- 1Department of Biology, University of Western Ontario, London, ON, Canada
- 2Environment and Climate Change Canada, Saskatoon, SK, Canada
Insect species that are nectivorous as adults acquire essential fatty acids almost exclusively from host plants during larval development. Thus, as essential fatty acids are important for a number of different biological processes, adult allocation of this limited resource may result in important trade-offs. Most lepidopteran species that migrate do so as sexually immature adults, so essential fatty acids used for migratory flight would not be available for subsequent reproduction. Using the true armyworm, Mythimna unipuncta, as a model system we analyzed fat body samples to test the hypothesis that environmental cues would influence the use of essential fatty acids during migratory flight. We used diets manipulated isotopically to trace origins and use of stored lipids and used chromatographic analyses to determine fatty acid composition. In the first experiments, 5-day old moths that had been reared in summer or fall (migratory) conditions and were force flown for different lengths of time (0–6 h) after which samples of the fat body were analyzed. Rearing conditions did not affect fatty acid loading however patterns of use during flight differed with essential fatty acids being conserved under fall but not summer conditions. As migratory flight can take several days, we repeated the experiment when 5-day old moths were flown for 8 h each day for up to 5 days. Some moths were provided access to sugar water after each flight while others were only given water or only given sugar water once. When sugar water was readily or sporadically available, moths reared under fall conditions conserved their essential fatty acids indicating that the environmental cues responsible for the onset of migratory flight result in physiological changes that modify lipid use. However, when moths had only water, the essential fatty acids were not conserved, highlighting the importance of nectar availability at stopovers for the conservation essential fatty acids during migration. Isotopic analysis of the moth fat body indicated a large contribution of adult-derived diet to lipids used as fuel. The implications of using isotopic approaches to other flight studies and future research on differential resource allocation in winged monomorphic migratory insects are discussed. Summary statement: Isotopic tracing methods and gas chromatography were used to demonstrate that environmental cues can impact patterns of fatty acid use in true armyworm moths. In particular, essential fatty acids are conserved during migratory flight. However, availability of adult food sources will determine the degree to which essential fatty acids are conserved.
1. Introduction
Diapause or seasonal migration in response to proximal cues indicating impending habitat deterioration, such as declining temperature, photoperiod, and host plant quality, are key components in the life history of many temperate insect species (Dingle, 1972, 2014; Saunders, 1987; Dixon et al., 1993). Whether entering a dormant state locally or emigrating to more favorable habitats, there is a need to acquire the appropriate energy reserves, as the failure to do so could result in death or a significant decline in the subsequent reproductive success of survivors.
Acquiring the appropriate energy reserves, predominantly in the form of lipids, is often associated with periods of hyperphagia (Beenakkers, 1969; Schneider and Dorn, 1994; McCue et al., 2015). The subsequent allocation of lipids during diapause or migration is important as they are also used for hormone synthesis, neuronal development, membrane integrity, defensive secretions, and reproduction (Stanley-Samuelson et al., 1988). Certain fatty acids (FAs) are considered as nonessential (NFAs) as they can be synthesized by the adult insect from other macronutrients, and so could be replaced through feeding post diapause or migration. However, others cannot be synthesized and are considered essential (EFAs) as they must be obtained directly from the diet. Thus, any EFAs used during diapause or migration that cannot be replaced could have negative consequences.
In the case of migratory Lepidoptera, the onset of migration is typically initiated by adults in reproductive diapause (Johnson, 1963; Dingle, 2014) and any subsequent replacement of lipids used during migration would have to be synthesized from nectar (i.e., carbohydrate) sources. However, as nectar only contains trace amounts of FAs (Nicolson and Thornburg, 2007; Krenn, 2010), the majority of EFA acquisition by Lepidoptera occurs during larval development. Thus, any EFAs used during migration would not be replaced. Previous studies on Lepidoptera have shown that EFAs are not conserved during flight under summer condition (Murata and Tojo, 2002; Sakamoto et al., 2004) but in their study comparing solitary and gregarious desert locusts (Schistocerca gregaria) (thus non migratory and migratory), Schneider and Dorn (1994) raised the possibility that EFAs may be conserved during migratory flight. If this were the case in migratory Lepidoptera, the conservation of EFAs during migratory flight would limit any negative post migration effects on reproduction. Therefore, we tested the hypothesis that the physiological changes in response to the environmental cues that induce the onset of migration would also influence the use of EFAs during flight. We predicted that adults reared under fall conditions would conserve EFAs while those under summer conditions would not.
We used the true armyworm, Mythimna unipuncta, a sporadic agricultural pest as a model system as it is a seasonal migrant (Guppy, 1961; Fields and McNeil, 1984; Hobson et al., 2018). Furthermore, previous research has shown that individuals reared under fall conditions have significant physiological differences than those reared under summer conditions, affecting sexual maturation, pheromone communication and lipid accumulation (Turgeon and McNeil, 1983; Delisle and McNeil, 1987; Cusson and McNeil, 1989). We reared insects under several different ecological conditions and then determined the fat body lipid content of newly emerged adults and 5-day old adults using gas chromatography and isotopically (δ13C) distinct larval and adult diets. In addition, moths were force flown for different lengths of time to determine if rearing conditions, flight duration and resource availability play a role in influencing fatty acid use during flight.
2. Methods
2.1. Insect colony
All individuals used were from a colony established using adults collected from light and pheromone traps (a minimum of 30 males and 30 females captured during the 2022 spring immigrant flight period) at the Environmental Sciences Western (ESW) Field Station (43.07°N, 81.34°W). The colony was maintained at 25°C, 16:8D, 70 ± 5% RH, with larvae being reared individually on a (C3: δ13C: ~−25.2‰) pinto bean diet (Shorey and Hale, 1965) while adults were provided an ad lib supply of 25% (C4: δ13C:~−12.2‰) cane sugar and tap water solution. The adults used for all experiments had not been in rearing for more than two generations.
Depending on the specific experiment, the larvae used were reared under the same laboratory conditions as the colony, or in an insectary under natural conditions in June/July and August/September, subsequently referred to as summer and fall conditions, respectively.
2.2. General protocol for fat body lipid extraction and chromatographic analysis
At the end of each experiment, adults were weighed (to nearest mg, Supplementary A2) and stored at −80°C until fat body tissue was analyzed. In all experiments, fat body tissue was excised (5–10 mg) and the lipids extracted in a 2:1 chloroform:methanol solution (Folch et al., 1957) containing 0.01% butylated hydroxytoluene. If they were used for GC analyses the extraction was performed under N2 flow to reduce oxidation of FAs. Twenty μL of an internal standard reference, margaric acid (17:0, 3 mg/mL), was added. One milliliter of 0.25% KCl was added to the sample to separate out aqueous solutes. The FAs were then converted into fatty acid methyl esters by adding 200 μL of 0.5 M methanolic hydrogen chloride and reacting at 90°C for 30 min. The solution was then reduced to dryness under nitrogen, resuspended in hexane and 100 μL samples subsequently analyzed using gas chromatography. Analyses were performed using a gas chromatography/flame ionization detector (Agilent Technologies® 6890 N G1530N, Santa Clara, United States) equipped with a DB23 column (Agilent DB23 122-2332, Santa Clara, United States). The injector temperature was 250°C and the flame ionization detector temperature was at 280°C. During each run, a 100 μL dichloromethane control was also included. The retention times of PUFA standards (Supelco® PUFA and 37 component) were averaged to create a library of known fatty acid peaks. The distinct and clear peaks of each sample chromatograph were compared to the known retention times to identify fatty acids. This, combined with the known concentration of the internal standard were used to calculate the concentration of each fatty acid (nmol/mL). When required separate fat body samples were used for isotopic analysis (see below).
2.3. General protocol for flight assays
The scales on the dorsal surface of the thorax were removed and a 3 cm piece of clear plastic tubing (2 mm diameter) was attached using EVO–STIK (Stafford, United Kingdom) instant contact adhesive. Each moth was then suspended from a copper wire placed perpendicular to flight apparatus base via the thoracic tether and force flown for the required period of time (Figure 1). If a moth stopped flying its back legs were gently touched with a camel hair brush but if the individual did not resume flight after three such stimulations it was not included in subsequent analyses.
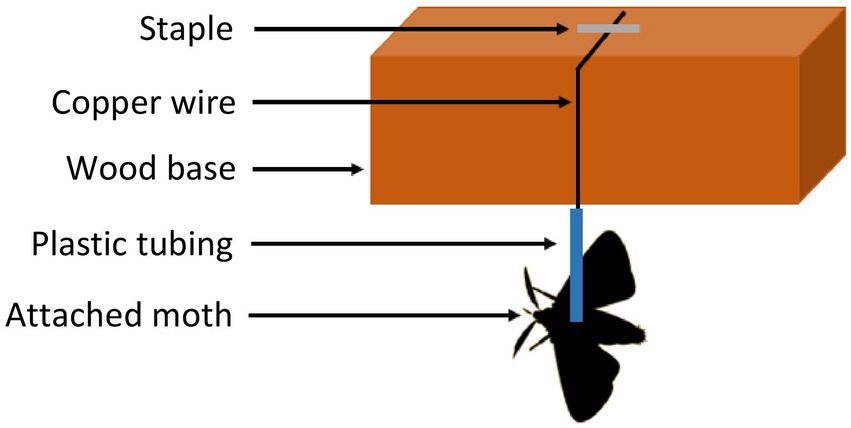
Figure 1. Set up used for forced flight experiments of tethered true armyworm moths. A video is provided as Supplementary Video S1.
2.4. Protocols of specific experiments
To test that the majority of lipid present in the fat body of mature individuals is acquired from adult resources, we reared cohorts under lab, summer, and fall conditions. Under each rearing condition 20 individuals (10 of each sex) were sacrificed at emergence and an equal number fed sugar water ad lib were sacrificed after 5 days. The fat body δ13C values of newly emerged individuals would reflect only the C3 larval diet while those of 5-day old individuals would also include the contribution from the C4 sugar water diet.
For δ13C analyses 0.91–0.99 mg of frozen lipid (see above) were placed into tin capsules (8×5 mm) and crushed. All prepared samples were shipped via expedited courier to the Cornell Isotope Laboratory (COIL, Ithaca, NY, United States) where isotopic measurements were performed. Here, crushed capsules were combusted at 1,000°C in a Carlo-Erba NC2500 Elemental Analyzer (Carlo Erba, Italy) and CO2 gas transferred via a ConFlo IV (Thermo Scientific, Bremen Germany) device to a Delta V Advantage Isotope Ratio Mass Spectrometer (Thermo Scientific, Bremen Germany). Data were normalized using internal calibrated lab standards (Cayuga brown trout: −25.58‰ and corn: −13.02‰); instrument linearity was assessed using in-house methionine (−27.2‰) and ground deer hair (−20.1‰) standards. All values are reported in standard delta (δ) notation relative to the Vienna Pee Dee Belemnite (VPDB) standard in parts per 1000 (‰). Measurement error based on within-run standards was estimated as ±0.1‰.
If lipids used during flight were principally of adult (C4) origin, then one would predict that as they were metabolized the δ13C values in the fat body would change, becoming closer and closer to the C3 larval diet value as a function of flight duration. To determine if this was the case, 10 five-day old adults (5 of each sex) were reared under either natural summer or fall conditions, then force flown for 0, 1, 4, or 6 h, after which time lipids were extracted from fat body tissue and prepared for δ13C isotopic analysis, as in the previous experiment.
Using the samples prepared for GC analyses, described above, we quantified the relative concentrations of specific FAs in fat body samples from the same individuals as the two previous experiments. We selected the NFAs oleic (OL), palmitic (PA), and stearic acid (ST), as they are found in high levels in Lepidoptera (Subramanyam and Cutkomp, 1987; Canavoso et al., 2001) and the EFAs alpha-linolenic acid (ALA) and linoleic acid (LA) as they cannot be de novo synthesized by Lepidopterans but are essential for adults, particularly for reproduction (Canavoso et al., 2001).
In light of the differences observed in EFA use between the summer- and fall-reared insects, and the fact that migratory flight generally occurs over more than one night, we conducted additional experiments using a cohort of 120 fall-reared individuals that were fed sugar water ad lib for 5 days. Then 20 (10 of each sex) were sacrificed without being flown, while the remaining 100 were flown for 8 h, after which 10 (5 of each sex) were sacrificed and fat body samples taken. A subset of 40 moths were flown 8h/day and fed sugar water ad lib after each flight period, with a subsample (five of each sex) sacrificed after 1, 2, 3, or 4 days. The same protocol was repeated with 40 moths only provided ad lib water after each flight. In addition, a subset of 20 moths receiving only water were provided sugar water ad lib once, after the third day of flight. Fat body samples were analysed, as described above, to determine the changes in relative FA concentrations as a function of the number of flight periods and the availability of adult resources.
2.5. Statistical analysis
All statistical tests (ANOVA, Tukey’s post-hoc analysis, unpaired t-tests) were performed using R Studio (Version 3.4.2 (2017-09-28)). ANOVA and Tukey’s post-hoc analysis were used to compare specific FA levels and δ13C values of the fat body (dependent variable) across different ages, rearing conditions and flight durations (independent variables). Unpaired t-tests were used to determine specific differences in FA patterns or δ13C values of the fat body between sexes. Normality of data was confirmed using Skew (−1 to +1) and Kurtosis (−4 to +4) analyses.
3. Results
While the δ13C values of the fat body from newly emerged adults reflected the C3 larval diet, after 5 days of ad lib feeding, the δ13C values increased regardless of rearing conditions (ANOVA, df, 5,112, F = 936.3, p < 0.001), and clearly reflected the addition of lipids derived from the C4 adult diet (Figure 2). In both age groups there were no differences in δ13C values of the fat body due to rearing conditions (Tukey HSD, Day 0 fall versus summer: p = 0.21, Day 0 fall versus lab: p = 0.99, Day 0 summer versus lab: p = 0.47, Day 5 fall versus summer: p = 0.93, Day 5 fall versus lab: p = 0.14, Day 5 summer versus lab: p = 0.17) or sex (ANOVA, df, 1,112, F = 0.631, p = 0.425). The concentration of total FAs increased significantly from day 0 to day 5 in non-flown adults across all rearing conditions (Table 1; ANOVA, df, 5,119, F = 27.72, p < 0.0001). This was due to the marked changes in the NFAs (Table 1; ANOVA, df, 5,119, FPA = 27.45, FOL = 26.52 FST = 8.74, p < 0.001), but there were no significant differences in FA concentrations between the three rearing conditions at either age (Tukey HSD, Day 0 fall versus summer: p = 0.99, Day 0 fall versus lab: p = 1.0, Day 0 summer versus lab: p = 1.0, Day 5 fall versus summer: p = 0.69, Day 5 fall versus lab: p = 0.25, Day 5 summer versus lab: p = 0.97). In contrast, while the concentration of the two EFAs did not differ between rearing conditions within any age group, both declined with age (Table 1; ANOVA, df, 5,119, FLA = 16.72, FALA = 21.48, p < 0.001).
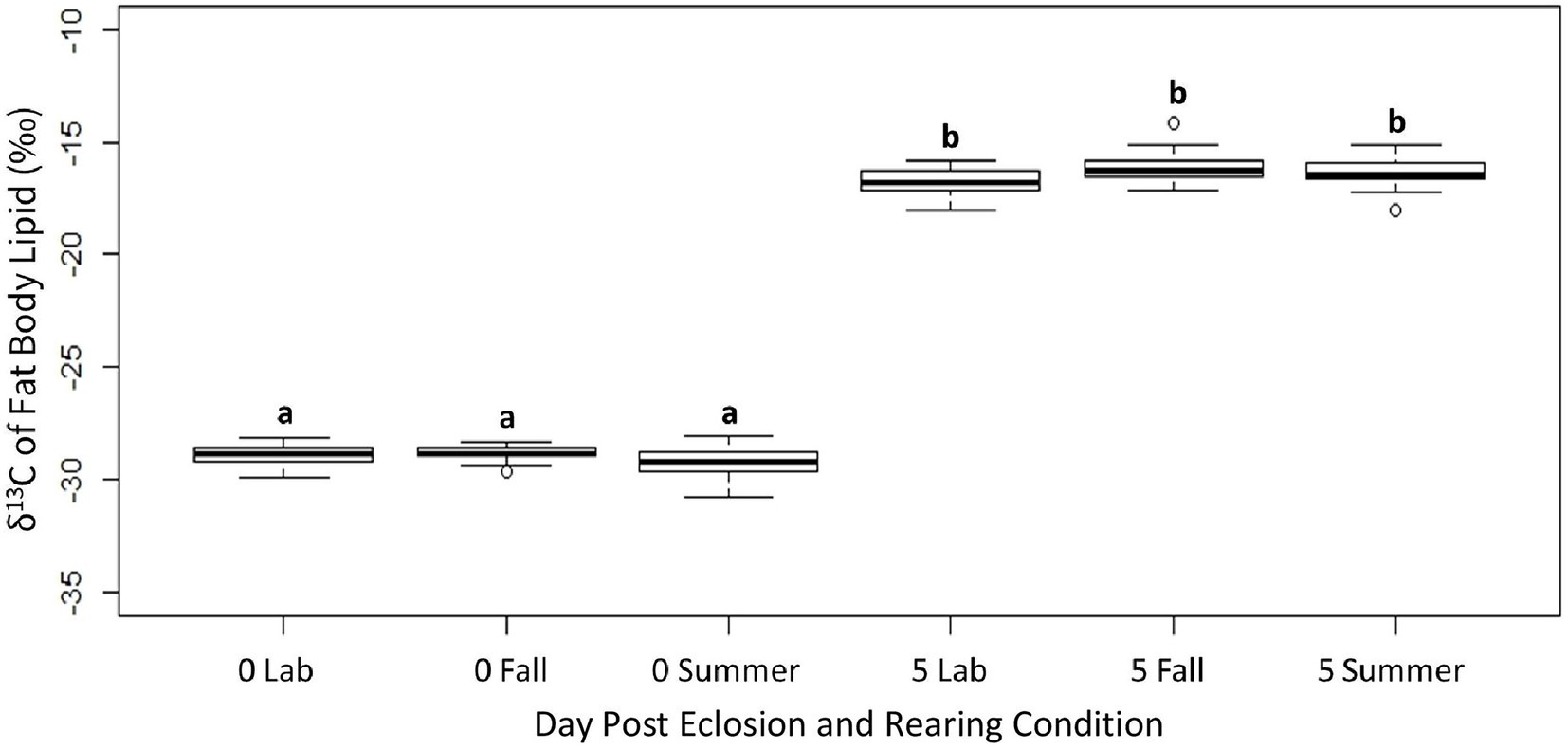
Figure 2. The δ13C values of the fat body from non-flown true armyworm adults fed sugar water ad lib as a function of age and rearing conditions. Significant differences are indicated by different letters (Tukey’s HSD, p < 0.05). N = 20 per sample group.
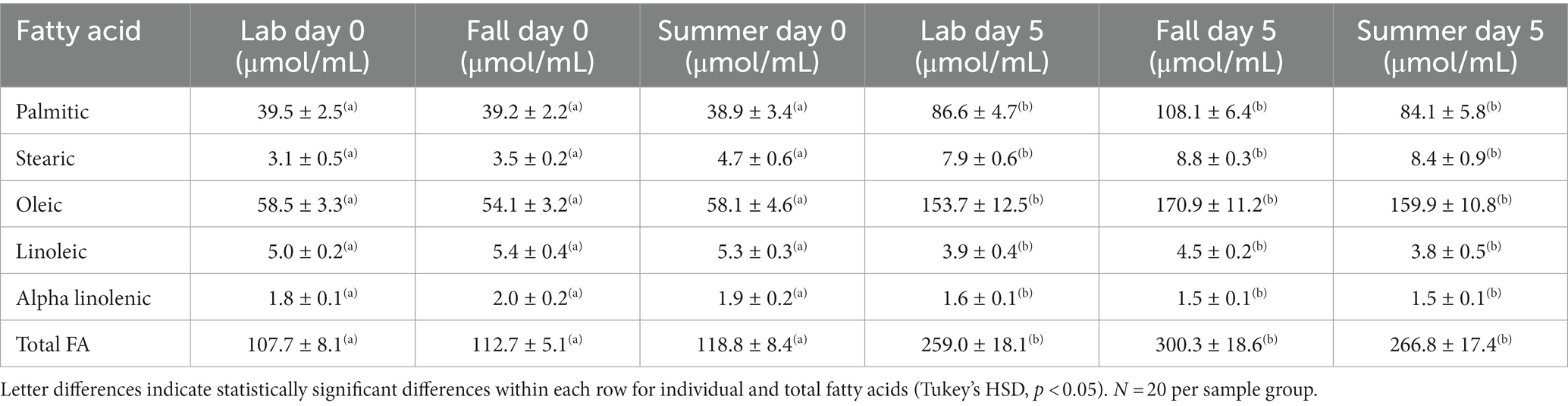
Table 1. Concentration of specific essential and non-essential fatty acids in the fat body of non-flown true armyworm adults as a function of age and rearing conditions.
There was a significant decline in the lipid δ13C values of 5-day old individuals as a function of flight duration under both rearing conditions, although the patterns differed. For moths reared under summer conditions, an overall decline was only significant after 6 h (Figure 3A; ANOVA, df, 3,49, F = 21.24, p < 0.05, Tukey HSD, p < 0.05) and was more pronounced in females than males (Supplementary A2; Unpaired t-test, t = 9.15, df = 1,19, p < 0.05). For fall moths a significant change was observed after 4 h (Figure 3B; ANOVA, df, 3,49, F = 21.634, p < 0.05, Tukey HSD, p < 0.05), and, as with summer moths, the change was more evident in females than males (Supplementary A3; Unpaired t-test, t4 = 3.84, t6 = 4.72 df = 1,19, p < 0.05).
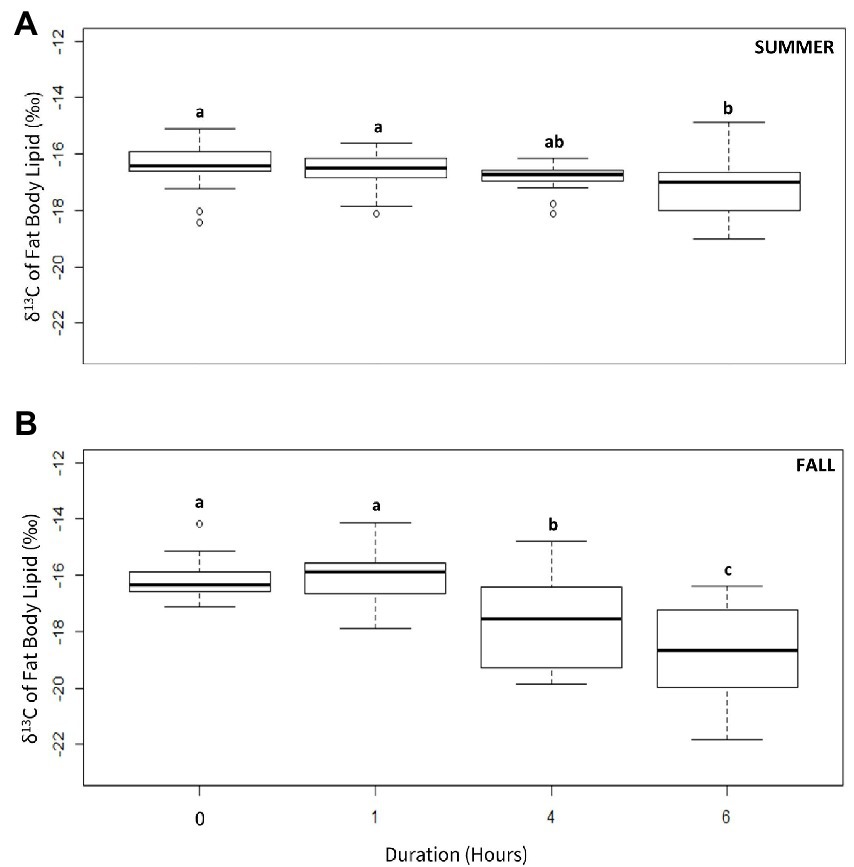
Figure 3. δ13C values of the fat body from 5-day old true armyworm moths after different periods of force flight when reared under (A) summer or (B) fall conditions. Letters indicate statistically significant differences in isotopic values as a function of flight time (Tukey’s HSD, p < 0.05). N = 10 per sample group (N = 20 in Day 5 no flight).
The total concentration of FAs declined as a function of flight duration in both summer (Table 2; ANOVA, df, 3,49, F = 10.321, p < 0.001, Tukey HSD, p < 0.05) and fall (Table 3; ANOVA, df, 3,49, F = 10.261, p < 0.001, Tukey HSD, p < 0.05) reared 5-day old moths. In summer moths, the decline in NFA was due to decreases in the concentrations of PA and OA, and both EFAs (Table 2; ANOVA, df, 3,49, FPA = 8.414 FOL = 6.301 FLA = 5.706 FALA = 4.910; p < 0.05, Tukey HSD, p < 0.05, for all). In all cases, the significant declines in FA concentration were observed after 4 h of sustained flight (Tukey HSD, p < 0.05). In fall-reared moths a significant decline in total FAs was detected after 6 h of sustained flight (Tukey HSD, p < 0.05), due to lower levels of all three NFA but with no change in the concentration of the two EFAs (Table 3; ANOVA, df, 3,49, FPA = 10.331, FST = 7.654, FOL = 9.864, p < 0.01, Tukey HSD, p < 0.05; FLA = 0.671, FALA = 1.802 PLA = 0.67, PALA = 0.31).

Table 3. Change in the concentration of essential and nonessential fatty acids in the fat body of 5- day old true armyworm moths as a function of flight duration under fall conditions.

Table 2. Change in the concentration of essential and nonessential fatty acids in the fat body of 5-day old true armyworm moths as a function of flight duration under summer conditions.
During multiple days of flight, the concentration of total FAs increased after the first day of flight and remained stable when fall moths were fed ad lib. This was due to an increase in OL, as the concentrations of the other NFAs and EFAs did not change significantly (Figures 4A, 5A; ANOVA, df, 5,69, FPA = 0.957, FST = 0.965, FLA = 0.512 FALA = 0.487, PPA = 0.42, PST = 0.41 PLA = 0.49 PALA = 0.51; Ftotal = 4.759, FOL = 5.704; p < 0.05). In contrast, when moths were only provided water during the rest periods there was an overall decline in the total concentration of FAs. This was due to lower levels of all NFAs and EFAs, with most significant differences being observed after 4 days of forced flight (Figures 4B, 5B; ANOVA, df, 5,69 F = 4.751, FPA = 6.847, FST = 2.533, FOL = 5.147, FLA = 3.716 FALA = 4.446, p < 0.05). However, if moths were provided ad lib sugar water after the third night of flight, the levels of total FAs remained stable on days 8 and 9 (Figures 4C, 5C; ANOVA, df, 2,29 F = 1.727, p = 0.33).
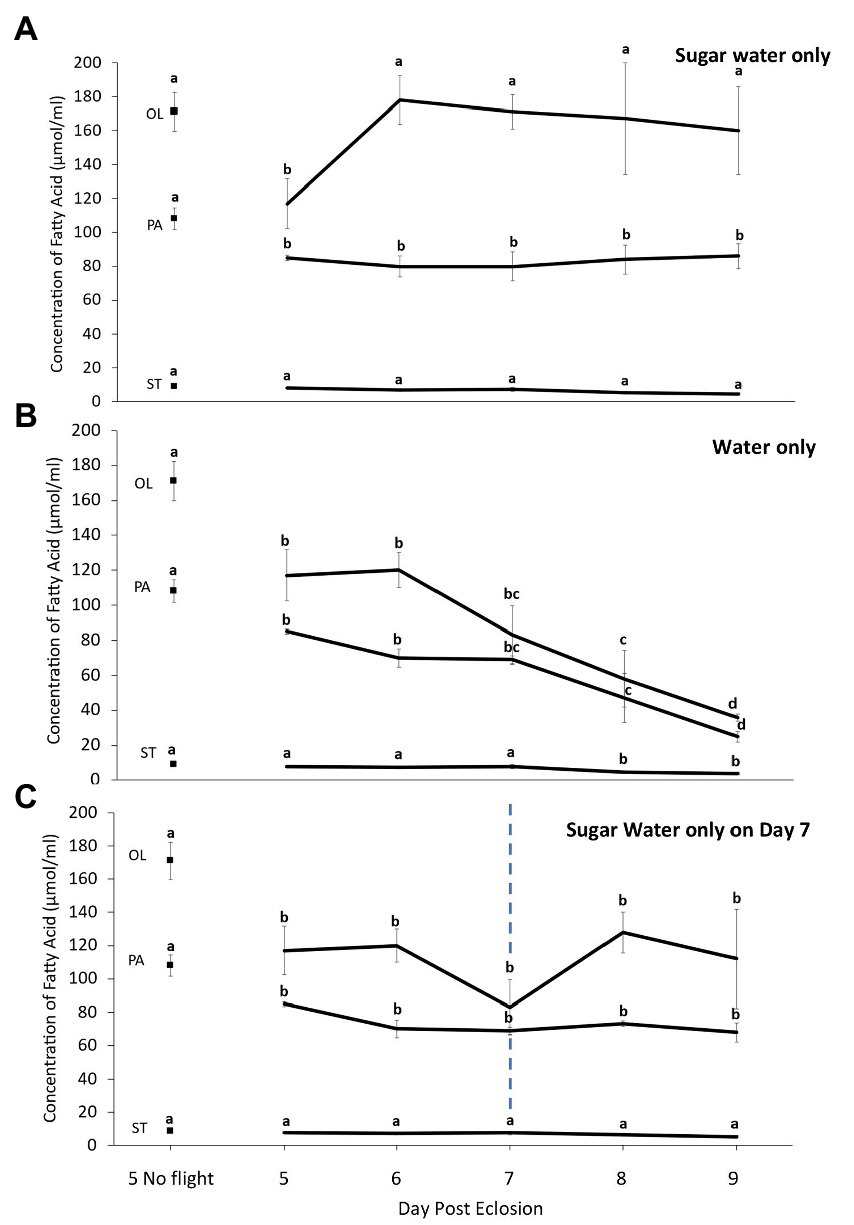
Figure 4. Change in the concentration of non-essential [palmitic (PA), oleic (OL) and stearic (ST)] fatty acids in fat body of true armyworm moths reared under fall conditions as a function of the number of 8-h flight periods when fed (A) sugar water ad lib daily, (B) provided water only, or (C) provided water on all days except at the end of Day 7 when they were provided sugar water ad lib during the rest period. The two values for Day 5 represent samples taken from un-flown moths prior to the flight period and from moths after 8 h of flight. In the other days samples were taken at the end of the flight period. Letters indicate statistically significant differences in each category of fatty acid (Tukey’s HSD, p < 0.05). N = 10 per sample group (N = 20 in Day 5 no flight).
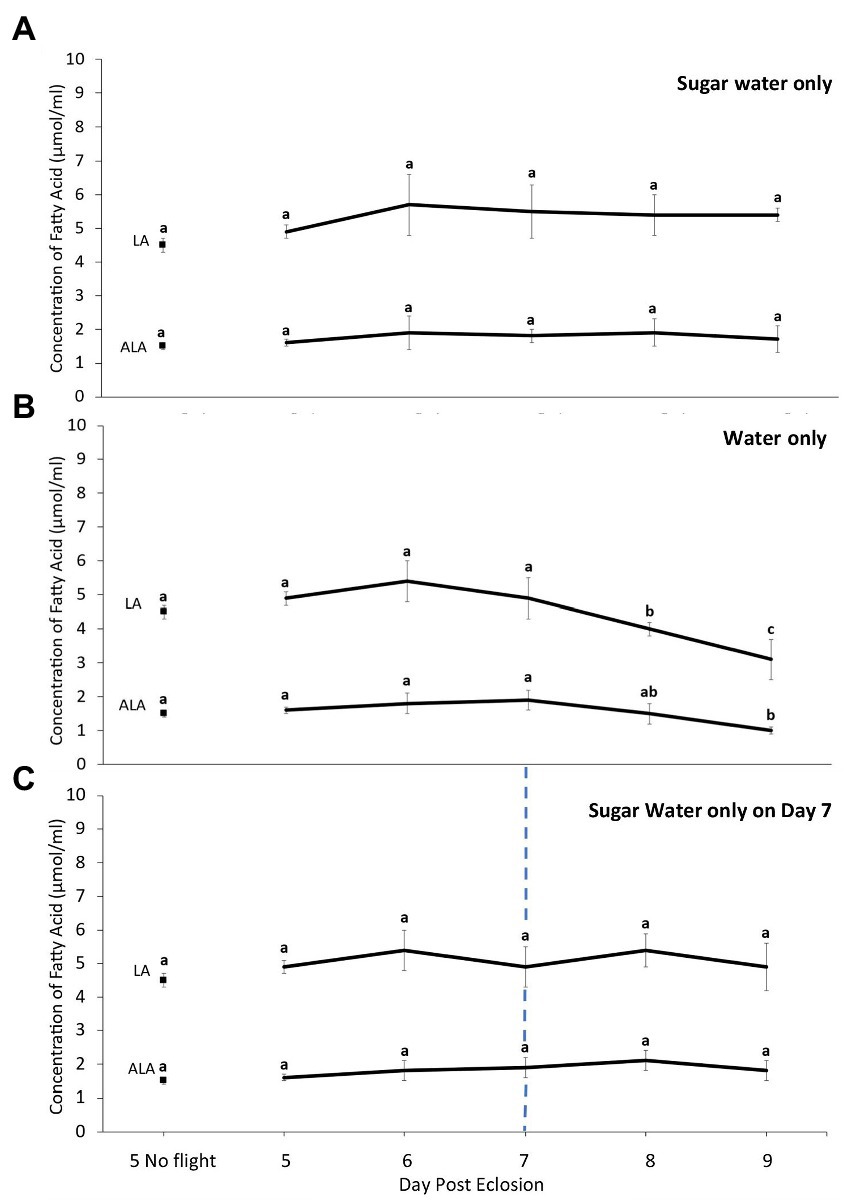
Figure 5. Change in the concentration of essential [linoleic (LA) and alpha linolenic (ALA)] fatty acids in the fat body of true armyworm moths reared under fall conditions as a function of the number of 8-h flight periods when fed (A) sugar water ad lib daily, (B) provided water only, or (C) provided water on all days except at the end of the flight period on Day 7 when they were provided sugar water ad lib during the rest period. The two values for Day 5 represent samples taken from un-flown moths prior to the flight period and from moths after 8 h of flight. In the other days samples were taken at the end of the flight period. Letters indicate statistically significant differences in each category of fatty acid (Tukey’s HSD, p < 0.05). N = 10 per sample group (N = 20 in Day 5 no flight).
4. Discussion
Anparasan et al. (2021) reported that non-flown TAW moths accumulated stored lipids under summer conditions and our current results show this occurs regardless of rearing conditions. This is driven by a rapid accumulation of adult-diet derived NFAs, reflected in the rapid rise in PA and OL (approximately three times the amount found at emergence; Table 1) and the change in δ13C values of the fat body (Figure 2). Concentrations of the EFAs declined slightly between 0 and 5 days in non-flown individuals under all rearing conditions (Table 1) but probably for different reasons. Under summer conditions, most males and females are sexually mature at 5 days post emergence (Delisle and McNeil, 1987; Cusson and McNeil, 1989; Dumont and McNeil, 1992) and thus some EFAs would be incorporated in different components of the reproductive systems, as reported in other Lepidoptera (Martin, 1969; Arrese and Soulages, 2010). In contrast, under fall conditions that initiate migratory behavior, adults take several weeks to become sexually mature (Delisle and McNeil, 1987; Cusson and McNeil, 1989; Dumont and McNeil, 1992). There is little or no development of the reproductive systems on day five under fall conditions, however as EFAs LA and ALA are found at high levels in the thorax muscles of other migratory insects (e.g., Turunen, 1974) the observed decline may be the result of their incorporation in flight muscle.
The decline in the NFAs OL and PA, as well as the δ13C value of the fat body, as a function of flight duration in 5-day old moths supports the idea that adult-derived FAs are preferentially used by the true armyworm to fuel flight, as reported for other insects (Schneider and Dorn, 1994; Wang and Ouyang, 1995; Murata and Tojo, 2002; Sakamoto et al., 2004; Levin et al., 2017). Furthermore, the fact that following flight the levels of LA and ALA in the fat body decline in moths reared under summer conditions but not in those reared under fall conditions (Tables 2, 3) support our hypothesis of differential FA allocation under different environmental conditions. As these EFAs are important for reproduction (Martin, 1969; Arrese and Soulages, 2010), the fact that they are conserved under the environmental conditions stimulating the onset of migration would ensure more are available once a suitable habitat is located and could reduce the costs of migration on future reproductive success.
The difference in the time taken to see a significant decline in FAs in summer- and fall-reared 5 day-old moths as a function of flight duration (Tables 2, 3; Figure 3) may reflect physiological differences related to fuel-use efficiency arising from differences in the physiology of the two seasonal morphs. These could include different levels of muscle FA binding proteins (Haunerland, 1997) and/or increased levels of and responsiveness to lipophorin molecules (Chino et al., 1992). Furthermore, insects reared under migratory conditions may have more developed flight muscles (Boggs, 2009) and/or differential wing loading (Roff and Fairbairn, 1991). The decline in the LA and ALA in the fat body of force-flown moths reared under summer conditions may be the result of their mobilization to flight muscles (see above). Under both rearing conditions, it took at least 4 h of forced flight to detect any significant decline in lipid levels and this was likely related to the initial phase of flight being fueled by carbohydrates, as reported in another migratory noctuid, Agrotis ipsilon (Sappington et al., 1995). The release of lipids from the fat body of the armyworm is modulated by adipokinetic hormone (AKH) and previous work has shown that it takes about an hour of forced flight for lipid levels to stabilize in the haemolymph (Orchard et al., 1991), a pattern also reported in the tobacco hornworm (Manduca sexta; Arrese and Wells, 1997).
As noted, the reduction in the EFAs in summer- but not fall-reared moths supports our hypothesis that physiological changes in response to environmental cues associated with onset of migratory behavior help conserve EFAs during migration. Schneider and Dorn (1994) suggested this may be the case in gregarious migratory locusts and proposed several possible mechanisms to explain such an adaptation. One included the involvement of juvenile hormone (JH), as higher titers may reduce fat body lipid storage in locusts. Previous research on the armyworm has shown that there are lower JH titers in moths reared under fall conditions resulting in the delayed development of the reproductive organs, as well as the production of, and response to, the female sex pheromone (Delisle and McNeil, 1987; Cusson and McNeil, 1989; Dumont and McNeil, 1992). However, additional research is required to determine if JH plays a role in the conservation of EFAs during migration.
The results obtained when fall-reared moths were flown for 8h/day on five consecutive days indicate that the EFAs are conserved over the entire period as long as moths have access to suitable resources. Interestingly, for 5-day-old moths flown for only 8h there was sharp decline in OL levels compared with those of non-flown individuals of the same age, while the post flight concentration on subsequent days were similar to the non-flown controls. This is undoubtedly due to adults increasing the amount of resources consumed following forced flight, possibly the result of increased levels of JH, which can affect feeding behaviour (Rankin, 1991; Dingle and Winchell, 1997). Min et al. (2004) reported a post flight increase in JH titers for migratory grasshoppers, and Cusson et al. (1990) reported increased JH production when TAW adults experienced an increase in ambient temperature. Thus, if increasing body temperature following flight resulted in higher JH levels and led to higher consumption of resources, it would result in greater changes in levels of OL compared with the other FAs, as it is one of the most readily used fuel sources in insects (Schneider and Dorn, 1994; Wang and Ouyang, 1995; Tomcala et al., 2010).
In the absence of such resources, the concentration of EFAs in the fat body decline significantly. However, when water fed moths were provided one ad lib meal of sugar water after 3 days of flight they only used NFAs when force flown for two additional days. This shows they only used EFAs when there was no other option and underlines the importance of nectar availability at stopover sites during the migratory process, as any shortages could significantly impact individual reproductive success and whether populations subsequently reach pest densities. Nectar availability could vary significantly at different sites along the migratory path due to different environmental conditions. For example, extreme weather events associated with climate change could not only affect the density of available flowers but also the quantity and quality of nectar (Takkis et al., 2018; Descamps et al., 2021). Furthermore, under hot, dry conditions the viscosity of nectar will increase and armyworm moths are less effective at acquiring resources when nectar concentrations are high (Pivnick and McNeil, 1985).
We have shown that if adults have access to nectar resources during migration, they are able to conserve the EFAs found in the fat body. However, if some are utilized to sustain flight there is potential for negative impacts on future reproduction as EFAs are a substantial component to the eggs of many lepidopterans (Martin, 1969; Forte et al., 2002) and thus a decline in the availability of these FAs can reduce the number of viable offspring. This possibility needs further investigation but when quantifying such potential impacts, one also needs to consider other EFA sources that adults might access following migration For example, bark beetles, break down their flight muscles post migration in response to an increase in JH titers (Borden and Slater, 1968; Sahota and Farris, 1980) and these authors have suggested that these resources are invested in reproduction. As noted above there is a significant increase in JH associated with the onset of reproduction in the true armyworm, and it is possible that this change in titer may stimulate post migratory release of EFA resources from flight muscle. However, unlike bark beetles which do not resume flight after colonizing a tree host, the armyworm and other lepidopterans need functional flight muscles for foraging and reproduction throughout adult life, thus obtaining resources through flight muscle degradation may be limited. Secondly, females of some migratory species are polyandrous (Torres-Vila et al., 2004) and thus obtaining several spermatophores from repeated mating could offer them an alternative source of resources. Both eggs and female somatic tissue contain male-derived resources (Boggs and Gilbert, 1979; Boggs, 1981; Wiklund et al., 1993), and males of some migratory species transfer JH at the time of mating (e.g., Park et al., 1998). As M. unipuncta is polyandrous, the spermatophores are potential nutrient sources (Marshall and McNeil, 1989) and repeated mating increases female reproductive output (Svärd and McNeil, 1994), so the potential importance of male derived EFAs merits further attention.
In addition to JH, various analogs of AKH have been shown to mobilize FAs differentially which may also be a mechanism by which resource limited TAW moths are allocating FAs. The migratory morph of Locusta migratoria expresses high levels of AKH II which mobilizes more OL and saturated fatty acids compared to other analogs (Tomcala et al., 2010). TAW moths may produce similar AKH analogs and merits investigation as the previous study on AKH in TAW did not explore the possible role of different AKH analogs (Orchard et al., 1991).
Our study demonstrates the power of using bulk isotopic tracing to examine the evolution of lipid allocation in migrant insects, particularly for insects such as the TAW where larval and adult diets are distinct. The use of carbon isoscapes, especially a compound-specific approach using δ13C in individual EFAs and NFAs (Whiteman et al., 2019; Pilecky et al., 2022) would allow for a greater understanding about nectaring stopover sites and sources of vital nutrients for migrant species in the wild. In fact, using a similar design setup to this experiment, tracing of isotopically labeled EFAs and NFAs could be used (as seen in studies on amino acids, e.g., Levin et al., 2017) to determine the fate, isotopic changes and confirmed source of FAs from the fat body, as well as the subsequent allocation to reproduction after flight. Clearly, future research will need to address all of these possibilities as this will not only contribute to our understanding of basic flight energetics but also how energy use would affect the population of migrants whether they are pests or ones, like the monarch butterfly (Danaus plexippus), that we wish to protect.
Data availability statement
The raw data supporting the conclusions of this article will be made available by the authors, without undue reservation.
Author contributions
LA, KH, and JM developed the protocols and wrote the manuscript, with LA performing the experiments. All authors contributed to the article and approved the submitted version.
Funding
LA was supported through a graduate stipend from the University of Western Ontario and the Queen Elizabeth II Graduate Scholarships in Science and Technology. This research was funded by Natural Science and Engineering Research Council of Canada (NSERC) Discovery grants to KH (#2017-04430) and JM (#2020-07203).
Acknowledgments
We thank (i) Blanca X. Mora-Alvarez and Erna Leclair for assistance in the lab, (ii) the Cornell University Stable Isotope Laboratory at Cornell University, Ithaca NY for stable isotope analyses, and (iii) C. Guglielmo for the use of the gas chromatography/flame ionization detector.
Conflict of interest
The authors declare that the research was conducted in the absence of any commercial or financial relationships that could be construed as a potential conflict of interest.
Publisher’s note
All claims expressed in this article are solely those of the authors and do not necessarily represent those of their affiliated organizations, or those of the publisher, the editors and the reviewers. Any product that may be evaluated in this article, or claim that may be made by its manufacturer, is not guaranteed or endorsed by the publisher.
Supplementary material
The Supplementary material for this article can be found online at: https://www.frontiersin.org/articles/10.3389/fevo.2023.1055534/full#supplementary-material
Abbreviations
FA, fatty acid; EFA, essential fatty acid; NFA, nonessential fatty acid; TAW, true armyworm; OL, oleic acid; PA, palmitic acid; ST, stearic acid; LA, linoleic acid; ALA, alpha linolenic acid; AKH, Adipokinetic hormone; JH, juvenile hormone.
References
Anparasan, L., McNeil, J. N., and Hobson, K. A. (2021). Tracing sources of carbon and hydrogen to stored lipids in the migratory moth, Mythimna unipuncta, using stable isotopes (δ2H, δ13C). Physiol. Entomol. 46, 45–51. doi: 10.1111/phen.12343
Arrese, E. L., and Soulages, J. L. (2010). Insect fat body: energy, metabolism, and regulation. Annu. Rev. Entomol. 55, 207–225. doi: 10.1146/annurev-ento-112408-085356
Arrese, E. L., and Wells, M. A. (1997). Adipokinetic hormone-induced lipolysis in the fat body of an insect, Manduca sexta: synthesis of sn-1,2-diacylglycerols. J. Lipid Res. 38, 68–76. doi: 10.1016/S0022-2275(20)37276-X
Beenakkers, A. M. T. (1969). Carbohydrate and fat as a fuel for insect flight. A comparative study. J. Insect Physiol. 15, 353–361. doi: 10.1016/0022-1910(69)90281-9
Boggs, C. L. (1981). Selection pressures affecting male nutrient investment at mating in heliconine butterflies. Evolution 35, 931–940. doi: 10.1111/j.1558-5646.1981.tb04959.x
Boggs, C. L. (2009). Understanding insect life histories and senescence through a resource allocation lens. Funct. Ecol. 23, 27–37. doi: 10.1111/j.1365-2435.2009.01527.x
Boggs, C. L., and Gilbert, L. E. (1979). Male contribution to egg production in butterflies: evidence for transfer of nutrients at mating. Science 206, 83–84. doi: 10.1126/science.206.4414.83
Borden, J. H., and Slater, C. E. (1968). Induction of flight muscle degeneration by synthetic juvenile hormone in Ips confusus (Coleoptera: Scolytidae). Z. Vergl. Physiol. 61, 366–368. doi: 10.1007/BF00428009
Canavoso, L. E., Jouni, Z. E., Karnas, K. J., Pennington, J. E., and Wells, M. A. (2001). Fat metabolism in insects. Annu. Rev. Nutr. 21, 23–46. doi: 10.1146/annurev.nutr.21.1.23
Chino, H., Lum, P. Y., Nagao, E., and Hiraoka, T. (1992). The molecular and metabolic essentials for long-distance flight in insects. J. Comp. Physiol. B. 162, 101–106. doi: 10.1007/BF00398334
Cusson, M., and McNeil, J. N. (1989). Ovarian development in female armyworms, Pseudaletia unipuncta: its relationship with pheromone release activities. Can. J. Zool. 67, 1380–1385. doi: 10.1139/z89-196
Cusson, M., McNeil, J. N., and Tobe, S. (1990). In vitro biosynthesis of juvenile hormone by corpora allata of Pseudaletia unipuncta virgin females as a function of age, environmental conditions, calling behaviour and ovarian development. J. Insect Physiol. 33, 139–146. doi: 10.1016/0022-1910(90)90185-I
Delisle, J., and McNeil, J. N. (1987). Calling behaviour and pheromone titre of the true armyworm Pseudaletia unipuncta (haw.) (Lepidoptera: Noctuidae) under different temperature and photoperiodic conditions. J. Insect Physiol. 33, 315–324. doi: 10.1016/0022-1910(87)90119-3
Descamps, C., Quinet, M., and Jacquemart, A. (2021). Climate change–induced stress reduce quantity and alter composition of nectar and pollen from a bee-pollinated species (Borago officinalis, Boraginaceae). Front. Plant Sci. 12:755843. doi: 10.3389/fpls.2021.755843
Dingle, H. (1972). Migration strategies of insects. Science 175, 1327–1335. doi: 10.1126/science.175.4028.1327
Dingle, H. (2014). Migration: the biology of life on the move, 2nd ed., Oxford, UK: Oxford University Press, 13–23.
Dingle, H., and Winchell, R. (1997). Juvenile hormone as a mediator of plasticity in insect life histories. Arch. Insect Biochem. Physiol. 35, 359–373. doi: 10.1002/(SICI)1520-6327(1997)35:4<359::AID-ARCH2>3.0.CO;2-N
Dixon, A. F. G., Horth, S., and Kindlmann, P. (1993). Migration in insects: cost and strategies. J. Anim. Ecol. 62, 182–190. doi: 10.2307/5492
Dumont, S., and McNeil, J. N. (1992). Responsiveness of Pseudaletia unipuncta (Lepidoptera: Noctuidae) males, maintained as adults under different temperature and photoperiodic conditions, to female sex pheromone. J. Chem. Ecol. 18, 1797–1807. doi: 10.1007/BF02751104
Fields, P. G., and McNeil, J. N. (1984). The overwintering potential of true armyworm Pseudaletia unipuncta (Lepidoptera: Noctuidae) populations in Quebec. Can. Entomol. 116, 1647–1652. doi: 10.4039/Ent1161647-12
Folch, J., Lees, M., and Stanley, G. H. S. (1957). A simple method for the isolation and purification of total lipids from animal tissues. J. Biol. Chem. 226, 497–509. doi: 10.1016/S0021-9258(18)64849-5
Forte, S. N., Ferrero, A. A., and Alonso, T. S. (2002). Content and composition of phosphoglycerols and neutral lipids at different developmental stages of the eggs of the codling moth, Cydia pomonella (Lepidoptera: Tortricidae). Arch. Insect Biochem. Physiol. 50, 121–130. doi: 10.1002/arch.10036
Guppy, J. C. (1961). Life history and behavior of the armyworm, Pseudaletia unipuncta (Haw.) (Lepidoptera: Noctuidae), in eastern Canada. Can. Entomol. 93, 1141–1153. doi: 10.4039/Ent931141-12
Haunerland, N. H. (1997). Transport and utilization in insect flight muscles. Comp. Biochem. Physiol. B 117, 475–482. doi: 10.1016/S0305-0491(97)00185-5
Hobson, K. A., Doward, K., Kardynal, K. J., and McNeil, J. N. (2018). Inferring origins of migrating insects using isoscapes: a case study using the true armyworm, Mythimna unipuncta, in North America. Ecol. Entomol. 43, 332–341. doi: 10.1111/een.12505
Johnson, C. G. (1963). Physiological factors in insect migration by flight. Nature 198, 423–427. doi: 10.1038/198423a0
Krenn, H. W. (2010). Feeding mechanisms of adult lepidoptera: structure, function, and evolution of the mouthparts. Annu. Rev. Entomol. 55, 307–327. doi: 10.1146/annurev-ento-112408-085338
Levin, E., McCue, M. D., and Davidowitz, G. (2017). More than just sugar: allocation of nectar amino acids and fatty acids in a lepidopteran. Proc. Royal Soc. B-Biol. Sci. 284, 2016–2126. doi: 10.1098/rspb.2016.2126
Marshall, L. D., and McNeil, J. N. (1989). Spermatophore mass as an estimate of male nutrient investment: a closer look in Pseudaletia unipuncta (Haworth) (Lepidoptera: Noctuidae). Funct. Ecol. 3, 605–612. doi: 10.1098/rspb.2016.2126
Martin, J. S. (1969). Lipid composition of fat body and its contribution to the maturing oöcytes in Pyrrhocoris apterus. J. Insect Physiol. 15, 1025–1045. doi: 10.1016/0022-1910(69)90142-5
McCue, M. D., Guzman, R. M., Passement, C. A., and Davidowitz, G. (2015). How and when do insects rely on endogenous protein and lipid resources during lethal bouts of starvation? A new application for 13C-breath testing. PLoS One 10:e0140053. doi: 10.1371/journal.pone.0140053
Min, K. J., Jones, N., Borst, D. W., and Rankin, M. A. (2004). Increased juvenile hormone levels after long-duration flight in the grasshopper, Melanoplus sanguinipes. J. Insect. Physiol. 50, 531–537. doi: 10.1016/j.jinsphys.2004.03.009
Murata, M., and Tojo, S. (2002). Utilization of lipid for flight and reproduction in Spodoptera litura (Lepidoptera: Noctuidae). Eur. J. Entomol. 99, 221–224. doi: 10.14411/eje.2002.031
Nicolson, S. W., and Thornburg, R. W. (2007). Nectaries and nectar. The Netherlands: Springer, 215–265.
Orchard, I., Cusson, M., and McNeil, J. N. (1991). Adipokinetic hormone of the armyworm, Pseudaletia unipuncta, immunohistochemistry, amino acid analysis, quantification and bioassay. Physiol. Entomol. 16, 439–445. doi: 10.1111/j.1365-3032.1991.tb00583.x
Park, Y. I., Shu, S., Ramaswamy, S. B., and Srinivasan, A. (1998). Mating in Heliothis virescens: transfer of juvenile hormone during copulation by male to female and stimulation of biosynthesis of endogenous juvenile hormone. Arch. Insect Biochem. Physiol. 38, 100–107. doi: 10.1002/(SICI)1520-6327(1998)38:2<100::AID-ARCH6>3.0.CO;2-X
Pilecky, M., Kämmer, S. K., Mathieu-Resuge, M., Wassenaar, L. I., Taipale, S. J., Martin-Creuzburg, D., et al. (2022). Hydrogen isotopes (δ2H) of polyunsaturated fatty acids track bioconversion by zooplankton. Funct. Ecol. 36, 538–549. doi: 10.1111/1365-2435.13981
Pivnick, K. A., and McNeil, J. N. (1985). Effects of nectar concentrations on butterfly feeding: measured feeding rates for Thymelicus lineola (Lepidoptera:Hesperiidae) and a general feeding model for adult Lepidoptera. Oecologia 66, 226–237. doi: 10.1007/BF00379859
Rankin, M. A. (1991). Endocrine effects on migration. Am. Zool. 31, 217–230. doi: 10.1093/icb/31.1.217
Roff, D. A., and Fairbairn, D. J. (1991). Wing dimorphisms and the evolution of migratory polymorphisms among the Insecta. Am. Zool. 31, 243–251. doi: 10.1093/icb/31.1.243
Sahota, T. S., and Farris, S. H. (1980). Inhibition of flight muscle degeneration by precocene II in the spruce bark beetle, Dendroctonus rufipennis (Kirby) (Coleoptera: Scolytidae). Can. J. Zool. 58, 378–381. doi: 10.1139/z80-048
Sakamoto, R., Murata, M., and Tojo, S. (2004). Effects of larval diets on flight capacity and flight fuel in adults of the common cutworm, Spodoptera litura (Lepidoptera: Noctuidae). Appl. Entomol. Zool. 39, 133–138. doi: 10.1303/aez.2004.133
Sappington, T. W., Fescemyer, H. W., and Showers, W. B. (1995). Lipid and carbohydrate utilization during flight of the migratory moth, Agrotis ipsilon (Lepidoptera: Noctuidae). Arch. Insect Biochem. Physiol. 29, 397–414. doi: 10.1002/arch.940290407
Saunders, D. S. (1987). Photoperiodism and the hormonal control of insect diapause. Sci. Prog. 71, 51–69.
Schneider, M., and Dorn, A. (1994). Lipid storage and mobilization by flight in relation to phase and age of Schistocerca gregaria females. Insect Biochem. Mol. Biol. 24, 883–889. doi: 10.1016/0965-1748(94)90017-5
Shorey, H. H., and Hale, R. L. (1965). Mass-rearing of the larvae of nine noctuid species on a simple artificial medium. J. Econ. Entomol. 58, 522–524. doi: 10.1093/jee/58.3.522
Stanley-Samuelson, D. W., Jurenka, R. A., Cripps, C., Blomquist, G. J., and Renobales, M. (1988). Fatty acids in insects: composition, metabolism, and biological significance. Arch. Insect Biochem. Physiol. 9:1. doi: 10.1002/arch.940090102
Subramanyam, B., and Cutkomp, L. K. (1987). Total lipid and fatty acid composition in male and female larvae of Indian-meal moth and almond moth (Lepidoptera: Pyralidae). Gt. Lakes Entomol. 20:10.
Svärd, L., and McNeil, J. N. (1994). Female benefit, male risk: polyandry in the true armyworm Pseudaletia unipuncta. Behav. Ecol. Sociobiol. 35, 319–326. doi: 10.1007/BF00184421
Takkis, K., Tscheulin, T., and Petanidou, T. (2018). Differential effects of climate warming on the nectar secretion of early- and late-flowering Mediterranean plants. Front. Plant Sci. 9:874. doi: 10.3389/fpls.2018.00874
Tomcala, A., Bártů, I., Simek, P., and Kodrík, D. (2010). Locust adipokinetic hormones mobilize diacylglycerols selectively. Comp. Biochem. Physiol. 156, 26–32. doi: 10.1016/j.cbpb.2010.01.015
Torres-Vila, L. M., Rodrguez-Molina, M. C., and Jennions, M. D. (2004). Polyandry and fecundity in the Lepidoptera: can methodological and conceptual approaches bias outcomes? Behav. Ecol. Sociobiol. 55, 315–324. doi: 10.1007/s00265-003-0712-2
Turgeon, J., and McNeil, J. N. (1983). Modifications in the calling behaviour of Pseudaletia unipuncta (Lepidoptera: Noctuidae), induced by temperature conditions during pupal and adult development. Can. Entomol. 115, 1015–1022. doi: 10.4039/Ent1151015-8
Turunen, S. (1974). Metabolism and function of fatty acids in a phytophagous lepidopteran. Ann. Zool. Fenn. 11, 170–184.
Wang, Z., and Ouyang, Y. (1995). Flight activity and fatty acid utilization in Mythimna separata (Walker) moths. Insect Sci. 2, 370–376. doi: 10.1111/j.1744-7917.1995.tb00061.x
Whiteman, J. P., Elliott-Smith, E. A., Besser, A. C., and Newsome, S. D. (2019). A guide to using compound-specific stable isotope analysis to study the fates of molecules in organisms and ecosystems. Diversity 11:8. doi: 10.3390/d11010008
Keywords: true armyworm, essential fatty acids, migration, carbon-13, trade off
Citation: Anparasan L, Hobson KA and McNeil JN (2023) Effect of rearing conditions on fatty acid allocation during flight in nectivorous lepidopteran Mythimna unipuncta. Front. Ecol. Evol. 11:1055534. doi: 10.3389/fevo.2023.1055534
Edited by:
Bing Chen, Hebei University, ChinaReviewed by:
Maya L. Evenden, University of Alberta, CanadaWei Guo, Institute of Zoology (CAS), China
Copyright © 2023 Anparasan, Hobson and McNeil. This is an open-access article distributed under the terms of the Creative Commons Attribution License (CC BY). The use, distribution or reproduction in other forums is permitted, provided the original author(s) and the copyright owner(s) are credited and that the original publication in this journal is cited, in accordance with accepted academic practice. No use, distribution or reproduction is permitted which does not comply with these terms.
*Correspondence: Libesha Anparasan, lanparas@uwo.ca
†These authors have contributed equally to this work