- Metaverse Research Unit, Institute for Intelligent Systems, University of Johannesburg, Johannesburg, South Africa
The COVID-19 pandemic challenged the status quo of higher education practices. With the transition to remote learning, students and faculty faced several challenges while making great strides in equalizing education access. Although theoretical aspects can be easily taught online, practical experiences are difficult to convey without dedicated laboratories and equipment. This is especially true in biological studies, where practical laboratory training significantly impacts the Student’s professional development. Presented here is an overview of some of the available resources that faculty can implement in their remote teaching curricula, a discussion on a possible approach toward creating in silico practicums, and potential challenges that could be experienced in creating such virtual laboratories (VL). To prevent another interruption in academic training, higher education institutions (HEIs) should focus on designing, developing, and implementing practical educational material. We propose that these resources be free and open-source, allowing for a global effort to create a standardized practical curriculum for basic biological technical skills.
Introduction
During the last 2 years, higher education institutions (HEIs) had to completely reimagine their curricula, mainly due to the global coronavirus disease of 2019 (COVID-19) pandemic. Efforts to curb the spread of this viral threat included the implementation of various public health measures. These actions included mainly the closure of borders, placing restrictions on intracountry movements, and closing facilities with a high aggregation of people in confined spaces, including schools, HEIs and other training facilities (Hale et al., 2020). Although such closures occurred with little prior notice, many institutions were agile enough to take their academic activities online; thus, enabling students to receive an education while the pandemic raged. The shift to online learning was relatively seamless in many countries with a high gross domestic product and high levels of computer literacy. However, economies with limited resources had to implement innovative solutions to ensure a high standard of academic activities. Indian HEIs implemented various online solutions, ranging from dedicated web-based radio and television stations to virtual laboratories (VL), as Jena (2020) reviewed. Learning also changed to a mainly online format in countries such as Egypt, Indonesia, Nigeria, Nepal, and the United Arab Emirates, among others (Crawford et al., 2020; Paudel, 2021), using various platforms ranging from social media (WhatsApp, YouTube, Facebook, and Twitter among other networks) to dedicated online teaching platforms (Google Classroom, Moodle, Blackboard, Zoom among others).
However, the COVID-19 pandemic was not the sole driving force in the shift toward online learning. HEIs saw the potential to take academia online prior to the pandemic, driven in part by an intensification for a continuously developed workforce adapting to ever-evolving digital economies and the global adoption of the Internet (Palvia et al., 2018), as well as the increasing popularity of online education (Dumford and Miller, 2018). e-Learning holds many benefits for students: giving them the ability to learn remotely while working to create an income; developing themselves regardless of geographical barriers; and investigating and developing new career prospects (Nguyen, 2015). Online learning is also not a lesser pedagogy compared to “traditional” face-to-face teaching. Whether classroom time can be reduced and replaced with an online learning environment without compromising learning outcomes was the focus of a meta-analysis conducted by Müller and Mildenberger (2021). They report that blended learning is on par with traditional classroom learning, despite a reduction in classroom time of up to 79% in some reports. Although the COVID-19 pandemic may have expedited the process of moving higher education online, predictions before the pandemic were that online education would become the main delivery method by 2025 (Palvia et al., 2018; World Economic Forum, 2020). There are many benefits to learning online; however, online learning also has challenges to overcome for students and faculty.
The shift to online learning occurred so quickly that many students and faculty felt inadequately prepared at the beginning of the pandemic. In Jordan, 40% of the faculty surveyed had no prior online teaching experience, while 66% reported receiving some form of online pedagogy training (Almahasees et al., 2021). Egyptian tertiary educators listed poor Internet connectivity, inadequate access to computers, and technical problems as the highest barriers to e-learning (Zalat et al., 2021). These barriers have also been reported in other countries, including South Africa (Mhlanga and Moloi, 2020; Mpungose, 2020), the Republic of Ireland (Cullinan et al., 2021), and the Philippines (Joaquin et al., 2020). There is evidence of ingenious approaches to overcome these challenges. For example, in South Africa, a country known for the high costs of mobile data, several universities banded together to bargain for reduced pricing on data from mobile operators, offering students access to 30 Gb of free monthly data to attend online video-based live classes. Many educational websites were also zero-rated (i.e., browsing these websites did not incur any cost for the browser). However, the most widely used online teaching service, Blackboard, could not be zero-rated at the beginning of the pandemic in South Africa (Hedding et al., 2020), thus requiring HEIs to subsidize mobile data. The University of Johannesburg, the University of Cape Town, and the University of the Witwatersrand (among others) implemented computer loan schemes to ensure that deserving students had access to the computing hardware required to complete their online studies.
Teaching theoretical aspects is quite easily done using online lecturing through live classes or in asynchronous modules when students are adequately equipped with the necessary support (technical, hardware, and access to broadband Internet) to access such learning opportunities. However, education is based not only on theoretical learning, but also on the practical implementation of techniques in practice, especially in the sciences. In an early review on how HEIs responded to the COVID-19 pandemic, Crawford et al. (2020) examined HEI strategies in 20 countries. Although providing a detailed examination of the pedagogical approaches implemented in these countries, the only mention of practical training was a singular notion that Hong Kong HEIs suspended practical training until further notice (Crawford et al., 2020). From this early report, it would seem that HEIs did not highly prioritize practical training, as was the case for theoretical learning. Students without such practical experience are likely to graduate with inadequate laboratory skills, a major long-term disadvantage compared to previous students who were able to study “normally” (Gamage et al., 2020). However, current technological advances have allowed virtual laboratory (VL) experiences to be created. VLs can be defined as “technology-mediated experiences in two or three dimensions that situate the student as being in an emulation of the physical laboratory with the capacity to manipulate virtual equipment and materials” through input devices (Reeves and Crippen, 2020).
In their extensive review of published VLs, Reeves and Crippen (Reeves and Crippen) describe that the majority of VLs before the COVID-19 pandemic were 2D desktop-based (92%) and acquired from external sources rather than developed for specific courses (75%). Udin et al. (2020) reviewed published VLs that improved Students’ understanding of abstract biology concepts and laboratory skills, with a total of 47 reports of 19 VLs dedicated to the topic. The students found the use of VLs to be mostly positive (Udin et al., 2020). The reported small number of self-developed VLs motivated the author’s investigation of whether the creation of VLs is feasible for an academic in their regular line of work. Here an approach toward creating two VL experiences is presented. One focused on creating a 3D laboratory environment where students could learn to perform a streak plate, a microbiological technique used to isolate colonies of a single strain of bacteria. The other aimed to educate students on micropipetting skills.
This paper investigates two aspects of the instructional design processes followed to create the VLs mentioned above.
(1) To provide an example, using mainly freely available software tools, of an approach toward developing different online laboratory learning methods.
(2) To report on the challenges we faced in creating an online laboratory training session and how these challenges were overcome.
This reflective paper is structured as follows. In section “An Approach to Virtual Laboratories,” the approach to creating VLs is described. In section “Challenges Experienced During the Creation of Virtual Laboratories During a Pandemic,” there is a discussion of challenges experienced in this endeavor to create VLs, and how these challenges were overcome. Lastly, in section “The Future of Virtual Laboratories and Conclusions,” we introduce the idea of creating an open access online repository where open-source applications can be shared for use by anyone. This report avoids being a technical report of creating a VL, as each VL will require a unique solution (i.e., creation of specific 3D models, programming of interactions, creation of assessment opportunities). The created VLs will soon be shared on a newly developed online repository (amakhono.org) Interested readers are welcome to contact the author for access to the source code and the VLs.
An Approach to Virtual Laboratories
The approach described below was developed based on the following self-formulated challenge: Create a teaching experience in which students can learn how to use a micropipette and how to perform a microbiological streak plate. The experiences should be available in various formats, including video, mobile applications, and virtual reality. The solution should be cost-effective and developed as far as possible without third-party payments.
Development Platform
At the beginning of this challenge, the author investigated several potential approaches for creating such VL experiences. The platforms are listed in Table 1, including the perceived benefits and caveats for using these platforms.
Game engines held the most promise in creating VLs in an agile manner, with the additional benefit of being able to export the content in various formats (i.e., PC, Linux, iOS, Android, WebGL). Several freemium game engines, including Unity3D,1 Unreal Engine2 and CryEngine3 offer 3D spatial representations and increasingly higher levels of immersion (Keil et al., 2021). Each one of these game engines offers different benefits to the programmer, and each has merits for using in the creation of VLs. Based on the author’s previous experience in programming in the C# language, Unity was chosen as the preferred game engine for development.
Various educational tools used Unity3D, a Unity Technologies-developed game engine, as a preferred development tool. The Open University OpenScience Laboratory implemented Unity3D to create different experiences, including a geology field trip based on Skiddaw in the Lake District (Minocha and Burden, 2013). High-level safety demonstrations in chemistry (Dholakiya et al., 2019), augmented reality of traditional foods for nutrition education (Yulia et al., 2018), fire safety training (Zhang and Shi, 2019), and virtual practice platforms for chemical engineering (Ouyang et al., 2018) are among a few examples of how HEIs have implemented this software in the creation of dedicated training software.
Unity3D offers the option to export to various platforms, including Apple iOS, Android, Windows, Linux, and WebGL. The ability to export to these formats enables educators to develop their VL once and make them available to a broader range of devices that students can access. Although Unity3D has a range of 2D tools, its approach to 3D helps developers create immersive environments.
Creating a 3D Laboratory Environment
Unity3D supports standard 3D file formats (.fbx, .dae, .dxf, and .obj), and although not recommended for production builds, Unity can also import proprietary file formats (Autodesk Maya, Blender, Modo, and Cheetah3D), which are internally converted to the .fbx format (Unity Technologies, 2021a). Generating a 3D world within Unity3D is done by placing 3D objects in a three-dimensional coordinate system. Each 3D object can be programmed to interact with other objects by adding the necessary physics objects, including object colliders and “rigid body” components, allowing objects to react in a simulated manner and expected physics.
When creating VLs, the developer should have a clear priority list, ensuring that his efforts are implemented optimally. In this presented case, the focus was on generating VLs as quickly as possible while ensuring that a high level of immersion was managed. Therefore, we opted to use the Unity Asset Store for some 3D models. The Unity Asset Store links potential buyers with sellers of Unity3D optimized add-ons and production elements. We found a modeled chemistry laboratory4 in this online store for the backdrop for our VLs. At the time of purchase, this model was on sale for US $5 (Figure 1).
Unity3D renders at various quality levels, depending on the render pipeline used. Since our range of devices had varying graphical outputs, we opted to use the Universal Render Pipeline (URP). This rendering pipeline is optimized for use on mobile platforms and works exceptionally well on high-end consoles. The 3D laboratory model was created for Unity’s High-Definition Render Pipeline (HDRP). The model conversion from HDRP to URP was done in Unity3D, by reassigning textures to the relevant materials in and with the appropriate shaders.
The purchased 3D laboratory model provided only the basic essential equipment and environment for a VL (table, blackboard, and aesthetic objects). All the additional equipment required for these applications needed to be sourced elsewhere. Various online marketplaces selling 3D models exist, including Turbosquid,5 CG Trader,6 Shapeways7 and Free3D,8 among other domains. When searching for 3D models of required laboratory equipment, we found that prices ranged between $2 US and $1,699 US per item on the various platforms (June 2021). As an example, the price of a micropipette varied between $9 US and $59 US on these markets, resulting in an increasing cost estimate in creating a whole laboratory experience. Instead of buying these 3D models, the author decided to design and develop the 3D models required for laboratory experiences in-house.
Creating a 3D Laboratory Equipment
On the basis of this decision, 3D modeling software was required. Many such packages exist in the market, ranging from high-end to extremely basic and carrying varying price tags from relatively expensive to free. One 3D modeling software package that stood out was Blender9 based on the high level of community support in the form of tutorials and open discussion forums, as well as the capabilities of the software to generate 3D models that range from comical to photo realistic. Blender is also available for free for any use. Creating 3D models of laboratory equipment in Blender was first described over a decade ago (Dere et al., 2010). With Project OSCAR (Open-Source Courseware Animations Repository), Dere and their colleagues created the first online repository of Blender-generated 3D models of laboratory equipment, licensed under a Creative Commons—Attribution-Non-Commercial ShareAlike 2.5 India license. Project OSCAR hosts 19 chemistry and 26 physics-related models. However, and perhaps better phrased, unfortunately, the project has not added many new models apart from the initial “more than 40” reported (Dere et al., 2010).
The author used Blender to generate 3D models of standard laboratory equipment, including an incubator, micropipette, Petri dishes, Bunsen burner, and inoculation loop. After completing a basic Blender tutorial on YouTube to learn how to use the software, the 3D laboratory equipment models were quickly created (averaging approximately 30 min per item). A demonstration of how to make a low-polygon Bunsen burner is presented in Supplementary Video 1. Other low-polygon 3D models were created in a similar fashion. It should be noted that the author has no formal training in 3D modeling prior to this endeavor, apart from watching free video tutorials on-line. The author is classically trained in environmental microbiology and genetics.10
Creating custom-made 3D objects for use in VLs has the benefit that it can be modeled to be similar to the equipment that students will most likely have used in a real laboratory, making asynchronous learning a feasible addition to the current practical curriculum. The main caveat is that it takes time to develop these 3D models and to learn how to use 3D modeling software such as Blender. However, the software has become much more user-intuitive with recent upgrades to the Blender user interface. The created models were easily imported into Unity3D and arranged in a 3D space to mimic a traditional practical training session (Figure 2). Once the models have been correctly placed in the 3D space, user interactions could be scripted.
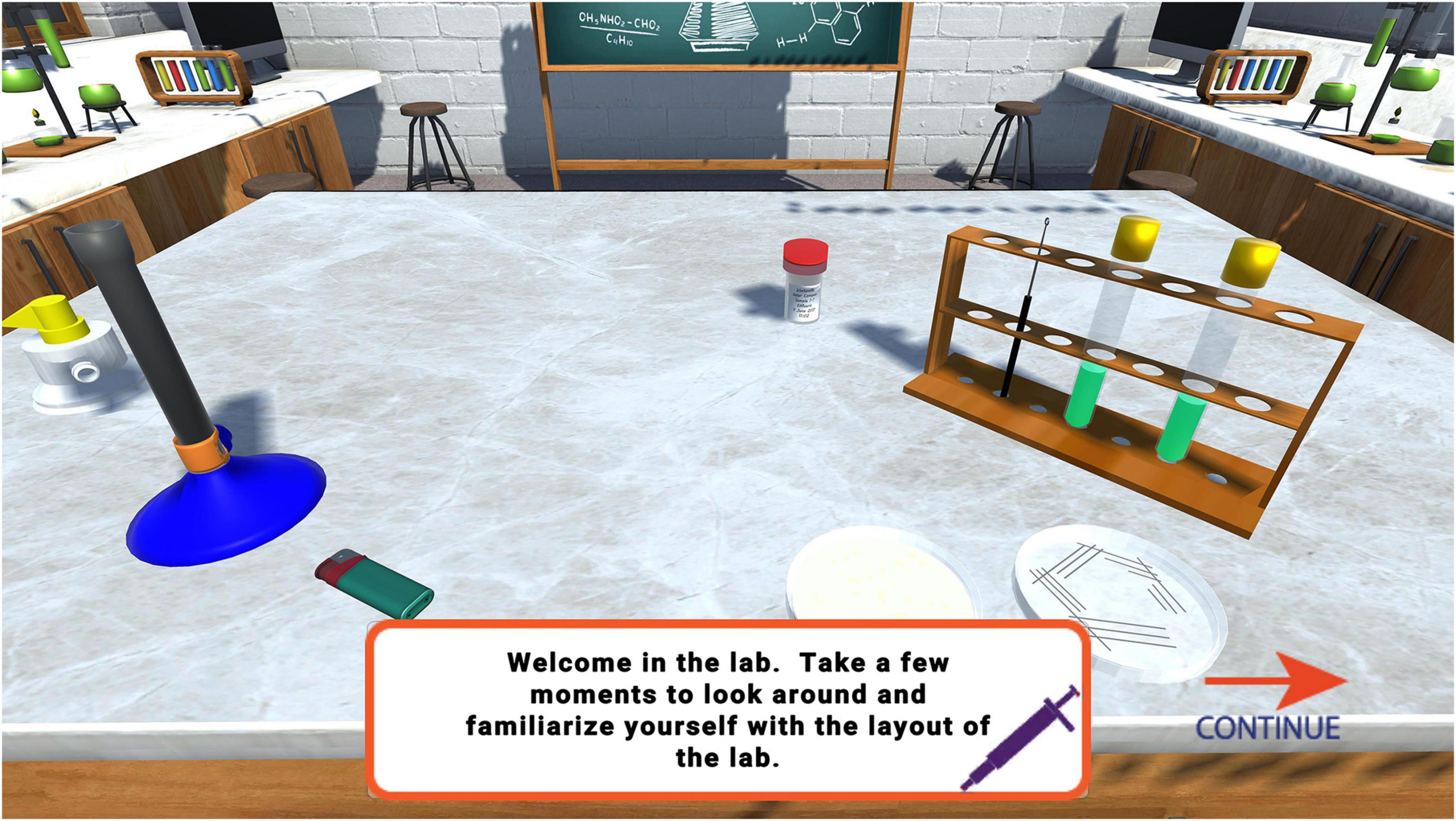
Figure 2. 3D Models placed in Unity3D. Several 3D models were generated in Blender, including the Bunsen burner, Petri dishes with agar, inoculation loop, green fluid test tubes, and the gas tap.
Animating, Scripting, and Interactions
Both Blender and Unity3D provide the developer with tools to animate 3D models, based on incremental movements or changes made to selected parts of the 3D object on a linear timeframe. Where possible, it is advised to animate objects rather than coding complex behaviors. For example, we decided to animate the Bunsen burner collar that adjusts the amount of oxygen entering the gas mix, as well as the gas tap that opens the flow of gas and the movement of the lighter to ignite the Bunsen burner and the opening/closing of the incubator door. These animations were done in Blender, and the exported 3D objects and animations were easily imported into Unity3D by a simple drag and drop.
However, to generate a sense of realism, not all objects can be animated to react in a predetermined fashion. Scripting is required for some objects to ensure that their behavior is as expected in a real-life laboratory. Some of the objects that we scripted to have additional behaviors included the flame of the Bunsen burner, the incubator temperature controls and light settings, and the pipette volume changer. Animating these objects would have been a momentous task, while scripting allowed for a far less laborious effort. An example is VR-based micropipette training, where the volume setting was scripted to be changed based on the number of turns on the volume setting dial. Animating this would have resulted in generating animation for each of the 9,999 possible volume settings on a 1,000 μL micropipette. For this solution, we used a Unity3D text object, overlain on the position where the text should be displayed, that could be changed from a C# script. The assigned script checks whether the volume changer is turned left or right and then adds or subtracts an arbitrary amount from the displayed volume of the pipette, updating the text value in each frame of the application.
In scripting, one is going to run into some conundrums as to how best to move forward. An example of one such problem that we faced was in modeling liquids in containers. Although game engines are competent in handling everyday objects’ physics, liquids (and, by extension, gases) remain a challenging physical state to model. Generally, two-dimensional modeling of these states of nature is done relatively easily by software such as Unity3D, but with an added dimension, it becomes more complicated. Since Unity3D does not support fluid handling in its standard format, the use of an additional package from the Unity Asset Store was investigated. Currently, there is only one fluid simulation package (called Obi Fluid)11 that mimics particles to generate accurate fluid simulations. The package retails for $60 US. However, one warning is that such fluid simulations are extremely demanding on computing resources, resulting in us opting rather for a shader-based solution for our application, as these applications were required to run on a wide variety of Students’ hardware. Various shader-based options are available in the Unity Asset Store; however, we opted for a solution reported by the Unity Community member—specifically “Binary Lunar” on YouTube.12 This free solution resulted in a primitive liquid that would take the shape of a container. Scripting the behavior of the liquid, based on the status of the pipette, resulted in a “virtual” fluid that could be manipulated at a fraction of the computing requirements. This enabled us to export the final applications to PC, Android (including Oculus), and WebGL.
The intended platform of the developed application is an important choice that needs to be well defined at the start of any similar projects. Although the export is relatively easy (a switch done by Unity3D), the interaction programming requires that the platform be kept in mind. For instance, input on a personal computer will require a different input framework compared to VR or mobile. In interaction coding, platform-specific alterations were incorporated to ensure an optimum user experience. These included changing the orientation of the camera (for mobile users with a gyroscope and VR devices, we incorporated the movement of the device to change the view in the game; mouse center button could be used to direct the camera for PC users, etc.) as well as the advancement methods of the tutorial steps, all to improve interactivity and ensure an engaging educational experience.
Tutorial and Guiding Student Through Experience
An important aspect of the created experiences is the tutorial, a guidance given to students to ensure that they achieve the targets of the VL experiment. There are two methods, both warranting further investigation as to which method aids students more. The first method is to provide step-by-step guidance and allow students to follow the tutorial in a specific order. Using this guided approach, students can focus on the steps in question. Second, students can be shown a video before the start of their virtual experiment, followed by a “free play” in the laboratory. We opted to investigate both styles to experience first-hand the implementation of these two guidance techniques in our VLs.
Practical Aspects of Implementing a Tutorial
By comparing students in three different tutorial scenarios, those who watched a video tutorial were better equipped to deal with a specific scenario compared to those who underwent static text-based instructions (Craig and Friehs, 2013). Interactive tutorials did not necessarily improve learning compared to static web-based tutorials (Craig and Friehs, 2013). However, Generation Z students are more inclined to innovative teaching styles, including visual and audience participation through simulation, case studies, and engaged learning (Hampton et al., 2020).
Practical Aspects of Implementing a Tutorial
In retrospect, the focus of this endeavor at the beginning of the development process was wrongly placed upon creating the experiences, without considering the implementation of a tutorial to guide students as an integral part of the experience. This resulted in an extended period required to create guided tutorials, including programming pauses in the experience where the user first needed to complete a specific task before advancing the tutorial to the next step. In the planning of any VL, special attention should be given to addressing how students will be guided, a valuable lesson that was learned from our experience.
Our implemented tutorial mechanism used the concept of “slides,” similar to PowerPoint or other slide presentation software. We programmed a tutorial manager to project images on the screen, play an audio voiceover (.mp3), and display the transcript (to include deaf and hard-of-hearing individuals). The tutorial manager would not allow advancement to the next phase until a set of criteria has been achieved. Therefore, it guides the student through their experience in a step-by-step manner. This method has the advantage of providing the student with short bursts of information, avoiding information overload, and ensuring that the student follows the tutorial exactly. The disadvantage of this tutorial method, at least for the programmer, is that it requires extensive programming to ensure that each step is coded correctly.
Audio quality is also an essential factor to include in the design of learning experiences. Here, the creator has three choices. First, they can record audio on their own devices and edit the audio to be of high quality. Various audio editing applications exist that can aid the developer in generating quality audio files, including open-source software packages, such as Audacity,13 which provides a robust workflow for editing audio files. However, recording requires a high-quality microphone to ensure that audio quality does not distract students. Furthermore, in many cases where recordings are made outside of a professional studio, background noise may be recorded as well. The highest quality audio content will be generated by recording audio in a dedicated studio, yet this increases production costs and may also result in an increase in the project development time. Additional recording or editing may further increase the cost of outsourcing audio.
The author believes that the most cost- and time-effective solution is to use an artificially intelligent text-to-speech generator to record audio. This allows the producer the opportunity to edit tutorial text and regenerate the audio files by merely pressing a button. Various text-to-speech services exist with varying price tags. Some free options include Amazon Polly14 and Balabolka.15 However, the free services that were evaluated were deemed monotonous by a small focus group (results not shown). Therefore, it was decided to investigate more advanced text-to-speech options. These include paid services such as murf.ai, nuance.com, and many others. Following a free trial of murf.ai, it was found that this platform provided for the immediate needs (although this is by no means supportive of murf.ai being the best platform for text-to-speech). Utilizing the paid-for service ($19 US per month, cancelation when no longer needed) allowed unlimited downloads of generated voiceovers, with 24 h worth of voice generation per year and commercial usage rights. Furthermore, it provided access to 60 different voices, allowing multiple “virtual” instructors to facilitate online tutorials. Unfortunately, one caveat of using text-to-speech generators is the absence of many languages and a lack of diversity in the ethnicity of the AI “voiceover artist”. An example of the realism achieved with AI text-to-speech generators is presented in Supplementary Audio 1 (Abstract of this article, narrated by “Edward”—a United Kingdom English voice on murf.ai).
Video and Free-Play Tutorial
Although the guided tutorial was created first, we found that the video tutorial was faster to produce, especially in Unity3D. One of the benefits of using Unity3D is its ability to manipulate and code camera(s) to render 3D recreated environments. Using the Unity Recorder add-in allows the user to capture and save data from Unity during play mode—including animations, videos (2D and 360 degrees), images, audio, and more (Unity Technologies, 2021b). Any editing required for the videos was done using Davinci Resolve 17,16 a free-to-use video editing software package. Such tutorials can then be uploaded to the educational content management system of choice or disseminated via online social platforms.
Challenges Experienced During the Creation of Virtual Laboratories During a Pandemic
In section “An Approach to Virtual Laboratories” we reported on our experience in creating a VL, which was done in a game engine and using as many freely available software packages and resources as possible. The monetary cost involved in the creation of the said VLs was $24 US ($5 US for the laboratory environment and $19 US for access to the murf.ai AI-generated text-to-speech service). However, in addition to monetary input, several other factors need to be taken into account in creating VLs as part of the normal daily activities of an academic at an HEI.
Time
The amount of time that it takes to develop depends on the prior knowledge that the developer has in using the software to create training opportunities. In our presented case study, the author was well experienced in the C# programming language and had some prior experience in creating and editing video, although neither of these experiences was the result of formal training. However, completing one VL took more than a 100 h despite prior knowledge of the software used. Academics wanting to explore VLs as a viable alternative to physical laboratory training should consider this in their planning.
However, although the creation of VLs requires an initial investment in time, the experience can be used in subsequent training sessions. VLs are also an excellent asynchronous training method, where students will be allowed to experiment without the need for supervision in a physical laboratory. Shifting some practical to an asynchronous delivery method frees up time in the academic schedule—potentially allowing for additional training modules that would be difficult to model in silico to be included in the curriculum.
Technical Skills
Above, we mentioned that the author had limited experience with some packages implemented in the VL creation process. Although the production of such experiences does not require formal training, it does help speed up the creation process. In our approach toward choosing appropriate tools for creating specific sections of the VL, we searched social media platforms and ensured that the investigated software had an active community of users. As students are turning toward social platforms to enrich their learning experience, so should academics. In doing so, faculty members develop their skillsets, which could then be transferred to new academics and thus transform the academic milieu into a complement of educators who are well versed in various technological solutions.
Working Remotely
When creating virtual laboratories while working remotely, one needs to ensure that communication between collaborators is in place. In this report, a single educator created the different VLs, based on his personal experience teaching the two laboratory techniques to students. This was due to a change in the working environment, where the researcher transferred to a new host institution and had yet to develop collaborative networks at the new host facility. However, the VL creation process can be substantially improved when working in a team environment. Furthermore, by splitting tasks and focusing on the strengths of a collective, VLs can be produced much faster than the mentioned 100 h.
Version control, as well as add-ons facilitating collaborative work for software packages such as Unity3D, will result in teams being agile in their creation of VLs for students. Therefore, although an educator can create their own VLs, the demanding nature of academic life makes it a rather heinous task to achieve while ensuring that all other demands on time are met. As the African saying goes: “If you want to go fast, go alone. If you want to go far, go together.”
The Future of Virtual Laboratories and Conclusion
Millions of biological sciences students are trained in basic laboratory skills, ranging from handling a micropipette to dissecting organisms. From recent events that transpired (and are pretty much still in play) during the global COVID-19 pandemic, access to laboratories for training students is not a given, as was the case a few years ago. With increasing student numbers, and poor access to equipment in resource-limited regions, now is an opportune time to develop an open-source, free-to-use set of virtual laboratories.
In the following months, the author’s team will be launching amakhono.org, intending to host and create free and open-access resources for VL creators. The platform, which is currently in development, will host 3D models of laboratory equipment under Creative Commons licensing; it will also provide practical guidelines on creating VLs and a community resource for hosting VLs for students and other educators to access. Having a free resource, in our opinion, will drive science education into the fourth industrial revolution while allowing students from all walks of life the opportunity to receive a high standard of practical training. Similar to how theoretical education has shifted its trajectory—from face-to-face to online teaching, so too will new technologies, such as extended reality devices, become the VL of tomorrow. However, to reach tomorrow, we need to start building today.
Author Contributions
The author confirms being the sole contributor of this work and has approved it for publication.
Funding
Financial support for this research was provided by the British Council under the Digital University funding mechanism. The researcher purchased the products (software or hardware), and no paid advertisements are included in the presented research. The opinions are based on personal experience using the software to create virtual laboratories.
Conflict of Interest
The author declares that the research was conducted in the absence of any commercial or financial relationships that could be construed as a potential conflict of interest.
Publisher’s Note
All claims expressed in this article are solely those of the authors and do not necessarily represent those of their affiliated organizations, or those of the publisher, the editors and the reviewers. Any product that may be evaluated in this article, or claim that may be made by its manufacturer, is not guaranteed or endorsed by the publisher.
Acknowledgments
The author gratefully acknowledge the on-line community who are actively generating tutorials, practical guidelines, and support channels for developers working on Unity3D, most of which are made freely available via social platforms such as YouTube and Stack Exchange.
Supplementary Material
The Supplementary Material for this article can be found online at: https://www.frontiersin.org/articles/10.3389/feduc.2022.796840/full#supplementary-material
Footnotes
- ^ https://unity.com
- ^ https://www.unrealengine.com/
- ^ https://www.cryengine.com/
- ^ https://assetstore.unity.com/packages/3d/environments/industrial/chemistry-laboratory-188274
- ^ https://www.turbosquid.com/
- ^ https://www.cgtrader.com/
- ^ https://www.shapeways.com/
- ^ https://free3d.com
- ^ https://www.blender.org/
- ^ https://orcid.org/0000-0001-8280-6718
- ^ https://assetstore.unity.com/packages/tools/physics/obi-fluid-63067
- ^ https://www.youtube.com/watch?v=eIZgPAZx56s
- ^ https://www.audacityteam.org/
- ^ https://aws.amazon.com/polly/
- ^ http://www.cross-plus-a.com/balabolka.htm
- ^ https://www.blackmagicdesign.com/hk/products/davinciresolve/
References
Almahasees, Z., Mohsen, K., and Amin, M. O. (2021). Faculty’s and students’ perceptions of online learning during COVID-19. Front. Educ. 6:638470. doi: 10.3389/feduc.2021.638470
Craig, C. L., and Friehs, C. G. (2013). Video and HTML: Testing Online Tutorial Formats with Biology Students. J. Web Librar. 7, 292–304. doi: 10.1080/19322909.2013.815112
Crawford, J., Butler-Henderson, K., Jurgen, R., Malkawi, B. H., Glowatz, M., Burton, R., et al. (2020). COVID-19: 20 countries’ higher education intra-period digital pedagogy responses. J. Appl. Learn. Teach. 3, 9–28. doi: 10.37074/jalt.2020.3.1.7
Cullinan, J., Flannery, D., Harold, J., Lyons, S., and Palcic, D. (2021). The disconnected: COVID-19 and disparities in access to quality broadband for higher education students. Int. J. Educ. Technol. High. Educ. 18:26. doi: 10.1186/s41239-021-00262-1
Dere, S., Sahasrabudhe, S., and Iyer, S. (2010). “Creating open source repository of 3D models of laboratory equipments using Blender,” in 2010 International Conference on Technology for Education, (Manhattan: IEEE), 149–156. doi: 10.1109/T4E.2010.5550044
Dholakiya, N. S., Kubík, J., Brozek, J., and Sotek, K. (2019). “Unity3D Game Engine Applied to Chemical Safety Education,” in Applied Physics, System Science and Computers III, eds K. Ntalianis, G. Vachtsevanos, P. Borne, and A. Croitoru (New York: Springer International Publishing), 81–87. doi: 10.1007/978-3-030-21507-1_12
Dumford, A. D., and Miller, A. L. (2018). Online learning in higher education: exploring advantages and disadvantages for engagement. J. Comp. High. Educ. 30, 452–465. doi: 10.1007/s12528-018-9179-z
Gamage, K. A. A., Wijesuriya, D. I, Ekanayake, S. Y., Rennie, A. E. W., Lambert, C. G., and Gunawardhana, N. (2020). Online Delivery of Teaching and Laboratory Practices: Continuity of University Programmes during COVID-19 Pandemic. Educ. Sci. 10:291. doi: 10.3390/educsci10100291
Hale, T., Petherick, A., Phillips, T., and Webster, S. (2020). Variation in government responses to COVID-19. Blavatnik School Gov. Working Paper 31, 2020–2011.
Hampton, D., Welsh, D., and Wiggins, A. T. (2020). Learning Preferences and Engagement Level of Generation Z Nursing Students. Nurse Educ. 45, 160–164. doi: 10.1097/NNE.0000000000000710
Hedding, D. W., Greve, M., Breetzke, G. D., Nel, W., and Van Vuuren, B. J. (2020). COVID-19 and the academe in South Africa: Not business as usual. South Afr. J. Sci. 116, 1–3. doi: 10.17159/sajs.2020/8298
Jena, P. K. (2020). Impact of Covid-19 on higher education in India. Int. J. Adv. Educ. Res. 5, 77–81.
Joaquin, J. J. B., Biana, H. T., and Dacela, M. A. (2020). The Philippine Higher Education Sector in the Time of COVID-19. Front. Educ. 5:576371. doi: 10.3389/feduc.2020.576371
Keil, J., Edler, D., Schmitt, T., and Dickmann, F. (2021). Creating Immersive Virtual Environments Based on Open Geospatial Data and Game Engines. KN J. Cartogr. Geogr. Inf. 71, 53–65. doi: 10.1007/s42489-020-00069-6
Mhlanga, D., and Moloi, T. (2020). COVID-19 and the Digital Transformation of Education: What Are We Learning on 4IR in South Africa? Educ. Sci. 10:180. doi: 10.3390/educsci10070180
Minocha, S., and Burden, D. (2013). “STEM education with Unity 3D,” in Virtual Worlds Education Roundtable (VWER). Available online at: http://oro.open.ac.uk/39529/
Mpungose, C. B. (2020). Emergent transition from face-to-face to online learning in a South African University in the context of the Coronavirus pandemic. Human. Soc. Sci. Commun. 7:113. doi: 10.1057/s41599-020-00603-x
Müller, C., and Mildenberger, T. (2021). Facilitating flexible learning by replacing classroom time with an online learning environment: a systematic review of blended learning in higher education. Educ. Res. Rev. 34:100394. doi: 10.1016/j.edurev.2021.100394
Nguyen, T. (2015). The effectiveness of online learning: beyond no significant difference and future horizons. MERLOT J. Online Learn. Teach. 11, 309–319.
Ouyang, S.-G., Wang, G., Yao, J.-Y., Zhu, G.-H.-W., Liu, Z.-Y., and Feng, C. (2018). A Unity3D-based interactive three-dimensional virtual practice platform for chemical engineering. Comp. Appl. Eng. Educ. 26, 91–100. doi: 10.1002/cae.21863
Palvia, S., Aeron, P., Gupta, P., Mahapatra, D., Parida, R., Rosner, R., et al. (2018). Online Education: Worldwide Status, Challenges, Trends, and Implications. J. Glob. Inform. Technol. Manage. 21, 233–241. doi: 10.1080/1097198x.2018.1542262
Paudel, P. (2021). Online education: benefits, challenges and strategies during and after COVID-19 in higher education. Int. J. Stud. Educ. 3, 70–85.
Reeves, S. M., and Crippen, K. J. (2020). Virtual Laboratories in Undergraduate Science and Engineering Courses: a Systematic Review, 2009–2019. J. Sci. Educ. Technol. 30, 16–30. doi: 10.1007/s10956-020-09866-0
Udin, W. N., Ramli, M., and Muzzazinah. (2020). Virtual laboratory for enhancing students’ understanding on abstract biology concepts and laboratory skills: a systematic review. J. Phys. 1521:042025. doi: 10.1088/1742-6596/1521/4/042025
Unity Technologies (2021a). Model file formats. Available online at: https://docs.unity3d.com/Manual/3D-formats.html (accessed on Sep 13, 2021).
Unity Technologies (2021b). Recorder. Available online at: https://docs.unity3d.com/Manual/com.unity.recorder.html (accessed on Sep 13, 2021).
World Economic Forum (2020). “Higher Education: In-Person or Online?”in 29-Country Ipsos Survey for the World Economic Forum. Paris: Ipsos. Available online at: https://www.ipsos.com/sites/default/files/ct/news/documents/2020-11/higher-education-in-person-or-online.pdf
Yulia, C., Hasbullah, H., Nikmawati, E. E., Mubaroq, S. R., Abdullah, C. U., and Widiaty, I. (2018). Augmented reality of traditional food for nutrition education. MATEC Web Conf. 197:16001. doi: 10.1051/matecconf/201819716001
Zalat, M. M., Hamed, M. S., and Bolbol, S. A. (2021). The experiences, challenges, and acceptance of e-learning as a tool for teaching during the COVID-19 pandemic among university medical staff. PLoS One 16:e0248758. doi: 10.1371/journal.pone.0248758
Keywords: Unity3D, gamification, remote higher education, online laboratory, laboratory skills
Citation: Myburgh PH (2022) Reflecting on the Creation of Virtual Laboratory Experiences for Biology Students. Front. Educ. 7:796840. doi: 10.3389/feduc.2022.796840
Received: 17 October 2021; Accepted: 22 June 2022;
Published: 14 July 2022.
Edited by:
Subramaniam Ramanathan, Nanyang Technological University, SingaporeReviewed by:
Spencer A. Benson, Education Innovations International, LLC, United StatesAlev Elçi, Aksaray University, Turkey
Copyright © 2022 Myburgh. This is an open-access article distributed under the terms of the Creative Commons Attribution License (CC BY). The use, distribution or reproduction in other forums is permitted, provided the original author(s) and the copyright owner(s) are credited and that the original publication in this journal is cited, in accordance with accepted academic practice. No use, distribution or reproduction is permitted which does not comply with these terms.
*Correspondence: Pieter Hermanus Myburgh, hermanm@uj.ac.za; orcid.org/0000-0001-8280-6718