- 1Institut Pasteur de Bangui, Bangui, Central African Republic
- 2Centre International de Recherches Medicales de Franceville (CIRMF), Franceville, Gabon
- 3UMR MIVEGEC, Univ. Montpellier, CNRS, IRD, Montpellier, France
The chromosomal rearrangement 2La has been directly involved in the ecological and deadly epidemiological success of the malaria mosquitoes Anopheles gambiae and Anopheles coluzzii in sub-Saharan Africa. However, little is known about the biological and ecological factors that drive the local and temporal dynamics of this inversion in both species. Here, we performed a year-round longitudinal survey in Bangui, Central African Republic. We monthly sampled A. gambiae and A. coluzzii mosquitoes indoor and outdoor using human landing catches (HLC) for 48 h non-stop. We molecularly karyotyped all specimens to study the 2La inversion frequency variations, and monitored the mosquito spatial and temporal biting behavior throughout the year. In total, we successfully karyotyped 5121 A. gambiae and 986 A. coluzzii specimens. The 2La inversion frequency was higher in A. coluzzii than in A. gambiae across the year. In A. gambiae and A. coluzzii, the inversion frequency or karyotypes did not influence the biting behavior, either location or time. Moreover, the inversion frequency variation in both species was also independent of local climatic changes. Overall, our results revealed that in Bangui, the 2La inversion segregates at different frequency in each species, but this is not influenced by their trophic behavior. Studying the impact of urban settings and the population genetic structure of these two A. gambiae complex members could bring insights into the intrinsic relationship between 2La inversion and local conditions. More studies are needed to understand the polymorphic equilibrium of this inversion in Bangui.
Introduction
The ability to rapidly adapt to environmental and anthropogenic conditions allowed some members of the Anopheles gambiae complex to become the most prominent malaria vectors in Africa (White et al., 2010). Within the Anopheles gambiae complex, two species, Anopheles gambiae and Anopheles coluzzii, stand out due to their ecological success, as indicated by their faculty to colonize a myriad of habitats across sub-Saharan Africa and their efficient transmission of malaria parasites (Costantini et al., 2009; Simard et al., 2009; Sinka et al., 2010). Their success is rooted in their virtually limitless genetic polymorphism (Fontaine et al., 2015; Miles et al., 2017), including chromosomal inversions (Ayala et al., 2014, 2017).
Among the polymorphic chromosomal rearrangements, the 2La inversion is particularly interesting in both species. This inversion spans along almost half (∼22 Mb) of the left arm of chromosome 2 (2L), and is polymorphic only in A. gambiae and A. coluzzii within the A. gambiae complex (Coluzzi et al., 1979; Sharakhov et al., 2006). It is acquired by adaptive introgression from the sister species An. arabiensis where it is fixed (Sharakhov et al., 2006; Fontaine et al., 2015), and has been associated with many adaptive traits that influence malaria transmission (Ayala et al., 2014). For decades, the 2La inversion has been essentially associated with a latitudinal cline in many parts of Africa (Coluzzi et al., 1979; Costantini et al., 2009; Simard et al., 2009). This reflects an aridity gradient, with increasing polymorphism from humid habitats (standard karyotype) to dry savannas (inverted karyotype) in Africa (Ayala et al., 2017). This aridity adaptation has been also associated with phenotypic traits, such as thermal tolerance in larvae and desiccation resistance in adults (Rocca et al., 2009; Fouet et al., 2012). A recent study on the genetic basis of this adaptation proposed that some candidate genes within the 2La inversion may explain the stronger desiccation resistance conferred by this inversion (Ayala et al., 2019). Moreover, seasonal fluctuations of the 2La inversion frequency (from high frequency in the dry season to low frequency in the wet season) have been recorded in different parts of the African continent (Toure et al., 1994; Ayala et al., 2014). Several studies showed that the 2La inversion could also affect the choice of biting and resting sites, leading to higher frequency of indoor than outdoor captures (Coluzzi et al., 1979; Ayala et al., 2014). This non-random association between 2La inversion and temporal/spatial behaviors may affect the effectiveness of vector control tools, such as indoor residual spraying and long-lasting insecticidal nets, at the local scale, increasing the level of malaria transmission in many parts of sub-Saharan Africa (Riehle et al., 2017). This is particularly true in areas where this inversion is highly polymorphic and both Anopheles species are the major malaria vectors.
In Bangui, the Central African Republic capital, two studies showed that A. gambiae and A. coluzzii are the only members of the A. gambiae complex present in the city, and that A. gambiae is the predominant vector (Ndiath et al., 2016; Ole Sangba et al., 2017; Kamgang et al., 2018). Ndiath et al. (2016) also showed that the 2La inversion frequency is ∼ 40%. To better understand the role of the 2La inversion in the population dynamics and behavior of these two mosquito species, we carried out an extensive longitudinal survey in Bangui throughout 1 year. We molecularly karyotyped both species and analyzed how the 2La inversion frequency varied across the year, and in function of the collection locality (indoor and outdoor), and biting time. These data are essential to better understand the local adaptation of these two malaria vectors and to assess the effectiveness of frontline interventions for malaria control in Bangui, Central African Republic.
Material and methods
Ethical approval
This study and the methods employed for mosquito collection, including human landing catch (HLC), were approved by the Institutional Review Board of the Health Sciences Faculty of Bangui University (authorization number 01/UB/FACSS/CSCVPER/17).
Study sites and climatic variables
Mosquitoes were collected at four sites in Bangui: Gbanikola (4.3311 N, 18.5400 W), Îles de singes (4.3549 N, 18.5667 W), Ouango (4.3767 N, 18.6082 W), and Pk10 (4.4304 N, 18.5468 W) (Figure 1A). Bangui is located at the southern border of the country and lies on the northern banks of the Ubangui River, along the Democratic Republic of Congo border. The climate is tropical with relative humidity varying from 47% in December to 72% in August. The rainy season extends from May to October, and the dry season from November to April.
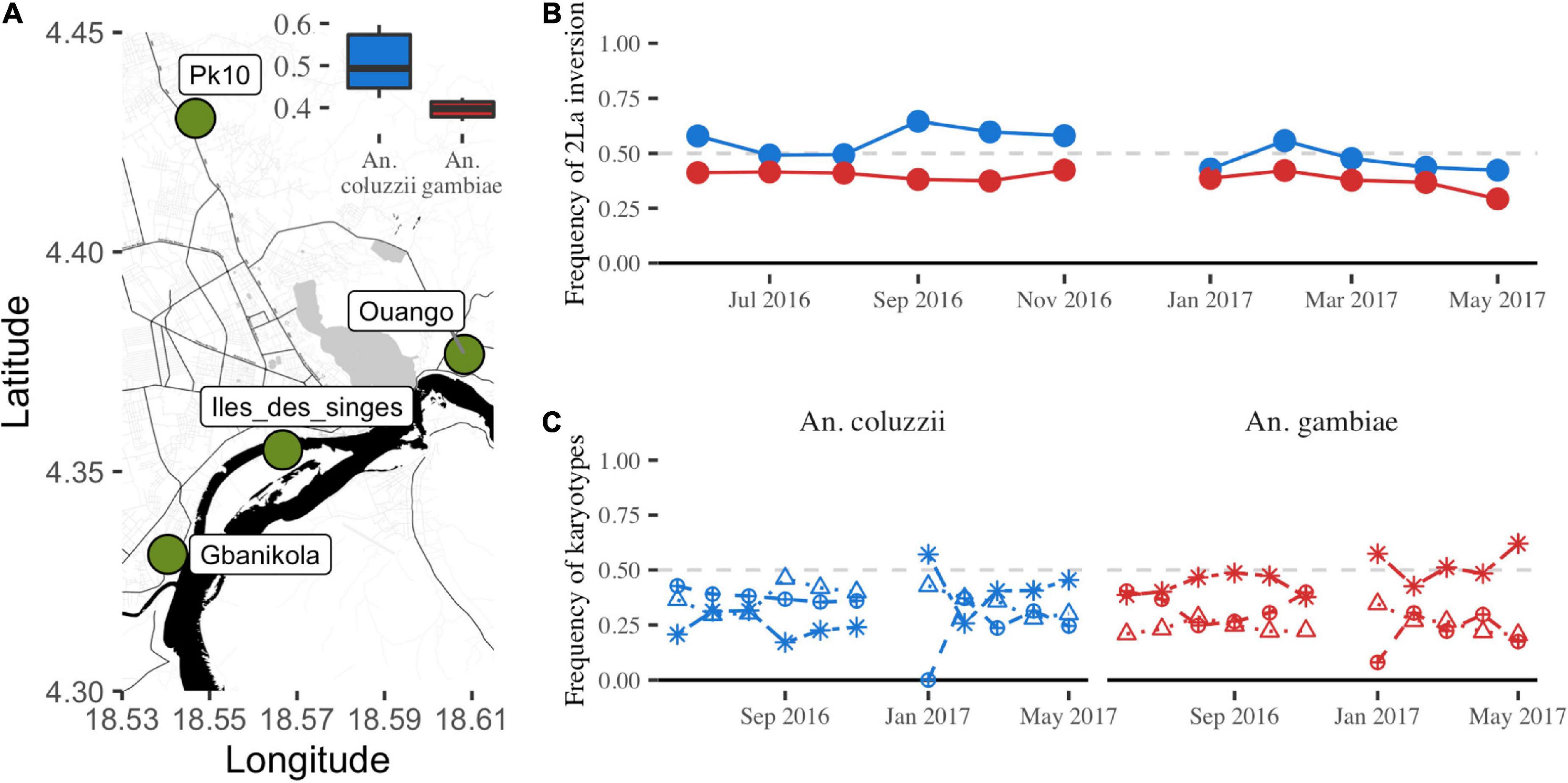
Figure 1. Collection sites and temporal dynamics of the 2La inversion frequency in Bangui. (A) Green circles indicate the mosquito collection sites in the city of Bangui (source: https://OpenStreetMap.org). The inset shows the mean frequency of the 2La inversion in the two species during the collection year. (B) Linear representation of the 2La inversion frequency variations for each species (blue line, A. coluzzii; red line, A. gambiae) during the collection year. (C) Linear representation of each karyotype (mean frequency) per species across the year. Blue lines, A. coluzzii; red lines, A. gambiae. Asterisks, standard karyotype; circles with a cross, heterozygote karyotype; triangles, inverted karyotype.
During the sampling period, meteorological data (rain, temperature, and relative humidity) were collected monthly by the Meteorological Station of Bangui (Supplementary Table 1).
Mosquito collection and morphological identification of species
All mosquitoes were collected during 11 months (from June 2016 to May 2017, except December due to logistical reasons). Each month, adult mosquitoes were field-collected by human landing capture (HLC) during two consecutive days (non-stop for 48 h). At each site, collections were carried out by two volunteers, inside and outside each house, for 6 h, who were then replaced by two new volunteers. The volunteers’ location and sampling time were changed several times to avoid bias in the collections. All samples were transported to the Institut Pasteur de Bangui. Mosquitoes were morphologically identified using the taxonomical identification keys described by Gillies and De Meillon (1968) and Gillies and Coetzee (1987).
DNA extraction, species identification, and molecular karyotyping of the 2La inversion
Total genomic DNA was extracted from all mosquitoes morphologically identified as A. gambiae complex members using 2% cetyl-trimethyl-ammonium-bromide following the protocol described by Morlais et al. (2004). DNA pellets were dissolved in 100 μl sterile water at 4°C for 24 h, and then stored at –20°C. A. gambiae complex members were then identified using species-specific PCR assays according to the protocols developed by Santolamazza et al. (2008). The molecular karyotyping of the 2La inversion was performed according to White et al. (2007) and based on amplicon band sizes that correspond to the standard homokaryotype (2L + a/2L + a, hereafter standard), the inverted homokaryotype (2La/a, hereafter inverted), or the heterokaryotype (2La/ + a, hereafter heterozygote).
Genetic, statistical and modeling analyses
Conformity to the Hardy-Weinberg equilibrium (HWE) was assessed using the “HardyWeinberg” package (Graffelman and Morales-Camarena, 2008; Graffelman, 2015) in R (R Core Team, 2021). The HWE was tested for each species at each site, location, and month. When the number of individuals was < 10, the test was not performed (“NA”).
Circular statistical analyses were carried out in R according to Pewsey et al. (2013) and Sangbakembi-Ngounou et al. (2022) and the functions available therein. As mosquitoes were aggregated over 1-h time slots at collection, biting events were transformed into a pseudo-continuous variable by adding a uniform random component over the [0, 1] interval of each time slot. Bites were grouped by species and by karyotype to compare the daily bite rhythmicity in the four grouped collection sites using circular statistics.
Then, to determine the variables that play a role in the 2La inversion and biting behavior dynamics in A. coluzzii and A. gambiae, a generalized linear model with a binomial distribution was parameterized (Hosmer et al., 1989). The response variable was the 2La inversion frequency in each species, and the explanatory variables were the climatic parameters listed in Supplementary Table 1.
All statistical analyses were performed using the R software, version 4.0.2,1 and the package “stats” in R (R Core Team, 2021).
Results
Frequency of the 2La inversion in Bangui
We collected 6,198 mosquitoes belonging to the A. gambiae complex at the four sites in Bangui (Figure 1A, Table 1, and Supplementary Table 2). We molecularly identified 5187 A. gambiae sensu stricto (hereafter A. gambiae), 991 A. coluzzii, and 20 hybrids. Only A. gambiae and A. coluzzii specimens were retained for the 2La inversion analysis. In total, we successfully molecularly karyotyped 5121 A. gambiae and 986 A. coluzzii samples (Table 1). The mean frequency of the arrangement across the year was significantly different between A. gambiae and A. coluzzii (0.38 and 0.51; Wilcoxon test, W = 120, p < 0.001) (Figure 1A). Moreover, 48.70 and 31.74% of samples harbored the standard, 24.74 and 34.69% the inverted, and 26.56 and 33.57% the heterozygote karyotype in A. gambiae and in A. coluzzii, respectively (Table 1). We observed a significant deficit of heterozygotes in both A. gambiae and A. coluzzii across the year (HWE, exact test Chi-Square = 974.31, p < 0.001, Table 1). When we estimated the HWE deviation for each month, we observed a constant deficit of heterozygotes in A. gambiae, and a deficit in more than the half of the months in A. coluzzii that coincided mostly with the dry season (Supplementary Table 3). We obtained similar results when we computed the HWE deviation by month and location for each species (Supplementary Table 4), or by month, location, and site (Supplementary Table 5).

Table 1. Summary of mosquito specimens collected and karyotyped in Bangui, Central African Republic.
The temporal dynamics of the 2La inversion
We calculated the 2La inversion frequency during 1 year (Figure 1B) and did not observe any significant difference among months for both A. gambiae (ANOVA: F-statistic = 1.2, p > 0.05) and A. coluzzii (ANOVA: F-statistic = 1.4, p > 0.05), and also between species (Wilcoxon test, p > 0.05) (Figure 1B). We obtained similar results when comparing the inversion karyotype frequencies for each month and for both species (Wilcoxon test, p > 0.05). Analysis of the karyotypes in each species across the year revealed different temporal patterns between species, particularly for the standard karyotype (Figure 1C).
Biting behavior and 2La inversion
To assess the role of the 2La inversion in the biting behavior of both malaria mosquito species, we estimated the inversion frequency in specimens collected indoor and outdoor in Bangui. We observed slight, but not significant, differences in its frequency between indoor and outdoor collections for each species during the sampling months (ANOVA: F-statistic = 0.007, p > 0.05) (Figure 2A). We did not detect any significant interaction between the inversion frequency and the sampling location for both species (ANOVA: F-statistic = 4.20, p > 0.05). Moreover, we asked whether the different inversion karyotypes were associated with the host-seeking behavior. Analysis of the sampling data showed no difference in the frequency of the different karyotypes per month between specimens captured indoor and outdoor for both species (Wilcoxon test, p > 0.05) (Figure 2B). For both species, mosquitoes harboring the standard karyotype were as likely to be captured indoor and outdoor as mosquitoes carrying the inverted karyotype (A. coluzzii: ANOVA: F-statistic = 9.618e-29, p > 0.05; A. gambiae: ANOVA: F-statistic = 5.349e-29, p > 0.05) (Figure 2B).
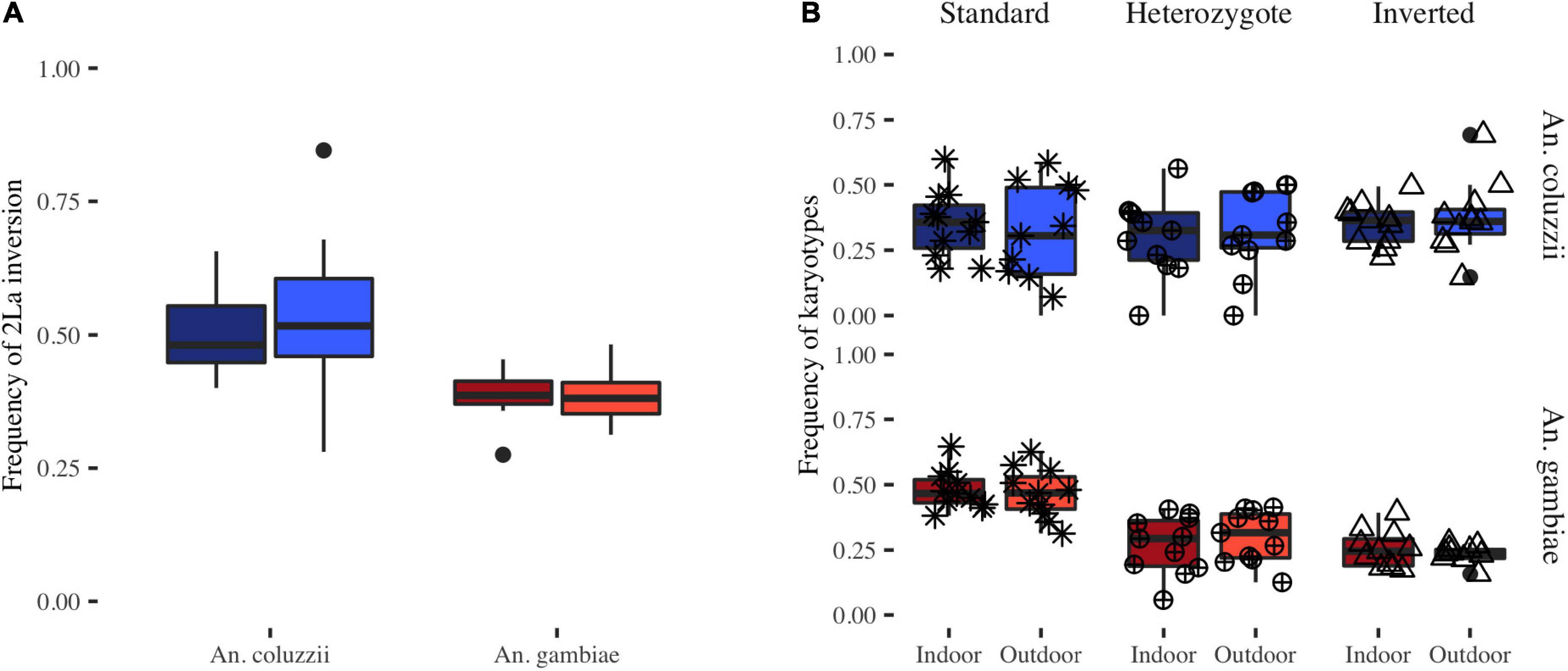
Figure 2. Spatial distribution of the 2La inversion in function of the collection location. (A) Boxplots show the 2La inversion frequency in mosquitoes collected indoor (darker colors) and outdoor (lighter colors) throughout the year (A. gambiae in red, A. coluzzii in blue). (B) Frequency of each 2La inversion karyotype (asterisk, standard; circle with cross, heterozygote; triangle, inverted) for A. gambiae and A. coluzzii specimens captured indoor and outdoor.
Then, we analyzed the daily mosquito bite rhythmicity as a continuous distribution of bite events that occurred during the entire diel cycle of 24 h. Despite the slight differences observed (Sangbakembi-Ngounou et al., 2022), we assumed that each karyotype exhibited similar spatial biting distributions, although more studies are needed to investigate variations according to spatial and temporal heterogeneities. We then estimated the trigonometric moments, such as the mean direction (θ) and the mean length (R), of each karyotype in the two species and the locations throughout the year and for each month. The θ computes the arithmetic mean of the different bite events during the 24 h cycle, and the resulting length varies between 0 and 1 and represents the variation –dispersion– in values of the angles (Pewsey et al., 2013; Sangbakembi-Ngounou et al., 2022). The estimation of these two parameters (Table 2) showed that mosquitoes (all karyotypes) tended to bite after midnight and before 3 a.m. (Table 2 and Figure 3). In A. coluzzii, all mosquitoes (the three inversion karyotypes) exhibited the same aggressivity pic, independently of the location. In A. gambiae, all mosquitoes (the three inversion karyotypes) showed the same aggressivity pic; however, mosquitoes tended to bite earlier outdoor than indoor (Figure 3). As previously observed (Sangbakembi-Ngounou et al., 2022), outdoor biting events were more concentrated compared with indoor biting events, independently of the karyotype (Table 2 and Figure 3). This pattern remained stable across the year with slight differences, mainly related to the low sample size (Supplementary Figures 1–3). Overall, we did not observe any difference in biting rhythm in function of the karyotype in both species. We also asked whether biting events were uniformly distributed around the 24-h diel cycle. The uniformity hypothesis was rejected for all groups, implying that there are different biting pics during the diel cycle (Table 2). Then, we tested whether the different biting distributions were reflective symmetric throughout the day. This hypothesis was systematically rejected for all A. gambiae specimens collected indoor (all karyotypes), and for A. gambiae and A. coluzzii specimens harboring the inverted karyotype and collected outdoor (Table 2), suggesting the existence of multiple distributions (Sangbakembi-Ngounou et al., 2022).
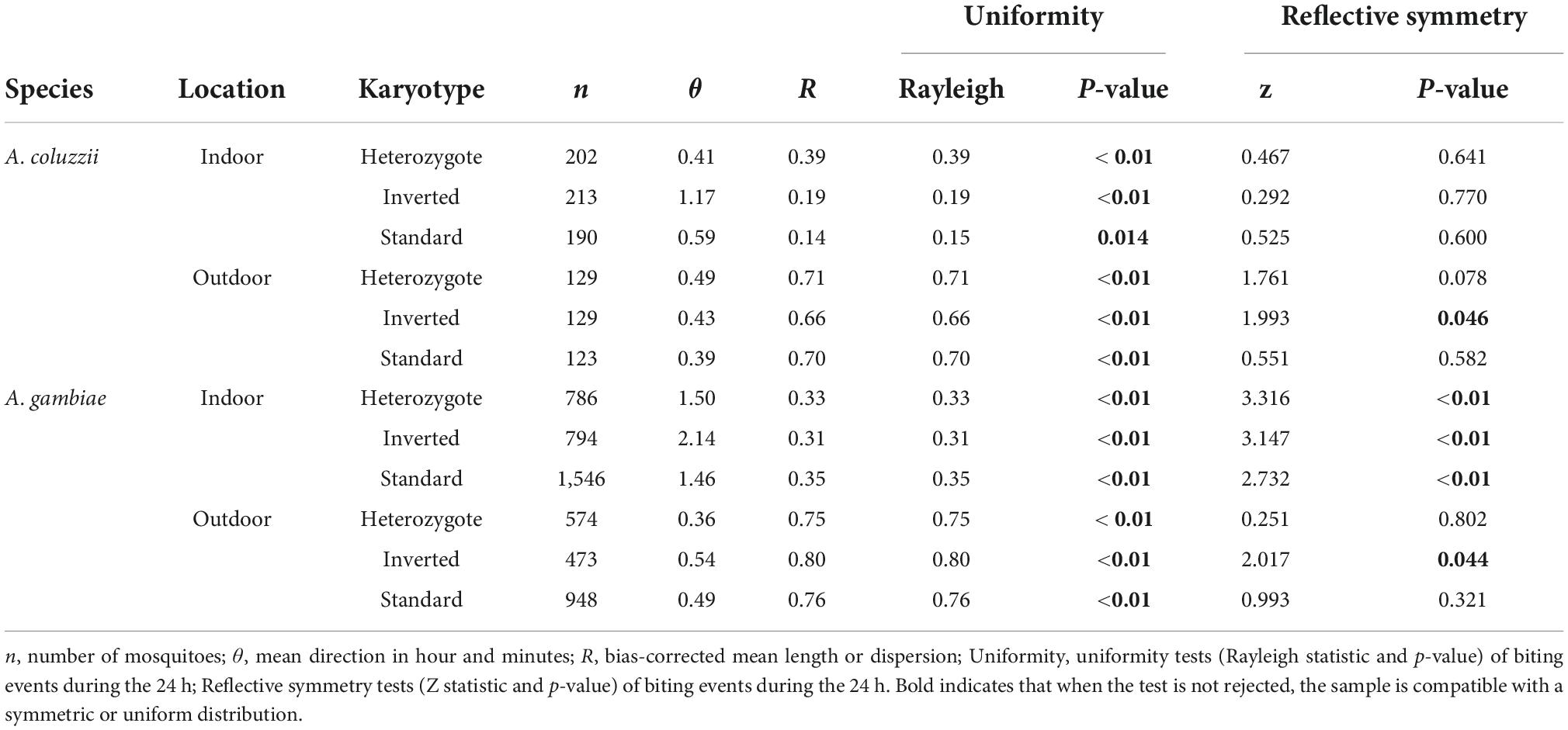
Table 2. Summary of the circular statistic results for each species, location, and 2La inversion karyotype across the year.
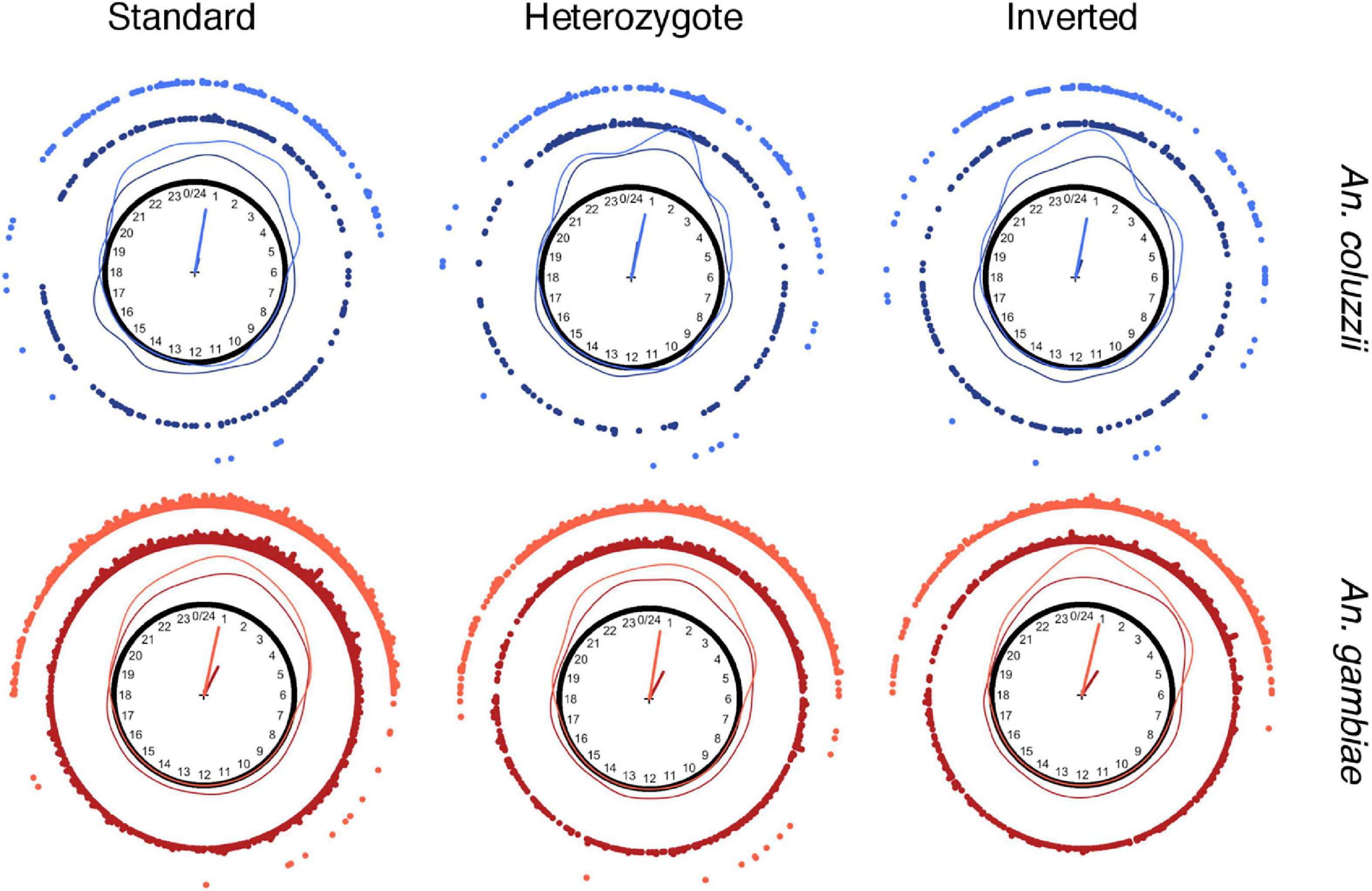
Figure 3. Circular representation of biting events for each karyotype and species. Biting events are represented by points, the kernel distributions by lines, and the mean directions (θ) and resulting lengths (R) are depicted as arrow vectors on the unit circle. Darker colors represent indoor collections, and lighter colors outdoor collections.
Environmental drivers of the 2La inversion
The 2La inversion frequency variations are governed by environmental factors (Simard et al., 2009). This is particularly relevant at the macrogeographical scale (Ayala et al., 2017). Therefore, we determined whether the 2La inversion frequency was affected by the temporal variations of the local climatic conditions in Bangui. Using a generalized linear model approach, we correlated the 2La inversion frequency with different climate parameters (Supplementary Table 1). Overall, our models revealed that the local changes in inversion frequency in both species were not related to variations in the local climatic factors (Supplementary Table 6). This remained true also when we analyzed the 2La inversion frequency variations in function of the sampling location (indoor or outdoor). Similarly, when we analyzed the frequency variations of the different karyotypes in each species, we observed the same pattern, without significant correlations with the local climatic factors in Bangui (Supplementary Table 6).
Discussion
Understanding the chromosomal 2La inversion dynamics in the malaria mosquitoes A. gambiae and A. coluzzii is required to better explain their capacity to adapt to local environmental conditions and predict future scenarios under global warming changes. Previous studies positioned the city of Bangui in the middle of the 2La inversion gradient (Ayala et al., 2017), with a mean frequency of 40% (Ndiath et al., 2016). In agreement, we found 2La inversion frequencies of 38 and 51% in A. gambiae and A. coluzzii, respectively. However, A. coluzzii exhibited significantly higher frequency that its sister species across the year (Figure 1). Although this frequency difference between species in favor of A. coluzzii has been already observed in other parts of Africa (Ayala et al., 2017), it was not investigated. Indeed, the fitness advantage for desiccation (Fouet et al., 2012; Ayala et al., 2019) and thermal tolerance linked to the 2La inversion (Gray et al., 2009) have been empirically demonstrated only in An gambiae. Looking at the existing gene flow between mosquitoes (we found 20 hybrids, Supplementary Table 1), we do not expect significant genetic differences. However, these results need to be confirmed by phenotypic tests in both species collected at the same location, and Bangui offers a compelling opportunity to this end. The expected similar frequencies in both species could be distorted also by the 2Rb inversion segregation. The chromosomal inversion 2Rb segregates in the right arm (2R) and is in linked disequilibrium with the 2La inversion (Costantini et al., 2009; Simard et al., 2009; Ayala et al., 2017), and exhibits signals of selection concerning desiccation resistance (Fouet et al., 2012; Ayala et al., 2019). Unfortunately, in our study we molecularly karyotyped only the 2La and not the 2Rb inversion and therefore, we cannot say whether this rearrangement segregates differently between species. Finally, the strategies to survive during the dry season are different in these two mosquito species, and they could affect the frequency of this rearrangement through the movement of populations. Indeed, A. gambiae migrate, whereas A. coluzzii stay in the same location during the dry season (Dao et al., 2014). We detected Hardy Weinberg Disequilibrium across most of the months in both species, revealing that populations experience asymmetric gene flow levels (Supplementary Tables 3–5). Although adaptation to local environments may affect also the gene flow (Lenormand, 2002), we could expect different connectivity levels for each mosquito species with the surroundings populations. Population genetic analysis across different spatial and time points could help to understand the population dynamics of both vectors across the year.
The 2La inversion exhibits a latitudinal gradient in A. gambiae and A. coluzzii across Africa that is significantly related to climatic variables (Ayala et al., 2017). We did not observe any significant correlation between the 2La inversion frequency and variations of local environmental factors (including precipitations) in both species (Supplementary Table 5). Moreover, we investigated whether seasonal changes in inversion frequencies were associated with environmental variables, as described for other Diptera species and also mosquitoes (Hoffmann et al., 2004; Guelbeogo et al., 2009; Ayala et al., 2014; Kapun and Flatt, 2019). Rishikesh et al. (1985) detected an association between the 2La inversion frequency in A. gambiae and the season, with higher frequency in the dry season. In our study, the 2La inversion frequency remained stable throughout the year. Slight frequency variations were not consistent with the dry (November to April) and rainy seasons (May to October) (Figure 1). Similarly, we did not observe any karyotype-season association (Figure 1). One potential explanation could be the local adaptation to urban habitats. Urban settings offer more stable habitats where mosquitoes can breed in all seasons (i.e., availability of suitable breeding sites). Therefore, aridity selection could be less strong than in rural areas. However, we observed a reduction of mosquitoes during the dry season (Supplementary Table 2). A comparison with rural sites close to Bangui could elucidate the role of urban settings in the 2La inversion frequency temporal dynamics.
Variations at the local scale can affect the behavioral patterns of mosquito populations. It has been shown that within the same mosquito population, individuals differ in their choice of biting or resting locations (Carnevale et al., 2009). These changes are governed by intrinsic (genetic) and extrinsic (environmental) factors (Pates and Curtis, 2005). The 2La inversion frequency may follow microclimatic changes. Coluzzi and co-workers (Coluzzi et al., 1979) found that dryer indoor conditions favor the collections of individuals carrying the 2La inversion. In Bangui, we did not observe any significant inversion and karyotype frequency variation between outdoor and indoor locations (Figure 2). Therefore, indoor and outdoor microclimatic conditions seem not to have any effect. However, a recent study in Western Kenya showed the impact of insecticide-treated nets (ITN) on the 2La inversion frequency (Matoke-Muhia et al., 2016), although it did not find any significant difference between indoor and outdoor collections (Matoke-Muhia et al., 2016). In the city of Bangui, two successive mass distribution of ITN occurred in 2006 and 2015 (MSF, 2020) that might have influenced the 2La inversion frequency dynamics by affecting the population size of both malaria mosquitoes. Unfortunately, we do not have data on the inversion frequency before bed-net implementation in order to appreciate the ITN potential effect on the inversion frequency. We then studied whether fluctuations in relative humidity during the day could explain changes in the biting times. To this end, we performed a circular analysis of the biting events by mosquitoes harboring each karyotype (Table 2, Figure 2, and Supplementary Figures 1–3). Our results did not highlight any biting pattern difference among karyotypes. As previously reported (Sangbakembi-Ngounou et al., 2022), mosquitoes bite earlier outdoor than indoor, independently of their karyotype. However, we observed slight differences across the months. Future studies should investigate potential spatial and temporal effects on the biting activity period.
Conclusion
In our study, we investigated the impact of the 2La inversion on the population dynamics and biting behavior of A. gambiae and A. coluzzii, the two main malaria vectors in Bangui, Central African Republic. We confirmed that this inversion is highly polymorphic, providing an unique opportunity for rapid adaption to changing environments in these two important malaria mosquitoes. However, the inversion frequency is not affected by changes at the microhabitat scale. In Bangui, this inversion seems to be governed by biological forces at a larger ecological scale. The specific urban features present in Bangui, such as light, temperature or vector control measures could affect the populations dynamics of the malaria vectors, by affecting gene flow with rural populations. Further research should investigate how reduction of gene flow could affect the local adaptation pattern of this inversion. Finally, our results need to be compared in other sites within the ecological range of this inversion.
Data availability statement
The original contributions presented in this study are included in the article/Supplementary material, further inquiries can be directed to the corresponding author/s.
Ethics statement
The studies involving human participants were reviewed and approved by the Institutional Review Board of the Health Sciences Faculty of Bangui University. The patients/participants provided their written informed consent to participate in this study.
Author contributions
CS-N and DA designed and conceptualized the study. CS-N and CN coordinated the fieldwork. CS-N and OA-E performed the molecular analysis. CS-N, CC, PK, and DA performed the statistical analysis and discussed the results. CS-N and DA wrote the first draft of the manuscript. CN, EN, and DA provided funding. All authors provided critical feedback on the manuscript.
Funding
Funding was provided by the International Division of Institut Pasteur Paris, Institut Pasteur in Bangui, and the Institut de Recherche pour le Développement. The funders had no role in the study design, data collection or analysis, decision to publish, or preparation of the manuscript.
Acknowledgments
We thank the volunteers in Gbanikola, Îles des Singes, Pk10, and Ouango in Bangui for their collaboration and their compliance during the field investigations. We also thank Neil Longo Pendy, Judicael Obame, Guy-André Kpilimbi, Jonas Mbiko-Tanza, Gildas Gonetomy, and Roger Detol for their assistance and discussions.
Conflict of interest
The authors declare that the research was conducted in the absence of any commercial or financial relationships that could be construed as a potential conflict of interest.
Publisher’s note
All claims expressed in this article are solely those of the authors and do not necessarily represent those of their affiliated organizations, or those of the publisher, the editors and the reviewers. Any product that may be evaluated in this article, or claim that may be made by its manufacturer, is not guaranteed or endorsed by the publisher.
Supplementary material
The Supplementary Material for this article can be found online at: https://www.frontiersin.org/articles/10.3389/fevo.2022.986925/full#supplementary-material
Supplementary Figure 1 | Circular representation of biting events for each month in each species (standard 2La inversion karyotype). Biting events are represented by points, the kernel distributions by lines, and the mean directions (θ) and resulting lengths (R) are depicted as arrow vectors in the unit circle. Darker colors represent indoor collections, and lighter colors are outdoor collections.
Supplementary Figure 2 | Circular representation of biting events for each month, in each species (heterozygote 2La inversion karyotype). Biting events are represented by points, the kernel distributions by lines, and the mean directions (θ) and the resulting lengths (R) are depicted by arrow vectors in the unit circle. Darker colors represent indoor collections, and lighter colors are outdoor collections.
Supplementary Figure 3 | Circular representation of biting events for each month, in each species (inverted 2La inversion karyotype). Biting events are represented by points, the kernel distributions by lines, and the mean directions (θ) and the resulting lengths (R) are depicted by arrow vectors in the unit circle. Darker colors represent indoor collections, and lighter colors are outdoor collections.
Supplementary Table 1 | Monthly summary of climatic data.
Supplementary Table 2 | List of Anopheles gambiae and Anopheles coluzzii specimens used in this study. Date: Year and month of collection; inv2La: 2La inversion karyotype.
Supplementary Table 3 | Hardy-Weinberg Equilibrium tests for each species and month. The p-value was computed with the exact test. Date: Year and month of collection; number of mosquitoes with the indicated 2La inversion karyotype; Freq_2La: Frequency of the inversion 2La; HWE: Hardy-Weinberg Equilibrium.
Supplementary Table 4 | Hardy-Weinberg Equilibrium tests for each species, month, and location. The p-value was computed with the exact test.
Supplementary Table 5 | Hardy-Weinberg Equilibrium tests for each species, month, location, and site. The p-value was computed with the exact test.
Supplementary Table 6 | Generalized linear model to assess the 2La inversion frequency correlation with different climate parameters in the two species (A. gambiae and A. coluzzii) and in function of their 2La inversion karyotype.
Footnotes
References
Ayala, D., Acevedo, P., Pombi, M., Dia, I., Boccolini, D., Costantini, C., et al. (2017). Chromosome inversions and ecological plasticity in the main African malaria mosquitoes. Evolution 71, 686–701. doi: 10.1111/evo.13176
Ayala, D., Ullastres, A., and Gonzalez, J. (2014). Adaptation through chromosomal inversions in Anopheles. Front. Genet. 5:129. doi: 10.3389/fgene.2014.00129
Ayala, D., Zhang, S., Chateau, M., Fouet, C., Morlais, I., Costantini, C., et al. (2019). Association mapping desiccation resistance within chromosomal inversions in the African malaria vector Anopheles gambiae. Mol. Ecol. 28, 1333–1342. doi: 10.1111/mec.14880
Carnevale, P., Robert, V., Manguin, S., Corbel, V., Fontenille, D., Garros, C., et al. (2009). Les anophèles: Biologie, transmission du Plasmodium et lutte antivectorielle. Marseille: IRD. doi: 10.4000/books.irdeditions.10374
Coluzzi, M., Sabatini, A., Petrarca, V., and Di Deco, M. A. (1979). Chromosomal differentiation and adaptation to human environments in the Anopheles gambiae complex. Trans. R. Soc. Trop. Med. Hyg. 73, 483–497. doi: 10.1016/0035-9203(79)90036-1
Costantini, C., Ayala, D., Guelbeogo, W. M., Pombi, M., Some, C. Y. I, Bassole, H., et al. (2009). Living at the edge: Biogeographic patterns of habitat segregation conform to speciation by niche expansion in Anopheles gambiae. BMC Ecol. 9:16. doi: 10.1186/1472-6785-9-16
Dao, A., Yaro, A. S., Diallo, M., Timbine, S., Huestis, D. L., Kassogue, Y., et al. (2014). Signatures of aestivation and migration in Sahelian malaria mosquito populations. Nature 516, 387–390. doi: 10.1038/nature13987
Fontaine, M. C., Pease, J. B., Steele, A., Waterhouse, R. M., Neafsey, D. E. I, Sharakhov, V., et al. (2015). Mosquito genomics. Extensive introgression in a malaria vector species complex revealed by phylogenomics. Science 347:1258524. doi: 10.1126/science.1258524
Fouet, C., Gray, E., Besansky, N. J., and Costantini, C. (2012). Adaptation to aridity in the malaria mosquito Anopheles gambiae: Chromosomal inversion polymorphism and body size influence resistance to desiccation. PLoS One 7:e34841. doi: 10.1371/journal.pone.0034841
Gillies, M. T., and Coetzee, M. (1987). A Supplement to the Anophelinae of Africa South of the Sahara. Johannesburg: South African Institute for Medical Research.
Gillies, M. T., and De Meillon, B. (1968). The Anophelinae of Africa South of the Sahara (Ethiopian zoogeographical region).” Ethiopian Zoogeographical Region. Wallingford: Centre for Agriculture and Bioscience International.
Graffelman, J. (2015). Exploring Diallelic Genetic Markers: The HardyWeinberg Package. J. Stat. Softw. 64, 1–23. doi: 10.18637/jss.v064.i03
Graffelman, J., and Morales-Camarena, J. (2008). Graphical tests for Hardy-Weinberg Equilibrium based on the ternary plot. Hum. Hered. 65, 77–84. doi: 10.1159/000108939
Gray, E. M., Rocca, K. A., Costantini, C., and Besansky, N. J. (2009). Inversion 2La is associated with enhanced desiccation resistance in Anopheles gambiae. Malar. J. 8:215. doi: 10.1186/1475-2875-8-215
Guelbeogo, W. M., Sagnon, N., Grushko, O., Yameogo, M. A., Boccolini, D., Besansky, N. J., et al. (2009). Seasonal distribution of Anopheles funestus chromosomal forms from Burkina Faso. Malar. J. 8:239. doi: 10.1186/1475-2875-8-239
Hoffmann, A. A., Sgro, C. M., and Weeks, A. R. (2004). Chromosomal inversion polymorphisms and adaptation. Trends Ecol. Evol. 19, 482–488. doi: 10.1016/j.tree.2004.06.013
Hosmer, D. W., Jovanovic, B., and Lemeshow, S. (1989). Best subsets logistic regression. Biometrics 45, 1265–1270. doi: 10.2307/2531779
Kamgang, B., Tchapga, W., Ngoagouni, C., Sangbakembi-Ngounou, C., Wondji, M., Riveron, J. M., et al. (2018). Exploring insecticide resistance mechanisms in three major malaria vectors from Bangui in Central African Republic. Pathog. Glob. Health 112, 349–359. doi: 10.1080/20477724.2018.1541160
Kapun, M., and Flatt, T. (2019). The adaptive significance of chromosomal inversion polymorphisms in Drosophila melanogaster. Mol. Ecol. 28, 1263–1282. doi: 10.1111/mec.14871
Lenormand, T. (2002). Gene flow and the limits to natural selection. Trends Ecol. Evol. 17, 183–189. doi: 10.1016/S0169-5347(02)02497-7
Matoke-Muhia, D., Gimnig, J. E., Kamau, L., Shililu, J., Bayoh, M. N., and Walker, E. D. (2016). Decline in frequency of the 2La chromosomal inversion in Anopheles gambiae (s.s.) in Western Kenya: Correlation with increase in ownership of insecticide-treated bed nets. Parasit. Vectors 9:334. doi: 10.1186/s13071-016-1621-3
Miles, A., Harding, N., Botta, G., Clarkson, C., Antao, T., Kozak, K., et al. (2017). Genetic diversity of the African malaria vector Anopheles gambiae. Nature 552, 96–100. doi: 10.1038/nature24995
Morlais, I., Ponçon, N., Simard, F., Cohuet, A., and Fontenille, D. (2004). Intraspecific nucleotide variation in Anopheles gambiae: New insights into the biology of malaria vectors. Am. J. Trop. Med. Hyg. 71, 795–802.
MSF (2020). In times of COVID-19, Malaria Remains the Number one Killer of Children in CAR. Geneva: MSF.
Ndiath, M. O., Eiglmeier, K., Ole Sangba, M. L., Holm, I., Kazanji, M., and Vernick, K. D. (2016). Composition and genetics of malaria vector populations in the Central African Republic. Malar. J. 15:387. doi: 10.1186/s12936-016-1431-2
Ole Sangba, M. L., Sidick, A., Govoetchan, R., Dide-Agossou, C., Osse, R. A., Akogbeto, M., et al. (2017). Evidence of multiple insecticide resistance mechanisms in Anopheles gambiae populations in Bangui, Central African Republic. Parasit. Vectors 10:23. doi: 10.1186/s13071-016-1965-8
Pates, H., and Curtis, C. (2005). Mosquito behavior and vector control. Annu. Rev. Entomol. 50, 53–70. doi: 10.1146/annurev.ento.50.071803.130439
R Core Team (2021). R: A Language and Environment for Statistical Computing. Vienna: R Foundation for Statistical Computing.
Riehle, M. M., Bukhari, T., Gneme, A., Guelbeogo, W. M., Coulibaly, B., Fofana, A., et al. (2017). The Anopheles gambiae 2La chromosome inversion is associated with susceptibility to Plasmodium falciparum in Africa. Elife 6:e25813. doi: 10.7554/eLife.25813
Rishikesh, N., Di Deco, M. A., Petrarca, V., and Coluzzi, M. (1985). Seasonal variations in indoor resting Anopheles gambiae and Anopheles arabiensis in Kaduna, Nigeria. Acta Trop. 42, 165–170.
Rocca, K. A., Gray, E. M., Costantini, C., and Besansky, N. J. (2009). 2La chromosomal inversion enhances thermal tolerance of Anopheles gambiae larvae. Malar. J. 8:147. doi: 10.1186/1475-2875-8-147
Sangbakembi-Ngounou, C., Costantini, C., Longo-Pendy, N. M., Ngoagouni, C., Akone-Ella, O., Rahola, N., et al. (2022). Diurnal biting of malaria mosquitoes in the Central African Republic indicates residual transmission may be “out of control”. Proc. Natl. Acad. Sci. U.S.A. 119:e2104282119. doi: 10.1073/pnas.2104282119
Santolamazza, F., Mancini, E., Simard, F., Qi, Y., Tu, Z., and della Torre, A. (2008). Insertion polymorphisms of SINE200 retrotransposons within speciation islands of Anopheles gambiae molecular forms. Malar. J. 7:163. doi: 10.1186/1475-2875-7-163
Sharakhov, I. V., White, B. J., Sharakhova, M. V., Kayondo, J., Lobo, N. F., Santolamazza, F., et al. (2006). Breakpoint structure reveals the unique origin of an interspecific chromosomal inversion (2La) in the Anopheles gambiae complex. Proc. Natl. Acad. Sci. U.S.A. 103, 6258–6262. doi: 10.1073/pnas.0509683103
Simard, F., Ayala, D., Kamdem, G. C., Pombi, M., Etouna, J., Ose, K., et al. (2009). Ecological niche partitioning between Anopheles gambiae molecular forms in Cameroon: The ecological side of speciation. BMC Ecol. 9:17. doi: 10.1186/1472-6785-9-17
Sinka, M. E., Bangs, M. J., Manguin, S., Coetzee, M., Mbogo, C. M., Hemingway, J., et al. (2010). The dominant Anopheles vectors of human malaria in Africa, Europe and the Middle East: Occurrence data, distribution maps and bionomic precis. Parasit. Vectors 3:117. doi: 10.1186/1756-3305-3-117
Toure, Y. T., Petrarca, V., Traore, S. F., Coulibaly, A., Maiga, H. M., Sankare, O., et al. (1994). Ecological genetic studies in the chromosomal form Mopti of Anopheles gambiae s.str. in Mali, west Africa. Genetica 94, 213–223. doi: 10.1007/BF01443435
White, B. J., Cheng, C., Simard, F., Costantini, C., and Besansky, N. J. (2010). Genetic association of physically unlinked islands of genomic divergence in incipient species of Anopheles gambiae. Mol. Ecol. 19, 925–939. doi: 10.1111/j.1365-294X.2010.04531.x
Keywords: inversion 2La, biting behavior, population dynamics, Anopheles, malaria, Bangui
Citation: Sangbakembi-Ngounou C, Ngoagouni C, Akone-Ella O, Kengne P, Costantini C, Nakouné E and Ayala D (2022) Temporal and biting dynamics of the chromosomal inversion 2La in the malaria vectors Anopheles gambiae and Anopheles coluzzii in Bangui, Central African Republic. Front. Ecol. Evol. 10:986925. doi: 10.3389/fevo.2022.986925
Received: 05 July 2022; Accepted: 23 September 2022;
Published: 11 October 2022.
Edited by:
Igor V. Sharakhov, Virginia Tech, United StatesReviewed by:
Rafael Kretschmer, Federal University of Pelotas, BrazilRolando Rivera-Pomar, Universidad Nacional del Noroeste de Buenos Aires, Argentina
Copyright © 2022 Sangbakembi-Ngounou, Ngoagouni, Akone-Ella, Kengne, Costantini, Nakouné and Ayala. This is an open-access article distributed under the terms of the Creative Commons Attribution License (CC BY). The use, distribution or reproduction in other forums is permitted, provided the original author(s) and the copyright owner(s) are credited and that the original publication in this journal is cited, in accordance with accepted academic practice. No use, distribution or reproduction is permitted which does not comply with these terms.
*Correspondence: Claire Sangbakembi-Ngounou, clairesangbakembi@gmail.com; Diego Ayala, diego.ayala@ird.fr