- Negaunee Integrative Research Center, The Field Museum, Chicago, IL, United States
The modern discussion of living fossils turns mostly on the persistence of archaic, or ancestral, traits in extant organisms. Prime examples mentioned by Darwin already—who also coined the term “living fossil”—include the platypus and the extant lungfishes. However, the identification of archaic traits in extant organisms requires a basis of comparison, i.e., it requires an estimate of the phylogenetic interrelationships of the living fossil in question. Phylogenetic relationships are determined not on the basis of the persistence of archaic traits, but on the basis of shared derived characters. The identification of persistent traits in an organism requires the same organism to also exhibit advanced, or specialized traits that allow its proper classification. The occurrence of such a mixture of primitive (plesiomorphic) or derived (apomorphic) traits in an organism, or species, is called the heterobathmy of characters. Willi Hennig recognized the heterobathmy of characters as quite a universal phenomenon, and made it the basis of his phylogenetic systematics.
1. Introduction
The term “living fossils” was coined by Darwin (1859: 486), who placed great hope on these “Species and groups of species, which are called aberrant, and which may fancifully be called living fossils,” which he thought “will aid us in forming a picture of the ancient forms of life.” Earlier in the Origin, Darwin (1859: 107) identified the “Ornithorhynchus [the platypus] and Lepidosiren” as such living fossils, which “connect to a certain extent orders now widely separated in the natural scale.” The South American lungfish Lepidosiren was discovered by the Viennese naturalist Johann Natterer (1787–1843), who from 1817 through 1835 explored remote areas in Brazil, collecting plants and animals for the precursor of the Vienna Natural History Museum. The two specimens of Lepidosiren collected by Natterer arrived in Vienna on 16 September 1836, prompting the volunteer curator at the Imperial Natural History Collections, Leopold Fitzinger (1802–1884), to comment on the strange creature in a letter that was read at the meeting of German Naturalists and Physicians in Jena on 26 September 1836, and subsequently published in Oken’s Isis (Fitzinger, 1837). In this letter, Fitzinger emphasized the overall similarity this new form shared with Muraena (e.g., the Mediterranean moray), which must have been the reason why Natterer had initially considered his catch to certainly be a fish. But Fitzinger also found similarities that the new form shared with certain limb-reduced salamanders that may retain permanent gills in adulthood. Fitzinger was able to identify remains of a lung, on which basis he concluded that the new form must definitively be classified as an amphibian near the North American salamander genus Amphiuma. He concluded his letter with naming the new form Lepidosiren paradoxa, which translates into the “paradoxical scaly Siren,” the latter again a genus of limb-reduced, perennibranchiate salamanders from North America, first described by John Ellis (1710–1776) in 1766 (Ellis, 1766). In his own monograph on Lepidosiren, Natterer (1837) supported Fitzinger’s conclusion that the animal was an amphibian, to be classified in the Ichthyodea, which is how he named the fish-like amphibians (Natterer, 1837: 267). Hence Darwin’s (1859: 107) remark that “living fossils” such as Lepidosiren “connect to a certain extent orders now widely separated in the natural scale.”
The coming years would see a growing controversy as to whether lungfish should be classified as fish, or as amphibians (the debate is reviewed in Conant, 1986), fueled by the discovery of the African lungfish Protopterus, described and classified as a fish by Owen (1839). The debate has perhaps never been better characterized than by Thomas H. Huxley (1825–1895) in his later remark on the Australian lungfish: “Equally good arguments might be adduced for the assertion that it is an amphibian or a fish, or both, or neither” (Huxley, 1880a: 660). In 28 April 1870, Gerald Krefft (1830–1881), then Curator and Secretary of the Australian Museum in Sydney, had a paper of his read in front of the Zoological Society of London on a “gigantic amphibian allied to the Genus Lepidosiren” from the Wide Bay area of Queensland (Australia), discovered by the honorable William Forster, after whom he named the new species Ceratodus forsteri” (Krefft, 1870: 221). Albert Günther (1830–1914), then Keeper of Zoology at the British Museum had been informed by a letter from Krefft of this new discovery, but before Günther had a chance to comment in a reply letter, Krefft had his paper delivered at the Zoological Society of London—with Günther in attendance. Günther judged Krefft’s specimen, which was eviscerated, to have been poorly preserved, and proceeded to write an extensive monograph on the anatomy of the new species, based on a complete and better preserved specimen that was acquired by the Australian Museum a few months later and forwarded to the British Museum (Natural History). Owen likewise “received a third (male) specimen … in the month of November; he very kindly handed it over to me” (Günther, 1871a: 512). Günther gave credit to Krefft for having correctly recognized the affinities of this new Australian form to Lepidosiren, but differed from Krefft in classifying these animals, including the African form Protopterus, as ganoid fishes in the suborder Dipnoi (lungfish). Krefft had named the Australian form on the basis of its dentition, which he found to correspond to fossil teeth described by Agassiz under the generic name Ceratodus, and which Agassiz had referred to sharks (Krefft, 1870: 221). Agassiz (1870: 166) later called his referral of Ceratodus teeth to selachians a “stupendous mistake.” Günther (1871a: 513) likewise noted the very close similarity, indeed near identity, of the teeth of the Australian lungfish with some of the Ceratodus teeth from Triassic and Jurassic deposits in Europe. It is probably for this reason that in his evaluation of the relationships of Ceratodus, Lepidosiren, and Protopterus, Günther made extensive reference to the fossil record. In his deliberations he focused on the Devonian genus Dipterus, as it shows characters “which appear to indicate an affinity to Ceratodus” (Günther, 1871a: 556): “Ceratodus, Lepidosiren, and Dipterus are most closely allied forms, and must remain together in the same suborder” (Günther, 1871a: 588). Given this conclusion, and the fact that there was no question that the Paleozoic fossil Dipterus was evidently a “ganoid” fish rather than an amphibian, a possible amphibian nature of the living lungfishes was thus conclusively rejected: “for we find that the Dipnoi reach back, with comparatively insignificant modifications, into one of the oldest epochs from which fish-remains are preserved” (Günther, 1871a: 560). The concluding sentence of his monograph reads: “The Dipnoi offer the most remarkable example of persistence of organization [through geologic time] not in Fishes only, but in Vertebrates” (Günther, 1871a: 561).
2. Neoceratodus as a living fossil
While admitting that “we have only the teeth for our guidance,” Günther (1871a: 513) was nevertheless struck by the close similarity the tooth plates of the extant Australian lungfish share with those of the fossil Ceratodus and concluded “that we should be better justified in making generic distinctions among the fossil forms, than in separating the living from the extinct.” But in 1891, Teller described a dipnoan skull complete with lower jaw from the Late Triassic of the northern Alps (Austria), Ceratodus sturii n. sp., and on that basis concluded that in spite of close similarities, the extant Australian lungfish nevertheless deserves to be referred to its own genus, which he called Epiceratodus (following the rules of nomenclature, the name Neoceratodus De Castelnau, 1876, takes priority over Epiceratodus Teller, 1891: Kemp, 1986: 182). Nevertheless, Teller (1891: 36) marveled at the “tenacity with which the dipnoan of the Australian rivers clung to the characteristic features of its ancient type. I do not know of a second example of such a persistence of characters in the vertebrate kingdom; and certainly we are entitled, in spite of the vast timespan which separates the periods of existence of the organisms here discussed, to talk of the direct descent of a living species from a fossil one.” And yet, the living lungfish from Queensland “no longer reaches the height of the development of its ancestors. With its lesser degree of ossification of its skeleton, but particularly with the primitive arrangement of the dermal bones in the skull, and with its superficially located lateral line canal system, it represents so as to say an embryonic stage of its ancient stem form” (Teller, 1891: 36). This is a very insightful statement, as it points to a mixture of characters in the anatomy of the Queensland lungfish, caused by a phenomenon that today is called heterochrony. While some parts of the anatomy of Neoceratodus reach the fully mature stage of development compared to the fossilized ancestral conditions, other parts lag behind in their development and remain in a juvenile, in Teller’s (1891) terms even embryonic, condition compared to the ancestor. Such arrested development is certainly not an ancestral, but a derived, i.e., specialized trait of Neoceratodus.
Both Günther (1871a), as well as Teller (1891) emphasized the astonishing persistence of form that is revealed in a comparison of Neoceratodus with its fossil relatives from the Triassic period. In fact, a case has been made that the species Neoceratodus forsteri existed in the Cretaceous of Australia already (Kemp and Molnar, 1981), while the genus Neoceratodus reaches back in its existence to the Triassic of Russia and Australia (Kemp, 1986: 181, and references therein). Neoceratodus may thus be considered as “probably the oldest surviving genus of vertebrate” (Kemp and Molnar, 1981: 216; see also Cavin and Kemp, 2011). But to call Neoceratodus forsteri for that reason a “living fossil” requires attention to detail. Whereas the Queensland lungfish does show parts in its anatomy that correspond to its ancestral Triassic relatives, other parts in its anatomy correspond to a juvenile, even embryonic stage of development compared to the ancestral condition. It thus exemplifies the fossilized ancestral condition in some, but not in all parts of its anatomy. Among the parts that do correspond to the ancestral condition is the skeleton of the paired appendages (pectoral and pelvic fins).
3. Carl Gegenbaur (1826–1903) and the origin of paired appendages in vertebrates
Gegenbaur was the leading comparative anatomist in Germany during the second half of the 19th Century. During the first stage of his career, Gegenbaur held the Chair for Comparative Morphology at the Friedrich Schiller University in Jena, an institution at which he met the “German Darwin” Ernst Haeckel (1834–1919), who became a close collaborator and friend (Kutschera et al., 2019). It was under the influence of Haeckel that Gegenbaur transformed idealistic morphology into an evolutionary research program in comparative anatomy (Nyhart, 2003: 163; Rieppel, 2011). Although transformationist in its outlook, the evolutionary morphology of Gegenbaur was still imbued by the 19th Century progressionism so characteristic of Haeckel’s thinking as well.
One of the many key evolutionary innovations in vertebrates Gegenbaur investigated was the origin of paired appendages in jaw-bearing vertebrates (gnathostomes), fins in fishes, limbs in tetrapods. The method he proposed to illuminate that question was still firmly rooted in idealistic morphology, although presented with a transformationist gloss: search for the fundamental type that is in its essence manifest in all paired appendages of gnathostomes. Once recognized, it enables the researcher “to distinguish what is constant from what is subject to continuous change, what is essential from what is non-essential, and thus reveals the continuity among the manifold and often very opaque conditions of form” (Gegenbaur, 1876: 400). That type, underlying the manifold modifications and manifestations of the paired appendages in gnathostome vertebrates, Gegenbaur called the archipterygium.
In his quest to uncover the archipterygium, Gegenbaur naturally looked first at sharks, representatives of the cartilaginous fishes or Chondrichthyes, thought to reside on the lowest, most primitive rung of the gnathostome ladder of life. This was so because in all vertebrates, the endoskeleton is first (in the embryo) preformed in cartilage, which is later partially, or entirely replaced by bone through a process called ossification. Since cartilage formation precedes bone formation in the endoskeleton of vertebrates, a fish with an entirely cartilaginous endoskeleton (a chondrichthyan) must be more primitive than a fish with an at least partially ossified endoskeleton (osteichthyan). This followed from Haeckel’s “biogenetic law,” according to which ontogeny recapitulates the sequence of transformations that characterize the successive links in the great chain of being: ontogeny recapitulates phylogeny (Haeckel, 1870: 361–362; palingenesis in Haeckel’s terms).
Accordingly, the archipterygium—the type that underlies all the manifold modifications of the paired appendages in gnathostome vertebrates—should be most immediately recognizable in the fins of the most primitive gnathostomes, the sharks. Given the sharks—fossil and extant—known to Gegenbaur at the time, the argumentation he deployed in his characterization of the archipterygium, derived from the anatomy of the paired fins of sharks, comes across as rather convoluted, but needs not to be untangled in the present context. Suffice it to say, with Gegenbaur (1870: 441), that “among fishes, [the archipterygium] is relatively most completely manifest in sharks, chimaras, and in the Dipnoi, in the latter with the least modifications.” But here is the problem: dipnoans (lungfish), classified as “bony fishes” (Osteichtyes), rank higher in Gegenbaur’s scale of gnathostome vertebrates than sharks, and yet preserve the archipterygium in a less modified condition of form than sharks do.
In most general terms, the archipterygium is an axially organized appendage (Figure 1). It is characterized by a central axis formed by a series of elements (mesomeres) that extend from the base of the fin into its distal tip. In Neoceratodus, radials fan out from this axis in two series, a preaxial and a postaxial one. This is Gegenbaur’s biserial (zweizieilig) archipterygium. Originally, and on the basis of the endoskeleton of the paired fins in sharks, Gegenbaur thought the uniserial archipterygium to represent the archetypical condition. Once he saw Günther’s (1871b: 224; Figure 1) illustration of the pectoral fin of Neoceratodus, he changed his mind, taking the biserial archipterygium as the archetypical configuration and set out for a search of remaining traces of the biserial archipterygium in sharks. Identifying such intermediate conditions of form, he declared the uniserial archipterygium in sharks to be the result of a process of reduction.
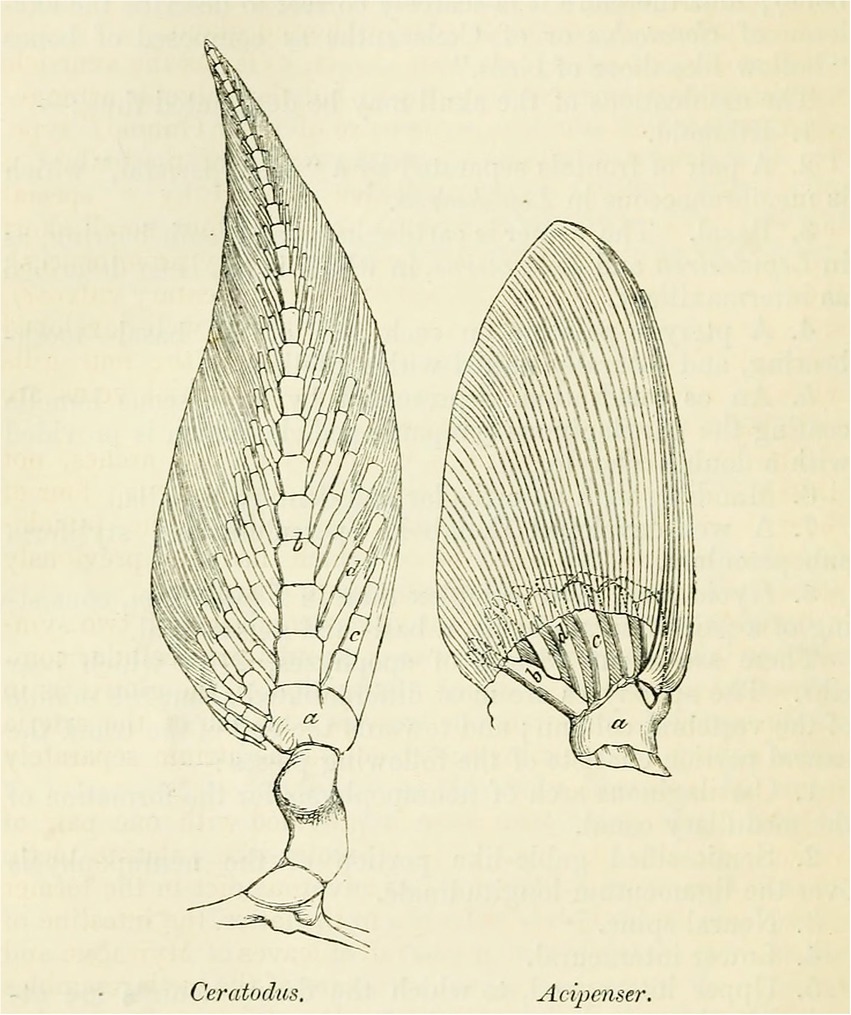
Figure 1. Günther’s rendition of the pectoral fin of the Australian lungfish “Ceratodus” (Neoceratodus) compared to that of the sturgeon Acipenser (from Günther, 1871b, p. 224).
“There are … in the pectoral fin of some sharks remains of a series of cartilaginous fin rays articulating on the medial aspect of the fin axis, which are more conspicuously developed in juvenile stages than in adult animals, and which therefore undergo reduction in the course of individual development. From there follows a partial correspondence to the fin skeleton of Ceratodus, such that a comparison of the two [i.e., Ceratodus and sharks] can be entertained” (Gegenbaur, 1873: 137).
“The persistence of the archipterygium in the fin skeleton of Ceratodus, as well as in part of the skeleton of the pectoral fin in some sharks, testifies to an originally widely distributed occurrence of this [archetypal] form” (Gegenbaur, 1873: 140). This follows from the fact that Neoceratodus ranks higher in the gnathostome scale than sharks, yet preserves the archipterygium in its most original form. Günther (1871a) (see Figure 1) had compared the pectoral fin of Neoceratodus with that of the sturgeon (Acipenser), to which Gegenbaur (1879) answered that selachians would have provided a better basis for comparison. Acipenser, an osteichthyan, again ranks higher than sharks on Gegenbaur’s scale of gnathostome vertebrates, but Gegenbaur (1879) admitted that the sturgeon also retains some very primitive features, as for example in its vertebral column.
“As far as sturgeons are concerned, one thing can be asserted with confidence, namely that they represent forms appearing later [in time] than sharks, with whom they share numerous similarities. But it would be erroneous to conclude therefrom, that all organ systems [of sturgeons] have undergone further progressive differentiation. This would be refuted by the condition of the vertebral column in sturgeons already” (Gegenbaur, 1879: 522).
Classified as bony fishes (osteichthyans), sturgeons rank higher in the scale of gnathostome vertebrates than sharks, and also appear later in time in earth history (in the fossil record), so they must be expected to have undergone further progressive transformation. While this is apparent in many traits of sturgeons, it is nevertheless not true of all their traits: the vertebral column, for example, remains in a very primitive stage of differentiation. Again, an evolutionary advanced organism is thus found to retain some very primitive parts in its anatomy, or, to put it differently: in sturgeons, as also in Ceratodus, advanced features of the anatomy mix with retained primitive features.
As Werth and Shear (2014: 438) said of living fossils: they are “mosaics combining older, retained features with newer, specialized ones.” This phenomenon ultimately underlies the potential for the “mixing of specializations” that is today technically known as the “heterobathmy of characters” (Takhtajan, 1959: 13; Hennig, 1965: 107; see discussion in Nelson, 2004). The concept of “living fossils” focuses attention on the retention of primitive features over long periods of (geologic) time, and motivates the search for a causal explanation for such evolutionary stasis in both genomic and phenomic traits. The concept of the “heterobathmy of characters” in contrast focuses attention on specialized traits, and their significance in an evolutionary context. The two concepts complement each other, as organisms generally exhibit a mosaic of primitive and specialized traits.
4. The heterobathmy of characters
The Belgian paleobiologist Louis Dollo (1857–1931) spoke of the chevauchement des spécialisations, the “overlap of specializations” when he described the “mixing” of primitive and specialized characters in an essay on the phylogeny of lungfishes:
“In reality, and in a general sense, it is always extremely rare that one can point to the real terms of descent in a direct linear succession; this is because of the insufficiency of the paleontological documents, and because of the overlap of specializations” (Dollo, 1895: 88).
He continued with remarks on the evolution of horses, noting that Hipparion is more specialized in its dentition than Equus, whereas Equus is more specialized in its limbs than Hipparion (Dollo, 1895: 88). This means that the series “Palaeotherium,—Anchitherium,—Hipparion,—Equus does not represent the ascent of horses in a direct line of ancestry and descent, but the series nevertheless illustrates very well the evolution of horses in its essential traits” (Dollo, 1895: 88). Dollo’s claim then was that the chevauchement des spécialisations forces us to think of a branching pattern of order in nature, rather than search for a linear sequence of ancestors and descendants.
It was Dollo’s younger admirer, friend and fellow paleobiologist Othenio Abel (1875–1946) from Vienna who most prominently picked up this thread of thought, and fleshed it out more completely (Rieppel, 2013). Looking for the seriability of phenomena in the natural world in search for underlying lawfulness (Abel, 1929: 51), Abel found that organisms–fossil and extant—can be arranged in transformation series from three different perspectives: adaptational (functional), morphological, and phylogenetic (Abel, 1913). An adaptational series (Anpassungsreihe), as is exemplified by the evolution of the limbs and dentition of horses, cannot be interpreted in genealogical terms as a sequential series of ancestors and descendants, as it may be marred by convergence. But it does allow the distinction of the more general (“primitive”) from the more specialized (“derived”) condition of form. But if the series of adaptational stages runs parallel to the appearance of these stages in the fossil record, “then at least the suspicion of a genealogical connection will be aroused” (Abel, 1913: 120). Should such suspicion be strengthened by ongoing research, the adaptational series acquires the status of a Stufenreihe, a morphological series of transformation rooted in phylogeny. It is at this juncture that the recognition of Spezialisationskreuzungen—the “crossing of specializations,” as Abel called Dollo’s chevauchement des spécialisations—becomes crucial. The possibility of a crossing of specializations implies the necessity to distinguish Stufenreihen (a sequential series of morphological transformation) from Ahnenreihen (a sequential series of ancestors and descendants). Abel’s (1913: 122) corresponding laws read:
1. If all the Stufenreihen within a group of species run parallel to one another, then every Stufenreihe at the same time also instantiates the Ahnenreihe.
2. But if, within a group of species, even only a single Stufenreihe of the investigated organs conflicts with all the others, then the forms in question cannot be considered to represent an Ahnenreihe.
Abel illustrated the consequences of these laws in two ways, one formal, one empirical, but both reflecting the consequences of the crossing of specializations. A Stufenreihe corresponds to a sequential series of morphological transformation from a more general to a less general, i.e., specialized condition of form. If the reconstruction of Stufenreihen for several, or better still many morphological traits results in the same sequential order of the genera under investigation for all the traits considered, then each Stufenreihe may be considered to also represent the Ahnenreihe, the sequence of ancestors and descendants among the genera under consideration. But if the transformation series of one morphological trait places four stipulated genera into the series A, C, D, B, and the transformation series of a second morphological trait orders the same genera into the series A, D, B, C, then the inferred phylogeny of the two traits cannot also depict the phylogeny of the genera that exhibit these traits (Abel, 1911: 246). The four genera can in no way form a lineal sequence of descent. Later, Abel (1929) illustrated this fundamental insight with reference to two mysticete whale families, the Balaenidae and the Balaenopteridae. The balaenids he found to combine a primitive forelimb skeleton with specializations in the cervical vertebrae, whereas the balaenopterids retain the primitive condition of the cervical vertebral column, but show specializations in the skeleton of the forelimb (Abel, 1929: 266f). The conclusion he drew was the same that Dollo (1895) had arrived at with his study of lungfish phylogeny: balaenids cannot be ancestral to the balaenopterids, nor can balaenopterids be ancestral to balaenids. The only option left is that the two families are derived from a hypothetical common ancestor. The heterobathmy of characters, the “mixing” of specializations, seemingly pits the branching tree of life against the linear sequence of descent.
5. Ancestors vs. sister-groups
The most important impact Dollo’s (1895: 88) chevauchement des spécialisations, the Spezialisationskreuzungen of Tschulok (1922: 205), Abel (1913), and Hennig (1950: 142), or the heterobathmy of characters of Takhtajan (1959: 13) and Hennig (1965: 107) had was to rob fossils of their privileged ontological status in phylogeny reconstruction. It has often been claimed that fossils provide the only direct clues to the history of life on earth. This claim in turn was grounded in the belief that phylogeny could be directly read off the fossil record.
In the year 1876 Thomas Henry Huxley (1825–1895), paleontologist and Professor of Natural History at the Royal School of Mines in London, traveled through parts of the United States—or “Yankee-land” as he called it (Randel, 1970: 97)—the primary purpose being a reunion with his sister in Nashville, Tennessee. Newspaper reports of his arrival in New York announced that he would deliver three lectures there on September 18, 20, and 22, 1876, “the subject being ‘Direct Evidence of Evolution’” (Randel, 1970: 78). Among the people enthusiastically greeting Huxley as he stepped off board the Germania was the paleontologist Othniel Charles Marsh (1831–1877) from the Peabody Museum of Yale University, who ushered Huxley to New Haven to show him his rich collection of fossils, notably the remains of fossil horses. In his third lecture held in New York, Huxley displayed a diagram provided by Marsh of fossil horse limbs and teeth representing specimens on display at the Peabody Museum that offered compelling insight into the continuous, step-wise transformations that were said to characterize the horse lineage through geologic time (Figure 2). “Thus, thanks to these important researches, it has become evident that … the history of the horse-type is exactly and precisely that which could have been predicted from the knowledge of the principles of evolution” (Huxley, 1877: 89). The claim implicit in this conclusion was that the course of evolution can be read off directly from the fossil record: “That is what I mean by demonstrative evidence of evolution” (Huxley, 1877: 90). On 19 March 1880, Huxley (1880b) addressed the Royal Institution in London in a celebration of the 20th birthday of Darwin’s book On the Origin of Species. He reiterated his firm belief that “primary and direct evidence in favor of Evolution can be furnished by palaeontology” (Huxley, 1880b: 3), and added: “if the doctrine of Evolution had not existed paleontologists must have invented it” (Huxley, 1880b: 4). Marsh (1879: 505) eventually published his illustration of the gradual, step-wise evolution of horses from a four-toed ancestral condition (Orohippus, Eocene) to the single-toed condition in living horses (Equus), in a paper issued in 1879. He had, however, already worked out the “natural line of descent” as illustrated by the North American fossil record of horses by 1874 (Marsh, 1874: 2655): “The line of descent appears to have been direct, and the remains now known supply every important intermediate form” (Marsh, 1874: 258). “Marsh’s illustration … has been reproduced … countless times in books dealing with paleontology, evolution, and natural history, and even today is seen in college-level textbooks in geology and zoology” (MacFadden, 1992: 39). Stephen J. Gould (1941–2002) called Marsh’s illustration “one of the most famous illustrations in the history of paleontology” (Gould, 1987: 18). In the end, however, the picture was recognized as misleading. George Gaylord Simpson (1902–1984), a pioneer in deciphering the complex phylogenetic history of the horse and its fossil relatives, recognized a pattern that shows “considerable phyletic branching, with at least 12 branches, aside from the direct Equus ancestry” (Simpson, 1944: 158). “There does not seem to be even one distinct character that evolved continuously in one direction among all the Equidae” (Simpson, 1944: 159).
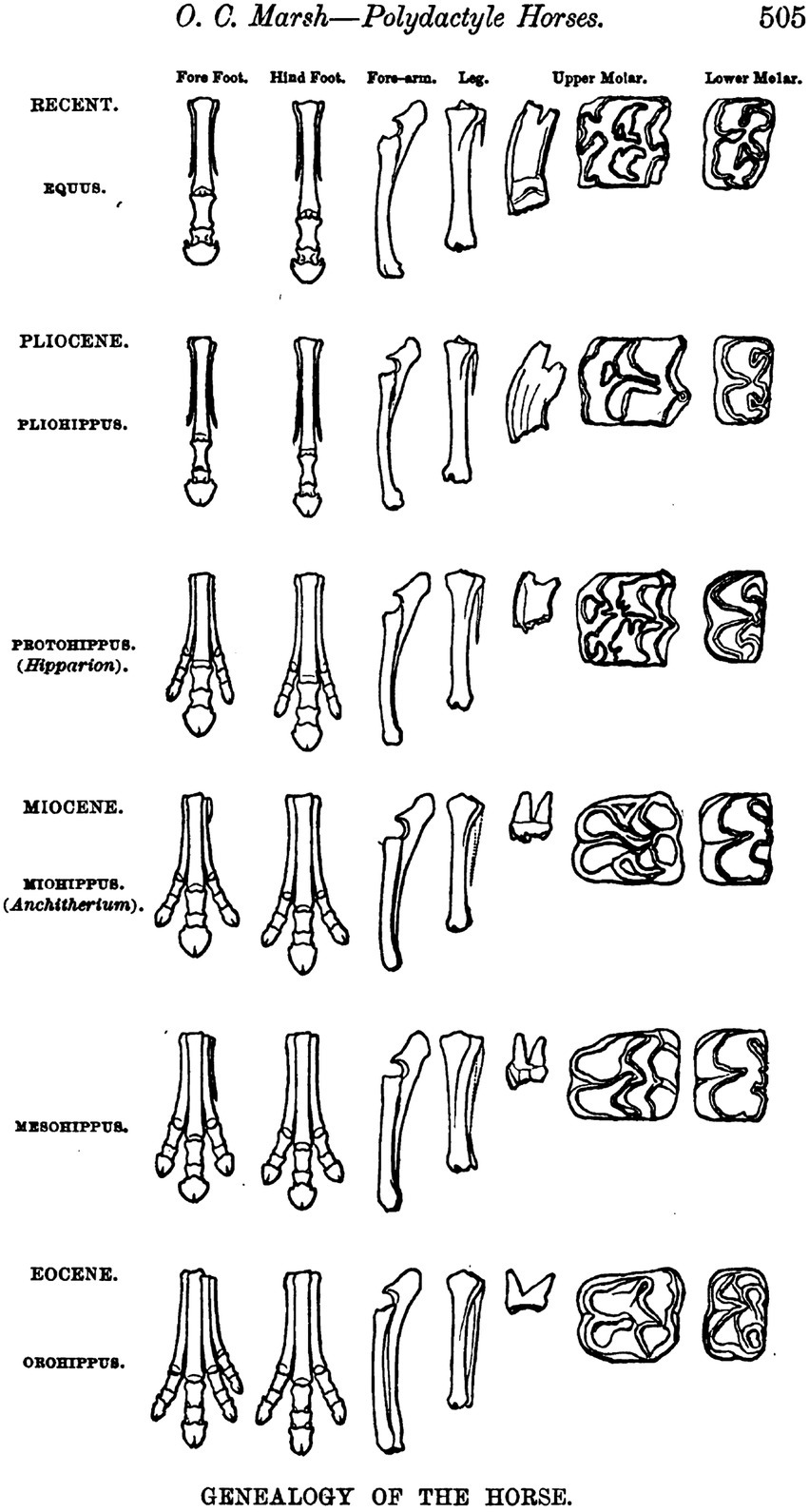
Figure 2. Othniel Marsh’s illustration of the “Genealogy of the Horse” in North America (from Marsh, 1879, Plate following page 504).
Too often, Abel (1920: 20) complained, has it been the case that putative ancestral forms were later recognized as side-branches of the phylogenetic tree (“it is easy to combat Haeckel’s Ahnenreihe of hominids …”: Abel, 1911: 245). The heterobathmy of characters spoilt the search for a linear sequence of ancestors and descendants stretching through geologic times, and enforced tree-thinking instead. Biodiversity originates from the sequential splitting of an ancestral species lineage, resulting in two descendant daughter species (relative to the ancestral species), or sister species (relative to one another). In the process, different organs evolve at different rates, which in turn gives rise to the “mixing” of specializations, the heterobathmy of characters also sometimes referred to as mosaic evolution (DeBeer, 1954; Werth and Shear, 2014). Sinai Tschulok (1875–1945), a pioneer of phylogenetic systematics (Rieppel, 2010), was keenly aware of conflicting character distribution, which he called the “transgression” of characters (Tschulok, 1922: 147). His proposal to untangle phylogenetic relationships in the face of such transgression of characters was: “the conditio sine qua non for the reconstruction of phylogenetic trees is the distinction of the primitive and derived condition of form” (Tschulok, 1922: 197). Recognizing further that the derived characters themselves show a conflicting distribution (Spezialisationskreuzungen: Tschulok, 1922: 205), Tschulok concluded that phylogeny cannot be read off the fossil record: “phylogenetic trees are by no means a ‘directly historical’ affair … the mere record of the temporal distribution [of taxa] cannot possibly allow the ‘direct assessment’ of phylogenetic history” (Tschulok, 1922: 242). The reason is that the distribution of derived characters blurs the relations between ancestors and descendants, as Abel (1911, 1929) had similarly realized. Tschulok (1922: 195) consequently reduced phylogeny reconstruction from the search of “direct ancestors” to the specification of “possible ancestors.” Willi Hennig (1913–1976), the acclaimed “founder” of phylogenetic systematics, went beyond Tschulok with his claim that the search for ancestors and descendants should be abandoned altogether, and replaced with a search for sister-group relationships on the basis of shared derived characters (Hennig, 1950, 1965, 1966): “Heterobathmy is therefore a precondition for the establishment of the phylogenetic relationships of species and hence a phylogenetic system” (Hennig, 1965: 107). Species A is more closely related to species B than either is to species C if the sister species A and B share a hypothetical common ancestral species that is not also ancestral to C. These relationships can be hypothesized if it can be shown that A and B share one or several derived character(s) that is/are not also shared by C.
6. Conclusion
Both Günther (1871a) and Teller (1891) stressed the close similarities Neoceratodus shares with Devonian lungfish, the result of “the most remarkable example of persistence of organization not in Fishes only, but in Vertebrates” (Günther, 1871a: 561). Both Westoll (1949: 173) and Simpson (1953: 23) likewise identified a remarkable degree of morphological stasis (bradytely: Simpson, 1944: 133) in post-Devonian lungfish following a relatively rapid diversification (tachytely: Simpson, 1944: 134) of the dipnoans during the Middle to the Late Devonian. “Are lungfish living fossils?” (Lee et al., 2006: 306). Lee et al. (2006: 312) found that “from a molecular evolution standpoint, the lungfishes do appear to be ‘living fossils’,” as their genome evolved at a lower rate in comparison to tetrapod genomes. However, when it comes to the genome as well as the phenome, relative rates of evolutionary change come in a mixed bag, with important theoretical consequences. The heterobathmy of characters forced the replacement of the search for lineal sequences of ancestors and descendants by the reconstruction of a branching phylogeny. As the search for ancestors was replaced by the search for sister-group relationships, fossils lost their privileged ontological status as the only direct evidence of evolution through geologic time. With that loss, the special evolutionary significance of the ‘living fossil’ vanished as well.
Darwin (1859: 486) had hoped that living fossils “will aid us in forming a picture of the ancient forms of life.” Today, living fossils are viewed as taxa that retain “a few prominent primitive traits” (Schopf, 1984: 246), or traits which have not changed “over long intervals of geologic time” (Schopf, 1984: 285). Indeed, the contemporary discussion of living fossils turns largely on relative rates in character evolution, both genomic and phenomic (see the contrasting discussion of coelacanths as living fossils: Casane and Laurenti, 2013; Cavin and Guinot, 2014). As living fossils are characterized by the retention of some strikingly ‘primitive’ or ancient features, these are nevertheless mixed with specializations of their own (e.g., the endoskeleton of the pectoral fin in the extant coelacanth: Friedmann et al., 2014). Just as any other taxon, fossil or extant, living fossils are subject to the heterobathmy of characters. For them to come out as surviving archaic relicts, or as a window into deep time as Darwin had hoped, they need to first find their proper place in the tree of life. Their place as terminal taxa at the tip of the branches of the phylogenetic tree according to their sister-group relationships will be determined by the derived (specialized) characters they share with their nearest neighbor Casane and Laurenti, 2013.
Data availability statement
The original contributions presented in the study are included in the article, further inquiries can be directed to the corresponding author.
Author contributions
The author confirms being the sole contributor of this work and has approved it for publication.
Acknowledgments
The author thanks Scott Lidgard and Alan Love for the invitation to contribute to the special issue of Frontiers in Earth Science on living fossils.
Conflict of interest
The author declares that the research was conducted in the absence of any commercial or financial relationships that could be construed as a potential conflict of interest.
Publisher’s note
All claims expressed in this article are solely those of the authors and do not necessarily represent those of their affiliated organizations, or those of the publisher, the editors and the reviewers. Any product that may be evaluated in this article, or claim that may be made by its manufacturer, is not guaranteed or endorsed by the publisher.
References
Abel, O. (1911). “Die Bedeutung der fossilen Wirbeltiere für die Abstammungslehre” in Die Abstammungslehre. Zwölf gemeinverständliche Vorträge über die Deszendenztheorie im Licht der neueren Forschung. ed. R. Hertwig (Jena: Gustav Fischer), 198–250.
Abel, O. (1913). Neuere Wege phylogenetischer Forschung. Verhandlungen der Gesellschaft Deutscher Naturforscher und Aerzte 85, 116–124.
Casane, D., and Laurenti, P. (2013). Why coelacanths are not living fossils. Bioessays 35, 332–338. doi: 10.1002/bies.201200145
Cavin, L., and Guinot, G. (2014). Coelacanths as “almost living fossils.”. Front. Ecol. Evol. 2, 1–5. doi: 10.3389/fevo.2014.00049
Cavin, L., and Kemp, A. (2011). The impact of fossils on the evolutionary distinctiveness and conservation status of the Australian lungfish. Biol. Conserv. 144, 3140–3142. doi: 10.1016/j.biocon.2011.08.014
Conant, E. B. (1986). An historical overview of the literature of Dipnoi: introduction to the biography of lungfishes. J. Morphol. 190, 5–13. doi: 10.1002/jmor.1051900404
De Castelnau, F. L. (1876). Mémoire sur les poissons appelés barramundi par les aborigènes du nord-est de l’Australie. J. Zool. 5, 129–136.
Dollo, L. (1895). Sur la phylogénie des dipneustes. Bulletin de la Société Belge de Géologie, de Paléontologie, et d’Hydrologie 9, 79–128.
Fitzinger, L. J. (1837). Vorläufiger Bericht über eine höchst tinteressante Entdeckung des in Brasilien befindlichen Dr. Natterer. Isis 1837, 379–380.
Friedmann, M., Coates, M. I., and Anderson, P. (2014). First discovery of a primitive coelacanth fin fills a major gap in the evolution of lobed fins and limbs. Evol. Dev. 9, 329–337. doi: 10.1111/j.1525-142X.2007.00169.x
Gegenbaur, C. (1870). Ueber das Skelet der Gliedmaassen der Wirbelthiere. Jena. Z. Med. Naturwiss. 5, 397–447.
Gegenbaur, C. (1876). Zur Morphologie der Gliedmassen der Wirbelthiere. Gegenbaurs Morphol. Jahrb. 2, 396–420.
Gould, S. J. (1987). Life’s little joke. The evolutionary histories of horses and humans share a dubious distinction. Nat. Hist. 96, 16–25.
Günther, A. (1871a). Description of ceratodus, a genus of ganoid fishes, recently discovered in rivers of Queensland, Australia. Philos. Trans. R. Soc. Lond. A 161, 511–571. doi: 10.1098/rstl.1871.0020
Günther, A. (1871b). Ceratodus, and its place in the system. Annals Magaz. Nat. Hist. 7, 222–227. doi: 10.1080/00222937108696351
Haeckel, E. (1870). Natürliche Schöpfungsgeschichte. Zweite, verbesserte und vermehrte Auflage. Georg Reimer, Berlin.
Hennig, W. (1950). Grundzüge einer Theorie der Phylogenetischen Systematik. Deutscher Zentralverlag, Berlin.
Hennig, W. (1965). Phylogenetic systematics. Annu. Rev. Entomol. 10, 97–116. doi: 10.1146/annurev.en.10.010165.000525
Huxley, T. H. (1877). American addresses, with a lecture on the study of biology. Macmillan and Co., London.
Huxley, T. H. (1880a). On the application of the laws of evolution to the arrangement of the vertebrata and more particularly of the Mammalia. Proc. Zool. Soc. London, 649–662.
Huxley, T. H. (1880b). The coming of age of the origin of species. Nature 22, 1–4. doi: 10.1038/022001a0
Kemp, A. (1986). The biology of the Australian lungfish, Neoceraodus forsteri. J. Morphol. 190, 181–198. doi: 10.1002/jmor.1051900413
Kemp, A., and Molnar, R. E. (1981). Neoceratodus forsteri from the lower cretaceous of New South Wales. J. Paleo. 55, 211–217.
Krefft, G. (1870). Description of a gigantic amphibian allied to the genus Lepidosiren, from the Wide-Bay district, Queensland. Proc. Zool. Soc. London 1870, 221–224.
Kutschera, U., Levit, G. S., and Hoßfeld, U. (2019). Ernst Haeckel (1834-1919): the German Darwin and his impact on modern biology. Theory Biosci. 138, 1–7. doi: 10.1007/s12064-019-00276-4
Lee, J., Alrubaian, J., and Dores, R. M. (2006). Are lungfish living fossils? Observation on the evolution of the opioid/orphanin gene family. Gen. Comp. Endocrinol. 148, 306–314. doi: 10.1016/j.ygcen.2006.07.010
MacFadden, B. J. (1992). Fossil horses. Systematics, Paleobiology and evolution of the family Equidae. Cambridge University Press, Cambridge.
Marsh, O. C. (1874). Notice of new equine mammals from the tertiary formation. Am. J. Sci. Arts 3, 247–258.
Marsh, O. C. (1879). Polydactyle horses, recent and extinct. Am. J. Sci. s3-17, 499–505. doi: 10.2475/ajs.s3-17.102.499
Natterer, J. (1837). Lepidosiren paradoxa eine neue Gattung aus der Familie der fischähnlichen Amphibien. Annalen des Wiener Museums der Naturgeschichte 2, 165–170.
Nelson, G. (2004). “Cladistics: its arrested development” in Milestones in systematics. eds. D. M. Williams and P. L. Forey (Boca Raton: CRC Press), 127–147.
Nyhart, L. K. (2003). The importance of the ‘Gegenbaur school’ for German morphology. Theory Biosci. 122, 162–173. doi: 10.1007/s12064-003-0051-x
Rieppel, O. (2010). Sinai Tschulok (1875-1945) – a pioneer of cladistics. Cladistics 26, 103–111. doi: 10.1111/j.1096-0031.2009.00274.x
Rieppel, O. (2011). The Gegenbaur transformation: a paradigm change in comparative biology. Syst. Biodivers. 9, 177–190. doi: 10.1080/14772000.2011.602754
Rieppel, O. (2013). Othenio Abel (1875-1946) and the “phylogeny of the parts.”. Cladistics 29, 328–335. doi: 10.1111/j.1096-0031.2012.00428.x
Schopf, T. J. M. (1984). Rates of evolution and the notion of “living fossils”. Annu. Rev. Earth Planet. Sci. 12, 245–292. doi: 10.1146/annurev.ea.12.050184.001333
Teller, F. (1891). Ueber den Schädel eines fossilen Dipnoers, Ceratodus Sturii nov. spec., aus den Schichten der oberen Trias der Nordalpen. Abhandlungen der Kaiserlich-Königlichen Geologischen Reichsanstalt 15, 1–38.
Werth, A. J., and Shear, W. A. (2014). The evolutionary truth about living fossils. Am. Sci. 102, 434–443.
Keywords: evolution, living fossils, heterobathmy, lungfish, Neoceratodus
Citation: Rieppel O (2023) “Living fossils” and the mosaic evolution of characters. Front. Ecol. Evol. 11:1119418. doi: 10.3389/fevo.2023.1119418
Edited by:
Alan C. Love, University of Minnesota Twin Cities, United StatesReviewed by:
Ronald Jenner, Natural History Museum, United KingdomJoyce Havstad, The University of Utah, United States
Copyright © 2023 Rieppel. This is an open-access article distributed under the terms of the Creative Commons Attribution License (CC BY). The use, distribution or reproduction in other forums is permitted, provided the original author(s) and the copyright owner(s) are credited and that the original publication in this journal is cited, in accordance with accepted academic practice. No use, distribution or reproduction is permitted which does not comply with these terms.
*Correspondence: Olivier Rieppel, b3JpZXBwZWxAZmllbGRtdXNldW0ub3Jn