- 1Department of Integrative Biology, University of California, Berkeley, Berkeley, CA, United States
- 2University and Jepson Herbaria, University of California, Berkeley, Berkeley CA, United States
- 3Departamento de Biologia, Universidade Federal do Amazonas -UFAM, Manaus, Brazil
- 4Programa de Pós-Graduação em Botânica, Instituto Nacional de Pesquisas da Amazônia, Manaus, Amazonas, Brazil
- 5Programa de Pós-Graduação em Entomologia, Instituto Nacional de Pesquisas da Amazônia, Manaus, Amazonas, Brazil
- 6Department of Biological Sciences, Binghamton University, Binghamton, NY, United States
Introduction: Plants and their insect herbivores represent a large fraction of the species in Amazonian forests and are often directly implicated in the origin and maintenance of biodiversity at local and regional scales. How these interactions may change over geographic distance is unknown because very few studies have investigated the herbivore fauna and defense chemicals of any host plant species at multiple sites in tropical forests. One hypothesis, the Geographic Mosaic Theory of Coevolution, predicts that if herbivore assemblages turn over in different parts of a plant’s range, then plant defense chemicals should also change, reflecting local selection pressures.
Methods: We tested this theory by studying 12 species of Protium (Burseraceae) trees that occur in both Iquitos, Peru, and Manaus, Brazil, in rainforests separated by 1500 km. We surveyed all insects observed directly feeding on the plants in both locations for 48 weeks in Manaus and 64 weeks in Iquitos. We analyzed the secondary metabolites in the leaves of all species in both locations using GC/MS and HPLC.
Results and Discussion: Although in both locations we found that Protium herbivores were dominated by insects from the orders Hemiptera, Coleoptera and Lepidoptera, we found almost complete turnover in the herbivore species composition in the two sites, and each host plant species had a different assemblage of herbivores in each location. Comparing the phylogenetic beta-diversity, we found low similarity in herbivore phylogenetic relatedness between host plant species in the two locations. However, the secondary metabolites found within a Protium species were similar across the two locations. We found no strong evidence that individuals from a host plant species in Iquitos or Manaus expressed locally-adapted defense chemicals, as individuals from geographic locations did not form clusters when looking at patterns of chemical similarity. These results are not consistent with the Geographic Mosaic Theory of Coevolution. The most intriguing pattern we found was a strong correlation between the diversity of herbivores per host plant species in both locations. We also found that plants with high chemical richness had lower numbers of herbivore species and numbers of total herbivores in both locations. We conclude that high chemical diversity is the most effective strategy for Protium trees to reduce insect herbivore attacks. We speculate that each secondary metabolite is effective at repelling only a few insect herbivores, and that different chemicals are likely effective in different parts of a plants’ geographic range. Future studies should investigate additional locations and additional natural enemies (i.e., fungal pathogens) to test the hypothesis that chemical diversity reduces attack from natural enemies and may explain the ecological and evolutionary success of rainforest trees over time and space.
Introduction
Natural enemies have been implicated in the origin of plant diversity, especially in hyperdiverse tropical forests where the species richness of plants (and their enemies) reaches its apex (Price, 2002). Insects are among the most important enemies of tropical tree species (Coley and Barone, 1996), and of those, caterpillars, beetles, and hemipterans have consistently been reported as the most common and diverse herbivores in tropical systems (Barone, 1998, Basset, 1999, Novotny et al., 2005; Novotny et al., 2006; Novotny et al., 2007; Sam et al. 2020, Richards et al., 2015; Salazar et al., 2018).
In turn, plants have evolved a suite of different chemical defenses to repel attack from insects, with each one of these specialized (i.e., secondary) metabolites having different efficacy against different kinds of herbivores (Volf et al., 2015; Salazar et al., 2018). Across major insect clades, large physiological and metabolic differences in taxa can affect the mechanisms of action of specialized metabolites. For example, caterpillar guts have very high pH compared to beetles, and thus the oxidative biological activity of tannins can differ significantly between these two taxa (Barbehenn and Constable, 2011). The effects of specific tannins on herbivores can range widely; from causing gut lesions, reducing overall nutrition, or sometimes having beneficial effects (Barbehenn and Constabel, 2011). Within herbivore groups, the evolution of specialization can allow some species to better overcome or circumvent plant host chemical defenses (Volf et al., 2015). Given the variation in specialized metabolite susceptibility across herbivores, plants would be expected to evolve chemical arsenals that match a particular biological context and herbivore community. In particular, plant species with wide geographical ranges are likely to be challenged by an equally-wide range of biological contexts, with significant changes in herbivore abundance and compositions across their range. Thus, it is reasonable to expect that, if two widely separated populations of plants contained different assemblages of associated herbivores, this may select for different concentrations (or different qualitative mixtures) of different defensive specialized metabolites (Volf et al., 2015).
Such coevolutionary interactions among plants and their enemies in tropical forests could lead to speciation when plants experienced different combinations of enemies in different parts of their range (Schemske, 2009). The Geographic Mosaic Theory of Coevolution (GMTC) posits that over an organism’s geographic range it is likely that it will be involved in complex coevolutionary relationships with a multitude of interacting partners (both mutualistic and antagonistic), and such biotic interactions will vary in strength among geographically-separated subpopulations, creating locally-adapted phenotypes (Thompson, 2005). In terms of plant defense and natural enemies, GMTC predicts that one would find natural variation in chemical defenses (qualitative and/or quantitative) in plant populations exposed to significantly different qualitative and quantitative herbivore pressures (Thompson, 2005; Salazar and Marquis, 2012), depending on the beta-diversity of natural enemies across a host plant’s range (Leimu et al., 2012).
To test this herbivore-chemistry GMTC, ideally one would evaluate defense chemistry and natural enemies attacking the same species of host plants at different locations throughout their geographic range (at similar elevations, habitats and latitudes to control for the effect on environmental changes and other confounding factors). To date, only a few studies have evaluated the beta-diversity of insect herbivores sampling the same host plant species at different geographic locations, with some studies showing low beta diversity (Novotny et al., 2006), and others showing the opposite pattern (Basset, 2000; Novotny et al., 2005; Salazar and Marquis, 2012; Fine et al., 2013). Even fewer studies have investigated host plant chemical defenses in conjunction with insect herbivore turnover, although there are many studies showing large variation in plant chemistry among populations (Mooney and Emboden, 1968; Macedo and Langenheim, 1989; Berenbaum and Zangerl, 1998; Zangerl and Berenbaum, 2003; Leimu et al., 2012). Most of these studies have shown that plant defense chemistry within a taxon varies among populations across geographic distances and habitats (but see Endara et al., 2018). Most of these previous studies have limited their comparisons to one or a few insect clades (i.e., Lepidoptera) and a subset of defense chemicals (i.e., alkaloids). A comprehensive evaluation of all insect herbivores and all specialized metabolites for widely-separated populations of host plants is needed to better understand the link between change in herbivore composition and host defense chemistry across large geographic distances. This will allow an evaluation of the selection pressures of the community of herbivores as well as the local adaptation of defense chemistry by the host plants.
Protium (Burseraceae) has been the subject of multiple studies regarding plant defense chemistry and/or insect herbivores (Siani et al., 1999, 2012; Fine et al., 2013; Lokvam et al., 2015; Salazar et al., 2018). It represents an ideal study system for investigating Amazonian tree diversity because of its high species richness (Daly et al., 2022), large number of coexisting species (Vleminckx et al., 2018), high relative abundance (ter Steege et al., 2013), up-to-date taxonomy (Daly and Fine 2011, 2018; Daly, 2019) and phylogeny (Fine et al. 2005, 2014).
Recently we reported on the chemical diversity and richness of Protium (Burseraceae) and its associated insect herbivore fauna in the Allpahuayo-Mishana National Reserve near Iquitos, Peru (Salazar et al., 2018). To test predictions of the GMTC and assess the link between plant herbivore turnover and specialized metabolite diversity we investigated Protium-insect herbivore interactions in a distant but comparable region in the Amazon basin. Here, we surveyed insect herbivores on 12 Protium species in rain forests near Manaus, Brazil for twelve-months (on average 15 Protium individuals per species). We selected 12 species also found in Iquitos, Peru, 1500 km away. We subjected each Protium species in Manaus to the same detailed analysis of their secondary metabolite composition performed in our Iquitos study.
We asked: 1) How much change in herbivore species composition can be observed in the tree genus Protium between Iquitos and Manaus?
2) How different is the Protium secondary metabolite composition in species occurring in both sites?
3) How are chemical richness and herbivore diversity related in the two locations?
Given the large distance between the two sites, we predicted to find significant differences in the insect herbivore fauna associated with Protium species. Because insect herbivores should contribute to divergent natural selection for effective chemical defenses in Protium species, we also predicted that the magnitude of the herbivore species turnover would be mirrored by similar changes in the host species defense chemistry across the two sites.
Methods
Background
In a recently published work, the authors studied 860 individuals from 31 sympatric species of Protium within a 10 ha area of intact rainforest in Iquitos, Peru, and surveyed the insect herbivores feeding on the plants for 62 weeks (Salazar et al., 2018). We analyzed the secondary metabolite composition of these plants, finding almost 600 specialized metabolites. We found 231 different insect herbivore taxa out of 4214 individual confirmed feeding records, mostly from the orders Hemiptera, Lepidoptera and Coleoptera. Protium species with high chemical diversity had significantly fewer numbers and fewer species of insect herbivores feeding on them (Salazar et al., 2018). Here we leveraged and expanded this dataset to a second site and used the combined dataset to investigate a new set of research questions.
Trees
Protium (Burseraceae) includes more than 140 species of mostly Neotropical trees, ranging from subcanopy treelets to canopy trees (Daly and Fine, 2018). The highest diversity and abundance of Protium is found in the Amazon basin where single sites can harbor up to 35 sympatric species (Rankin-de-Morona et al., 1992; Daly et al., 2022), and where Protium is often among the top five genera in terms of abundance throughout the basin (ter Steege et al., 2013). A phylogenetic analysis has proposed a well-resolved phylogeny of Protium (Fine et al., 2014). This work includes all species used for the current study, although some of the species names have changed. Thus, some of the species we reported in Salazar et al. (2018) and Fine et al. (2014) have been updated, and we include a table in the Appendix that lists all species in our study with how their names may have changed since the previous publications (Table S1).
Protium herbivore surveys in Peru
Complete details on this dataset are published in Salazar et al. (2018). In short, we sampled 31 species of Protium in the Allpahuayo-Mishana Reserve using forest transects. To balance our sampling effort across all Protium species, 18–25 individuals of each species were sampled (a total of 860 Protium plants). Individuals were censused for herbivores at least once a week for 64 consecutive weeks (May 2013 to September 2014). Each plant census included two steps: observation and collection. The observation step was implemented to record and collect only the herbivores that were observed feeding directly on the host plant. Collected herbivores were preserved in 80% ethanol and later assigned to a morphospecies using an “in-house” reference collection (deposited in the Essig museum of Entomology, UC Berkeley; Berkeley, CA). In total, the herbivore surveys quantified 4,214 feeding events from 231 herbivore morphospecies. Of all morphospecies in the dataset, 90 were singletons (found only once) and the 50 most abundant species accounted for 87% of total records. This sampling scheme was designed to accurately assess the quantitative and qualitative herbivore pressure experienced by the focal host-plant species, not to determine the ‘complete’ diet breadth of each herbivore observed.
Protium herbivore surveys in Brazil
In Manaus, we surveyed juvenile Protium trees at two locations, the Reserva Ducke and Reserva da Campina. The Reserva da Campina contains white-sand forests similar to the white-sand forests found in the Allpahuayo-Mishana Reserve in Iquitos; the Reserva Ducke has terra firme forests on sandy soil and clay soils similar to Allpahauyo-Mishana terra firme forests. We selected 12 Protium species for weekly monitoring also found in Iquitos. Data and specimen collection mirrored the protocol followed in Iquitos, Peru (Salazar et al. (2018), see above). In short, we surveyed 8-17 individuals per Protium species once a week (from November 2014 to October 2015) and collected insects that were observed feeding directly on the plants. Reserva da Campina is 36 km north of Reserva Ducke and was included in the weekly monitoring to increase the number of plant species surveyed. We sampled the two areas on the same day and in sequence but with alternating visit order. One week we visited Reserva Ducke first; the following week, it was Reserva da Campina to avoid sampling the plants at the same time of the day. We monitored 68 plants in Reserva da Campina and 112 in Reserva Ducke. Two species were only found at Campinas: P. heptaphyllum and P. subserratum morphotype 3. Only P. calanense and P. hebetatum were sampled at both places. We observed 966 feeding records from 163 insect morphospecies.
Insect molecular work (Manaus and Iquitos)
Insect DNA barcoding was conducted at the “morphospecies” level. For each putative insect species (morphospecies) we sequenced at least 5 individuals (more samples were used for morphologically variable taxa and recalcitrant morphospecies). A small sample from each insect (a leg or a small fraction the abdomen) was washed with distilled water and dried to remove all traces of ethanol. DNA extraction and PCR amplification of the COI gene were accomplished using standard methods (see Salazar et al. (2018)). Alignment was done using the MUSCLE algorithm (Edgar, 2004). To determine the best substitution model for our data we used the R package Phangorn via AIC (Schliep, 2011). Phylogenetic reconstruction was carried out using 10 independent runs under a GTR+G+I model for 3 million generations (four chains, 25% burn-in, sampled every 100 generations) using MrBayes 3.2.6 (Ronquist and Huelsenbeck, 2003).
Plant secondary metabolite analysis (Iquitos and Manaus)
The Burseraceae (Frankincense and Myrrh family), are well known for the production of terpene resins, and Protium is no exception. Before this study, almost 100 different terpene compounds had been characterized for Protium by gas-chromatography mass-spectroscopy (Rüdiger et al., 2007). These compounds have been shown to have medicinal properties (Rüdiger et al., 2007, Siani et al., 2012) and are widely believed to have defensive function against pathogens and insect herbivores (Langenheim, 2003). Protium species also produce a wide array of non-volatile secondary compounds, including polymeric flavans, flavones, oxidized terpenes, and quinic acids, among others (Siani et al., 2012, Lokvam and Fine 2012, Lokvam et al., 2015).
Chemical analysis was conducted at the plant species level. Between six and nine different individuals per species were analyzed. Young and mature leaf samples were collected in silica gel from the same marked individuals within the herbivore observation transects and transported to the University of California, Berkeley for analysis. To assess the maximum number of secondary compounds, we performed separate analyses for high- and low-molecular-weight metabolites (GCMS and HPLC-MS respectively) using a non-targeted metabolomic approach. Find more details of the methods and chemical composition of the study species in Salazar et al. (2018).
Low-molecular-weight metabolite chemistry (GC/MS)
Of the twelve species surveyed for herbivores, only 11 could be used for the final GC/MS analysis. Protium rhoifolium was not included due to having fewer than 3 samples. GC/MS methods follow Salazar et al. (2018). In short, 100 mg of dry leaf material was pulverized and extracted using 150 microliters of a 1:4 solution of ethanol: dichloromethane with 0.075 mg/l of Piperine as an internal standard. We injected 2.5 microliters of plant extract. The inlet was kept at a constant temperature of 275°C (split injection 1:40). The oven was programmed as follows: 85°C, hold for 2 min; ramp 1: 10°C/min; 155°C, hold for 1 min; ramp 2: 6°C/min; 260°C, hold for 1 min; ramp 3: 2°C/min; 300°C hold for 14 min (total run time 60 min). MS conditions: EI @ 70eV, scanning range 40-550 amu. Raw GC/MS data were processed in R with the package XCMS (script available upon request) using the approach by Salazar et al. (2016a); Salazar et al. (2016b). Chromatographic features from species were putatively classified using AMDIS (Automated Mass Spectral Deconvolution and Identification System) and the NIST/EPA/NIH and MassBank Databases (Horai et al., 2010). Metabolites that did not have a match from the available mass spectra databases or in the available literature were classified as unknown.
High-molecular-weight metabolite chemistry (HPLC-MS)
Leaf samples from our study species were pulverized and 100 mg samples were extracted using 1.5 mL of hexane (three times), 4:1 (v/v) ethanol/aq 0.5% acetic acid (4 times) and 7:3 (v/v) acetone/aq 0.5% acetic acid (3 times). Aqueous ethanol and acetone extracts were combined and dried under nitrogen. The mass of each extract was recorded before analysis by HPLC. Samples were dissolved in 1:1 methanol and methyl sulfoxide (0.1% (v/v) formic acid) at the rate of 100 µg/µL. The sample solvent included gallic acid, 4-hydroxy benzoic acid, sinapinic acid, flavone, chrysin and α-tocopherol as internal standards. Injection volumes were 3. HPLC analyses were carried out using an Agilent 1100 system (ESIMS) using an ion trap mass detector (LCQ Fleet, Thermo Scientific, Waltham, MA, USA). Separation was done using an Atlantis T3 2 × 150 mm 3µ ODS HPLC column (@ 40°C; Waters Corp., Milford, MA, USA). Gradient elution was performed as follows: (Solvent A) 1:1 methanol and acetonitrile and (Solvent B) water, both acidified with 0.1% (v/v) formic acid. At time (t, min) = 0, 5% A in >B; at t = 10, 15% A in B; at t = 60, 30% A in B; at t = 75, 70% A in B; at t = 100, 95% A in B; at t = 135, 100% A. This was followed by an isocratic step of 100% A to t = 150. ESIMS data were collected in the negative ion mode from t = 0-80, positive ion mode t = 80-114 and negative ion mode t = 114-150. HPLC raw data was processed using MZmine (Pluskal et al., 2010).
Data analysis
Protium herbivore and chemical turnover
To visualize the differences of Protium herbivore composition between our two sites we used R (packages Picante, Ape, and Bipartite) to map the number of observed and confirmed feeding records of each morphospecies on the herbivore phylogeny. Similarly, we used R to generate a Sankey Network to visualize the per-host species differences in herbivore community composition between Manaus and Iquitos. We assessed the herbivore community turnover by calculating the length-based phylogenetic beta diversity using the Phylosor algorithm (Bryant et al., 2008; Leprieur et al., 2012). To determine if our empirical values of phylogenetic beta diversity were within the random expectation, for each Protium species/site combination we created a null distribution by estimating the phylogenetic beta diversity on a set of 1000 randomly-generated herbivore communities (phylogeny label swapping). To quantify the turnover in Protium species chemical composition we calculated the Morisita chemical similarity index (Salazar et al., 2016a; Salazar et al. (2016b). In short, we used the MZmine workflow to create matrices of shared chromatographic features across all samples of the same species. These matrices are made by comparing the m/z data across the retention time component. These matrices were compared both using presence absence and abundance-weighted data. To visualize the changes in chemical composition of the focus Protium species between our two distance sites we performed chemical hierarchical clustering of multiple individuals of each species/site combination.
Relationship between herbivore community and host species chemistry
We performed a simple linear regression between plant species chemical richness (number of chemical compounds detected across our two analytical approaches) and the plant host herbivore species load (total number of herbivore species observed feeding on a Protium species). Finally, to assess the similarity between herbivore loads for each species across our two sites we regressed the Protium species herbivore richness found in Manaus with the one found in Iquitos.
Results
Turnover in the Protium herbivore assemblages between Iquitos and Manaus
We found very high species turnover between sites. Only four herbivore species were found at both sites (3 Lepidoptera and one Hemiptera, see Figure 1). However, three of these species were only found once at one of the two sites. Therefore, we found only one common species at both sites, a tortricid leaf roller (morphospecies “Cabeza Negra -LJ04-”, Tortricidae). At both sites the most diverse and abundant group of herbivores were the Hemipterans (mainly leaf hoppers – Cicadellidae-), followed by Coleopterans (mainly leaf beetles -Chrysomelidae) and caterpillars (Lepidoptera – various families-; Figure 1).
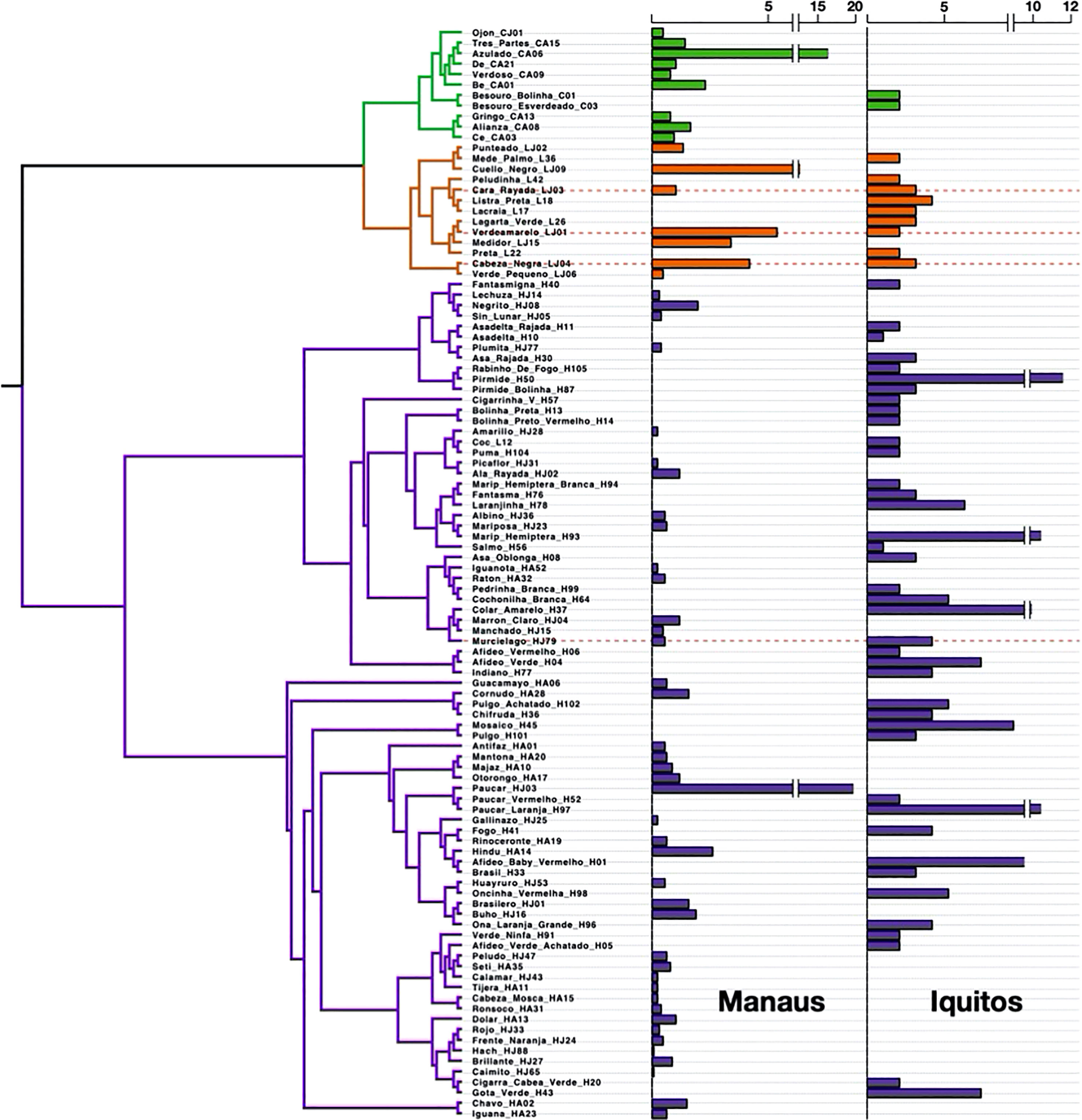
Figure 1 Phylogenetic structure of the herbivore community assemblies for the Iquitos and Manaus field sites. Bar and clade color represent the taxonomic order of the herbivores (Green= Coleoptera, Orange=Lepidoptera, Purple=Hemiptera. Bars represent the relative abundance (% of total abundance) of each herbivore species. Red horizontal lines indicate the four species found at both sites.
Given the high species turnover we assessed branch-length based phylogenetic beta diversity (Phylosor) (Bryant et al., 2008; Leprieur et al., 2012). Here, the phylogenetic beta diversity for an herbivore assemblage in the same host species in the two locations was lower than the average random expectation, although these differences were not statistically significant for most Protium species (Figure S1). Differences in herbivore composition for one site are not based on subsets of herbivore composition of the other site; they derive from completely different herbivore species that are found at one site or the other (Figure S2). Most insect herbivores were associated with multiple species of Protium (Figure 2).
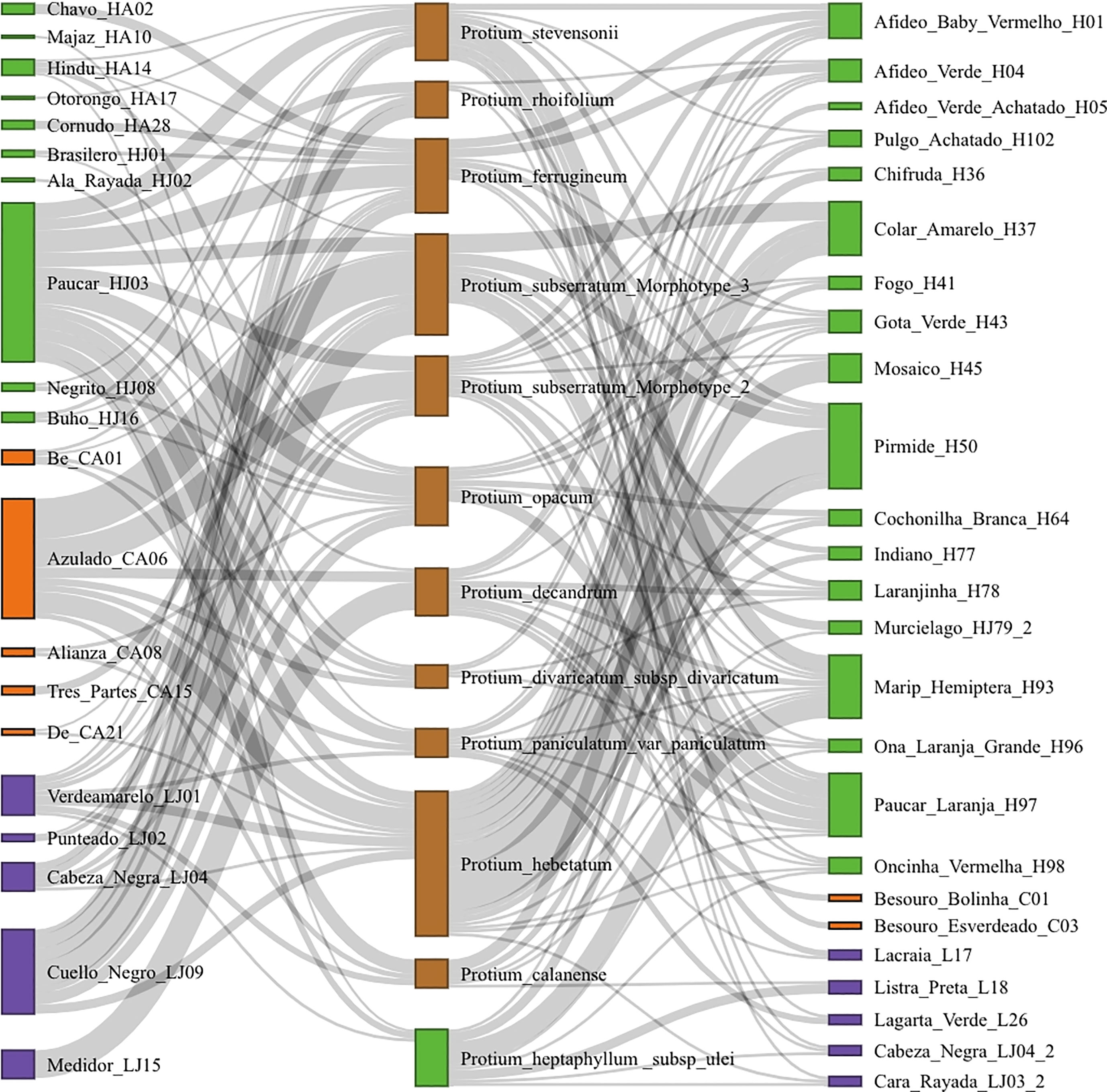
Figure 2 Plant-herbivore community networks from Iquitos and Manaus. The left column shows herbivores from Iquitos, the central column shows Protium species sampled in both sites, and the right column shows herbivores from Manaus. Data shows only herbivores above the 25th abundance percentile, to exclude singletons and less abundant species. Green = Hemiptera, Orange = Coleoptera, Purple = Lepidoptera.
Change in Protium defense chemical composition between Iquitos and Manaus
Contrary to our expectations, we did not find large differences in secondary metabolite composition between the Manaus and Iquitos populations for the twelve focal species. Although we did find some intraspecific variation, the variation in secondary metabolites between individuals of different populations (Iquitos and Manaus) was not larger than the variation within the same population. A non-targeted metabolomic analysis of both GC/MS and HPLC-MS data shows that individuals of one population appear nested within the clusters of the other population (Figure 3). The presence/absence chemical similarity composition (Morisita chemical similarity index) for the most dominant chromatographic features (specialized metabolites that comprise at least 0.1% of the total chromatogram integrated area) is 1 for most sample pairs (range=0.97-1). This result highlights that, in the vast majority of cases, the specialized metabolites expressed by a Protium species in Iquitos are the same metabolites expressed in Manaus (in Figure S3, we provide as an example of chromatograms of Protium ferrugineum from both sites) Similarly, when we accounted for the changes in relative abundance of specialized secondary compounds between our two sites, similarity between samples ranged from 0.74 to 0.98, with no obvious difference between and across populations. (Table S2).
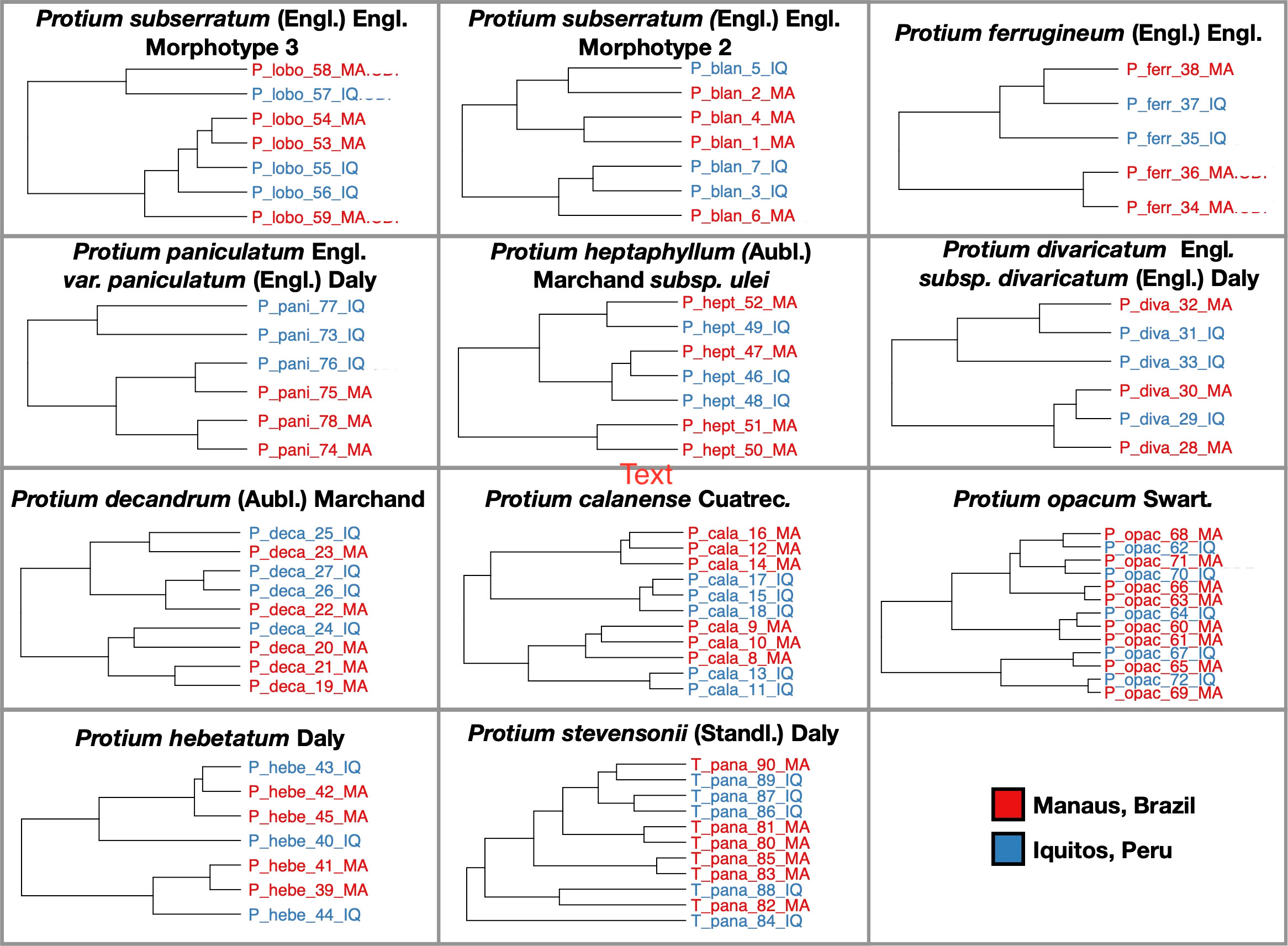
Figure 3 Chemical similarity of Protium species found in both Manaus and Iquitos. A hierarchical clustering (Ward’s method) based on the chemical composition of each individual sample in the two populations. Samples are colored by site.
The link between Protium herbivore community and host species chemistry
The most notable finding of this study is that, despite the large changes in herbivore community composition, the relationship between a Protium species’ chemical diversity (measured here are chemical richness) and its herbivore species richness was very consistent in both Iquitos and Manaus. In both locations we found a negative relationship between host species chemical richness and the number of herbivore species found feeding on a particular Protium taxon (Figure 4). Moreover, our most fascinating finding was, despite the extremely large herbivore species turnover, the herbivore species richness of a Protium species in one site predicts the species richness of the same species in a different site (Figure 4).
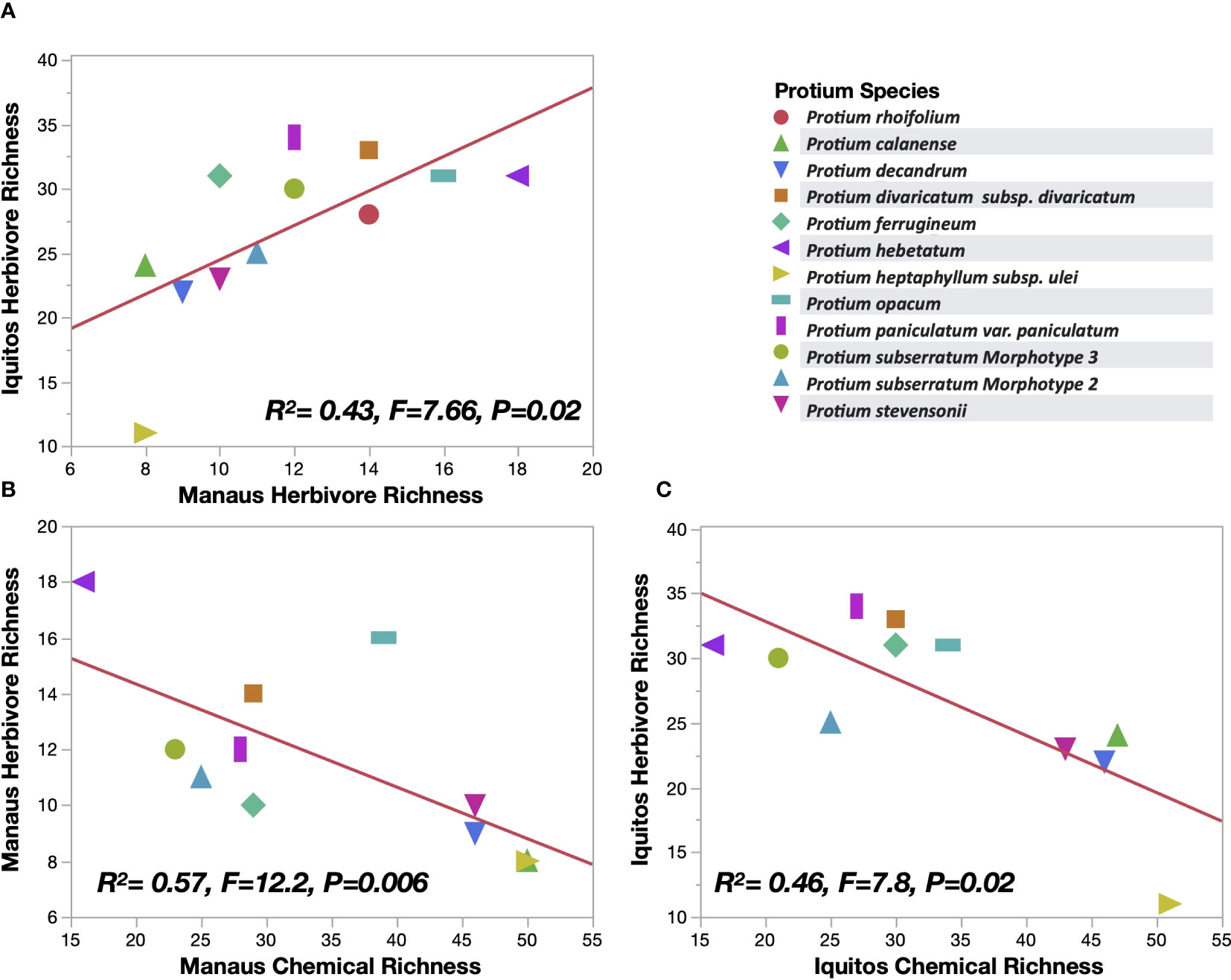
Figure 4 (A) association between Protium species herbivore species richness in Iquitos and Manaus (12 species shown). (B) (Manaus) and (C) (Iquitos): The relationship between a Protium species metabolite richness and the species’ herbivore richness (11 species shown).
Discussion
We found almost complete turnover in insect herbivore species composition between hosts of the same species of Protium in Iquitos and Manaus. In both locations, insects from the orders Hemiptera, Coleoptera and Lepidoptera were the most common herbivores feeding on the plants, in that rank order (Figure 1). However, there was minimal phylogenetic similarity in herbivore assemblages within a species across the two sites. These differences in herbivore faunas in host plants in the two locations therefore do not represent a simple replacement by herbivore sister taxa or close relatives. For example, insects associated with Protium hebetatum in Iquitos were phylogenetically dissimilar to insects associated with Protium hebetatum in Manaus; much more dissimilar than a random expectation (Figure S1). This pattern was repeated in all of the twelve species that we surveyed in the two locations. This result is contrary to the expectation of the co-evolutionary arms race hypothesis (Ehrlich and Raven, 1964) where closely related specialist herbivores would be associated with the same (or closely-related) host plant species and instead is more in agreement with the host-tracking hypothesis of Endara et al. (2017). Also similar in both sites was the pattern of insect herbivores feeding on multiple species of Protium. Strict monophagy was extremely rare in both locations (Figure 2).
The degree of host specialization and spatial turnover of herbivorous insect communities in tropical forests remains a subject of intense interest, ever since Erwin (1982) published his estimate of total herbivore species richness based on fogging several canopies of a single species of tropical tree. Novotny et al. (2007) found that in contrast to Erwin’s expectation, there was very little species turnover in four guilds of herbivorous insects even across more than 500 km of Papua New Guinea lowland forest. However, when sampling across an elevational gradient, Novotny et al. (2005) found strong differences in herbivore assemblages in three widespread species of Ficus. Each tree species was associated with a different dominant lepidopteran herbivore species in the lowlands compared to montane habitats. Similar results of high beta-diversity across elevational habitats have been reported in lepidopteran herbivores specialized on Piper (Rodriguez-Castañeda et al., 2010).
Fine et al. (2013) studied two morphotypes of Protium subserratum (the same two as in this paper) in four geographic sites. Two of these sites were white-sand forests and two were terra firme forests. They found strong patterns of high beta-diversity of insect herbivores between habitats and also between the same habitat on opposite sides of the Ucayali River, a large biogeographic barrier. Even though the sites were only 150 km apart, terra-firme vs terra-firme comparisons were 62% dissimilar and white-sand vs white-sand comparisons were 70% dissimilar. This represented a greater dissimilarity than the white-sand vs terra firme comparisons on the same side of the river (37% and 51% dissimilarity, respectively). These findings and the findings in the current study are very different from the low beta-diversity of insect assemblages reported in Novotny et al. (2006) in Papua New Guinea lowland forest. However, although the lowland forest sites in the Papua New Guinea study were 500 km apart they did not cross any major biogeographic barriers like wide rivers or mountains.
Chemical defense turnover is much lower than herbivore assemblage turnover
We found a striking similarity of secondary metabolite composition within Protium species in qualitative and quantitative investment in two sites separated by 1500 km. The twelve Protium species have much more similar chemical defenses across the two sites than their associated insect assemblages. In addition, there were no population-level signatures in chemical composition (Figure 3). However, we did find some individual-level differences in both unique specialized metabolites and concentrations of shared metabolites, and at this point we do not know how important these differences are or whether they deter herbivores differently. We can confirm that the “herbivore active metabolites” (HAMs) that we identified with the least absolute shrinkage and selection operator (LASSO) approach in Iquitos (Salazar et al., 2018) also do appear in Manaus. Still, we cannot draw any conclusions about whether these metabolites are also associated with repelling herbivores in Manaus. The LASSO method requires a larger sample size than the limited subset of species we surveyed in Manaus as compared to Iquitos (Hastie et al., 2009).
Similar consistent chemical defense allocation within species across large distances has been reported in other tropical tree genera. Endara et al. (2018) characterized metabolomes from eight species of Inga (Fabaceae) that had widespread geographic ranges and found that a majority of them had very similar secondary metabolite profiles even comparing conspecifics as far away as Panama, southern Peru, French Guiana, Manaus, and Amazonian Ecuador. Bursera showed very little differences in terpenes in populations of the same species across 18 different locations in Mexican tropical dry forest (Becerra, 2007). Trees, with a much slower generation time than insect herbivores, may not be able to adapt to local herbivore communities, which, with their faster generation times, are likely to change rapidly across time and space. Moreover, Neotropical trees have a great dispersal ability and many appear able to maintain gene flow across huge distances (Dexter et al., 2017).
Evolution of chemical diversity
Comparing two forests 1500km apart, we found strong patterns of turnover in insect herbivores feeding on the same species of plant hosts but correspondingly small differences in secondary metabolites. This does not fit predictions of the Geographic Mosaic Theory of Coevolution perhaps in large part because the most important herbivores in this system appear to be generalists, not specialists. Previous studies reporting evidence of GMTC have studied specialist herbivores and pollinators and reported variation in chemical defenses known to mediate specialist interactions (Thompson, 1997; Berenbaum and Zangerl, 2006). The scenario underlying the GMTC assumes that either local selective pressures or geographical/environmental barriers will be strong enough to prevent gene flow over generations across populations of host plants. If this is not the case, gene flow could help prevent speciation events in host plants while spreading new defensive alleles/genes across the range. The outcome of such a mechanism would be species with large geographical ranges, high regional (gamma) chemical diversity (but low beta chemical diversity), and a high beta diversity for their associated herbivore and pathogen communities, which is consistent with the findings of this study.
The most intriguing result in our study is the strong, significant correlation showing that a Protium species that was attacked by the fewest herbivore species in Iquitos was also attacked by the fewest herbivore species in Manaus, despite the fact that almost 100% of the insect herbivores were different in the two locations (Figure 4). Our hypothesis to explain this phenomenon is that having high chemical diversity is the best strategy available for Protium plants to prevent attack by a large number of different natural enemies. Having a high chemical diversity (here quantified as chemical richness) correlates with having the largest number of effective chemicals (Salazar et al., 2018), and these effective chemicals are likely to be effective only in a subset of a plant’s geographic distribution, meaning that plants with high chemical diversity are more likely to be successful in more biotic contexts across space and time.
Other studies on diverse Neotropical woody plant clades have concluded that secondary metabolites are key to understanding local plant diversity and abundance (Salazar and Marquis, 2012; Kursar et al., 2009; Coley and Kursar, 2014; Endara et al., 2015; Sedio et al., 2017; Coley et al., 2018). Richards et al. (2015) found chemical diversity to be key in understanding local diversity patterns of Piper, although the relationship between chemical abundance and herbivore diversity they found is opposite of the patterns we report in Protium. A key point to underline is that the Protium system is dominated by generalists and therefore, evolutionary pressure on particular defense chemicals is not likely as strong as it would be with a plant clade mainly attacked by specialist herbivores. We encourage investigators of other tropical plant clades to expand their sampling of natural enemies to include non-Lepidopteran herbivores in order to be able to make comparisons with the patterns we have uncovered in Protium.
We still need more evidence to test the hypothesis that high chemical diversity is an effective strategy to reduce attack from natural enemies, as we have only been able to associate a small number of the secondary metabolites in Protium with deterring particular herbivores (Salazar et al., 2018). Yet, it is well known that there are other important natural enemies which are deterred by secondary metabolites (Langenheim, 2003). For example, future studies could test how Protium secondary metabolites are associated with fungal pathogens across their geographic distribution to get a more comprehensive picture of the forces influencing the evolution of chemical diversity. Finally, it will be important to test the hypothesis that high chemical diversity drives plant relative abundance and geographic distribution by studying these species’ enemy–secondary metabolite interactions at more locations throughout the Amazon basin and relating this to local and regional abundance.
Data availability statement
The datasets presented in this study can be found in online repositories. The names of the repository/repositories and accession number(s) can be found below: https://www.ncbi.nlm.nih.gov/, KY509049–KY509292. Please also see Supplementary Material.
Author contributions
PF, FB, IM, and DS designed the study, PF, FB, IM, MP, JZ, EM, MS, CN, and DS conducted field work, JL, DS conducted lab work, PF and DS wrote the manuscript. All authors contributed to the article and approved the submitted version.
Funding
Funding for this project was provided by NSF DEB (award numbers 1254214 and 1952378) to PF. FB has been continuously supported by CNPq grant (#313986/2020-7).
Acknowledgments
We thank the C. Rivera for help with research permits at the Allpahuayo-Mishana National Reserve in Iquitos, Peru and SERNANP for research and export permits. In Brazil, we thank IBAMA for research permit TRTM 125530/2015. Publication made possible in part by support from the Berkeley Research Impact Initiative (BRII) sponsored by the UC Berkeley Library. We thank N. Tsutsui for laboratory space and sample archiving. We thank M. Metz, B. Castro Escobar, G. Figueroa, G. Trujillo, and F. Wildtruth for comments on the manuscript.
Conflict of interest
The authors declare that the research was conducted in the absence of any commercial or financial relationships that could be construed as a potential conflict of interest.
Publisher’s note
All claims expressed in this article are solely those of the authors and do not necessarily represent those of their affiliated organizations, or those of the publisher, the editors and the reviewers. Any product that may be evaluated in this article, or claim that may be made by its manufacturer, is not guaranteed or endorsed by the publisher.
Supplementary material
The Supplementary Material for this article can be found online at: https://www.frontiersin.org/articles/10.3389/fevo.2023.1180274/full#supplementary-material
References
Barbehenn R. V., Constabel C. P. (2011). Tannins in plant-herbivore interactions. Phytochemistry 72, 1551–1565. doi: 10.1016/j.phytochem.2011.01.040
Barone J. A.(1998). Host-specificity of folivorous insects in a moist tropical forest. J. Anim. Ecol. 67, 400–409. doi: 10.1046/j.1365-2656.1998.00197.x
Basset Y. (1999). Diversity and abundance of insect herbivores foraging on seedlings in a rainforest in Guyana. Ecol. Entomology 24, 245–259. doi: 10.1046/j.1365-2311.1999.00197.x
Basset Y. (2000). Insect herbivores foraging on seedlings in an unlogged rain forest in Guyana: spatial and temporal considerations. Stud. Neotropical Fauna Environ. 35, 115–129. doi: 10.1076/0165-0521(200008)35:2;1-9;FT115
Becerra J. X. (2007). The impact of herbivore-plant coevolution on plant community structure. Proc. Natl. Acad. Sci. 104, 7483–7488. doi: 10.1073/pnas.0608253104
Berenbaum M., Zangerl A. R. (1998). Chemical phenotype matching between a plant and its insect herbivore. Proc. Natl. Acad. Sci. United States America 95, 13743–13748. doi: 10.1073/pnas.95.23.13743
Berenbaum M., Zangerl A. R. (2006). Parsnip webworms and host plants at home and abroad: trophic complexity in a geographic mosaic. Ecology 87, 3070–3081. doi: 10.1890/0012-9658(2006)87[3070:PWAHPA]2.0.CO;2
Bryant J. A., Lamanna C., Morlon H., Kerkhoff A. J., Enquist B. J., Green J. L. (2008). Microbes on mountainsides: Contrasting elevational patterns of bacterial and plant diversity. Proc. Natl. Acad. Sci. United States America 105, 11505–11511. doi: 10.1073/pnas.0801920105
Coley P. D., Barone J. A. (1996). Herbivory and plant defenses in tropical forests. Annu. Rev. Ecol. Systematics 27, 305–335. doi: 10.1146/annurev.ecolsys.27.1.305
Coley P. D., Endara M. J., Kursar T. A. (2018). Consequences of interspecific variation in defenses and herbivore host choice for the ecology and evolution of Inga, a speciose rainforest tree. Oecologia 187, 361–376. doi: 10.1007/s00442-018-4080-z
Coley P. D., Kursar T. A. (2014). On tropical forests and their pests. Science 343, 35–36. doi: 10.1126/science.1248110
Daly D. C. (2019). A new identity for Tetragastris panamensis. Studies in Neotropical Burseraceae XXIX. Brittonia 71, 345–346. doi: 10.1007/s12228-019-09577-w
Daly D. C., Fine P. V. A. (2011). A new Amazonian section of Protium (Burseraceae) including both edaphic and specialist and generalist taxa. Stud. Neotropical Burseraceae XVI. Systematic Bot. 36, 939–949. doi: 10.1600/036364411X604958
Daly D. C., Fine P. V. A. (2018). Generic limits re-visited and an updated sectional classification for Protium (tribe Protieae). Stud. Neotropical Burseraceae XXV. Brittonia 70, 418–426. doi: 10.1007/s12228-018-9533-5
Daly D. C., Perdiz R. O., Fine P. V. A., Damasco G., Martinez-Habibe M. C., Calvillo-Canadell. L. (2022). A review of neotropical burseraceae. Braz. J. Bot. 45, 103–137. doi: 10.1007/s40415-021-00765-1
Dexter K. G., Lavin M., Torke B. M., Twyford A. D., Kursar T. A., Coley P. D, et al. (2017). Dispersal assembly of rain forest tree communities across the Amazon basin. Proc. Natl. Acad. Sci. 114, 2645–2650. doi: 10.1073/pnas.1613655114
Edgar R. C. (2004). MUSCLE: multiple sequence alignment with high accuracy and high throughput. Nucleic Acids Res. 32, 1792–1797. doi: 10.1093/nar/gkh340
Ehrlich P. R., Raven P. H. (1964). Butterflies and plants: A study in coevolution. Evolution 18, 586–608. doi: 10.2307/2406212
Endara M. J., Coley P. D., Ghabash G., Nicholls J. A., Dexter K. G., Donoso D. A., et al. (2017). Coevolutionary arms race versus host defense chase in a tropical herbivore–plant system. Proc. Natl. Acad. Sci. 114, E7499–E7505. doi: 10.1073/pnas.1707727114
Endara M. J., Coley P. D., Wiggins N. L., Forrister D. L., Younkin G. C., Nicholls J. A., et al. (2018). Chemocoding as an identification tool where morphological- and DNA-based methods fall short: Inga as a case study. New Phytol. 218, 847–858. doi: 10.1111/nph.15020
Endara M. J., Weinhold A., Cox J. E., Wiggins N. L., Coley P. D., Kursar T. A. (2015). Divergent evolution in antiherbivore defences within species complexes at a single Amazonian site. J. Ecol. 103, 1107–1118. doi: 10.1111/1365-2745.12431
Erwin T. L. (1982). Tropical forests, their richness in Coleoptera and other arthropod species. Coleopterists Bull. 36, 74–75.
Fine P. V. A., Daly D. C., Villa Muñoz G., Mesones I., Cameron K. M. (2005). The contribution of edaphic heterogeneity to the evolution and diversity of Burseraceae trees in the Western Amazon. Evolution 59, 1464–1478. doi: 10.1554/04-745
Fine P. V. A., Metz M. R., Lokvam J., Mesones I., Ayarza Zuñiga J. M., Lamarre G. P. A., et al. (2013). Insect herbivores, chemical innovation and the evolution of habitat specialization in Amazonian trees. Ecology 94, 1764–1775. doi: 10.1890/12-1920.1
Fine P. V. A., Zapata F., Daly D. C. (2014). Investigating processes of Neotropical rain forest tree diversification by examining the evolution and historical biogeography of the Protieae (Burseraceae). Evolution 68, 1988–2004. doi: 10.1111/evo.12414
Hastie T., Tibshirani R., Friedman J. H. (2009). The elements of statistical learning: data mining, inference, and prediction. 2nd (New York: Springer).
Horai H., Arita M., Kanaya S., Nihei Y., Ikeda T., Suwa K., et al. (2010). MassBank: a public repository for sharing mass spectral data for life sciences. J. Mass Spectrometry 45, 703–714. doi: 10.1002/jms.1777
Kursar T. A., Dexter K. G., Lokvam J., Pennington R. T., Richardson J. E., Weber M. G., et al. (2009). The evolution of anti-herbivore defenses and their contribution to species coexistence in the tropical tree genus Inga. Proc. Natl. Acad. Sci. 106, 18073–18078. doi: 10.1073/pnas.0904786106
Langenheim J. H. (2003). Plant resins: chemistry, evolution, ecology, ethobotany (Portland: Timber Press).
Leimu R., Muola A., Laukkanen L., Kalske A., Prill N., Mutikainen P. (2012). Plant-herbivore coevolution in a changing world. Entomol. Exp. Appl. 144, 3–13. doi: 10.1111/j.1570-7458.2012.01267.x
Leprieur F., Albouy C., De Bortoli J., Cowman P. F., Bellwood D. R., Mouillot D. (2012). Quantifying phylogenetic beta diversity: distinguishing between 'true' turnover of lineages and phylogenetic diversity gradients. PloS One 7. doi: 10.1371/annotation/6fe0199e-7916-4bb9-9c9e-b854c5cee029
Lokvam J., Fine P. V. A. (2012). An oxidized squalene derivative from Protium subserratum Engl. (Engl.) growing in Peru. Molecules 17, 7451–7457. doi: 10.3390/molecules17067451
Lokvam J., Metz M. R., Takeoka G. R., Nguyen L., Fine P. V. A. (2015). Habitat-specific divergence of procyanidins in Protium subserratum (Burseraceae). Chemoecology 25, 293–302. doi: 10.1007/s00049-015-0198-1
Macedo C. A., Langenheim J. (1989). Intra and interplant leaf sesquiterpene variability in Copaifera langsdorfii: relation to microlepidopteran herbivory. Biochem. Systematics Ecol. 17, 551–557. doi: 10.1016/0305-1978(89)90098-7
Mooney H. A., Emboden W. A. (1968). The relationship of terpene composition, morphology, and distribution of populations of Bursera microphylla. Brittonia 20, 44–51. doi: 10.2307/2805460
Novotny V., Drozd P., Miller S. E., Kulfan M., Janda M., Basset Y, et al. (2006). Why are there so many species of herbivorous insects in tropical rainforests? Science 313, 1115–1118. doi: 10.1126/science.1129237
Novotny V., Miller S. E., Basset Y., Cizek L., Darrow K., Kaupa B., et al. (2005). An altitudinal comparison of caterpillar (Lepidoptera) assemblages on Ficus trees in Papua New Guinea. J. Biogeography 32, 1303–1314. doi: 10.1111/j.1365-2699.2005.01225.x
Novotny V., Miller S. E., Hulcr J., Drew R. A. I., Basset Y., Janda M., et al. (2007). Low beta diversity of herbivorous insects in tropical forests. Nature 448, 692–695. doi: 10.1038/nature06021
Pluskal T., Castillo S., Villar-Briones A., Orešič M. (2010). MZmine 2: modular framework for processing, visualizing, and analyzing mass spectrometry-based molecular profile data. BMC Bioinf. 11, 1. doi: 10.1186/1471-2105-11-395
Price P. W. (2002). Resource-driven terrestrial interaction webs. Ecol. Res. 17, 241–247. doi: 10.1046/j.1440-1703.2002.00483.x
Rankin-de-Morona J. M., Prance G. T., Hutching R. W., Silva M. F., Rodrigues W. A., Uehling M. E. (1992). Preliminary results of a large-scale tree inventory of upland rain forest in the central Amazon. Acta Amazônica 22, 493–534. doi: 10.1590/1809-43921992224534
Richards L. A., Dyer L. A., Forister M. L., Smilanich A. M., Dodson C. D., Leonard M. D., et al. (2015). Phytochemical diversity drives plant-insect community diversity. Proc. Natl. Acad. Sci. 112, 10973–10978. doi: 10.1073/pnas.1504977112
Rodriguez-Castañeda G., Dyer L. A., Brehm G., Connahs H., Forkner R. E., Walla T. R. (2010). Tropical forests are not flat: how mountains affect herbivore diversity. Ecol. Lett. 13, 1348–1357. doi: 10.1111/j.1461-0248.2010.01525.x
Ronquist F., Huelsenbeck J. P. (2003). MrBayes 3: Bayesian phylogenetic inference under mixed models. Bioinformatics 19, 1572–1574. doi: 10.1093/bioinformatics/btg180
Rüdiger A. L., Siani A. C., Veiga Junior V F. (2007). The chemistry and pharmacology of the South America genus Protium Burm. f. (Burseraceae). Pharmacogn Rev 1, 93–104.
Salazar D., Jaramillo M. A., Marquis R. J. (2016a). The impact of plant chemical diversity on plant herbivore interactions at the community level. Oecologia 181, 1199–1208. doi: 10.1007/s00442-016-3629-y
Salazar D., Jaramillo M. A., Marquis R. J. (2016b). Chemical similarity and local community assembly in the species rich tropical genus Piper. Ecology 97, 3176–3183. doi: 10.1002/ecy.1536
Salazar D., Lokvam J., Mesones I., Ayarza Zuñiga J. M., Vásquez Pilco M., de Valpine P., et al. (2018). Origin and maintenance of chemical diversity in a species-rich tropical tree lineage. Nat. Ecol. Evol. 2, 983–990. doi: 10.1038/s41559-018-0552-0
Salazar D., Marquis R. J. (2012). Herbivore pressure increases towards the equator. Proc. Natl. Acad. Sci. 109, 12616–12620. doi: 10.1073/pnas.1202907109
Sam K., Koane B., Sam L., Mrazova A., Segar S., Volf M, et al. (2020). Insect herbivory and herbivores of Ficus species along a rain forest elevational gradient in Papua New Guinea. Biotropica 52, 263–276. doi: 10.1111/btp.12741
Schemske D. W. (2009). “Biotic interactions and speciation in the tropics,” in Speciation and Patterns of Diversity. Eds. Butlin R. K., Bridle J. R., Schluter D. (Cambridge, United Kingdom: Cambridge Univ. Press), 219–239.
Schliep K. P. (2011). Phangorn: phylogenetic analysis in R. Bioinformatics 27, 592–593. doi: 10.1093/bioinformatics/btq706
Sedio B. E., Rojas Echeverri J. C., Boya P. C. A., Wright S. J. (2017). Sources of variation in foliar secondary chemistry in a tropical forest tree community. Ecology 98, 616–623. doi: 10.1002/ecy.1689
Siani A. C., Nakamura M. J., Tappin M. R., Monteiro S. S., Guimaraes A. C., Ramos M. F. (2012). Chemical composition of South American Burseraceae non-volatile oleoresins and preliminary solubility assessment of their commercial blend. Phytochemical Anal. 23, 529–539. doi: 10.1002/pca.2351
Siani A. C., Ramos M. F. S., Menezes-de-Lima O. Jr., Ribeiro-dos-Santos R., Fernandez-Ferreira E., Soares R. O. A., et al. (1999). Evaluation of anti-inflammatory-related activity of essential oils from the leaves and resins of species of Protium. J. Ethnopharmacology 66, 57–69. doi: 10.1016/S0378-8741(98)00148-2
ter Steege H., Pitman N. C. A., Sabatier D., Baraloto C., Salomão R. P., Guevara J. E, et al. (2013). Hyperdominance in the Amazonian tree flora. Science 342, 325(6156). doi: 10.1126/science.1243092
Thompson J. N. (1997). Evaluating the dynamics of coevolution among geographically structured populations. Ecology 78, 1619–1623. doi: 10.1890/0012-9658(1997)078[1619:ETDOCA]2.0.CO;2
Thompson J. N. (2005). The Geographic Mosaic of Coevolution (Chicago, IL, USA: University of Chicago Press).
Vleminckx J., Salazar D., Fortunel C., Mesones I., Dávila N., Lokvam J., et al. (2018). Divergent secondary metabolites and habitat filtering both contribute to tree species coexistence in the Peruvian Amazon. Front. Plant Sci. 9, 836. doi: 10.3389/fpls.2018.00836
Volf M., Hrcek J., Julkunen-Tiitto R., Novotny V. (2015). To each its own: differential response of specialist and generalist herbivores to plant defence in willows. J. Anim. Ecol. 84, 1123–1132. doi: 10.1111/1365-2656.12349
Keywords: rainforest plants, secondary metabolites, insects, chemical ecology, beta-diversity, phylobetadiversity
Citation: Fine PVA, Baccaro FB, Lokvam J, Mesones I, Vásquez Pilco M, Ayarza Zuñiga JM, Merkel E, Sanches M, Nogueira CA and Salazar D (2023) A test of the Geographic Mosaic Theory of Coevolution: investigating widespread species of Amazonian Protium (Burseraceae) trees, their chemical defenses, and their associated herbivore faunas. Front. Ecol. Evol. 11:1180274. doi: 10.3389/fevo.2023.1180274
Received: 05 March 2023; Accepted: 13 July 2023;
Published: 23 August 2023.
Edited by:
María-José Endara, University of the Americas, EcuadorReviewed by:
Massuo Jorge Kato, University of São Paulo, BrazilJuan Ernesto Guevara Andino, Field Museum of Natural History, United States
Copyright © 2023 Fine, Baccaro, Lokvam, Mesones, Vásquez Pilco, Ayarza Zuñiga, Merkel, Sanches, Nogueira and Salazar. This is an open-access article distributed under the terms of the Creative Commons Attribution License (CC BY). The use, distribution or reproduction in other forums is permitted, provided the original author(s) and the copyright owner(s) are credited and that the original publication in this journal is cited, in accordance with accepted academic practice. No use, distribution or reproduction is permitted which does not comply with these terms.
*Correspondence: Paul V. A. Fine, paulfine@berkeley.edu