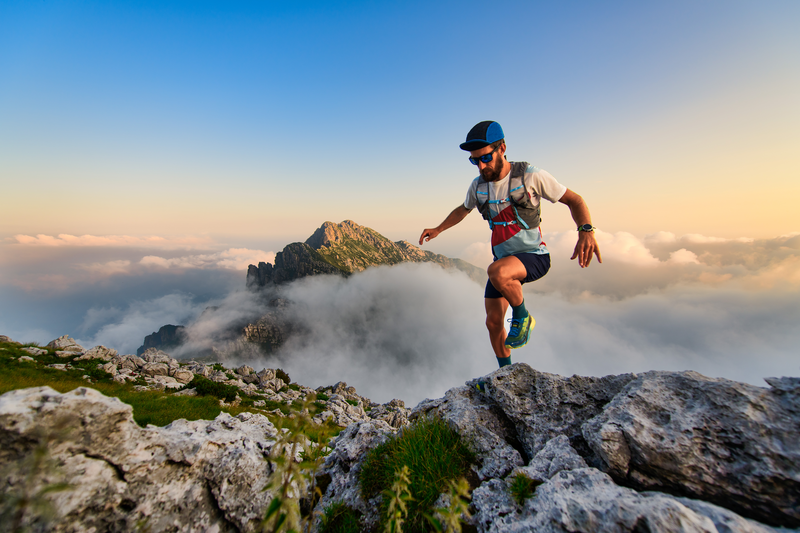
95% of researchers rate our articles as excellent or good
Learn more about the work of our research integrity team to safeguard the quality of each article we publish.
Find out more
ORIGINAL RESEARCH article
Front. Ecol. Evol. , 27 July 2022
Sec. Behavioral and Evolutionary Ecology
Volume 10 - 2022 | https://doi.org/10.3389/fevo.2022.948324
This article is part of the Research Topic Social Functions of Bat Vocalizations View all 15 articles
Bat populations employ rich vocal repertoires for social communication in addition to emitting sound pulses for echolocation. Acoustic parameters of echolocation pulses can vary with the context in which they are emitted, and also with the individual and across populations as a whole. The acoustic parameters of social vocalizations, or “calls”, also vary with the individual and context, but not much is known about their variation across populations at different geographic locations. Here, we leveraged the detailed acoustic classification of social vocalizations available for the Great Himalayan leaf-nosed bat, Hipposideros armiger, to examine geographic variation in five commonly emitted simple syllable types. We hypothesized that individuals within geographically dispersed populations communicate using spectrographically similar constructs or “syllable types”. We also examined whether call syllables vary discordantly with the correlation pattern observed for echolocation pulses across those same geographic regions. Furthermore, we postulated that the acoustic boundaries of a syllable type are not uniquely constrained to its variation within a particular population of the same subspecies. To test our hypotheses, we obtained recordings of social calls of H. a. armiger from nine locations within the oriental region. These locations were consolidated into five geographic regions based on previously established region-specific differences in the peak frequency of echolocation pulses. A multivariate cluster analysis established that unlike echolocation pulses, syllable types exhibit a relatively large variance. Analysis of this variance showed significant differences in Least Squares Means estimates, establishing significant population-level differences in the multiparametric means of individual syllable types across geographic regions. Multivariate discriminant analysis confirmed the presence of region-specific centroids for different syllable constructs, but also showed a large overlap of their multiparametric boundaries across geographic regions. We propose that despite differences in the population-specific core construct of a syllable type, bats maximize acoustic variation across individuals within a population irrespective of its overflow and overlap with other populations.
Morphometric variation is a key substrate for natural selection (Darwin, 1859). Similarly, acoustic variation for audiovocal social communication has also been useful for determining whether a population represents a subspecies or an acoustically isolated, separate species (Wright, 1996; MacDougall-Shackleton and MacDougall-Shackleton, 2001; Hoskin et al., 2005; Puechmaille et al., 2011; Toews, 2017; Liu et al., 2019; Garcia et al., 2020). Non-verbal aspects of variations in speech sounds in humans, identified as dialects, are an example of population-level differences, resulting from the rapid and geographically diverse diaspora of humankind (Lee and Hasegawa, 2014; Hua et al., 2019). In songbirds, the ecological and evolutionary advantages of the presence of dialects and variations in song production across different populations have been elucidated in a few species (Krebs and Kroodsma, 1980; Naguib et al., 2009), but rarely quantified within a statistical framework. In gray mouse lemurs, a nocturnal primate species, advertisement calls diverge but also overlap among sympatric and allopatric species (Braune et al., 2008).
Studies of systematic variation in acoustic characteristics of human-imperceptible, ultrasonic communication sounds, such as those emitted by whales, dolphins and bats, have lagged behind the description of human-perceptible vocalization of non-human species. It can be challenging to both record and quantify social vocalizations in bat species given the many call types and their relatively infrequent emission within a colony of hundreds to thousands of bats roosting in locations that are difficult to access. Advances in high bandwidth sampling and mobile technologies and the computational power affording ease of analyses within a multivariate statistical framework have only recently opened the possibility of examining ecological and evolutionary mechanisms at play in bat species vocalizing in the ultrasonic range (Bradbury and Vehrencamp, 2011). We are, therefore, now in a position to examine the presence of geographic variation in acoustic traits for ultrasonic social vocal communication.
Among mammals, many bat species show a wide geographic distribution under varying climatic and physical conditions owing to flight (Fleming and Eby, 2003; Luo et al., 2019). Their wide-bandwidth vocalizations extend to the ultrasonic range and are used for echolocation as well as social communication. Bats continuously emit echolocation pulses during foraging and in other contexts so as to visualize their environment. A vast number of studies in many bat species demonstrate acoustic diversity and variation in the echolocation pulses (produced for navigation and foraging) at the individual level (Kazial et al., 2001; Hiryu et al., 2006; Luo et al., 2012; Matthew et al., 2014; Wu et al., 2021), and most recently at the population/geographic levels (Gillam and McCracken, 2007; Yoshino et al., 2008; Jiang et al., 2010; Lin et al., 2015a; Zhang et al., 2018; López-Bosch et al., 2021; Rossoni et al., 2021). Sounds emitted for social communication also vary with the individual, the specific audience or context, the physiological/emotional state of the emitter and the time of day in which they are emitted (Behr and von Helversen, 2004; Bohn et al., 2008, 2009). Acoustic characteristics of social vocalizations may also correlate with phenotypic variation across geographically isolated populations. Only in the last few years, the communication sounds made by a few different species of bats have been recorded, identified and systematically characterized from an acoustics standpoint (Kanwal et al., 1994; Davidson and Wilkinson, 2002; Behr and von Helversen, 2004; Ma et al., 2006; Melendez et al., 2006; Bohn et al., 2008; Gadziola et al., 2012; Lin et al., 2015b), making it now possible to examine their geographic variation.
The Great Himalayan leaf-nosed bat, Hipposideros armiger (Chiroptera: Hipposideridae) emits echolocation pulses that are characterized by a constant frequency (CF) component followed by a downward frequency modulation (FM) component. This species is widely distributed in South China (IUCN, 2014), where they are characterized by a high degree of environmental heterogeneity. From a morphological characterization of 16 external measurements, including forearm length, 25 skull measurements and the sequence analysis of two mitochondrial genes (cytochrome-b and D-loop), it has been established that H. armiger is represented in China by only one subspecies, H. a. armiger (Bu et al., 2017). Substantial gene flow is present across populations from different geographic regions (Lin et al., 2014). Our previous study demonstrated that the structural diversity of communicative vocalizations in H. armiger rival those of the most vocal bat species (Lin et al., 2016). Its well-organized syllabic repertoire consists of 35 distinct “syllable types” with 18 being classified as simple syllables and 17 as composites. Simple syllables contained 4 CF syllables, 13 FM syllables, and 1 noise burst type syllable. H. armiger usually strings together either the same (as in a syllable train) or different syllable types when vocalizing for social communication. The syntactic rules for these combinations have not been systematically studied. From a purely subspecies standpoint, no statistically significant geographic differences in multiparametric acoustic boundaries are expected for any syllable type.
Here we leverage the spectrographic diversity of social vocalizations in H. armiger and the quantitative data available on the statistically verifiable acoustic classifiers of syllable types (Lin et al., 2016) to test the hypothesis that individuals within and across geographically isolated populations communicate using the same syllable set. Toward this end, we recorded daytime social vocalizations and retrieved similar data on echolocation vocalizations within each population. We were interested in knowing whether communication and echolocation vocalizations vary discordantly across populations. More importantly, we wanted to test (1) that individuals within geographically separate regions communicate using acoustically similar constructs or syllable types, and (2) the acoustic variation in each syllable type is universal across populations, i.e., the multiparametric acoustic centroids and upper bounds of a particular syllable type are not uniquely constrained within a parent population (null hypothesis). Alternately, the geographic variation of acoustic parameters within a syllable type may be regionally constrained and originate from an acoustic structure that is unique to each population. Given the constraints elaborated above, this is the first systematic and in-depth analysis of geographic variation in multiple syllable types in a bat subspecies. The results of this study pave the way for a deeper understanding of the ecologic, genetic, morphologic, and physiologic factors influencing acoustic variation for audiovocal communication in mammals, including humans.
This study builds on the data on echolocation vocalizations within the same populations in H. a. armiger (Lin et al., 2015a). Briefly, bats from each population were collected and taken into a temporary laboratory (9 m long × 9 m wide × 5 m high) set up near the roost. A total of 139 individuals from nine localities were collected for sound recording, with 19, 20, 11, 15, 13, 9, 19, 23, and 10 for Anlong (AL), Beichuan (BC), Chongyi (CY), Fanchang (FC), Guilin (GL), Lingshui (LS), Hanzhong (HZ), Jiangkou (JK), and Simao (SM), respectively. Resting echolocation pulses were recorded for all individuals, with the microphone located at ∼2 m from the bat. Pulses were recorded using the UltraSoundGate 116, with a sample rate of 375 kHz at 16 bits/sample.
During March to June 2011, vocalizations of H. armiger were recorded from nine localities in China, i.e., AL, BC, CY, FC, GL, LS, HZ, JK, and SM (Figure 1A inset), from where echolocation vocalizations of this species have been reported by Lin et al. (2015a). We conducted sound recordings in caves during 16:30–19:50 h when the bats were the most active prior to their nightly emergence from caves. Vocalizations were recorded using an ultrasound recording system (UltraSoundGate 116, Avisoft Bioacoustics, Berlin, Germany), with a sample rate of 375 kHz at 16 bits/sample. A microphone was positioned with a distance 3–7 m far from bat colonies, and the microphone position was changed every day to minimize the possibility of obtaining recordings from the same group of bats each time. Vocalizations were recorded every 2–3 days, to achieve a total of 7–10 days of recordings for each locality. This study followed ASM guidelines and was approved by National Animal Research Authority in Northeast Normal University, China.
Figure 1. (A) Spectrographic patterns plotted to scale and labeled by name and marker (in parenthesis as used in B) for each syllable. (B) Scatterplot of the first two canonical variables capturing the multiparametric variation for each of the five syllable types recorded for all locations within the South China region. The discriminant analysis tested for misclassification of observations for the syllable types. The point corresponding to each multivariate mean is denoted by a plus (“+”) marker. Bold lines denote the 95% confidence ellipses and dashed lines indicate boundaries containing ∼50% of the observations. Inset: Map of geographic locations of different habitats from where sound recordings were obtained from different bat populations. (C) A scatterplot of the first two principal components from a K-means cluster analysis of acoustic parameters in the five syllable types and echolocation pulses. A best fit was obtained for the four color-coded clusters shown (numbers in parentheses in the key indicate syllable count in each cluster). Numbers as marker labels represent different syllable types illustrated by their spectrograms. Cluster 2 (green) was made up entirely of echolocation pulses, whereas clusters 1, 3, and 4 represent the five syllable types within social vocalizations. The scatterplots indicate the larger variation captured by each syllable cluster compared to the pulses and also the relatively large distance in multivariate acoustic space between acoustic characteristics of pulses vs. syllables. Centroids for each cluster are indicated by bold ellipses and dashed lines represent boundaries of 50% confidence ellipses. Numbers in parenthesis in the legend indicate the number of observations placed within each cluster. Numbers as marker labels correspond to each syllable type (“1” to “5” and “14” for echolocation pulse). A spectrographic plot of the echolocation pulse is also included.
A syllable is defined as a discrete part of a call which is surrounded by periods of silence (Kanwal et al., 1994). It is the smallest unit of a bat communication call. A simple syllable consists of a single predominant sound element, and a composite syllable is made up of two or more types of distinct components. The classification of simple syllabic calls followed the scheme introduced by Kanwal et al. (1994) that was based on quantitative and geometric descriptions of the sound spectrograms. We previously selected the vocalizations of the population in AL for syllable identification and classification (Lin et al., 2016). We selected the same syllable type from a daily dataset of vocalizations with recordings obtained as far apart in time as possible. We selected only one high-quality (signal-to-noise ratio > 40 dB) example from a call sequence. This minimized the possibility of pseudo-replication given that each vocal sample could not be unambiguously matched to an individual. Each syllable analyzed was initially normalized to amplitudes of 0.75v. We determined the duration, peak frequency (fpeak), minimum frequency (fmin), maximum frequency (fmax), and bandwidth, and calculated center frequency (fcent = fmax-bandwidth/2) of the first harmonic of each syllable using Avisoft SASLab pro software (Lin et al., 2016). Frequency parameters were measured from spectrograms using a sample rate of 250 kHz with a 1,024 pt. FFT (Hamming window, 93.75% overlap), resulting in a frequency resolution of 244 Hz, and duration was measured from the oscillogram.
Earlier, echolocation pulse parameters were characterized for local populations. Mean peak frequencies of echolocation pulses with a maximum variation of about 6 kHz (66.80–72.51 kHz), showed significant differences across populations, clustering into three distinct groups: Eastern and Western China, Hainan (HN), and Southern Yunnan (SY). This geographic distinction across populations was shown to result from the action of both indirect ecological selection and cultural drift (Lin et al., 2015a). Some populations of H. armiger were grouped together, however, within wider geographic regions based purely on either maternally inherited markers (HN and EC populations) or on bi-parentally inherited markers (HN and WC populations; Lin et al., 2014).
Using location proximity from a multidimensional scaling (MDS) analysis of the echolocation pulse data (variation in peak frequency values) available from the 17 original locations; Lin et al., 2015a), we grouped the nine populations relevant for this study into five non-overlapping regions/zones represented on the first dimension of the MDS plot (see Figure 2 in Lin et al., 2015a). These regions include Northeastern China (FC), Central South China (SC; AL and JK populations in the West and those of GL and CY in the East), Northern China (NC; BC and HZ populations), Southwestern Yunnan (SM), and Hainan-island (LS). Syllable types that were the most frequently observed and shared among these five regions were selected for geographic variation analysis.
The variation of the syllables derives from three levels, i.e., the region level, population (or location) level, and individual level (irrespective of region or location). Multivariate K-means cluster analysis was used to compare the variation between syllable types within each region and between syllables and pulses and the multivariate distances within syllable types and between syllables and echolocation pulses for each region. To determine whether the nine populations differed significantly in the basic construction of syllable types, we first performed cluster analysis followed by independent principal component analysis (PCA) with the eight spectro-temporal parameters (fpeak, fmin, fmax, fcent, duration, bandwidth, and FM rate and direction), and used the obtained PCA scores to perform discriminant function analysis (DFA) for each syllable type.
We also calculated acoustic Euclidean distance between population mean values of each acoustic parameter for each syllable type using JMP Pro software (v. 16.2; SAS Institute Inc., United States) to test geographic effects for syllable types, following a comparison of means for individual parameters (fpeak and duration) across the five geographic locations. These parameters showed high factor loadings for the first two factors in acoustic factor analysis of the five simple syllable types. Since General Linear Model analysis for fpeak in echolocation pulses revealed a significant effect for geographic location but not for sex (Lin et al., 2015a), we used the same parameter (fpeak) together with syllable duration (from Factor analysis) for performing analysis of variance (ANOVA) using Least Squares Fit to test for an effect of geographic region for each of five simple syllabic calls for which we had data from populations at all nine locations.
To compare the extent of geographic variation in communication vs. echolocation vocalizations, we calculated and statistically compared the overall coefficients of variation (CV; irrespective of localities) and percentages of variation attributed to geographic differences of each acoustic parameter of these two kinds of sounds. Significance of the differences was evaluated based on One Sample T-tests. Multivariate ANOVA (using Least Squares Means), based on the fpeak and duration parameters, was used to test if syllable type distribution patterns were discordant from those of echolocation pulses and their correlation with geographic distances and body size (forearm length measures) across the five geographic regions using Mantel tests performed in PASSaGE v 2 (Rosenberg and Anderson, 2011). To test whether communication and echolocation vocalizations would vary in discordance geographically, we used Mantel tests to test the correlations between matrices of acoustic Euclidean distance between populations of each syllable type and of echolocation pulses. Moreover, we used simple linear regression models to test the correlations between acoustic parameters of echolocation pulses and those of common syllable types. Multiple Correspondence (XLSTAT, Addinsoft, Inc.) was used to produce a simplified (low- dimensional) representation of the acoustic information in a Burt table. MDS (JMP Pro v. 16.2; SAS Institute Inc., United States) analysis was performed to compute acoustic distances between geographic locations and show their proximity to each other as well as how they parse across larger regions.
As reported earlier, H. armiger exhibits a diversity of syllable types within their social vocalizations. The frequency band carrying the predominant energy in the five FM commonly emitted syllable types, i.e., upward chevron FM (uCFM), upward paraboloid FM (uPFM), bent upward FM (bUFM), linear upward FM (lUFM), and plateaued paraboloid FM (pPFM), used for analysis of geographic variation was typically in the second or third harmonic with peak frequencies > 50 kHz. The basic forms of the spectrographic patterns are shown in Figure 1A (for quantitative details, see Lin et al., 2016). For this study, a total of 2,768 examples of the five syllable types were recorded with the number of samples for each syllable type analyzed ranging from 224 to 417 syllables. Altogether, these syllables represented more than 80% of the vocalizations recorded, and were commonly present within the nine populations. Acoustic parameters for each syllable type exhibited remarkable variation. We investigated the classification accuracy of the five syllable types at each of the five geographic regions. In each case, discriminant analysis showed a perfect to near-perfect fit for each syllable type (misclassification < 0.5%) when mapping the variance based upon the six measured syllable parameters as well as FM rate [computed from (fmax-fmin)/FM duration for the predominant direction of FM and its typical duration] and categorically encoded FM direction (Figure 1B).
For call syllables, the bandwidth parameter showed the highest overall variation across all locations with a mean coefficient of variation of 27.20%, ranging from 17.36 to 37.15% (see Supplementary Table 1). CV for fpeak (21.18%) and fmin (21.54%) were also relatively high. The fmax parameter (together with fcent) showed the lowest overall variation, with the mean CV of 14.21%, ranging from 5.72 to 18.14%. Overall, all six directly measured acoustic parameters (FM rate and direction excluded) in these syllables exhibited a greater variation than in echolocation pulses. One Sample T-tests indicated that the overall CVs of each acoustic parameter were significantly greater in syllable types than in echolocation pulses (all P ≤ 0.01), indicating greater variability and lower regional parsing of geographic variation in social vocalizations.
To get a general sense of how the variation in vocalizations is distributed across all acoustic parameters for syllable types vs. echolocation pulses, we performed K-means cluster analysis using data on the seven acoustic parameters for both echolocation and social vocalizations. We obtained a best fit for 4 clusters, which clearly separated echolocation vocalizations from those of calls within a biplot of the first two principal components (Figure 1C). The other three clusters were in closer proximity to each other (with clusters 1 and 3 showing a minor overlap) compared to the echolocation cluster and exhibited a much larger parametric variation. Cluster 1 clearly separated the uCFM syllable from other syllable types. Cluster 4 included all examples of the simple syllabic, uPFM syllable that was routinely emitted as a train of syllables, and also included examples of the structurally similar pPFM syllable type. This analysis allowed us to establish that simple syllables within social vocalizations form their own parametric acoustic boundaries and therefore might not follow geographic variation patterns established for echolocation sounds (Lin et al., 2015a).
Factor analysis revealed the underlying acoustic structure in calls by extracting parametric combinations that represent the common variability across observed variables. Across all syllables tested, maximum likelihood estimates indicated that the first factor captured nearly 43% of the variation ranging from a high of 68.9% for bUFM and a low of 33.0% for both lUFM and uCFM. The average variation explained by the second factor was approximately 26.6% with a max of 32.3% for uPFM and a low of 16.3% in case of bUFM. Overall, fmax and fcent had the highest factor loadings and FM rate had the lowest. It should be noted that the contribution of the acoustic parameters to the first two factors varied with the syllable type and also the relative factor loadings of each parameter for the same syllable type across the five geographic regions. The first factor was typically made up of frequency parameters (fcent, fmax, fmin, and fpeak) and the second (orthogonal) factor included syllable duration and either FM rate or bandwidth for most syllables though duration also contributed predominantly to a third factor, which across all syllables explained 14.4% of the variation. Since fpeak exhibited a relatively high variance in syllable types and was also tested for geographic variation in echolocation pulses, we first focused on the geographic variation for fpeak and duration parameters across the geographic regions sampled.
Given the various ways in which acoustic variation may be parsed across recorded locations, we used a non-parametric statistical approach to first test whether call syllables followed a concordant pattern of geographic variation across the same populations targeted for echolocation sounds. Figure 2 shows box plots for comparing the means for fpeak and duration for each of the five syllable types across the five geographic regions. Our results for fpeak across these geographic locations revealed a significant effect [F4 = 21.28, 4.77, 4.53, and 8.29 for bUFM, lUFM, uCFM, and uPFM syllable types, respectively (p < 0.001), but an insignificant one for pPFM (F4 = 0.903, P = 0.462)]. However, pPFM showed a significant effect (F4 = 9.725, P < 0.001) for the duration parameter across the same geographic locations. In fact, duration revealed a significant effect (P < 0.001) across all locations for this syllable type. A comparison of means of fpeak across all pairs revealed significant separation between SC, FC, and SM regions for bUFM, between SC, and NC for lUFM, between FC and SC regions for uCFM, and between NC, LS, and SC regions for the uPFM syllable type, and no separation between regions for pPFM (see Table 1). Similarly, a comparison of means of the duration parameter revealed significant differences between LS, SC, NC, and SM regions for bUFM, between FC and NC regions for lUFM, only the FC region for pPFM, between LS and SC regions for uCFM, and between LS and FC regions for uPFM syllable type. All comparisons were tested for all pairs using the non-parametric Tukey-Kramer HSD (P < 0.001). These results demonstrated that the acoustic variance in syllable types within at least some regions was largely constrained by the geographic boundaries of that region.
Figure 2. Side-by-side box and whisker plots showing the mean and standard deviation of fpeak and duration parameters in five syllable types tested for analysis of geographic variation in this study. Numbered markers indicate parameter observations for syllable types and colors indicate geographic regions from where they were recorded. FC and SM regions in the far northeast and far southwest, respectively, show the maximum divergence from the grand mean (gray line) of the medians (green lines) for nearly all syllables, suggesting that geographic distance can increase difference between means. A systematic pattern of difference between means proportional to geographic distance, however, is absent. The width of the box plots is based upon the number of data points at each location. See Table 1 for statistical significance of differences between geographic regions.
Table 1. A comparison of the means of fpeak and duration in syllable types across all pairs showing statistical significance (P < 0.001) of differences, indicated by asterisks, between geographic regions.
Fpeak in the echolocation pulse varied with morphological features, particularly body size characterized by forearm length (Lin et al., 2015a). Therefore, we also tested each of the simple syllabic calls to examine any correlation with forearm length. We computed the first principal component as a composite index for fpeak and duration parameters together because they capture the maximum independent sources of acoustic variation in all vocalizations. Moreover, both of these parameters require more energy and ventilatory volume to produce a vocalization and therefore may correlate with body size that is known to vary across geographic populations. For the same geographic regions, the echolocation pulse showed a significant (P < 0.05) correlation with forearm length and 60% of the variation was explained by forearm length. None of the syllable types, however, showed a significant (P > 0.05) correlation with forearm length. The bUFM, pPFM, and uPFM syllable types showed a trend of change in the same direction as the pulse, but uCFM and lUFM calls exhibited a trend to change in the opposite direction with increase in forearm length, as indicated by the regression plot in Figure 3A. Also, only 15% to 33% of the variation in syllables was accounted for with forearm length as the regressor. None of the variation was explained for the lUFM syllable. Mantel tests also revealed that the matrix of acoustic distance for syllables and that for body size between populations were not significantly correlated (Figure 3B). These analyses demonstrated that geographic variation in syllabic parameters was discordant with the pattern of parsing variation in acoustic parameters for echolocation pulses when tested across the same set of geographic regions. Correlation in syllabic variation was also not significant (P > 0.05) for either acoustic vs. geographic or acoustic vs. genetic distances.
Figure 3. (A) Scatterplot of data points and regression line plots for model fit with body size (forearm length), incorporating all acoustic parameters measured for each syllable type at different geographic locations. (B) Scatterplot for Mantel test of correspondence between the matrix of acoustic distances for echolocation pulses and that of multiparametric acoustic distances for all syllable types. No significant correspondence was observed between the two acoustic distance matrices.
For non-parametric tests of geographic divergence and visualization of geographic variance in each syllable type, we performed Discriminant analysis (using Mahalonobis distances) based on all six acoustic parameters. Here the geographic location of a syllable cluster is represented by the mean of all emissions at that location as well as from the group mean that they are actually closest to. This gives an estimate of how well a syllable type matched its geographic identity. When testing for syllabic classification (cluster boundaries for individual syllable types) discriminant analysis revealed a near-perfect match with <0.05% of the syllables showing a misclassification at any one or when tested for all geographic locations combined (Wilks’ lambda < 0.0001).
Discriminant analysis (quadratic method with different co-variances) performed for the five aforementioned regions revealed that the centroids of echolocation pulses were clearly segregated across regions (Wilks’ lambda < 0.0001). However, partially overlapping representation of the centroids of acoustic parameters across the five locations for the simple syllabic types showed a high level of misclassification across the same aggregated locations (Figure 4). Percent misclassification for echolocation pulses was 23.74%, whereas that for calls ranged from 34.69% (for uPFM) to 68.97% (for bUFM). Compared to a –2LogLikelihood estimate of 188.024 for echolocation pulses, that for each syllable type was 3668.1 for bUFM, 110.19 for lUFM, 1117.4 for pPFM, 19210.6 for uCFM and 556.2 for uPFM. ROC curves indicated the poorest sensitivity for uCFM, with area under the curve averaging <0.5 for different locations despite a significant difference between the centroids or multiparametric means (Wilks’ lambda < 0.0001; F = 4.83, P < 0.0001 for first eigenvalue capturing 77.75% of the variance) for different geographic locations. ROC curves for other syllable types showed a high level of sensitivity and specificity with respect to geographic location.
Figure 4. Scatterplot of the first two canonical variables capturing the multiparametric variation for echolocation pulses and each of the five syllable types recorded for all geographic regions. The discriminant analysis tested for misclassification of observations for each geographic region. The point corresponding to each multivariate mean is denoted by a plus (“+”) marker. Bold lines denote the 95% confidence ellipses and dashed lines indicate boundaries containing ∼ 50% of the observations. Echolocation pulses and three syllables show good separation between FC (red ellipses) and SM regions (bluish-green ellipses).
Although Multiple Correspondence analysis is typically used for dimensional reduction of categorical variables, here we used an algorithm for ranking continuous data (JMP Pro software, v. 16.2; SAS Institute Inc., United States), which revealed a two-dimensional plot showing the proximity of different geographic regions within acoustic space and how the acoustic parameters contributed to the inertia keeping populations’ relative placement in close proximity (Figure 5). Thus, FC and LS were placed in the same quadrant with fpeak, and FM rate contributing to the inertia, whereas SC and SM, placed close together, were distanced largely by frequency parameters (fmax and fcent), and NC and FC regions were placed far apart because of fpeak and bandwidth. Bandwidth contribution varied largely along the first dimension, whereas the duration parameter contributed to acoustic separation of geographic regions along both dimensions. A large part of the inertia (64.7%) was captured by the first dimension; the second dimension captured 18.6% of the inertia and the third dimension (not shown) captured another 10.7%. Among geographic regions, LS and NC contributed the most to the inertia for the first dimension, FC contributed the most to the second dimension and SM contributed the most to the third dimension. Statistical tests using likelihood ratios and Pearson’s correlation coefficient revealed a high level of independence (P < 0.0001).
Figure 5. A symmetric, two-dimensional multiple correspondence plot mixing observations (parametric values) with variables (geographic regions) to explain the acoustic basis of regional proximity. The five geographic regions are well separated into four quadrants of the plot contingent upon the values of the acoustic parameters placed in the same quadrant. Proximity of parameters and geographic locations are governed by the inertia determined by the commonality of the values or rankings for each level of a variable. Proximity of acoustic variables is highlighted gray ellipses enclosing red dots with corresponding parameters labeled. Negative values of a parameter indicate lower than expected proportion of contribution of that parameter.
Finally, we performed MDS to estimate the acoustic dissimilarity among all original geographic locations of populations from which calls were recorded. The final configuration provided a good fit (R2 = 0.999) with the data (Figure 6). Most populations showed a clear spatial separation, though groupings obtained from MDS of echolocation pulses (encircled with dashed lines; Lin et al., 2015a) did not conform well with those obtained for calls. In terms of acoustic distances, our data suggested potentially five geographic clusters (gray ovals), somewhat different from those used to test main effect of location on population means based on the divergence for echolocation pulse in the previous study (Lin et al., 2015a) and tested here using ANOVA for individual parameters (fpeak and duration; see Figure 2) and composite parameters in the DFA for syllable types (see Figure 3). Based on acoustic parameters for syllable types, BC and HZ locations in the north and SM location in the southwest were all grouped surprisingly close to each other. GL and CY locations were relatively close as expected, but JK and FC locations were relatively closer than indicated by their geographic distance and acoustic distance estimated from fpeak in echolocation pulses. The AL location appeared relatively isolated acoustically even though geographically it is close to the JK and GL location and the LS location kept its distance as expected from H. armiger populations at other locations.
Figure 6. A MDS plot showing acoustic proximity of all nine geographic populations in two-dimensional space organized according to acoustic distance between syllable types estimated from all measured parameters. Boundaries indicated by dashed lines enclose the five geographic regions tested for independence (significant differences between means of multiple parameters). Gray ellipses indicate relatively close proximity visualized in two dimensions based on the correlations between the means of the seven continuous parameters representing each syllable type.
Communication and echolocation are acoustically, behaviorally and functionally distinct, requiring the production and auditory processing of complex sounds expressed as two distinct phenotypic-acoustic traits. The acoustic parameters of echolocation vocalizations have been extensively studied for characterizing variation across species and within a species during foraging behavior and other physiologic, climatic and geographic factors (Kazial et al., 2001; Hiryu et al., 2006; Gillam and McCracken, 2007; Yoshino et al., 2008; Jiang et al., 2010; Luo et al., 2012; Matthew et al., 2014; Lin et al., 2015a; Zhang et al., 2018; López-Bosch et al., 2021; Rossoni et al., 2021; Wu et al., 2021). This study provided a window into how the two types of vocalizations (communication vs. echolocation) co-vary or not with geographic region in the same subspecies. Our results can offer new insights into the plasticity of the vocal apparatus and the neural circuits controlling vocalizations and factors that influence acoustic divergence.
The patterns and causes of geographic variation in echolocation pulses have been uncovered for more than two dozen species of bats and depend on species-specific morphological and/or environmental characteristics (reviewed in Lameira et al., 2010; Jiang et al., 2015). Thus, empirical studies have shown remarkable geographic variation in echolocation pulses of many bat species, with peak frequency spread over a range of 5 to 10 kHz among individuals within a species, such as Hipposideros larvatus (84.5–91.8 kHz, Jiang et al., 2010), Tadarida brasiliensis (25.2–30.5 kHz, Gillam and McCracken, 2007), Craseonycteris thonglongyai (70.1–83.6 kHz, Puechmaille et al., 2011), and Rhinolophus cornutus pumilus (105.9–118.9 kHz, Yoshino et al., 2008). The results of the earlier study in Himalayan Leaf-nosed bats were consistent with these findings (Lin et al., 2015a).
The geographic variation in echolocation pulses of H. armiger resulted from population differences in body size, which resulted from adaptation to local ecological conditions (Lin et al., 2015a). The spectrotemporal structure of bat echolocation vocalizations are shaped both by evolutionary constraints on the vocal structure and by physiological mechanisms influencing sound structure. Especially in CF-bats, the frequencies of echolocation pulses are strictly constrained to maintain their echo within the acoustic fovea in the cochlea (Neuweiler, 1980; Rübsamen et al., 1988). Population divergence in morphological traits correlated with sound-producing structures can thus result in geographic variation in echolocation vocalizations, as observed in many bat species (reviewed in Lameira et al., 2010; Jiang et al., 2015). In H. armiger, variation in body size among and within populations can explain the variation of echolocation pulses among populations but not the observed pattern of acoustic proximity across geographic locations for syllable types.
Geographic variation in social vocalizations in bats has been reported only in a few species and limited to only one or two call types, i.e., Phyllostomus hastatus (Boughman and Wilkinson, 1998), Phyllostomus discolor (Esser and Schubert, 1998), Saccopteryx bilineata (Davidson and Wilkinson, 2002), and T. brasiliensis (Bohn et al., 2009). In the former three species, screech calls and maternal directive calls were significantly divergent. In T. brasiliensis, features of advertisement songs were similar across regions, i.e., exhibited almost no geographic variation, but varied within and among individuals. Our results closely follow these findings though only of the population as a whole since we were unable to examine within individual variation due to limitations of field recordings.
More broadly, geographic variation in calls has been documented in a wide array of taxa, spanning arthropod (e.g., Claridge et al., 1985; Lampe et al., 2014), amphibian (e.g., Pröhl et al., 2007; Jang et al., 2011; Velásquez et al., 2014), birds (e.g., Marler and Tamura, 1962; Irwin, 2000; Robin et al., 2011), and a few species of mammals (e.g., Mitani et al., 1999; Eiler and Banack, 2004; Amano et al., 2014), but rarely reported in bats though they have rich vocal repertoires for communication. The studies available on bats showed that only a few types of communication vocalizations were divergent across populations/groups (Boughman and Wilkinson, 1998; Esser and Schubert, 1998; Davidson and Wilkinson, 2002; Bohn et al., 2009; Sun et al., 2020). In this study, we found that the most frequent syllable types exhibited significant population divergence, suggesting that geographic variation could be commonly observed in the communication vocalizations of bats.
In the five syllable types tested in this study, inter-population multiparametric variation was greater, but not by far, than intrapopulation variation. Based on the multiparametric means, indicated by their centroids, syllables were significantly different across several populations. The multiparametric boundaries at the 50% confidence interval, however, were largely overlapping. Geographic variation can result from the action of various evolutionary forces such as ecological selection, sex selection, genetic drift, cultural drift, or a combination of these factors (Podos and Warren, 2007; Wilkins et al., 2013; Jiang et al., 2015). Significant variation in morphological traits constrained by geographic boundaries can result in reproductive isolation between diverging populations and eventually contribute to speciation (Gould and Johnston, 1972; Endler, 1977; Boughman, 2002). The trait of social vocalizations is of particular interest in this respect because sound characteristics are not only influenced by morphometric parameters of the vocalization apparatus of the emitter, but also depend upon the physical characteristics of the hearing apparatus of the receiver as well as attributes of the physical environment through which sound must be propagated before it reaches the receiver. These emitter-receiver characteristics are a hallmark of social audiovocal communication in any species.
Our results overall supported the hypothesis that communication vocalizations exhibit lower geographically classifiable divergence than echolocation vocalizations. The acoustic variation within calls in H. armiger was largely attributed to differences within rather than across the geographic regions tested. This is consistent with observations in Brazilian free-tailed bat, T. brasiliensis (Bohn et al., 2009), Thick-billed Parrot, Rhynchopsitta pachyrhyncha (Guerra et al., 2008), and Eastern Phoebes, Sayornis phoebe (Foote et al., 2013). One reason for why across-population variation accounts for a relatively small percentage of the total variation in calls in H. armiger may be the frequent dispersal among populations. Significant gene flow has been detected between populations from different regions in H. armiger (Lin et al., 2014). Mixing during dispersal could reduce vocal differences among populations, particularly if vocalizations play a significant role in mediating social behaviors (e.g., Ellers and Slabbekoorn, 2003; Wright et al., 2005; Guerra et al., 2008; Papale et al., 2014). Regardless, gene flow applies equally to both echolocation and social vocalizations and echolocation pulses do show divergence among different geographic regions. Another possibility is that convergence in the acoustic structure of social vocalizations among populations reduces errors in detection and/or interpretation by a migrant or visiting receiver, ensuring effective communication between individuals from different populations (Anholt and Mackay, 2009). Stability of signals is necessary for individual recognition and unambiguous communication, which may be important for species such as H. armiger with frequent dispersals across populations.
Traits with similar functions, commonly assumed to respond to similar selection pressures, may follow similar patterns of spatial variation, whereas those with distinct functions may exhibit discordant patterns. This happens because each functional trait is sensitive to a different set of selection pressures (Armbruster and Schwaegerle, 1996; Byers, 1996; Baker, 2011). In this study, we found that most syllable types varied discordantly with echolocation pulses across populations in H. armiger. The patterns of geographic variation in phenotypic traits are associated with the evolutionary forces driving trait divergence (Gould and Johnston, 1972; Endler, 1977; Podos and Warren, 2007; Wilkins et al., 2013; Jiang et al., 2015). A previous study found that population divergence in echolocation pulses of H. armiger resulted from morphological variation (due to ecological selection) and cultural drift (Lin et al., 2015a). Although little is known about the evolutionary forces driving population divergence in social calls of H. armiger, the results found here suggest that morphological variation and cultural drift may not be the primary causes contributing to geographic variation in most of the syllables. Further studies are necessary to determine the causes of geographic divergence in calls vs. echolocation vocalizations.
In songbirds, song and other territorial calls may develop or be modified during the mating season because of hormonal changes and a surge of growth of the song nucleus in the brain (DeVoogd and Nottebohm, 1981; Mooney and Prather, 2005). Echolocation pulses in bats are also known to vary with season. For example, weather conditions can affect the echolocation signals via their effects on body temperature and on atmospheric attenuation (Wiley and Richards, 1978; Wu et al., 2021). In addition to age and season, echolocation pulses are known to change non-seasonally, depending on audio-vocal feedback from conspecific echolocation pulses and contribute to short- or long-term intra-individual variation in the resting frequency (Hiryu et al., 2006). There is no evidence as yet of variations in social calls, particularly syllable types, with season though new calls may emerge during the mating season (Sun et al., 2021). Our recordings were obtained over a short interval of a few weeks from all geographic locations. Therefore, we could not address this potential source of acoustic variation in this study.
Echolocation sound pulses are produced by tracheal chambers and in some species via nasal passages (Suthers et al., 1988) that are anatomically less elaborate than the laryngeal and oropharyngeal structures responsible for shaping the final acoustic form of a social call (Kanwal et al., 1994; Bohn et al., 2008, 2009; Lin et al., 2015b). Therefore, body size may affect echolocation pulses differently than syllable types. This may partly explain why the vocal trajectories of echolocation and social vocalizations differ for geographical distribution despite a morphologic and partial anatomic overlap in their production apparatus (Liu et al., 2013).
Unlike the relatively homogenous bony and muscular tissues, the cellular organization and connectivity in the nervous system can change rapidly with experience at multiple time scales, from seconds to years (Ji and Suga, 2008; Jiang et al., 2019; Kanwal et al., 2021). Differences in the contribution (factor loadings) of individual parameters across geographic regions point to different trajectories for modifying syllable types within a location or region. This indicates plasticity in brain networks contributing to different vocal motor trajectories for the construction of a syllable type. These differences in vocal motor trajectories within syllable types across geographic regions and between syllable types and echolocation pulses are directly influenced by vocal control circuits within the frontal, limbic and brainstem vocal premotor networks and auditory feedback loops (Smotherman and Metzner, 2005; Fenzl and Schuller, 2007; Kanwal, 2021). Therefore, our findings on geographic variation reported here may provide new insights into the brain mechanisms for controlling variation in the production of call syllables vs. echolocation pulses in bats.
In summary, our data show that all syllable types varied discordantly with echolocation pulses across geographically separated populations of Great Himalayan leaf-nosed bats. Our data suggest that the acoustic boundaries defining a geographically isolated population of H. a. armiger are largely parsimonious such that the acoustic variation of most simple syllable types is maximized within each population, reducing heterogeneity between populations. The core construct of simple syllable types within their social calls is significantly different within at least half of all possible regional comparisons. This may happen because the multiparametric nucleus of a syllable is learned from either a parental (Esser, 1994; Esser and Schmidt, 2010) or a social group template (Prat et al., 2017). As in songbirds, this template may gradually be modified with the growth of each individual, depending on morphological, hormonal and other factors and thus contribute to individual identity via group-distinctive calls (Whaling et al., 1995; Boughman and Wilkinson, 1998; Kanwal, 2021). This divergence, however, can cause an overflow of the upper bounds of acoustic variance to another population and geographic region despite population-specific differences in body size and climatic conditions. This is not surprising given that the nature of social interactions is the same across populations. Both migration and conspecific feedback may also contribute to overlap and convergence of acoustic variance across neighboring populations (Boughman, 1998).
This study revealed the presence of diffuse boundaries across large geographic regions and relatively isolated populations for acoustic parameters defining simple syllable types that are key components of social calls in the Great Himalayan leaf-nosed bats, H. a. armiger. Our results also showed that acoustic variation in simple syllable types develops discordantly from that observed for echolocation pulses. In general, our results provide insights into vocal plasticity and its neural control at the individual, population and evolutionary levels in mammalian species. We conclude that together with behavioral interactions within and across geographically distributed populations, morphology of the vocal apparatus and developmental changes in it as well as vocal learning can play a role in the dynamics of variation in the acoustic constructs for social communication, which is critical for survival and reproduction. The potential contribution of multiple factors governing natural variation in the construction of social calls stresses the importance of maintaining the delicate balance between ecological, morphological, neurohormonal and behavioral factors, including an animal’s internal state (Kanwal, 2021; Kanwal et al., 2021). A sudden disruption in this balance via introduction of harmful chemicals in the environment and climate change that affect normal growth, call production or social behavior can negatively impact the survival of any species.
The raw data supporting the conclusions of this article will be made available by the authors, without undue reservation.
The animal study was reviewed and approved by National Animal Research Authority in Northeast Normal University, China.
AL conducted the field work, participated in the study design and data analysis, and contributed to the write the original draft. JF conceived the study design and provided support for the study. JK contributed to the study design, conducted data analysis, and wrote the manuscript. All authors contributed to the article and approved the submitted version.
This study was supported by the National Natural Science Foundation of China (grant numbers 31030011, 31872681, and 32071492).
The authors declare that the research was conducted in the absence of any commercial or financial relationships that could be construed as a potential conflict of interest.
All claims expressed in this article are solely those of the authors and do not necessarily represent those of their affiliated organizations, or those of the publisher, the editors and the reviewers. Any product that may be evaluated in this article, or claim that may be made by its manufacturer, is not guaranteed or endorsed by the publisher.
We appreciate Guanjun Lu, Jinhong Luo, Xuewen Wei, and Bo Luo for their help in the field work without which obtaining the data may not have been possible.
The Supplementary Material for this article can be found online at: https://www.frontiersin.org/articles/10.3389/fevo.2022.948324/full#supplementary-material
Amano, M., Kourogi, A., Aoki, K., Yoshioka, M., and Mori, K. (2014). Differences in sperm whale codas between two waters off Japan: possible geographic separation of vocal clans. J. Mammal. 95, 169–175.
Anholt, R. R. H., and Mackay, T. F. C. (2009). Principles of Behavioral Genetics. Oxford: Elsevier Academic Press.
Armbruster, W. S., and Schwaegerle, K. E. (1996). Causes of covariation of phenotypic traits among populations. J. Evol. Biol. 9, 261–276.
Baker, M. C. (2011). Geographic variation of three vocal signals in the Australian ringneck (Aves: Psittaciformes): do functionally similar signals have similar spatial distributions? Behaviour 148, 373–402.
Behr, O., and von Helversen, O. (2004). Bat serenades-complex courtship songs of the sac-winged bat (Saccopteryx bilineata). Behav. Ecol. Sociobiol. 56, 106–115.
Bohn, K. M., Schmidt-French, B., Ma, S. T., and Pollak, G. D. (2008). Syllable acoustics, temporal patterns, and call composition vary with behavioral context in Mexican free-tailed bats. J. Acoustical Soc. Am. 124, 1838–1848. doi: 10.1121/1.2953314
Bohn, K. M., Schmidt-French, B., Schwartz, C., Smotherman, M., and Pollak, G. D. (2009). Versatility and stereotypy of free-tailed bat songs. PLoS One 4:e6746. doi: 10.1371/journal.pone.0006746
Boughman, J. W. (1998). Vocal learning by greater spear-nosed bats. Proc. R. Soc.: Biol. Sci. 265, 227–233. doi: 10.1098/rspb.1998.0286
Boughman, J. W., and Wilkinson, G. S. (1998). Greater spear-nosed bats discriminate group mates by vocalizations. Animal Behav. 55, 1717–1732. doi: 10.1006/anbe.1997.0721
Bradbury, J. W., and Vehrencamp, S. L. (2011). Principles of Animal Communication, 2nd Edn. Sunderland, MD: Sinauer Associates Press.
Braune, P., Schmidt, S., and Zimmermann, E. (2008). Acoustic divergence in the communication of cryptic species of nocturnal primates (Microcebus ssp.). BMC Biol. 6:19. doi: 10.1186/1741-7007-6-19
Bu, Y., He, X., Zhou, H., Zhou, H., Zhang, Z., Zhang, C., et al. (2017). Morphology and mitochondrial gene divergence in Hipposideros armiger armiger occurs only in China. Mammalia 81, 135–146.
Byers, B. E. (1996). Geographic variation of song form within and among Chestnut-sided warbler populations. Auk 113, 288–299.
Claridge, M. F., Hollander, J. D., and Morgan, J. C. (1985). Variation in courtship signals and hybridization between geographically definable populations of the rice Brown planthopper, Nilaparvata lugens (Stål). Biol. J. Linnean Soc. 24, 35–49.
Darwin, C. (1859). On the Origin of Species by Means of Natural Selection, or the Preservation of Favoured Races in the Struggle for Life. London: John Murray.
Davidson, S. M., and Wilkinson, G. S. (2002). Geographic and individual variation in vocalizations by male Saccopteryx bilineata (Chiroptera: Emballonuridae). J. Mammal. 83, 526–535.
DeVoogd, T., and Nottebohm, F. (1981). Gonadal hormones induce dendritic growth in the adult avian brain. Science 214, 202–204.
Eiler, K. C., and Banack, S. A. (2004). Variability in the alarm call of golden-mantled ground squirrels (Spermophilus lateralis and S. saturatus). J. Mammal. 85, 43–50.
Ellers, J., and Slabbekoorn, H. (2003). Song divergence and male dispersal among bird populations: a spatially explicit model testing the role of vocal learning. Animal Behav. 65, 671–681.
Endler, J. A. (1977). Geographic Variation, Speciation and Clines. Princeton, NJ: Princeton University Press.
Esser, K. H. (1994). Audio-vocal learning in a non-human mammal: the lesser spear-nosed bat Phyllostomus discolor. Neuroreport 5, 1718–1720. doi: 10.1097/00001756-199409080-00007
Esser, K. H., and Schmidt, U. (2010). Mother-infant communication in the lesser spear-nosed bat phyllostomus discolor (Chiroptera, Phyllostomidae) - evidence for acoustic learning. Ethology 82, 156–168. doi: 10.1111/j.1439-0310.1989.tb00496.x
Esser, K. H., and Schubert, J. (1998). Vocal dialects in the lesser spear-nosed bat Phyllostomus discolor. Naturwissenschaften 85, 347–349.
Fenzl, T., and Schuller, G. (2007). Dissimilarities in the vocal control over communication and echolocation calls in bats. Behav. Brain Res. 182, 173–179. doi: 10.1016/j.bbr.2006.12.021
Fleming, T. H., and Eby, P. (2003). “Ecology of bat migration,” in Bat Ecology, eds T. H. Kunz and M. B. Fenton (Chicago, IL: University of Chicago Press).
Foote, J. R., Palazzi, E., and Mennill, D. J. (2013). Songs of the Eastern Phoebe, a suboscine songbird, are individually distinctive but do not vary geographically. Bioacoustics 22, 137–151.
Gadziola, M. A., Grimsley, J. M., Faure, S. P., and Wenstrup, A. J. J. (2012). Social vocalizations of big brown bats vary with behavioral context. PLoS One 7:e44550. doi: 10.1371/journal.pone.0044550
Garcia, M., Theunissen, F., Sèbe, F., Clavel, J., Ravignani, A., Marin-Cudraz, T., et al. (2020). Evolution of communication signals and information during species radiation. Nat. Commun. 11:4970.
Gillam, E. H., and McCracken, G. F. (2007). Variability in the echolocation of Tadarida brasiliensis: effects of geography and local acoustic environment. Animal Behav. 74, 277–286.
Gould, S. J., and Johnston, R. F. (1972). Geographic variation. Ann. Rev. Ecol. Systemat. 3, 457–498.
Guerra, J. E., Cruz-Nieto, J., Ortiz-Maciel, S. G., and Wright, T. F. (2008). Limited geographic variation in the vocalizations of the endangered thick-billed parrot: Implications for conservation strategies. Conder 110, 639–647. doi: 10.1525/cond.2008.8609
Hiryu, S., Katsura, K., Nagato, T., Yamazaki, H., Lin, L. K., Watanabe, Y., et al. (2006). Intra-individual variation in the vocalized frequency of the Taiwanese leaf-nosed bat, Hipposideros terasensis, influenced by conspecific colony members. J. Comp. Physiol. Neuroethol. Sensory Neural Behav. Physiol. 192, 807–815. doi: 10.1007/s00359-006-0118-5
Hoskin, C. J., Higgie, M., McDonald, K. R., and Moritz, C. (2005). Reinforcement drives rapid allopatric speciation. Nature 437, 1353–1356.
Hua, X., Greenhill, S. J., Cardillo, M., Schneemann, H., and Bromham, L. (2019). The ecological drivers of variation in global language diversity. Nat. Commun. 10:2047. doi: 10.1038/s41467-019-09842-2
Irwin, D. E. (2000). Song variation in an avian ring species. Evolution 54, 998–1010. doi: 10.1111/j.0014-3820.2000.tb00099.x
Jang, Y., Hahm, E. H., Lee, H. J., Park, S., Won, Y. J., and Choe, J. C. (2011). Geographic variation in advertisement calls in a tree frog species: gene flow and selection hypotheses. PLoS One 6:e23297. doi: 10.1371/journal.pone.0023297
Ji, W., and Suga, N. (2008). Tone-specific and nonspecific plasticity of the auditory cortex elicited by pseudoconditioning: role of acetylcholine receptors and the somatosensory cortex. J. Neurophysiol. 100, 1384–1396. doi: 10.1152/jn.90340.2008
Jiang, T., Guo, X., Lin, A., Wu, H., Sun, C., Feng, J., et al. (2019). Bats increase vocal amplitude and decrease vocal complexity to mitigate noise interference during social communication. Animal Cogn. 22, 199–212. doi: 10.1007/s10071-018-01235-0
Jiang, T., Liu, R., Metzner, W., Yuyan, Y., Li, S., Liu, S., et al. (2010). Geographical and individual variation in echolocation calls of the intermediate leaf-nosed bat, Hipposideros larvatus. Ethology 116, 691–703.
Jiang, T., Wu, H., and Feng, J. (2015). The patterns and causes of geographic variation in bat echolocation pulses. Int. Zool. 10, 241–256. doi: 10.1111/1749-4877.12129
Kanwal, J. K., Coddington, E., Frazer, R., Limbania, D., Turner, G., Davila, K. J., et al. (2021). Internal state: dynamic, interconnected communication loops distributed across body, brain, and time. Int. Comp. Biol. 61, 867–886. doi: 10.1093/icb/icab101
Kanwal, J. S. (2021). Sonic and ultrasonic communication in bats: acoustics, perception, and production. Neuroendocrine Regulation Animal Vocalization 2021, 239–265.
Kanwal, J. S., Matsumura, S., Ohlemiller, K., and Suga, N. (1994). Analysis of acoustic elements and syntax in communication sounds emitted by mustached bats. J. Acoustical Soc. Am. 96, 1229–1254. doi: 10.1121/1.410273
Kazial, K. A., Burnett, S. C., and Masters, W. M. (2001). Individual and group variation in echolocation calls of big brown bats, Eptesicus fuscus (Chiroptera: Vespertilionidae). J. Mammal. 82, 339–351.
Krebs, J. R., and Kroodsma, D. E. (1980). Repertoires and geographical variation in bird song. Adv. Study Behav. 11, 143–177.
Lameira, A., Delgado, R., and Wich, S. (2010). Review of geographic variation in terrestrial mammalian acoustic signals: human speech variation in a comparative perspective. J. Evol. Psychol. 8, 309–332.
Lampe, U., Reinhold, K., and Schmoll, T. (2014). How grasshoppers respond to road noise: developmental plasticity and population differentiation in acoustic signalling. Funct. Ecol. 28, 660–668.
Lee, S., and Hasegawa, T. (2014). Oceanic barriers promote language diversification in the Japanese Islands. J. Evol. Biol. 27, 1905–1912. doi: 10.1111/jeb.12442
Lin, A., Csorba, G., Li, L.-F., Jiang, T.-L., Lu, G.-J., Thong, V. D., et al. (2014). Phylogeography of Hipposideros armiger (Chiroptera: Hipposideridae) in the oriental region: the contribution of multiple Pleistocene glacial refugia and intrinsic factors to contemporary population genetic structure. J. Biogeography 41, 317–327.
Lin, A., Jiang, T., Feng, J., and Kanwal, J. S. (2016). Acoustically diverse vocalization repertoire in the Himalayan leaf-nosed bat, a widely distributed Hipposideros species. J. Acoustical Soc. Am. 140, 3765–3774. doi: 10.1121/1.4966286
Lin, A., Jiang, T., Kanwal, J. S., Lu, G., Luo, J., Wei, X., et al. (2015a). Geographical variation in echolocation vocalizations of the Himalayan leaf-nosed bat: contribution of morphological variation and cultural drift. Oikos 124, 364–371.
Lin, H., Kanwal, J. S., Jiang, T., Liu, Y., and Feng, J. (2015b). Social and vocal behavior in adult greater tube-nosed bats (Murina leucogaster). Zoology 118, 192–202. doi: 10.1016/j.zool.2014.12.005
Liu, T., Sun, K., Csorba, G., Zhang, K., Zhang, L., Zhao, H., et al. (2019). Species delimitation and evolutionary reconstruction within an integrative taxonomic framework: a case study on Rhinolophus macrotis complex (Chiroptera: Rhinolophidae). Mol. Phylogenet. Evol. 139:106544. doi: 10.1016/j.ympev.2019.106544
Liu, Y., Feng, J., and Metzner, W. (2013). Different auditory feedback control for echolocation and communication in horseshoe bats. PLoS One 8:e62710. doi: 10.1371/journal.pone.0062710
López-Bosch, D., Huang, J. C., Wang, Y., Palmeirim, A. F., Gibson, L., and López-Baucells, A. (2021). Bat echolocation in continental China: a systematic review and first acoustic identification key for the country. Mammal Res. 66, 405–416.
Luo, B., Santana, S. E., Pang, Y., Wang, M., Xiao, Y., and Feng, J. (2019). Wing morphology predicts geographic range size in vespertilionid bats. Sci. Rep. 9:4526.
Luo, J., Ou, W., Liu, Y., Wang, J., Wang, L., and Feng, J. (2012). Plasticity in echolocation calls of Myotis macrodactylus (Chiroptera: Vespertilionidae): implications for acoustic identification. Acta Theriol. 57, 137–143.
Ma, J., Kobayasi, K., Zhang, S., and Metzner, W. (2006). Vocal communication in adult greater horseshoe bats, Rhinolophus ferrumequinum. J. Comp. Physiol. Neuroethol. Sensory Neural Behav. Physiol. 192, 535–550. doi: 10.1007/s00359-006-0094-9
MacDougall-Shackleton, E. A., and MacDougall-Shackleton, S. A. (2001). Cultural and genetic evolution in mountain white-crowned sparrows: song dialects are associated with population structure. Evolution 55, 2568–2575. doi: 10.1111/j.0014-3820.2001.tb00769.x
Marler, P., and Tamura, M. (1962). Song “dialects” in three populations of white-crowned sparrows. Condor 64, 368–377. doi: 10.1006/anbe.1999.1118
Matthew, E. G., Stephen, C. B., and Mary, T. M. (2014). Sex and season differences in the echolocation pulses of big brown bats (Eptesicus fuscus) and their relation to mating activity. Acta Chiropterol. 16, 379–386.
Melendez, K. V., Jones, D. L., and Feng, A. S. (2006). Classification of communication signals of the little brown bat. J. Acoustical Soc. Am. 120, 1095–1102.
Mitani, J. C., Hunley, K. L., and Murdoch, M. E. (1999). Geographic variation in the calls of wild chimpanzees: a reassessment. Am. J. Primatol. 47, 133–151. doi: 10.1002/(SICI)1098-2345(1999)47:2<133::AID-AJP4>3.0.CO;2-I
Mooney, R., and Prather, J. F. (2005). The HVC microcircuit: the synaptic basis for interactions between song motor and vocal plasticity pathways. J. Neurosci. 25, 1952–1964. doi: 10.1523/JNEUROSCI.3726-04.2005
Naguib, M., Janik, V., Clayton, N., and Zuberbuhler, K. (2009). Vocal Communication in Birds and Mammals. New York, NY: Academic Press.
Neuweiler, G. (1980). “Auditory processing of echoes: peripheral processing,” in Animal Sonar Systems, eds R. Busnel and J. Fish (New York, NY: Plenum Press), 519–548.
Papale, E., Azzolin, M., Cascão, I., Gannier, A., Lammers, M., Martín, V., et al. (2014). Macro- and micro-geographic variation of short-beaked common dolphins whistles in the mediterranean sea and atlantic ocean. Ethol. Ecol. Evol. 26, 392–404.
Podos, J., and Warren, P. S. (2007). The evolution of geographic variation in birdsong. Adv. Study Behav. 37, 403–458.
Prat, Y., Azoulay, L., Dor, R., and Yovel, Y. (2017). Crowd vocal learning induces vocal dialects in bats: playback of conspecifics shapes fundamental frequency usage by pups. PLoS Biol. 15:e2002556. doi: 10.1371/journal.pbio.2002556
Pröhl, H., Hagemann, S., Karsch, J., and Höbel, G. (2007). Geographic variation in male sexual signals in strawberry poison frogs (Dendrobates pumilio). Ethology 113, 825–837.
Puechmaille, S. J., Ar Gouilh, M., Piyapan, P., Yokubol, M., Mie Mie, K., and Jeremy James, et al. (2011). The evolution of sensory divergence in the context of limited gene flow in the bumblebee bat. Nat. Commun. 2:573. doi: 10.1038/ncomms1582
Robin, V. V., Katti, M., Purushotham, C., Sancheti, A., and Sinha, A. (2011). Singing in the sky: song variation in an endemic bird on the sky islands of southern India. Animal Behav. 82, 513–520.
Rosenberg, M. S., and Anderson, C. D. (2011). PASSaGE: pattern analysis, spatial statistics and geographic exegesis. Version 2. Methods Ecol. Evol. 2, 229–232.
Rossoni, D. M., Demos, T. C., Goodman, S. M., Yego, R. K., Mohlman, J. L., Webala, P. W., et al. (2021). Genetic, morphological and acoustic differentiation of African trident bats (Rhinonycteridae: Triaenops). Zool. J. Linnean Soc. 192, 236–257.
Rübsamen, R., Neuweiler, G., and Sripathi, K. (1988). Comparative collicular tonotopy in two bat species adapted to movement detection, Hipposideros speoris and Megaderma lyra. J. Comp. Physiol. A: Neuroethol. Sensory Neural Behav. Physiol. 163, 271–285.
Smotherman, M., and Metzner, W. (2005). Auditory-feedback control of temporal call patterns in echolocating horseshoe bats. J. Neurophysiol. 93, 1295–1303. doi: 10.1152/jn.00653.2004
Sun, C., Jiang, T., Gu, H., Guo, X., Zhang, C., Gong, L., et al. (2020). Geographical variation of social calls and vocal discrimination in male Himalayan leaf-nosed bats. Animal Behav. 170, 15–26.
Sun, C., Zhang, C., Lucas, J. R., Lin, A., Feng, J., and Jiang, T. (2021). Territorial calls of the bat Hipposideros armiger may encode multiple types of information: body mass, dominance rank and individual identity. Animal Cogn. 24, 689–702. doi: 10.1007/s10071-020-01455-3
Suthers, R. A., Hartley, D. J., and Wenstrup, J. J. (1988). The acoustic role of tracheal chambers and nasal cavities in the production of sonar pulses by the horseshoe bat, Rhinolophus hildebrandti. J. Comp. Physiol. A 162, 799–813. doi: 10.1007/BF00610969
Toews, D. P. L. (2017). From song dialects to speciation in white-crowned sparrows. Mol. Ecol. 26, 2842–2844. doi: 10.1111/mec.14104
Velásquez, N. A., Opazo, D., Díaz, J., and Penna, M. (2014). Divergence of acoustic signals in a widely distributed frog: relevance of inter-male interactions. PLoS One 9:e87732. doi: 10.1371/journal.pone.0087732
Whaling, C. S., Nelson, D. A., and Marler, P. (1995). Testosterone-induced shortening of the storage phase of song development in birds interferes with vocal learning. Dev. Psychobiol. 28, 367–376. doi: 10.1002/dev.420280703
Wiley, R. H., and Richards, D. (1978). Physical constraints on acoustic communication in the atmosphere: implications for the evolution of animal vocalizations. Behav. Ecol. Sociobiol. 3, 69–94.
Wilkins, M. R., Seddon, N., and Safran, R. J. (2013). Evolutionary divergence in acoustic signals: causes and consequences. Trends Ecol. Evol. 28, 156–166.
Wright, T. F. (1996). Regional dialects in the contact call of a parrot. Proc. R. Soc. London. Series B: Biol. Sci. 263, 867–872.
Wright, T. F., Rodriguez, A. M., and Fleischer, R. C. (2005). Vocal dialects, sex-biased dispersal, and microsatellite population structure in the parrot Amazona auropalliata. Mol. Ecol. 14, 1197–1205. doi: 10.1111/j.1365-294X.2005.02466.x
Wu, H., Gong, L., Jiang, T., Feng, J., and Lin, A. (2021). Echolocation call frequencies of bats vary with body temperature and weather conditions. Animal Behav. 180, 51–61.
Yoshino, H., Armstrong, K. N., Izawa, M., Yokoyama, J. U. N., and Kawata, M. (2008). Genetic and acoustic population structuring in the Okinawa least horseshoe bat: are intercolony acoustic differences maintained by vertical maternal transmission? Mol. Ecol. 17, 4978–4991. doi: 10.1111/j.1365-294X.2008.03975.x
Keywords: bioacoustic signals, social communication, echolocating bats, population divergence, vocalizations, dialects, geographic variation, speech
Citation: Lin A, Feng J and Kanwal JS (2022) Geographic Variation in Social Vocalizations of the Great Himalayan Leaf-Nosed Bat, Hipposideros armiger: Acoustic Overflow Across Population Boundaries. Front. Ecol. Evol. 10:948324. doi: 10.3389/fevo.2022.948324
Received: 19 May 2022; Accepted: 21 June 2022;
Published: 27 July 2022.
Edited by:
Kirsten Bohn, Johns Hopkins University, United StatesReviewed by:
Angeles Salles, University of Illinois at Chicago, United StatesCopyright © 2022 Lin, Feng and Kanwal. This is an open-access article distributed under the terms of the Creative Commons Attribution License (CC BY). The use, distribution or reproduction in other forums is permitted, provided the original author(s) and the copyright owner(s) are credited and that the original publication in this journal is cited, in accordance with accepted academic practice. No use, distribution or reproduction is permitted which does not comply with these terms.
*Correspondence: Jagmeet S. Kanwal, a2Fud2FsakBnZW9yZ2V0b3duLmVkdQ==
Disclaimer: All claims expressed in this article are solely those of the authors and do not necessarily represent those of their affiliated organizations, or those of the publisher, the editors and the reviewers. Any product that may be evaluated in this article or claim that may be made by its manufacturer is not guaranteed or endorsed by the publisher.
Research integrity at Frontiers
Learn more about the work of our research integrity team to safeguard the quality of each article we publish.