- Physical Education and Sports Science Academic Group, National Institute of Education, Nanyang Technological University, Singapore, Singapore
This paper illustrates how movement analysis could be performed using publicly available videos and freeware to generate meaningful information for sports practitioners and researchers. Using acrobatic sports as a case, we performed kinematic analysis on 206 YouTube videos of high-level competitions in diving and gymnastics using Kinovea. Results revealed good to excellent inter-rater reliability of variables analyzed. Significant differences in angular speed (p < 0.001, η2p = 0.213) and flight time (p < 0.001, η2p = 0.928) were found among eight different events. Divers had longer flight time (p < 0.001, η2p = 0.569) and were somersaulting faster than gymnasts (p = 0.021, η2p = 0.026). Angular speed was higher in tuck than pike somersaults (p < 0.001, η2p = 0.214). Shorter the flight time was significantly correlated with faster angular speed (rho = −0.533, p < 0.001) in gymnastics events. Coaches and scientists can consider applying the proposed method to monitor the athletes’ performance and to identify errors (e.g., insufficient flight time). The kinematics measurements can also be used to guide the transition plan across different apparatus and categories (e.g., 10-m platform to 3-m springboard). In conclusion, the present study highlights the potential of using readily available information and open-source freeware to generate scientific data for sports applications. Such data analysis approach can accommodate a wide range of video qualities, is easily accessible, and not restricted by situations such as social distancing, quarantine, lockdown or other restrictive measures.
Introduction
In the field of sports science, biomechanical analysis of athletes’ movements can provide useful information for coaches, scientists and athletes to evaluate performance, identify errors, and develop training and competition plans (Ae, 2020; Barbosa et al., 2021). To obtain detailed and accurate movement data, athletes are often tested comprehensively in a biomechanical laboratory using expensive and advanced equipment such as multi-camera 3D motion capture systems and force platforms (Ferdinands et al., 2009; Augustus et al., 2021). COVID-19 and the associated restrictions impose great challenge to sports (Bobo-Arce et al., 2021). Many laboratories around the world were closed for a sustained period of time. Others required users to adhere to strict safe management measures such as limiting the number of people per group, maintaining social distancing, and avoiding physical contact. These restrictions make it difficult if not impossible to perform standard biomechanical movement analysis in the laboratory. Fortunately, there are alternative solutions to perform meaningful movement analysis in sports (Morgulev et al., 2018; Pan et al., 2021). This paper presents a method that makes use of publicly available videos and free software to conduct biomechanical movement analysis. When using publicly available videos, the researchers have no control over the video recording process such as camera position, sampling rate, shutter speed and field of view. The wide range of video qualities imposes challenges on movement analysis, for example, blurry images due to slow shutter speeds and temporal errors associated with low frame rate. Despite these technical difficulties, it is yet possible to perform remote video analysis by carefully selecting variables that can be determined with good confidence even when the videos were taken from different sources with sub-optimal qualities. The present study will illustrate a practical data analysis approach that can accommodate a wide range of videos of varied qualities.
To demonstrate the idea of video-based movement analysis, a case of acrobatic sports was selected. Acrobatic sports athletes such as divers and gymnasts performed highly skilled moves across different events (e.g., platform, springboard, floor, and gymnastic apparatus) in specialized competition venues (e.g., gymnasium and diving pool) (King and Yeadon, 2004; Vladimir et al., 2015; Sayyah et al., 2018, 2020; King et al., 2019, 2022). While great efforts have been made to develop inertial measurement units for on-water data collection (Walker et al., 2017, 2019), kinematic information of diving movements are mostly obtained from videos recorded at diving pools (Sayyah et al., 2018, 2020; King et al., 2019, 2022). Similarly in gymnastics, video-based analysis has been a useful means to assess movement quality, provide feedback and inform training plans (Mizutori et al., 2021; Fujihara, 2022). Analyzing publicly available competition videos is a promising approach to offer insights into these very difficult moves that are approaching human limit. At high-level international competitions, it is interesting to observe that acrobatic sports athletes perform similar somersaulting moves despite competing in different sport events. For example, triple back somersaults are seen in floor routines and dismounts from apparatus in artistic gymnastics as well as the armstand dive group from the 10-m platform. Although the numbers of rotation appear similar, one cannot assume that the biomechanical characteristics of these moves are the same for divers and gymnasts. Factors that can influence the somersaulting speed may include the approach speed, compliance and height of the take-off and landing surfaces, flight time available to complete the required number of somersaults, body shape, and moment of inertia of the athlete while somersaulting (Hamill et al., 1986; Gronbech, 1993; Miller and Sprigings, 2001; Cheng and Hubbard, 2008; Kong, 2010; Mkaouer et al., 2013; Mikl, 2018). Previous biomechanical studies on acrobatic sports typically reported detailed kinematics of one move (Vladimir et al., 2015; Park and Yoon, 2017; Walker et al., 2019) or comparing across a few somersaulting moves (Sanders and Gibson, 2003; Walker et al., 2017; King et al., 2022) within a single sport. To the authors’ best knowledge, no studies have compared the somersaulting moves performed by athletes across different acrobatic sports events.
The purpose of this study was to use acrobatic sports as a case to illustrate a video-based movement analysis approach using open-source freeware to perform meaningful analysis from publicly available footages of varied qualities. In specific, key biomechanical variables of divers and gymnasts when performing similar acrobatic moves in real competition settings would be compared. Findings from this study can help us better understand the mechanical demand of the highly difficult skills and the transferability of skills in acrobatic sports. Such information may also be useful for coaches and sports scientists to develop training plan and select movements for competitions. The proposed data analysis approach has economic and practical benefits for scientists, coaches and athletes because the measurements can potentially be performed by anyone in any location, without the need to access specialized and expensive facilities, equipment or software.
Materials and Methods
This study was approved by the Nanyang Technological University Institutional Review Board (Protocol Number: IRB-2021-02-029). Publicly available videos of from high-level diving and gymnastics competitions in the past 6 years were retrieved for kinematics analysis.
Selection of Video
To systematically retrieve videos for analysis, a set of pre-set criteria should be developed. These criteria should be objective and clearly defined such that other analysts can repeat the search if they follow the described methods. In the present study, the acrobatic sports videos were included for analyses if they fulfilled all the following criteria:
(1) Publicly available on the YouTube site, uploaded by the competition organizers (e.g., Olympics YouTube Channel) and/or the international federation of diving or gymnastics [e.g., Fédération Internationale de Natation (FINA), Fédération Internationale de Gymnastique (FIG)].
(2) Athletes performed either a 3.0 or 3.5 back somersault move in a tuck or pike position without any twists in the diving or gymnastics competitions. These moves were considered very difficult moves that were near the athletes’ limits. We did not limit the move to only the triple somersault because divers do not usually land feet first at high level competition. In the backward dive group, divers typically perform 3.5 somersaults and entered the water with their hands first to minimize splash.
(3) The move had to be executed in a high-level competition setting (e.g., Olympic Games, world championships, European championships, British Gymnastics Championships, United States Gymnastics Championships, China Diving Star, and China National Games).
(4) The competitions took place in the past 6 years (2016–2021).
(5) The videos must be in normal time and not slow motion.
For diving videos, the search terms on the YouTube site included dive number “207C” (backward 3.5 somersaults in tuck), “207B” (backward 3.5 somersaults in pike), “626C” (arm stand 3.0 somersault in tuck), “626B” (arm stand 3.0 somersault in pike), followed by the term “diving”. For gymnastics videos, the terms used were “triple somersault,” “triple back tuck,” “triple back pike,” followed by the term “gymnastics.” In addition, the search also included terms related to official high-level competitions such as “Olympics Gymnastics,” “Olympics Diving,” “World Championship Gymnastics,” “FINA Diving,” and “Diving World Cup.” The selected videos consisted of two categories in diving – 3-m springboard and 10-m platform (backward group and armstand group), and five categories in gymnastics – floor exercise, rings, horizontal (high) bar, double-mini trampoline, and tumbling. Both male and female athletes were included in the video search process.
In the present study, a tuck position was considered when there is hip and knee flexion whereby the knees should be bent to a maximum range and pulled toward the chest (Mitchell et al., 2002). A pike position was considered when there is hip flexion (less than 90°) and straight legs, and this is also known as a closed pike position (Mitchell et al., 2002). If the athlete attempted to somersault in a pike position but bent the knees midway, the video would be excluded from the analysis because no distinct body position could be identified. Every eligible video was extracted from YouTube through screen recording at 30 Hz through these two software – OBS Studio 27.0.1, Xbox Game Bar 5.721.6282.0. This allows slow motion viewing, frame-to-frame navigation, and kinematic analysis.
Biomechanical Analysis
Biomechanical analysis of the extracted videos was performed using the Kinovea software (version 0.8.15, available for download at: http://www.kinovea.org). Kinovea is an open source software under GPLv2 license for 2D motion analysis. It can be downloaded for free, and is widely used in the field of kinematic analyses in sports (Nor Adnan et al., 2018). This software has been demonstrated to show good accuracy in measuring angles and distances (Puig-Diví et al., 2019). Specific to acrobatic sports, excellent intra-rater and inter-rater reliability was found in angle measurements and identification of key events in dynamic gymnastics movements (Khong and Kong, 2016). Moreover, the authors reported good agreement among three independent raters with varying levels of gymnastics experience suggesting that 2D video analysis can be readily adopted by users regardless of their personal experience in acrobatic sport.
When selecting biomechanical variables to be analyzed, we need to consider the recording rate, camera position and scaling factors. Temporal or timing variables can be easily obtained as long as the key events (e.g., takeoff and landing) can be visually seen from the videos. The accuracy of timing data will depend on the video recording frame rates (Tay and Kong, 2018). Angular kinematic measurements (e.g., angle and angular speed) require the movement plane to lie perpendicular to the optical axis of the camera (Tay and Kong, 2018). Any out-of-plane angle measurements would result in perspective errors. Linear kinematic variables (e.g., displacement and velocity) are the most difficult ones since calibration procedures must be performed to obtain appropriate scaling factors. In some sports, the known dimensions of equipment (e.g., billiards table) can be used for calibration purposes (Pan et al., 2021).
In the present study on acrobatic sports, there are technical challenges associated with the online videos which were captured under different camera settings with varied qualities. First, the cameras were moving to follow the athletes and that no consistent calibration objects were available. Second, the cameras were not always positioned perpendicular to the movement plane and therefore angle measurements would be subjected to perspective errors. Third, most videos were recorded at low sampling rate and slow shutter speeds. This temporal limitation restricted us from analyzing frame-by-frame instantaneous kinematics such as joint angle-time history or peak angular speed. Having consider these technical challenges, the present study proposed to measure flight time and average angular speed of the somersaulting moves. Flight time is a temporal variable which does not require calibration. Compared with instantaneous kinematic measurements (e.g., maximum hip flexion angle, peak angular speed), taking the average angular speed over a longer period of time would be more accurate because this method was less affected by the temporal errors associated with low frame rate. We believe that flight time and average angular speed information can be determined with good confidence across videos of varied qualities to facilitate remote analysis of acrobatic sports. Details of each variable are described below.
Flight Time
Flight time was defined as the duration of the athlete in the air (Figure 1). It started from last video frame that any part of the athlete’s body is in contact with the take-off surface (springboard, platform, floor, and tumbling track) or apparatus (rings, high bar, and mini trampoline) before somersaulting in the air, to the first video frame that the athletes’ hands breaking the water (diving) or feet touching the landing surfaces (gymnastics).
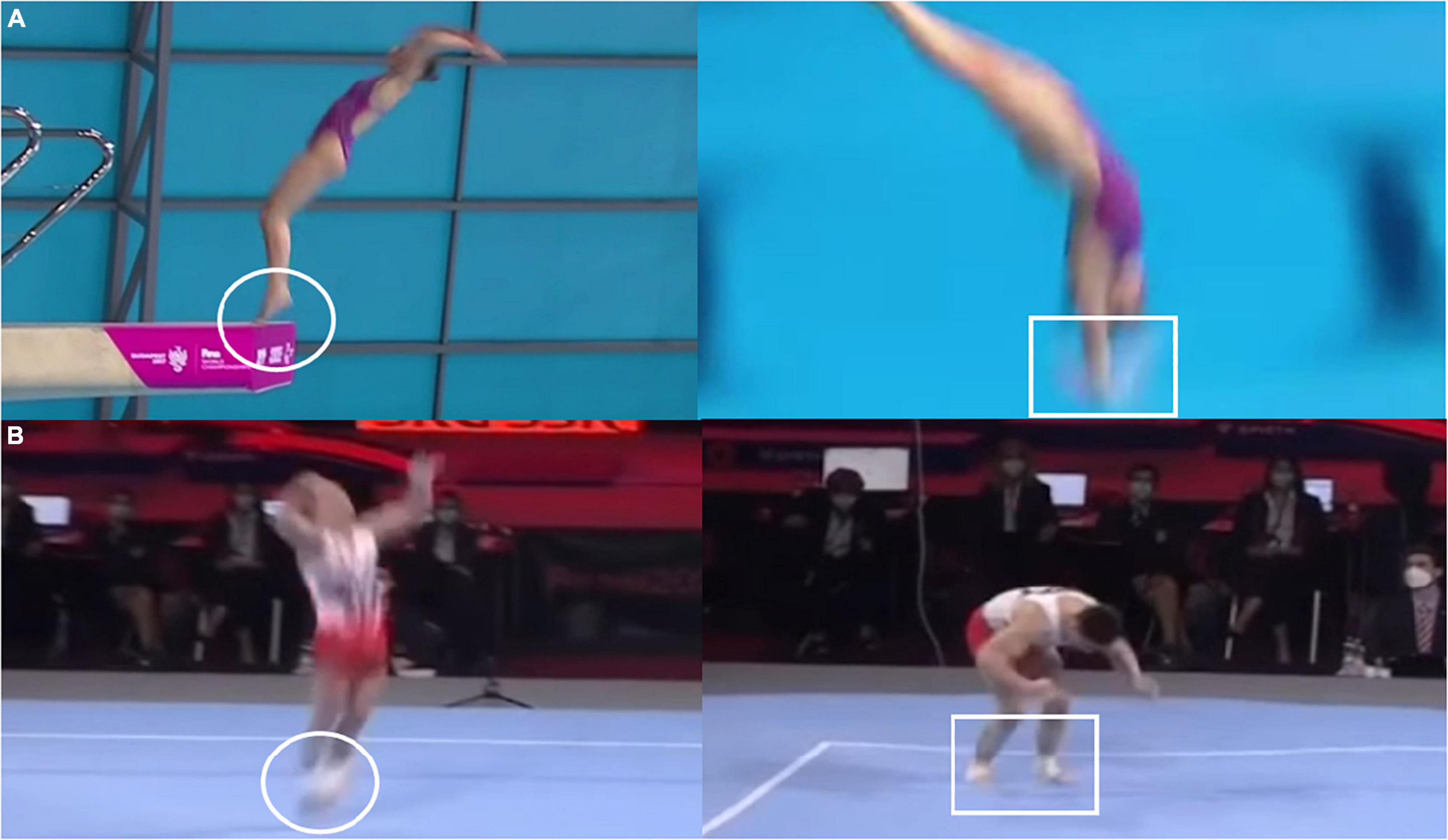
Figure 1. An example of measurement of flight time in (A) diving and (B) gymnastics. The start of flight time is denoted by the circle, while the end of flight time is denoted by the rectangle.
Average Angular Speed
Previous studies illustrated that when divers performed multiple somersaulting dives, the angular speed increased sharply in the beginning of the flight phase, remained fairly unchanged in the somersault phase when a tight body position was formed, and decreased toward the end when the body extended from the tight somersault position (Walker et al., 2017, 2019). In the present study, the average angular speed was quantified while the athlete was somersaulting in a tight and rigid body position with minimal joint flexion or extension movements. While there are variations in the way how divers and gymnasts hold a tuck or pike position (Mikl, 2018), we considered a tight and rigid position was assumed when the athlete pulled the legs closest to the chest during the rotation. Once the athlete loosened from the tight tuck or pike position and started to extend the body, the tight and rigid body was no longer assumed. Among all back somersaulting videos retrieved for analysis, the tight and rigid body position was commonly seen during or slightly after the completion of the first 360° rotation. A straight line passing through the athlete’s hip and knee joints were drawn to represent the orientation of the athlete in first and last frame of the tight and rigid shape duration (Figure 2). Based on these two lines, the total degree of rotation that the athlete performed were measured using the angle tool in the Kinovea software.
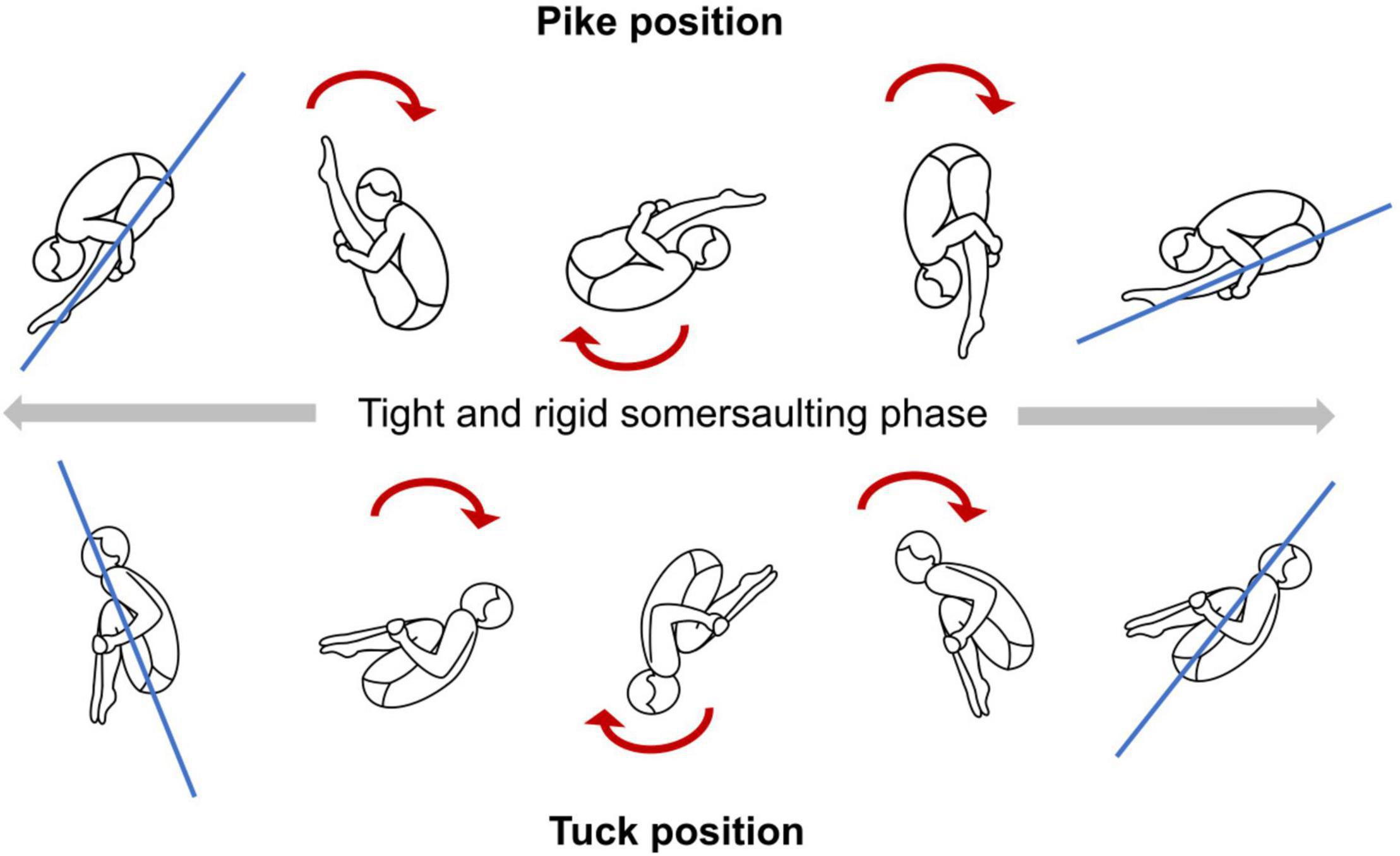
Figure 2. A straight line passing through the hip and knee joints of the athletes was drawn in indicate the orientation of the athlete in the beginning and end of the tight and rigid somersaulting phase.
The average angular speed was calculated as the total degrees of rotation divided by the time in which the athlete held a tight and rigid body position. In some tumbling videos, the cameras were not placed perpendicular to the movement plane, and hence measuring angles from these camera views would lead to prospective errors. To overcome this limitation, we determined the time required for the athlete to complete either 0.5, 1.0, or 1.5 somersaults in a tight and rigid body position. We then use the known degrees of rotation of 180°, 360°, or 540° in the computation of angular speed to avoid the perspective errors associated with camera position.
Video analyses were performed by two raters under the guidance of the senior author who has a Ph.D. in sports biomechanics and competitive background in springboard diving. All gymnastics videos were analyzed by rater 1 who has a bachelor’s degree in sports science and 10 years of competitive background in artistic gymnastics. All diving videos were processed by rater 2 who has a bachelor’s degree in sports science, 4 years of competitive background in artistic gymnastics, and 1 year of competitive background in cheerleading.
Statistical Analysis
A total of 206 videos (105 diving and 101 gymnastics) were retrieved, including 150 in tuck and 56 in pike positions (Figure 3). The athletes took off from their feet in most moves (75 diving and 95 gymnastics), with 30 armstand dives and 6 dismounts from apparatus. All divers entered the water with their hands first while all gymnasts landed the triple somersaults on their feet.
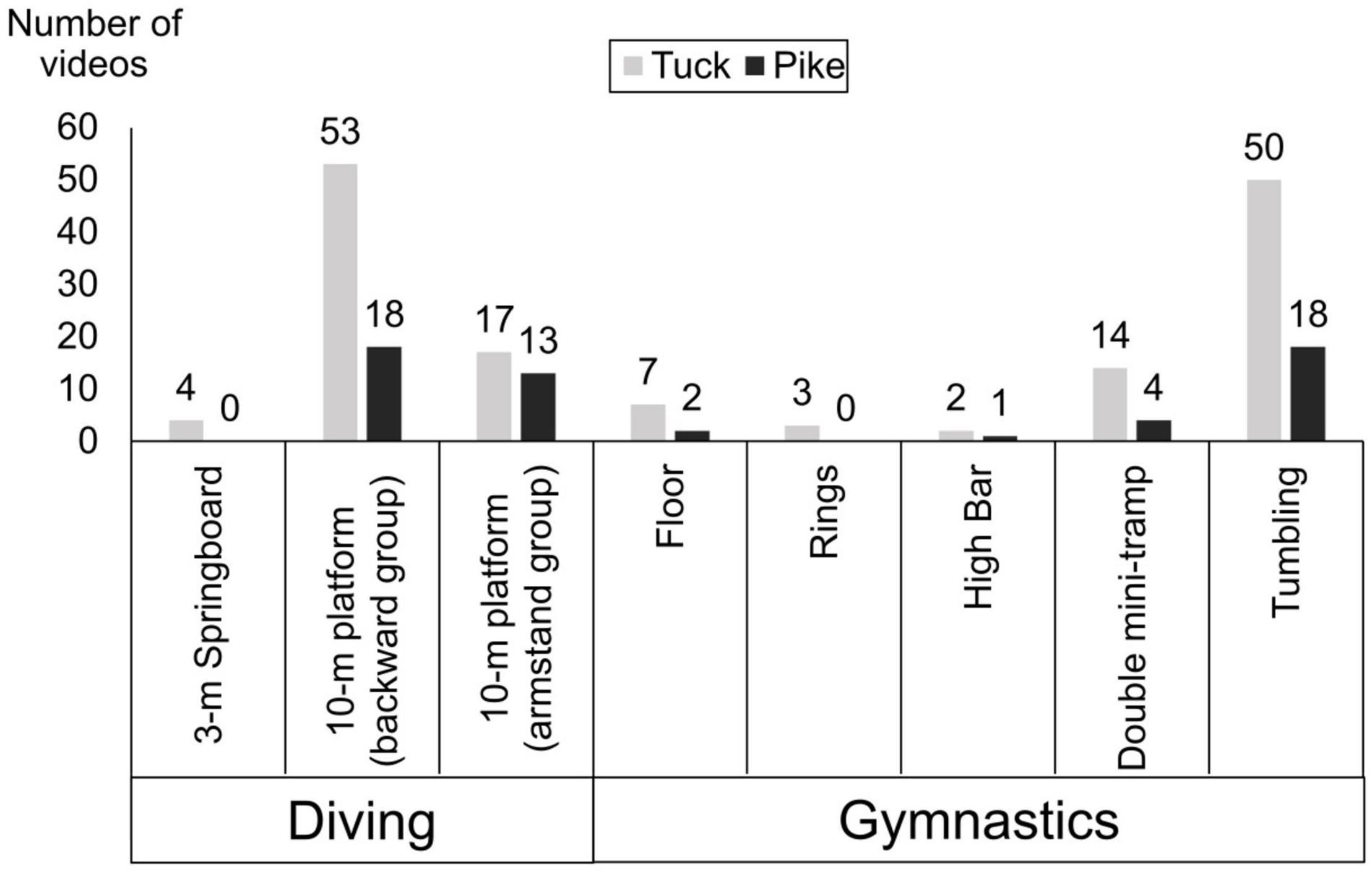
Figure 3. Number of tuck and pike multiple (3.0 or 3.5) back somersault moves in competitive diving (n = 105) and gymnastics (n = 101) videos retrieved in years 2016–2021 (total = 206 video).
All gymnastics triple somersaults were performed by male gymnasts and hence no female data were available. In diving, there were only four female divers performing backward 3.5 somersaults from the 10-m platform. Since the flight time (1.40–1.74 s) and angular speed (831°/s to 1169°/s) of these four female divers fell within the range of those in their male counterparts (flight time: 1.39–1.80 s; angle speed: 818°/s to 1,200°/s), all divers’ data were analyzed as a group regardless of sex.
To check the inter-rater reliability, rater 1 and rater 2 independently analyzed a sub-sample of 34 videos (17 diving and 17 gymnastics). The intraclass correlation coefficients (ICC) of average angular speed and flight time was calculated using IBM SPSS Statistics for Windows (Version 27.0. Armonk, NY, United States: IBM Corp.). Subsequently, the standard error of measurements (SEM) of each variable were computed.
For the main dataset, JASP (version 0.14.1) was used for statistical analysis with significance level set at p < 0.05. The two outcome variables were angular speed and flight time. As the data were not normally distributed, descriptive data are expressed as median [interquartile range (IQR)]. Data were log transformed prior to running Analysis of Variance (ANOVA). First, a One-way Analysis of Variance (ANOVA) was performed to detect differences across all diving and gymnastics events. In addition, two-way ANOVA was used to compare between sports (diving/gymnastics) and body position (tuck/pike). Effect size was calculated as partial eta-squared (η2p). Post hoc tests with Bonferroni adjustments were performed where appropriate. To examine the relationship between angular speed and flight time, Spearman rho’s correlation analysis was performed.
Results
The inter-rater reliability between rater 1 and rater 2 were excellent for flight time (ICC = 0.994, SEM = 0.02 s) and good for angular speed (ICC = 0.788, SEM = 54°/s). Typical tight and rigid body positions during the somersaulting phase of divers and gymnasts are illustrated in Figure 4. In general, divers hold a tighter shape than gymnasts especially in the pike position. There were significant differences in angular speed (p < 0.001, η2p = 0.213) and flight time (p < 0.001, η2p = 0.928) among the different events in diving and gymnastics (Table 1). The 3.5 back somersaults in 3-m springboard diving were the fastest, while the triple back somersaults in double mini-tramp was the slowest. Flight time was the longest in 3.5 backward somersaults from the 10-m platform and the shortest when dismounting from rings.
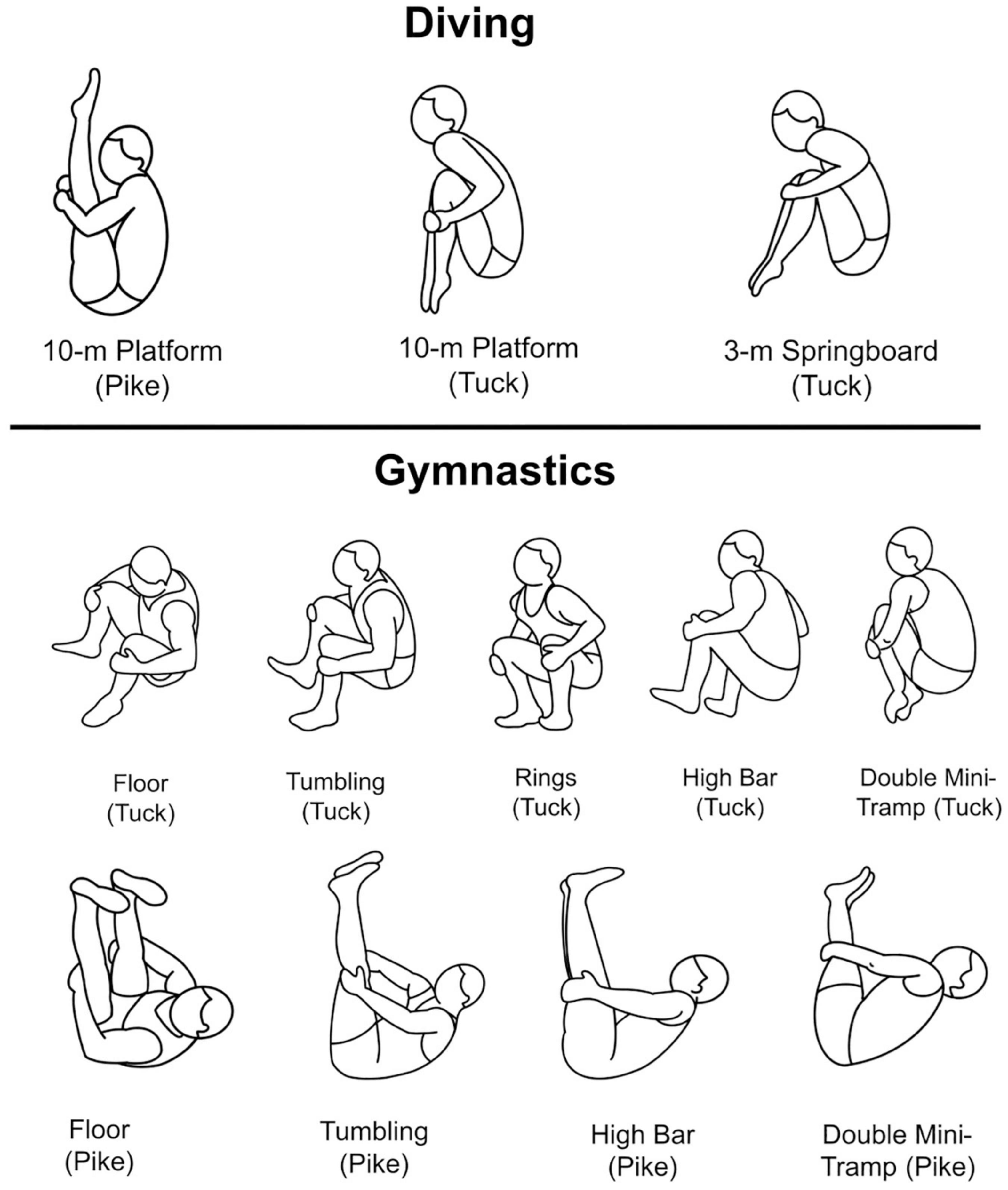
Figure 4. Illustration of typical tuck and pike positions of divers and gymnastics during the tight and rigid somersaulting phase of 3.0 or 3.5 back somersaults at high-level competitions.
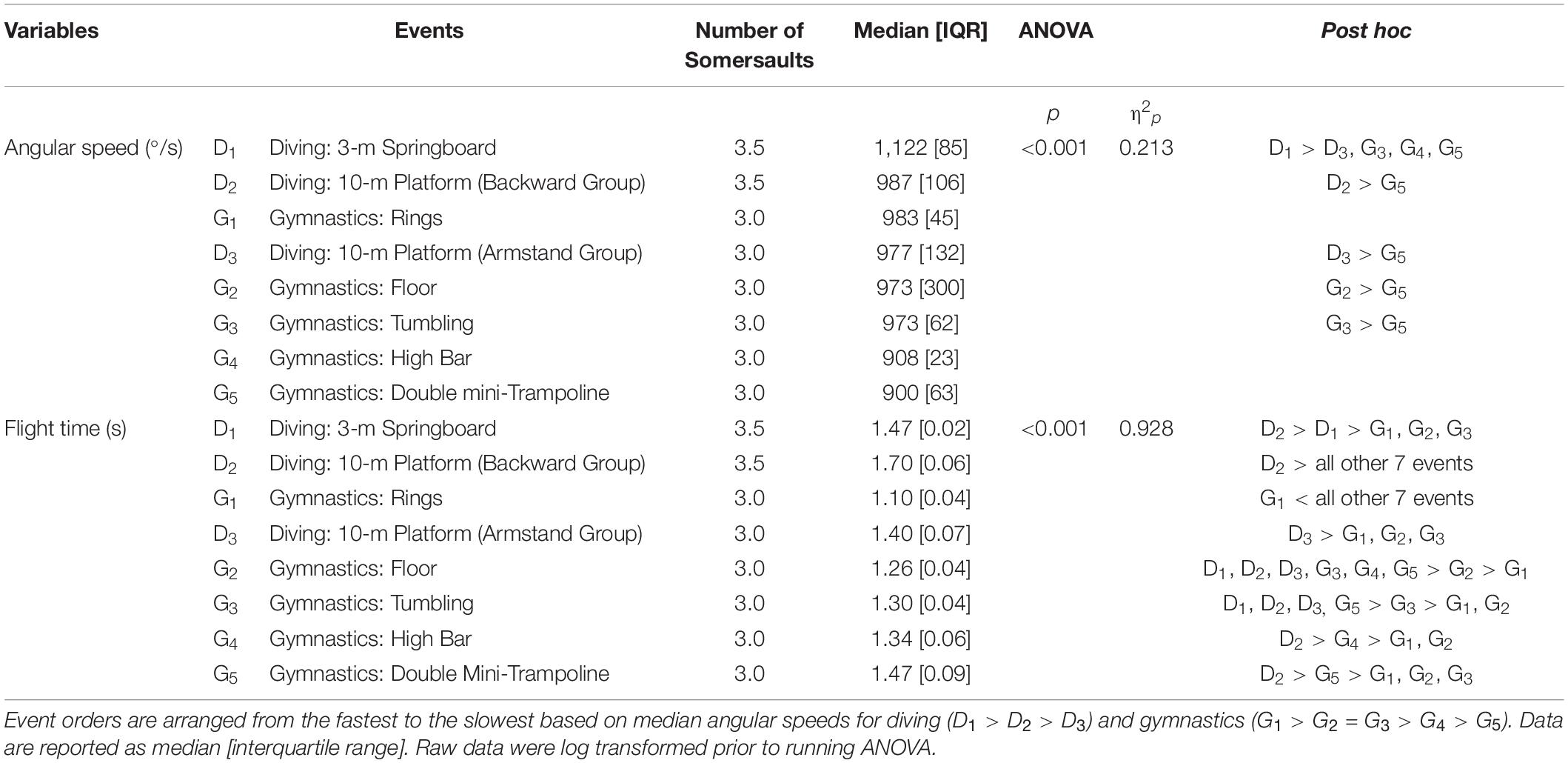
Table 1. Angular speed and flight time of multiple back somersaults in diving and gymnastics events.
When comparing between sports, ANOVA results revealed that divers had longer flight time (p < 0.001, η2p = 0.569) and were somersaulting faster than gymnasts (p = 0.021, η2p = 0.026, Table 2). While the flight time did not differ between body positions (p = 0.627), the angular speed was higher in tuck than pike somersaults (p < 0.001, η2p = 0.214, Table 2). No significant interaction effect between sport and body shape was found in angular speed or flight time variables.
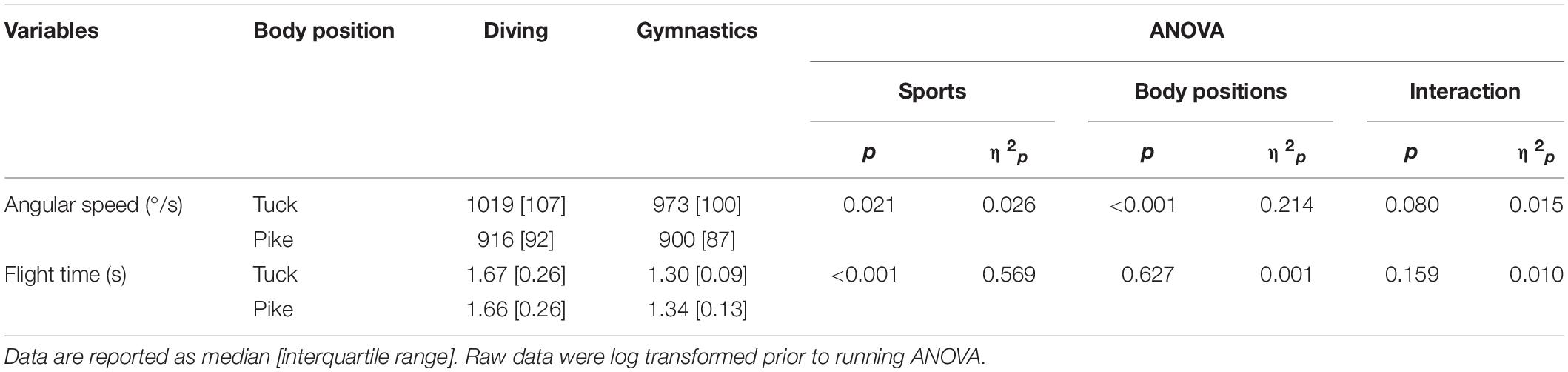
Table 2. Comparison of angular speed and flight time by sports (diving/gymnastics) and body positions (tuck/pike).
Regarding the relationship between angular speed and flight time, there was a significant negative correlation in gymnastics (rho = −0.533, p < 0.001) but not in diving (rho = −0.016, p = 0.873). The shorter the flight time available, the faster the gymnasts’ angular speed in order to complete required degrees of rotation (Figure 5).
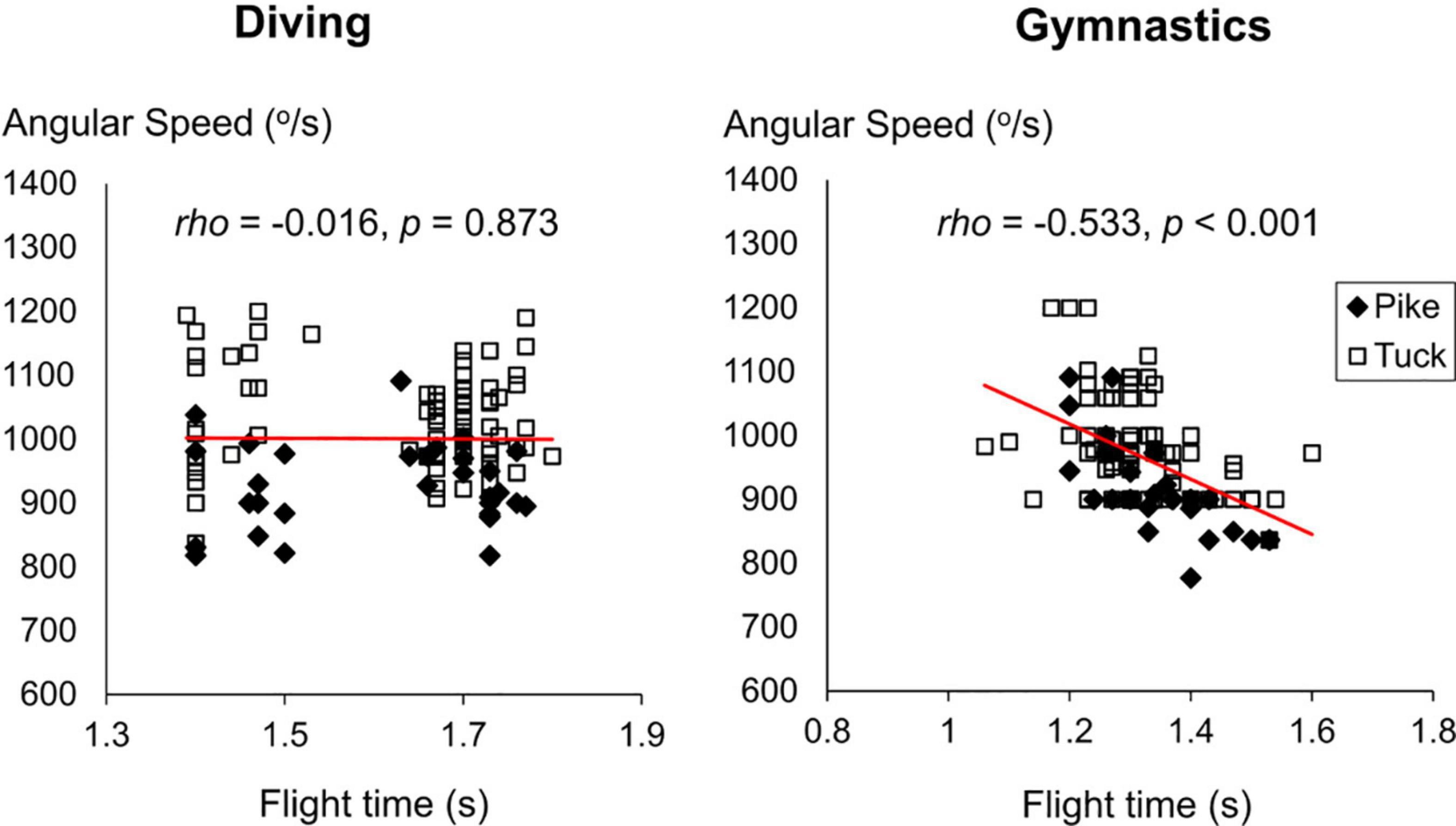
Figure 5. Spearman’s correlations between angular speed and flight time for multiple back 3.0 or 3.5 somersaults in diving and gymnastics.
Discussion
Using acrobatic sports as an example, this study illustrated how movement analysis can be performed using publicly available videos and free software to offer insights into high performance sports. The findings showed that the biomechanical characteristics of multiple back somersaults differed among various diving and gymnastics events despite the numbers of rotation were similar. The source videos were real performance of elite athletes at high-level competitions, providing greater ecological validity over analyzing training videos (Sayyah et al., 2018, 2020; King et al., 2022) or laboratory biomechanical tests (Mkaouer et al., 2013; Mizutori et al., 2021; Fujihara, 2022). While the videos were recorded with different camera settings and of varied qualities, the proposed data analysis approach yields good to excellent inter-rater reliability when measuring average angular velocity and flight time of acrobatic moves. We demonstrate that it is possible to make good use of readily available information such YouTube videos to enhance our understanding of sport movements at low cost. Although we used acrobatic sports as a case to illustrate the method, the potential applications of video-based analysis are certainly not limited to diving and gymnastics. In surfing, for example, time-motion analysis from videos have also been shown to reveal the timing of various activity that are deem useful for the development of tailored condition training programs (Minghelli et al., 2019).
Comparison Across Diving and Gymnastics Events
The present study found significant differences in angular speed and flight time across eight events in competitive diving and gymnastics. The range of angular speeds (median 900°/s to 1,122°/s) observed from our video analysis were similar to those quantified using inertial measurement units for forward 3.5 piked somersaults (855°/s to 904°/s) and forward 4.5 tuck somersaults (1,090°/s) in 3-m springboard diving (Walker et al., 2019). The observed differences in biomechanical characteristics can be explained by the varied approach speeds, compliance and height of the take-off and landing surfaces. For example, tumblers and artistic gymnasts have long run-up to build up momentum for their somersaults (Mkaouer et al., 2013) where divers perform backward and armstand dives from a stationary start (Hamill et al., 1986; Cheng and Hubbard, 2008). Platform divers have 10-m distance to travel before entering the water while 3-m springboard divers can take advantage of the springboard to increase flight time (Sanders and Wilson, 1988; Miller et al., 1989).
It was observed that divers rotated faster than gymnasts when performing multiple back somersaults of similar numbers of rotation. With the 3.5 back somersaults in 3-m springboard diving being the fastest move (1,122 [85]°/s), it is not surprising that only four male divers performed this move at high-level competitions. While four female divers chose to perform 3.5 back somersault from the 10-m platform, females are yet to master this dive on the 3-m springboard. Previous studies on springboard diving observed that males achieved greater heights than females (Miller et al., 1989; Sanders and Gibson, 2003). When performing 2.5 backward somersaulting dives, females were suggested to increase the amount of work done on the springboard to generate sufficient dive height and angular momentum (Sanders and Gibson, 2003).
The 3.5 back somersaults back somersaults from the 10-m platform was the second fastest move (987 [987, 106]°/s) and had the longest flight time (1.70 [0.06]°/s) among all events. This was the only event in which female athletes were able to perform 3.5 backward somersaults at high-level competitions. Starting from a stationary position with no run-up, platform divers must take off powerfully to generate sufficient height and angular momentum for rotation (Sanders and Wilson, 1988). The 10 m free-falling distance from the platform to the water provides the advantage of long flight time even if the athletes do not generate a high vertical velocity at take-off. Interestingly, even without a powerful take-off from the legs, the flight time of the armstand 3.0 somersaults (1.44 [1.42, 1.46]°/s) was comparable to other events such as 3-m springboard (1.48 [1.43, 1.53]°/s) and double-mini trampoline (1.47 [1.44, 1.50]°/s). The advantage of having a long flight time resulted in many divers being able to perform highly difficult moves from the 10-m platform.
In gymnastics, the triple back somersaults dismount from rings was the fastest move (983 [45]°/s) and only three male gymnasts managed to perform this difficult move. In the literature, there are very few detailed biomechanical studies on triple back somersaults in gymnastics. One case study on the double back somersaults in women’s artistic gymnastics floor routine reported a range of flight heights of the center of mass from 1.85 to 2.06 m across a few competitions (Vladimir et al., 2015). The author did not examine the flight time or angular speed during the somersaulting phase and hence no direct comparison could be made. In the current study, the flight time for the triple back somersault on floor was rather short (1.26 [0.04]s, the second shortest among all events). As a result, the gymnasts must rotate at a high angular speed in order to complete the required number of rotations before extending the body to land safely on their feet. Gymnasts build momentum from the run-up, round-off and back handspring(s) before taking off to perform the triple back somersaults. It is postulated that the full tumbling sequence in floor routines contributed to generating sufficient angular momentum required to complete the difficult move of triple back somersaults despite the short flight time.
Why Do Divers Rotate Faster Than Gymnasts?
With the exception of rings dismount, the multiple back somersaults in diving events required faster angular speeds than all other gymnastics events (Table 1). As illustrated in Figure 3, divers tend to hold a tighter shape during the somersaulting phase. Holding a tight body position while somersaulting require muscular efforts from the arms, torso, and legs (Gronbech, 1993; Miller and Sprigings, 2001; Kong, 2010; Mikl, 2018). Mikl (2018) used an 11-segment model to calculate the joint forces and moments required to maintain a tuck and pike shape while somersaulting. For both body positions, it was found that the greatest joint moments are at the hip, followed by the pelvis-abdomen segment. The author discussed that isometric strength at various joints may play a role in limiting the diver or gymnast’s ability to hold a rigid body shape while somersaulting. In addition to strength, the flexibility of the athletes can also determine whether one can form a tight shape, especially for the pike position. Gymnastics is a sport that is generally characterized by high levels of strength and power relative to body weight, as well as high flexibility (Donti et al., 2014), especially for male artistic gymnasts who are required to perform strength elements in various events (Jemni et al., 2006; Mkaouer et al., 2018). It is unlikely that gymnasts are limited by their isometric strength or poor flexibility to form tighter tuck and pike shapes during the somersaults.
One possible reason for why gymnasts do not hold a tighter shape to rotate faster may be related to the margin of error for landing. In diving, if a diver makes a mistake in the timing of come-out from the tight somersaulting shape, he/she cannot enter the water in a desirable near-vertical position. While a poor dive entry may lead to a low performance score and sometimes physical pain or bruising, the chance of severe injuries is low when entering the water. In gymnastics, the margin of error for landing is very narrow (Hiley and Yeadon, 2003, 2005). A poor landing resulting from mis-judging the timing of extending the body from a tight somersault position may lead to severe injuries or even fatal consequences. Thus, gymnasts may be more cautious toward pushing for the limit in their somersaulting speed. Vision may also play a role (Natrup et al., 2020, 2021; Barreto et al., 2021) as it is difficult to spot for landing when the athlete is rotating in a deeply closed pike position. During a back tuck somersault on a trampoline, the gymnasts’ gaze behavior depended on their position in the bed (Natrup et al., 2020). Kicking-out from a somersault early allows gymnasts to orient themselves to prepare for landing. A final point to note is that there is no advantage in the performance score for holding a very tight shape. According to gymnastics code of points for gymnastics, there will be no deduction as long the hip angle is less than 90° for the pike position. It is therefore not surprising to note that the typical pike position across all gymnastics events were much more open than that in 10-m platform diving (Figure 3). Within the requirements in code of points, gymnasts can regulate the tightness of the shape and not necessarily tucking or piking to the maximum extent. Holding a not-too-tight shape would mean that the gymnasts rotate at a slower angular speed, allowing a larger window of error to open their body and land safely on their feet.
Tuck Versus Pike Positions
To maintain a tight body position while somersaulting at fast speeds, muscular efforts from the arms, torso and legs are required to provide the necessary centripetal force (Gronbech, 1993; Miller and Sprigings, 2001; Kong, 2010; Mikl, 2018). Differences are noted in the way how divers and gymnast hold a tuck or pike shape while somersaulting and the joint moments required to hold the shape are influenced by the location and direction of how the arms pulling on the leg (Mikl, 2018). Since the angular momentum of the athlete in the flight phase is constant due to no external torque, the tightness of the body shape can substantially influence the rotational speed. The present study showed that somersaulting in a tuck shape was faster than that in a pike shape in which the athlete’s legs are extended and further away from the center of mass. This increases the moment of inertia about the transverse axis, making it more difficult to somersault (Mikl, 2018). Interesting, flight time was similar regardless of the body position during somersaulting. This reflects that athletes who were able to perform the same number of somersaults in a pike shape did not compromise the height of move, compared with those who chose to somersault in the easier tuck shape. These results align with previous data on female divers which showed similar dive height (mean value, pike: 0.82 m, tuck: 0.81 m) and flight time (pike: 1.29 s, tuck: 1.29 s) regardless of body positions when performing backward 2.5 somersaults from a 3-m springboard (Sanders and Gibson, 2003). When divers and gymnasts progress from tuck to pike somersaulting moves, they should aim to maintain the same jump height and expect a slower rotating speed.
Relationship Between Angular Speed and Flight Time
Among all gymnastics events, shorter the flight time was significantly correlated with faster angular speed. The shorter the flight time available, the faster the gymnasts must rotate to complete required degree of rotation. When taking off from a compliant surface – double mini-trampoline, the gymnasts gained the longest flight time and therefore could rotate more slowly when compared with the other gymnastics events. In diving, however, there was no significant relationship between angular speed and flight time. The lack of relationship may be due to the narrow range of flight time data in diving when compared with gymnastics (Figure 5). Only four dives were performed from the 3-m springboard and the other 101 dives were from 10-m platform in which the flight time was rather similar due to the long free-falling distance. From the same 10-m platform, divers performed 0.5 less somersault in the armstand group than the backward group. Despite significant less flight time in the armstand 3.0 somersaults (1.40 [0.07]s) than backward 3.5 somersaults (1.70 [0.06]s), the somersaulting speed were similar in these two dive groups (armstand: 977 [132]°/s; backward: 987 [106]°/s). Thus, when combing all diving moves as a group, the relationship between angular speed and flight time was unclear.
Transferability of Acrobatic Sports Skills
Diving coaches and athletes should be aware the high somersaulting speeds required to perform multiple back somersaults from the 3-m springboard. If a diver can perform 3.5 back somersaults from 10-m platform, he/she may not be able to execute the same move on 3-m springboard. Similarly, gymnasts can compete in different events and should be aware of the different rotational requirements. A gymnast who can dismount with a triple back somersault from the high bars may not be able to do so from the rings which require a faster angular speed. As different disciplines of acrobatic sports share common techniques and training approaches, some athletes may crossover from one sport to another. There are examples of retired gymnasts who pick up diving and continue to compete after. While we can reasonably expect high transferability of similar acrobatic skills, one previous study has showed that the kinematics of divers performing somersaulting moves differed between dry-land training and actual on-water diving (Barris et al., 2013). Thus, it is important to acknowledge the subtle biomechanical differences between similar acrobatic moves which can be critical at high performance level.
Limitations
There are a few limitations to the present study. First, the kinematic analysis was done on publicly available YouTube videos that were screen recorded at 30 Hz. Some videos were not filmed with fast shutter speeds and hence the extracted images were blurry at times. While higher quality videos recorded at higher sampling rate will allow more accurate kinematic analysis, access to data collection at high level competitions are often restricted. The kinematic video analysis in the present study was performed in a consistent manner and the inter-rater reliability was good to excellent. It is believed that the data obtained could provide sufficiently accurate and reliable information and allow a fair comparison across different acrobatic sports events. Second, the present study observed that divers in general hold a tighter shape than gymnasts and the typical body positions were illustrated in Figure 4. However, we were unable quantify the moments of inertia of the athletes owing to the limitation of video analysis.
Conclusion
This study demonstrated how movement analysis of elite sport performance can be done via analysis of publicly available videos using free software without the need of specialized laboratory, equipment or software. In the case of acrobatic sports, findings from this study showed that the biomechanical variable multiple back somersaults differed among various diving and gymnastics moves that appeared similar in the numbers of rotation. In general, divers rotated faster than gymnasts and that somersaulting in a tuck position has higher angular speed that the pike position. Gymnasts tended to hold a less tight shape than divers, possibly because a slower somersaulting speed allows larger margin of error in the timing of opening up for safe landing. Coaches can consider applying the proposed method to monitor the athletes’ performance and to identify errors. For example, if a gymnast struggles to complete a move, is it due to insufficient flight time or slow angular speed? The kinematics measurements can also be used to guide the transition plan across different apparatus (e.g., dismount from high bar and rings) and categories (e.g., 10-m platform to 3-m springboard). In conclusion, the present study highlights the potential of using readily available information and open-source freeware to generate scientific data for sports applications. Such data analysis approach can accommodate a wide range of video qualities, is easily accessible, and not restricted by situations such as social distancing, quarantine, lockdown or other restrictive measures.
Data Availability Statement
The datasets presented in this study can be found in online repositories. The names of the repository/repositories and accession number(s) can be found below: NIE Data Repository (https://doi.org/10.25340/R4/HRQ4PS).
Ethics Statement
The studies involving human participants were reviewed and approved by the Nanyang Technological University Institutional Review Board (Protocol Number: IRB-2021-02-029). Written informed consent for participation was not required for this study in accordance with the National Legislation and the Institutional Requirements.
Author Contributions
AS and MC performed the material preparation and data collection and analysis. PK wrote the first draft of the manuscript. All authors commented on previous versions of the manuscript, contributed to the study conception and design, and read and approved the final manuscript.
Funding
AS was supported by the Nanyang President’s Graduate Scholarship, Nanyang Technological University.
Conflict of Interest
The authors declare that the research was conducted in the absence of any commercial or financial relationships that could be construed as a potential conflict of interest.
Publisher’s Note
All claims expressed in this article are solely those of the authors and do not necessarily represent those of their affiliated organizations, or those of the publisher, the editors and the reviewers. Any product that may be evaluated in this article, or claim that may be made by its manufacturer, is not guaranteed or endorsed by the publisher.
Acknowledgments
We would like to thank Stefanie C. Lee and Jing W. Pan for their assistance with the preparation of figures.
References
Ae, M. (2020). The next steps for expanding and developing sport biomechanics: winner of the 2019 ISBS Geoffrey Dyson Award. Sports Biomech. 19, 701–722. doi: 10.1080/14763141.2020.1743745
Augustus, S., Hudson, P. E., and Smith, N. (2021). The effect of approach velocity on pelvis and kick leg angular momentum conversion strategies during football instep kicking. J. Sports Sci. 39, 2279–2288. doi: 10.1080/02640414.2021.1929008
Barbosa, T. M., Barbosa, A. C., Simbaña Escobar, D., Mullen, G. J., Cossor, J. M., Hodierne, R., et al. (2021). The role of the biomechanics analyst in swimming training and competition analysis. Sports Biomech. [Epub ahead of print]. doi: 10.1080/14763141.2021.1960417
Barreto, J., Casanova, F., Peixoto, C., Fawver, B., and Williams, A. M. (2021). How task constraints influence the gaze and motor behaviours of elite-level gymnasts. Int. J. Environ. Res. Public Health 18:6941. doi: 10.3390/ijerph18136941
Barris, S., Davids, K., and Farrow, D. (2013). Representative learning design in springboard diving: is dry-land training representative of a pool dive? Eur. J. Sport Sci. 13, 638–645. doi: 10.1080/17461391.2013.770923
Bobo-Arce, M., Sierra-Palmeiro, E., Fernández-Villarino, M. A., and Fink, H. (2021). Training in rhythmic gymnastics during the pandemic. Front. Psychol. 12:658872. doi: 10.3389/fpsyg.2021.658872
Cheng, K. B., and Hubbard, M. (2008). Role of arms in somersaulting from compliant surfaces: a simulation study of springboard standing dives. Hum. Mov. Sci. 27, 80–95. doi: 10.1016/j.humov.2007.05.004
Donti, O., Tsolakis, C., and Bogdanis, G. C. (2014). Effects of baseline levels of flexibility and vertical jump ability on performance following different volumes of static stretching and potentiating exercises in elite gymnasts. J. Sports Sci. Med. 13, 105–113.
Ferdinands, R. E., Kersting, U., and Marshall, R. N. (2009). Three-dimensional lumbar segment kinetics of fast bowling in cricket. J. Biomech. 42, 1616–1621. doi: 10.1016/j.jbiomech.2009.04.035
Fujihara, T. (2022). Real-time video and force analysis feedback system for learning strength skills on rings in men’s artistic gymnastics. Sports Biomech. [Epub ahead of print]. doi: 10.1080/14763141.2021.2024873
Gronbech, E. (1993). Sport-specific strength training for the rotation about the transverse axis in diving and gymnastics. Natl. Strength Cond. Assoc. J. 15, 62–66.
Hamill, J., Ricard, M. D., and Golden, D. M. (1986). Angular momentum in multiple rotation nontwisting platform dives. Int. J. Sport Biomech. 2, 78–87. doi: 10.1123/ijsb.2.2.78
Hiley, M. J., and Yeadon, M. R. (2003). The margin for error when releasing the high bar for dismounts. J. Biomech. 36, 313–319. doi: 10.1016/S0021-9290(02)00431-1
Hiley, M. J., and Yeadon, M. R. (2005). The margin for error when releasing the asymmetric bars for dismounts. J. Appl. Biomech. 21, 223–235. doi: 10.1123/jab.21.3.223
Jemni, M., Sands, W. A., Friemel, F., Stone, M. H., and Cooke, C. B. (2006). Any effect of gymnastics training on upper-body and lower-body aerobic and power components in national and international male gymnasts? J. Strength Cond. Res. 20, 899–907. doi: 10.1519/R-18525.1
Khong, S., and Kong, P. A. (2016). Simple and objective method for analyzing a gymnastics skill. Eur. J. Physic. Educ. Sport 12, 46–57. doi: 10.13187/ejpe.2016.12.46
King, M. A., Kong, P. W., and Yeadon, M. R. (2019). Maximising forward somersault rotation in springboard diving. J. Biomech. 85, 157–163. doi: 10.1016/j.jbiomech.2019.01.033
King, M. A., Kong, P. W., and Yeadon, M. R. (2022). Differences in the mechanics of takeoff in reverse and forward springboard somersaulting dives. Sports Biomech. [Epub ahead of print]. doi: 10.1080/14763141.2022.2034929
King, M. A., and Yeadon, M. R. (2004). Maximising somersault rotation in tumbling. J. Biomech. 37, 471–477. doi: 10.1016/j.jbiomech.2003.09.008
Kong, P. W. (2010). Hip extension during the come-out of multiple forward and inward pike somersaulting dives is controlled by eccentric contraction of the hip flexors. J. Sports Sci. 28, 537–543. doi: 10.1080/02640411003628030
Mikl, J. (2018). Joint moments required to hold a posture while somersaulting. Hum. Mov. Sci. 57, 158–170. doi: 10.1016/j.humov.2017.12.001
Miller, D. I., Hennig, E., Pizzimenti, M. A., Jones, I. C., and Nelson, R. C. (1989). Kinetic and kinematic characteristics of 10-m platform performances of elite divers: I. Back takeoffs. Int. J. Sport Biomech. 5, 60–88. doi: 10.1123/ijsb.5.1.60
Miller, D. I., and Sprigings, E. J. (2001). Factors influencing the performance of springboard dives of increasing difficulty. J. Appl. Biomech. 17, 217–231. doi: 10.1123/jab.17.3.217
Minghelli, B., Paulino, S., Graça, S., Sousa, I., and Minghelli, P. (2019). Time-motion analysis of competitive surfers: Portuguese championship. Rev. Assoc. Méd. Bras. 65, 810–817. doi: 10.1590/1806-9282.65.6.810
Mitchell, D., Davis, B., and Raim, L. (2002). Teaching Fundamental Gymnastics Skills, 1st Edn. Champaign, IL: Human Kinetics.
Mizutori, H., Kashiwagi, Y., Hakamada, N., Tachibana, Y., and Funato, K. (2021). Kinematics and joints moments profile during straight arm press to handstand in male gymnasts. PLoS One 16:e0253951. doi: 10.1371/journal.pone.0253951
Mkaouer, B., Hammoudi-Nassib, S., Amara, S., and Chaabène, H. (2018). Evaluating the physical and basic gymnastics skills assessment for talent identification in men’s artistic gymnastics proposed by the International Gymnastics. Biol. Sport 35, 383–392. doi: 10.5114/biolsport.2018.78059
Mkaouer, B., Jemni, M., Amara, S., Chaabène, H., and Tabka, Z. (2013). Kinematic and kinetic analysis of two gymnastics acrobatic series to performing the backward stretched somersault. J. Hum. Kinet. 5, 17–26. doi: 10.2478/hukin-2013-0021
Morgulev, E., Azar, O. H., and Lidor, R. (2018). Sports analytics and the big-data era. Int. J. Data Sci. Anal. 5, 213–222. doi: 10.1007/s41060-017-0093-7
Natrup, J., Bramme, J., de Lussanet, M. H. E., Boström, K. J., Lappe, M., and Wagner, H. (2020). Gaze behavior of trampoline gymnasts during a back tuck somersault. Hum. Mov. Sci. 70:102589. doi: 10.1016/j.humov.2020.102589
Natrup, J., de Lussanet, M. H. E., Boström, K. J., Lappe, M., and Wagner, H. (2021). Gaze, head and eye movements during somersaults with full twists. Hum. Mov. Sci. 75:102740. doi: 10.1016/j.humov.2020.102740
Nor Adnan, N. M., Ab Patar, M. N. A., Lee, H., Yamamoto, S. I., Jong-Young, L., Mahmud, J., et al. (2018). Biomechanical analysis using Kinovea for sports application. IOP Conf. Ser. Mater Sci. Eng. 342:012097. doi: 10.1088/1757-899X/342/1/012097
Pan, J. W., Komar, J., Sng, S. B. K., and Kong, P. W. (2021). Can a good break shot determine the game outcome in 9-ball? Front. Psychol. 12:691043. doi: 10.3389/fpsyg.2021.691043
Park, J., and Yoon, S. (2017). Kinematic analysis of back somersault pike according to skill level in platform diving. Korean J. Sport Biomech. 27, 157–164. doi: 10.5103/KJSB.2017.27.3.157
Puig-Diví, A., Escalona-Marfil, C., Padullés-Riu, J. M., Busquets, A., Padullés-Chando, X., Marcos-Ruiz, D., et al. (2019). Validity and reliability of the Kinovea program in obtaining angles and distances using coordinates in 4 perspectives. PLoS One 14:e0216448. doi: 10.1371/journal.pone.0216448
Sanders, R., and Gibson, B. (2003). Diving: technique and timing in women’s backward two and one half somersault tuck (205C) and the men’s backward two and one half somersault pike (205B) 3m springboard dives. Sports Biomech. 2, 73–84. doi: 10.1080/14763140308522809
Sanders, R. H., and Wilson, B. D. (1988). Factors contributing to maximum height of dives after takeoff from the 3M springboard. Int. J. Sport Biomech. 4, 231–259. doi: 10.1123/ijsb.4.3.231
Sayyah, M., Hiley, M. J., King, M. A., and Yeadon, M. R. (2018). Functional variability in the flight phase of one metre springboard forward dives. Hum. Mov. Sci. 59, 234–243. doi: 10.1016/j.humov.2018.04.014
Sayyah, M., King, M., Hiley, M. J., and Yeadon, M. R. (2020). Functional variability in the takeoff phase of one metre springboard forward dives. Hum. Mov. Sci. 72:102634. doi: 10.1016/j.humov.2020.102634
Tay, C. S., and Kong, P. W. (2018). A video-based method to quantify stroke synchronisation in crew boat sprint kayaking. J. Hum. Kinet. 65, 45–56. doi: 10.2478/hukin-2018-0038
Vladimir, P., Carmen, M., and Daniel, N. (2015). Andreyeva No. Didactic technologies of learning the double back somersault on floor based on the biomechanical analysis of sports technique in women’s artistic gymnastics. J. Phys. Educ. Sport 15, 120–127. doi: 10.7752/jpes.2015.01020
Walker, C., Sinclair, P., Graham, K., and Cobley, S. (2017). The validation and application of inertial measurement units to springboard diving. Sports Biomech. 16, 485–500. doi: 10.1080/14763141.2016.1246596
Keywords: YouTube, Kinovea, somersaults, sport, diving, gymnastics
Citation: Kong PW, Sim A and Chiam MJ (2022) Performing Meaningful Movement Analysis From Publicly Available Videos Using Free Software – A Case of Acrobatic Sports. Front. Educ. 7:885853. doi: 10.3389/feduc.2022.885853
Received: 28 February 2022; Accepted: 18 May 2022;
Published: 10 June 2022.
Edited by:
Aaron Williamon, Royal College of Music, United KingdomReviewed by:
Germina Cosma, University of Craiova, RomaniaJosé Eugenio Rodríguez-Fernández, University of Santiago de Compostela, Spain
Copyright © 2022 Kong, Sim and Chiam. This is an open-access article distributed under the terms of the Creative Commons Attribution License (CC BY). The use, distribution or reproduction in other forums is permitted, provided the original author(s) and the copyright owner(s) are credited and that the original publication in this journal is cited, in accordance with accepted academic practice. No use, distribution or reproduction is permitted which does not comply with these terms.
*Correspondence: Pui Wah Kong, cHVpd2FoLmtvbmdAbmllLmVkdS5zZw==; Alexiaa Sim, bmllMTg0NzA0QGUubnR1LmVkdS5zZw==