in 1998 (UNESCO Division of Philosophy Ethics, 1998): “Transdisciplinarity is the intellectual space where the nature of the manifold links among isolated issues can be explored and unveiled, the space where issues are rethought, alternatives reconsidered, and interrelations revealed” (p. IV). Areas of study and research groups—often isolated and geographically distant—can contribute to the creation of an educational ecosystem for the study of robotics in the areas analyzed in the following sections.
Educational robotics and computational thinking
Robots entered our everyday life. Sometimes they appear just like toys. Other times they appear simply as machines that, if programmed correctly, help solve problems. Tangible robots (Funk et al., 2021) can move, recognize faces or barcodes, and use colors to identify different situations. They can be humanoid or more similar to vehicles and animals; they are programmable or linked to apps and software to do it; they have screens, sensors, motors, and wheels.
Educational Robotics use this variety of instruments in formal and informal contexts that are recognized as a tool for working on STEAM disciplines (Science, Technology, Engineering, Art e Mathematics) and enhancing students' motivation and engagement in learning activities while contributing to creating problem-solving and team working skills. The use of robotics in education has its bases in LOGO programming language and in constructivism ideas that learning is generated by working on real problems in teams and knowledge is produced by interaction among peers and with the environment (Anwar et al., 2019).
The procedures on which robots are constructed and the “simple” mechanism with which we use them to make these tools a way to work on programming and coding from an early age, thanks to the possibility they give to decompose complex problems, solve problems, think algorithms, apply the mechanism of debugging, and so on.
Public opinion considers programming synonymous with computational thinking (CT). In a widely known text by Wing (2006), she explained that CT is something more: inherent to everyday actions, it represents the new ability to add to 3 R—reading, writing, and arithmetic. She said: “Computational thinking involves solving problems, designing systems, and understanding human behavior, by drawing on the concepts fundamental to computer science” (ivi, p. 33). In her words, we find the reasons that make CT a necessary ability to integrate into the curriculum at school since the first stages: CT is reformulating, thinking recursively, using abstraction and decomposition, separation of concern, choosing an appropriate representation for a problem, having the confidence to influence complex system, using massive amounts of data, and so on.
So, “the promise of CT is that it can improve problem-solving and critical thinking by harnessing the power of computing” [Computer Science Teachers Association (CSTA) and the Int. Society for Technology in Education (ISTE) (2011), p. 7], the International Society for Technology in Education (ISTE) and the Computer Science Teachers Association (CSTA) affirmed. The skills that, according to the two associations, are essential dimensions of CT include confidence in dealing with complexity; persistence in working with difficult problems; tolerance for ambiguity; the ability to deal with open-ended problems; and the ability to communicate and work with others to achieve a common goal or solution.
CT seems to be a complex concept that, based on computer science, can be applied to more disciplines, and involve them in designing curricula that use digital tools, create collaboration, and work on feedback and error connection. In our review, we try to describe the teaching methods and solutions that can contribute to its development in ER.
Educational robotics and health training
Recent studies categorized medical robots into three main classes: (1) medical devices including surgery robotic devices, diagnosis and drug delivery devices, (2) assistive robotics including wearable robots and rehabilitation devices, and (3) robots mimicking the human body including prostheses, artificial organs, and body-part simulators (Boubaker, 2020). Surgery is one of the medical specialties that can offer a truer mirror of the state-of-the-art of robotics applied to healthcare (Puliatti et al., 2022a,b). It has been a pioneer in this field that involved urology, followed by general surgery, gynecology, thoracic surgery, and otolaryngology. Such significant use has enhanced the benefits of such technologies for surgeons, increasing accuracy in the interventions by enlarging the image of the area they need to operate on and taking advantage of the robotic arm's rotation, which is superior to that of the human hand.
The applications of robotic technology in surgical practice have grown exponentially over the last 15 years. In the last five years, nearly the entire spectrum of surgical practice has adopted robotic technology (Green et al., 2019) to assist physicians with complex surgical tasks by minimizing human error while increasing the technical ease of performing surgical interventions. According to the World Robotics 2021—Service Robots report presented by the International Federation of Robotics (IFR), the market for professional service robots reached a turnover of 6.7 billion U.S. dollars worldwide (sample method)—up 12% in 2020. At the same time, the turnover of new consumer service robots grew 16% to 4.4 billion U.S. dollars.
It can be inferred from these early statements that robotics in medical scenarios is in a strong moment of development.
Can we say the same in the area of robotics education in medicine and health?
What teaching practices dealing with robots can be observed in the medical and professional area?
We are particularly interested in finding answers because of the strong presence of surgical professors and residents in the group of RECE program, which offers the possibility of research and experience at a high level on these topics. We, therefore, added the robotic surgical experiences to the scoping review focusing on the educational aspects, although it is difficult for the authors, but at the same time interesting, to understand the scientific language of the healthcare contributions in the literature.
From a first impression over the years of research in digital education, it seems that the more discussed link between health and education is the use of humanoid robots, particularly for children's education in health. As we will see in the discussion, this perception is confirmed by the review of the international literature, which indeed has returned in the educational field, schooling mostly, few results other than this topic. However, there is also a wide range of postgraduate experiences in robotics education that make the picture more interesting, thus presenting two groups of evidence that we discuss in the remainder of this paper.
Educational robotics and special needs
In the last UNESCO report (UNESCO Global Education Monitoring Report, 2021), you can read that “statistical measurement of disability is catching up with the social model, in nine education systems, the share of 5- to 17-year-olds with a functional difficulty in at least one domain was 7.5%, on average”.
The data on the estimates of functional difficulty among 5- to 17-year-olds vary by domain and context: “in the sensory domain, the average prevalence of seeing difficulties was 0.3%. In the mobility domain, walking difficulties affected 0.8% of children. Cognitive and psycho-emotional difficulties were far more common. In total, 1.6% of children and adolescents were depressed and 4.4% suffered from anxiety” (UNESCO Global Education Monitoring Report, 2021, p. 57). In relation to sensory, physical, or intellectual disability, “increases the out-of-school rate by 2 percentage points. For adolescents of lower secondary school age, the gap is 4 percentage points, while for youth of upper secondary school age, the gap is 11 percentage points” (ivi, p. 58).
The number of students with special education needs can differ considerably in different national contexts, in fact, if in “Europe, it ranges from 1% in Sweden to 21% in Scotland (United Kingdom); in Central and Eastern Europe, it varies from 3.3% in Poland to 13% in Lithuania” (ivi, p. 59).
In the Italian context, according to the latest report published by the Istituto Nazione di statistica—ISTAT (2022), there is an increase in the number of pupils with disabilities attending Italian schools (+4 thousand, 3.6% of those enrolled). In the Istituto Nazione di statistica—ISTAT (2022), there are critical issues related to training associated with inclusive models that are still not fully disseminated among teachers. Only 24% of curricular teachers have participated in training courses on these issues. The percentage rises to 28% among support teachers. Training among secondary school teachers is less frequent (21% of subject teachers and 25% of support teachers). As stated in the same report, 75% of schools have computer workstations suited to the needs of pupils with disabilities, with the largest number in the central regions (78%).
These differences are also linked to the different definitions of “special education needs” which in turn depend on other aspects such as decentralization, population density and demographic factors, economic, social, and cultural contexts, and the structure and details of education systems (European Agency for Development in Special Needs Education, 2021, p. 13). In numerous national contexts analyzed by the UNESCO report (UNESCO Global Education Monitoring Report, 2021), you can read the link between “disability and special education needs [and] vulnerability in national laws and plans for education” (ivi, p. 125). Some European countries have standardized and not always flexible curricula, with direct consequences on the inclusion of children and young people with SEN (Special Educational Needs).
The European Agency for Development in Special Needs Education has conducted numerous research and surveys in recent years (European Agency for Development in Special Needs Education, 2021, (European Agency for Special Needs Inclusive Education, 2022a,b)—to cite some recent publications) to develop a shared framework on the use of information and communication technology (ICT) in special needs education (SEN) in different agency member states and for the implementation of inclusive education policies. This provided data, information, and resources “on policies, key issues, examples of interesting and innovate practice, key information sources plus future challenges in the field” (European Agency for Development in Special Needs Education, 2022, p. 5).
The educational contexts assume a strategic role in some processes of early detection of possible signs of difficulty and in the subsequent implementation of didactic and methodological strategies to be flanked by possible rehabilitation and reinforcement paths (Berninger et al., 2013; Cornoldi et al., 2018; Bray et al., 2021). This close relationship can make it possible not only to act on aspects and situations that are already present in the various educational contexts but also to prevent experiences of failure and frustration concerning the school environment, the emergence of critical issues related both to relationships with the peer group and to the emotional and motivational sphere of the subjects (Sannicandro, 2013, 2017): “Fully embracing the concept of inclusion in education, when it runs against deeply held and divisive views on issues such as disability, ethnicity, religion or sexuality, requires teachers to become agents of change and overcome social biases and prejudices” (UNESCO Global Education Monitoring Report, 2021, p. 25).
The link between didactics and digital technologies can facilitate the construction of meaningful learning; these are tools and resources capable of fostering autonomy in children and young people (Sannicandro, 2013, 2017). It is a question of designing teaching paths to respond effectively to the educational and developmental needs of the subjects, favoring the development of empowerment skills also through digital tools and technologies. It becomes necessary to select the tools and resources to enhance processes linked to the principles of inclusion and personalization. These elements are also related to the specific context of educational robotics, as highlighted by Alimisis (2013): “educational robotics creates a learning environment in which children can interact with their environment and work with real-world problems” (ivi, p. 63). From the study carried out by the author, it is possible to trace three different approaches to Educational Robotics: (1) Theme-Based Curriculum Approach, (2) Project-Based Approach, and (3) Goal-Oriented Approach.
Due to technological advancement, research has been directed to developing and analyzing resources and tools related to educational robotics with particular attention to the field of special needs and training actions aimed at learners, teachers, professionals, and families and conducted in school contexts. Teacher training and, at the same time, the development of professional figures (e.g., psychologists, speech therapists, etc.) involved in various capacities in these processes are not always carried out in line with the paradigms of inclusive education. Consequently, the elements in play referring to the relationship between educational robotics and special needs must consider multiple aspects linked: to teacher training, didactic planning, the constant updating of didactic and inclusive technologies, and the awareness on the part of the various subjects involved of the differences linked to special educational needs. Educational robotics can act as a resource and tool to foster the development and enhancement of transversal competences and, at the same time, act on learning processes. In recent years, several studies have investigated the relationship between robotics, disabilities, and/or special educational needs, especially in the educational context. However, as pointed out by Negrini (2020), “teachers need to be trained and, more importantly, teachers have to build a positive attitude toward robotics” (ivi, p. 78). Educational robotics and special needs are therefore closely related to the role played by inclusion processes related to digital technologies, by teachers, by school context, and “the need to identify effective ways to include the voices of all learners and their families in decision-making processes” (European Agency for Special Needs Inclusive Education, 2022b, p. 7).
Method
The contribution focuses on the first phase of the project RECE. It aims to carry out a scoping review to build a theoretical and methodological framework on the relationship among robotics, teaching, and e-health in educational contexts and, at the same time, identify practices developed at the international level, preferably with transdisciplinary approaches.
The research process followed the steps proposed by Arksey and O'Malley (2005) and the revision by Levac et al. (2010) and Khalil et al. (2016, p. 120) in the field of Proposed Methodology of the Scoping Reviews Based on the JBI Framework of Evidence Synthesis and the successive methodological guidelines of Peters et al. (2020a,b, 2021). In the authors' recent publication (Peters et al., 2021), it is highlighted that “the latest guidance for scoping reviews includes the JBI methodology and the Preferred Reporting Items for Systematic Reviews and Meta-Analyses—Extension for Scoping Reviews” (ivi, p. 1). The scoping reviews “can provide end users with important insights into the characteristics of a body of evidence, the ways, concepts or terms have been used, and how a topic has been reported upon” (ivi, p. 2).
As said by Arksey and O'Malley (2005), scoping review process “is not linear but iterative, requiring researchers to engage with each stage reflexively and, where necessary, repeat steps to ensure that the literature is totally covered” (ivi, p. 8). The authors identify the main stages in Table 1 as summarized in JBI Manual for Evidence Synthesis (Peters et al., 2020, p. 412).
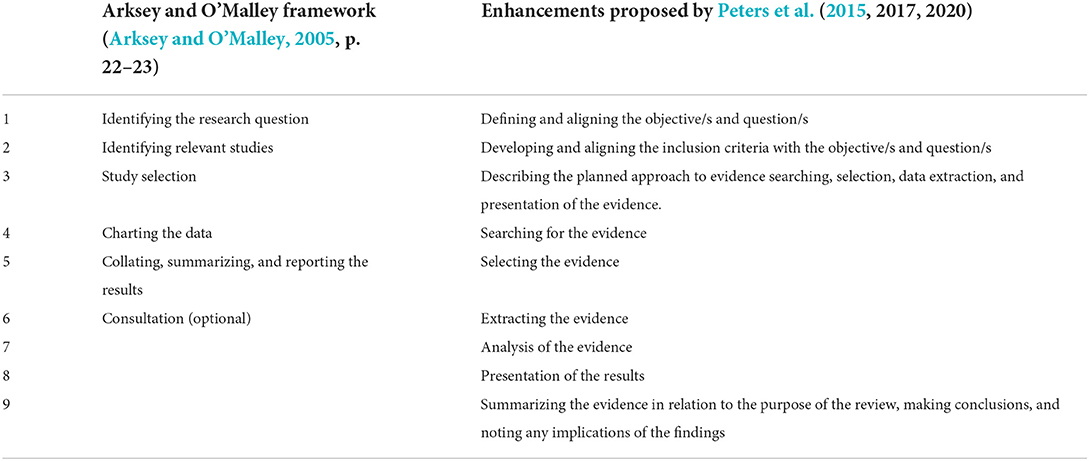
Table 1. Scoping review frameworks (Peters et al., 2020b, p. 412).
Scopus and Web of Science are the electronic databases examined for the scoping review. The search ended in April 2022. In the selection of papers, non-matching studies and research were excluded, considering the correspondence between identified articles, research topics, and the following eligibility criteria:
• Period: 2016–April 2022
• Language: English
• Review: peer-reviewed
• Article type: articles and reviews.
We chose the period between 2016 and 2022 to identify studies and research focusing on recently developed tools and models. In particular, in the medical field, some solutions have been implemented in recent years, both in response to the growing demand for telemedicine (not only for medical emergencies) and the spread of widespread technologies such as the Internet of Things (IoT). We decided to exclude previous studies (albeit interesting) because we are oriented to mapping recent evidence-based studies and practices to seek a starting point for new experiences within RECE center.
Three independent reviewers screened and analyzed the papers published in open-access journals and those that could be downloaded through our university subscription (in particular, journals by Elsevier). The authors performed data extraction; checked titles, abstracts, and full texts; assessed the risk of bias; selected; and consequently excluded articles not relevant to the three areas of analysis, also for “developing and aligning the inclusion criteria with the objective/s and question/s” according to the methodological indications of Peters et al. (2020b). Each reviewer checked data extracts only in one area; subsequently, the three reviewers discussed the results together.
The three areas of inquiry described above in paragraphs 1.1, 1.2, and 1.3 were investigated through the following research strings used in combination with the Boolean expression AND: (1) robot AND teaching AND “computational thinking”, (2) robotics AND education AND health AND teaching, (3) robotics AND education AND special needs. We chose to start with a limited number of terms to verify the earliest elements of connection among the three areas that we aim to explore in the future in a transdisciplinary way.
We applied a two-stage check process for eligibility criteria: at the beginning of the search, we automatically applied criteria querying the database; after identifying duplicate records, we manually checked the records for the selection of eligible studies. This procedure has at least two advantages: double checking of eligibility criteria by automated database systems and by researchers; faster reproducibility of records selection mechanisms in the study.
The research questions that guided the review are:
1. What are educational and experimental experiences conducted through robotics in a transdisciplinary field?
2. What tools and resources are most used in such experiments (educational robotics kit, humanoid robots, telepresence robots etc.)?
3. What are the constitutive elements of the experiments and studies involving robotics and health in educational contexts?
4. What are those explicitly related to students with special needs?
Figure 1 illustrates a detailed description of the scoping review process for the three strings and the number of papers selected and analyzed. We describe in the following three sections the results for each string. See Appendix (Supplementary material) for a complete list of the papers.
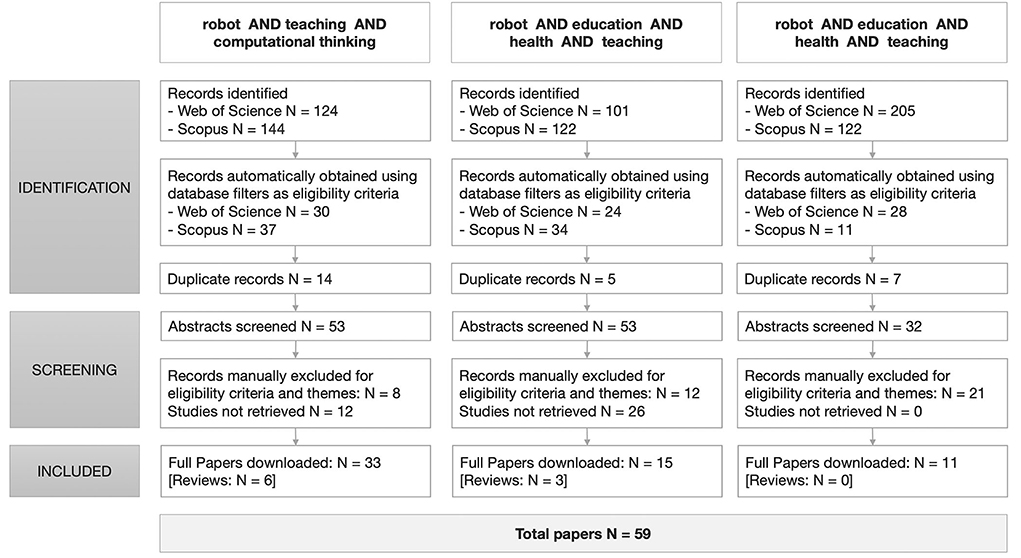
Figure 1. Process of scoping review related to the three strings about ER: (1) teaching and computational thinking, (2) training in the health sector, and (3) education and special needs.
Results
Robot, teaching, and computational thinking
What is the scenario around teaching and robotics? This section investigates teaching practices (for students and teachers) that affect the use of robots in the educational context concerning computational thinking.
The keywords we used for the search are Robot, Teaching, Computational thinking. Using the three keywords, we obtained 144 documents on Scopus (Filter: Article title, Abstract, Keywords) and 124 on Web of Science (Filter: All fields). We applied the criteria described in the Methods section to these first results (period, language, peer-reviewed, article type) automatically through filters on database systems. The used filters were Year/Year published (2016–2022), Language (English), and Document Type (Article, Review). We decided not to add limits for Subject area on Scopus. Instead, some limitations were done in the research on Web of Science where we selected papers belonging to the following categories (Web of Science Categories): Education Educational Research, Computer Science Interdisciplinary Applications, Education Scientific Disciplines, Instruments Instrumentation, and Educational Special.
At the end of this procedure, 37 records were found on Scopus, 30 on Web of Science of which 14 were duplicates. We excluded eight papers because they did not reply to the criteria after the automated selection (written in English or belonging to Proceeding), and the themes were not fully compliant with our questions. We couldn't download 12 papers because they were not in open access or available through university subscriptions. The number of papers downloaded is 33.
Even if computational thinking and integration of technology tools are not new, the publication on the theme seems to grow in 2020: the number of papers published between 2016 and 2019 is 7 (no studies published in 2016 were selected in our search). In 2020, the number grows to 10 as it happened in 2021. Until April 2022, 8 papers have already been published.
Where possible in the papers, we reported the countries where the studies took place: most of the studies (15) were realized in Europe (six in Spain) and Asia followed with six papers. Papers are published in 25 scientific journals in the categories of Social sciences (16) and Computer Science (12); 14 of the 25 are in both categories. Categories also included are Psychology (4) and Engineering (3) to show that these are the main sectors of interest in the field that lead to educational, psychological, informatic, technical issues, and STEM (Science, Technology, Engineering, and Mathematics). Of course, limitations in Web of Science can affect these numbers. However, the journals “Computers and Education” and “International Journal of Child-Computer Interaction” (among the 16 categorized in areas of both Social sciences and Computer Science) published four papers for each in the review period.
Six papers, among those selected, are reviews. Yang et al. (2020a) produced a literature review using snowball rolling methods where papers published between 2009 and 2019 were selected using the keywords CT and robot and a bibliometric analysis on global research trends in robot education in the same journal and with the same period Yang et al. (2020b). Robots used and costs, proposed activities (goal-oriented, open-ended, free play), and CT assessment with recommendations for future studies and research are in the systematic review by Bakala et al. (2021) on computational thinking in preschoolers. In this case, the keywords chosen are robot, computational thinking, and preschool education, with their synonyms. Zhong and Xia are the authors of two reviews: one on the potential of educational robotics in mathematics education at all educational levels (Zhong and Xia, 2020), the second on teaching and learning robotics content knowledge in K-12 (Xia and Zhong, 2018). Jamal et al. (2021) proposed a qualitative analysis, where research and other sources are analyzed through grounded theory to design a framework to integrate CT and ER. Our review differs from the others in the method, period, keywords, and school level because we aim to describe teaching practices more generally.
We now describe the results from the analysis of the other 27 papers based on the targets of educational interventions. Even if some research involved students of different ages and school levels, we can round and say that five studies have been realized with children in pre-school/kindergartens, 7 with students between 6 and 10 years (primary school), 7 with students between 10 and 16 years, and only three in the university. Five papers investigated teachers' training related to educational robotics.
For pre-school children, the robot used is Bee-bot, and in only one case, its evolution, Blue-bot. Both robots are without screen programmable robots particularly useful in acquiring programming processes and spatial relations. Their use regards the acquisition of abilities in programming (for example, understanding algorithms and decomposition strategy for complex tasks), logical–mathematical sectors, spatial thinking, meaning of maps and symbols, communication, collaboration, and engagement. In only one case, the educational intervention includes children with communication difficulties (Critten et al., 2021). Besides these themes, the studies in primary schools deepened emotional intelligence and team workings. Bee-bots is used only in one case: other robot names like Thymio, a small visual programming robot characterized by colors; mBot, Arduino-based and screen-programmable with a Scratch-like environment, Cozmo, a robot with sensors and camera, linked to an app. In two cases, the framework used is CAL (Coding as a language), “a pedagogical approach for early childhood computer science” by Bers (2019, p. 499).
In secondary schools, Lego kits are used in three cases. A particular mention can be made to RoboKar, a small mobile robot developed by Universiti Teknologi Malaysia (UTM) and used to teach problem-solving (Jawawi et al., 2022). In one case, researchers involved students in creating a robot on paper through unplugged activities that focus on processes and not technologies (Merino-Armero et al., 2022).
From a didactic point of view, the experiment conducted in a high school with 38 students of 15–16 years old is interesting: it proposes a comparison between two classes where pair learning versus individual learning. At this school level, we found two papers targeting two opposite student types: Díaz-Lauzurica and Moreno-Salinas (2019) addressed the intervention to students with apathy and demotivation to develop a methodology to teach robots that can also engage this category of students; Sen et al. (2021) to gifted and talented students to construct a robot able to solve Rubik's cube and promote creative and original thinking. Santos and Lopes (2020) presented the creation of a virtual community (DROIDE), while students in secondary school used LEGO Mindstorms, a multi-language programming platform, and eight problems between Mathematics and Informatics to solve.
At the university, we have three research studies in three different geographical areas (Spain, Panama, and Taiwan) that involved engineering students in two cases and students in science and technology in the third one. The core focus of the teaching content is STEM and technical skills in the realization of robots. In the studies, students are asked to test platform based on MATLAB/Simulink package and LEGO EV3 (Montes et al., 2021) to realize a BEAM robot working on hardware and using robots as a tool to acquire STEM knowledge and skills (Boya-Lara et al., 2022), to realize projects using Scratch or NUWA, a tangible robot used to provide teaching materials (Hsieh et al., 2021). In two cases (Hsieh et al., 2021; Montes et al., 2021), the teaching method proposed is project-based learning. In particular, the research by Hsieh et al. (2021) investigated the teaching method that best fits the improvement of CT abilities: besides unplugged teaching methods with the use of books and traditional activities (control group), experimental groups of students used project-based learning together with an environment Scratch or NUWA robot.
At all educational levels, experimental or quasi-experimental research with pre-post tests are proposed and based on different tests: some standardized as CT assessment test by Román-González et al.and Map Test for Children by Peter et al. (Diago et al., 2021), others constructed for specific cases. Only Chen et al. (2017) describe the development of an instrument to assess CT levels for fifth-grade students for coding in robotics and reasoning of everyday events made of 15 multiple-choice and eight open-ended questions. Together with quantitative studies, qualitative researches are proposed: in the contribution of Chevalier et al. (2022), video analysis of four experimental conditions on guidance and feedback by teachers are recorded and analyzed in primary schools; in the same way, in pre-schools, the analysis of words related to programming and computational thinking are recorded and analyzed (Fridberg and Redfors, 2021).
Five researches (realized in Australia and Europe—Switzerland, Spain, Cyprus, Austria) related to teachers start from the idea that there is a strong necessity to integrate robotics/computer science/digital citizenship from the first years at school. In some other papers in the review, even if the teaching interventions are targeted at students, teacher training was implemented and considered part of the research. The role of teachers is fundamental to doing this, even if teachers do not always have sufficient skills and knowledge to integrate CT curriculum and activities.
The teachers can be in service or students preparing to be teachers (Esteve-Mon et al., 2019; Angeli, 2022), mainly in primary school (one case also in kindergarten). The number of subjects involved in the studies varies from 4 and 350, just like the kind of research that can be organized as experimental or quasi-experimental with pre-post tests on computational thinking, digital competencies, algorithmic thinking, and debugging skills (IN-COTIC and CT test in Esteve-Mon et al., 2019, Technology Usage Inventory, TUI in Tengler and Sabitzer, 2022) or according to qualitative or mixed approaches. For example, the study of Chalmers (2018), one of the three most cited among those selected in the review, is structured as multiple case studies on four teachers in Australia whose goal is to examine the perceptions of teachers in the implementation of WeDo 2.0 robot kits in their classrooms. In this case, teachers completed an initial questionnaire, two journal reflections, and a final semi-structured interview. The papers focus on the organization (methods and contents) of the training for teachers, the models for teaching robotics to students, and teachers' perceptions. Papers cite T-PACK as a model for teachers' training.
The methods are related to using robotics to work on CT; the only interdisciplinary method to teach robotics together with storytelling is Tell, Draw, and Code (Tengler and Sabitzer, 2022). In the papers, together with unplugged activities, we find the use of robots such as Bee-bots, ozobot, mBots, software such as Scratch, and systems such as Makey-Makey and Arduino. The paper by El-Hamamsy et al. (2021) describes a model to integrate CS in primary school (1–4 grade) and its validation in ten schools through the training of 350 teachers on computer science unplugged activities, robotics unplugged activities, robotics activities involving visual programming, and non-robotic activities involving visual programming that can be easily transferable to classrooms (about 5,000 pupils). The model requires the presence of 23 purveyors that are teachers and attended different training to support colleagues. Researchers collected teachers' opinions on their representation of the discipline and, finally, the adoption of the CS content in their classrooms. Two key elements are teacher training and curriculum design.
We concluded this overview by listing the content of the three most cited papers according to Scopus. The three papers are referred to primary school and cover the main components of didactics:
• Assessment: “Assessing elementary students' computational thinking in everyday reasoning and robotics programming” (Chen et al., 2017, Computer and Education): an instrument to assess students' CT level (163 citations);
• Activities and interaction: “Developing young children's computational thinking with educational robotics: An interaction effect between gender and scaffolding strategy” (Angeli and Valanides, 2020, Computers in Human Behavior): a study using Bee-bot and two scaffolding techniques (84 citations);
• Teachers' role: “Robotics and computational thinking in primary school” (Chalmers, 2018, International Journal of Child-Computer Interaction): a research on teachers' strategies and opinion in educational robotics (81 citations).
Robotic, education, and health
The first consideration after the research on scientific references that include robotics and education in the health sector is that there are two groups of evidence that literature shows: one regards the experiences of post-base training for healthcare professionals in the use of robotics for medical treatment (often surgical), one on school education for teacher in the use of robots for children. In these results, we consider both intending to pursue the transdisciplinary nature of this work.
Given the likely paucity of potentially eligible studies related to this topic, we considered experimental designs (randomized controlled trials) and other study designs (non-randomized trials, observational, cohort, case–control, and qualitative). We excluded editorials, conference abstracts, opinion pieces, studies not published in English, and studies not fully compliant with our focus. The target population included school students and healthcare professionals.
As said, the electronic bibliographic databases searched were: Scopus and Web of Science.
A structured search strategy was created for use on two bibliographic databases with keywords used according to each database's specific requirements.
Keywords included in the review for this area are: “robotic”, “education”, “health”, and “teaching” used in combination with the Boolean expression AND. The first results on Scopus are 122 papers. The first results on Web of Science are 101 papers, instead. Next, we entered inclusion criteria (period, language, peer-reviewed, article type) and set additional filters automatically on database systems. We applied a filter for areas that, because of differences between the two databases, are different:
- For scopus (Subject areas) they were social, health, nursery;
- For web of science (Web of Science categories): robotics, education, education scientific disciplines, health policy, social science interdisciplinary, multidisciplinary sciences, and healthcare sciences services.
Items resulting on Scopus were 34; 7 were deleted after reading the abstract because they were not fully compliant with our focus and 18 weren't in open access. Items on the Web of science resulted were 24; 5 were already present in the Scopus repository, 5 were deleted after reading the abstract because they were not fully compliant with our focus, 8 weren't open access. The total number of papers downloaded is 15.
Data extraction included the type of study/design, date of publication, main objectives, country and specific medical discipline, and contextual recurrent themes (i.e., Artificial Intelligence; Simulation; Virtual Reality).
Other types of contributions (books, etc.) were excluded; in addition, works already published were selected and works in the process of publication were excluded.
The abstracts were read to evaluate whether the studies seemed to correspond to the purpose of our review. The total number of papers downloaded after reading the abstract is 15 (9 Scopus, 6 WoS).
Group 1—The studies focusing on experiences in robot education for health. Two studies focused on the educational intervention of robots in school. An interesting Italian study (Rosi et al., 2016) proposes an evaluation of the presence of a humanoid robot (NAO) to improve the efficacy of a game-based, nutritional education intervention. Target is fourth-grade school children (8 and 10 years old), for a total of 112. Also, a literature review regarding social assistive robots was considered. Robaczewski et al. (2021) consider the specific case of NAO in literature, defining six categories in the surveys: social interactions, affectivity, intervention, assisted teaching, mild cognitive impairment/dementia, and autism/intellectual disability. In line with our discussion, we report the conclusion on NAO as a teacher: “The robot was an efficient teacher or a coach assistant. Its greatest advantage is its use of multiple learning modalities (visual, auditive and kinaesthetic). However, disadvantages consisted of adapting to the rhythm of the children, technical issues and physical limitations”. Instead, Hughes-Roberts et al. (2019) explore the potential effects of robots on eleven learners by examining engagement and goal achievement within teaching sessions. This study differs from others in the literature in its use of a single-subject design methodology utilizing a more appropriate control condition. Despite the thoroughness and the originality of these investigations, the presence of a robot to support teaching activity did not result in any significant learning improvement. It's not found a significant difference between the robot and the control sessions for any of the outcome measures utilized, suggesting robots are as effective as teaching tools as traditional methods. A transversal study by Harris et al. (2020) introduces the use of neurorobots—robots controlled by computer models of biological brains—as an introduction to computational neuroscience in the classroom. Unlike the results of using robots for disabilities, this experience demonstrates that neurorobotics offers students a unique opportunity to learn neuroscience and computational methods by building and interacting with embodied models of neurons and brains. There are two studies in the sector of children's rehabilitation. Both reported that children enjoyed participating in the training with a robotic presence, despite the experience being different. A study was conducted in a children's rehabilitation hospital with the purpose of a program to help young disabled children in STEM fields by engaging them in a group-based robotics program while also building essential skills (Lindsay, 2020). Results highlight that children agreed that the perceived impact of the program was good, particularly in helping them understand teamwork, solve real-world problems, and gain an interest in science. The second one presents a robotic intervention system for training manual dexterity with the potential to be used within a school or home environment without needing one-to-one administration (Shire et al., 2016). The review of the authors shows that no measures focus on assessing the impact of a robotics program on youth with disabilities, addressing an important gap in the literature by exploring the participation of youth with disabilities in a group-based robotics program.
Group 2—The research on the best training methods to teach the use of robotic medical instruments. There are a lot of post-base training programs and experiences in the literature on robot training in the medical discipline that often involves specialization and residency. In these types of experiences, different from school studies, the use of robotic instruments applied in the medical discipline is the main subject of training: it's not the education to be “robotic” but the instrument. Most of them, considered for the second group, face the experience of robot-assisted surgery. As Green et al. (2019) said, “the discipline is growing over the last 15 years and because this expansion includes academic medical centers, surgical trainees are increasingly exposed to this technology”. So, structured and validated training programs for surgeons became one of the main targets that need to be developed.
A particular experience reported by Beulens et al. (2018) shows results on 1-day robotic surgery training. The training consisted of pre-training preparation, a theoretical session, a practical session on the robot set-up, and a simulation session on virtual reality simulators. The participants filled out an online questionnaire at the end of the training, and the results showed that novice robot surgeons are too optimistic in self-assessing their dexterity skills after 1-day training. Self-assessment revealed uncertainty of the obtained knowledge level on requirements for the safe use of the surgical robot. An unexpected topic found during the readiness is the use of video for training on robotic instruments. For this second group of scientific evidence, three studies analyze the importance of video content for robotic training in health: an educational video can help the trainees, especially with a relatively complex simulator module, and reflect the module developer's intention. Studies highlight that many surgeons preparing for robotic surgery receive some help from an educational video (Shim et al., 2018; Adorisio et al., 2021). Consequently, video-sharing platforms have become a significant source of visual information for health care providers. Shim et al. (2018) show no significant difference between subjects with expert proctoring and those who viewed an educational video only in achieving skill acquisition, but both groups yielded significant improvement compared to those with independent training. Kun et al. (2019) show that using an integrated video recording system makes the self-manipulated protocol with its own smartphone feasible to improve training efficiency and overcome skill decay during robotic surgical training.
More generic papers below on surgical experience are considered for the completeness of the review. Two studies assess technical skills in surgeon performance robot-assisted. The study by Yu et al. (2021) assesses the association between an assistant's technical skill on surgeon performance in Robotic-Assisted Radical Prostatectomy (RARP) with an often-cited scale: Global Evaluative Assessment of Robotic Skills (GEARS). The next study by Beulens et al. (2019) says that various authors suggested that systematic evaluation of skills, both technical (surgical) and non-technical (communication and teamwork), may be more effective in improving the surgeons' skills than a quota alone. So, the authors proposed an assessment instrument for evaluating surgical videos to elucidate the association between surgical skills and postoperative outcomes after a robot-assisted radical prostatectomy (RARP). More on the surgical skill topic, a review by Childs et al. (2019) provides an update on surgical simulators and tools for urological trainee education. Hung et al. (2018) review research about telemedicine and minimally invasive surgery. Robotics provides an ideal environment for surgical telementoring and telesurgery, given its endoscopic optics and mechanized instrument movement.
Robotics, education, and special needs
This section investigates strategies and educational robotics resources in the inclusive education processes. Inclusive education allows the connection between “researchers and teachers on shared and reciprocal research and training tracks: research competence is essential for an inclusive teacher, evidence-based school practices are indispensable for a researcher studying special educational needs” (Tessaro, 2011, p. 115, our translation).
String identified with the research group, related to robotics AND education AND special needs, is searched on the two already mentioned databases (Scopus and Web of Science). In detail:
Scopus: We searched the keywords within Article Title, Abstract, and Keywords in the database to focus and narrow our analysis; 122 contributions resulted from our query. We then added the mentioned inclusion criteria (period, language, peer-reviewed, article type) and set filters in the database related to the choice to include only articles from the social sciences area [filter: subjects area], articles and reviews in English [filter: language], and types of contributions [filter: document type]. Furthermore, works already published were selected and works in the publication stage were excluded [filter: publication stage]. The total number of articles is 11 (please note that works published after April 2022 were excluded manually).
Web of Science: The initial search with the filter All fields produced a total of 205 papers. We then inserted the filters mentioned for the inclusion criteria (period, language, peer-reviewed, article type, articles and reviews in English [filter: language], and types of contributions [filter: document type]). The total number of articles is 28 (please note that papers published after April 2022 have been manually excluded).
Seven duplicate contributions were identified.
In the subsequent reading analysis of the selected works, contributions available in open-access format were also retrieved from sources external to Scopus and Web of Science if evaluated compliant with our research (e.g., from the journal website, authors' pages, or institutional websites, by searching the version with google scholar). After reading the abstracts, 21 records considered not compliant (Peters et al., 2020b) with the research questions or not available in the open-access version were excluded; 11 contributions were selected.
The research shows the importance of the teacher and professional figures working in different roles to redesign the educational setting, in the implementation of experiments in different educational contexts involving the use of social robots in education, human–robot interaction, and robot-assisted. Many studies focus on special education needs or disabilities, specific developmental disorders, autism spectrum, and so on.
With respect to the close link between educational robotics and special needs, the studies by Bargagna et al. (2019) and Catlin and Blamires (2019) deal with:
• Down syndrome (DS): “this experience is the first attempt to evaluate the feasibility of ER-Lab using one of the most utilized robots in educational technology, the Bee-Bot robot, in a group of DS children. Limitations of this study are mainly related to the heterogeneity of the sample in terms of age, cognitive developmental profiles and comorbidity and the qualitative interpretation of data” (Bargagna et al., 2019, p. 322).
• Special needs education (SEN): The paper starts with a historical analysis of educational robots and their use in SEN and the meaning of special needs education in the research. The contribution aims to strengthen the link between robotics and SEN. In the authors' proposal, “Papert's Paradigm links together education and technology within a pedagogic framework that is now well supported by a view of difficulty and disability. Both these give agency to the learner and teachers who have the potential enhanced skills and knowledge to exploit the power offered by educational robots” (Catlin and Blamires, 2019, p. 310). In the contribution, the principles related to characteristics of special needs robots (ERA) are presented (see Table 2).
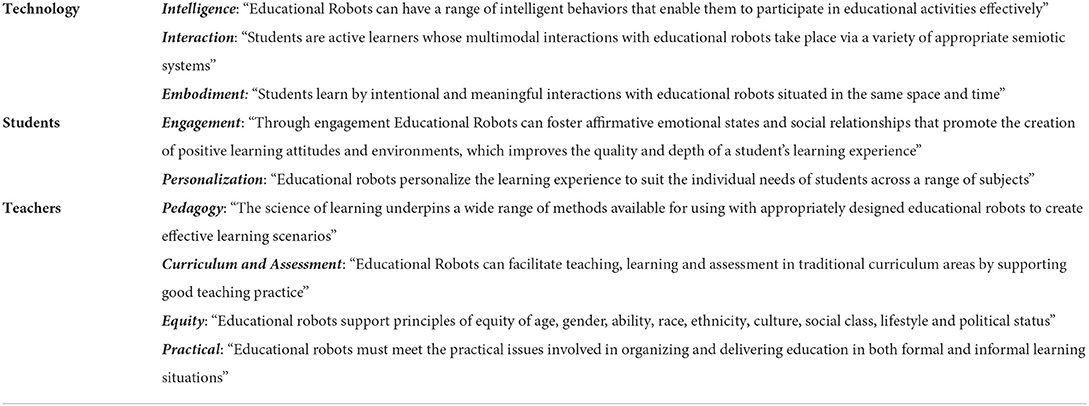
Table 2. Summary of the ERA principles (Catlin and Blamires, 2019, p. 306–307).
The importance of teacher's training and, at the same time, their involvement and participation in experiments is the subject of other studies. Alcorn et al. (2019) conducted a study to investigate the potential “of social robots in education for learners on the autism spectrum”. They collected, through semi-structured interviews and focus groups, the perspectives of educators on the potential use of humanoid robots with autistic students. The research involved “thirty-one autism education staff, representing a range of special education settings and age groups as well as multiple professional roles (e.g., teachers, teaching assistants, speech, and language therapists)”. The study refers to the context of special classes (not present, for example, in the Italian context) where “classes are small (often 5–10 children), with a highly trained teacher and a team of teaching assistants”. This is something to consider in the analysis of best practices and for the replicability of studies related to these themes in different contexts. The authors have identified four critical themes related to the central theme:
1. Autistic children are likely to find robots engaging
2. Robots offer predictable, consistent interactions; children know what to expect
3. Roles of robots in autism education
4. Children ultimately need to interact with people, not robots.
The study shows the willingness of participants to explore and experiment with the use of humanoid robots in the autism education context. “Interviewees proposed robot uses that supported existing curricular goals and volunteered a range of established educational strategies that could be applied to introduce robots and support their use. Suggested robot activities and roles built on existing classwork (e.g., practicing turn-taking in a small group) and staff roles” (Alcorn et al., 2019, p. 9). Robots are considered a “stepping stone” for learning, although the ability of humanoid robots to support children with autism is not currently supported by extensive research evidence. The study by Anisimova et al. (2017) investigates effective strategies and methods of training the professional competence of future teachers to work with gifted students. In this case, the training course includes modules dedicated to educational robotics to “promote the development of skills in using robotic art technology in classroom and extracurricular activities for the development of students' creative abilities in the process of designing and programming robots” (p. 1).
In their contribution, Schina et al. (2021) offer an overview of the research on teacher education in Educational Robotics (ER), and some recommendations for institutions considering implementing teacher education programs. Their study suggests that:
• “ER teacher training programs are often not founded on theory. When they are based on pedagogical principles;
• The lack of uniformity regarding the duration and requirements of training programs and the fact that information on trainer and trainee profiles is not always documented,
• The most prominent best practices for ER teacher training fall into five categories (collaboration, materials, pedagogy, practice and feedback/support)” (p. 2831).
Their analysis leads to “recommendations for future ER teacher training programs” in Table 3.
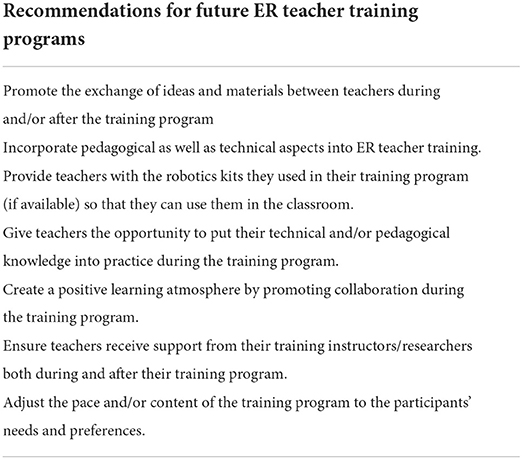
Table 3. Recommendations for future ER teacher training programs (Schina et al. (2021), p. 2,845).
Chootongchai et al. (2021) identified and confirmed quality dimensions in robotic education from the teachers' perspectives. Research was conducted with 510 teachers observed in Thai elementary schools. They have identified “six dimensions of robotic education quality as Social interaction, Cognitive function, Teaching method, Learner characteristics, Main features and Content” (p. 1). These dimensions have emerged in the literature and in other studies, but they can contribute to the construction of a complex methodological and theoretical framework that is useful for implementing research and experimentation related to educational robotics.
The focus on the inclusion of robotics in diverse training contexts is also investigated by Aniskin et al. (2020) and Ao and Yu (2022); in the first case for STEM disciplines with “the introduction of robotic and cyber-physical systems in the training of future specialists” (Aniskin et al., 2020) and in the second case Ao and Yu (2022) for robot-assisted language learning in the school setting to improve learning processes through human–robot interaction.
In addition, it is possible to identify several studies on STEM disciplines concerning areas of cross-cutting applications helpful in building a more articulated framework. In the contribution by Potkonjak et al. (2016), starting with the application of virtual labs and virtual worlds in science, technology, and engineering, the research focuses on the field of robotics. The authors' choice is motivated not only by diffusion due to the maturity of this area within the virtual education community but also because of its widely transdisciplinary character; robotics is a perfect example to which all the other fields of engineering and physics can contribute.
Still, for the complex multidisciplinary framework, the contributions of Scaradozzi et al. (2019) and Fridberg et al. (2022) propose studies that link STEM disciplines to educational robotics and, at the same time, help to confirm the close link with learning processes and instructional choices. Choices related, for example, to “two other methodologies, Coding and Tinkering, characterized by a similar hands-on approach. In order to fully exploit their inclusive features, teachers need to be prepared to introduce them into the classroom. It is noticed that in-service teachers are not yet fully prepared to face this challenge” (Scaradozzi et al., 2019, p. 249). The training aimed at teachers is once again one of the main variables, intending to evaluate the effect of a training course on in-service teachers' knowledge and self-confidence in using technology in practice (ivi, p. 263).
Finally, the work of Fridberg et al. (2022) is part of ERASMUS + project Robotics and STEM education for children and primary schools (Spain—coordinators, Sweden, Italy, and Cyprus). Also, in this case, actions aimed at teachers were implemented. Was developed “botSTEM developed a research- and evidence-based toolkit with teaching guidelines for teachers working with integrated STEM and scaffolded by robotics in preschool and primary school (children 4-8 years old)” (ivi, p. 2).
Discussion
In this research, we conducted a scoping review using three different strings to investigate three aspects of educational practices with ER in a broader way. We referred to teaching elements concerning computational thinking, training features on health, and the use of ER for special needs students. We studied the teaching experiences and the research conducted between 2016 and 2022. We used Scopus and Web of Science as databases and defined exclusion criteria to choose the papers to include in the review. Fifty-nine papers were selected and analyzed.
The analysis of the contributions suggested the need to foster a transdisciplinary approach in ER to transfer models and practices from different areas of research and technological development. As emerged from some of the contributions analyzed, models, protocols, and practices could guarantee an Evidence-Based Education (EBE) approach (Hattie and Marsh, 1996; Calvani et al., 2018) and strengthen the relationship and exchange between research and educational contexts. Consequently, “scientific knowledge should not descend from above, stifling practical wisdom and sensitivity to the socio-cultural context, but rather should help to give voice to them, thus contributing to the achievement of effective expert competence” (Calvani and Vivanet, 2014, p. 132, our translation).
Robotics and simulation technologies have proven to be worthy components of available educational resources. The use of these technologies in the educational environment has demonstrated their value in the everyday learning and specialized education of students with disabilities and health professionals caring for patients with learning or behavioral disorders (RECE project originated from this consideration confirmed by the review).
The studies from the first string (robot AND teaching AND “computational thinking”) identified some wellknown teaching methods in ER (such as project-based learning, problem-based learning, pair learning, learning by inquiry, and game-based learning) and a series of strategies to structure activities and propose exercises according to the identified framework of computational thinking with core concepts and skills that need a bigger deepening by an educational and methodological point of view. ER is used at all educational levels (from preschool to university) to teach programming, STEM, problem-solving, emotional intelligence, creativity, and teamwork. The whole element can be associated with computational thinking. A very high number of robots are listed in the research analyzed. Bee-bot and Blue-bot—tangible and mobile robots programmable through buttons—are the most frequently cited. Other programming platforms as Scratch, Blockly or MATLAB, robots—ozobot, Thymio, NUWA, mBot, BEAM, Kibo, and so on—and kits/systems (Makey-Makey, Lego Mindstorm, Arduino) can be retrieved in the experiences at the origin of the researches and need a more deepening. Unplugged activities (without technology) can be effective tools to acquire programming and computational thinking. Researches are conducted through qualitative and quantitative methods: experimental or quasi-experimental experiments, action research, design-based research with video analysis, content analysis, interview, observation, and rubrics represent some of the research tools provided.
The second string (robotics AND education AND health AND teaching) describes two different groups of evidence in using ER in the health sector. In a more evident way in this sector, as said in the results paragraph, robots can be a tool for education for health in formal and informal contexts or the objects of training for health professionals. So, also, in this case, ER appears diffused at all educational levels but with different aims. The robot Nao is cited in school experience, but more studies are necessary to identify other robots that can be used in the sector besides those used by physicians and professionals.
The results from the third string (robotics AND education AND special needs) highlight a fair diffusion of experimentation and research that shows a good synergy between research and educational contexts (school, training, teacher training). After the analysis conducted, it has been possible to identify the strengths and critical elements related, in particular, to the design of training courses aimed at teachers on educational robotics. This is one of the points to be developed within the RECE project. As said Bettencourt et al. (2021) “although research on the use of robotics as an effective tool to help children [e.g.] with ASD and other DNS is promising, these studies indicate that there are still significant limitations in this field that need to be overcome” (p. 7). In fact, many studies are based on very small samples. At the same time, “robotics is a perfect example where all the other fields of engineering and physics can contribute” (Potkonjak et al., 2016, p. 309).
The review gave us the possibility to collect some recommendations to consider during the design of educational activities in ER:
• Remember that robots can be a tool or a product of training, choose the right robot/environment according to educational goals, and also accept the idea of realizing unplugged activities using dolls, soft toys, cards, pictures, and maps;
• Choose the proper teaching methods among those usually performed in other disciplines (project-based learning, problem-based learning, pair learning, learning by inquiry, game-based learning) or among strategies according to the identified framework of computational thinking/computer science/health themes;
• Personalize er activities to realize particular categories of students, just as demotivated, disadvantaged, or gifted students with difficulties;
• Remember that probably involved teachers could need specific training on content, tools, and method in the experience;
• Define the role of adults/teachers: guide in the interaction or scaffolder in the activities and production of materials.
Despite our findings, we recognize that the study has several limitations. In the review, only two electronic databases, Scopus and Web of Sciences, were chosen. Because of the transdisciplinary aim of this scoping review, specific databases (i.e., PubMed) were excluded, and other “gray literature” database (i.e., Scholar) was not considered in order to avoid scattering of discourse, given the three already broad research topics. Additionally, we are aware that it would have been appropriate to use other keywords and combinations in the search to have more complete results: a deeper analysis is needed to explore robot features and computer science procedures.
Future research and activities can focus on:
• defining the connection in the teaching strategies between CT and Learning Analytics or Instructional Design, disciplines that have in common interdisciplinary approaches and skills and decomposition of complex tasks in simple actions;
• proposing interventions to children of kindergartens and primary schools together, working with students of secondary school and the degree in Digital Education as guides and scaffolders;
• Deepening the role of video in the training of physicians and as an observation tool;
• Investigating the differences in experiences for health professionals, medicine students, medical practitioners, and students.
Data availability statement
The original contributions presented in the study are included in the article/Supplementary material, further inquiries can be directed to the corresponding author/s.
Author contributions
The contribution represents the result of a joint work of the authors who collaborated in all the phases of the research work. KS wrote introduction—research background, educational robotics and special needs, methods, robotics, education, and special needs sections. AD wrote educational robotics and computational thinking and robot, teaching, and computational thinking sections. CB wrote educational robotics and health training and robotic, education, and health sections. TM is scientific co-responsible for the planning of the research. Discussion section is the result of the comparison between the authors. All authors contributed to the article and approved the submitted version.
Funding
This work was part of the robotics and E-Health: a challenge for education (RECE) research program funded by Department of Surgery, Medicine, Dentistry and Morphological Sciences with interest in Transplant, Oncology and Regenerative Medicine of the University of Modena and Reggio Emilia, Italy.
Acknowledgments
The authors acknowledge members of the RECE center for the sharing of ideas and experiences that gave us the possibility to think and study ER according to an interdisciplinary approach.
Conflict of interest
The authors declare that the research was conducted in the absence of any commercial or financial relationships that could be construed as a potential conflict of interest.
Publisher's note
All claims expressed in this article are solely those of the authors and do not necessarily represent those of their affiliated organizations, or those of the publisher, the editors and the reviewers. Any product that may be evaluated in this article, or claim that may be made by its manufacturer, is not guaranteed or endorsed by the publisher.
Supplementary material
The Supplementary Material for this article can be found online at: https://www.frontiersin.org/articles/10.3389/feduc.2022.955572/full#supplementary-material
References
Adorisio, O., Silveri, M., and Torino, G. (2021). Evaluation of educational value of YouTube videos addressing robotic pyeloplasty in children. J. Pediatr. Urol. 17, 390–e1. doi: 10.1016/j.jpurol.2021.02.009
Alcorn, A. M., Ainger, E., Charisi, V., Mantinioti, S., Petrovic, S, Schadenberg, B. R., et al. (2019). Educators' views on using humanoid robots with autistic learners in special education settings in England. Front. Robot. AI. 6, 107. doi: 10.3389/frobt.2019.00107
Alimisis, D. (2013). Educational robotics: open questions and new challenges. Themes Sci. Technol. Educ. 6, 63−71. Available online at: http://earthlab.uoi.gr/theste/index.php/theste/article/view/119
Angeli, C. (2022). The effects of scaffolded programming scripts on pre-service teachers' computational thinking: developing algorithmic thinking through programming robots. Int. J. Child Comput. Interaction 31, 100329. doi: 10.1016/j.ijcci.2021.100329
Angeli, C., and Valanides, N. (2020). Developing young children's computational thinking with educational robotics: an interaction effect between gender and scaffolding strategy. Comput. Hum. Behav. 105, 105954. doi: 10.1016/j.chb.2019.03.018
Anisimova, T. I., Latipova, L. N., Sergeeva, A. B., Sharafeeva, L. R., and Shatunova, O. V. (2017). Formation of teachers' readiness to work with technically gifted children. Modern J. Lang. Teach. Method. 7, 143–150. doi: 10.26655/mjltm.2017.9.1
Aniskin, V. N., Korostelev, A. A., Busygina, A. L., Kurochkin, A. V., and Sobakina, T. G. (2020). Teaching potential of integrated learning technologies Smart, Stem and Steam. Revista de la Universidad del Zulia. 11, 328–336. Available online at: https://elibrary.ru/item.asp?id=44935236
Anwar, S., Bascou, N. A., Menekse, M., and Kardgar, A. (2019). A systematic review of studies on educational robotics. J. Pre Coll. Eng. Educ. Res. J-PEER 9, 2. doi: 10.7771/2157-9288.1223
Ao, Y., and Yu, Z. (2022). Exploring the relationship between interactions and learning performance in robot-assisted language learning. Educ. Res. Int. 2022, 1958317. doi: 10.1155/2022/1958317
Arksey, H., and O'Malley, L. (2005). Scoping studies: towards a methodological framework. Int. J. Soc. Res. Methodol. 8, 19–32. doi: 10.1080/1364557032000119616
Bakala, E., Gerosa, A., Hourcade, J. P., and Tejera, G. (2021). Preschool children, robots, and computational thinking: A systematic review. Int. J. Child Comput. Interaction 29, 100337. doi: 10.1016/j.ijcci.2021.100337
Bargagna, S., Castro, E., Cecchi, F., Cioni, G., Dario, P., Dell'Omo, M., et al. (2019). Educational robotics in down syndrome: a feasibility study. Technol. Knowl. Learn. 24, 315–323. doi: 10.1007/s10758-018-9366-z
Berninger, V. W., Lee, Y. L., Abbott, R. D., and Breznitz, Z. (2013). Teaching children with dyslexia to spell in a reading-writers' workshop. Ann. Dyslexia 63, 1–24. doi: 10.1007/s11881-011-0054-0
Bers, M. U. (2019). Coding as another language: a pedagogical approach for teaching computer science in early childhood. J. Comput. Educ. 6, 499–528. doi: 10.1007/s40692-019-00147-3
Bettencourt, C., Grossard, C., Anzalone, S., Chetouani, M., and Cohen, D. (2021). Robotica e trattamento dei disturbi del neurosviluppo: revisione della letteratura. Prosp. Pediatr. 51, 1–9. Available online at: http://speapsl.aphp.fr/pdfpublications/2021/2021-19.pdf
Beulens, A. J. W., Brinkman, W. M., Porte, P. J., Meijer, R. P., Van Merriënboer, J. J., Van der Poel, H. G., et al. (2019b). The value of a 1-day multidisciplinary robot surgery training for novice robot surgeons. J. Robot Surg. (2018) 13:435–47. doi: 10.1007/s11701-018-0894-2
Beulens, A. J. W., Brinkman, W. M., Van der Poel, H. G., Vis, A. N., van Basten, J. P., Meijer, R. P., et al. (2019). Linking surgical skills to postoperative outcomes: a Delphi study on the robot-assisted radical prostatectomy. J. Robot. Surgery 13, 675–687. doi: 10.1007/s11701-018-00916-9
Boubaker, O. (2020). “Medical robotics,” in Control Theory in Biomedical Engineering, ed O. Boubaker (Elsevier), 153–204. doi: 10.1016/C2019-0-01984-X
Boya-Lara, C., Saavedra, D., Fehrenbach, A., and Marquez-Araque, A. (2022). Development of a course based on BEAM robots to enhance STEM learning in electrical, electronic, and mechanical domains. Int. J. Educ. Technol. High. Educ. 19, 1–23. doi: 10.1186/s41239-021-00311-9
Bray, L., Skubik-Peplaski, C., and Ackerman, K. B. (2021). A systematic review of the effectiveness of interventions to improve handwriting and spelling in children with specific learning disabilities. J. Occup. Ther. Schools Early Interv. 437–465. doi: 10.1080/19411243.2021.1934227
Calvani, A., Trinchero, R., and Vivanet, G. (2018). Nuovi orizzonti della ricerca scientifica in educazione. Raccordare ricerca e decisione didattica: il Manifesto S.Ap.IE. J. Educ. Cult. Psychol. Stud. ECPS J. 18, 311–339. doi: 10.7358/ecps-2018-018-cal1
Calvani, A., and Vivanet, G. (2014). Evidence based education e modelli di valutazione formativa per le scuole. J. Educ. Cult. Psychol. Stud. ECPS J. 1, 127–146. doi: 10.7358/ecps-2014-009-calv
Catlin, D., and Blamires, M. (2019). Designing robots for special needs education. Technol. Knowl. Learn. 24, 291–313. doi: 10.1007/s10758-018-9378-8
Chalmers, C. (2018). Robotics and computational thinking in primary school. Int. J. Child Comput. Interaction 17, 93–100. doi: 10.1016/j.ijcci.2018.06.005
Chen, G., Shen, J., Barth-Cohen, L., Jiang, S., Huang, X., and Eltoukhy, M. (2017). Assessing elementary students' computational thinking in everyday reasoning and robotics programming. Comput. Educ. 109, 162–175. doi: 10.1016/j.compedu.2017.03.001
Chevalier, M., Giang, C., El-Hamamsy, L., Bonnet, E., Papaspyros, V., Pellet, J. P., et al. (2022). The role of feedback and guidance as intervention methods to foster computational thinking in educational robotics learning activities for primary school. Comput. Educ. 180:104431. doi: 10.1016/j.compedu.2022.104431
Childs, B. S., Manganiello, M. D., and Korets, R. (2019). Novel education and simulation tools in urologic training. Current urology reports, 20, 1-8. doi: 10.1007/s11934-019-0947-8
Chootongchai, S., Songkram, N., and Piromsopa, K. (2021). Dimensions of robotic education quality: Teachers' perspectives as teaching assistants in Thai elementary schools. Educ. Infm. Technol. 26, 1387–1407. doi: 10.1007/s10639-019-10041-1
Computer Science Teachers Association (CSTA) and the Int. Society for Technology in Education (ISTE) (2011). Computational Thinking, Leadership Toolkit, 1st edn. Available online at: https://cdn.iste.org/www-root/2020-10/ISTE_CT_Leadership_Toolkit_booklet.pdf
Cornoldi, C., Capodieci, A., Colomer Diago, C., Miranda, A., and Shepherd, K. G. (2018). Atteggiamenti degli insegnanti di scuola primaria in tre paesi occidentali verso i disturbi dell'apprendimento. J. Learn. Disabil. 51, 43–54. doi: 10.1177/0022219416678408
Critten, V., Hagon, H., and Messer, D. (2021). Can pre-school children learn programming and coding through guided play activities? A case study in computational thinking. Early Childhood Educ. J. 50, 1–13. doi: 10.1007/s10643-021-01236-8
Diago, P. D., González-Calero, J. A., and Yáñez, D. F. (2021). Exploring the development of mental rotation and computational skills in elementary students through educational robotics. Int. J. Child Comput. Interaction 32:100388. doi: 10.1016/j.ijcci.2021.100388
Díaz-Lauzurica, B., and Moreno-Salinas, D. (2019). Computational thinking and robotics: a teaching experience in compulsory secondary education with students with high degree of apathy and demotivation. Sustainability 11, 5109. doi: 10.3390/su11185109
El-Hamamsy, L., Chessel-Lazzarotto, F., Bruno, B., Roy, D., Cahlikova, T., Chevalier, M., et al. (2021). A computer science and robotics integration model for primary school: evaluation of a large-scale in-service K-4 teacher-training program. Educ. Infm. Technol. 26, 2445–2475. doi: 10.1007/s10639-020-10355-5
Esteve-Mon, F. M., Adell-Segura, J., Llopis Nebot, M. Á., Valdeolivas Novella, M. G., and Pacheco Aparicio, J. (2019). The development of computational thinking in student teachers through an intervention with educational robotics. J. Inf. Technol. Educ. Innov. Pract. 18, 139–152. doi: 10.28945/4442
European Agency for Special Needs and Inclusive Education. (2021). Key Principle-Supporting Policy Development and Implementation for Inclusive Education, eds V. J. Donnelly and A. Watkins. Odense: European Agency for Special Needs Inclusive Education. Available online at: https://www.european-agency.org/resources/publications/keyprinciples-supporting-policy-development-implementation
European Agency for Special Needs and Inclusive Education. (2022a). Profile for Inclusive Teacher Professional Learning: Including All Education Professionals in Teacher Professional Learning for Inclusion, eds A. De Vroey, A. Lecheval, and A. Watkins. Odense: European Agency for Special Needs and Inclusive Education.
European Agency for Special Needs and Inclusive Education. (2022b). Voices into Action-The Voices of Learners and their Families in Educational Decision-Making: Literature Review, eds D. C. Murdoch, A. Mangiaracina, and A. Kefallinou. Odense: European Agency for Special Needs and Inclusive Education.
Fridberg, M., and Redfors, A. (2021). Teachers' and children's use of words during early childhood STEM teaching supported by robotics. Int. J. Early Years Educ. 29, 1–15. doi: 10.1080/09669760.2021.1892599
Fridberg, M., Redfors, A., Greca, I. M., and Terceño, E. M. G. (2022). Spanish and Swedish teachers' perspective of teaching STEM and robotics in preschool-results from the botSTEM project. Int. J. Technol. Design Educ. 2022, 1–21. doi: 10.1007/s10798-021-09717-y
Funk, M. A., Cascalho, J. M., Santos, A. I., and Mendes, A. B. (2021). Educational robotics and tangible devices for promoting computational thinking. Front. Robot. AI 340. doi: 10.3389/frobt.2021.713416
Green, C. A., Mahuron, K. M., Harris, H. W., and O'Sullivan, P. S. (2019). Integrating robotic technology into resident training: challenges and recommendations from the front lines. Acad. Med. J. Assoc. Am. Med. Colleg. 94, 1532. doi: 10.1097/ACM.0000000000002751
Harris, C. A., Guerri, L., Mircic, S., Reining, Z., Amorim, M., Jovi, et al. (2020). Neurorobotics workshop for high school students promotes competence and confidence in computational neuroscience. Front. Neurorobot. 14, 6. doi: 10.3389/fnbot.2020.00006
Hattie, J., and Marsh, H. W. (1996). The relationship between research and teaching: A meta-analysis. Rev. Educ. Res. 66, 507–542. doi: 10.3102/00346543066004507
Hsieh, M. C., Pan, H. C., Hsieh, S. W., Hsu, M. J., and Chou, S. W. (2021). Teaching the concept of computational thinking: a STEM-based program with tangible robots on project-based learning courses. Front. Psychol. 12, 828568. doi: 10.3389/fpsyg.2021.828568
Hughes-Roberts, T., Brown, D., Standen, P., Desideri, L., Negrini, M., Rouame, A., et al. (2019). Examining engagement and achievement in learners with individual needs through robotic based teaching sessions. Br. J. Educ. Technol. 50, 2736–2750. doi: 10.1111/bjet.12722
Hung, A. J., Chen, J., Shah, A., and Gill, I. S. (2018). Telementoring and telesurgery for minimally invasive procedures. J. Urol. 199, 355–369. doi: 10.1016/j.juro.2017.06.082
Istituto Nazione di statistica—ISTAT (2022). L'inclusione Scolastica Degli Alunni con Disabilità | A.S. 2020–2021. Available online at: https://www.istat.it/it/archivio/251409
Jamal, N. N., Jawawi, D. N., Hassan, R., and Mamat, R. (2021). Conceptual model of learning computational thinking through educational robotic. Int. J. Emerg. Technol. Learn. 16, 91–106. doi: 10.3991/ijet.v16i15.24257
Jawawi, D., Jamal, N., Halim, S. A., Sa'adon, N., Mamat, R., Isa, M., et al. (2022). Nurturing secondary school student computational thinking through educational robotics. Int. J. Emerg. Technol. Learn. iJET 17, 117–128. doi: 10.3991/ijet.v17i03.27311
Khalil, H., Peters, M., Godfrey, C. M., McInerney, P., Soares, C. B., and Parker, D. (2016). An evidence-based approach to scoping reviews. Worldviews Evid. Based Nurs. 13, 118–123. doi: 10.1111/wvn.12144
Kun, Y., Hubert, J., Bin, L., and Huan, W. X. (2019). Self-debriefing model based on an integrated video-capture system: an efficient solution to skill degradation. J. Surg. Educ. 76, 362–369. doi: 10.1016/j.jsurg.2018.08.017
Levac, D., Colquhoun, H., and O'Brien, K. K. (2010). Scoping studies: advancing the methodology. Impl. SCI. 5, 1–9. doi: 10.1186/1748-5908-5-69
Lindsay, S. (2020). Exploring skills gained through a robotics program for youth with disabilities. OTJR Occup. Participat. Health. 40, 57–63. doi: 10.1177/1539449219868276
Merino-Armero, J. M., González-Calero, J. A., Cózar-Gutiérrez, R., and del Olmo-Muñoz, J. (2022). Unplugged activities in cross-curricular teaching: effect on sixth graders' computational thinking and learning outcomes. Multimod. Technol. Interaction 6, 13. doi: 10.3390/mti6020013
Montes, N., Rosillo, N., Mora, M. C., and Hilario, L. (2021). A novel real-time MATLAB/Simulink/LEGO EV3 platform for academic use in robotics and computer science. Sensors 21, 1006. doi: 10.3390/s21031006
Negrini, L. (2020). Teachers' attitudes towards educational robotics in compulsory school. Ital. J. Educ. Technol. 28, 77–90. doi: 10.17471/2499-4324/1136
Neophytou, R., and Eteokleous, N. (2022). “The impact of the professional development training of primary education teachers on issues of educational robotics as a learning tool,” in Designing, Constructing, and Programming Robots for Learning (pp. 69–89). IGI Global. doi: 10.4018/978-1-7998-7443-0.ch004
Papadakis, S., Vaiopoulou, J., Sifaki, E., Stamovlasis, D., and Kalogiannakis, M. (2021). Attitudes towards the use of educational robotics: Exploring pre-service and in-service early childhood teacher profiles. Educ. Sci. 11, 204. doi: 10.3390/educsci11050204
Peters, M. D., Marnie, C., Tricco, A. C., Pollock, D., Munn, Z., Alexander, L., et al. (2020a). Updated methodological guidance for the conduct of scoping reviews. JBI Evid. Synth. 18, 2119–2126. doi: 10.11124/JBIES-20-00167
Peters, M. D. J., Godfrey, C., McInerney, P., Munn, Z., Tricco, A. C., and Khalil, H. (2020b). “Scoping reviews,” in JBI Manual for Evidence Synthesis, eds. E. Aromataris, and Z. Munn (JBI). Available online at: https://synthesismanual.jbi.global
Peters, M. D. J., Godfrey, C. M., Khalil, H., McInerney, P., Parker, D., and Soares, C. B. (2015). Guidance for conducting systematic scoping reviews. Int. J. Evid. Based Healthc. 13, 141–146. doi: 10.1097/XEB.0000000000000050
Peters, M. D. J., Marnie, C., Colquhoun, H., Garritty, C. M., Hempel, S., Horsley, T., et al. (2021). Scoping reviews: reinforcing and advancing the methodology and application. Syst. Rev. 10, 263. doi: 10.1186/s13643-021-01821-3
Potkonjak, V., Gardner, M., Callaghan, V., Mattila, P., Guetl, C., Petrovi, V. M., et al. (2016). Virtual laboratories for education in science, technology, and engineering: A review. Comput. Educ. 95, 309–327. doi: 10.1016/j.compedu.2016.02.002
Puliatti, S., Amato, M., Farinha, R., Paludo, A., Rosiello, G., De Groote, R. G., et al. (2022b). Does quality assured eLearning provide adequate preparation for robotic surgical skills; a prospective, randomized and multi-center study. Int. J. Comput. Assist. Radiol. Surg. 17, 1–9. doi: 10.1007/s11548-021-02545-4
Puliatti, S., Amato, M., Mazzone, E., Rosiello, G., De Groote, R., Berquin, C., et al. (2022a). Development and validation of the metric-based assessment of a robotic dissection task on an avian model. J. Surg. Res. 277, 224–234 doi: 10.1016/j.jss.2022.02.056
Robaczewski, A., Bouchard, J., Bouchard, K., and Gaboury, S. (2021). Socially assistive robots: the specific case of the NAO. Int. J. Soc. Robot. 13, 795–831. doi: 10.1007/s12369-020-00664-7
Rosi, A., Dall'Asta, M., Brighenti, F., Del Rio, D., Volta, E., Baroni, I., et al. (2016). The use of new technologies for nutritional education in primary schools: a pilot study. Public Health 140, 50–55. doi: 10.1016/j.puhe.2016.08.021
Sannicandro, K. (2013). “Didattica e disturbi specifici dell'apprendimento: proposte operative per i docenti,” in E-learning e Risorse Didattiche Online per la Formazione Continua Degli Insegnanti sui DSA, eds R. Pace (Bari: Progedit), pp. 52–72.
Sannicandro, K. (2017). Tecnologie Compensative e Didattiche: possibilità e criticità. Progettare percorsi didattici, risorse digitali e buone prassi. Scuola Italiana Moderna. Brescia: La Scuola.
Santos, E. A., and Lopes, L. G. (2020). Learning programming with robots: a study on students' participation in a virtual community of practice. Univ. J. Educ. Res. 8, 313–320. doi: 10.13189/ujer.2020.080138
Scaradozzi, D., Screpanti, L., Cesaretti, L., Storti, M., and Mazzieri, E. (2019). Implementation and assessment methodologies of teachers' training courses for STEM activities. Technol. Knowl. Learn. 24, 247–268. doi: 10.007/s10758-018-9356-1
Schina, D., Esteve-González, V., and Usart, M. (2021). An overview of teacher training programs in educational robotics: characteristics, best practices and recommendations. Educ. Inf. Technol. 26, 2831–2852. doi: 10.1007/s10639-020-10377
Sen, C., Ay, Z. S., and Kiray, S. A. (2021). Computational thinking skills of gifted and talented students in integrated STEM activities based on the engineering design process: The case of robotics and 3D robot modeling. Think. Skills Creat. 42, 100931. doi: 10.1016/j.tsc.2021.100931
Shim, J. S., Kim, J. Y., Pyun, J. H., Cho, S., Oh, M. M., Kang, S. H., et al. (2018). Comparison of effective teaching methods to achieve skill acquisition using a robotic virtual reality simulator: Expert proctoring versus an educational video versus independent training. Medicine 97. doi: 10.1097/MD.0000000000013569
Shire, K. A., Hill, L. J., Snapp-Childs, W., Bingham, G. P., Kountouriotis, G. K., Barber, S., et al. (2016). Robot guided ‘Pen Skill' training in children with motor difficulties. PloS ONE 11, 1–14. doi: 10.1371/journal.pone.0151354
Tengler, K., and Sabitzer, B. (2022). Examining teachers' intention to integrate robotics-based storytelling activities in primary schools. Int. J. Interactive Mob. Technol. 16, 221–240. doi: 10.3991/ijim.v16i06.28905
Tessaro, F. (2011). Formazione alla ricerca attraverso la ricerca nell'inclusive education. formazione and insegnamento. Rivista Internazionale di Scienze Dell'educazione e Della Formazione 9, 115–122.
Trigo, M. J. G., Standen, P. J., and Cobb, S. V. G. (2019). Robots in special education: reasons for low uptake. J. Enabl. Technol. 13, 59–69. doi: 10.1108/JET-12-2018-0070
UNESCO Division of Philosophy and Ethics (1998). Transdisciplinarity—“Stimulating Synergies, Integrating Knowledge”. Available onlien at: https://unesdoc.unesco.org/ark:/48223/pf0000114694
UNESCO Global Education Monitoring Report (2021). Central and Eastern Europe, Caucasus and Central Asia: Inclusion and education: All means all. Paris: UNESCO. Available online at: en.unesco.org/gem-report/eurasia2021inclusion
Xia, L., and Zhong, B. (2018). A systematic review on teaching and learning robotics content knowledge in K-12. Comput. Educ. 127, 267–282. doi: 10.1016/j.compedu.2018.09.007
Yang, K., Liu, X., and Chen, G. (2020a). The influence of robots on students' computational thinking: a literature review. Int. J. Inf. Educ. Technol. 10, 5. doi: 10.18178/ijiet.2020.10.8.1435
Yang, K., Liu, X., and Chen, G. (2020b). Global research trends in robot education in 2009-2019: a bibliometric análisis. Int. J. Inf. Educ. Technol. 10, 476–481. doi: 10.18178/ijiet.2020.10.6.1410
Yu, N., Saadat, H., Finelli, A., Lee, J. Y., Singal, R. K., Grantcharov, T. P., et al. (2021). Quantifying the “assistant effect” in robotic-assisted radical prostatectomy (RARP): measures of technical performance. J. Surg. Res. 260, 307–314. doi: 10.1016/j.jss.2020.11.037
Keywords: educational robotics, scoping review, special needs, inclusive education, teaching, computational thinking, robotic healthcare, digital education
Citation: Sannicandro K, De Santis A, Bellini C and Minerva T (2022) A scoping review on the relationship between robotics in educational contexts and e-health. Front. Educ. 7:955572. doi: 10.3389/feduc.2022.955572
Received: 28 May 2022; Accepted: 30 September 2022;
Published: 02 November 2022.
Edited by:
Giovanni Bonaiuti, University of Cagliari, ItalyReviewed by:
Charles R. Doarn, University of Cincinnati, United StatesJoão Piedade, University of Lisbon, Portugal
Copyright © 2022 Sannicandro, De Santis, Bellini and Minerva. This is an open-access article distributed under the terms of the Creative Commons Attribution License (CC BY). The use, distribution or reproduction in other forums is permitted, provided the original author(s) and the copyright owner(s) are credited and that the original publication in this journal is cited, in accordance with accepted academic practice. No use, distribution or reproduction is permitted which does not comply with these terms.
*Correspondence: Katia Sannicandro, a2F0aWEuc2FubmljYW5kcm9AdW5pbW9yZS5pdA==