- 1Digital Education Research Centre, Moray House School of Education and Sport, University of Edinburgh, Edinburgh, United Kingdom
- 2Learning Sciences Research Institute, School of Education, University of Nottingham, Nottingham, United Kingdom
Covid-19 has significantly impacted children’s lives, requiring them to process multiple messages with significant emotional, social, and behavioural implications. Yet, the vast majority of these messages solely focus on behaviour. This is an oversight as children and young people can understand the biological properties and mechanisms of viruses when supported appropriately, thereby presenting an important opportunity for educators. However, like many other invisible scientific phenomena, understanding of viruses greatly depends upon how they are represented. Thus, we sought to understand the relative benefits and limitations of different forms for learning about the underlying biology of Covid-19. Applying an embodied learning lens, we analysed pictures, 3d models, gestures, dynamic visualisations, interactive representations, and extended reality identified through a state-of-the art-review. In so doing, we address the affordances and limitations of these forms in general and variation within them. We used this to develop a representational checklist that teachers and other adults can use to help them support children and young people’s learning about the biology of Covid-19.
1 Introduction
Covid-19 * has significantly impacted children’s lives within and beyond the classroom, requiring them to process multiple messages that have significant emotional, social and behavioural implications. Whilst these messages are predominately focused on health behaviours, we arguably have a responsibility to help children’s understanding of the biology underpinning these messages. Greater knowledge can positively influence attitudes, and may also (although not yet clear) foster improved health behaviour (Myant and Williams, 2005). This increased need to support children and young people’s knowledge about Covid-19, and viruses more generally, calls for greater consideration of the resources available to teachers.
Understanding viruses is challenging; yet children’s difficulties are less likely to be about inherent cognitive limitations and more about how we explain viruses and the way that they are represented. Yet, there is a lack of critical examination of the differences between representations of Covid-19, and their relative merits and limitations for supporting learning. The contribution of this paper is to offer a new perspective concerning the support of children’s learning of viruses through a framework informed analysis of representations of Covid-19 and viruses more broadly identified by a state-of-the-art review. Then, from this analysis, draw implications for education to help teachers in making appropriate representational choices. The question underpinning the contribution of this paper is: What are the relative benefits and limitations of different forms of representations for learning about the underlying biology of Covid-19?
2 Representing Covid-19 for Learning
Given the impact of Covid-19 on children’s lives, it is encouraging that the pandemic has already been met with a plethora of resources, from websites and video explanations to books and even games, to help teachers and parents explain the Covid-19 pandemic for children and young people. Examination of these resources, however, reveals their dominant focus: the observable consequences of Covid-19, such as symptoms and healthy behaviours. Typically, there is less explanation of biological information underlying the virus itself. As an example, in a comic for children developed from interviews by the independent non-profit media organisation NPR with experts, biological information is limited to a simple reference to the virion’s visual appearance (Figure 1).
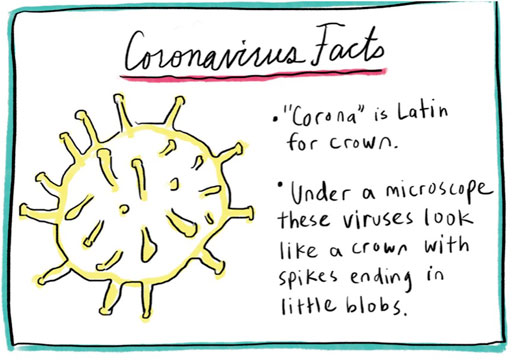
FIGURE 1. Coronavirus facts (Gharib & Turner, 2020).
There are various possible explanations for why the majority of resources for children concerning Covid-19 make little effort to help them understand biological aspects of the virus: for example, it might be considered frightening or simply not a current priority. Yet, there is good reason to believe that greater understanding offers children greater agency in interpreting and managing the social and emotional impact as well as positive attitudes towards health and health promotion (Piko and Bak, 2006). Knowing how the virus replicates, for example, may help children understand why you may become ill days after becoming infected. Understanding how the spikey shapes depict proteins may help children towards understanding how the virus enters our cells, and how vaccines operate.
Another explanation for this gap is that the biological properties and mechanisms of Covid-19 might be considered too complex for children, especially younger children. From a Piagetian perspective, younger children are considered to struggle to comprehend biological concepts to which they have no sensory access (Bibace and Walsh, 1981). Indeed, this raises a significant challenge in learning about viruses: children cannot see, hear, touch or interact with them directly. Yet, contemporary theories suggest that children are capable of developing naïve (nascent) theories when given appropriate experience (Hatano and Inagaki, 1994) and interventions to improve young children’s knowledge of illness are effective (e.g., Williams and Binnie, 2002). Such naïve theories emerge early; for example, work has shown that children as young as 3 years old can begin to understand concepts such as contagion and contamination of food (Siegal, 1988).
Research has continually demonstrated the potential to engage children with complex scientific ideas through appropriate models, guidance and scaffolding. So, the challenge is less about children’s cognitive limitations and more about how potentially complex information is presented to children (Bruner, 1960). From this perspective, current publicity surrounding viruses might be perceived as a valuable educational opportunity – a rich set of resources with which to engage and learn about viruses. Thus, like many other invisible scientific phenomena (e.g., atoms, galaxies, inside our bodies) our understanding of viruses depends greatly upon how they are represented (Gilbert, 2005). In the case of Covid-19, these include webpage images, everyday language, anthropomorphised toys and video games. This poses some fundamental questions for research. In what ways have viruses in general, and Covid-19 specifically, been represented? And to what extent can these representations support or hinder, children’s understanding? To start to address these questions, we first need to consider the learning domain.
2.1 The Learning Domain: What Needs to be Represented
Children’s, young people’s, and even university students’ understanding of viruses has been found to be limited and fragmented (e.g., Byrne, 2011; Simon et al., 2017). A fundamental challenge is to understand what a virus is. Children and young people have a tendency to see viruses from an anthropocentric perspective: how they affect and harm humans rather than their more neutral role as micro-organisms within an ecosystem (e.g., Byrne et al., 2009); for example, attributing them with free will and a malevolent desire to spread and infect humans (Byrne et al., 2009; Simon et al., 2017). Idoiaga et al. (2020) found that children represented Covid-19 as an enemy that doctors are fighting. Learners can be confused as to whether a virus is alive. Jones and Rua (2006) found few adults and no high school students understood that viruses are not considered as living as they lack key characteristics of life such as reproductive autonomy. In fact, in Byrne (2011), children argued that viruses were alive as they needed to move and kill people.
As well as potential misunderstanding of the difference between a virus and a viral disease (arguably not helped by using the term Covid-19 to refer to both), many children do not understand the difference between micro-organisms such as bacteria, viruses, and fungi (Simonneaux, 2000; Jones and Rua, 2006; Byrne, 2011). Furthermore, they may believe that bacteria cause viruses (Simonneaux, 2000; Jones and Rua, 2006). A related challenge is understanding viruses’ structural properties. Findings have shown that students rarely knew what viruses consist of, and young people confuse the structure of viruses with other microscopic organisms (e.g., Simonneaux, 2000; Jones et al., 2003) or, when younger, even with small creatures such as insects (Byrne, 2011).
A key challenge for understanding viruses is their size. A typical Covid-19 particle (60–140 nm) is around 1/400th the diameter of a single human hair (60–120 µm). Jones and Rua (2006) found that children and young people often believed that viruses are bigger than bacteria. Tretter et al. (2006) report that younger children thought viruses were bigger than small visible objects like grains of rice. Misunderstanding of size is associated with further misconceptions such as the virulence of a disease or that, as in Byrne (2011), children thought it was easier to catch viral diseases because viruses are bigger. Jones et al. (2003) also found that only 12% of their American high school student sample understood viruses to be three-dimensional.
Learners can be unclear about how viruses replicate. Simon et al. (2017) found that high-school students often held misconceptions such as a virus replicates by division. Jones and Rua (2006) found only 2 of 63 interviewed students understood the basics of replication. This might explain why students can interpret a virus’s growth in terms of its size rather than quantity. Children also may not understand how viruses cause illness. They can, for example, conflate getting ill with direct environmental factors: “I got a cold because I was cold” (e.g., Byrne, 2011). They may also apply a social justice rationale: your own misdeeds cause you to become ill (e.g., Raman and Winer, 2004). It is unlikely that younger children will know how the immune system responds to viral infections and how the symptoms of viral illness are often due to immune system response (Simonneaux, 2000). High school students did show greater understanding, but this was typically anthropomorphised and couched in terms of war (Simonneaux, 2000).
It is unclear the extent to which frequent home, school and media discussions of the Covid-19 virus may have influenced these findings. However, this brief review does illustrate the problems that children can face in defining viruses, describing their morphology (size, shape and structure), replication and association with illness and immune system responses. The representations they experience may help them learn about these concepts but, in the worst case, could possibly reinforce misconceptions.
2.2 Analytic Review
The question underpinning the contribution of this paper is: What are the relative benefits and limitations of different forms of representation for learning about the underlying biology of Covid-19? Our focus is children and young people aged 4–16 (reflecting a typical age range for compulsory education), although for brevity we often use just the term “children.” To address this question, it was first necessary to articulate a framework that could be used to examine these differences, and which then informed a state-of-the art review of existing representations of Covid-19 (or where these could not be found, representations of viruses more generally).
The framework for representations presented in this paper is situated within the theoretical paradigm of Embodied Cognition. In contrast to information processes theories of cognition, Embodied Cognition is an umbrella term capturing growing claims that cognitive processes are deeply rooted in, and inseparable from, the body’s sensorimotoric interaction with the world (Wilson, 2002; Barsalou, 2008). Given the implications for designing embodied experiences to foster conceptual development, this theoretical paradigm has fuelled increasing research in education (e.g., Kontra et al., 2012; Lindgren and Johnson-Glenberg, 2013; Abrahamson et al., 2020). Embodied cognition also emphasises the metaphors and analogies that help learners understand new concepts by basing them upon familiar interactions in the world (Glenberg, 2010). Recently, Pande (2020) has drawn upon this paradigm to argue that to understand learning with representations of scientific phenomena we need to understand their interactive affordances. Adopting this theoretical framing for this paper directs our attention to perceptual information and the actions that representations allow the learners to perform, as well as the social and affective processes the representations engage. It therefore motivates our categories of representational form. Finally, it should be noted we do not consider written or spoken language in isolation, instead assuming they are an invariant part of the wider context of the representations. Consequently, the representational forms we consider are:
1) visual (static) representations (e.g., pictures or diagrams);
2) physical 3d representations (e.g., model or toys);
3) gestures (representational);
4) visual dynamic representations (e.g., animation);
5) interactive representations (e.g., computer games and simulations), and;
6) extended reality (representational forms that integrate digital information with the physical world such as haptics and tangibles).
We analyse representations of Covid-19 by utilising a state-of-the-art review drawing upon both peer-reviewed academic publications and publicly available online grey literature (e.g., websites, blogs, resource downloads, news items) that were described in English. For the review, we drew upon our framework as well as other more general search terms to minimize the risk of missing out examples, including those capturing the media (e.g., image, representation, animation, toy, physical, picture, video), the pedagogical intention (e.g., learning, educational, explanation, children) and the domain (Covid-19, coronavirus, virus). Our intent was not to analyse all representations of Covid-19 available as this would be impossible given their rapidly increasing numbers. Instead, we intended to find, analyse and illustrate the variety of forms of representation of the virus concepts described in Section 1.2. The availability of examples varied significantly between forms. For representational forms where there were many examples available (e.g., visual static, visual dynamic), typical cases were purposely selected by the authors to draw attention to specific representational features that educators need to evaluate. For other forms (e.g., physical, gesture, interactive, extended), there were far fewer examples available and consequently there was less choice about what to focus upon and illustrate.
3 Representations of Covid-19: Case Analysis
Each of the six forms of representations has different affordances and constraints shaping how learners interpret, interact, construct, communicate and ultimately learn about Covid-19. For each representational form, we follow the following approach:
1) Definition and illustration of its key properties;
2) Presentation of Covid-19 or virus case examples;
3) Detailed analysis of benefits and limitations through an embodied learning lens highlighting properties common to the form and variation within it.
Our search criterion was representations that included information content relevant to the learning domain for children aged 4–16 years as described in Section 2.1. Our selection criterion for case examples was those that were able to illustrate representational features for discussion. Although analysis does consider benefits and limitations of representational features, the intention is not to evaluate individual representations or forms, nor indicate which are more apt for learners. This is because their value will greatly depend upon the context of use, including the learning objectives, preferences, ability and prior experiences of learners, knowledge and pedagogical approach of the teacher and the availability and use of other representations.
3.1 Visual (Static) Representations
Visual representations encompass a broad range of representations, including pictures, diagrams, images, drawings and photographs, which use a 2-dimensional space (e.g., paper, screen) in meaningful ways. Whilst they typically provide visuo-spatial resemblance to the represented concept, certain features may be selected or exaggerated in order to make salient particular properties (Tversky, 2011). They permit limited abstractions but in contrast to language, these abstractions are specific: i.e., they have to commit to many explicit choices (Stenning and Oberlander, 1995). They often contain text in the form of keys and labels, but visual information is the most central feature. They can have high information density (particularly in the case of scientific “textbook” diagrams) but also can be a simple low-information density sketch. Visual representations can excel at supporting social interaction, they disambiguate communication between collaborators when they can be jointly viewed or gestured towards (Roschelle and Teasley, 1995). Moreover, they are (mostly) easy for learners to construct for themselves. They can also be collaboratively constructed, acting as “Negotiation Potentials” as collaborators become more aware of the need to discuss and agree on changes to them (Suthers, 2014).
The static-visual case example in Figure 2A is a popularly shared representation (highest ranked image of Covid-19 on Google Image search on fifth May 2021) created at the Center for Disease Control and Prevention (CDC). The representation reveals the external architecture of the virion when viewed via an electron microscope. It makes visible a number of key structural aspects of Covid-19 with the most salient being a focus on the virion’s distinctive shape – i.e., multiple protein spikes around the circular envelope of the virion. It emphasises that these spikes are of a different nature to the envelope by using colour coding, and that these spike proteins are not the only protrusions from the envelope. To help readability, it is presented alone (a single virion) against a dark neutral background.
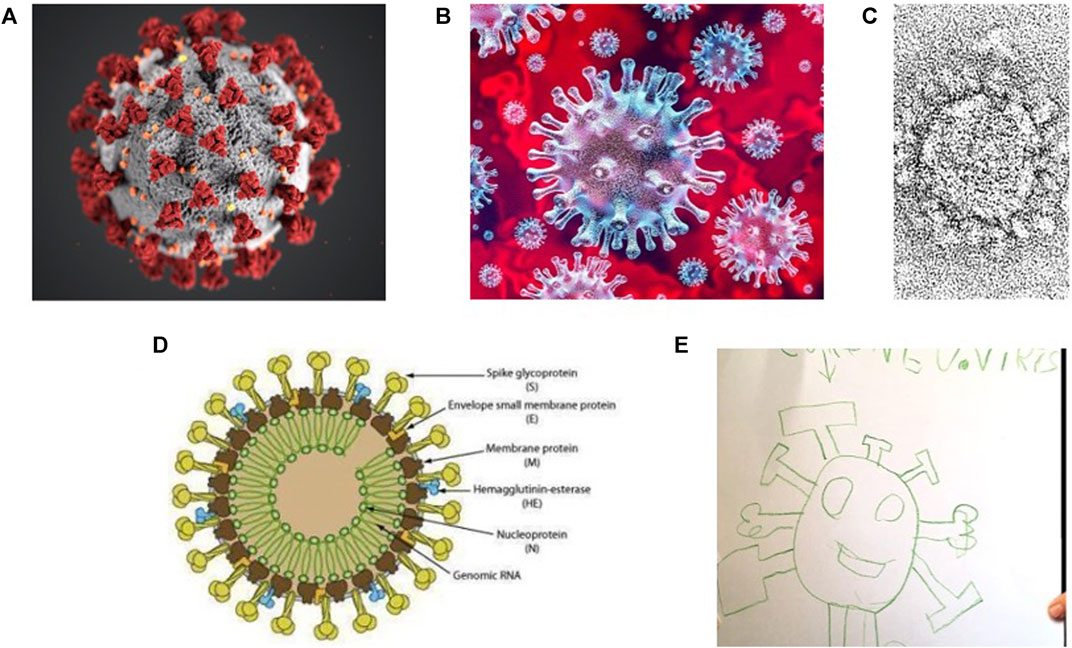
FIGURE 2. Static Visual representations (A) Stylised Covid-19 ultrastructural morphology (Eckert & Higgins, 2020) (B) Stylised and decorative illustration of Covid-19 virions, (WebMD, 2021) (C) Electron microscopic image of a negatively stained particle of Covid-19 (Goldsmith & Tamin, 2020) (D) Scientific “textbook” type image including labels and cutaways (Kamde et al., 2020) (E) Child’s drawing of the virus (Gaulkin, 2020).
Whilst Figure 2A clearly illustrates Covid-19 shape and form, size is more problematic as no scale or comparison is offered. The focus on a single virion obscures the multitudinous nature of the virus, which is key to understanding concepts such as replication. Other representations (e.g., Figure 2B) address this limitation although the use of size to represent perspective in 2d risks confusing understanding of size.
Figures 2A,B exemplify the way many visual representations of Covid-19 are simplified and use colour for effect. They are frequently used as background for media articles. This use of colour can be contrasted with the black and white transmission electron microscope image of Figure 2C. This form of image is rarely presented although it provides the source for many others. Colour can help make different aspects of visual representations salient – from the protein spikes (Figure 2A) to the outline of multiple virions against the ambiguous bloodlike background of Figure 2B. However, the arbitrary nature of the colour choices is almost invariably not described. Consequently, it is possible that, as with children’s interpretation of other invisible objects (e.g., atomic element and modules) the macroscopic concepts of colour might be imported to the microscopic level (Barke et al., 2009). Moreover, if this colour becomes conventional (like in molecule building sets where green is always chlorine, yellow is always sulphur) it is likely that children will believe that protein spikes are red. It is noticeable how often red is used for either the spike proteins or the whole virion – possibly leveraging associations of the colour red with danger or energy (Kress and Van Leeuwen, 2016). It is therefore important to consider how arbitrary visual choices such as colour can encapsulate certain affective metaphors, and possibly lead to misconceptions (e.g., that viruses only travel in blood).
By focusing on a singular virion without surrounding context or other virions, Figure 2A has moderate information density. Significantly, like most pictures, it does not show inside the protein envelope, RNA is not depicted, and it would be easy to believe the virion is either “solid” or “empty.” This picture may therefore not support understanding of much of viral morphology or replication. Moreover, it is rarely presented with a caption (or a key as in textbooks) and thus for many, the original intent to show different protein forms (though colour and shape) may be lost. This can be contrasted with Figure 2D which offers greater information density – a cutaway to illustrate RNA inside the virion, and labels to identify different parts. Clearly, such labels require further explanation for learners unfamiliar with these terms.
Whilst Figures 2A–D are expert representations, Figure 2E illustrates a very different form - a pen and paper drawing constructed by a child. As a constructed representation, it offers insight into the child’s understanding (Ainsworth et al., 2011), although the context and task instructions would need to be taken into account. The representation echoes many of the features of the expert created illustrations (different proteins are signified by the different shaped proteins from the spherical envelope, single virion, no background). The key difference is the anthropomorphic nature of the representation: legs, eyes and a smiley face, although, angry ones are equally if not more common as Covid-19 is often depicted as a hostile agent, intently angry or gleeful about infecting humans. Representing viruses like this could lead children to be unwilling to consider viruses neutrally, independent of their impact on other life forms, or the benefits that viruses bring to human life (Byrne et al., 2009).
3.2 Physical Representations
The two-dimensional nature of visual representations can be contrasted with more tangible forms of representations from toys to three-dimensional scientific models. The use of such physical representations has a long history in education although there continues to be debate over what benefits, if any, physicality offers learners (e.g., McNeil & Jarvin, 2007). Some of the proposed mechanisms refer to how the unique sensory and manipulative properties of physical representations help support certain cognitive tasks. Physical representations, for example, provide three dimensional and tactile information and can typically be explored through simple physical manipulation in the space around the learner (Manches and O’Malley, 2011). Whilst evidence is limited, the tactile nature of physical representations may offer emotional engagement (Moyer, 2001), whilst the ability to view from multiple angles and physically pass between individuals can help support a shared, social, experience. It is also possible that actions afforded by physical materials have more direct cognitive benefits; from an embodiment perspective, certain actions may themselves help develop or strengthen key motoric representations underpinning concepts at hand (Pouw et al., 2014).
Our review revealed a range of physical representations, from toys to more scientific models, often created through 3D printouts. Figure 3A is an indicative example of a Covid-19 toy. It is a plush (soft) toy, measuring 15 cm and is sold as an adult gimmick rather than educational toy. Similarly to the visual representations, colour is used arbitrarily to distinguish the virion body from protein spikes. Again, red is dominant, although for the main body here. As is common with many microbe toys, the representation is anthropomorphised, although in this example only with eyes. Similar to many visual representations, it consists of a single virion; although arguably this is even more likely for physical representations given the costs and practicalities associated with presenting multiple objects. In contrast to most representations where the virion size is typically unspecified, the physical nature of the representation commits to a size: that of a hand which has a clear potential to be misleading.
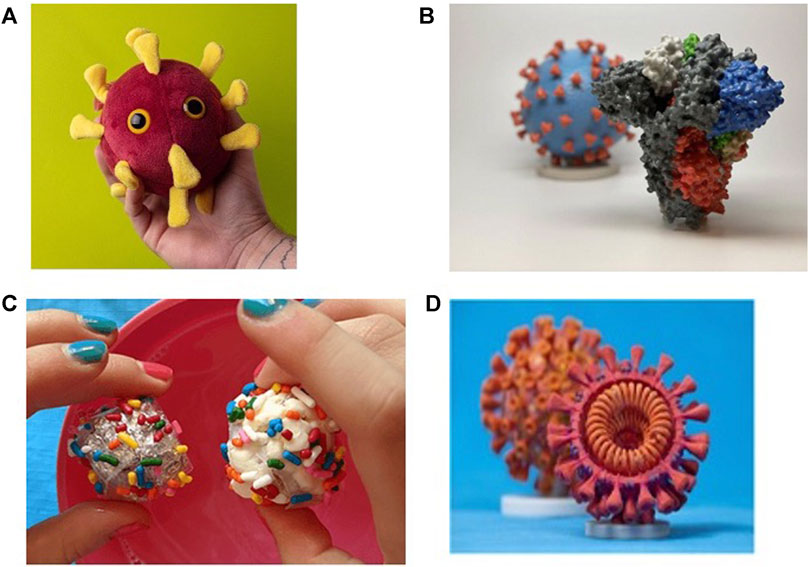
FIGURE 3. Physical representations (A) Covid-19 plush toy (Firebox, 2021) (B) 3D Covid-19 spike protein (NIAID, 2020) (C) 3D printed model of Covid-19 (Tino et al., 2020) (D) Constructed “enveloped” virus model (Slutz, 2020).
The toy in Figure 3A offers tactile information: it allows children to explore key features (e.g., protein spike) through touch rather than just visually. Such tactile information enables children to free up visual attention: they can inspect the representation through touch whilst attending visually to other information – such as the teacher or peers talking (Manches & O’Malley, 2011). Tactile information also includes the feel of the material, although the soft fabric here is more indicative of a generic plush toy than any relevant biological information (the ubiquity of soft toys for younger children though suggests this may offer some emotional engagement). Most relevant, from an embodied learning perspective, are the manipulative properties of this physical representation. It is possible to represent and communicate ideas with actions using the representations, for example, tracing the movement of the virus from the mouth toward surfaces, or from one person to another to represent transmission (literally “giving someone the virus”). Importantly, such physical actions can be co-presented with explanatory speech, helping ground language in a similar way to gestures (See 2.3).
In contrast to the more playful representation of Figures 3A,B, exemplifies a more formal scientific physical representation of Covid-19, predominately designed for clinical settings. Here we see a 3D print of a spike protein of Covid-19 in front of a virus particle. The example illustrates how more recent technologies such as 3D printers offer opportunities to create and share more detailed physical representations. The model further illustrates the potential of conveying 3-dimensional information, which is significant in light of evidence (e.g., Wu and Shah, 2004) that learners can have difficulties in visualising three dimensions from 2d representations and that children can struggle to understand viruses as 3d (Jones et al., 2003). Figure 3C also illustrates the potential to provide more detailed 3-dimensional representation of the inside of a virion. Compared to many representations, the ability to provide this greater detail is more complicated and has limitations – such as the difficulties of annotation with text. However, recent work in information visualisation has argued for the emotional and communicative benefits of physicalization (Jansen, et al., 2015).
Where Figures 3A–C are “presented” representations, physical materials present a range of opportunities for learners to construct their own. The Medical Research Council, UK, for example, provides an activity pack for children (7 + years) to build a virus model. Here, the value of construction should be understood to be in the meaning-making process rather than scientific accuracy of the outcome. This is exemplified in a blog post (Slutz, 2020) describing a parent who led an activity at home with her child following a video-based resource by the organisation “Science Buddies” to explain differences between non-enveloped and enveloped viruses (Figure 3D). The parent chose the activity in response to her child not understanding why she had to thoroughly wash with soap after being in public during the pandemic. The video explains the activity: create two balls using aluminium foil each covered in double sided sticky tape. Coat the first directly with sprinkles, for the second, coat in butter (representing a lipid membrane) and then sprinkles. Then place both in hot soapy water. By seeing how the “protein spikes” are moved on the buttered model, the parent had a highly meaningful context to talk about washing hands with respect to the underlying biological processes. This example illustrates an important point of an embodied view of representational learning; that it involves physical activity, dialogue, and relation to personal experience. The pedagogical value of such learner-led construction is recognised in initiatives such as the “maker movement” which applies informal hands-on making activity to more formal education in areas such as STEM (Science Technology Engineering and Mathematics) (Halverson and Sheridan, 2014). Whilst constructing science representations can involve a range of media (e.g., paper, digital), physical materials arguably offer more learner choice with respect to materials and design, discussion of which can offer opportunities for meaningful dialogue with respect to what is being represented.
3.3 Gesture
In the last few decades, there has been increasing attention to a form of holistic visuo-spatial representation that we generate, often spontaneously, when speaking – our gestures (Kontra, et al., 2012). Whilst there are various factors shaping if and how we gesture, such as culture, context or individual differences (Hostetter and Alibali, 2008), research has shown that gestures often represent the concepts being communicating (directly or metaphorically) (McNeill, 1992) and are often used to explain ideas – notably when the subject matter is considered difficult for the learner (Hostetter and Alibali, 2008).
We now know that an important function of gestures is to support the speaker as well as the listener (Goldin-Meadow (2011) - gestures helps us to externalise the embodied nature of how we think, where they often simulate (Hostetter and Alibali, 2008) or represent (Novack and Goldin-Meadow, 2017) our prior sensory and action experiences in the world. Empirical work has indicated the potential value of gestures for learning - from capturing learners’ understanding and “readiness to learn,” to the positive influence of teachers’ gesture both directly (by influencing children’s thinking) or indirectly (by helping teachers clarify their own thinking when communicating) [see Goldin-Meadow (2011) for overview].
In comparison to other representational media, the information represented in gestures is highly constrained to what can be depicted by the body and predominately by our hands. Yet, whilst lacking in informational density, gestures can readily produce dynamic visuo-spatial schematical information quickly and easily. Importantly, they can complement information in speech, which is why the meaning of gestures is typically interpreted in conjunction with co-produced speech (Parrill and Sweetser, 2004). For younger learners, gestures often convey information that children cannot yet produce in speech, hence, work has examined their potential to reveal conceptual understanding (Kim et al., 2011). Indeed, mismatches between speech and gestures have been shown to indicate readiness to learn (Goldin-Meadow, 1997). Unfortunately, because gestures are created, and disappear, quickly, they do not offer teachers or learners much time to inspect as a representation. However, like speech, they can be produced to support engagement with other representations, for example, gesturing with videos or pictures (Scheiter et al., 2020).
Identifying the types of gesture generated to represent biological knowledge about Covid-19 is difficult. From our review, most explainer videos feature voice over animated content. Where the speaker is visible, the camera often cuts off at the shoulders, so any gesturing is not visible. This itself indicates that the potential value of gestures as representations may be under-recognized. However, we did find several videos where the speakers’ hands are visible. In each case, the speaker is gesturing. Figure 4A is screen shot of one explainer video by a well-known children’s UK TV presenter. In the video, he creates a range of gestures; in the frame illustrated, the speaker is gesturing when referring to the virus mutating. As with many other gestures in the video, the shape of their hand appears to hold a spherical shape – representing the virion. In this particular gesture, the speaker simulates rotating the virus clockwise then anti-clockwise like a dial whilst referring verbally to the virus “mutating.” Whilst mutating and rotating are clearly different actions, “mutating” is likely a difficult and unfamiliar term for children and this simple dynamic gesture offers a metaphorical representation of something varying to provide meaning.
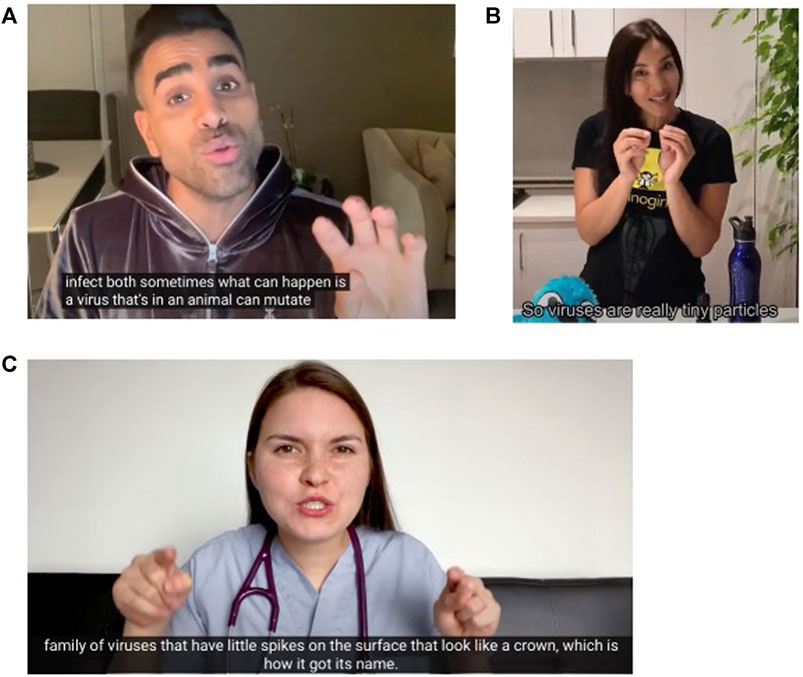
FIGURE 4. Representational gestures (A) Mutate gesture (DrRanjOnline, 2020) (B) Tiny particles gesture (DrMichelleDickinson, 2020) (C) Spikes on the surface gesture (ViolinMD, 2020).
Whilst a key goal of the speaker in Figure 4A is to provide reassurance to young children, other explainer videos intend to provide more scientific explanation. In Figures 4B,C for example, the speakers are practicing doctors. The frame in Figure 4B shows the speaker emphasising how small the virus is; in Figure 4C the speaker uses her hands to outline the shape of protein spikes on the virus. Whilst her gestures correspond to speech, they provide additional information to help visualise the virion. She includes gestures to communicate dynamic processes, such as rolling hands to communicate the ongoing replication of the virus inside a body or pointing fingers from away to her body and then toward her body to depict the process of infection. Whilst informationally simple, these gestures help explain co-produced linguistic terms that may be less familiar to learners - such as replicate, infect, or incubation. Importantly, from an embodied perspective, this form of communication is providing children with dynamic visuo-spatial resources to construct their own meaning and subsequently communicate understanding of the virus.
3.4 Dynamic Visualisation
Dynamic representations are frequently used to represent important biological processes. Hence, our focus now turns to (pictorial) animation using Bétrancourt and Tversky (2000) definition, i.e., “a series of frames so each frame appears as an alternation of the previous one” (p. 313). Animations are typically used to make salient changes over time. Animations are also determined with respect to time, i.e., they cannot be ambiguous about the sequence in which something occurs just like a 2d picture cannot be ambiguous about the shape of something. Animations can contain text or labels and vary in information density; additionally, they can also include audio information, like explanatory voiceovers. The example in Figure 5 illustrates four frames selected from a narrated medical animation created by a US company Scientific Animations (Scientific Animations, 2020). This particular sequence (around 10 s from the 6.08-min animation) shows multiple virions in the context of other cells, then one attachment of the spike protein to a bigger cell, then entry of the virion into the cell, before moving on to describe how the virion spreads its RNA.

FIGURE 5. Dynamic processes of virion (Scientific Animations, 2020).
Animations such as Figure 5 excel at showing the dynamic processes that are not easily observed in real life because of the spatial properties (e.g., size) or temporal scales at which these processes occur. Hence, they are particularly useful for representing microscopic dynamic processes such as viral replication. Whilst a series of static pictures could be used (as in Figure 5), an animation conveys the dynamic nature clearly as it need not show discrete steps but instead displays a continuous process. This can be helpful if learners are unable to mentally simulate intermediate stages (e.g., Schnotz and Rasch, 2005) or when they benefit from the opportunities to develop a fuller mental simulation of the phenomena (Black, 2010). Transitions can help clarify changes, such as why the background is different in the final figure (the virion is now inside the cell). By following a single virion through time it also can become easier to understand the virus lifecycle and the different sizes of virion, proteins and cell components are clearer. The accompanying narration provides much of the information needed to help understand this process and such narrations enhance the effectiveness of animations (Mayer and Sims, 1994). It is also the medium where uncertainty is communicated (in this case the mechanism of adsorption) as it is difficult for animations to convey ambiguity. This example also illustrates two problems with animations. Firstly, to simplify the explanation, a single virion becomes the focus of animation. This reduces the considerable perceptual demands of simultaneously viewing multiple moving images (e.g., Bétrancourt and Tversky, 2000). But, in so doing may lead viewers to consider the process of viral replication as involving only one virion/cell at a time. Secondly, it explains changes over time (replication for example) at a rate adjusted to need of the viewer to understand these complex dynamic processes and not at the speeds that they occur in reality. It is not easy therefore to understand the timescale of replication from such a representation.
We have chosen a second animation (Figure 6) of how infection spreads through a population to illustrate other benefits and disadvantages of this representational form. It shows the number of people who get infected over time, how this is associated with contact and how this accelerates when the barrier is open (representing going from quarantine to no quarantine). It thus fulfils what Schnotz and Rasch (2005) refer to as the facilitating function of animation by helping people build dynamic mental models of situations.
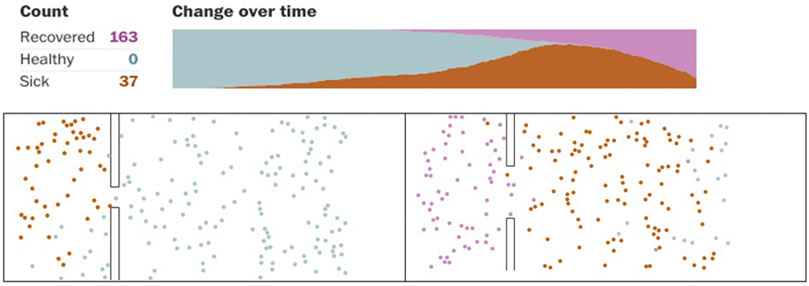
FIGURE 6. An animation of the spread of Simulitis depicting individuals as infected (orange), recovered (pink) and healthy (green) balls that move around randomly. As the underlying simulation runs, over time, more people become infected and then recover. Infection occurs when two balls bump into each other (Stevens, 2020).
Figure 6 also demonstrates some of the particular challenges associated with animations. It runs through a single cycle from start to finish and cannot be stopped or restarted. So earlier states of the animation must be remembered if they are to be compared to the current state – something that can easily overwhelm working memory (Lowe, 1999). It also is impossible to accurately count the numbers of healthy, infected, and recovered individuals as the balls rapidly change. Both of these issues are addressed by the inclusion of two extra representations placed above the animation – the first is an animated number count which provides the current state of the simulation and the second is a form of a dynamic area graph (although without labels or axis) which shows current and previous values. Thus, as a representational system these problems are somewhat ameliorated; although it would be difficult to pay attention to all three sources simultaneously and integrate them (Ainsworth, 2006). A further fundamental problem when with animations is that attention can be drawn to obvious changes, which may not be the most meaningful (Lowe, 1999). The authors of the animation in Figure 6 point out that as the simulation is random you will get different results when you look at the animation on subsequent visits, this however would not be at all easy to see given the working memory demands required. A beneficial design feature (not included in Figure 6 but in similar ones) would be to allow people to pause and restart the animation at will to allow more time to perceptually inspect and mentally interrogate what they are looking at (Bétrancourt, 2005).
3.5 Interactive Visualisations
Interactive visualisations respond to learners’ interactions as they manipulate and explore existing visualisations, perhaps providing feedback across forms as learners act on one representation and see a response on others when representations are dyna-linked (Ainsworth, 2006). Simulations allow learners to generate hypotheses and test the accuracy of their predictions with the provided representations (Rutten et al., 2012). When implemented within educational games, they can support playful yet serious learning (Clark et al., 2016). As embodied theorists argue, these actions with and on visualisations support learning when they are well aligned with key features of the domain to be learnt (Alibali and Nathan, 2018) and consequently we will review some of these possibilities below.
At the basic level, interactive visualisations can simply allow learners to manipulate visual-spatial aspects of a representation to gain a fuller understanding of the form. They are capable of displaying just-in-time information, allowing both overviews and zooming in and filtering (Ware, 2013). The interactive visualisation shown in Figure 7 allows the user to rotate the virion in any direction, which should enhance learners’ understanding of its 3d nature (e.g., Plass et al., 2009). Learners can zoom in to enable a more detailed focus on visible structures such spike, envelope and membrane proteins. It also has five interactive numbered spots that provide further textual explanation (e.g., lipid membrane) providing just-in-time information in an appropriate representation. This particular example does not allow zooming out to see the surrounding context and other virions or zooming in to see inside the envelope. CellPAINT (e.g., Gardner et al., 2018) on the other hand does so by using a cross-sectional approach (Figure 8). It allows users to create interactive illustrations using tools that look like those provided by typical digital painting programmes, but that are illustrated accurately, move appropriately and are consistent with available scientific data.
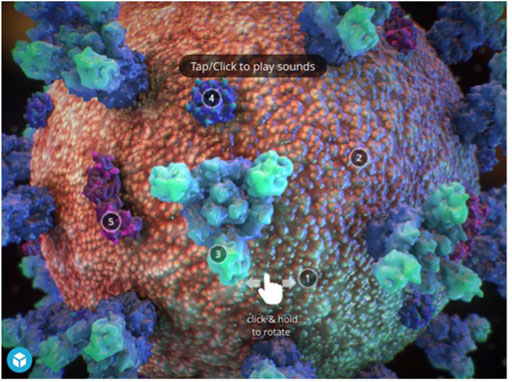
FIGURE 7. Interactive 3D model of Covid (Fusion Animation, 2020).
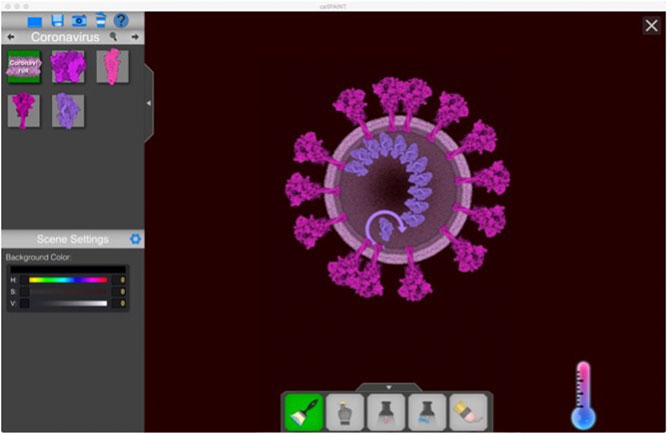
FIGURE 8. CellPAINT-coronavirus (CellPAINT-Coronavirus, 2021).
Computer-based simulations allow learners’ actions to have meaningful consequences for representations. For example, learners can interact with a simplified model by changing values for variables and see the consequences reflected in provided visualisations. Such visualisations typically include both abstract representations of the phenomena (such as equations or tables) but also concrete visual forms (Rutten et al., 2012). As such, they can be used to support the generation of hypotheses, data collection and then subsequent inspection of their effects to see if new hypotheses are needed. When well designed, such multi-representational simulations have been shown to enhance learning (e.g., Plass et al., 2009). They are particularly valuable in situations where it is impossible to observe phenomena in the real world because they do not operate at human time scales or size, or where it not safe or practical to experiment with them (de Jong and van Joolingen, 1998). Clearly, simulations of Covid-19 are therefore well suited to help young people learn in this difficult domain.
Some examples of virus simulations that we have found allow learners to manipulate biological variables such as replication or mutation rates or behavioural interventions such as length of lockdowns or degree of social distancing to explore consequences for infection. In Figure 9 for example, users can manipulate multiple parameters, from the length and intervention length and strategy, to explore the resulting changes for various outcomes such as the total infections over time. Comparable interactives, that we did not identify in our searches, might focus on the relationship between biological properties and various impacts, for example, manipulating virus mutations to explore the potential impact on vaccine efficiency or population spread.
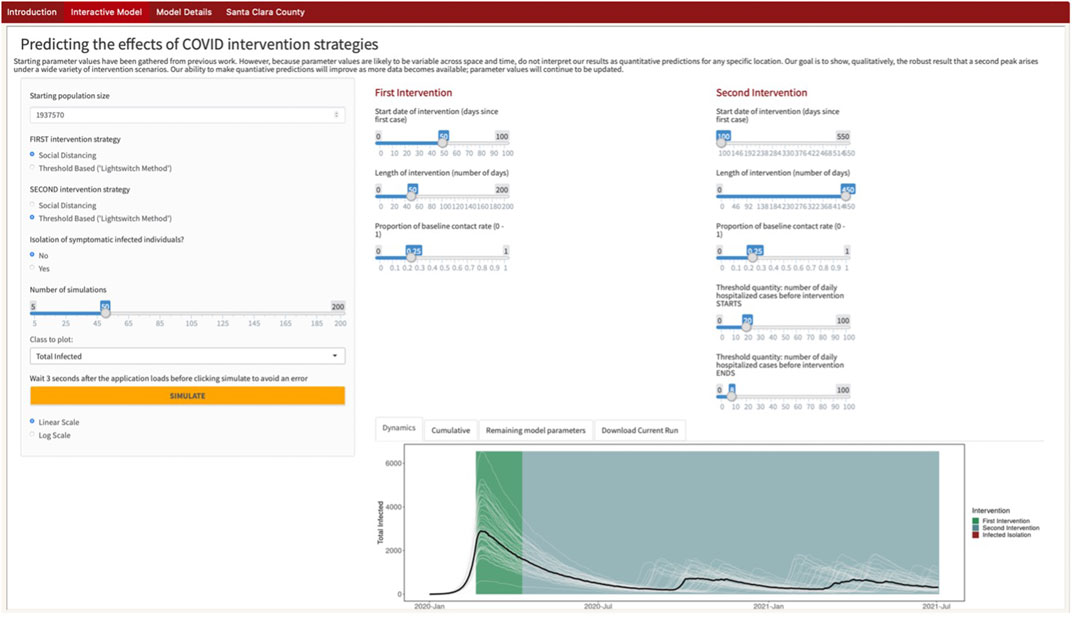
FIGURE 9. Interactive model for predicting effect of Covid-19 Intervention strategies (Childs et al., 2020).
These examples show that educational simulations must make a number of simplifying assumptions, which may not be apparent to learners unless teachers help scaffold their understanding (e.g., de Jong and van Joolingen, 1998; Rutten et al., 2012). Learners also tend to need support to understand the representations in the simulations (Ainsworth, 2006) and, in particular, how the representations are related (van der Meij and de Jong, 2006).
The final example of interactive visualisation we discuss in this section are educational games. Educational simulations and games share many features but perhaps the core difference is that people typically expect to enjoy playing games, at least some of the time (Malone, 1981). Games themselves come in many different genres, although there is not a commonly agreed approach to their classification (Clarke et al., 2017) and as such it is difficult to make generalised claims about how they support learning. However, there is compelling evidence that well designed games are effective (Clark, et al., 2016) and have much to offer learners through features such as allowing identity development, problem sequencing that supports a pleasantly frustrating experience, cycles of expertise and just-in-time instruction (Gee, 2003).
One example of this is Virulent (Anderson et al., 2018); a role-playing strategy game designed to help children learn about viral replication and immune system response. The players act as a fictional simplified virus that infects hosts cells, takes resources and engages in “battles” with the immune system. Players can trial strategies that allow their virus to avoid cell defences and central to the game design is the idea that these need not always be successful – that failures can teach. Gameplay is supported by a range of collaborative activities including researching viruses, constructing visual models and developing solutions which were presented to one another. Evaluations showed the benefits of this game for increasing understanding of virology and that ‘failures’ were effective in helping children learn.
Perhaps one of the biggest challenges with using games for learning about Covid-19 is how to balance the features designed to evoke an emotional response (the joy on winning, the frustration at losing) with their clearly emotionally-charged context. For example, in Can you Save the World users walk their character down the street, dodging passers-by whose contagion is represented by a green circle around them (Figure 10). Players gain points for collecting masks and lose them for passing too closely to someone infected. This example raises important questions about accuracy and the possibility of counter-productive messaging (e.g., the fun of going out to a busy street). One of its most sensitive aspects is the young children, for whom it is intended, may not have agency in their everyday lives to make decisions about distancing or mask wearing. They may also have experienced distressing consequences of the pandemic such as social isolation, economic impact or serious family health concerns. Consequently, the use of games such as these are perhaps best, as the authors suggest, as a stimulus to prompt facilitated discussion and should not be seen as educational tools in their own right.
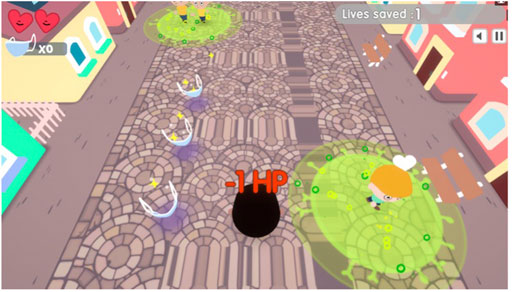
FIGURE 10. Interactive Covid-19 game (Wiseman and Martin, 2020).
3.6 Extended Reality
A common concern is how digital interaction, via a graphical user interface (i.e., screens), constrains some of the value of whole-body, real-world, physical interaction, which embodied cognitive accounts of learning argue are so important. However, this physical/virtual dichotomy is gradually being eroded through the emergence of new interactive representational systems. These include virtual reality that offers a greater level of immersivity by overlapping digital information/interactivity onto the physical world such as in augmented and mixed reality. Interfaces can augment physical objects with digital information (tangibles). People can interact with digital interfaces using their whole body not just their hands and can receive physical feedback from the interface (haptics). We will use extended reality (XR) as an umbrella term for all these technologies which offer more embodied experiences by bringing together physical and virtual worlds.
At the present time, the novelty of XR representations is likely to have general affective benefit for learners and we do not wish to dismiss the value of novel engaging experiences. It is not obvious that a hologram of a virus floating around the classroom presents cognitive benefits, but the experience is likely to be a memorable one and one that children are likely to talk about with friends and family. However, from an embodied learning perspective, there are very specific benefits that XR technology may offer when sensory-motor experiences are productively aligned with the desired features of learning (Abrahamson, et al., 2020; Lindgren and Johnson-Glenberg, 2013).
One advantage of augmented reality is that digital representations can be manipulated or overlaid on physical representation. For example, in Figure 11, the explainer uses a 3d model to represent the virus spike protein and his hand as the cell surface. During the clip, the digital image representing an (ACE 2) receptor is overlaid on his hand to help explain how proteins connect. It also shows the potential to bring together the complementary benefits of digital information and a real-world representation – in this case a detailed labelled digital representation mapped onto the explainer’s hand as they gesture dynamically. Gillet et al. (2005) describe how tangible user interfaces allow learners to interact with a physical model that simultaneously projects a 3d virtual representation (such as a direct representation of what they are manipulating or other labels or views). This combination allows the benefits of direct physical exploration but where the overlaid augmented reality means that multiple forms of representation can also be leveraged to support understanding (Lindgren and Johnson-Glenberg, 2013).
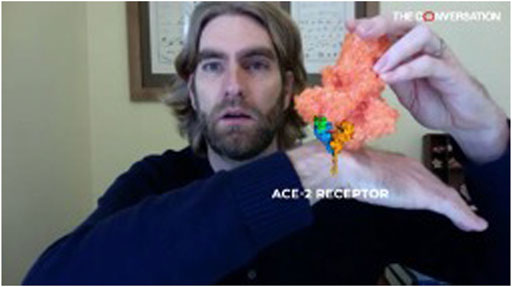
FIGURE 11. Augmented reality representation of Covid-19 (Ahlgren, 2021).
Extended reality also permits learners to engage in digital activities with their whole bodies and there are some examples of this being used successfully for virus education. Colella (2000) describes a participatory simulation where learners become viruses by wearing small computers (tags) that keep track of others they meet in their classroom. Tags become infected if other infected tags come close to them and they are not immune. Over a series of lessons, students (who did not know how infection occurred) began to investigate their own experiences, collaborating and becoming increasingly more systematic to investigate this simple model. Colella suggests that some of the success of this clearly engaging activity was that the students were collecting data about and experimenting on their own physical, embodied, experiences.
A further extended reality example includes the use of haptics (sensory feedback of virtual objects) to support children’s biological understanding. Whilst most frequently used in domains that require fine motor skills (e.g., surgery), it has also been used to help students understand biological phenomena such as proteins (Bivall et al., 2011) and, of particular relevance to this paper, viruses (e.g., Jones, et al., 2003; Jones et al., 2006). In these studies, high school students were able to use a microscope, desktop computer, and either high or low fidelity haptic devices (PHANToMs or joysticks) to learn virus characteristics such as shape, composition, size, and the nature of genetic material. Students could cut and poke a virus to discover its shape as part of a wider series of activities that includes animations about virus replication and microchemistry simulations. Researchers found that these activities, in general, enhanced students’ engagement and specifically understanding of virus morphology, diversity of forms, and the three-dimensional nature of virus. In line with Bivall et al. (2011), they also noted that students used more body-based metaphors as they talked about the phenomena (e.g., “roll like the paper tube,” Jones et al., 2006 p 119).
In our review of extended realities to support learning of coronavirus, and viruses more generally, we did encounter more examples described online. These included augmented reality to explore mRNA vaccine process (Figure 12A), virtual reality to enhance shared learning experiences (Figure 12B), and whole-body interactive exhibits (Figure 12C). Unfortunately, the limited description provided makes it difficult to evaluate the extent to which these examples move beyond a more superficial focus on gamifying learning experiences as opposed to offering unique representational benefits. They do however indicate the potential for emerging technologies to offer novel forms of immersive and embodied experiences for designers.
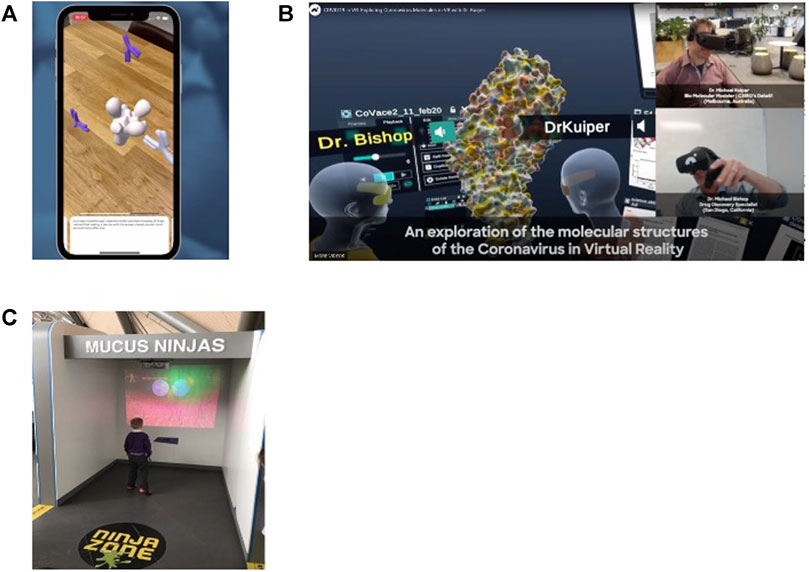
FIGURE 12. Extended Reality examples (A) Augmented reality for mRNA exploration (USA Today, 2021) (B) Virtual Reality for co-exploring of Covid-19 (Kulper, 2020) (C) Whole body interactive game at Glasgow Science Centre, United Kingdom.
4 Conclusion: Summary and Implications
It was not our intention when writing this paper to reduce the complexity of teaching about Covid-19 to be a solely representational issue. However, we maintain that to help children understand these invisible phenomena, representations that make explicit key aspects of Covid-19 so learners are able to engage in beneficial cognitive, social and affective actions are vital. In addition, there are practical issues that will influence choice and success of representations when used in classrooms. For example, whilst teachers might prefer the educational possibilities of interactive or extended forms, the realities of accessing suitable representations will often mitigate against their use. Hence, in Table 1 we summarise the advantages and disadvantages of the forms discussed above and include a final column addressing some of the practical considerations that are inherent when such representations are intended for use in the classroom. As stated earlier in the paper, whilst we draw attention to certain representational benefits and limitations, we refrain from advocating particular examples or forms on the basis that their value will ultimately depend upon a complex range of factors, not least the use alongside other representation forms.
What this summary Table 1 helps clarify is that it also almost inevitable that we will need to draw upon multiple representations of Covid-19 to address limitations and increase overall value for learners. For example, a picture showing virions and cells could be complemented by gesturing whilst a teacher explains the process of replication. Thus, the disadvantages of one representational form, in this case the difficulty of representing a 4d process (virus replication) in 2d can be compensated for by gesturing and explaining and, in turn, the lack of specific detail in the gesture is made concrete in the 2d picture.
4.1 Implications for Education
As research on learning with representations has matured, the question has turned from “is this representation effective?,” to addressing a wider set of issues coalescing around how to help different learners succeed when learning with representations. Research has shown how learners need support to learn the conventions of representations (e.g., Cromley et al., 2013), that they can lack metacognitive insight into how to learn with representations, and can be at risk of processing them only superficially (Salomon, 1984) or focus on the less useful aspects of a representation (Lowe, 1994). The activities that learners do with the representations and the feedback and scaffolding they receive is increasingly recognised as paramount (Tippett, 2016). When using multiple representations, we know it can be difficult for learners to see relationships between them (e.g., Ainsworth, 2006). This will be even more challenging if there are differences in design features (for example, the colour of proteins in a model may be different to those in an accompanying picture). In general, this is best avoided but where this is not possible, this should be addressed directly and could even, where appropriate, provide an opportunity to discuss what are inherent features of the virus and what are simply representational choices. Keeping these considerations in mind, we propose a representational checklist in Table 2 that teachers and other adults can use, in conjunction with Table 1, to support children’s learning about Covid-19.
4.2 Conclusion
Identifying what is important to teach and how to teach it is a complex and evolving challenge. The argument made in this paper is that the impact of Covid-19 on children’s lives warrants greater efforts to support children’s understanding of the biology of virus and to achieve this, we need to understand and improve educational resources for teachers. The limited focus of this paper has been to extend our knowledge of the relative benefits and limitations of different forms of representations for learning about the underlying biology of Covid-19. More specifically we have focussed on representations of morphology and viral replication rather than the wider biological and social aspects of virus education which also requires further exploration. We have rarely been able to draw upon research that has directly addressed these issues. We have instead conducted an analytic review using a synthesis of approaches that have previously considered how representations influence learning brought together using an embodied lens. Therefore, different researchers and theoretical frameworks may well highlight other issues. We also have not attempted to analyse all representations of Covid-19 that could be found, as given their continuing growth, particularly in non-peer reviewed media, such a goal would be impossible. We also acknowledge that our evidence concerning children’s understanding of viruses is likely to be changing rapidly. However, until new studies can be conducted, we hope that our approach can be useful for articulating likely sources of misunderstandings and difficulties that representation can address.
In this paper, we have used this to propose practical suggestions for teachers. Some implications may be challenging for teachers to address given the immediacy of Covid-19; yet the principles extend to the use of representations for teaching other biological concepts and indeed learning more generally. Furthermore, where suggestions are difficult for teachers to address (e.g., finding the best interactive representations), they may inform those who help curate and design resources for teachers.
Whilst the paper contributes to wider goals of understanding the relative merits of different representations (and the potential of technology), the more immediate goal is to contribute timely and accessible knowledge that will help children understand the current Covid-19 pandemic as well as potential future ones. Importantly, this knowledge might help children disassociate the term virus from the health impact of Covid-19, to be aware that viruses have always been around and within us (and hence not to be scared), and that some viruses can even benefit us. Future work may help identify whether increased biological knowledge can also encourage children’s health behaviours (where they have agency).
Amidst the tragic impact of Covid-19, one encouraging outcome can be found in a report by the organisation Kids Insights (2020) which indicates that the pandemic may have positively impacted young people’s interests in careers that involve deeper knowledge of viruses (such as doctors, or scientists). A further positive outcome would be to improve the way we improve the design and use of resources, such as representations, to improve all children and young people’s biological understanding.
Data Availability Statement
The original contributions presented in the study are included in the article/supplementary material, further inquiries can be directed to the corresponding author.
Author Contributions
All authors listed have made a substantial, direct, and intellectual contribution to the work and approved it for publication.
Funding
This research was funded in whole, or in part, by the Wellcome Trust (206205/Z/17/Z). For the purpose of open access, the author has applied a CC BY public copyright licence to any Author Accepted Manuscript version arising from this submission.
Conflict of Interest
The authors declare that the research was conducted in the absence of any commercial or financial relationships that could be construed as a potential conflict of interest.
Publisher’s Note
All claims expressed in this article are solely those of the authors and do not necessarily represent those of their affiliated organizations, or those of the publisher, the editors, and the reviewers. Any product that may be evaluated in this article, or claim that may be made by its manufacturer, is not guaranteed or endorsed by the publisher.
References
Abrahamson, D., Nathan, M. J., Williams-Pierce, C., Walkington, C., Ottmar, E. R., Soto, H., et al. (2020). The Future of Embodied Design for Mathematics Teaching and Learning. Front. Educ. 5, 147. doi:10.3389/feduc.2020.00147
Ahlgren, N. (2021). The Body’s Fight against COVID-19 Explained Using 3D-Printed Model. Retrieved from: https://theconversation.com/the-bodys-fight-against-covid-19-explained-using-3d-printed-models-153766 (Accessed April 15, 2021).
Ainsworth, S., Prain, V., and Tytler, R. (2011). Science Education. Drawing to Learn in Science. Science 333 (6046), 1096–1097. doi:10.1126/science.1204153
Ainsworth, S. (2006). Deft: A Conceptual Framework for Considering Learning with Multiple Representations. Learn. Instr. 16 (3), 183–198. doi:10.1016/j.learninstruc.2006.03.001
Alibali, M. W., and Nathan, M. J. (2018). “Embodied Cognition in Learning and Teaching,” in International Handbook of the Learning Sciences. Editors F. Fischer, C. Hmelo-Silver, S. Goldman, and P. Reimann (New York: Routledge), 75–85. doi:10.4324/9781315617572-8
Anderson, C. G., Dalsen, J., Kumar, V., Berland, M., and Steinkuehler, C. (2018). Failing up: How Failure in a Game Environment Promotes Learning through Discourse. Thinking Skills Creativity 30, 135–144. doi:10.1016/j.tsc.2018.03.002
Barke, H.-D., Hazari, A., and Yitbarek, S. (2009). “Students’ Misconceptions and How to Overcome Them,” in Misconceptions in Chemistry. Editors H.-D. Barke, A. Hazari, and S. Yitbarek (Berlin: Springer), 21–36.
Barsalou, L. W. (2008). Grounded Cognition. Annu. Rev. Psychol. 59, 617–645. doi:10.1146/annurev.psych.59.103006.093639
Bétrancourt, M., and Tversky, B. (2000). Effect of Computer Animation on Users’ Performance: A Review. Le Trav. Hum. 63 (4), 311–330.
Bétrancourt, M. (2005). “The Animation and Interactivity Principles in Multimedia Learning,” in Cambridge Handbook of Multimedia Learning. Editor R. E. Mayer (New York: Cambridge University Press), 287–296. doi:10.1017/cbo9780511816819.019
Bibace, R., and Walsh, M. E. (1981). Children's Conceptions of Illness. New Dir. Child Adolesc. Dev. 1981 (14), 31–48. doi:10.1002/cd.23219811404
Bivall, P., Ainsworth, S., and Tibell, L. A. E. (2011). Do haptic Representations Help Complex Molecular Learning? Sci. Ed. 95 (4), 700–719. doi:10.1002/sce.20439
Black, J. B. (2010). “An Embodied/grounded Cognition Perspective on Educational Technology,” in New Science of Learning. Editors M. S. Kine, and K. M. Saleh (New York, NY: Springer), 45–52. doi:10.1007/978-1-4419-5716-0_3
Byrne, J., Grace, M., and Hanley, P. (2009). Children's Anthropomorphic and Anthropocentric Ideas about Micro-organisms. J. Biol. Educ. 44 (1), 37–43. doi:10.1080/00219266.2009.9656190
Byrne, J. (2011). Models of Micro‐Organisms: Children's Knowledge and Understanding of Micro‐organisms from 7 to 14 Years Old. Int. J. Sci. Educ. 33 (14), 1927–1961. doi:10.1080/09500693.2010.536999
CellPAINT-Coronavirus (2021). Center for Structural Biology (Center for Structural Biology). Available from: https://ccsb.scripps.edu/cellpaint/cellpaint-coronavirus/(Retrieved May 5, 2021).
Childs, M., Kain, M., Kirk, D., Harris, M., Ritchie, J., Couper, L., et al. (2020). Predicting the Effects of COVID Intervention Strategies. Retrieved from:Available at: http://covid-measures.stanford.edu (Accessed April 2, 2021.
Clark, D. B., Tanner-Smith, E. E., and Killingsworth, S. S. (2016). Digital Games, Design, and Learning: A Systematic Review and Meta-Analysis. Rev. Educ. Res. 86 (1), 79–122. doi:10.3102/0034654315582065
Clarke, R. I., Lee, J. H., and Clark, N. (2017). Why Video Game Genres Fail: A Classificatory Analysis. Games Cult. 12 (5), 445–465. doi:10.1177/1555412015591900
Colella, V. (2000). Participatory Simulations: Building Collaborative Understanding through Immersive Dynamic Modeling. J. Learn. Sci. 9 (4), 471–500. doi:10.1207/s15327809jls0904_4
Cromley, J. G., Bergey, B. W., Fitzhugh, S., Newcombe, N., Wills, T. W., Shipley, T. F., et al. (2013). Effects of Three Diagram Instruction Methods on Transfer of Diagram Comprehension Skills: The Critical Role of Inference while Learning. Learn. Instr. 26, 45–58. doi:10.1016/j.learninstruc.2013.01.003
de Jong, T., and van Joolingen, W. R. (1998). Scientific Discovery Learning with Computer Simulations of Conceptual Domains. Rev. Educ. Res. 68 (2), 179–201. doi:10.3102/00346543068002179
DrMichelleDickinson (2020). Coronavirus Explained! (For Kids). Retrieved from: https://www.youtube.com/watch?v=OPsY-jLqaXM (Accessed May 23, 2021).
DrRanjOnline (2020). Dr. Ranj's Coronavirus Advice for Kids. Retrieved from: https://www.youtube.com/watch?v=oMHacLHchI0 (Accessed May 14, 2021).
Eckert, A., and Higgins, D. (2020). Ultrastructural Morphology Exhibited by Coronaviruses. ID#23311 [Online Image]. Centre for Disease Control and Prevention. Available at: https://phil.cdc.gov/Details.aspx?pid=23311 (Retrieved May 2, 2021).
Firebox (2021). Covid-19 plush [Online Image]. Available from: https://firebox.com/products/covid-19-plush (Retrieved May 5, 2021).
Fusion Animation (2020). Interactive 3D Model of SARS-CoV-2 Surface Released. Retrieved from https://www.drugtargetreview.com/news/72344/interactive-3d-model-of-sars-cov-2-surface-released/.
Gardner, A., Autin, L., Barbaro, B., Olson, A. J., and Goodsell, D. S. (2018). Cellpaint: Interactive Illustration of Dynamic Mesoscale Cellular Environments. IEEE Comput. Graph Appl. 38 (6), 51–66. doi:10.1109/MCG.2018.2877076
Gaulkin, T. (2020). Kids Are Drawing Pictures of the New Coronavirus. That’s a good thing [Online Image]. Retrieved from: https://thebulletin.org/2020/03/kids-are-drawing-pictures-of-the-new-coronavirus-thats-a-good-thing/ (Accessed May 4, 2021).
Gee, J. P. (2003). What Video Games Have to Teach Us about Learning and Literacy. New York: Palgrave Macmillan.
Gharib, M., and Turner, C. (2020). A Comic Just for Kids: How to Stay Safe from the Coronavirus [Online Image]. NPR. Retrieved from: https://www.npr.org/sections/goatsandsoda/2020/11/16/934679210/just-for-kids-how-to-stay-safe-from-the-coronavirus (Accessed May 14, 2021).
Gillet, A., Sanner, M., Stoffler, D., and Olson, A. (2005). Tangible Interfaces for Structural Molecular Biology. Structure 13 (3), 483–491. doi:10.1016/j.str.2005.01.009
Glenberg, A. M. (2010). Embodiment as a Unifying Perspective for Psychology. Wiley Interdiscip. Rev. Cogn. Sci. 1 (4), 586–596. doi:10.1002/wcs.55
Goldin-Meadow, S. (1997). When Gestures and Words Speak Differently. Curr. Dir. Psychol. Sci. 6 (5), 138–143. doi:10.1111/1467-8721.ep10772905
Goldin-Meadow, S. (2011). Learning Through Gesture. Wiley Interdiscip. Rev. Cogn. Sci. 2 (6), 595–607. doi:10.1002/wcs.132
Goldsmith, C., and Tamin, A. (2020). Electron Microscopic Image of a Negatively Stained Particle of SARS-CoV-2ID#23640 [Online Image]. Centre for Disease Control and Prevention. Available at: https://phil.cdc.gov/Details.aspx?pid=23640 (Retrieved May 2, 2021).
Halverson, E. R., and Sheridan, K. (2014). The Maker Movement in Education. Harv. Educ. Rev. 84 (4), 495–504. doi:10.17763/haer.84.4.34j1g68140382063
Hatano, G., and Inagaki, K. (1994). Young Children's Naive Theory of Biology. Cognition 50 (1-3), 171–188. doi:10.1016/0010-0277(94)90027-2
Hostetter, A. B., and Alibali, M. W. (2008). Visible Embodiment: Gestures as Simulated Action. Psychon. Bull. Rev. 15 (3), 495–514. doi:10.3758/pbr.15.3.495
Idoiaga, N., Berasategi, N., Eiguren, A., and Picaza, M. (2020). Exploring Children's Social and Emotional Representations of the Covid-19 Pandemic. Front. Psychol. 11, 1952. doi:10.3389/fpsyg.2020.01952
Jansen, Y., Dragicevic, P., Isenberg, P., Alexander, J., Karnik, A., Kildal, J., et al. (2015). “Opportunities and Challenges for Data Physicalization,” in Paper presented at the Proceedings of the 33rd Annual ACM Conference on Human Factors in Computing Systems.
Jones, M. G., and Rua, M. J. (2006). Conceptions of Germs: Expert to Novice Understandings of Microorganisms. Electron. J. Sci. Educ. 101—40.
Jones, M. G., Andre, T., Superfine, R., and Taylor, R. (2003). Learning at the Nanoscale: The Impact of Students' Use of Remote Microscopy on Concepts of Viruses, Scale, and Microscopy. J. Res. Sci. Teach. 40 (3), 303–322. doi:10.1002/tea.10078
Jones, M. G., Minogue, J., Tretter, T. R., Negishi, A., and Taylor, R. (2006). Haptic Augmentation of Science Instruction: Does Touch Matter? Sci. Ed. 90, 111–123. doi:10.1002/sce.20086
Kamde, K., Kasliwal, R., Gholse, Y., Gajbhiye, P., Bisane, K., and Chaple, D. (2020). Scoping Review: Life Cycle, Diagnosis and Current Treatment Strategies of Coronavirus Disease (COVID-19) Outbreak. Int. J. Pharm. Sci. Rev. Res. 63 (1), 67–72. Retrieved from https://globalresearchonline.net/journalcontents/v63-1/11.pdf.
Kim, M., Roth, W.-M., and Thom, J. (2011). Children's Gestures and the Embodied Knowledge of Geometry. Int. J. Sci. Math. Educ. 9 (1), 207–238. doi:10.1007/s10763-010-9240-5
Kontra, C., Goldin-Meadow, S., and Beilock, S. L. (2012). Embodied Learning across the Life Span. Top. Cogn. Sci. 4 (4), 731–739. doi:10.1111/j.1756-8765.2012.01221.x
Kress, G., and Van Leeuwen, T. (2016). Colour as a Semiotic Mode: Notes for a Grammar of Colour. Vis. Commun. 1 (3), 343–368. doi:10.1177/147035720200100306
Kulper, M. (2020). The Coronavirus and Virtual Reality. Retrieved from: https://www.digitalbodies.net/virtual-reality/the-coronavirus-and-virtual-reality/ (Accessed May 3, 2021).
Lindgren, R., and Johnson-Glenberg, M. (2013). Emboldened by Embodiment. Educ. Res. 42 (8), 445–452. doi:10.3102/0013189x13511661
Lowe, R. K. (1999). Extracting Information from an Animation during Complex Visual Learning. Eur. J. Psychol. Educ. 14 (2), 225–244. doi:10.1007/BF03172967
Lowe, R. K. (1994). Selectivity in Diagrams: Reading beyond the Lines.. Educ. Psychol. 14 (14), 467–491. doi:10.1080/0144341940140408
Malone, T. (1981). “What Makes Computer Games Fun,” in Paper presented at the Proceedings of the Joint Conference on Easier and More Productive Use of Computer Systems. (Part-II): Human Interface and the User Interface-Volume 1981.
Manches, A., and O’Malley, C. (2011). Tangibles for Learning: A Representational Analysis of Physical Manipulation. Pers Ubiquit Comput. 16 (4), 405–419. doi:10.1007/s00779-011-0406-0
Mayer, R. E., and Sims, V. K. (1994). For Whom Is a Picture worth a Thousand Words? Extensions of a Dual-Coding Theory of Multimedia Learning. J. Educ. Psychol. 86 (3), 389–401. doi:10.1037/0022-0663.86.3.389
McNeil, N., and Jarvin, L. (2007). When Theories Don't Add up: Disentangling He Manipulatives Debate. Theor. Into Pract. 46 (4), 309–316. doi:10.1080/00405840701593899
McNeill, D. (1992). Hand and Mind: What Gestures Reveal about Thought. Chicago: University of Chicago press.
Moyer, P. S. (2001). Are We Having Fun yet? How Teachers Use Manipulatives to Teach Mathematics. Educ. Stud. Math. 47 (2), 175–197. doi:10.1023/a:1014596316942
Myant, K. A., and Williams, J. M. (2005). Children's Concepts of Health and Illness: Understanding of Contagious Illnesses, Non-contagious Illnesses and Injuries. J. Health Psychol. 10 (6), 805–819. doi:10.1177/1359105305057315
NIAID (2020). Novel Coronavirus SARS-CoV-2 Spike Protein. Retrieved from: https://www.flickr.com/photos/niaid/49584124196/ (Accessed May 3, 2021).
Novack, M. A., and Goldin-Meadow, S. (2017). Gesture as Representational Action: A Paper about Function. Psychon. Bull. Rev. 24 (3), 652–665. doi:10.3758/s13423-016-1145-z
Pande, P. (2020). Learning and Expertise with Scientific External Representations: An Embodied and Extended Cognition Model. Phenom Cogn. Sci. 20, 463–482. doi:10.1007/s11097-020-09686-y
Parrill, F., and Sweetser, E. (2005). What We Mean by Meaning: Conceptual Integration in Gesture Analysis And Transcription. Gest 4 (2), 197–219. doi:10.1075/gest.4.2.05par
Piko, B. F., and Bak, J. (2006). Children's Perceptions of Health and Illness: Images and Lay Concepts in Preadolescence. Health Educ. Res. 21 (5), 643–653. doi:10.1093/her/cyl034
Plass, J. L., Homer, B. D., and Hayward, E. O. (2009). Design Factors for Educationally Effective Animations and Simulations. J. Comput. High Educ. 21 (1), 31–61. doi:10.1007/s12528-009-9011-x
Pouw, W. T. J. L., van Gog, T., and Paas, F. (2014). An Embedded and Embodied Cognition Review of Instructional Manipulatives. Educ. Psychol. Rev. 26 (1), 51–72. doi:10.1007/s10648-014-9255-5
Raman, L., and Winer, G. A. (2004). Evidence of More Immanent justice Responding in Adults Than Children: A challenge to Traditional Developmental Theories. Br. J. Dev. Psychol. 22 (2), 255–274. doi:10.1348/026151004323044609
Roschelle, J., and Teasley, S. D. (1995). “The Construction of Shared Knowledge in Collaborative Problem Solving,” in Computer Supported Collaborative Learning. Editor C. O’Malley (Berlin: Springer), 69–97. doi:10.1007/978-3-642-85098-1_5
Rutten, N., van Joolingen, W. R., and van der Veen, J. T. (2012). The Learning Effects of Computer Simulations in Science Education. Comput. Educ. 58 (1), 136–153. doi:10.1016/j.compedu.2011.07.017
Salomon, G. (1984). Television Is "Easy" and Print Is "Tough": The Differential Investment of Mental Effort in Learning as a Function of Perceptions and Attributions. J. Educ. Psychol. 76 (4), 647–658. doi:10.1037/0022-0663.76.4.647
Scheiter, K., Brucker, B., and Ainsworth, S. (2020). "Now Move like that Fish": Can Enactment Help Learners Come to Understand Dynamic Motion Presented in Photographs and Videos? Comput. Educ. 155, 103934. doi:10.1016/j.compedu.2020.103934
Schnotz, W., and Rasch, T. (2005). Enabling, Facilitating, and Inhibiting Effects of Animations in Multimedia Learning: Why Reduction of Cognitive Load Can Have Negative Results on Learning. ETR&D 53 (3), 47–58. doi:10.1007/BF02504797
Scientific Animations (2020). Coronavirus Outbreak (Covid 19) Explained through 3D Medical Animation. Retrieved from: https://www.youtube.com/watch?v=I-Yd-_XIWJg&list=PLRuLO8d3L-MA5ieaZnVbGs6XIGzTlgiOX (Accessed May 4, 2021).
Siegal, M. (1988). Children's Knowledge of Contagion and Contamination as Causes of Illness. Child. Dev. 59 (5), 1353–1359. doi:10.2307/1130497
Simon, U. K., Enzinger, S. M., and Fink, A. (2017). "The Evil Virus Cell": Students' Knowledge and Beliefs about Viruses. PLoS One 12 (3), e0174402. doi:10.1371/journal.pone.0174402
Simonneaux, L. (2000). A Study of Pupils' Conceptions and Reasoning in Connection with 'microbes', as a Contribution to Research in Biotechnology Education. Int. J. Sci. Educ. 22 (6), 619–644. doi:10.1080/095006900289705
Slutz, S. (2020). Answering Kids' COVID-19 Questions: Doing Fun Science at Home during School Closures. Retrieved from: https://www.sciencebuddies.org/blog/doing-fun-science-at-home-activity12-kids-coronavirus-questions-answered (Accessed April 2, 2021).
Stenning, K., and Oberlander, J. (1995). A Cognitive Theory of Graphical and Linguistic Reasoning: Logic and Implementation. Cogn. Sci. 19 (1), 97–140. doi:10.1207/s15516709cog1901_3
Stevens, H. (2020). Why Outbreaks like Coronavirus Spread Exponentially, and How to “Flatten the Curve”. Washington Post. Retrieved from: https://www.washingtonpost.com/graphics/2020/world/corona-simulator/ (Accessed May 23, 2021).
Suthers, D. D. (2014). “Empirical Studies of the Value of Conceptually Explicit Notations in Collaborative Learning,” in Knowledge Cartography: Software Tools and Mapping Techniques. Editors A. Okada, S. J. Buckingham Shum, and T. Sherborne (London: Springer London), 1–22. doi:10.1007/978-1-4471-6470-8_1
Tino, R., Moore, R., Antoline, S., Ravi, P., Wake, N., Ionita, C. N., et al. (2020). COVID-19 and the Role of 3D Printing in Medicine. 3d Print Med. 6, 11. doi:10.1186/s41205-020-00064-7
Tippett, C. D. (2016). What Recent Research on Diagrams Suggests about Learningwithrather Than Learningfromvisual Representations in Science. Int. J. Sci. Educ. 38 (5), 725–746. doi:10.1080/09500693.2016.1158435
Tretter, T. R., Jones, M. G., and Minogue, J. (2006). Accuracy of Scale Conceptions in Science: Mental Maneuverings across many Orders of Spatial Magnitude. J. Res. Sci. Teach. 43 (10), 1061–1085. doi:10.1002/tea.20155
Tversky, B. (2011). Visualizing Thought. Top. Cogn. Sci. 3 (3), 499–535. doi:10.1111/j.1756-8765.2010.01113.x
USA Today (2021). Explore How COVID-19 Vaccine Works: An Augmented Reality Journey into the Body. Available from https://eu.usatoday.com/story/augmented-reality/2021/01/27/interactivestory-experienceid-mrna/6701446002/(Retrieved May 5th, 2021).
van der Meij, J., and de Jong, T. (2006). Supporting Students' Learning with Multiple Representations in a Dynamic Simulation-Based Learning Environment. Learn. Instr. 16 (3), 199–212. doi:10.1016/j.learninstruc.2006.03.007
ViolinMD (2020). Doctor Explains Covid-19 (Coronavirus). Retrieved from: https://www.youtube.com/watch?v=3Uugj-zxA5E&t=90s (Accessed May 3, 2021).
WebMD (2021). Stylised and Decorative Illustration of Covid-19 Virions. [Untitled image of Covid-19] [Online Image]. Retrieved from: https://www.webmd.com/lung/coronavirus.
Williams, J. M., and Binnie, L. M. (2002). Children's Concepts of Illness: An Intervention to Improve Knowledge. Br. J. Health Psychol. 7 (Pt 2), 129–147. doi:10.1348/135910702169402
Wilson, M. (2002). Six Views of Embodied Cognition. Psychon. Bull. Rev. 9 (4), 625–636. doi:10.3758/bf03196322
Wiseman, R., and Martin, J. (2020). Can You Save the World? Available from: https://martin-jacob.itch.io/can-you-save-the-world (Retrieved May 5th, 2021).
Keywords: COVID-19, representation, review, education, teachers, embodied learning
Citation: Manches A and Ainsworth S (2022) Learning About Viruses: Representing Covid-19. Front. Educ. 6:736744. doi: 10.3389/feduc.2021.736744
Received: 05 July 2021; Accepted: 24 November 2021;
Published: 03 January 2022.
Edited by:
Dor Abrahamson, University of California, Berkeley, United StatesReviewed by:
Kim Nichols, The University of Queensland, AustraliaAditi Wagh, Massachusetts Institute of Technology, United States
Copyright © 2022 Manches and Ainsworth. This is an open-access article distributed under the terms of the Creative Commons Attribution License (CC BY). The use, distribution or reproduction in other forums is permitted, provided the original author(s) and the copyright owner(s) are credited and that the original publication in this journal is cited, in accordance with accepted academic practice. No use, distribution or reproduction is permitted which does not comply with these terms.
*Correspondence: Andrew Manches, YS5tYW5jaGVzQGVkLmFjLnVr
†These authors have contributed equally to this work