- 1Graduate Institute of Science Education and Environmental Education, National Kaohsiung Normal University, Kaohsiung, Taiwan
- 2Collections and Research Division, National Science and Technology Museum, Kaohsiung, Taiwan
Up until now, there have been several different viewpoints on creativity in general and creativity in the science field in particular. Furthermore, STEAM (science, technology, engineering, arts, and mathematics) education is increasingly successful and widespread around the world; however, few studies on its impact on scientific creativity exist. As a result, research on the influence of STEAM-based curriculum on students’ scientific creativity is critical. Elementary school students were chosen to be investigated in this research, and the main topic of the STEAM-based curriculum was about a house-shaped money-saving tube with the concept of lock science, which was developed and created by the authors’ team. This research produced two phases of courses: Lock Science Courses (2 weeks) and STEAM-based courses (2 weeks). In this study, sixty-six elementary students from two separate courses were divided into two groups: control and experimental. This research used a counterbalanced design. The control group took Lock Science Courses first and then STEAM-based courses, while the experimental group did the opposite. As a pretest and posttest, students in both groups were asked to complete the “scientific creativity test” (Cronbach’s α, 0.87). The findings of the paired t-test study indicate that both the control and experimental groups have shown significant improvement in their scientific creativity. However, only the fluency and flexibility components of scientific creativity (consisting of fluency, flexibility, and originality) showed considerable development, whereas the originality component remained unchanged. This research also found that after engaging in a STEAM-based curriculum, there was no substantial difference in scientific creativity between males and females. Further discussion is provided.
Introduction
According to the Organization for Economic Cooperation and Development (OECD, 2019a) study on the Future of Education and Skills 2030 initiative, creativity is one of the most significant and necessary factors in generating new value and seeking solutions to difficult challenges and it is also becoming an increasingly important aspect to ensure sustainable development (Said-Metwaly et al., 2018). Furthermore, according to Meador (2003), someone who has learned to think creatively while working with scientific tasks is able to apply these skills in other ways.
Although there are several approaches to creativity, there are mainly four elements of creativity as observed in most of the prior research. They are action, production, disequilibrium, and sensitivity to a problem. In summary, creativity can be defined as a specific human capacity, an act that results from a perception of the environment that admits a certain unbalance, which results in productive activity that challenges patterned processes and norms of thought, then explores and creates something new in the form of a physical object or even an emotional structure, and solves real-world problems in a creative manner (Guilford, 1950; Huang et al., 2017; Walia, 2019). Most educators believe that creativity can be achieved through learning (Ford & Harris, 1992; Hoffman et al., 2021). Therefore, numerous scholars and educators around the world believe that creativity is one of the goals of education and that it is vital for the future (Shi et al., 2017; Suyidno et al., 2019).
PISA 2021, in keeping with this perspective, centered on the topic of creative thinking in schools (OECD, 2019b). In formal education, however, there are less standardized creativity-training courses and Newton and Newton (2010) found that teachers’ conceptions of scientific creativity in elementary schools are either narrow or inappropriate. Thus, the aim of this research was to develop a systematic curriculum for elementary school students in order to increase their creativity.
As previously mentioned, the definition of creativity has a range of meanings based on the area of study, and it is presented with domain-specific interpretations. Despite the fact that cognitive structure of creativity is identical, the essence of domain-specific creativity differs significantly (Csikszentmihalyi, 1996). Hence, the diverse points of view will influence the conversation about creativity. In this research, creativity in the scientific field, also known as scientific creativity, is concerned.
Scientific creativity is a subset or form of scientific giftedness, which is a type of domain-specific creativity in which humans combine their science context expertise with domain-relevant creativity to achieve scientific vision (Amabile, 1996; Sternberg and Lubart, 1993; Hu and Adey, 2002; Ayas and Sak, 2014; Author, 2019). More specifically, according to Ayas and Sak (2014), scientific creativity is considered as a result of the convergence of several cognitive and noncognitive variables such as intelligence, skills associated with creation, scientific abilities, characteristics and motivations of personality, interest, concentration, and the search for knowledge and chance permutation of mental elements. It may be seen as a problem-solving process that involves three different stages. These stages include the interaction of hypothesis generation, the design and conduct of experiments, and the assessment of evidence. One of the most important characteristics of scientific creativity is the ability to generate a large number of hypotheses for a particular issue or circumstance. One crucial criterion for science creativity, according to Kind and Kind (2007), is that it should be focused on what “real” scientists do. In order to address scientific and environmental issues that are becoming global problems and threats (Dunlap and Jorgenson, 2012), not just scientists but also humans must use their scientific creativity. As a result, this study confirms the importance of focusing the definition of creativity on scientific creativity to a greater extent.
Many previous studies have found that people with high creativity skills have a deep sense of interest and a strong correlation between their knowledge and experiences in order to generate new ideas (Lubart, 1994; Feldhusen and Goh, 1995; Thuneberg et al., 2018; Conradty and Bogner, 2019). To put it another way, the interdisciplinary thinking skill or integrated learning will be a critical element in honing human creativity and making knowledge more holistic, long-lasting, and versatile (Newton, 2000). STEAM (science, technology, engineering, art, and mathematics) subjects, according to Conradty and Bogner (2019), are a form of interdisciplinary education strategy. Ngo and Phan (2019) also note that the multidisciplinary approach in the project-based learning strategy fits into the STEAM concept. According to some studies, students’ scientific creativity improved significantly after taking STEAM courses (Kim et al., 2014; Genek and Doğança Küçük, 2020; Ozkan and Topsakal, 2021). However, Perignat and Katz-Buonincontro (2019) claimed in an integrative literature review that research is required to consider the effects of STEAM in practice in order for STEAM education to develop as an efficient pedagogy; several scholars posit that the STEAM concept is a model for enhancing creativity, but there is not much evidence to support this notion.
As a result, the aim of this research was to determine a STEAM-based curriculum design that is best suitable for helping elementary school students develop their scientific creativity. Using project-based learning methods, this study created a two-stage STEAM-based curriculum. The key focus of this STEAM-based curriculum is finishing a project—assembling a house-shaped money-saving tube and investigating the causes for varying outcomes under various conditions. “Lock Science Courses” and “STEAM-based courses” are the two stages of this curriculum. The next section will go into the basics of program design (Methods section).
To summarize, this study elicits two key research questions:
i) Which kind of sequence of the course stage design has the remarkable impact on improving scientific creativity of elementary school students, as measured by the constituent scores of scientific creativity (fluency, flexibility, and originality)?
ii) Is there a discrepancy in the effects of STEAM-based curriculum on various genders after they participated in the study?
This study has a few scientific limitations. All participants in this research will write down their responses to the scientific creativity test at the same time in class. However, if the number of responses is low, this study would be unable to determine whether this is due to the students’ lack of commitment to complete the exam. Simply put, this study will count all data in the research article that includes terms. Besides, the findings of the paired t-test study indicate that both the control and experimental groups have shown improvement in their scientific creativity significantly.
Materials and Methods
Participants
This research was carried out in an urban elementary school in Taiwan. Sixty-six elementary school students from two elementary schools in the south of Taiwan voluntarily took part in the study, enrolled in the course, and participated in the survey. They were randomly divided into experimental and control groups. The participants’ details are described in Table 1. Photos of students participating in the experiment are shown in (Figure 1). Before and after the whole course, all participants were asked to complete the scientific creativity test (Hu and Adey, 2002). The two groups of students, on the contrary, went through different stage designs. The next segment would go into the aspects of the curriculum design.
Research Design and STEAM-Based Curriculum Design
The aim of this research was to see how the STEAM-based curriculum affects scientific creativity of elementary school students. The key subject of the STEAM-based curriculum is a house-shaped money-saving tube that was designed and produced by the authors’ team. In addition to serving the same role as other money-saving tubes in helping to keep coins, the house-shaped money-saving tube is also a useful tool for automatically sorting coins of various denominations into different internal storage compartments when deposited. Students must learn about the lock science, technology, engineering, and mathematics principles in order to comprehend the complexity of this. Furthermore, this study included STEAM-based courses to help students grasp the overall principles of the house-shaped money-saving tube. Both groups of students must mount, install, and paint their own house-shaped money-saving tubes and use their own tubes to investigate various outcomes from various condition settings. Hence, this research produced a STEAM-based curriculum of two stages and four steps (Figure 2). The curriculum design was reviewed and validated by three experts (male = 2, female = 1; all experts majored in science education).
Despite the fact that all students went through these two stages of the program, the aim of this research was to establish “the influences of the STEAM-based curriculum on students’ scientific creativity” as well as “which kind of sequence of the course stage design is more successful to boost students’ scientific creativity.” This study used a counterbalance design to determine the potential outcomes of key research questions. Students in the control group were required to participate in stage 1 (Lock Science Courses) before going on to stage 2 (STEAM-based courses). This type of curriculum design aids students in constructing science principles first and then guiding them to incorporate these concepts through participation in STEAM courses. Students in the experimental group, on the contrary, were required to join stage 2 first and then stage 1 (Figure 3). This style of curriculum design assists students in self-learning of interdisciplinary expertise in STEAM courses and then leads them in generalizing their science concepts.
The control group style (stage 1 to stage 2) involves building students’ science principles first and then guiding them to incorporate these concepts by participation in STEAM courses. The experimental group style (stage 2 to stage 1) allows students to learn on their own.
The research design is a kind of cross-study method. There are two reasons to use the cross-study method: 1) We did not know if the sequence of different teaching methods affected the results. 2) Students in both groups need to accept the same teaching method for better research ethics.
As can be seen in Figure 3, the comparison of pretest and posttest data were used to reflect about research questions “which kind of sequence of the course stage design has the remarkable impact on improving scientific creativity of elementary school students, as measured by the constituent scores of scientific creativity (fluency, flexibility, and originality)” and “is there a discrepancy in the effects of STEAM-based curriculum on various genders after they participated in the study.”
Instruments and Scoring
The scientific creativity test (Hu and Adey, 2002) was used as the main instrument in this study; its details are mentioned in Table 2. Author (2019) retested and validated this test, and the findings showed that science performances and creativity of students in both groups correctly represented their scientific creativity.
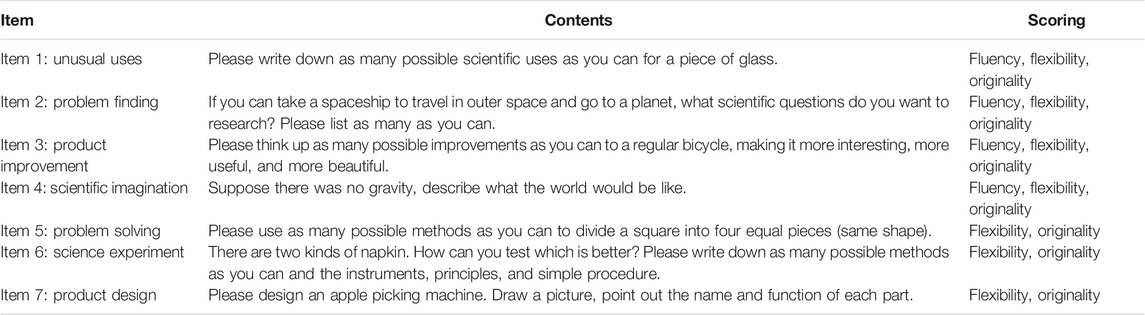
TABLE 2. The scientific creativity test (Hu and Adey, 2002; Huang and Wang, 2019).
The scientific creativity test was used in the research of Hu and Adey to investigate the scientific creativity of high school students, and reliability of Cronbach’s α reached 0.89. The test was converted into Chinese and retested in middle school students (n = 82, 38 males, 44 females; mean age ±SD = 14.1 ± 1.1 years) in Taiwan, and the revised reliability of Cronbach’s α reached 0.87. The test was designed for group administration with a time limit of 60 min. The examiner sought to make the students feel at ease but also wanted them to work hard to complete the tasks. Table 2 presents each of the seven items in the test.
The definition of scoring (Author, 2019; Hu and Adey, 2002) is as follows:
i) Fluency score: to count all of the separate responses given by the subjects, regardless of the quality.
ii) Flexibility score: to count the number of approaches or areas used in the answer.
iii) Originality score: scored based on the probability of a response among all responses, detailed in Table 3.
All answers by the students were read by three professional experts, and the results were the individual scores of fluency, flexibility, and originality. Each expert could then read the scores of the other two experts and make remarks or changes of their own. The three experts came to a unanimous decision after reviewing three times.
All of the data selected by the study was approved by the volunteering students, and they all had provided volunteer citation.
Results and Discussion
This study aimed to explore the influences of STEAM-based curriculum on the scientific creativity of elementary school students. There are two main research questions in this study: “which kind of sequence of the course stage design has the remarkable impact on improving scientific creativity of elementary school students” and “is there a discrepancy in the effects of the STEAM-based curriculum on various genders after they participated in the study.”
On the whole, the results from Table 3 show that not only all participants but also the control group and experimental group obtained significant higher scores of scientific creativity after joining the whole STEAM-based curriculum than before. The result supports that the curriculum design in this study could improve students’ scientific creativity.
The findings from Table 4 show in general that after joining the entire STEAM-based curriculum, not only all participants but also the control and experimental groups achieved significant higher scores than before. The outcome supports that the curriculum design in this study could improve students’ scientific creativity. This could be confirmed by previous studies which indicated that a multidisciplinary approach of project-based STEAM curriculum design would improve students’ scientific creativity (Erdoğan et al., 2013; Knezek et al., 2013; Kim et al., 2014; Ugras, 2018; Ngo and Phan, 2019; Akhmad et al., 2019; Genek and Doğança Küçük, 2020; Ozkan and Topsakal, 2021). In addition, Ozkan and Topsakal (2021) have concluded in their research that the STEAM design curriculum will boost scientific creativity in students’ verbal and figure domain-relevant skills. While using the scientific creativity test (Hu and Adey, 2002), the examiners need to clearly explain, motivate, and encourage students to try to promote their scientific creativity and problem-solving skills. That being said, in order for students to develop scientific creativity, teachers, who will be in contact with students for a long time, need to have a clear understanding of scientific creativity and STEAM courses and take active measures to create motivation and interest in students. This is consistent with the findings of Ugras (2018).
Data analyses of components of scientific creativity are also conducted in order to better understand the influence of the STEAM curriculum on participating students (Table 5).
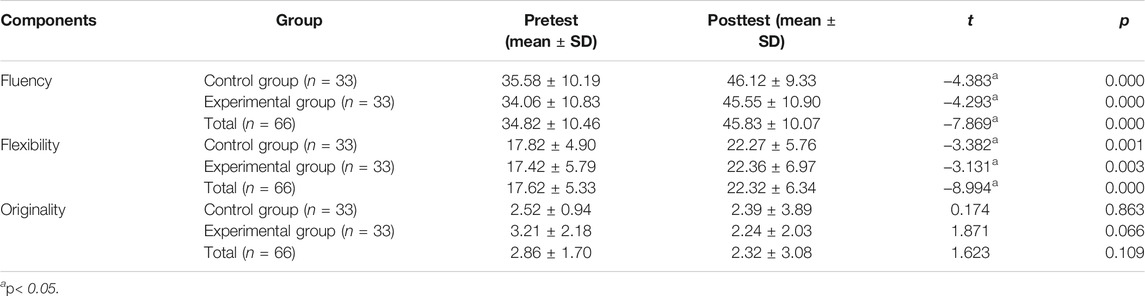
TABLE 5. The comparison table of pretest and posttest data of scores of the three components of scientific creativity.
The findings of Table 5 demonstrate that, after engaging in the STEAM-based program, the fluency and flexibility of students in both experimental and control groups were significantly higher. However, the originality score did not vary significantly in the pretest and posttest. This is in line with the findings by Darvishi and Pakdaman (2012). This means that, after participating in the STEAM-based curriculum, the development of elements of scientific creativity in students is different. The STEAM-based curriculum has a major impact on fluency and flexibility but does not significantly change the originality component. Many explanations can be given for this. Previous studies have shown that cultural background and students’ attitudes towards science and technology courses have an influence on students’ scientific creativity (Usta and Akkanat, 2015; De Vries and Lubart, 2019). In addition, other factors, such as the nature of the STEAM curriculum, the quality of the courses, the characteristics of the participating students, and cultural background can also influence students’ scientific creativity. Further study is therefore essential to confirm the above concerns and find ways to enhance all the three components of scientific creativity.
Next, this study wanted to find out which kind of sequence of the course stage design has more influence on improving scientific creativity of elementary school students. There are two kinds of curriculum designs in this study (see in Figure 3). The first one is used in the control group; students were required to participate in stage 1 course (Lock Science Courses) before going on to stage 2 (STEAM-based courses). This type of curriculum design aids students in constructing science principles first and then guiding them to incorporate these concepts through participation in STEAM-based courses. The second one is used in the experimental group; students were required to join stage 2 first and then stage 1. This style of curriculum design assists students in self-learning of interdisciplinary expertise in STEAM courses and then leads them in generalizing their science concepts. To answer this question, ANCOVA analysis to compare scientific creativity among different groups of students was performed (Table 6).
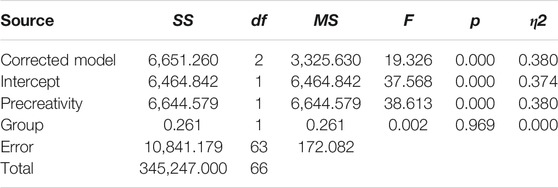
TABLE 6. ANCOVA analysis to compare scientific creativity among different groups of students (n = 66).
Table 5 shows that, after joining all STEAM-based curriculums, there were no significant differences in the control group and the experimental group. The different course sequence of the design, in other words, has little effect on the final results of scientific creativity of the students. According to Perignat and Katz-Buonincontro (2019), despite the variety of models and pedagogical approaches for STEAM education, they almost educate students to utilize cross-disciplinary knowledge to solve real-world problems. Besides, most contents of the scientific creativity test (Hu and Adey, 2002) were concerned with real-life problems; this could be a reason to understand why the students’ scientific creativity performances could be significantly improved by the STEAM-based curriculum participation no matter which stage was used first. However, these implications and hypotheses should be supported by further research.
Finally, to answer the question “is there a discrepancy in the effects of STEAM-based curriculum on various genders after they participated in the study,” an analysis of scientific creativity scores by gender before and after participating in the experiment has been performed (Table 7).
While there was no substantial difference in scientific creativity of females in the control group for pretest and posttest scores (p = 0.351 > 0.05), the other findings showed substantially higher scientific creativity scores regardless of gender in the control group, the experimental group, and all of the participants. The effects of the STEAM-based curriculum on various genders are obviously similar in this study. This result is consistent with that of previous studies (Darvishi and Pakdaman, 2012; Genek and Doğança Küçük, 2020). This indicates that males and females have the same potential to improve scientific creativity after participating in STEAM education and also contributes to affirming and reinforcing the goal of equality in education between men and women in the sustainable development goals (UN, 2015), where both genders are equally capable of developing scientific creativity. It is important to create conditions for both material and learning programs so that they have the ability to perfect themselves to the fullest.
Conclusion and Suggestion
This study aimed to investigate the influences of STEAM (science, technology, engineering, arts, and mathematics)-based curriculum on scientific creativity of elementary school students. The two core research questions are as follows:
i) Which kind of sequence of the course stage design has the remarkable impact on improving scientific creativity of elementary school students, as measured by the constituent scores of scientific creativity (fluency, flexibility, and originality)?
ii) Is there a discrepancy in the effects of STEAM-based curriculum on variousgenders after they participated in the study?
The main project of STEAM-based curriculum in this study is the house-shaped money-saving tube which was designed and produced by the authors’ team. Furthermore, the main concept of house-shaped money saving-tube is about lock science. This study adopted the counterbalance design. The control group joined Lock Science Courses first and then enrolled in STEAM-based courses, and the experimental group joined in the reverse sequence.
Data analyses show that the STEAM-based curriculum could increase scientific creativity of elementary school students, regardless of the kind of sequence of the course stage design. Specifically, in the three components of scientific creativity (fluency, flexibility, and originality), students, after participating in the whole STEAM-based curriculum, showed a significant improvement in fluency and flexibility components but the same result was not observed in the originality component.
This research also found no substantial difference in science creativity between males and females following STEAM-based curriculum participation. It is indicated that the development of scientific creativity of males and females participating in STEAM education is the same.
While the findings of ANCOVA analysis demonstrate that there are no significant differences between scientific creativity performances of students in the control group and experimental group after joining the whole STEAM-based curriculum, this study does not indicate which stage supports the scientific creativity of students more actively. Further research on these two stages (Lock Science Courses stage and STEAM-based courses stage) is therefore needed to verify.
The results suggested that both the STEAM courses and traditional science courses could help students preserve or continue their scientific creativity. Furthermore, considering gender differences, the STEAM course could improve female students’ scientific creativity; however, the traditional course did not show significant improvement in female students’ scientific creativity. This study suggests the investigators to extend the investigation period or to do the delayed posttest to validate the statements of implications.
Data Availability Statement
The raw data supporting the conclusions of this article will be made available by the authors, without undue reservation.
Ethics Statement
Written informed consent was obtained from the minors’ legal guardian/next of kin for the publication of any potentially identifiable images or data included in this article.
Author Contributions
All authors contributed to conception and design of the study. N-HT, C-FH, K-HH, and K-LL organized the database and collected data. N-HT performed the statistical analysis and wrote the first draft of the manuscript. C-FH, K-HH, K-LL, and J-FH listed sections of the manuscript. All authors contributed to manuscript revision and read and approved the submitted version.
Conflict of Interest
The authors declare that the research was conducted in the absence of any commercial or financial relationships that could be construed as a potential conflict of interest.
Publisher’s Note
All claims expressed in this article are solely those of the authors and do not necessarily represent those of their affiliated organizations, or those of the publisher, the editors, and the reviewers. Any product that may be evaluated in this article, or claim that may be made by its manufacturer, is not guaranteed or endorsed by the publisher.
References
Akhmad, Y., Masrukhi, M., and Indiatmoko, B. (2019). The Effectiveness of the Integrated Project-Based Learning Model STEM to Improve the Critical Thinking Skills of Elementary School Students. Educ. Manage. 9 (1), 9–16.
Ayas, M. B., and Sak, U. (2014). Objective Measure of Scientific Creativity: Psychometric Validity of the Creative Scientific Ability Test. Think. Skills. Creat. 13, 195–205. doi:10.1016/j.tsc.2014.06.001
Conradty, C., and Bogner, F. X. (2019). From STEM to STEAM: Cracking the Code? How Creativity & Motivation Interacts with Inquiry-Based Learning. Creativity Res. J. 31 (3), 284–295. doi:10.1080/10400419.2019.1641678
Csikszentmihalyi, M. (1996). Creativity: Flow and the Psychology of Discovery and Invention. New York: Harper/Collins.
Darvishi, Z., and Pakdaman, S. (2012). “Fourth Grade Slump in Creativity”: Development of Creativity in Primary School Children. GSTF J. L. Soc. Sci. (Jlss) 1 (2), 40–48. doi:10.1037/e527322013-009
Dunlap, R. E., and Jorgenson, A. K. (2012). “Environmental Problems,” in The Wiley-Blackwell Encyclopedia of Globalization, Editor G. Ritzer Wiley Online Library. doi:10.1002/9780470670590.wbeog174
Erdoğan, N., Çorlu, M. S., and Capraro, R. M. (2013). Defining Innovation Literacy: Do Robotics Programs Help Students Develop Innovation Literacy Skills? Int. Online J. Educ. Sci. 5 (1), 1–9.
Feldhusen, J., and Goh, B. E. (1995). Assessing and Accessing Creativity: An Integrative Review of Theory, Research, and Development. Hcrj 8 (3), 231–247. doi:10.1207/s15326934crj0803_3
Ford, D. Y., and Harris, J. J. (1992). The Elusive Definition of Creativity. J. Creat. Behav. 26 (3), 186–198. doi:10.1002/j.2162-6057.1992.tb01175.x
Genek, S. E., and Doğança Küçük, Z. (2020). Investigation of Scientific Creativity Levels of Elementary School Students Who Enrolled in a STEM Program. İlköğretim Online. 19 (3), 1715–1728. doi:10.17051/ilkonline.2020.734849
Hoffmann, J. D., Ivcevic, Z., and Maliakkal, N. (2021). Emotions, Creativity, and the Arts: Evaluating a Course for Children. Empirical Stud. Arts. 39 (2), 123–148. doi:10.1177/0276237420907864
Hu, W., and Adey, P. (2002). A Scientific Creativity Test for Secondary School Students. Int. J. Sci. Educ. 24 (4), 389–403. doi:10.1080/09500690110098912
Huang, P.-S., Peng, S.-L., Chen, H.-C., Tseng, L.-C., and Hsu, L.-C. (2017). The Relative Influences of Domain Knowledge and Domain-General Divergent Thinking on Scientific Creativity and Mathematical Creativity. Think. Skills. Creat. 25, 1–9. doi:10.1016/j.tsc.2017.06.001
Huang, C.-F., and Wang, K.-C. (2019). Comparative Analysis of Different Creativity Tests for the Prediction of Students’ Scientific Creativity. Creat. Res. J. 31 (4), 443–447. doi:10.1080/10400419.2019.1684116
Kim, D.-H., Ko, D. G., Han, M.-J., and Hong, S.-H. (2014). The Effects of Science Lessons Applying STEAM Education Program on the Creativity and Interest Levels of Elementary Students. J. Korean Assoc. Res. Sci. Educ. 34 (1), 43–54. doi:10.14697/jkase.2014.34.1.1.00043
Kind, P. M., and Kind, V. (2007). Creativity in Science Education: Perspectives and Challenges for Developing School Science. Stud. Sci. Educ. 43 (1), 1–37. doi:10.1080/03057260708560225
Knezek, G., Christensen, R., Wood, T. T., and Periathiruvadi, S. (2013). Impact of Environmental Power Monitoring Activities on Middle School Student Perceptions of STEM. Sci. Educ. Int. 24 (1), 98–123.
Lubart, T. I. (1994). “Creativity,” in Handbook of Perception and Cognition: Thinking and Problem Solving. Editor R. J. Sternberg (New York: Academic Press), 289–332. doi:10.1016/b978-0-08-057299-4.50016-5
Meador, K. S. (2003). Thinking Creatively about Science Suggestions for Primary Teachers. Gifted Child. Today. 26 (1), 25–29. doi:10.4219/gct-2003-93
Newton, L., and Newton, D. (2010). Creative Thinking and Teaching for Creativity in Elementary School Science. Gifted Talented Int. 25 (2), 111–124. doi:10.1080/15332276.2010.11673575
Ngo, H. Q. T., and Phan, M.-H. (2019). Design of an Open Platform for Multi-Disciplinary Approach in Project-Based Learning of an EPICS Class. Electronics 8 (2), 200. doi:10.3390/electronics8020200
OECD (2019a). Future Of Education and Skills 2030: OECD Learning Compass 2030. Paris: OECD Publishing.
OECD (2019b). Framework for the Assessment of Creative Thinking in Pisa 2021 (Third Draft). Paris: OECD Publishing.
Ozkan, G., and Umdu Topsakal, U. (2021). Exploring the Effectiveness of STEAM Design Processes on Middle School Students' Creativity. Int. J. Technol. Des. Educ. 31 (1), 95–116. doi:10.1007/s10798-019-09547-z
Perignat, E., and Katz-Buonincontro, J. (2019). STEAM in Practice and Research: An Integrative Literature Review. Think. Skills. Creat. 31, 31–43. doi:10.1016/j.tsc.2018.10.002
Said-Metwaly, S., Fernández-Castilla, B., Kyndt, E., and Van den Noortgate, W. (2018). The Factor Structure of the Figural torrance Tests of Creative Thinking: A Meta-Confirmatory Factor Analysis. Creativity Res. J. 30 (4), 352–360. doi:10.1080/10400419.2018.1530534
Shi, B., Cao, X., Chen, Q., Zhuang, K., and Qiu, J. (2017). Different Brain Structures Associated with Artistic and Scientific Creativity: a Voxel-Based Morphometry Study. Sci. Rep. 7 (4), 42911–42918. doi:10.1038/srep42911
Sternberg, R. J., and Lubart, T. I. (1993). Creative Giftedness: A Multivariate Investment Approach. Gifted Child. Q. 37 (1), 7–15. doi:10.1177/001698629303700102
Suyidno, S., Susilowati, E., Arifuddin, M., Misbah, M., Sunarti, T., and Dwikoranto, D. (2019). Increasing Students' Responsibility and Scientific Creativity through Creative Responsibility Based Learning. J. Penelit. Fis. Apl. 9 (2), 178–188. doi:10.26740/jpfa.v9n2.p147-157
Thuneberg, H. M., Salmi, H. S., and Bogner, F. X. (2018). How Creativity, Autonomy and Visual Reasoning Contribute to Cognitive Learning in a STEAM Hands-On Inquiry-Based Math Module. Think. Skills. Creat. 29, 153–160. doi:10.1016/j.tsc.2018.07.003
Ugras, M. (2018). The Effect of STEM Activities on STEM Attitudes, Scientific Creavity and Motivation Beliefs of the Students and Their Views on STEM Education. Int. Online J. Educ. Sci. 10 (5), 165–182.
UN (2015). Transforming Our World: The 2030 Agenda for Sustainable Development. United Nations. Available at: https://sdgs.un.org/2030agenda
Usta, E., and Akkanat, Ç. (2015). Investigating Scientific Creativity Level of Seventh Grade Students. Proced. - Soc. Behav. Sci. 191, 1408–1415. doi:10.1016/j.sbspro.2015.04.643
Vries, H. B., and Lubart, T. I. (2019). Scientific Creativity: Divergent and Convergent Thinking and the Impact of Culture. J. Creat. Behav. 53 (2), 145–155. doi:10.1002/jocb.184
Keywords: scientific creativity, science education, steam, STEAM-based curriculum, elementary school students
Citation: Tran N-H, Huang C-F, Hsiao K-H, Lin K-L and Hung J-F (2021) Investigation on the Influences of STEAM-Based Curriculum on Scientific Creativity of Elementary School Students. Front. Educ. 6:694516. doi: 10.3389/feduc.2021.694516
Received: 13 April 2021; Accepted: 20 August 2021;
Published: 05 October 2021.
Edited by:
Weihua Niu, Pace University, United StatesReviewed by:
Olivia López Martínez, University of Murcia, SpainChih Ming Chu, National Ilan University, Taiwan
Copyright © 2021 Tran, Huang, Hsiao, Lin and Hung. This is an open-access article distributed under the terms of the Creative Commons Attribution License (CC BY). The use, distribution or reproduction in other forums is permitted, provided the original author(s) and the copyright owner(s) are credited and that the original publication in this journal is cited, in accordance with accepted academic practice. No use, distribution or reproduction is permitted which does not comply with these terms.
*Correspondence: Jeng-Fung Hung, dDE4NzNAbmtudWNjLm5rbnUuZWR1LnR3