- 1Key Laboratory of Animal Ecology and Conservation Biology, Chinese Academy of Sciences, Beijing, China
- 2Illinois Natural History Survey, Prairie Research Institute, University of Illinois, Champaign, IL, United States
Life histories involving transitions between differing habitats (i.e., aquatic to terrestrial or marine to freshwater) require numerous anatomical, physiological, and behavioral changes. Often, the traits associated with these changes are thought to come in suites, but all traits thought to be associated with particular life histories may not be required. While some traits are found in all species with a particular habitat transition, a grab bag approach may apply to other traits in that any trait may be sufficient for successful habitat transitions. We examine patterns of morphological traits associated with prolonged emersion in mudskipper, an amphibious fishes clade, where prolonged emersion appears twice. We test the evolutionary history of multiple characteristics associated with cutaneous respiration. We find most traits thought to be key for prolonged emersion show no phylogenetic signal and no tight correlation with prolonged emersion. Such traits appear in species with prolonged emersion but also non-emerging species. Only capillary density, which, when increased, allows for increased oxygen absorption, shows strong phylogenetic signal and correlation with prolonged emersion. Further experimental, functional genomics, and observational studies are needed to fully understand the mechanisms associated with each of these traits. With respect to traits associated with other particular behaviors, a comparative framework can be helpful in identifying evolutionary correlates.
Introduction
The ability to survive in a new environment often requires numerous behavioral, anatomical, and physiological traits. Life histories that allow for movement between habitats, such as transitions between marine and freshwater, aquatic and terrestrial, and flight and flightless, are all common across the tree of life (Shubin et al., 2006; Corush, 2019; Sackton et al., 2019). In some cases, the traits associated with these life histories, or simply occupying a new environment in the case of migration or invasion, are thought to come in suites (Dingle, 2006; Colmer and Voesenek, 2009; Carnicer et al., 2013; Chuang and Riechert, 2021; but also see Piersma et al., 2005). It is convenient to consider the full suite of traits associated with each life history to be necessary. But parsing apart the traits that are necessary for persistence, as opposed to additional adaptive traits that are not necessary for survival in a new environment, is important in order to understand the evolution of such life histories. This is especially problematic in a single-species framework where a group of traits is associated with a life history and then each trait is assumed to be necessary. Examining suites of traits in a phylogenetic framework can be more useful in identifying specific traits that may be required for each life history.
Traits associated with aquatic–terrestrial movement in fishes have been examined with respect to fin kinematics (Pace and Gibb, 2009), morphological and molecular basis of vision (Sayer, 2005; Hu et al., 2022), vasculatures of skin, gills, and bucco-opercular cavities associated with respiration (Zhang et al., 2000; Gonzales et al., 2011), body elongation and compressions (Polgar et al., 2017; Egan et al., 2021), specialization in feeding and diet (Kruitwagen et al., 2007; Ord and Hundt, 2020; Tran et al., 2022), shifts in dominant bacteria in the gut (Guan et al., 2021), vocalizations (Polgar et al., 2011), burrowing behaviors (Ishimatsu et al., 1998; Dinh et al., 2021), candidate genes associated with emersion (You et al., 2014), and the effects of amphibious behavior on population structure (Corush et al., 2022). In many of these examples, multiple behavioral, physiological, and anatomical traits work together to allow fish to emerge. While few studies do take a comparative approach, e.g., two studies looking at body shape and diet of blennies (Blenniidae) in supralittoral and intertidal zones (Ord and Hundt, 2020; Egan et al., 2021), these traits are typically examined in a single, if not a few, species, and also in species that show a more extreme level of transition, making it tempting to assume that the suite of traits are all required for the evolution of the behavior. Speciose groups such as mudskippers (Gobiidae: Oxudercidae: subfamily Oxudercinae), however, have a complex evolutionary history with respect to the polyphyletic distribution of prolonged emersion (Steppan et al., 2022). This raises questions regarding the evolutionary history of the various traits associated with emersion. Understanding the phylogenetic signal of these traits and their correlation to emersion behavior is important for a broader understanding of how and why fish move onto land.
Respiration in emerging fishes is of interest because most fish species are unable to maintain necessary oxygen levels out of water. Across fishes that can utilize atmospheric oxygen, a number of organs are used including gills, skin, gastrointestinal tract, labyrinthine organs, and the swim bladder [for an in-depth review of air breathing and gas exchange in fishes see Graham (1997); Sayer (2005); Ishimatsu (2017) and Zaccone et al. (2018)]. Mudskippers, however, lack organs such as the gas bladder (Polgar et al., 2011) which is used by other air-breathing fishes including gars (Lepisosteus and Atractosteus). The skin is important in mudskippers as cutaneous respiration is a primary respiration method for some species when out of water, although gills are also used (Tamura et al., 1976) as well as bucco-opercular cavity (Ishimatsu, 2017). Within mudskippers, there is variation in gas exchange. For example, Periophthalmodon schlosseri are more effective in oxygen uptake out of water compared to in water (Kok et al., 1998) even after “exhaustive exercise” (Takeda et al., 1999). Others, such as Boleophthalmus boddaerti, rely more heavily on aquatic respiration, although they are capable of surviving long periods out of water (Kok et al., 1998). Other species within the mudskipper clade are fully aquatic, although some such as Apocryptodon punctatus may gulp air as suggested by Graham and Lee (2004).
One organ that has received particularly wide taxonomical attention is the skin (Al-Kadhomiy and Hughes, 1988; Yokoya and Tamura, 1992; Zhang et al., 2000; Park, 2002; Park et al., 2003; Zhang et al., 2003; Park et al., 2006; Beon et al., 2013; Jaafar and Parenti, 2017; Kim and Park, 2020). Within the dermal layers, there is a suite of traits that increases the ability to absorb oxygen when fishes are out of water. These traits include an increase in capillary density in the skin allowing for more absorption, as well as a decrease in the distance between those capillaries and the surface of the skin, and decrease in the distance oxygen must travel to get to the blood vessels (Al-Kadhomiy and Hughes, 1988; Beon et al., 2013). Additionally, dermal bulges often contain additional capillaries and are associated with cutaneous respiration (Beon et al., 2013). The presence of mucous cells has been observed to decrease within species during emersion (Sayer and Blackstock, 1988) as the mucus may restrict oxygen flow (Zhang, 2001) although it is also suggested that this may help increase diffusion (Graham, 1997). Chloride cells are associated with ion regulation and are often associated with respiratory organs (Copeland, 1948; Komnick, 1986). Middle cells may also act as a barrier to water loss and store large amounts of water (Yokoya and Tamura, 1992; Figure 1). Because most of these traits do not require a novel feature, but rather an increase or decrease in an already existent trait (i.e., more blood capillary or thicker cells), the “gain” (increase in the trait) and “loss” (decrease in the trait) is likely more reversible as opposed to traits typically thought of in the context of Dollo’s law (Dollo, 1893) which states that once a complex trait is lost, it should not be reacquired (e.g., the regain of a digit in the lizard genus Bachia: Galis et al., 2010).
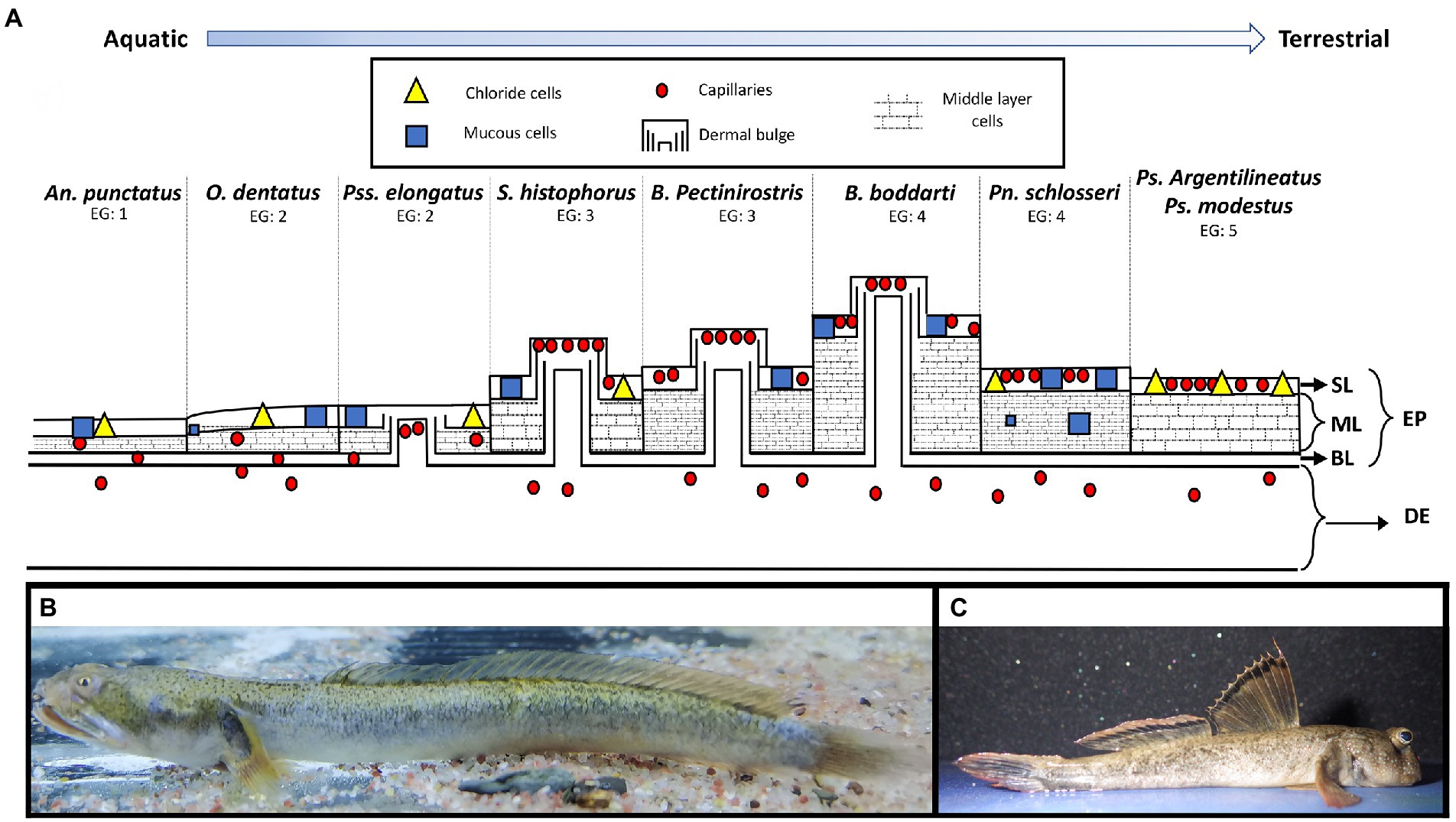
Figure 1. (A) Schematic representation of the variation in histological features from the head of mudskipper species representing different emission groups from fully aquatic (emersion group EG1) (left) to highly terrestrial (EG5) (Right). Layers include the dermis (DE) and epidermis (EP), which included a superficial layer (SL), middle layer (ML), and basal layer (BL). Figure modified from Zhang (2001). Morphological variation between EGs can be seen between (B), Oxuderces dentatus (EG2) collected in Panyu, Guangdong Province, China, in September 2022 (Photo credit: Minzheng Li) and (C), Periophthalmus magnuspinnatus (EG5) (not used in this study, but representative of the “P clade” in figure 2) collected from the Bohai Sea, China, in July 2016 (Photo credit: Joel B. Corush).
Of the seven genera of mudskippers, there is a wide range of emersion behavior from fully aquatic, emersion group (EG) 1, to primarily terrestrial, EG 5 (See Supplementary Table 1 for the description of each EG). According to recent phylogenetic analyses (McCraney et al., 2020; Steppan et al., 2022), there are two monophyletic groupings containing the species known for prolonged emersion (EG 4–5). The first clade contains the species of the genera Periophthalmus and Periophthalmodon (clade P: EG 4–5) and the second clade, nested within a less frequent, less prolonged emersion species, is the genus Boleophthalmus (clade B: EG 4, but also contains species in EG 3; Figure 2). It is unknown how the traits associated with prolonged emersion have changed across the phylogeny. Additionally, it is unknown if the same suite of traits is found in all species with prolonged emersion. If only a few adaptive traits are needed to successfully spend time out of water, different species might exhibit different traits. These points can be addressed by understanding the phylogenetic signal of traits associated with emersion.
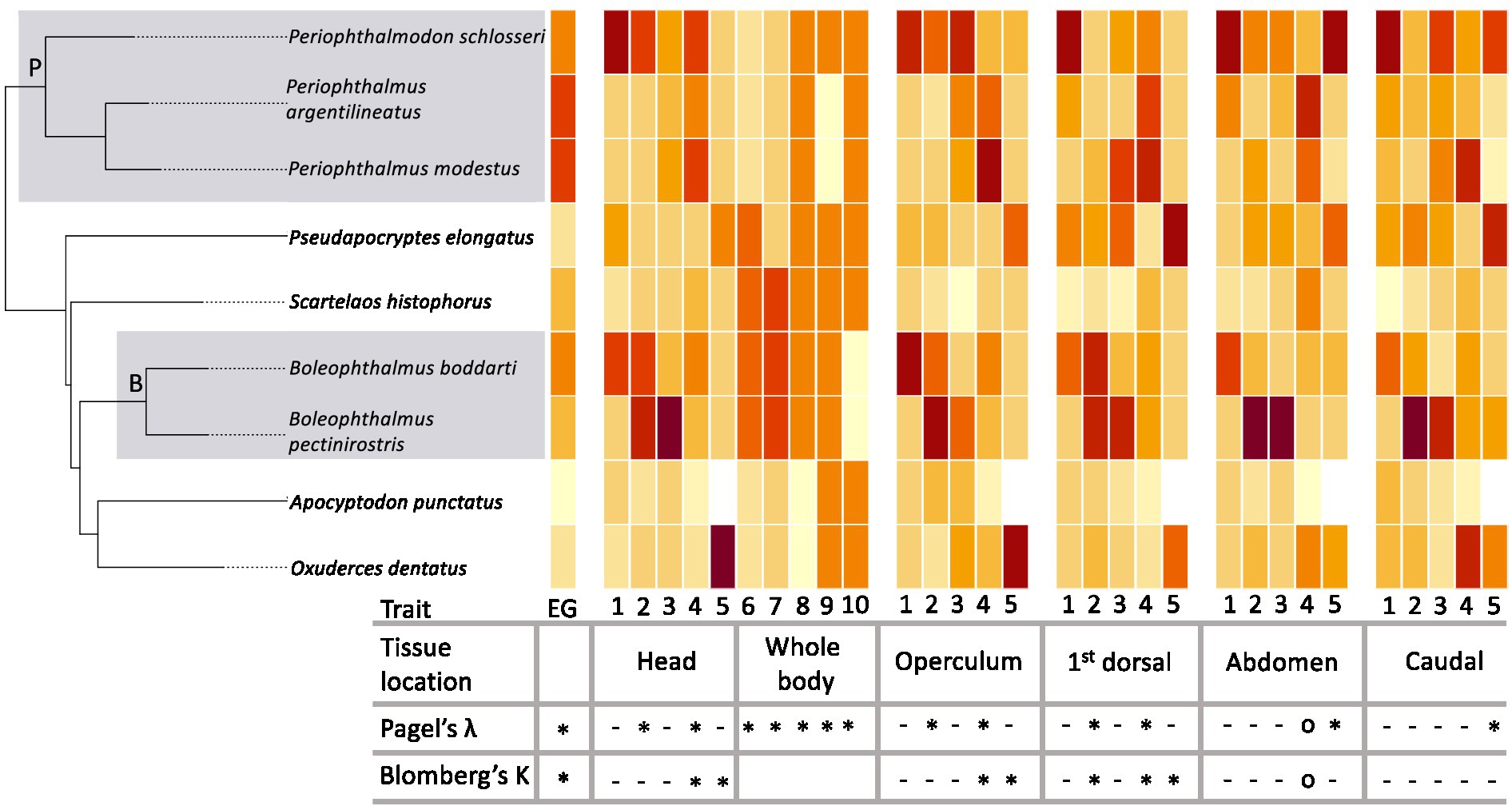
Figure 2. Pruned phylogeny (from McCraney et al., 2020) and a heatmap of associated trait values. Traits estimation based on mean values of each species which is based on mean values for each. Traits include: (1) number of scale layers (#), (2) tier of middle cells (#), (3) middle layer thickness (μm), (4) capillary density (#/mm), (5) diffusion distance (μm), (6) dermal bulge, (7) papilla, (8) Intra-epidermal capillary (skin), (9) mucous cells, and (10) chloride cell. Traits 6–10 are presence/absence across the full specimen. Dark colors = higher/presence. Light colors = low/absence trait values (see Supplementary Table 2 for actual values). Shaded boxes indicate clade P and clade B which have prolonged emersion. Table below represents phylogenetic signal based on Pagel’s λ and Blomberg’s K (See supplementary Table 4 for actual values). Traits and tissues combinations that show a strong phylogenetic signal are denoted with an “∗” (λ > 0.9, K > 1.0), traits and tissues combinations that show an intermediate phylogenetic signal are denoted with an “o” (0.5 < λ < 0.9, 0.9 < K < 1.0), and traits and tissues combinations that show a little to no phylogenetic signal are denoted with a “-” (λ < 0.5, K < 0.9). Blomberg’s K was not calculated for the whole body binary traits.
Phylogenetic signal is a measurement of the randomness of a trait’s distribution across a phylogeny. If a trait is randomly distributed across a phylogeny, then there is no signal. If this is the case, traits are not often shared by sister species or closely related species, but instead found randomly across the phylogeny. However, if a trait shows a nonrandom pattern with respect to phylogeny, then we could consider there to be a strong phylogenetic signal. In this case, traits tend to be found in closely related species or conversely, not found in closely related species. Two standard metrics of phylogenetic signal include Pagel’s λ (Pagel, 1997; Pagel, 1999) and Blomberg’s K (Blomberg et al., 2003). Pagel’s λ ranges from 0 (no phylogenetic signal) to 1 (strong phylogenetic signal). Blomberg’s K starts from 0 (no phylogenetic signal) and continues infinitely with K = 1 being a threshold for phylogenetic signal and increasing values indicating a stronger signal. Strength of phylogenetic signal alone is not an indicator of an association between traits. Two traits may both have strong phylogenetic signals because they are restricted to different clades. For example, in this study, we found a strong phylogenetic signal for dermal bulges and emersion behavior, but these traits appear in different clades. Additional tests are needed to show the association between traits.
We used histological data from Zhang (2001), coupled with a published phylogeny (McCraney et al., 2020) to identify the evolutionary pattern of skin adaptations across multiple species of mudskippers. This suite of traits was used in part because of the availability of data on multiple traits across species that do and do not emerge. We address the following questions: (1) Are the specific traits that are thought to be associated with prolonged emersion present in all species with prolonged emersion and absent in species without prolonged emersion? (2) Do the traits appear in suites or are these traits irregularly distributed across species with prolonged emersion? (3) Is there a phylogenetic signal associated with each of the traits?
Materials and methods
Histology
Histological data were summarized by Zhang (2001) for the giant mudskipper (Periophthalmodon schlosseri; Pallas, 1774), the barred mudskipper (Periophthalmus argentilineatus; Cuvier and Valenciennes, 1837), shuttles hoppfish (Periophthalmus modestus; Cantor, 1842), the elongate mudskipper (Pseudapocryptes elongatus; Cuvier, 1816), the walking goby (Scartelaos histophorus; Cuvier and Valenciennes, 1837), Boddart’s goggle-eyed goby (Boleophthalmus boddarti; Pallas, 1774), the great blue-spotted mudskipper (Boleophthalmus pectinirostris; Linnaeus, 1758), the spotted hidden-teeth goby (Apocryptodon punctatus; Tomiyama, 1934), and the crocodile-face goby (Oxuderces dentatus; Eydoux and Souleyet, 1852). For each sample, measurements were taken from the following regions of the body: (1) top of the head (H), (2) outer surface of operculum (O), (3) dorso-lateral body near the first dorsal fin (D), (4) posterior portion of the abdomen (A), and (5) the lateral side of the caudal peduncle (C). Traits measured at each of the regions included: (1) number of scale layers (#), (2) tier of middle cells (#), (3) middle layer thickness (μm), (4) capillary density (#/mm), and (5) diffusion distance (μm). Additionally, the following traits were examined as presence/absence across the full specimen: (6) drmal bulge, (7) papilla, (8) intra-epidermal capillary, (9) mucous cells, and (10) chloride cell. Lastly, each species was assigned an emersion period (EG 1–5) based on how much time they spent out of water. Values used for phylogenetic analyses were the means across all individuals of each species as recorded in Zhang (2001). If minimum and maximum values were measured in Zhang (2001), as in tier of middle cells and middle layer thickness, the mean of the minimum and maximum was used (Supplementary Table 2). The number of scale layers was considered 0 if there was not a complete layer of scales (i.e., noncontinuous scales that are completely embedded in the skin with spacing between scales; Figure 3). A heatmap of trait values was constructed using the ‘heatmap’ function in the basic stats package in R (R Core Team, 2019).
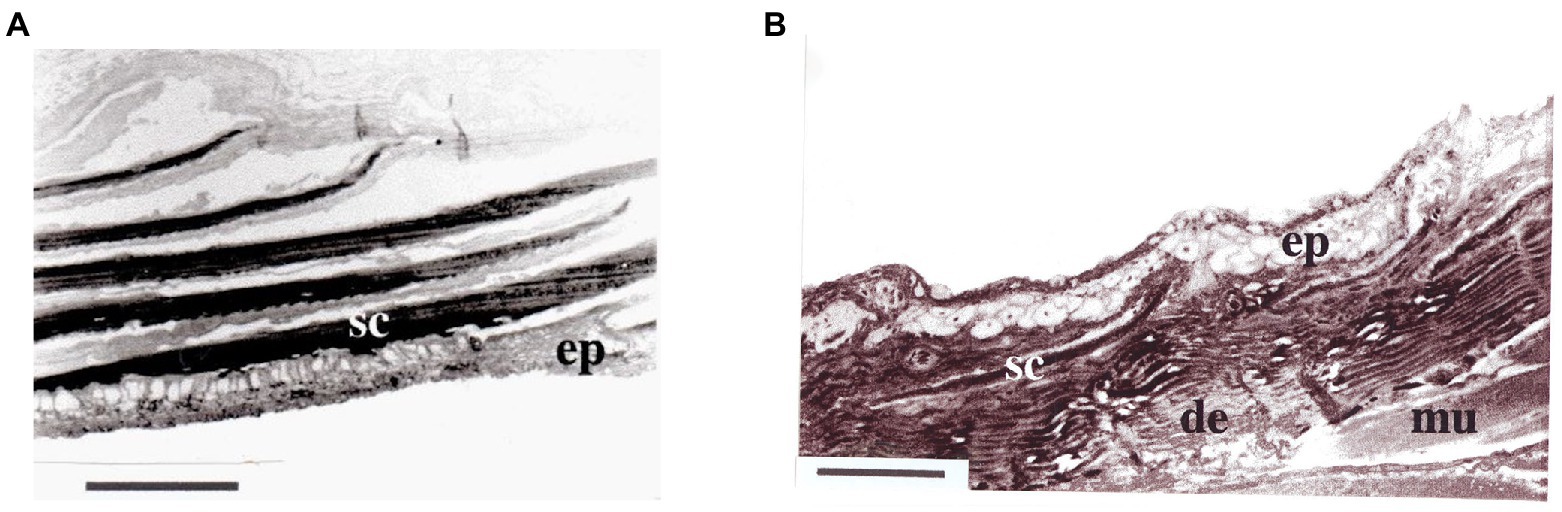
Figure 3. Examples of variation in scale counts from different species of mudskipper from Zhang (2001). Images depict the (A), transverse section of the operculum of Periophthalmodon septemradiatus (not included in this study) and (B), lateral side of the caudal peduncle of Scartelaos histophorus. sc, scales; ep, epidermis; de, dermis; mu, muscle. Scale bars indicate 100 μm. Pn. septemradiatus has continuous multilayered scales, whereas S. histophorus has isolated embedded scales.
Phylogenetic analysis
A recent goby phylogeny (McCraney et al., 2020) was pruned to include only the taxa of interest (Figure 2) using the “drop.tip” function in the ape package (Paradis et al., 2004). Phylogenetic signal was calculated using the “phylosig” function in the phytools package with both method = “K” and “lambda” (Revell, 2012). For discrete traits, the “fitDiscrete” function in Geiger (Harmon et al., 2008) was used to calculate Pagel’s λ. Because of the small number of taxa in this study, a permutation test was performed to assess the significance of these values. The permutation test was performed by randomly arranging the data and recalculating both metrics of phylogenetic signal. This was done 1,000 times for each trait and tissue type using Pagel’s λ and Blomberg’s K. Observed values were compared to the distribution of simulated values.
To identify the correlation between traits, Pearson’s product-moment correlation coefficient (r) was calculated using the “cor.test” with methods = “pearson” in the basic stats package in R. In order to incorporate evolutionary history, two separate methods were used depending on the type of data. To look at correlations, phylogenetic generalized least squares (PGLS) were used to identify significant associations between pairs of traits. This was done using the “glm” function in the nlem package (Pinheiro et al., 2017). For the correlation argument, phylogeny was incorporated with the “corBrownian” function in the ape package. Because PGLS should not be used between two binary traits, phylogenetic generalized linear mixed models (PGLMM) were used to identify significant associations between pairs of binary traits (Ives and Garland, 2014; Symonds and Blomberg, 2014). To look at the correlation between binary traits (all the non-tissue specific traits - dermal bulge, papilla, intra-epidermal capillary, mucous cells, and chloride cells), the “binaryPGLMM” function in the ape package (Pearse et al., 2015) was used. Because of the number of independent correlations, a Bonferroni correction for multiple comparisons was completed using the “p.adjust” function in the basic stats function in R (see supplementary file for example code).
Results/discussion
We find that while some of the traits associated with prolonged emersion do tend to show up in all species with prolonged emersion and not others, this is not the observed pattern across most traits. Many traits thought to be associated with emersion are randomly distributed in species in both high and low EGs. This is evident by the β coefficient of only one trait, capillary density, showing a significant effect of emersion groups when incorporating phylogenetic history (Supplementary Table 3). This suggests that increased capillary density may be a key component of emersion in mudskippers. It is also worth noting that this trait showed a significant correlation in tissues H (β = 0.109, p = 0.011), O (β = 0.137, p = 0.026), D (β = 0.109, p = 0.005), but not A (β = 0.349, p = 1.0), and C (β = 0.167, p = 1.0) after corrections for multiple comparisons. This is biologically meaningful because even species such as Periophthalmus modestus and Periophthalmus argentilineatus, which are both in EG 5, often have their abdominal region and their tails in, or pressed against, water as they sit on wet mud, or on a rock or log with the posterior part of their body submerged. Because those body parts are not being exposed to air, individuals may not allocate resources to increase capillary density in those regions.
Traits associated with prolonged emersion do not appear to show up in suites and very few traits showed any correlation with other traits. Two traits that did show near significant correlation in tissues A (β = 0.034, p = 0.055) but not C (β = 0.083, p = 1.0), D (β = 0.082, p = 1.0), H (β = 0.054, p = 0.22), or tissues O (β = 0.062, p = 1.0; Supplementary Table 3), were tier of middle cells and middle layer thickness, which would be expected since an increased number of cells in a layer should increase the overall layer thickness. Additionally, tier of middle cells shows a significant association with chloride cells, but only in tissue D (β = −9.545, p < 0.001). The importance of incorporating phylogenetic independent contrasts can be seen by the numerous traits that did show a significant r, but not when incorporating the phylogenetic relationship. The overall lack of correlation in traits implies that, while there may be various traits that are adaptive with respect to emersion behavior, different lineages may make use of different combinations of traits (Supplementary Table 3).
Lastly, many of the traits do appear to show a phylogenetic signal (Figure 2). Emersion group did show a strong phylogenetic signal with both Pagel’s λ (λ = 0.999) and Blomberg’s K (K = 1.608) as did dermal bulge, papilla, mucous cells, intra-epidermal capillary (skin), and chloride cell. Some traits, such as dermal bulges, have a very apparent phylogenetic signal, but are found in the species with the highest EG (e.g., Clade B) as well as species with the lowest EG (e.g., Pseudapocryptes elongatus). Capillary density also showed a strong phylogenetic signal according to both metrics in tissues H, O, and D, but not in A or C (Figure 2, Supplementary Table 4). This is also the only trait that shows a strong correlation with EG. The permutation tests of all traits showing a strong phylogenetic signal resulted in observed values of Pagel’s λ and Blomberg’s K to be significantly higher than randomized data (p < 0.05; Supplementary Figure 1).
Both phylogenetic and histological data are subject to some level of variation based on the methods used. We acknowledge that scales may be lost during handling of specimens, but the lack of scales on the operculum of many species is consistent with Boleophthalmus species’ descriptions by Murdy (1989). The lack of scales on the head of Oxuderces dentatus is also consistent with previous descriptions (Jaafar and Parenti, 2017). Additionally, Murdy (1989) describes a widespread lack of scales on Zappa, while not a represented genus in our study, this suggests there is variability within the clade. Therefore, the lack of scales in the Scartelaos is assumed to be correct, not a product of handling the specimens. A larger number of samples would be helpful in a better understanding of the species-specific variation found in these traits. This could be particularly important in species that are found in both temperate and tropical regions (e.g., Periophthalmus argentilineatus) as they may alter their morphology to adjust to local conditions. Additional data on the remaining taxa in the clade, particularly unsampled genera (e.g., Apocryptes, Parapocryptes, Zappa, Taenioides, and Odontamblyopus) as well as a more complete phylogeny will better inform our understanding of how these adaptive traits have evolved across the Oxudercinae radiation. The increase in annotated mudskipper genomes (Li et al., 2022; Yang et al., 2022) may also help identify specific candidate genes associated with specific traits. Furthermore, the location of the sampled skin is important to consider (Zhang et al., 2003), species such as those in the genus Oxuderces may not fully emerge, with the ventral portion of their body remaining submerged all the time. This should result in the skin on their head having traits more closely associated with emersion whereas the skin on the ventral side has traits more closely related with a typical fish.
These results can be helpful in identifying specific adaptive traits worth further examination when addressing questions about emersion behavior. If the patterns observed in this study are in fact causal, we expect to see the change in capillary density expanded across the full mudskipper clade. Similar mechanisms may also be observed across other groups that emerge, such as blennies and catfishes, although these groups may utilize other traits. This is also interesting in the context of individual and species-specific variation. Mudskippers have a fully aquatic larval phase which may require less vascularization. Additionally, humidity and temperature differences in temperate compared to tropical environments may lead to different mechanisms in oxygen uptake in species that span both environments such as Periophthalmus argentilineatus. This methodology can also be applied to identify behavioral, anatomical, and physiological correlates to other life histories involving transitions between habitats such as marine to freshwater, shifts in thermal tolerance, invasive species, or species’ response to climate change.
Data availability statement
The original contributions presented in the study are included in the article/Supplementary material, further inquiries can be directed to the corresponding authors.
Author contributions
JC and JZ designed the study. JZ collected the histological data as part of her dissertation research (Zhang, 2001). JC conducted the phylogenetic analysis. JC prepared the manuscript with input from JZ. All authors contributed to the article and approved the submitted version.
Funding
Travel for this collaboration was made possible by NSF-EAPSI 2016 (Award#1614100) to JBC. This study was partly funded by the JSPS Invitation Fellowship Program for Research in Japan and the Strategic Priority Research Program of Chinese Academy of Sciences (XDA19050202), both to JZ.
Acknowledgments
We would like to thank Minzheng Li for the use of the Oxuderces dentatus photograph. We would like to thank Milton Tan for providing comments on the manuscript. We would also like to thank two reviewers for their insightful comments.
Conflict of interest
The authors declare that the research was conducted in the absence of any commercial or financial relationships that could be construed as a potential conflict of interest.
Publisher’s note
All claims expressed in this article are solely those of the authors and do not necessarily represent those of their affiliated organizations, or those of the publisher, the editors and the reviewers. Any product that may be evaluated in this article, or claim that may be made by its manufacturer, is not guaranteed or endorsed by the publisher.
Supplementary material
The Supplementary material for this article can be found online at: https://www.frontiersin.org/articles/10.3389/fevo.2022.967067/full#supplementary-material
References
Al-Kadhomiy, N. K., and Hughes, G. M. (1988). Histological study of different regions of the skin and gills in the mudskipper, Boleophthalmus boddarti with respect to their respiratory function. J. Mar. Biol. Assoc. U. K. 68, 413–422. doi: 10.1017/S0025315400043319
Beon, M. S., Oh, M. K., Lee, Y. J., Kim, C. H., and Park, J. Y. (2013). A comparative study on vascularization and the structure of the epidermis of an amphibious mudskipper fish, S cartelaos gigas (G obiidae, T eleostei), on different parts of the body and the appendages. J. Appl. Ichthyol. 29, 410–415. doi: 10.1111/jai.12038
Blomberg, S. P., Garland, T. Jr., and Ives, A. R. (2003). Testing for phylogenetic signal in comparative data: behavioral traits are more labile. Evolution 57, 717–745. doi: 10.1111/j.0014-3820.2003.tb00285.x
Cantor, T. (1842). General features of Chusan, with remarks on the flora and fauna of that island. J. Nat. Hist. 9, 265–278.
Carnicer, J., Barbeta, A., Sperlich, D., Coll, M., and Peñuelas, J. (2013). Contrasting trait syndromes in angiosperms and conifers are associated with different responses of tree growth to temperature on a large scale. Front. Plant Sci. 4:409. doi: 10.3389/fpls.2013.00409
Chuang, A., and Riechert, S. E. (2021). Personality shifts are inconsistent across two rapid range expansions of a non-native spider. Anim. Behav. 173, 67–79. doi: 10.1016/j.anbehav.2020.12.018
Colmer, T. D., and Voesenek, L. A. C. J. (2009). Flooding tolerance: suites of plant traits in variable environments. Funct. Plant Biol. 36, 665–681. doi: 10.1071/FP09144
Copeland, D. E. (1948). The cytological basis of chloride transfer in the gills of Fundulus heteroclitus. J. Morphol. 82, 201–227. doi: 10.1002/jmor.1050820204
Corush, J. B. (2019). Evolutionary patterns of diadromy in fishes: more than a transitional state between marine and freshwater. BMC Evol. Biol. 19, 1–13. doi: 10.1186/s12862-019-1492-2
Corush, J. B., Pierson, T. W., Shiao, J. C., Katayama, Y., Zhang, J., and Fitzpatrick, B. M. (2022). Amphibious mudskipper populations are genetically connected along coastlines, but differentiated across water. J. Biogeogr. 49, 767–779. doi: 10.1111/jbi.14345
Cuvier, G. (1816). Le règne animal distribué d’après son organisation: pour servir de base à l’histoire naturelle des animaux et d’introduction à l’anatomie compare. (Paris, Chez Déterville: Louis Hauman et Compe., libraires-éditeurs), Vol 2.
Cuvier, G. L. C. F. D., and Valenciennes, A. (1837). Histoire naturelle des poissons. Tome douzième. Suite du livre quatorzième. Gobioïdes. Livre quinzième. Gobioïdes. Livre quinzième. Acanthoptérygiens à pectorales pédiculées. (Paris: Pitois-Levrault), Vol 12, 344–368.
Dingle, H. (2006). Animal migration: is there a common migratory syndrome? J. Ornithol. 147, 212–220. doi: 10.1007/s10336-005-0052-2
Dinh, Q. M., Tran, L. T., Phan, T. T., Bui, M. T., Nguyen, T. T. K., Tran, D. D., et al. (2021). Burrow structure and utilization in the mudskipper Periophthalmodon septemradiatus from the Mekong Delta. J. Zool. 314, 72–83. doi: 10.1111/jzo.12861
Egan, J. P., Buser, T. J., Burns, M. D., Simons, A. M., and Hundt, P. J. (2021). Patterns of body shape diversity and evolution in intertidal and subtidal lineages of combtooth blennies (Blenniidae). Integrative Organismal Biology 3:obab004. doi: 10.1093/iob/obab004
Eydoux, F., and Souleyet, L. F. A. (1852). Voyage autour du monde exécuté pendant les années 1836 et 1837 sur la corvette “La Bonite”: Zoologie. Arthus Bertrand, éditeur.
Galis, F., Arntzen, J. W., and Lande, R. (2010). Dollo’s law and the irreversibility of digit loss in Bachia. Evolution: Intern. J. Organic Evolution 64, 2466–2476. doi: 10.1111/j.1558-5646.2010.01041.x
Gonzales, T. T., Katoh, M., Ghaffar, M. A., and Ishimatsu, A. (2011). Gross and fine anatomy of the respiratory vasculature of the mudskipper, Periophthalmodon schlosseri (Gobiidae: Oxudercinae). J. Morphol. 272, 629–640. doi: 10.1002/jmor.10944
Graham, J. B. (Ed.) (1997). Air-breathing fishes: Evolution, diversity, and adaptation. Netherland: Elsevier.
Graham, J. B., and Lee, H. J. (2004). Breathing air in air: in what ways might extant amphibious fish biology relate to prevailing concepts about early tetrapods, the evolution of vertebrate air breathing, and the vertebrate land transition? Physiol. Biochem. Zool. 77, 720–731. doi: 10.1086/425184
Guan, F., Shen, L., Zhou, X., Chen, Z., Yu, C., Zhang, J., et al. (2021). Effects of underwater and semi-aquatic environments on gut tissue and microbiota of the mudskipper Boleophthalmus pectinirostris. J. Comp. Physiol. B. 191, 741–753. doi: 10.1007/s00360-021-01380-y
Harmon, L. J., Weir, J. T., Brock, C. D., Glor, R. E., and Challenger, W. (2008). GEIGER: investigating evolutionary radiations. Bioinformatics 24, 129–131. doi: 10.1093/bioinformatics/btm538
Hu, W., Mu, Y., Lin, F., Li, X., and Zhang, J. (2022). New insight into visual adaptation in the mudskipper cornea: from morphology to the cornea-related COL8A2 gene. Front. Ecol. Evol. 10:370. doi: 10.3389/fevo.2022.871370
Ishimatsu, A. (2017). “Respiratory and circulatory adaptations,” in Fishes Out of Water. eds. Z. Jaafar and E. O. Murdy (CRC Press), 111–136.
Ishimatsu, A., Hishida, Y., Takita, T., Kanda, T., Oikawa, S., Takeda, T., et al. (1998). Mudskippers store air in their burrows. Nature 391, 237–238. doi: 10.1038/34560
Ives, A. R., and Garland, T. (2014). Phylogenetic regression for binary dependent variables. In modern phylogenetic comparative methods and their application in evolutionary biology (pp. 231–261). Springer, Berlin, Heidelberg.
Jaafar, Z., and Parenti, L. R. (2017). Systematics of the mudskipper genus Oxuderces Eydoux & Souleyet 1848 (Teleostei: Gobiidae: Oxudercinae) with resurrection from synonymy of O. nexipinnis (cantor 1849). Zool. J. Linnean Soc. 180, 195–215. doi: 10.1111/zoj.12482
Kim, H. T., and Park, J. Y. (2020). Microscopic research on the olfactory organ of the blue spotted mudskipper Boleophthalmus pectinirostris, compared to a related sympatric mudskipper. Ocean Sci. J. 55, 563–572. doi: 10.1007/s12601-020-0042-6
Kok, W. K., Lim, C. B., Lam, T. J., and Ip, Y. K. (1998). The mudskipper Periophthalmodon schlosseri respires more efficiently on land than in water and vice versa for Boleophthalmus boddaerti. J. Exp. Zool. 280, 86–90. doi: 10.1002/(SICI)1097-010X(19980101)280:1<86::AID-JEZ10>3.0.CO;2-U
Komnick, H. (1986). “Chloride cells and salt glands,” in Biology of the integument. eds. J. Bereiter-Hahn, A. G. Matoltsy, and K. Richards (Berlin, Heidelberg: Springer), Vol 11, 499–516.
Kruitwagen, G., Nagelkerken, I., Lugendo, B. R., Pratap, H. B., and Wendelaar Bonga, S. E. (2007). Influence of morphology and amphibious life-style on the feeding ecology of the mudskipper Periophthalmus argentilineatus. J. Fish Biol. 71, 39–52. doi: 10.1111/j.1095-8649.2007.01508.x
Li, X. Z., Qi, J., Corush, J., Chen, J., and Zhang, J. (2022). A chromosome-level genome assembly of the walking goby (Scartelaos histophorus). Front. Mar. Sci. 9:966275. doi: 10.3389/fmars.2022.966275
McCraney, W. T., Thacker, C. E., and Alfaro, M. E. (2020). Supermatrix phylogeny resolves goby lineages and reveals unstable root of Gobiaria. Mol. Phylogenet. Evol. 151:106862. doi: 10.1016/j.ympev.2020.106862
Murdy, E. O. (1989). A taxonomic revision and cladistic analysis of the oxudercine gobies (Gobiidae: Oxudercinae). Records of the Australian Museum, Supplement 11, 1–93. doi: 10.3853/j.0812-7387.11.1989.93
Ord, T. J., and Hundt, P. J. (2020). Crossing extreme habitat boundaries: Jack-of-all-trades facilitates invasion but is eroded by adaptation to a master-of-one. Funct. Ecol. 34, 1404–1415. doi: 10.1111/1365-2435.13600
Pace, C. M., and Gibb, A. C. (2009). Mudskipper pectoral fin kinematics in aquatic and terrestrial environments. J. Exp. Biol. 212, 2279–2286. doi: 10.1242/jeb.029041
Pagel, M. (1997). Inferring evolutionary processes from phylogenies. Zool. Scr. 26, 331–348. doi: 10.1111/j.1463-6409.1997.tb00423.x
Pagel, M. (1999). Inferring the historical patterns of biological evolution. Nature 401, 877–884. doi: 10.1038/44766
Pallas, P. S. (1774). Spicilegia zoologica: Quibus Novae Imprimis Et Obscurae Animalium Species Iconibus, Descriptionibus Atque Commentariis Illustrantur. Fasciculus Decimus. (Lange). Vol. 1.
Paradis, E., Claude, J., and Strimmer, K. (2004). APE: analyses of phylogenetics and evolution in R language. Bioinformatics 20, 289–290. doi: 10.1093/bioinformatics/btg412
Park, J. Y. (2002). Structure of the skin of an air-breathing mudskipper, Periophthalmus magnuspinnatus. J. Fish Biol. 60, 1543–1550. doi: 10.1111/j.1095-8649.2002.tb02446.x
Park, J. Y., Kim, I. S., and Lee, Y. J. (2006). A study on the vascularization and structure of the epidermis of the air-breathing mudskipper, Periophthalmus magnuspinnatus (Gobiidae, Teleostei), along different parts of the body. J. Appl. Ichthyol. 22, 62–67. doi: 10.1111/j.1439-0426.2006.00696.x
Park, J. Y., Lee, Y. J., Kim, I. S., and Kim, S. Y. (2003). A comparative study of the regional epidermis of an amphibious mudskipper fish, Boleophthalmus pectinirostris (Gobiidae, Pisces). Folia Zoologica-Praha 52, 431–440.
Pearse, W. D., Cadotte, M. W., Cavender-Bares, J., Ives, A. R., Tucker, C. M., Walker, S. C., et al. (2015). Pez: Phylogenetics for the environmental sciences. Bioinformatics 31, 2888–2890. doi: 10.1093/bioinformatics/btv277
Piersma, T., Perez-tris, J., Mouritsen, H., Bauchinger, U. L. F., and Bairlein, F. (2005). Is there a “migratory syndrome” common to all migrant birds? Ann. N. Y. Acad. Sci. 1046, 282–293. doi: 10.1196/annals.1343.026
Pinheiro, J., Bates, D., DebRoy, S., Sarkar, D., Heisterkamp, S., Van Willigen, B., et al. (2017). Package ‘nlme’. Linear and nonlinear mixed effects models, version, 3.
Polgar, G., Ghanbarifardi, M., Milli, S., Agorreta, A., Aliabadian, M., Esmaeili, H. R., et al. (2017). Ecomorphological adaptation in three mudskippers (Teleostei: Gobioidei: Gobiidae) from the Persian Gulf and the Gulf of Oman. Hydrobiologia 795, 91–111. doi: 10.1007/s10750-017-3120-8
Polgar, G., Malavasi, S., Cipolato, G., Georgalas, V., Clack, J. A., and Torricelli, P. (2011). Acoustic communication at the water’s edge: evolutionary insights from a mudskipper. PLoS One 6:e21434. doi: 10.1371/journal.pone.0021434
R Core Team. (2019). R: A language and environment for statistical computing. R Foundation for Statistical Computing, Vienna, Austria.
Revell, L. J. (2012). Phytools: an R package for phylogenetic comparative biology (and other things). Methods Ecol. Evol. 3, 217–223. doi: 10.1111/j.2041-210X.2011.00169.x
Sackton, T. B., Grayson, P., Cloutier, A., Hu, Z., Liu, J. S., Wheeler, N. E., et al. (2019). Convergent regulatory evolution and loss of flight in paleognathous birds. Science 364, 74–78. doi: 10.1126/science.aat7244
Sayer, M. D. (2005). Adaptations of amphibious fish for surviving life out of water. Fisheries 6, 186–211. doi: 10.1111/j.1467-2979.2005.00193.x
Sayer, M. D. J., and Blackstock, N. (1988). The relationship between nitrogen output and changes in mucous cell function in the amphibious teleost Blennius pholis L. during aerial exposure. J. Fish Biol. 32, 765–776. doi: 10.1111/j.1095-8649.1988.tb05415.x
Shubin, N. H., Daeschler, E. B., and Jenkins, F. A. (2006). The pectoral fin of Tiktaalik roseae and the origin of the tetrapod limb. Nature 440, 764–771. doi: 10.1038/nature04637
Steppan, S. J., Meyer, A. A., Barrow, L. N., Alhajeri, B. H., Al-Zaidan, A. S. Y., Gignac, P. M., et al. (2022). Phylogenetics and the evolution of terrestriality in mudskippers (Gobiidae: Oxudercinae). Mol. Phylogenet. Evol. 169:107416. doi: 10.1016/j.ympev.2022.107416
Symonds, M. R., and Blomberg, S. P. (2014). “A primer on phylogeneteneralizedsed least squares,” in Modern phylogenetic comparative methods and their application in evolutionary biology. eds. L. Z. Garamszegi (Berlin, Heidelberg: Springer), 105–130.
Takeda, T., Ishimatsu, A., Oikawa, S., Kanda, T., Hishida, Y., and Khoo, K. H. (1999). Mudskipper Periophthalmodon schlosseri can repay oxygen debts in air but not in water. J. Exp. Zool. 284, 265–270. doi: 10.1002/(SICI)1097-010X(19990801)284:3<265::AID-JEZ3>3.0.CO;2-X
Tamura, S. O., Morii, H., and Yuzuriha, M. (1976). Respiration of the amphibious fishes Periophthalmus cantonensis and Boleophthalmus chinensis in water and on land. J. Exp. Biol. 65, 97–107. doi: 10.1242/jeb.65.1.97
Tomiyama, I. (1934). Four new species of gobies from Japan. J. Fac. Sci. Tokyo. Imp. Univ. 3, 325–334.
Tran, L. X., Soyano, K., and Ishimatsu, A. (2022). Morphology of the feeding apparatus in two oxudercine gobies, Parapocryptes serperaster (Richardson 1846) and Pseudapocryptes elongatus (Cuvier 1816). Zoomorphology 141, 183–196. doi: 10.1007/s00435-022-00554-8
Yang, Y., Yoo, J. Y., Baek, S. H., Song, H. Y., Jo, S., Jung, S. H., et al. (2022). Chromosome-level genome assembly of the shuttles hoppfish, Periophthalmus modestus. Gigascience 11:089. doi: 10.1093/gigascience/giab089
Yokoya, S., and Tamura, O. S. (1992). Fine structure of the skin of the amphibious fishes, Boleophthalmus pectinirostris and Periophthalmus cantonensis, with special reference to the location of blood vessels. J. Morphol. 214, 287–297. doi: 10.1002/jmor.1052140305
You, X., Bian, C., Zan, Q., Xu, X., Liu, X., Chen, J., et al. (2014). Mudskipper genomes provide insights into the terrestrial adaptation of amphibious fishes. Nat. Commun. 5, 1–8. doi: 10.1038/ncomms6594
Zaccone, G., Lauriano, E. R., Capillo, G., and Kuciel, M. (2018). Air-breathing in fish: air-breathing organs and control of respiration: nerves and neurotransmitters in the air-breathing organs and the skin. Acta Histochem. 120, 630–641. doi: 10.1016/j.acthis.2018.08.009
Zhang, J. (2001). A comparative study on the morphological adaptations in Oxudercine gobies to their terrestrial life (Doctoral dissertation, 長崎大学 Nagasaki University).
Zhang, J., Taniguchi, T., Takita, T., and Ali, A. B. (2000). On the epidermal structure of boleophthalmus and scartelaos mudskippers with reference to their adaptation to terrestrial life. Ichthyol. Res. 47, 359–366. doi: 10.1007/BF02674263
Keywords: amphibious, fish out of water, goby, histology, Pagel’s lambda, phylogenetic comparative methods, trait evolution, water-to-land transition
Citation: Corush JB and Zhang J (2022) One size does not fit all: Variation in anatomical traits associated with emersion behavior in mudskippers (Gobiidae: Oxudercinae). Front. Ecol. Evol. 10:967067. doi: 10.3389/fevo.2022.967067
Edited by:
Paolo Franchini, Sapienza University of Rome, ItalyReviewed by:
Quang Dinh, Can Tho University, VietnamPeter Hundt, University of Minnesota Twin Cities, United States
Christine Thacker, Natural History Museum of Los Angeles County, United States
Copyright © 2022 Corush and Zhang. This is an open-access article distributed under the terms of the Creative Commons Attribution License (CC BY). The use, distribution or reproduction in other forums is permitted, provided the original author(s) and the copyright owner(s) are credited and that the original publication in this journal is cited, in accordance with accepted academic practice. No use, distribution or reproduction is permitted which does not comply with these terms.
*Correspondence: Joel B. Corush, SmNvcnVzMkBpbGxpbm9pcy5lZHU=; Jie Zhang, emhhbmdqaWVAaW96LmFjLmNu