Corrigendum: Dam removal enables diverse juvenile life histories to emerge in threatened salmonids repopulating a heterogeneous landscape
- 1Ocean Associates Inc., Under Contract to Northwest Fisheries Science Center, National Marine Fisheries Service, NOAA, Seattle, WA, United States
- 2Natural Resources Department, Lower Elwha Klallam Tribe, Port Angeles, WA, United States
- 3Fish Ecology Division, Northwest Fisheries Science Center, National Marine Fisheries Service, NOAA, Seattle, WA, United States
- 4The Conservation Angler, Port Angeles, WA, United States
Human stressors block, eliminate, and simplify habitat mosaics, eroding landscapes’ life history diversity and thus biological resilience. One goal of restoration is to alleviate human stressors that suppress life history diversity, but life history responses to these efforts are still coming into focus. Here, we report life history diversity emerging in threatened salmonids (Oncorhynchus spp.) repopulating the recently undammed Elwha River (WA, United States) in adjacent but environmentally distinct tributaries. The ~20 km tributaries entered the Elwha River <1 km apart, but one had a colder stream temperature regime and swifter waters due to its high, snow-dominated elevation and steep valley gradient (~3%), while the other had a warmer stream temperature regime and slower waters because it drained a lake, was at lower elevation, and had a lower stream gradient (~1.5%). Following the 2012 removal of Elwha Dam, the tributaries’ salmonids generally became more abundant and expressed diverse life histories within and among species. The warmer, low-gradient tributary produced more age-1+ coho salmon while the colder, steeper tributary produced a notably high abundance of steelhead smolts in 2020. Additionally, salmonids exiting the warmer tributary were older and possibly larger for their age class, emigrated ~25 days earlier, and included age-0 Chinook salmon that were larger. Also, assemblage composition varied among years, with the most abundant species shifting between Chinook salmon and coho salmon, while steelhead abundances generally increased but were patchy. These patterns are consistent with a newly accessible, heterogeneous landscape generating life history diversity against the backdrop of patchy recruitment as salmonids—some with considerable hatchery-origin ancestry—repopulate an extirpated landscape. Overall, dam removal appears to have promoted life history diversity, which may bolster resilience during an era of rapid environmental change and portend positive outcomes for upcoming dam removals with similar goals.
Introduction
Diversity promotes ecological resilience and efforts to restore connected, functional habitats often seek to rebuild aspects of diversity that have been depleted. Diverse habitats enable varied life histories, creating biological systems that spread risk, locally process disturbances, and exploit unpredictable opportunities (Levin, 1992; Tilman and Downing, 1994; Schindler et al., 2015). In this way, diversity helps promote stability over greater temporal and spatial scales, which is key to reliable ecosystem services (Greene et al., 2010; Schindler et al., 2010). Human stressors can suppress diversity within and among species, which has prompted attempts to rehabilitate biological diversity by reconnecting and restoring impaired habitats (e.g., Boughton et al., 2022; Soulodre et al., 2022). However, empirical outcomes of such efforts are still coming into focus.
Rebuilding diversity is critical for Pacific salmon and trout (Oncorhynchus spp.; hereafter: salmonids). Salmonids are culturally, ecologically, and economically significant taxa native to the Pacific Rim. They are often migratory and adapted to diverse habitat mosaics across a wide range of landscapes (Quinn, 2018). Within and among species, life history attributes such as differences in timing (e.g., age at ocean entry, age at maturity, and spawning season) and habitat use (e.g., low elevation vs. high elevation spawning) are sustained through variable physical environments and genetics, contributing to population stability and increasing the reliability of fisheries (Greene et al., 2010; Schindler et al., 2010; Hodge et al., 2016). A suite of diverse life histories—using a variety of habitats at different times for different reasons—can help buffer annual and seasonal variation in population survival, which is critical for salmon given their naturally high mortality rates (Quinn, 2018). For example, Alaska’s comparatively undeveloped landscape and its varied, functional habitats support substantial life history diversity that contributes to more sustainable production and more consistent fisheries (Hilborn et al., 2003; Schindler et al., 2010; Brennan et al., 2019). In contrast, human stressors across highly-modified habitats in California have eroded the diversity and resilience of what was once a reliable fishery and made it more susceptible to drought and temperature fluctuation (Carlson and Satterthwaite, 2011; Munsch et al., 2022).
Human modifications and stressors have imperiled salmonids, particularly across their southern range (Nehlsen et al., 1991). Consequently, many efforts have sought to restore lost habitat and rebuild life history diversity to improve productivity, resilience, and viability, especially against the backdrop of increasing climatic impacts (e.g., rising temperatures and shifting streamflow patterns; Beechie et al., 2013). One particularly promising approach to improve salmonid life history diversity is the removal of impassable dams to restore formerly connected habitats. Restored connectivity can increase the variety of habitats salmon use, within a species range of preference, and provide salmon with greater potential for expressing diversity across landscapes (Pess et al., 2014). For instance, deep-bodied coho salmon (O. kisutch) prefer slow-moving pools in low-gradient streams whereas cylindrical-shaped steelhead (O. mykiss) prefer shallower, faster waters in steep streams, and Chinook salmon (O. tshawytscha) are more intermediate (Bisson et al., 1988). Further, each species generally remains in freshwater for different periods of time and migrates to sea at different ages and sizes (Quinn, 2018). Because survival, movement, and migration timing partly depends on juvenile growth in freshwater, however, different habitats can produce slightly different life history trajectories within each species. For example, water temperature strongly influences incubation and growth rates, and thermal regimes can vary extensively within and across stream networks (Hawkins et al., 2020). Given adequate food, salmonids tend to grow fastest as water temperatures increase up to and within their thermal optimum (Brett et al., 1969). Additionally, eggs incubate faster in warmer water (Murray and McPhail, 1988). Salmonids often move and migrate when they exceed a threshold size (e.g., reaction norm: Bjornn et al., 1968; Peven et al., 1994), ostensibly to optimize differential tradeoffs in growth and mortality regimes in freshwater and marine habitats, which is mediated by size-selective mortality (Quinn, 2018). As a result, juvenile salmonids often outmigrate earlier (Roper and Scarnecchia, 1999) and at younger ages (Cline et al., 2019) in warmer years and warmer systems that do not frequently exceed the species thermal optimum (Liermann et al., 2017). Accordingly, different temperature regimes across landscapes are likely to promote different growth and migration patterns, and restoring access to a broader range of temperature regimes may therefore help salmon increasingly realize greater life history diversity.
Here, we quantify the life history attributes of salmonid assemblages in two newly accessible, adjacent, but environmentally-distinct tributaries following dam removal. We focus on the species-specific outmigration timing, age structure, and length at date of seaward migration in juvenile coho salmon, Chinook salmon, and steelhead. The research was conducted in the Elwha River (WA, United States) where dams previously blocked 95% of anadromous habitat and dam removal in 2012 restored habitat access for the first time since 1912. Previous work indicates the diversity and abundance of salmonids has increased as they access former habitats and resume life histories that require a connected river system (e.g., Quinn et al., 2017; Duda et al., 2021; Fraik et al., 2021). However, most of that research was centered on the adult life stage. For juveniles, Liermann et al. (2017) found a warmer, low-gradient stream produced more coho salmon smolts and earlier-outmigrating coho salmon fry compared to a colder stream. Building on this, our goal was to use new data to quantify and compare juvenile life history expression in newly opened habitats with contrasting features. Specifically, we focus on the juvenile life stage and analyze data collected from 2016 to 2021 to characterize and compare the timing of juvenile migration, age structure, and growth trajectories of all salmonid species that were commonly found during sampling. These results may inform expectations in other systems where dam removal and other restoration actions seek to improve access to diverse landscapes and increase life history diversity.
Methods
Study region and species
The 72 km Elwha River drains an old-growth, forested landscape within Washington State that connects perennial snowfields in the Olympic Mountains to the Salish Sea (Figure 1). The Elwha River’s tributaries are generally shaded, primarily by Douglas fir (Pseudotsuga menziesii) and western hemlock (Tsuga heterophylla), while understory vegetation includes sword fern (Polystichum munitum) and Oregon grape (Mahonia spp.), among others (Munn et al., 1999). Substrate size varies across the basin with local habitat type (e.g., riffle and run) and stream order, with mean sizes ranging from ~10–170 mm (Munn et al., 1999; their Figure 11). For millennia, people including the Lower Elwha Klallam Tribe shared this landscape with an abundance and diversity of salmonids that enabled sustenance, identity, and culture (reviewed by Guarino, 2013). In 1912, Olympic Power and Development Company constructed the Elwha Dam on the river’s mainstem at rkm 7.9, blocking ~95% of accessible habitat to salmonids (Olympic Power also constructed an additional dam, Glines Canyon, upstream in 1925; Brenkman et al., 2019). In 1992, following declines in salmonid production, tribal advocacy, and legal proceedings, U.S. Congress passed the Elwha River Ecosystem and Fisheries Restoration Act, authorizing the eventual removal of the dams in 2012. Notably, much of the watershed drained by the Elwha River remains undeveloped as it is located in Olympic National Park. This effort constituted the largest dam removal project in the world and is arguably the most direct attempt ever to recover a human-stressed landscape’s natural potential to produce abundant, diverse salmonids.
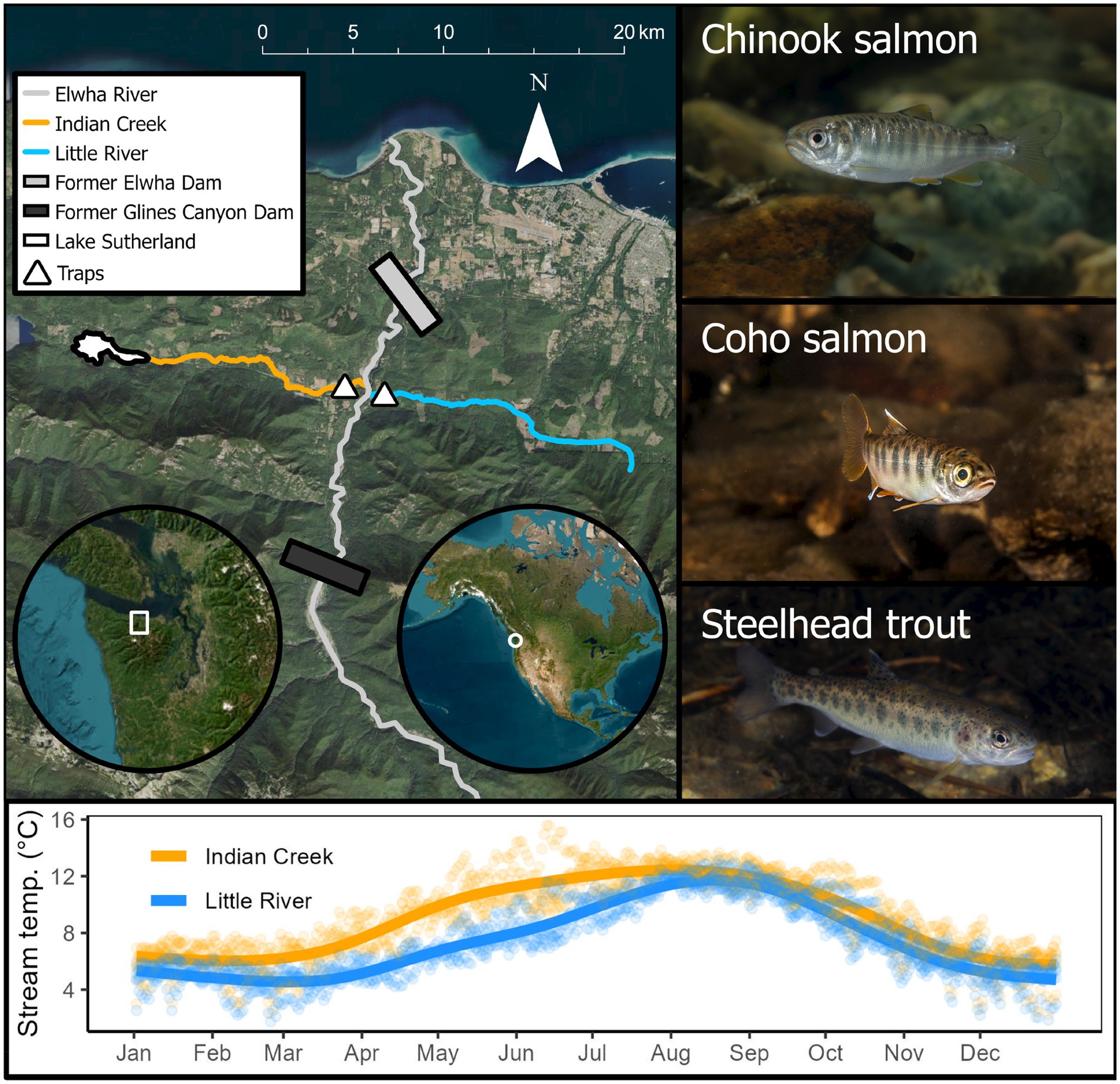
Figure 1. Study region, focal species, and tributary temperature regimes. Lines describing temperature regimes are cubic regression splines fit to the points as a visual aid. Here and after, we follow the convention that Indian Creek, which is warmer, is depicted in orange, while Little River, which is cooler, is depicted in blue. Photographs by Morgan H. Bond.
Despite its history of stressors, the Elwha River is inhabited by many species of Pacific salmon and trout (Duda et al., 2021). Among these species are Chinook salmon, coho salmon, and steelhead, which rear in streams and are the focus of this study (Figure 1). The Chinook salmon and steelhead populations in this system are listed as threatened under the U.S. Endangered Species Act. These anadromous species express considerable variation in their juvenile life histories, including their seasonal outmigration timing and length of freshwater rearing (Quinn, 2018). In addition to the species that we focus on here because they were abundant in the observed tributaries, chum salmon (O. keta), pink salmon (O. gorbuscha), cutthroat trout (O. clarkii), bull trout (Salvelinus confluentus), sculpins (Cottidae), and lamprey (Entosphenus tridentatus) inhabit the Elwha River system.
Dam removal restored access for salmonids to the Elwha River’s environmentally-distinct tributaries Indian Creek and Little River. They are the first major tributaries encountered by salmon swimming upstream, enter the Elwha River mainstem within ~1 km of one another, and drain similar areas (Indian Creek: 60 km2, Little River: 52 km2). However, they support markedly different habitats—one being cold and steep, the other being warmer and lower-gradient. Little River has a colder stream temperature regime (annual mean: 7.5°C Washington Department of Ecology, 2016; Figure 1) due to its elevation, including the snow dominated zone with perennial snowfields, and steeper valley gradient (~3%). In contrast, Indian Creek has a warmer stream temperature regime (annual mean: 9°C, Washington Department of Ecology, 2016; Figure 1) because it drains Lake Sutherland, is at lower elevation, has a lower stream gradient (~1.5%), and has ample beaver activity. Note: the tributaries’ daily temperature values in Figure 1 are a combination of empirical observations and imputed values estimated by quantifying statistical relationships between temperature in the tributaries and the Elwha River mainstem and neighboring Quinault River, for which more complete time series were available (details: Liermann et al., in review, this issue).
Also of note, hatcheries have in some years transported adults from the lower Elwha River to Indian Creek and Little River. Specifically, 179 and 117 Chinook salmon were relocated to Indian Creek in 2012 and 2013, respectively; 11 and 35 steelhead were relocated to Indian Creek in 2012 and 2016, respectively; and 35, 88, and 59 steelhead were relocated to Little River in 2012, 2013, and 2014, respectively (details: Pess et al. in review; this issue). Additionally, hatcheries seeded Indian Creek in 2011–2014, 2016, 2017, and 2021 and Little River in 2011–2013 with surplus adult coho salmon to accelerate recovery (McHenry et al., 2022). Furthermore, adult Chinook salmon returning to the Elwha River system at present are predominantly hatchery origin (Pess et al., in review, this issue). That is, juveniles emigrating from Indian Creek and Little River are natural-origin, but their ancestors, including some of their parents, were likely raised by hatcheries.
Data collection
Screw trap observations quantified attributes of salmonids migrating from Indian Creek and Little River. Traps were located at river kilometer (rkm) 0.5 in Little River and 0.7 rkm in Indian Creek and were monitored from late winter to late summer 2016–2021. Fieldworkers identified and measured individual lengths, and Chinook and coho salmon were classified as age-0 or age-1+ based on length cutoffs (80 and 60 mm for coho and Chinook salmon, respectively). However, in a separate analysis on a subset of these outmigrants, we explored the possibility that some coho salmon and steelhead were age-2. Indeed, steelhead were not assigned age classes in the field because of their complex age structure and phenology that produces a less obvious break in length distributions (given the date) between age classes. Instead, we attempted to make these assignments and make primary inference about individual ages in a more rigorous modeling analysis described below.
Tributaries were inhabited by the anadromous (steelhead) and resident (rainbow trout) forms of O. mykiss. Fieldworkers followed the convention of only identifying smolting individuals (characterized by silver coloration adapted to marine habitats) as steelhead and not identifying individuals under 55 mm as steelhead, because O. mykiss and sympatric cutthroat trout (O. clarkii) are not visually distinguishable below that length.
Trap efficiency was measured by mark-recapture procedures whereby fieldworkers dyed 100–200 age-0 fish with Bismarck brown and released them upstream of the trap, enabling calculation of weekly efficiency as the proportion of recovered marked fish. The procedure was repeated weekly and marked fish recaptured in the trap usually arrived within 3–4 days after release. Typically, marked fish were natural-origin (i.e., not hatchery-origin) Chinook salmon, coho salmon, and steelhead. To calculate capture efficiencies during periods of low natural abundance, juveniles transported from hatcheries were used as surrogates. We divided daily counts by efficiency to estimate total outmigration counts. We inferred that juveniles were natural origin because (1) hatcheries on the Elwha River are below the former Elwha Dam location and thus below the tributary mouths and (2) hatchery coho salmon and steelhead were identifiable via clipped adipose fins and traps caught zero clipped fish.
In addition, fieldworkers enumerated Chinook salmon redds (egg nests) across the tributaries during the spawning season. We chose to include these data post hoc after noticing substantial annual variation in juvenile Chinook salmon that we thought may be due to variation in adult spawning abundances.
Analyses
We used mixed effects models to (1) compare the abundances of salmonids between tributaries, (2) compare the timing of median annual outmigration date between tributaries, and (3) examine for effects of density dependence on Chinook salmon length. Models comparing abundances and outmigation timing were similarly parameterized as:
where log(abundance+1) or median migration date μ was a function of an intercept β0, an effect β1 of tributary X1, and random intercepts ac. Here and after, tributaries were categorical variables, meaning their model parameters were informed by binary vectors of whether (1) or not (0) observations occurred in a given tributary. For abundance models, c indicated different years and for the outmigration model, c indicated different combinations of years, species, and fishes’ identifiable age class (e.g., 2016 Chinook age-0, 2020 steelhead smolt). For the latter, we combined these variables rather than including separate random intercept parameters for year and salmonid type to avoid a scenario whereby models attempted to fit random effects to variables with few levels for each variable (Bolker et al., 2009), while following the guiding premise that the model should account for fish of the same type and within the same year outmigrating at similar times to better isolate differences in timing among tributaries. Also for the outmigration timing model, we only compared combinations of years, species, and identifiable age classes when abundances in both tributaries’ traps were in the top 33rd percentile of abundances (5,804 individuals) relative to each combination of year, species, age class, and tributary to focus on comparisons with more robust sample sizes. Initial explorations suggested that this was a natural cutoff below which counts were too few or patchy for medians and cumulative distribution functions to informatively describe outmigration timing.
Then, we used non-metric multidimensional scaling (NMDS) to compare the composition of salmonid types (each species and identifiable age class; hereafter “assemblage”) between tributaries and among years. The NMDS was fit to two dimensions using a Bray–Curtis dissimilarity matrix. The format of this data was rows: years, columns: salmonid types (e.g., Chinook age-0, Coho age-1), and cell data: summed abundance. To test for differences in composition between assemblages, we used a permutational ANOVA (PERMANOVA; Anderson, 2001), which was also implemented using a Bray–Curtis dissimilarity matrix and included the categorical explanatory variables of tributary and year.
Next, we used Bayesian mixture models to assign salmonids to age classes and quantify differences in length between tributaries. Formally, these models were parameterized where the age, , of fish , followed a categorical distribution with a dirichlet prior on . The resulting age then determined the age specific length distributions.
The mean age specific fish length was modeled using year and tributary specific effects ( and ) along with a linear relationship with julian date ( ).
The year effect, , was modeled hierarchically using a normal distribution with mean 0 and estimated standard deviation. The tributary effect, , was modeled with normal priors. See Supplementary material for more details.
While steelhead often outmigrate at age-2 (Busby et al., 1996) and preliminary data explorations suggested their lengths followed a relatively clear trimodal distribution (ages 0–2), this was not the case with coho salmon. This assemblage included distinct age-0 and age-1 individuals, but also—particularly in Indian Creek—markedly larger individuals. We therefore fit coho salmon lengths to a trimodal mixture model as we did for the steelhead, but refer to the largest coho salmon tentatively as “age 2?” and address this uncertainty in the Discussion.
Additionally, in the case of Chinook salmon, which were almost entirely age-0, we used a linear mixed effects model to quantify differences between tributaries in size at date. Formally, this model was parameterized as
where Chinook salmon length μ was a function of an intercept β0, an effect β1 of day of year X1 an effect β2 of tributary X2, an interactive effect β3 between day of year and tributary, and a random intercept a of year c. In plain terms, this model quantified how much larger Chinook salmon in Indian Creek were than in Little River, while accounting for seasonal growth that increasingly separated juvenile lengths between tributaries as winter progressed into summer as well as interannual differences in length among years due to factors not explicitly accounted for.
We also investigated effects of density dependence on Chinook salmon length within each tributary. These models were parameterized as:
where Chinook salmon length μ was a function of an intercept β0, an effect β1 of total annual Chinook salmon outmigrants X1, an effect β2 of day of year X2, the interactive effect β3 of total annual Chinook salmon outmigrants and the day of year, and random intercepts a for each year c. We used this model structure because we anticipated that Chinook salmon would be smaller in years with more conspecific migrants due to competition, that salmon would be larger later in the year, and that potential effects of competition on salmon size would be more apparent later in the year as salmon had experienced more time to grow. Ideally, we would also have examined for effects of density dependence on lengths of other salmonid species, but we decided against this because other species’ longer rearing times complicated our ability to quantify competition. For coho salmon and steelhead, multiple age classes competed year-round while growth and mortality occurred prior to measurements at the traps, introducing much uncertainty in attempts to describe how many fish were present and how high their resource demands were during years prior. This challenge was less concerning in the case of Chinook salmon because nearly all juveniles outmigrated at age-0 before summer, making total annual counts more conducive to estimating competition.
Results
Indian Creek and Little River supported a diversity of abundances, outmigration timings, and ages across Chinook salmon, coho salmon, and steelhead (Figures 2–4). Salmonids outmigrated from January to November, mostly within late winter to early summer. Chinook salmon generally migrated earliest, followed by a relatively protracted outmigration of coho salmon that began before and ended after steelhead outmigrations. Abundance and composition also varied among years, with greater abundances in 2019–2021 than 2016–2018, coho salmon dominating the assemblage in 2017 and 2018, and Chinook salmon dominating the assemblage in 2016, 2019, and 2020. Juveniles were generally more abundant in Indian Creek than Little River, except in 2020 when juveniles were much more abundant in Little River and in 2018 when abundances were approximately equal between tributaries. Chinook salmon were dominated by small individuals presumably age-0 whereas coho salmon and steelhead included a wider range of sizes that presumably reflected multiple age classes. Overall, while distinct patterns in assemblages were present between tributaries (discussed below), there was also considerable variation among years, with each year and tributary supporting different assemblages. Altogether, this variation meant that the timing, shape, and number of migration peaks was markedly different among years and between tributaries.
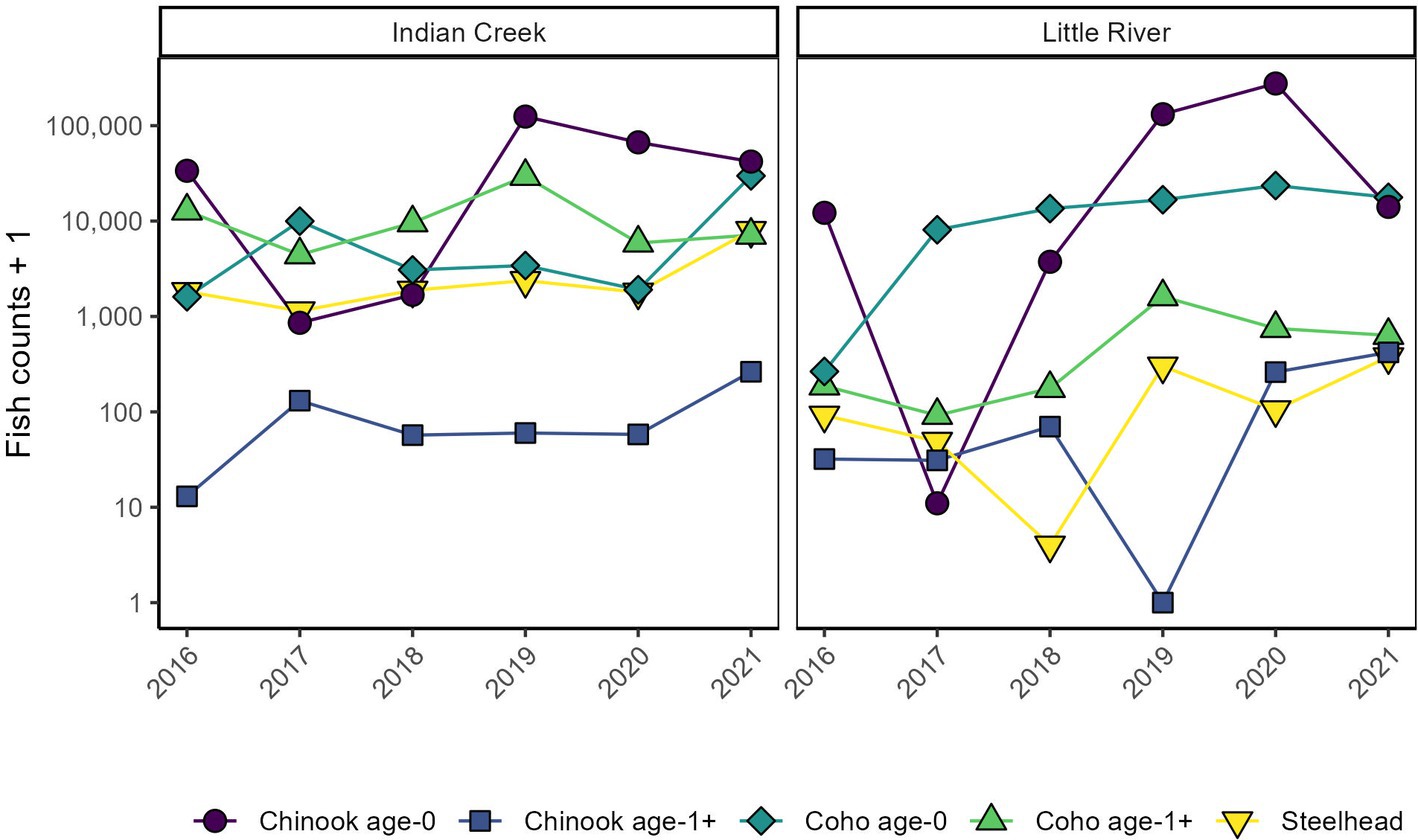
Figure 2. Assemblage composition compared between tributaries and among years. Y axes are log transformed to improve visibility of smaller counts.
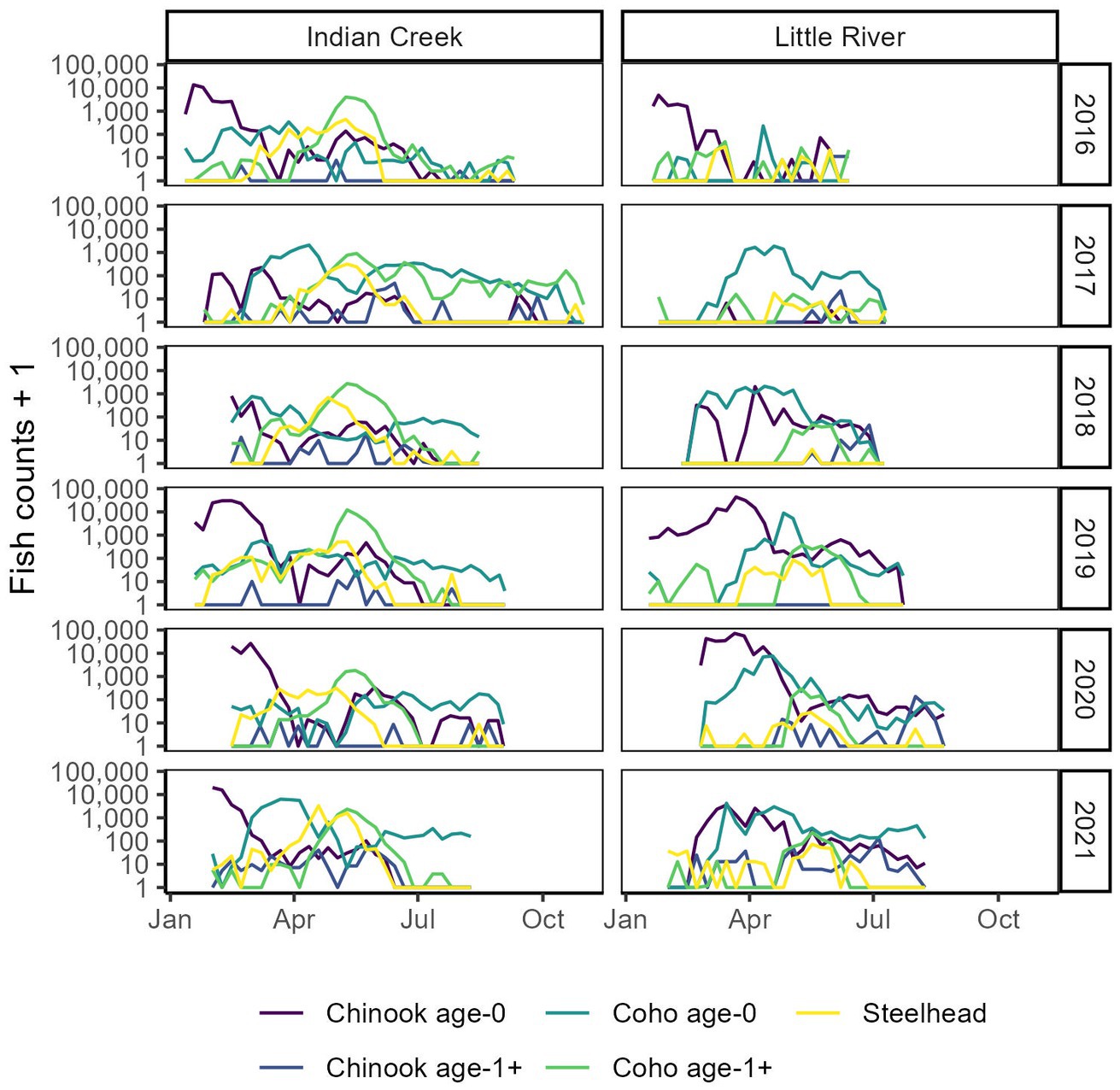
Figure 3. Timing and assemblage composition compared between tributaries and among years. Within-year counts are summed by week. Y axes are log transformed to improve visibility of smaller counts.
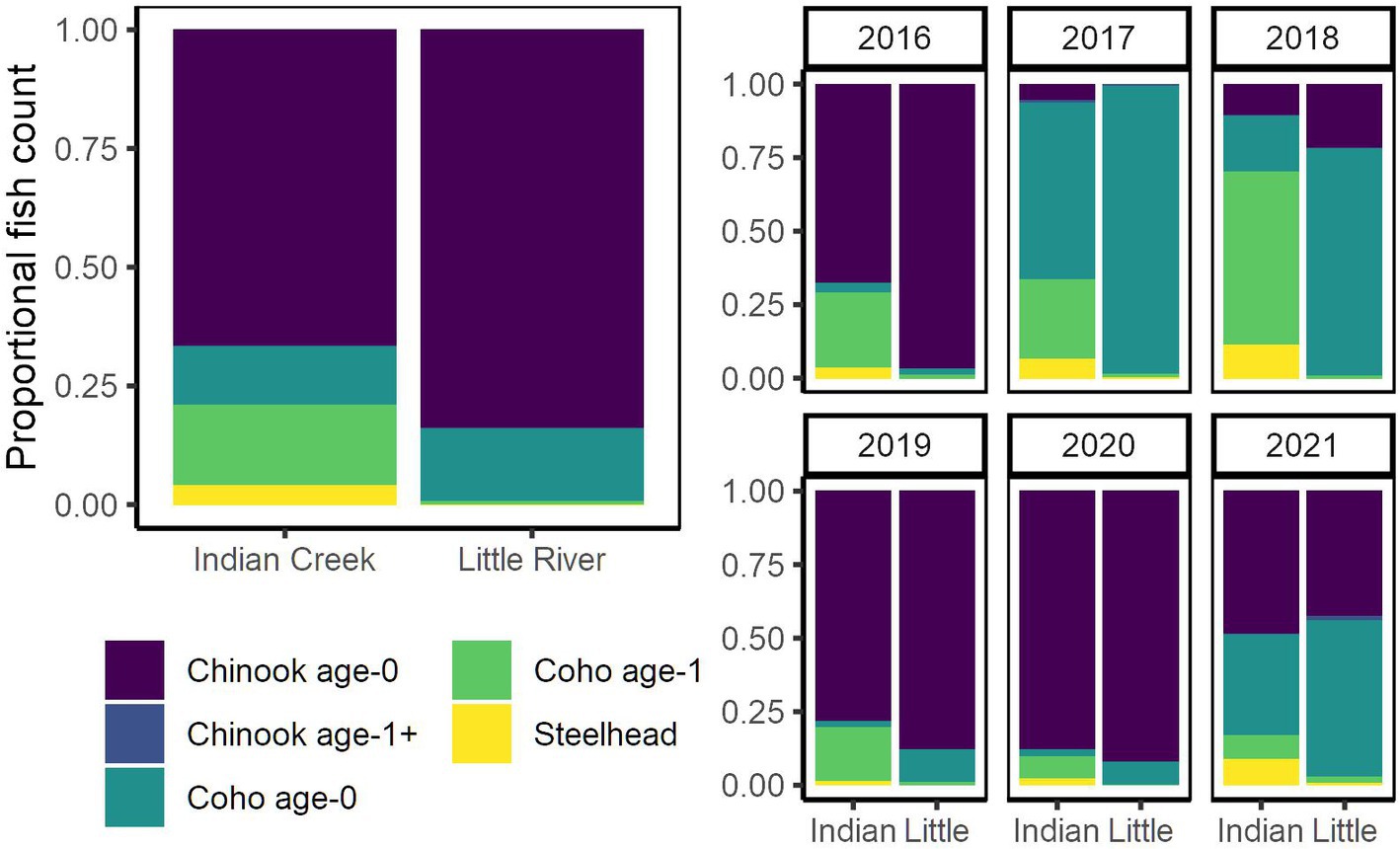
Figure 4. Proportional composition of salmonid assemblage compared between Indian Creek and Little River for all years (left) and individual years (right).
Indian Creek and Little River supported distinct salmonid assemblages (Figures 2–5). Indian Creek supported greater abundances of coho salmon, especially age 1+ individuals, and steelhead. Indeed, age-1 coho salmon and steelhead were significantly more abundant in Indian Creek than Little River (Table 1, p < 0.001 [coho salmon], p = 0.0021 [steelhead]). Abundances of other salmonids did not significantly differ between tributaries (Table 1, p ≥ 0.44). In both tributaries, age-0 Chinook salmon were numerically dominant.
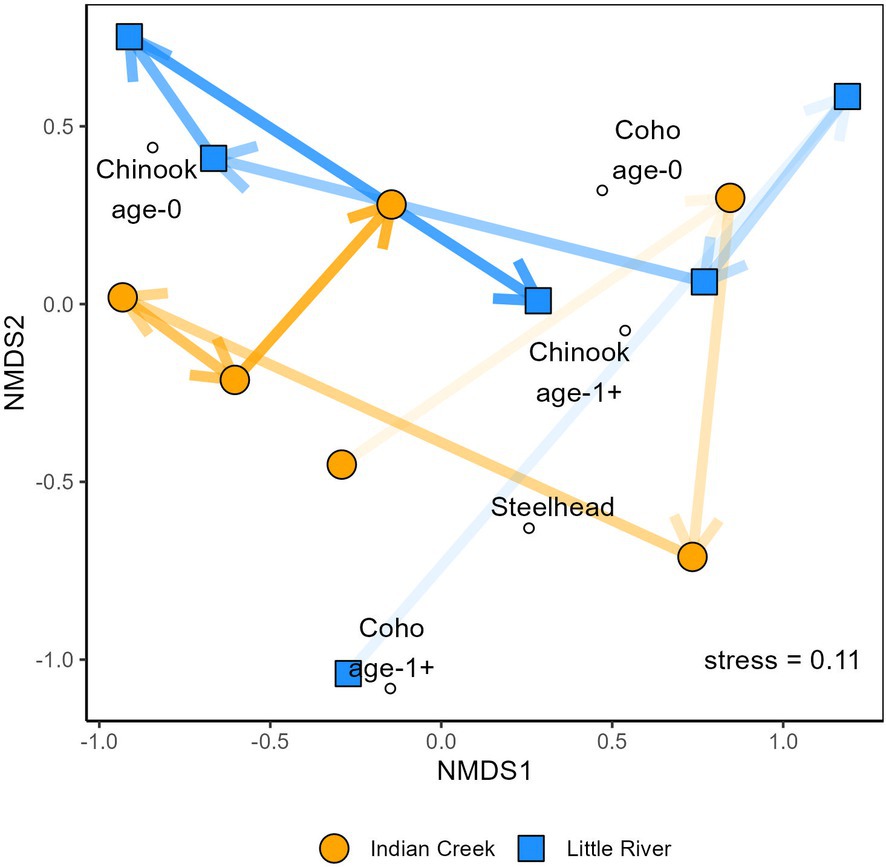
Figure 5. NMDS comparing salmonid assemblages between Indian Creek and Little River. Lines and arrows track the tributaries’ changes from 2016–2021 and darken across time.
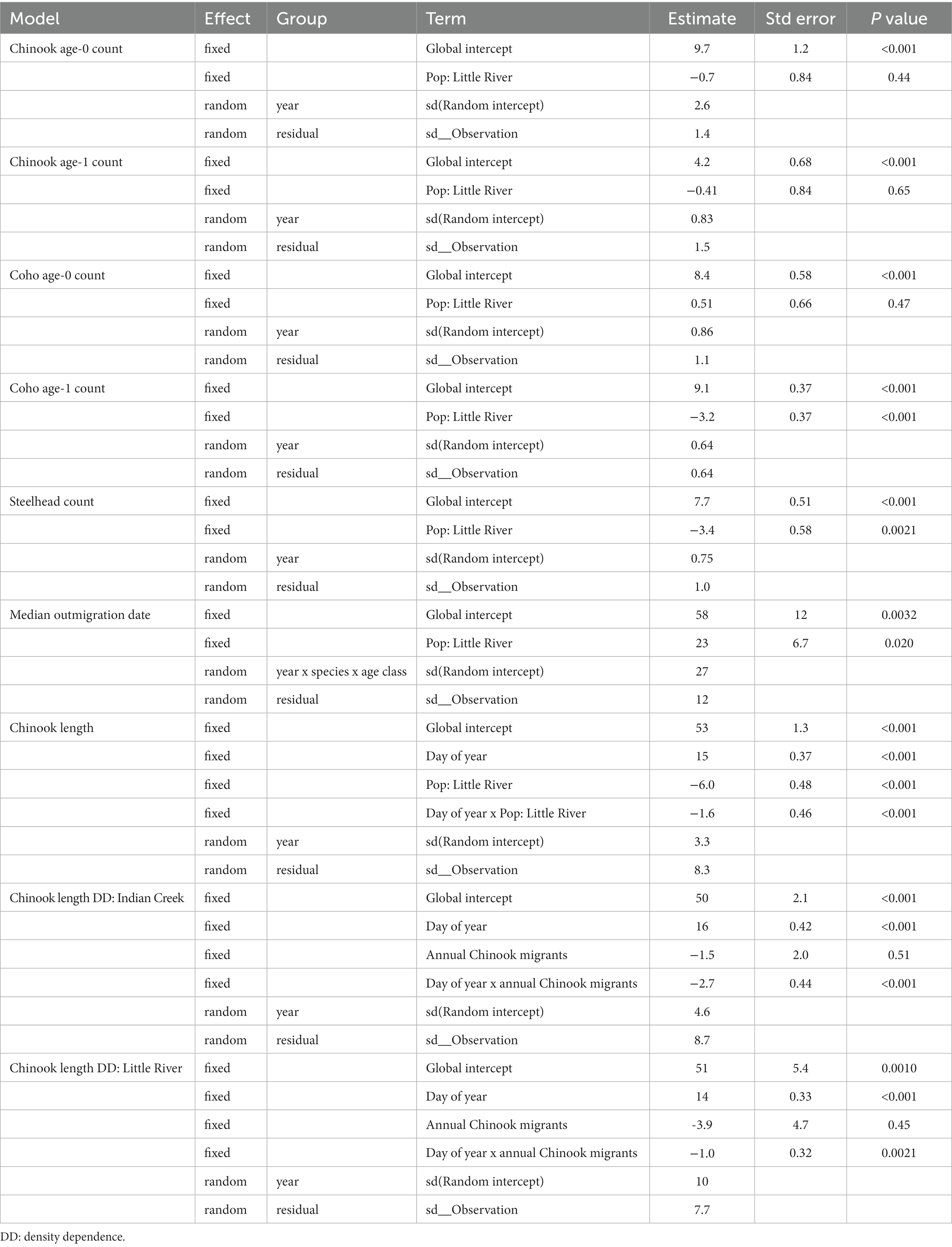
Table 1. Summary statistics of mixed effects models quantifying salmonid abundance, outmigrations timing, and length.
The tributaries’ assemblages differed significantly (Table 2, p = 0.001) but also shared significant temporal patterns (Figure 5, Table 2). Both tributaries’ assemblage trajectories generally went up and right, then down, then left, then right in NMDS space from 2016–2021 (Figure 5). Perhaps the most striking variation shared by the tributaries over time was in Chinook salmon abundances, which were relatively high in 2016 and 2019–2021. In addition to this, steelhead abundances generally increased over time while coho salmon dominated assemblages in 2017 and 2018. Finally, both tributaries supported greater total salmonid abundances in each year of 2019–2021 than each year of 2016–2018.
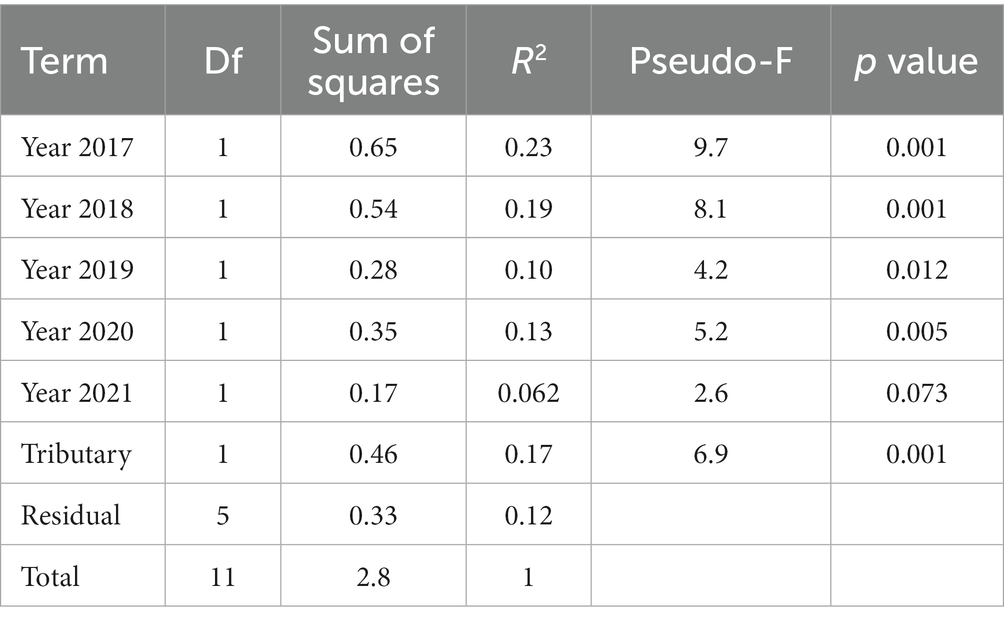
Table 2. Summary statistics of PERMANOVA comparing salmonid assemblages between tributaries and among years.
Salmonids outmigrated ~23 days earlier from Indian Creek than Little River (Figure 6, Table 1, p = 0.020). Notably, monitoring in Indian Creek in some years appeared to begin after annual outmigrations had begun, suggesting the difference in median outmigration dates between the tributaries was likely an underestimate. For all species combined, the outmigration timing was also more protracted in Indian Creek compared to a more pulsed and shorter migration period in Little River, which was often due to its abundance of later-migrating coho salmon.
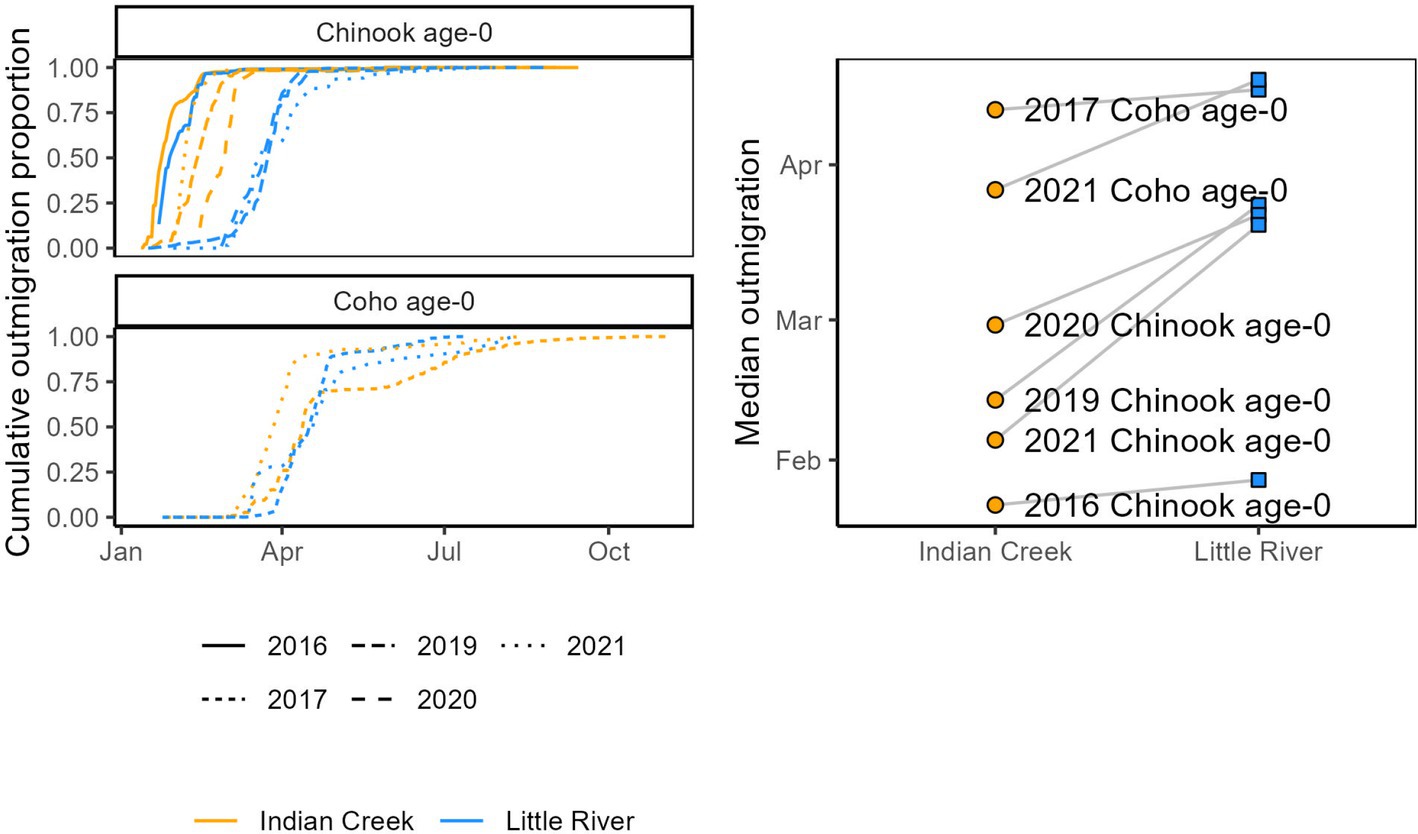
Figure 6. Salmonid outmigration timing compared between Indian Creek and Little River. Left: cumulative outmigrations as a proportion of total outmigrations. Right: median outmigration date, with dashed lines connecting observations of the same salmonid type and year.
Age at outmigration also varied between tributaries. Both tributaries supported multiple outmigrant age classes, but Indian Creek supported proportionally older (or markedly larger—see Discussion) coho salmon and steelhead (Figure 7). Ages (or possibly only sizes, in the case of larger individuals) of coho salmon were especially different, with Little River supporting predominantly age-0 outmigrants whereas age-0 outmigrants comprised only ~50% of outmigrants in Indian Creek.
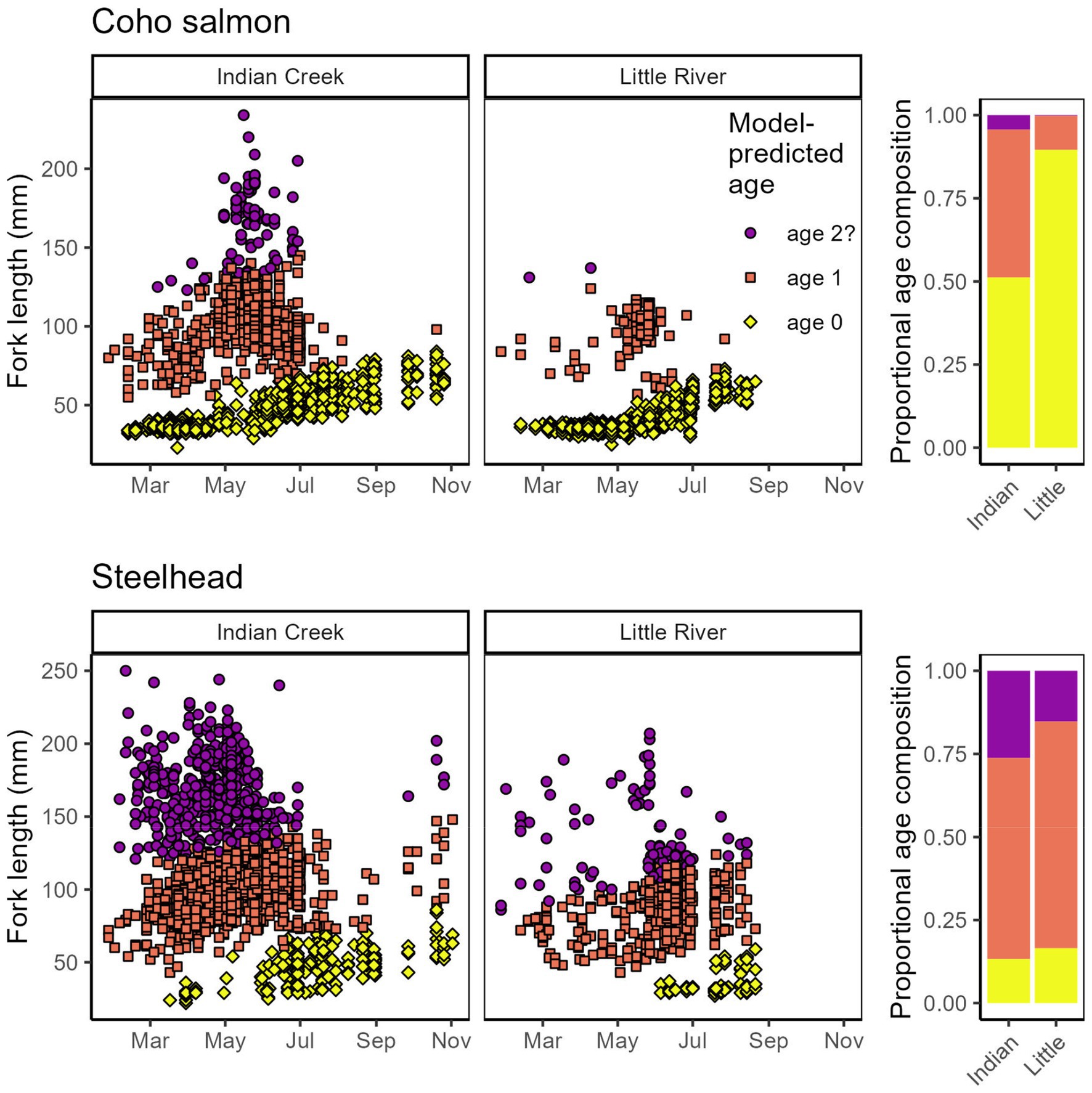
Figure 7. Model predicted ages of coho salmon and steelhead compared between tributaries. (Chinook salmon omitted because of their negligible juvenile age structure).
Some salmonid lengths differed between tributaries and were constrained by competition. Lengths of coho salmon (tributary parameter 95% CI posteriors: −0.57–0.55; Supplementary material) and steelhead (−0.83−0.71; Supplementary material) were not detectably different between tributaries. Notably, the complex age structure and multimodal length distributions of these species may have made differences between tributaries harder to detect. Chinook salmon, however, were significantly smaller in Little River than Indian Creek (p < 0.001; Table 1) and these differences widened as winter progressed through summer (p < 0.001; Table 1) (Figure 8).
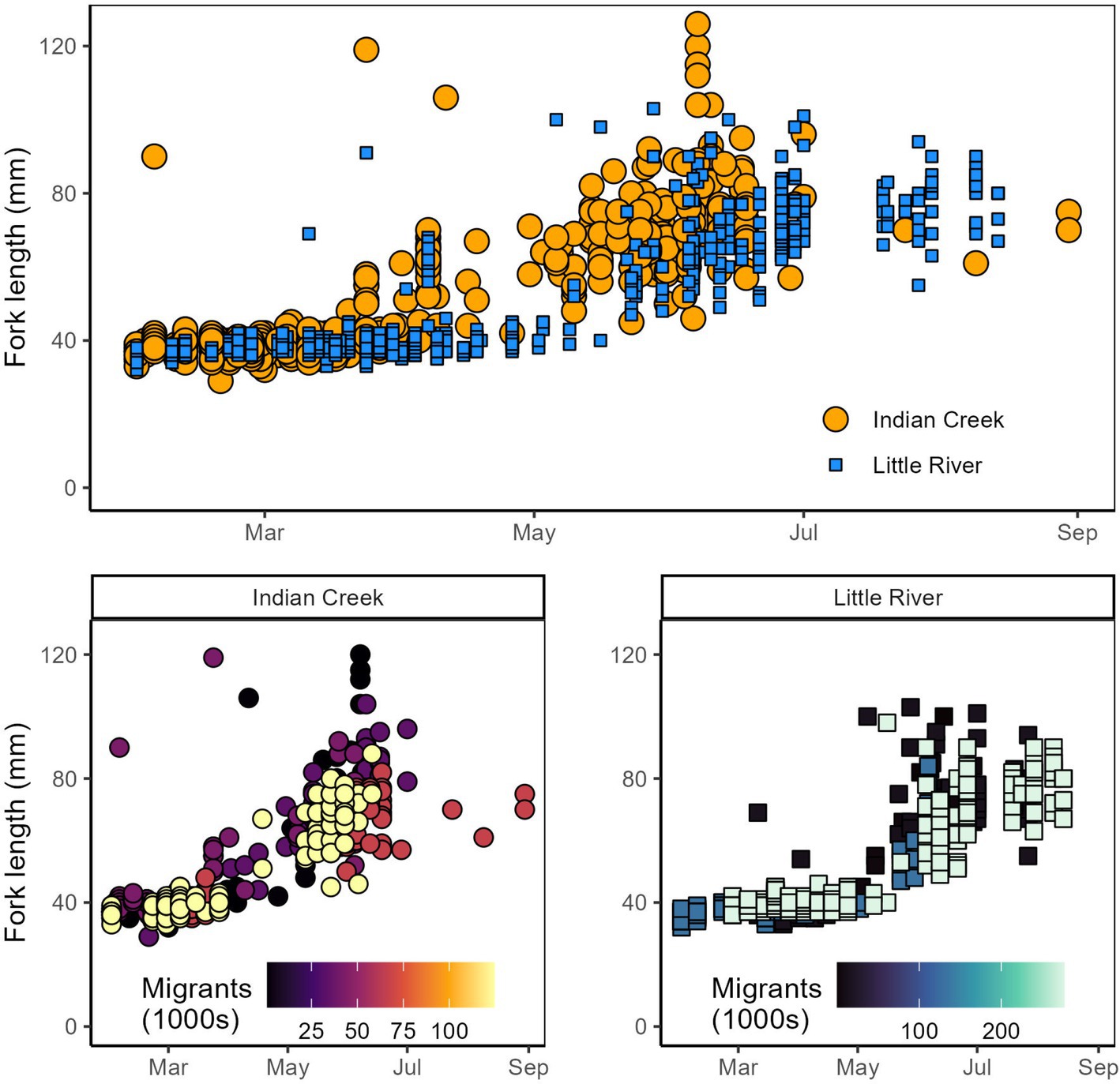
Figure 8. Top: Chinook salmon lengths compared between Indian Creek and Little River. Points describing Indian Creek fish lengths are larger and plotted behind Little River to improve visual comparison between tributaries. Bottom: Chinook salmon lengths compared within tributaries among years with different abundances of Chinook salmon outmigrants.
The model detecting this relationship indicated that salmon were 6 mm smaller in Little River on April 5, the average day of year that Chinook salmon were measured. Effects of competition on salmon length (i.e., density dependence) were also evident in Chinook salmon. Specifically, Chinook salmon in both tributaries were smaller later in the year during years when tributaries’ total Chinook outmigrants were greater (Figure 8, bottom). There was statistical evidence for density-dependent effects on growth in Indian Creek (p < 0.001; Table 1) and Little River (p = 0.0021; Table 1), with effect size being greater in Indian Creek than Little River (parameter estimate: −2.7 vs. −1.0; Table 1). Visual patterns suggested that density dependent effects on length were particularly apparent during May–July (Figure 8, bottom).
Chinook salmon redd counts generally increased across the study period, with especially high counts in 2018 and 2019 and greater counts in Indian Creek than Little River in 2016–2021 (Figure 9). For context, from 2014 to 2016, recruitment of Chinook salmon into Little River was impacted by the position of the river. During that time, the channel was on the west side of the valley and Little River flowed across a broad gravel bar, which restricted access to the relatively large Chinook salmon spawners. Notably, high Chinook salmon redd counts in 2018 and 2019 were followed by higher juvenile Chinook abundances in 2019 and 2020 (Figure 2).
Discussion
We quantified the demographics of juvenile salmonids outmigrating from a heterogeneous landscape made accessible by dam removal. The tributaries supported different species assemblages, outmigration timings, age structures, and population-level growth trajectories. Specifically, the warmer tributary produced salmonids that outmigrated ~23 days earlier, more age-1 coho salmon, more steelhead, some notably large or old coho salmon, and larger Chinook salmon given the date. Additionally, species composition and abundance varied substantially among years and between streams. Moreover, the colder, steeper tributary generally produced fewer juveniles, except in 2020 when abundances were dramatically higher in the colder, steeper tributary than in other years and in 2018 when abundances were approximately equal between tributaries. The patterns we observed were potentially due to multiple processes: (a) different stream gradients and temperature regimes favoring different species, (b) warmer temperature regimes accelerating incubation and growth, thus shifting seasonal outmigration timing forward, (c) stochastic, patchy adult recruitment and juvenile production during early phases of salmon repopulating the landscape, and (d) hatcheries sustaining baseline abundances of domesticated Chinook salmon that are not locally-adapted to the tributaries and happen to perform better in one than the other. Overall, restoring connectivity to tributaries with different characteristics rapidly enabled species to express diverse life histories. Such biological diversity is known to emerge from diverse habitat mosaics and promote resilience (e.g., Schindler et al., 2010; Lisi et al., 2013).
Temperature, stream gradient, and lake presence are fundamental to salmonid habitat mosaics, vary across landscapes, and likely drove some differences in demographics between the tributaries. High abundances of coho salmon being produced by a warmer, low-gradient tributary with lake access and ample beaver wetlands was consistent with this species’ known habitat preferences (Bisson et al., 1988; Bugert and Bjornn, 1991). Additionally, temperature regimes vary across landscapes and influence all phases of salmonid life histories (e.g., Brett et al., 1969; Richter and Kolmes, 2005; Lisi et al., 2013; FitzGerald et al., 2021). Our estimate that salmonids outmigrate ~23 days earlier in the warmer tributary extends similar findings on coho fry (Liermann et al., 2017) to Chinook salmon in these systems. Warm or increasing temperatures can cue earlier migrations in juvenile salmonids (Spence and Dick, 2014 and references therein), which is consistent with our observations of earlier migrations in warmer Indian Creek. In warmer waters that accelerate metabolism, juveniles can incubate and – given sufficient food – grow faster, which may enable them to reach outmigration size thresholds earlier in warmer environments (Brett et al., 1969, Murray and McPhail, 1988, Peven et al., 1994, Cline et al., 2019). Put together, warmer waters and presumably sufficient prey may have enabled Chinook salmon inhabiting Indian Creek to grow faster, promoting earlier outmigration. Interestingly, salmonids in warmer Indian Creek included greater proportions of older (age-1+) coho salmon. Plausibly, threshold lengths may determine outmgiration timing more directly in individuals genetically predisposed to enter the sea at age-0 while migration timing in individuals predisposed to rear for a year depends less on individual length and thus temperature regime (unfortunately ago-1 coho were not abundant enough in both tributaries during the same years to robustly compare outmigration timings between them). Moreover, mosaics of habitat types, and the associated differences in the environmental conditions such as water temperature, depth, and velocity, can affect the life history diversity and age structure of coho salmon populations (Jones et al., 2021; Sethi et al., 2021). Coho salmon with access to lake environments (e.g., Lake Sutherland connected to Indian Creek) in higher latitude watersheds can utilize both lentic and lotic habitats, resulting in differences in life history, age structure, and freshwater migration patterns (Sethi et al., 2017, 2021, 2022). Conversely, there can be differences in life history and size at migration (yearling vs. parr, vs. fry migrants) and associated age structure (age 0 vs. age 1 – spring, summer, or fall/winter) in systems that have freshwater, estuarine, and ocean habitats connected or disconnected (Jones et al., 2021). Overall, the distinct environments in Indian Creek and Little River appear to have driven distinct life histories in juvenile salmonids.
We observed greater abundances of steelhead in Indian Creek than Little River, despite the latter’s steeper, colder environment. While steelhead generally predominate among species in higher gradient habitats (McMillan et al., 2013), this may reflect their evolutionary ability to hold in faster currents (Bisson et al., 1988) and their comparatively broad spatial distribution within a watershed (McMillan et al., 2013) rather than a tendency to avoid warmer, lower gradient areas. Indeed, steelhead as a species can certainly tolerate rearing areas that are warm for salmonids (Richter and Kolmes 2005; Sloat and Osterback 2013) because they have evolved a scope for activity that is maximized at relatively warmer temperatures (Dickson and Kramer 1971). And, as outlined above, warmer streams within tolerable temperature ranges offer greater potential for growth, given adequate prey availability. Additionally, Indian Creek is larger and therefore presumably provides greater habitat capacity and its lake head dampens flow variation that can cause scour and flooding. For these reasons, Indian Creek’s environment may be more conducive to steelhead production than Little River.
In addition to landscapes generating diversity, some assemblage patterns among years and between tributaries may be attributable to adult recruitment and hatchery processes. During this study’s timeframe, salmonids were becoming more abundant and widely distributed across the landscape (Duda et al., 2021, Pess et al., in review, this issue). Notably, Chinook salmon tend to spawn in mainstems unless high spawner abundances (and adequate flows) promote expansion into tributaries. This appeared to happen in 2018 and 2019, when Chinook salmon escapement was high (Pess et al., in review, this issue) and redd counts in Indian Creek and Little River were especially high, resulting in high juvenile abundances the following years. Indeed, some of the synchronized variation in juvenile assemblage composition between tributaries among years appeared to be attributable to variation in adult spawning the year prior. If Chinook salmon abundances continue to increase across the landscape, annual spawner distributions may more routinely expand into the tributaries, resulting in greater abundances the following years. However, an important nuance to understanding Chinook salmon in this system is that returning adults are, to date, overwhelmingly hatchery-origin and presumably descend from a hatchery lineage that originated in 1930 (Pess et al., in review, this issue). It therefore seems unlikely that juvenile Chinook salmon are locally adapted to Indian Creek or Little River. One interpretation of Chinook salmon outmigrating at smaller sizes from Little River may be that the current stock’s genetically-determined traits happen to align more with niches in Indian Creek than Little River. Over decades as natural productivity has an opportunity to eclipse hatchery productivity, it remains to be seen whether natural selection will produce locally-adapted populations that exploit localized environments and opportunities, potentially driving more divergence in life histories between tributaries.
Competition also appeared to influence juvenile lengths and interact with the tributaries’ different growth opportunities. Chinook salmon were smaller when conspecific abundances were higher, and such density dependent effects are common for salmonids in general (Grossman and Simon 2020). Notably, these effects were greater in Indian Creek, which appeared to support faster growth than Little River. That fish appeared to grow faster but experience greater density-dependent constraints on growth in Indian Creek is consistent with Indian Creek’s warmer temperatures offering greater potential for growth, but possibly also greater potential for prey limitation by increasing salmonids’ metabolic rates. Indeed, whether warmer waters within tolerable ranges promote growth depends on the interaction of temperature, prey availability, and competition (Crozier et al., 2010). While examining for the effects of competition on coho salmon and steelhead was beyond the scope of our study because of their more complex residence times, similar dynamics may influence growth in these species as well.
Many of the assemblage changes among years and between tributaries were unpredictable and yet appeared to offset one another, underscoring the concept that ecosystems are often stabilized by diverse options (Brennan et al., 2019; Munsch et al., 2022). Specifically, assemblages shifted back and forth among years from dominance by Chinook salmon to coho salmon and in 1 year. Little River produced a large abundance of steelhead smolts. Indeed, if the systems lacked Chinook salmon very few fish would have been present in 2016 and if they lacked coho salmon very few fish would have been present in 2017 and 2018. Because the dynamics of these three species were not synchronized among years, they generated portfolio effects whereby the abundance of the total assemblage was more stable than the more volatile abundances of individual species and age classes (Schindler et al., 2015). As is typically the case in ecology, our ability to predict or explain such fine-scale ecosystem changes will always be limited. However, we can count on stability to emerge from diverse systems on aggregate as their components spread risk and respond differentially to disturbances and opportunities (Schindler and Hilborn, 2015). Additionally, as alluded to above, this system remains in a recovery phase and its dynamics perhaps also reflect recruitment trends (i.e., generally increasing, but patchy abundances over time) and “trial and error” in natural selection contexts as populations attempt to inhabit and locally adapt to newly accessible areas. Overall, salmon production across the landscape may be stabilized by environmentally-distinct tributaries enabling salmon to stagger their life cycles across time and space, attributes that enable systems to spread risk and take advantage of unpredictable opportunities (Schindler et al., 2015).
Our findings are relevant to other attempts to increase salmonid diversity via habitat reintroduction. For example, preliminary observations suggest that the removal of San Clemente Dam (CA, United States) has enabled a diversity of steelhead (and perhaps rainbow trout) size classes to repopulate the landscape. Researchers attribute this biological diversity similarly as in our study to the diversity of newly-accessible habitats (Williams et al., 2018). Additionally, an upcoming project removing dams on the Klamath River will constitute the largest dam removal to date. This project will target the recovery of distinct Chinook salmon life histories that return in the spring and fall to different areas within the watershed (Oregon Department of Fish and Wildlife and the Klamath Tribes, 2021). The Klamath River watershed supports a remarkably heterogeneous landscape of stream temperatures and stream gradients that could give rise to life history diversity similar to that observed in our study. Additionally, preliminary work has investigated the feasibility of reintroducing steelhead and early-migrating Chinook salmon to tributaries of the San Joaquin River and Eel River (CA, United States; Boughton et al., 2022; FitzGerald et al., 2022). Provided that the diversity of unlocked habitats in these systems are within the thermal tolerance of their salmonids’ life stages, there is no obvious reason why diverse juvenile life histories would not emerge from these systems as happened in our study.
Similarly, we may expect the different environmental conditions that are distributed across the Elwha River system to generate juvenile life history beyond the tributaries we studied. The Elwha River system consists of hundreds of anadromous stream kilometers and thousands of overall kilometers that are nested across its environmentally heterogeneous landscape (Munn et al., 1999, Pess et al. in review, this issue). Its large elevation range, coupled with its variation in confined and alluvial valley bottoms, allows for considerable variation across space in conditions that determine habitat characteristics. Notably, high elevations experience quadruple the precipitation of lower elevations, experience a greater proportion of precipitation as snow, and are cooler (Munn et al., 1999). Furthermore, an array of habitat types such as high-gradient streams and floodplains are distributed across this template (Munn et al., 1999). Based on our observations in Indian Creek and Little River and a fundamental understanding that salmonid life history is linked to environmental conditions that vary across landscapes, we may expect life history diversity to be emerging across the diversity of many newly-accessible habitats in the Elwha River system.
Our mixture models that assigned fish to age classes include uncertainty that should be considered in the interpretation of our results. Our mixture models intended to parse out three age classes by leveraging a priori knowledge that these three age classes are often present in juvenile salmonid habitats, especially in the case of steelhead (Quinn, 2018), and will create three different length distributions after accounting for various covariates (i.e., day of year, tributary, or year). Importantly, age assignments were probabilistic, meaning we can make some of these assignments more confidently than others, but for simplicity we presented age composition based on the most likely outcome for each fish. Thus, the proportion of fish assigned to each age class includes uncertainty and more important than the absolute proportions of age classes is the qualitative pattern that Indian Creek was inhabited by proportionally more larger and older individuals, which is corroborated by visually comparing length at date values between tributaries (Figure 7). Additionally, the complex age structure of coho salmon and steelhead may impair model detection of differences in length at date of these species between Indian Creek and Little River, relative to the model used to examine Chinook salmon length. That is, coho salmon and steelhead may have differed in length at date between the two tributaries, but we may have been less able to detect these differences due to the more complicated data arising from their diverse age structure. Finally, without direct age sampling (e.g., examining scales) and given that coho are not known to outmigrate at older ages as often do steelhead (Hodge et al., 2016; Quinn, 2018), we cannot confidently interpret whether the largest coho salmon in Indian Creek were age-2 or simply large age-1 individuals. Instead, we note that Indian Creek’s coho salmon included many uniquely large individuals, which may reflect tributary-specific rearing durations or growth opportunities and contributed to differences in life histories between the two tributaries. Directly measuring the age of juveniles repopulating environmentally diverse tributaries following dam removal in this river system and others would be a natural extension of this study.
To conclude, diverse landscapes enable diverse life histories that promote resilience (Hilborn et al., 2003; Schindler et al., 2010; Hodge et al., 2016; Brennan et al., 2019), but human stressors that simplify and degrade landscapes suppress life history diversity and erode resilience (Munsch et al., 2022). Dams and other modes of habitat fragmentation contribute substantially to lost life history diversity (e.g., Yoshiyama et al., 1998), prompting efforts to restore diversity via dam removal. Our study—which addressed a knowledge gap regarding responses in juvenile life stages—and others (e.g., Quinn et al., 2017; Williams et al., 2018; Duda et al., 2021) demonstrate that dam removal can enable salmonids to repopulate heterogeneous landscapes and express local differences in their relative species abundance, outmigration timing, age classes, and growth rates, thus enabling landscapes’ natural capacities to express diverse life histories. By actualizing diverse, complex systems that spread risk (Schindler et al., 2015), dam removal may promote resilience in salmonids and other species during an era of rapid and uncertain environmental change.
Data availability statement
The raw data supporting the conclusions of this article will be made available by the authors, without undue reservation.
Ethics statement
The animal study was reviewed and approved by Washington State (Washington State Scientific Collection Permit), National Park Service (Scientific Research and Collecting Permit), U.S. Fish and Wildlife Service (Native Threatened Specific-Recovery), and NOAA-NMFS (take permit).
Author contributions
SM: led writing, analyses, fieldwork, ideas, and revisions. MM and GP: design, fieldwork, project supervision, ideas, and revisions. ML: analyses, fieldwork, ideas, and revisions. TB: fieldwork, ideas, and revisions. JM: ideas and revisions. RM: design, fieldwork, ideas, and revisions. All authors contributed to the article and approved the submitted version.
Funding
Funding was provided to the Lower Elwha Klallam Tribe by the Environmental Protection Agency/Puget Sound Partnership through the Northwest Indian Fisheries Commission (contract no. PA-01J64601-1). Funding was provided by the Lower Elwha Klallam Tribe to the National Oceanic and Atmospheric Administration – Northwest Fisheries Science Center for the contributions of George Pess, Todd Bennett, John McMillan, and Martin Liermann (contract no. PA-01J64601-1).
Acknowledgments
We thank Vanessa Castle, Mel Elofson, Allyce Miller, Ernest Sampson, Justin Stapleton, and mcKenzie Taylor for fieldwork, and Joshua W. Chamberlin, Sarah A. Morley, two reviewers, and handling editor J-MR for critiques that improved our manuscript. We also thank John Mahan for alerting us to an error in abundance values in a previous version of the article.
Conflict of interest
SM is employed by Ocean Associates Inc.
The remaining authors declare that the research was conducted in the absence of any commercial or financial relationships that could be construed as a potential conflict of interest.
Publisher’s note
All claims expressed in this article are solely those of the authors and do not necessarily represent those of their affiliated organizations, or those of the publisher, the editors and the reviewers. Any product that may be evaluated in this article, or claim that may be made by its manufacturer, is not guaranteed or endorsed by the publisher.
Supplementary material
The Supplementary material for this article can be found online at: https://www.frontiersin.org/articles/10.3389/fevo.2023.1188921/full#supplementary-material
References
Anderson, M. J. (2001). A new method for non-parametric multivariate analysis of variance. Austral. Ecol. 26, 32–46. doi: 10.1111/j.1442-9993.2001.01070.pp.x
Beechie, T., Imaki, H., Greene, J., Wade, A., Wu, H., Pess, G., et al. (2013). Restoring salmon habitat for a changing climate. River Res. Appl. 29, 939–960. doi: 10.1002/rra.2590
Bisson, P. A., Sullivan, K., and Nielsen, J. L. (1988). Channel hydraulics, habitat use, and body form of juvenile coho salmon, steelhead, and cutthroat trout in streams. Trans. Am. Fish. Soc. 117, 262–273. doi: 10.1577/1548-8659(1988)117<0262:CHHUAB>2.3.CO;2
Bjornn, T. C., Craddock, D. R., and Corley, D. R. (1968). Migration and survival of redfish Lake, Idaho, sockeye salmon, Oncorhynchus nerka. Trans. Am. Fish. Soc. 97, 360–373. doi: 10.1577/1548-8659(1968)97[360:MASORL]2.0.CO;2
Bolker, B. M., Brooks, M. E., Clark, C. J., Geange, S. W., Poulsen, J. R., Stevens, M. H. H., et al. (2009). Generalized linear mixed models: a practical guide for ecology and evolution. Trends Ecol. Evol. 24, 127–135. doi: 10.1016/j.tree.2008.10.008
Boughton, D. A., Harrison, L. R., John, S. N., Bond, R. M., Nicol, C. L., Legleiter, C. J., et al. (2022). Capacity of two Sierra Nevada Rivers for reintroduction of anadromous Salmonids: insights from a high-resolution view. Trans. Am. Fish. Soc. 151, 13–41. doi: 10.1002/tafs.10334
Brenkman, S. J., Peters, R. J., Tabor, R. A., Geffre, J. J., and Sutton, K. T. (2019). Rapid recolonization and life history responses of bull trout following dam removal in Washington's Elwha River. N. Am. J. Fish Manag. 39, 560–573. doi: 10.1002/nafm.10291
Brennan, S. R., Schindler, D. E., Cline, T. J., Walsworth, T. E., Buck, G., and Fernandez, D. P. (2019). Shifting habitat mosaics and fish production across river basins. Science 364, 783–786. doi: 10.1126/science.aav4313
Brett, J. R., Shelbourn, J. E., and Shoop, C. T. (1969). Growth rate and body composition of fingerling sockeye salmon, Oncorhynchus nerka, in relation to temperature and ration size. J. Fish. Board Can. 26, 2363–2394. doi: 10.1139/f69-230
Bugert, R. M., and Bjornn, T. C. (1991). Habitat use by steelhead and coho salmon and their responses to predators and cover in laboratory streams. Trans. Am. Fish. Soc. 120, 486–493. doi: 10.1577/1548-8659(1991)120<0486:HUBSAC>2.3.CO;2
Busby, P. J., Wainwright, T. C., Bryant, G. J., Lierheimer, L. J., Waples, R. S., Waknitz, F. W., et al. (1996). Status review of west coast steelhead from Washington, Idaho, Oregon, and California.
Carlson, S. M., and Satterthwaite, W. H. (2011). Weakened portfolio effect in a collapsed salmon population complex. Can. J. Fish. Aquat. Sci. 68, 1579–1589. doi: 10.1139/f2011-084
Cline, T. J., Ohlberger, J., and Schindler, D. E. (2019). Effects of warming climate and competition in the ocean for life-histories of Pacific salmon. Nat. Ecol. Evol. 3, 935–942. doi: 10.1038/s41559-019-0901-7
Crozier, L. G., Zabel, R. W., Hockersmith, E. E., and Achord, S. (2010). Interacting effects of density and temperature on body size in multiple populations of Chinook salmon. Journal of Animal Ecology 79, 342–349.
Dickson, I. W., and Kramer, R. H. (1971). Factors influencing scope for activity and active and standard metabolism of rainbow trout (Salmo gairdneri). Journal of the Fisheries Board of Canada 28, 587–596. doi: 10.1111/eff.12523
Duda, J. J., Torgersen, C. E., Brenkman, S. J., Peters, R. J., Sutton, K. T., Connor, H. A., et al. (2021). Reconnecting the Elwha River: spatial patterns of fish response to dam removal. Front. Ecol. Evol. 9:765488. doi: 10.3389/fevo.2021.765488
FitzGerald, A. M., Boughton, D. A., Fuller, J., John, S. N., Martin, B. T., Harrison, L. R., et al. (2022). Physical and biological constraints on the capacity for life-history expression of anadromous salmonids: an Eel River, California, case study. Can. J. Fish. Aquat. Sci. 79, 1023–1041. doi: 10.1139/cjfas-2021-0229
FitzGerald, A. M., John, S. N., Apgar, T. M., Mantua, N. J., and Martin, B. T. (2021). Quantifying thermal exposure for migratory riverine species: phenology of Chinook salmon populations predicts thermal stress. Glob. Chang. Biol. 27, 536–549. doi: 10.1111/gcb.15450
Fraik, A. K., McMillan, J. R., Liermann, M., Bennett, T., McHenry, M. L., McKinney, G. J., et al. (2021). The impacts of dam construction and removal on the genetics of recovering steelhead (Oncorhynchus mykiss) populations across the Elwha River watershed. Gene 12:89. doi: 10.3390/genes12010089
Greene, C. M., Hall, J. E., Guilbault, K. R., and Quinn, T. P. (2010). Improved viability of populations with diverse life-history portfolios. Biol. Lett. 6, 382–386. doi: 10.1098/rsbl.2009.0780
Grossman, G. D., and Simon, T. N. (2020). Density-dependent effects on salmonid populations: a review. Ecol. Freshw. Fish 29, 400–418. doi: 10.1111/eff.12523
Guarino, J. (2013). Tribal advocacy and the art of dam removal: the lower Elwha Klallam and the Elwha dams. Am. Ind. Law J. 2, 114–145.
Hawkins, B. L., Fullerton, A. H., Sanderson, B. L., and Steel, E. A. (2020). Individual-based simulations suggest mixed impacts of warmer temperatures and a nonnative predator on Chinook salmon. Ecosphere 11:e03218. doi: 10.1002/ecs2.3218
Hilborn, R., Quinn, T. P., Schindler, D. E., and Rogers, D. E. (2003). Biocomplexity and fisheries sustainability. Proc. Natl. Acad. Sci. U. S. A. 100, 6564–6568. doi: 10.1073/pnas.1037274100
Hodge, B. W., Wilzbach, M. A., Duffy, W. G., Quiñones, R. M., and Hobbs, J. A. (2016). Life history diversity in Klamath River steelhead. Trans. Am. Fish. Soc. 145, 227–238. doi: 10.1080/00028487.2015.1111257
Jones, K. K., Cornwell, T. J., Bottom, D. L., Stein, S., and Starcevich, S. (2021). Interannual variability in life-stage specific survival and life history diversity of coho salmon in a coastal Oregon basin. Can. J. Fish. Aquat. Sci. 78, 1887–1899. doi: 10.1139/cjfas-2020-0306
Levin, S. A. (1992). The problem of pattern and scale in ecology: the Robert H MacArthur award lecture. Ecology 73, 1943–1967.
Liermann, M., Pess, G., McHenry, M., McMillan, J., Elofson, M., Bennett, T., et al. (2017). Relocation and recolonization of coho salmon in two tributaries to the Elwha River: implications for management and monitoring. Trans. Am. Fish. Soc. 146, 955–966. doi: 10.1080/00028487.2017.1317664
Lisi, P. J., Schindler, D. E., Bentley, K. T., and Pess, G. R. (2013). Association between geomorphic attributes of watersheds, water temperature, and salmon spawn timing in Alaskan streams. Geomorphology 185, 78–86. doi: 10.1016/j.geomorph.2012.12.013
McHenry, M., McMillan, J., Moses, R., and Pess, G. (2022). Coho salmon relocations and redd surveys in the Elwha River 2011–2021: Summary report provided to Olympic National Park by the lower Elwha Klallam tribe as part of the final settlement for Elwha monitoring.
McMillan, J. R., Liermann, M. C., Starr, J., Pess, G. R., and Augerot, X. (2013). Using a stream network census of fish and habitat to assess models of juvenile salmonid distribution. Transactions of the American Fisheries Society 142, 942–956.
Munn, M., Black, D., Haggland, R.W., Hummling, M. A. A. L., and Huffman, R. L. (1999). An assessment of stream habitat and nutrients in the Elwha River basin: Implications for restoration. Report 98–4223. US Department of the Interior, US Geological Survey.
Munsch, S. H., Greene, C. M., Mantua, N. J., and Satterthwaite, W. H. (2022). One hundred-seventy years of stressors erode salmon fishery climate resilience in California’s warming landscape. Glob. Chang. Biol. 28, 2183–2201. doi: 10.1111/gcb.16029
Murray, C. B., and McPhail, J. D. (1988). Effect of incubation temperature on the development of five species of Pacific salmon (Oncorhynchus) embryos and alevins. Can. J. Zool. 66, 266–273. doi: 10.1139/z88-038
Nehlsen, W., Williams, J. E., and Lichatowich, J. A. (1991). Pacific salmon at the crossroads: stocks at risk from California, Oregon, Idaho, and Washington. Fisheries 16, 4–21. doi: 10.1577/1548-8446(1991)016<0004:PSATCS>2.0.CO;2
Oregon Department of Fish and Wildlife and the Klamath Tribes (2021). Implementation plan for the reintroduction of anadromous fishes into the Oregon portion of the upper Klamath Basin. (eds.) M.E. Hereford, T.G. Wise, and A. Gonyaw.
Pess, G. R., Quinn, T. P., Gephard, S. R., and Saunders, R. (2014). Re-colonization of Atlantic and Pacific rivers by anadromous fishes: linkages between life history and the benefits of barrier removal. Rev. Fish Biol. Fish. 24, 881–900. doi: 10.1007/s11160-013-9339-1
Peven, C. M., Whitney, R. R., and Williams, K. R. (1994). Age and length of steelhead smolts from the mid-Columbia River basin, Washington. N. Am. J. Fish Manag. 14, 77–86. doi: 10.1577/1548-8675(1994)014<0077:AALOSS>2.3.CO;2
Quinn, T. P. (2018). The Behavior and Ecology of Pacific Salmon and Trout, Seattle, Washington, USA: University of Washington Press.
Quinn, T. P., Bond, M. H., Brenkman, S. J., Paradis, R., and Peters, R. J. (2017). Re-awakening dormant life history variation: stable isotopes indicate anadromy in bull trout following dam removal on the Elwha River, Washington. Environ. Biol. Fish 100, 1659–1671. doi: 10.1007/s10641-017-0676-0
Richter, A., and Kolmes, S. A. (2005). Maximum temperature limits for Chinook, coho, and chum salmon, and steelhead in the Pacific northwest. Rev. Fish. Sci. 13, 23–49. doi: 10.1080/10641260590885861
Roper, B. B., and Scarnecchia, D. L. (1999). Emigration of age-0 Chinook salmon (Oncorhynchus tshawytscha) smolts from the upper South Umpqua River basin, Oregon, USA. Can. J. Fish. Aquat. Sci. 56, 939–946. doi: 10.1139/f99-023
Schindler, D. E., Armstrong, J. B., and Reed, T. E. (2015). The portfolio concept in ecology and evolution. Front. Ecol. Environ. 13, 257–263. doi: 10.1890/140275
Schindler, D. E., and Hilborn, R. (2015). Prediction, precaution, and policy under global change. Science 347, 953–954. doi: 10.1126/science.1261824
Schindler, D. E., Hilborn, R., Chasco, B., Boatright, C. P., Quinn, T. P., Rogers, L. A., et al. (2010). Population diversity and the portfolio effect in an exploited species. Nature 465, 609–612. doi: 10.1038/nature09060
Sethi, S. A., Ashline, J., Harris, B. P., Gerken, J., and Restrepo, F. (2021). Connectivity between lentic and lotic freshwater habitats identified as a conservation priority for coho salmon. Aquat. Conserv. Mar. Freshwat. Ecosyst. 31, 1791–1801. doi: 10.1002/aqc.3504
Sethi, S. A., Carey, M. P., Gerken, J., Harris, B. P., Wolf, N., Cunningham, C., et al. (2022). Juvenile salmon habitat use drives variation in growth and highlights vulnerability to river fragmentation. Ecosphere 13:e4192. doi: 10.1002/ecs2.4192
Sethi, S. A., Gerken, J., and Ashline, J. (2017). Accurate aging of juvenile salmonids using fork lengths. Fish. Res. 185, 161–168. doi: 10.1016/j.fishres.2016.09.012
Sloat, M. R., and Osterback, A. M. K. (2013). Maximum stream temperature and the occurrence, abundance, and behavior of steelhead trout (Oncorhynchus mykiss) in a southern California stream. Canadian Journal of Fisheries and Aquatic Sciences 70, 64–73. doi: 10.1139/cjfas-2012-0479
Soulodre, E. M., Dhar, A., and Naeth, M. A. (2022). Plant community development trends on mixed grass prairie well sites 5 years after reclamation. Ecol. Eng. 179:106635. doi: 10.1016/j.ecoleng.2022.106635
Spence, B. C., and Dick, E. J. (2014). Geographic variation in environmental factors regulating outmigration timing of coho salmon (Oncorhynchus kisutch) smolts. Can. J. Fish. Aquat. Sci. 71, 56–69. doi: 10.1139/cjfas-2012-0479
Tilman, D., and Downing, J. A. (1994). Biodiversity and stability in grasslands. Nature 367, 363–365. doi: 10.1038/367363a0
Washington Department of Ecology (2016). Flow monitoring network. Available at: https://fortress.wa.gov/ecy/eap/flows/regions/state.asp
Williams, T. H., East, A. E., Smith, D. P., Boughton, D. A., Mantua, N., and Harrison, L. R. (2018). Removal of San Clemente dam did more than restore fish passage. Osprey 89, 1–4.
Keywords: Elwha river, habitat mosaic, restoration, thermal regime, phenology
Citation: Munsch SH, McHenry M, Liermann MC, Bennett TR, McMillan J, Moses R and Pess GR (2023) Dam removal enables diverse juvenile life histories to emerge in threatened salmonids repopulating a heterogeneous landscape. Front. Ecol. Evol. 11:1188921. doi: 10.3389/fevo.2023.1188921
Edited by:
Jean-Marc Roussel, INRAE Rennes, FranceReviewed by:
Peter J. Auster, Mystic Aquarium, United StatesSimon Blanchet, Center National de la Recherche Scientifique (CNRS), France
Copyright © 2023 Munsch, McHenry, Liermann, Bennett, McMillan, Moses and Pess. This is an open-access article distributed under the terms of the Creative Commons Attribution License (CC BY). The use, distribution or reproduction in other forums is permitted, provided the original author(s) and the copyright owner(s) are credited and that the original publication in this journal is cited, in accordance with accepted academic practice. No use, distribution or reproduction is permitted which does not comply with these terms.
*Correspondence: Stuart H. Munsch, Stuart.Munsch@NOAA.gov