- 1Department of Physiology, Southern Illinois University School of Medicine, Carbondale, IL, United States
- 2Department of Anatomy, Southern Illinois University School of Medicine, Carbondale, IL, United States
Evolutionary adaptation to a subterranean habitat consisting of extensive underground tunnel systems would presumably require adept spatial learning and memory, however, such capabilities have not been characterized to date in naked mole-rats (Heterocephalus glaber) which, like other members of Bathyergidae, are subterranean rodents. The goal of this study was to develop a method for effectively assessing spatial learning and memory by modifying a Hebb-Williams maze for use with these subterranean rodents. Established behavioral tests to assess spatial learning and memory have primarily focused on, and have been optimized for, more typical laboratory rodent species such as mice and rats. In the current study, we utilized species-appropriate motivators, analyzed learning curves associated with maze performance, and tested memory retention in naked mole-rats. Using a modified Hebb-Williams maze, naked mole-rats underwent 3 days of training, consisting of five trials per day wherein they could freely explore the maze in search of the reward chamber. Memory retention was then tested 1 day, 1 week, and 1 month following the last day of training. Performance was analyzed based on latency to the reward chamber, errors made, and distance traveled to reach the reward chamber. Overall, this study established a behavioral paradigm for assessing maze navigation, spatial learning, and spatial memory in subterranean rodents, including optimization of rewards and environmental motivators.
Introduction
The elaborate tunnel systems maintained by bathyergids are indicative of adept spatial navigation skills. This is supported by studies that have been conducted in other subterranean species (Kimchi and Terkel, 2001, 2004; Costanzo et al., 2009; Oosthuizen et al., 2013; Brachetta et al., 2014; Oosthuizen, 2020), including one study that demonstrated that Damaraland mole-rats outperformed voles and rats in long-term memory tests (Kimchi and Terkel, 2001). However, limited research has focused on maze optimizations for testing spatial learning and memory in subterranean species, and no such studies have been conducted in naked mole-rats to date.
Naked mole-rats are a rodent species native to eastern Africa (Sherman et al., 1991). Like other bathyergids, they are subterranean rodents that thrive in hot, humid environments and construct complex burrows of interconnected underground tunnels (Sherman et al., 1991). More extensive burrow systems are associated with greater spatial memory performance in other mole-rat species, likely due to the daily reliance on spatial cues required to navigate these underground labyrinths (Costanzo et al., 2009). These systems include designated living areas that consist of toilet chambers, food chambers, and nesting chambers (Faulkes and Bennett, 2021; Smith and Buffenstein, 2021), the complexity of which may also warrant well-developed spatial memory. Naked mole-rats establish subterranean tunnel systems that can extend several kilometers in length (Brett, 1991), which may be attributable in part to their large colony sizes of up to approximately 300 animals (Faulkes and Bennett, 2021). Both naked mole-rats and Damaraland mole-rats exhibit a social hierarchy characterized by set divisions of labor, as well as generally having one queen as the only reproductively active female (Wilson, 1971; Buffenstein et al., 2022). In Damaraland mole-rats, large colony sizes contributed to increased spatial navigation skills (du Toit et al., 2012), but this has yet to be experimentally investigated in naked mole-rats.
The subterranean habitats of Bathyergidae have resulted in evolutionary adaptations of sensory capabilities and related anatomical and neuroanatomical systems which must be considered when developing and interpreting behavioral tests. Traditionally, behavioral paradigms within a laboratory setting have been designed for more typical animal models (e.g., mouse and rat) and have incorporated aspects suited to their visual and hearing capabilities. These designs are often not translatable to more atypical research models, such as the subterranean naked mole-rat (Heterocephalus glaber), for whom sensory limitations such as functional blindness play a role (Hetling et al., 2005). In particular, spatial memory testing paradigms such as the Morris water maze (Morris, 1984) and radial arm maze (Olton and Samuelson, 1976; Brown and Cook, 1986) often rely on visual cues that provide a basis for navigation while in the maze.
Designing behavioral tasks for subterranean rodents requires taking into account their divergent sensory abilities from other members of Rodentia, as well as incorporating appropriate motivators. In the current study, we developed an effective paradigm for investigating spatial learning and memory in naked mole-rats, with consideration of a range of factors that may affect navigational memory abilities among colony members including age, sex, body mass, and dominance ranking within the social hierarchy. We hypothesized that naked mole-rats would exhibit spatial learning in a customized maze design by reaching the reward chamber faster (reduced latency) and with optimized navigation (shorter total distance traveled and fewer errors committed) across training trials, and that spatial memory would be robust based on the naked mole-rat’s adaptations to a subterranean habitat.
Materials and Methods
Animals
Forty-three naked mole-rats (H. glaber; Rüppell, 1842) (25 female, 18 male) encompassing a range of body masses and ages were used in this study. For a preliminary study using a non-continuous maze design, eight animals were used (Table 1). Following improvements to the maze design and incorporation of a continuous maze, thirty-five animals were used. Six of these animals were excluded due to missing data for their dominance ranking. Eleven additional animals had no more than one missing trial data point. For these eleven animals, the missing data point was replaced with linear interpolation. One additional animal was excluded as an outlier due to data points in the first learning trial exceeding ten standard deviations of the mean, while another was excluded due to passing away before the end of data collection. Thus, 27 animals were included in the continuous maze study (Table 1). For the memory probe analysis, two additional animals were excluded, one as an outlier with values that exceed 10 standard deviations of the mean and the other excluded for missing multiple data points. Thus, 25 animals of the initial 27 were included in the memory probe analysis. Body masses of the animals included in the analysis ranged from 23.0 to 101.0 g (Braintree Scientific compact portable scale model CB 1,001 with 0.1 g precision), with ages ranging from 0.39 to 6.68 years at the start of testing. All of the naked mole-rats were members of one of two laboratory breeding colonies (Colony A and Colony B) maintained in-house, with varying status within the colony (queens, breeders, non-breeders). Not all members of each colony were used. Animals were excluded based on involvement in other ongoing studies. The vivarium housing for each colony was maintained at approximately 27.8–30°C and at least 40% relative humidity, without a light/dark cycle due to the subterranean habitat of the animals (Sherman et al., 1991). Animal housing consisted of a series of chambers interconnected with tubing and designed to mimic their natural burrow systems. Free access to food and ample bedding were provided (for further housing details, see Artwohl et al., 2002). All aspects of the research performed for this study complied with our protocol approved by the Institutional Animal Care and Use Committee at Southern Illinois University, Carbondale, IL, United States, and were in accordance with the National Institutes of Health Guide for the Care and Use of Laboratory Animals (NIH Publication Nos. 8023 and 1978).
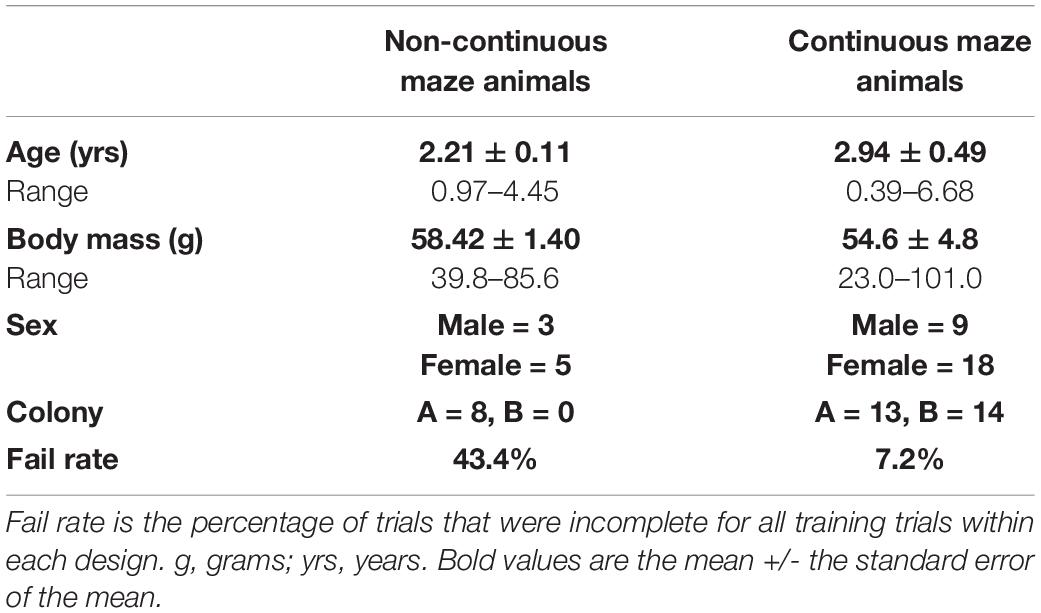
Table 1. Descriptive statistics (values shown are mean ± SEM, and range) for naked mole-rats used across two different spatial maze designs.
Maze Design
We designed modified versions of the Hebb-Williams maze, a common paradigm for testing spatial learning and memory in rodents. Modifications to the Hebb-Williams maze were based on studies that used similar tests of spatial navigation in other atypical rodent animal models, including the subterranean tuco-tuco (Antinuchi and Schleich, 2003; Schleich, 2010; Brachetta et al., 2014) and the blind mole-rat (Kimchi and Terkel, 2001, 2004). The mazes were constructed using PVC pipes that were cut in half longitudinally and covered with a sheet of clear acrylic, creating a trough for the animals to navigate through while allowing visibility for video recordings. The tubing had a 2” diameter, equal to the tubing in the housing chamber tunnel systems for the naked mole-rats. This tubing width was chosen based on previous research that showed greater learning and memory performance in the blind mole-rat when using narrower pathways that provide tactile cues in mazes (Kimchi and Terkel, 2004).
The first maze design we tested was non-continuous and included dead-ends (Figure 1A) similar to other Hebb-Williams-based mazes (Kimchi and Terkel, 2001, 2004; Costanzo et al., 2009; du Toit et al., 2012). Preliminary testing using a cohort of eight animals was conducted for this version of the maze (Table 1). Any dead-ends within the maze were sealed and a clear acrylic sheet was placed on top of the maze, allowing visualization of animal behavior and performance in the mazes from above. The reward chamber consisted of a cylindrical housing chamber made of clear cast acrylic with 8” diameter, 10” height, and 1/4” wall thickness. This reward chamber was heated with a heat lamp and filled with bedding from the animal’s home colony. It was connected to the maze via a clear cast acrylic 2” diameter connector tube with 1/4” wall thickness (US Plastics, Item #44530). Performance was measured using latency to the reward chamber, total distance traveled before reaching the reward chamber, and number of entries into the error zones. An entry into an error zone was counted if over 50% of the animal’s body passed into the zone, regardless of the direction of entry.
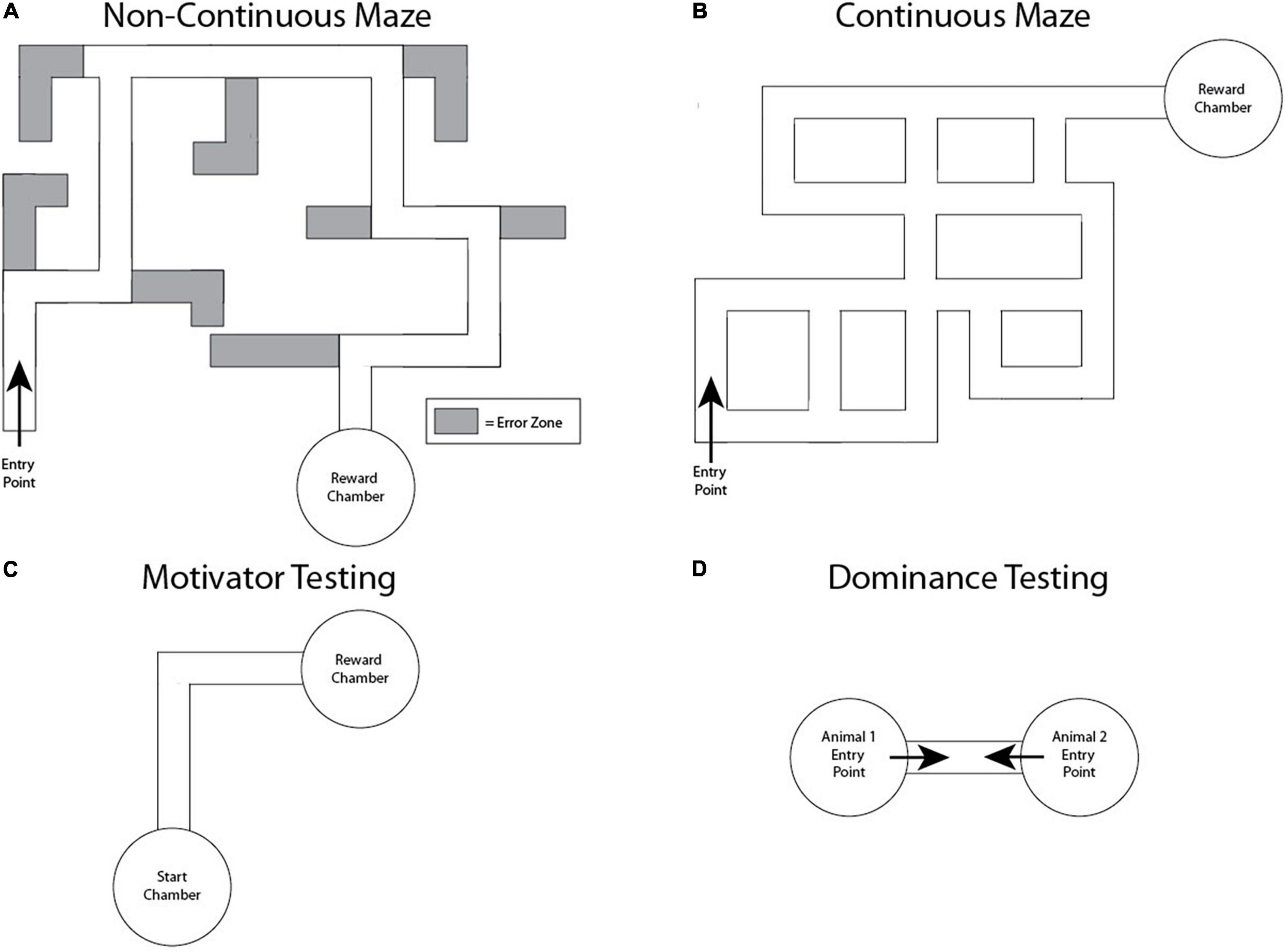
Figure 1. Naked mole-rat behavioral testing configurations. (A) Modified Hebb-Williams maze with a non-continuous maze design that included dead ends. Gray regions indicate areas where an error was recorded upon entry. (B) Modified Hebb-Williams maze with a continuous design lacking dead ends and sharing more similarities with the extensive, interconnected underground tunnel systems of the naked mole-rat’s habitat. (C) Motivator testing setup. Animals were placed in the start chamber and allowed 2 min to freely explore. Animal performance was tested using a range of motivators in the reward chamber and compared to performance when that chamber was empty. (D) Dominance testing setup. Animals were placed at the indicated entry points of each chamber, facing toward one another, and analyzed for how many times one passed over the other through the tubing across three trials.
Naked mole-rats react strongly to blockages in tunnels by persistently digging and biting in an attempt to get past the blockage (Sherman et al., 1991). During initial maze design testing (Figure 1A), we found that this distraction affected completion of the maze (“Fail Rate,” Table 1). Fail rate was calculated as the percentage of learning trials (Trial 1–15) in which animals did not complete the maze in the allotted 5 min (Table 1).
To address this, we redesigned the maze to a continuous configuration that lacked dead-ends (Figure 1B) and tested thirty-five animals in this maze configuration (Table 1). This allowed us to more effectively investigate whether the animals would be more motivated to complete the maze without having attention diverted from seeking the reward chamber due to dead-end distractors.
Reward Testing
In order to test the effectiveness of a range of motivators in naked mole-rats, and subsequently use the most effective motivator in the reward chamber, a separate experiment was performed prior to using the continuous maze. The same eight animals used in the testing of the first maze design were utilized to determine appropriate motivators for naked mole-rats. First, as a control, a start chamber was connected to a separate empty chamber using an L-shaped connector tube. Each animal’s behavior was first assessed in this control condition (see Data Analysis below). To test the effectiveness of a range of motivators, a motivator was added to the empty chamber, making it a reward chamber (Figure 1C). For the control test, each animal was placed in the start chamber and observed for one trial of 2 min in duration. Time spent in each chamber and latency to enter the empty chamber were recorded. This was then compared to each animal’s performance with a motivator present in the reward chamber. All animals were tested with one motivator (e.g., heat lamp) for one trial of 2 min in duration before switching to the next motivator, and motivators were tested in the following order: heat lamp, darkened chamber (shielded from outside light with black construction paper), food (sweet potato, banana baby food, and ProNutro—all items placed together in the food chamber, for all animals tested), home colony bedding, and foreign colony bedding (Table 2). The start and empty or reward chambers were wiped with 70% isopropyl ethanol between testing sessions with different animals. The resulting optimized motivator, a combination of home colony bedding and a heat lamp, was used for the continuous maze reward chamber.
Determination of Dominance Rank
Social hierarchy rank was determined using a dominance testing paradigm modeled after similar testing in previous studies in naked mole-rats (Clarke and Faulkes, 1997, 1998). All animals used in the continuous version of the maze were marked with their ID numbers on their backs using a permanent marker to allow for visual tracking of behaviors during dominance testing. Two researchers placed one naked mole-rat each in separate, circular chambers. Both chambers were connected via a clear polycarbonate tube. The animals were oriented at the open ends of the tube such that they met face to face when they moved in a forward direction (Figure 1D). Encounters were only rated if one animal passed over the top of another while in the tube. The test session ended after three instances of face-to-face pass-over behaviors were recorded. After each session, the chambers and tubing were cleaned with 70% isopropyl alcohol. Animal pairings were randomly generated with no pair undergoing more than one testing session together. Each animal underwent at least three testing sessions, each with a novel paired animal.
Dominance ranking was determined for the continuous maze animals using previously described methods with naked mole-rats (Clarke and Faulkes, 1997; Gilbert et al., 2020). Using a package extension for use with R Studio (R Core Team, 2021) to calculate the Elo rating (Neumann and Kulik, 2020), the results of each testing session across multiple days were entered to yield the rank of each individual animal tested. The higher the ranking, the more dominant the animal.
Experimental Paradigm
All spatial learning trials occurred within the same 4 h time block across every day of testing (8 a.m.–12 p.m.). All testing was performed in a brightly lit behavior room, maintained at 18.5–21°C and at least 40% relative humidity. Animals were brought into the room in transfer chambers and allowed to acclimate in the reward chamber of the maze for 5 min. Animals were then removed from the reward chamber and placed at the predetermined entry point of the maze, facing forward (Figures 1A,B). The naked mole-rat was allowed to explore the maze until it reached the reward chamber. The trial was considered complete when 5 min elapsed or when the animal successfully navigated to the reward chamber, whichever came first. If the animal was unable to reach the reward chamber before 5 min elapsed, it was gently guided to the chamber by the experimenter following cessation of the trial and the trial counted as a failed trial. Upon entry into the reward chamber, a block was placed at the entrance to prevent reentry into the maze. The animal was allowed 5 min to rest in and explore the chamber, after which time the next trial began. Performance was recorded with a camcorder (FHD 1080P, YEHOOVJIANGER) and analyzed in real-time using ANY-maze software (Stoelting Co., Wood Dale, IL, United States).
Animals underwent spatial learning/training for three consecutive days with five trials per day for a total of fifteen trials (Figure 2). Between each trial, the maze was cleaned with 70% isopropyl alcohol to reduce the effect of olfactory cues on performance in subsequent trials. After each day of testing, the maze was deconstructed and washed with non-scented soap and water. Spatial memory retention was tested 1 day and 1 week following the last day of training for the non-continuous maze and 1 day, 1 week, and 1 month for the continuous maze (Figure 2). On memory probe days, the animal freely explored the maze for one trial of 5 min or until it reached the reward chamber, whichever occurred first. In memory probe trials, the reward chamber contained the same motivators used in learning trials. If the animal was unable to complete the maze within the 5 min allotted, it was returned to the colony without being guided to the reward chamber.
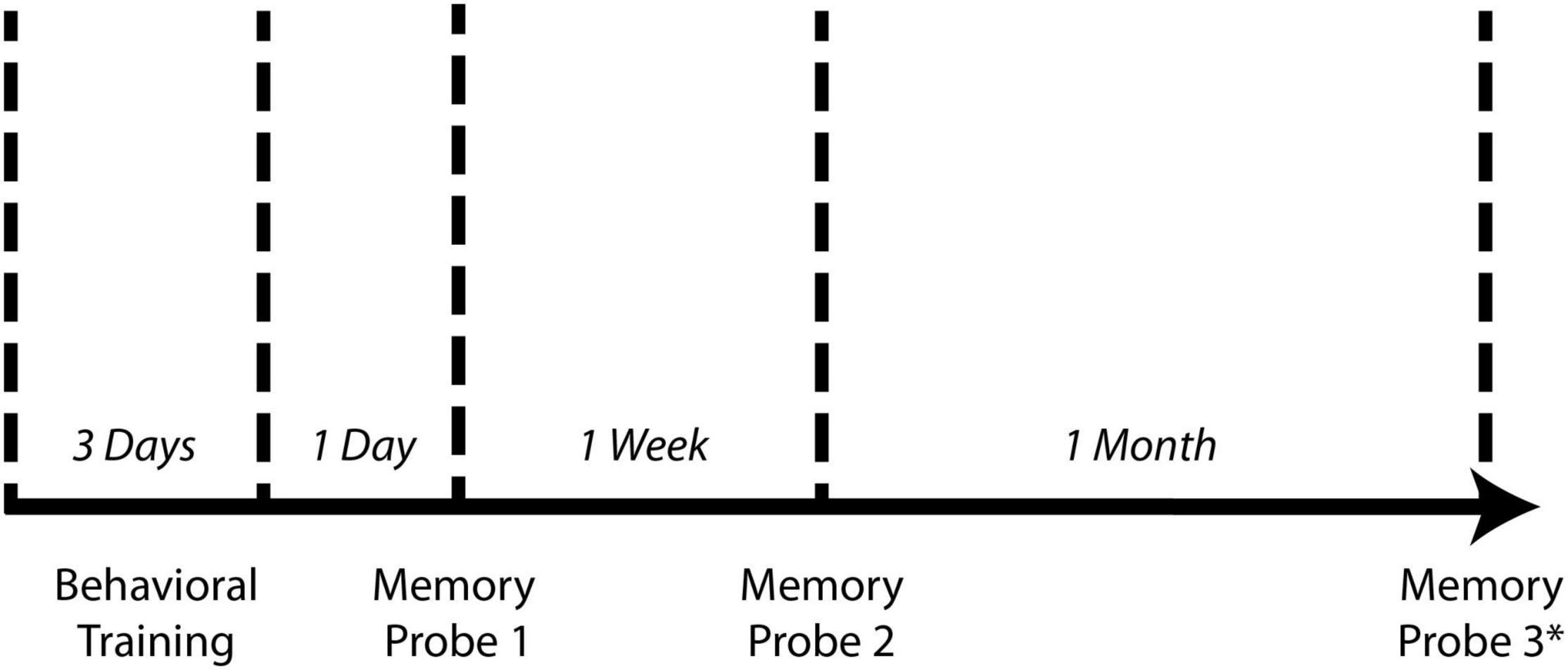
Figure 2. Spatial learning and memory testing timeline. For both the non-continuous maze and the continuous maze, animals underwent 3 days of behavioral training consisting of five trials per day. One day, 1 week, and 1 month following the last day of training, animals underwent a single memory probe trial. *One month memory probe trial was performed for the continuous maze but was not performed for the non-continuous maze due to the lack of a learning curve (see Figure 6).
Data Analysis
Paired sample t-tests were first used to examine the effectiveness of different motivators for use in the reward chamber. P-values were first calculated by using raw values to compare latency to the reward chamber vs. latency to the empty chamber (Figure 3A) and dwell time in the reward chamber vs. dwell time in the empty chamber (Figure 3B). The dwell time ratio for reward (DTRR) and control conditions with an empty chamber (DTRE) were calculated as follows, where DTS represents the dwell time spent in the start chamber under reward (DTSR) vs. control conditions with an empty chamber (DTSE), and DT represents the dwell time in the finish chamber between control (DTE, dwell time spent in the empty chamber) vs. reward conditions (DTR, dwell time spent in the reward chamber):
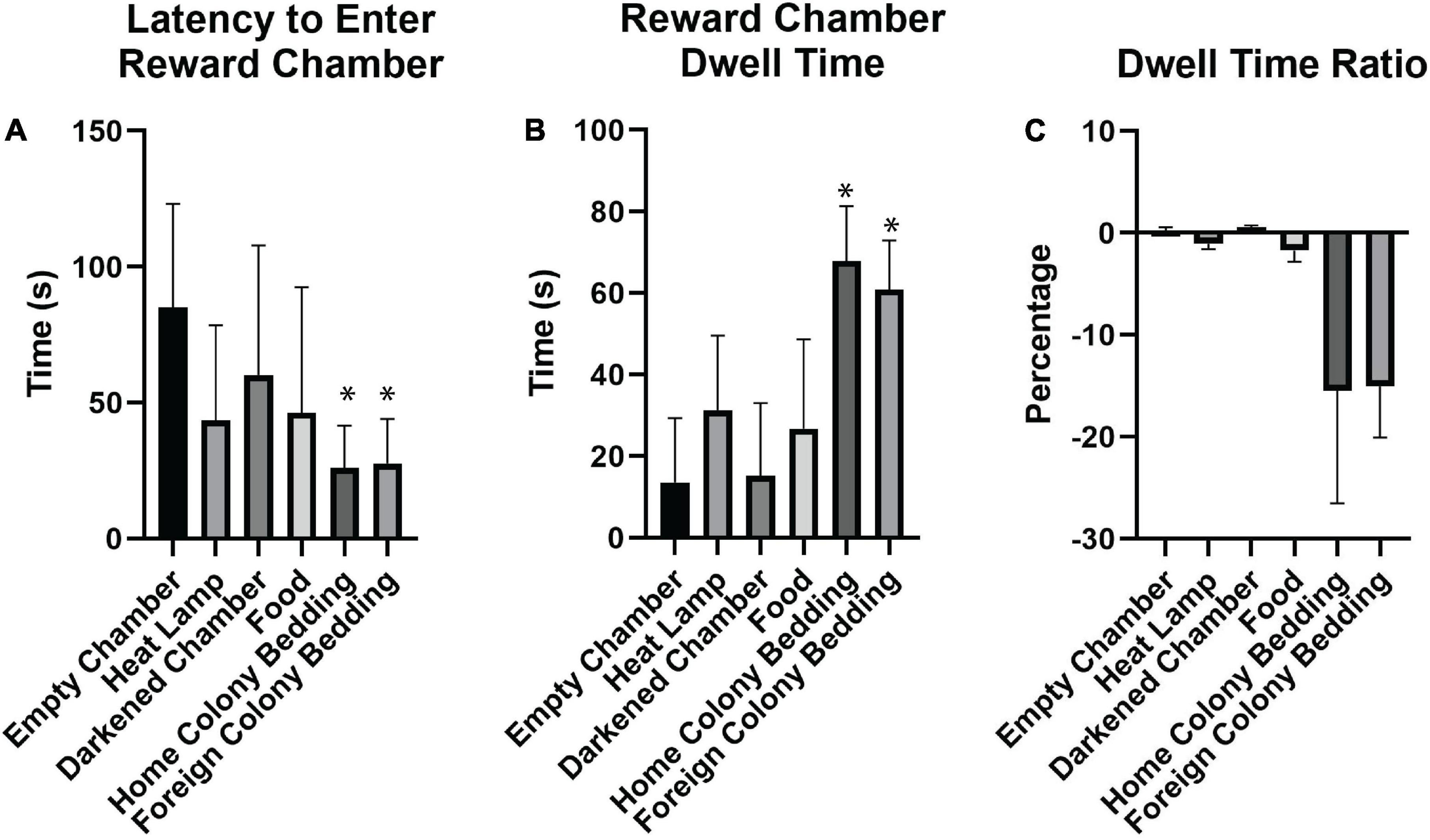
Figure 3. Effectiveness of different motivators for naked mole-rats. (A) Of the motivators tested, home colony bedding, foreign colony bedding, and heat resulted in the shortest latencies to enter the reward chamber compared to an empty chamber. (B) Both home and foreign colony bedding resulted in the greatest increase in dwell time in the reward chamber compared to the start chamber. (C) In assessing the Dwell Time Ratio, animals spent relatively more time in the reward chamber compared to the start chamber when either home or foreign colony bedding was present. A negative percentage indicates more time spent in the reward chamber than the start chamber. *Indicates a significant (p < 0.05) difference from the empty chamber value. s, seconds.
A paired sample t-test was also conducted for DTRR vs. DTRE (Figure 3C). Bonferroni corrected p-values were used to determine significance. A summary of results from the paired sample t-tests can be found in Table 2.
For the continuous maze, latency to the reward chamber and distance traveled were analyzed to investigate spatial learning and memory performance (analyses adapted from Vallianatou et al., 2020). For animals with one trial’s worth of missing data points, the missing points were linearly interpolated by averaging the values for the trial preceding the missing point and the trial following the missing point (e.g., a Trial 4 data point was interpolated by averaging the values of the animal on Trial 3 and Trial 5). To investigate differences in the learning phase of the continuous maze, we used two different multivariate repeated measures factorial MANOVAs. These models incorporated body mass and age as covariates; sex and colony as fixed factors; and latency and distance as dependent measures. These models were used to determine any main effects or interactions on performance in a manner similar to previous studies (Costanzo et al., 2009; Oosthuizen et al., 2013; Oosthuizen, 2020). The first repeated measures MANOVA used both Day and Trial as within-subjects factors, wherein each day was subdivided into its respective 5 trials. The second model was used for ease of interpretation and modeled only Trials without distinguishing by days. In the second model, Trials were analyzed as Trial 1 through Trial 15 rather than Day 1 through 3 each with Trial 1 through Trial 5. Estimated marginal means and confidence intervals from these models were used to graph the adjusted performance values (see Figures 5, 6). These models adjusted for the covariates at Body Mass = 54.60 g and Age = 2.94 yrs. The repeated measure MANOVA was also conducted separately for each colony in order to incorporate dominance ranking as a covariate. These colony specific analyses were done because no dominance testing can be performed between animals of different colonies, as this testing results in lethal confrontations. The colony-specific models used body mass, age, and dominance ranking as covariates; sex as a fixed factor; and latency and distance as dependent measures. These analyses were also performed in two separate ways, one with two repeated measures Day and Trial and the second with Trials as the only within-subject factor. Colony An estimated marginal means were computed using: Mass = 54.63 g, Age = 2.35 years, Rank = 957.40. Colony B estimated marginal means were computed using: Mass = 54.56 g, Age = 3.49 years, Rank = 1002.96. All aforementioned statistical analyses were also conducted as separate repeated measures ANOVAs with speed as the dependent variable to complement the latency and distance analyses. These models were conducted separately because speed is equal to distance divided by latency. Bonferroni correction was utilized for post hoc pairwise comparison for all Day and Trial effects.
Performance percentages on memory probe trials were computed for both maze configurations (non-continuous and continuous), comparing performance (latency and distance traveled) from individual memory probe days to the last training day. Latency and distance traveled were analyzed for both continuous and non-continuous maze designs, whereas errors were analyzed only for the non-continuous maze design. These were calculated such that the value from the memory probe trial (1, 2, or 3) was divided by the last training day trial (Trial 5 on training Day 3; Figure 2 and Table 3). A Repeated Measures MANOVA using latency performance and distance performance as dependent measures; body mass and age as covariates; sex and colony as fixed factors; and memory probe trial (1, 2, or 3) as the within-subjects factor. This analysis was conducted for each colony separately and combined. This set of statistical analyses for memory probe trials were also conducted as repeated measures ANOVAs with speed as the dependent variable in lieu of latency and distance.
Results
Testing the Relative Effectiveness of a Range of Behavioral Motivators in Naked Mole-Rats
We tested different motivators in order to optimize subsequent spatial maze testing performance (Figure 1C). Using paired sample t-tests for the raw values of latency, dwell time, and dwell time ratio compared to the empty chamber, similar motivators proved to be significant (Table 2 and Figure 3). Latency was enhanced with the presence of home colony bedding [t(7) = 5.904, p = 0.003] or foreign colony bedding [t(7) = 5.352, p = 0.005], with heat approaching significance [t(7) = 3.21, p = 0.075]. Dwell Time Ratio was not significant for any motivator tested, but Dwell Time was significantly greater when home colony bedding [t(7) = −9.623, p < 0.001] or foreign colony bedding [t(7) = −7.904, p < 0.001] were present in the reward chamber. Based on these sets of analyses, heat and the presence of either bedding type (foreign or home bedding) were the most effective motivators when presented independently. Thus, both heat and home bedding were chosen for the reward chamber in subsequent continuous maze testing. Foreign colony bedding, though also effective, was not selected based on the potential risk of an experimental animal being attacked upon reintroduction to the home colony due to smelling like an intruder (Braude et al., 2021).
Spatial Learning in Naked Mole-Rats
For the preliminary data set collected using a non-continuous maze design (n = 8; Figure 1A), no learning curves were evident across training trials for latency to the reward chamber (Figure 4A), total number of errors (wrong turns) made (Figure 4B), or total distance traveled (Figure 4C). Fail rate was also high (Table 1). The poor performance of naked mole-rats in this non-continuous maze design, along with the observation that the non-continuous maze design may have created a confound (i.e., dead-ends proving to be strong distractors that obscured the true potential for naked mole-rats to exhibit spatial learning, and memory improvements), led us to redesign the maze in a continuous layout with no dead-ends.
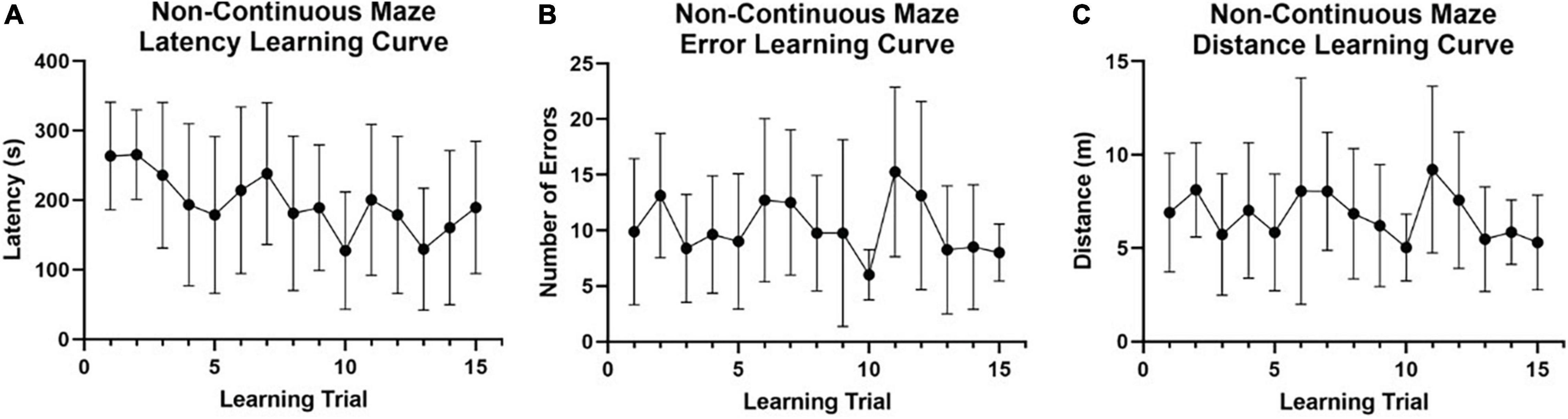
Figure 4. Non-continuous spatial maze learning performance in naked mole-rats. Preliminary data were collected (n = 8) using a non-continuous modified version of the Hebb-Williams maze (see Figure 1A) to investigate spatial learning and memory capabilities in naked mole-rats across 15 training trials (5 trials per day for 3 days). (A) Latency to reach the reward chamber was averaged across animals for each of the training/learning trials. Non-continuous maze performance exhibited a very gradual latency learning curve with a trend toward decreased latency across trials but large variability in individual performance. (B) The average number of entries into an error zone was plotted for all animals tested across the training/learning trials. No error reduction learning curve was present for the non-continuous maze. (C) Total distance traveled prior to reaching the reward chamber was averaged across animals for each trial and plotted for all training/learning trials. Non-continuous maze performance exhibited large variability in individual performance and no learning curve related to decreases in distance traveled (optimizing route to the reward chamber). m, meters; s, seconds.
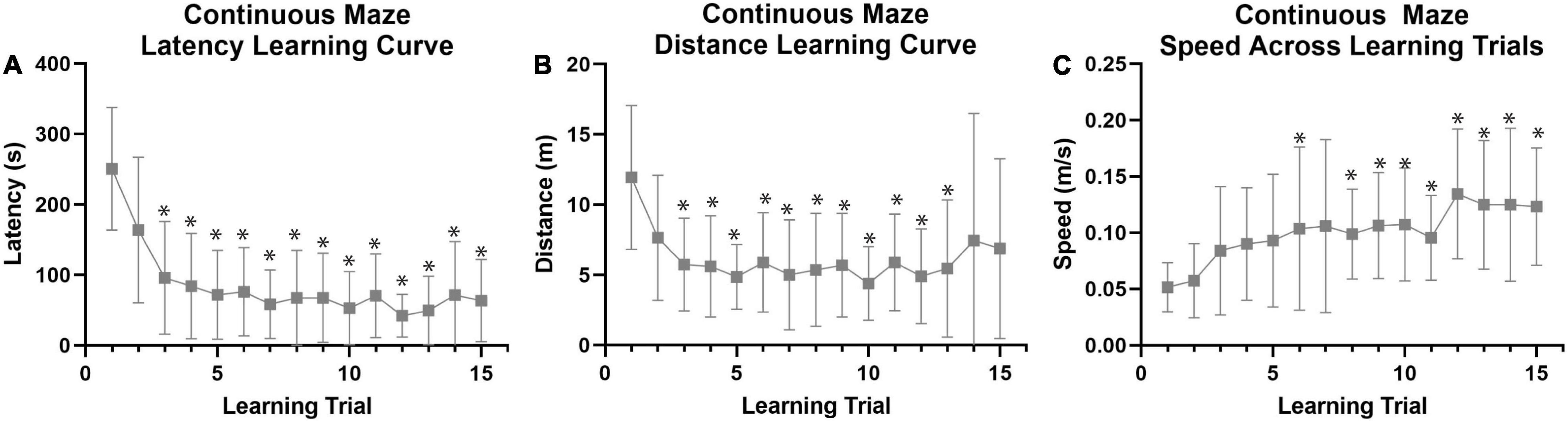
Figure 5. Continuous spatial maze learning performance in naked mole-rats. Data were collected (n = 27) using a continuous maze design (see Figure 1B) to investigate spatial learning and memory capabilities in naked mole-rats across 15 training trials (5 trials per day for 3 days). (A) Average latency to the reward chamber revealed a distinct learning curve across training/learning trials. Comparison of trial 1 to trials 2–15 revealed a significant decrease in latency by trial 3. (B) Average total distance traveled also exhibited a learning curve across training/learning trials. Comparison of trial 1 to trials 2–15 revealed a significant decrease in distance by trial 3. (C) Average speed traveled significantly increased across training trials by Trial 6. *p < 0.05 when compared to Trial 1. m, meters; s, seconds.
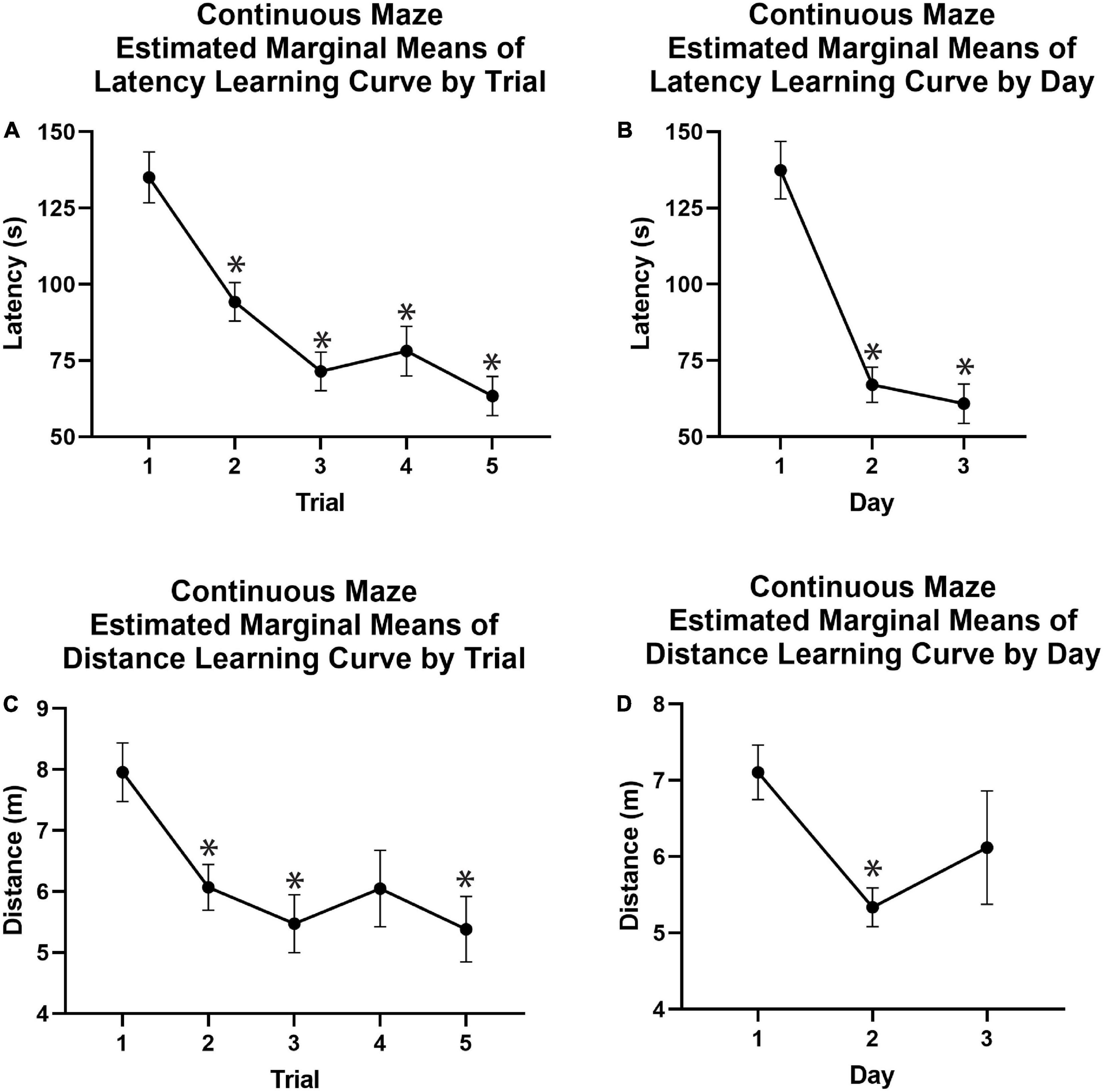
Figure 6. Continuous spatial maze learning curves using estimated marginal means. (A) Latency to the reward chamber was significantly reduced on average in Trials 2, 3, 4, and 5 as compared to Trial 1 across Days 1–3, demonstrating a main effect of trial independent of day for latency. (B) The mean latency for each learning day showed that animals reached the reward chamber in significantly less time on Days 2 and 3 compared to Day 1. (C) Animals exhibited significant learning effects when comparing distance traveled on Trials 2, 3, and 5 to that of Trial 1 across Days 1–3, demonstrating a main effect of Trial independent of Day for distance. (D) Animals traveled significantly less distance on Day 2 compared to Day 1. Covariates appearing in the model were evaluated at the following values: Mass = 54.60, Age = 2.94.*p < 0.05. m, meters; s, seconds.
Both colonies (Colony A and Colony B) were analyzed together for maximum power with the continuous maze design (n = 27; Figure 1B), and using a repeated measures MANOVA that did not model the effect of testing on separate days for simplicity of interpretation. This analysis revealed a distinct learning curve across the 15 training trials (Figure 5A) that was absent in the non-continuous maze. There was a multivariate effect of trial number on performance [F(28, 588) = 3.074, p < 0.001, Figure 5A and Supplementary Table 1]. The multivariate effect of Trial was driven by main effects on both latency and distance. Repeated pairwise comparisons of Trial 1 with Trials 2–15 revealed that latency (Bonferroni corrected p < 0.05, Figure 5A and Supplementary Table 1) and distance (Bonferroni corrected p < 0.05, Figure 5B and Supplementary Table 1) decreased significantly by the 3rd trial, whereas speed increased significantly by the 6th trial (Bonferroni corrected p < 0.05, Figure 5C and Supplementary Table 3). In addition, female animals had a significantly larger increase in speed across learning trials than males [F(14, 294) = 2.811, p = 0.001, Supplementary Table 3], though there were no significant differences between sexes for latency and distances across trials.
When utilizing a model that did incorporate separate testing days into the model similar results were obtained. There was a multivariate effect of Trial [F(8, 168) = 3.732, p < 0.001, Supplementary Table 2] driven by significant main effects of trial on both latency [F(4, 84) = 5.494, p = 0.001, Supplementary Table 2] and distance [F(4, 84) = 2.809, p = 0.031, Supplementary Table 2], but not speed [F(4, 84) = 2.396, p = 0.051, Supplementary Table 4]. Bonferroni-corrected pairwise comparisons showed that animals traveled significantly less during Trials 2, 3, and 5 of any given day vs. Trial 1 (−1.888, −2.4843, −1.910, and −2.574 m respective changes; p < 0.05; Figure 6C and Supplementary Table 2). Similarly, latency significantly decreased from Trial 1 to Trials 2, 3, and 5 (−43.515, −61.82, and −72.84 s respective changes; p < 0.05; Figure 6A and Supplementary Table 2).
There was no multivariate effect of Day, independent of trial number. There was also no univariate main effect of Day on latency, speed, or distance traveled to reach the reward chamber. However, pairwise comparisons demonstrated that distance decreased significantly between Day 1 and 2 of training (−1.768 m, Bonferroni corrected p = 0.002; Figure 6D and Supplementary Table 2). There was no significant decrease between distance traveled Day 1 and 3 of training. The average latency across trials on Day 1 of the learning period was significantly longer than latencies on Day 2 (70.376 s, p < 0.05; Figure 6B and Supplementary Table 2) and Day 3 (76.556 s, p < 0.05; Figure 6B and Supplementary Table 2).
There was a significant multivariate Day by Trial interaction [F(16, 36) = 3.534, p < 0.001, Supplementary Table 2] driven by significant interactions for both latency [F(8, 168) = 6.006, p < 0.001, Supplementary Table 2] and distance [F(8, 168) = 4.084, p < 0.001, Supplementary Table 2]. There was also a significant interaction of Day and Trial on speed [F(8, 168) = 2.40, p = 0.018, Supplementary Table 4]. Both latency and distance demonstrated the greatest performance improvements across trials on Day 1 of training with performance improvements plateauing across trials in Days 2 and 3, showing that most learning occurred on Day 1.
There was no multivariate interaction between Day and Body Mass, however, there was a univariate effect on latency [F(2, 42) = 4.137, p = 0.023, Supplementary Table 2], but not distance. Larger animals had higher latencies to reach the reward chamber in early days of training with these differences decreasing across days despite all animals traveling comparable distances.
No multivariate interaction of Trial by Age was present. However, there was a univariate effect of Trial by Age on distance [F(4, 84) = 2.580, p = 0.043, Supplementary Table 2] indicating better performance for younger animals in Trials 2 and 3, but for older animals in Trials 4 and 5. There was also a significant multivariate 3-way Day by Trial by Body Mass interaction [F(16, 336) = 1.988, p = 0.013, Supplementary Table 2] driven by significant interactions on both latency [F(8, 168) = 3.245, p = 0.002, Supplementary Table 2] and distance [F(8, 168) = 2.398, p = 0.018, Supplementary Table 2]. This effect indicated that the shape of the learning curve across days and trials was impacted by body mass with smaller animals showing faster learning initially, with more variable performance in later training trials.
There was no Day by Trial by Sex multivariate interaction on performance, nor univariate effects on latency and distance. However, there was a significant 3-way univariate interaction on speed. Female animals increased speed throughout learning trials significantly more than males [F(8, 168) = 3.443, p = 0.001, Supplementary Table 4].
No other multivariate or univariate effects or interactions (Day by Age, Day by Sex, or Day by Colony, Trial by Mass, Trial by Sex, and Trial by Colony) were significant for latency to reach the reward chamber, distance traveled, or speed.
In order to incorporate dominance ranking (a colony specific measure), into the GLM, the data set was split by colony for analysis. There were no significant effects of dominance rank on latency or distance across trials and days, nor were there any interactions of dominance rank with any of the other variables in the model (for detailed statistics see Supplementary Tables 1, 2). Speed traveled varied by dominance rank for Colony B with subordinate animals traveling faster across trials than dominant animals (for detailed statistics see Supplementary Tables 3, 4).
These findings indicate that naked mole-rats exhibited significant spatial learning in the continuous maze. The bulk of the performance improvements occurred within the first 3 trials, indicating rapid spatial learning. Both latency and distance calculations were informative in assessing spatial learning gains in this particular maze configuration in naked mole-rats, suggesting that naked mole-rats were able to rapidly learn to navigate the continuous maze design.
Spatial Memory in Naked Mole-Rats
Mean performance was analyzed across memory probe trials for both the non-continuous maze and the continuous maze (Table 3). There were no significant differences between the last trial of the last learning day vs. memory probe trials 1, 2, or 3 in assessing latency (Figure 7A) or distance traveled (Figure 7B), nor any effects of age, sex, ranking, or mass. This indicates that spatial memory was retained for at least 1 month in naked mole-rats.
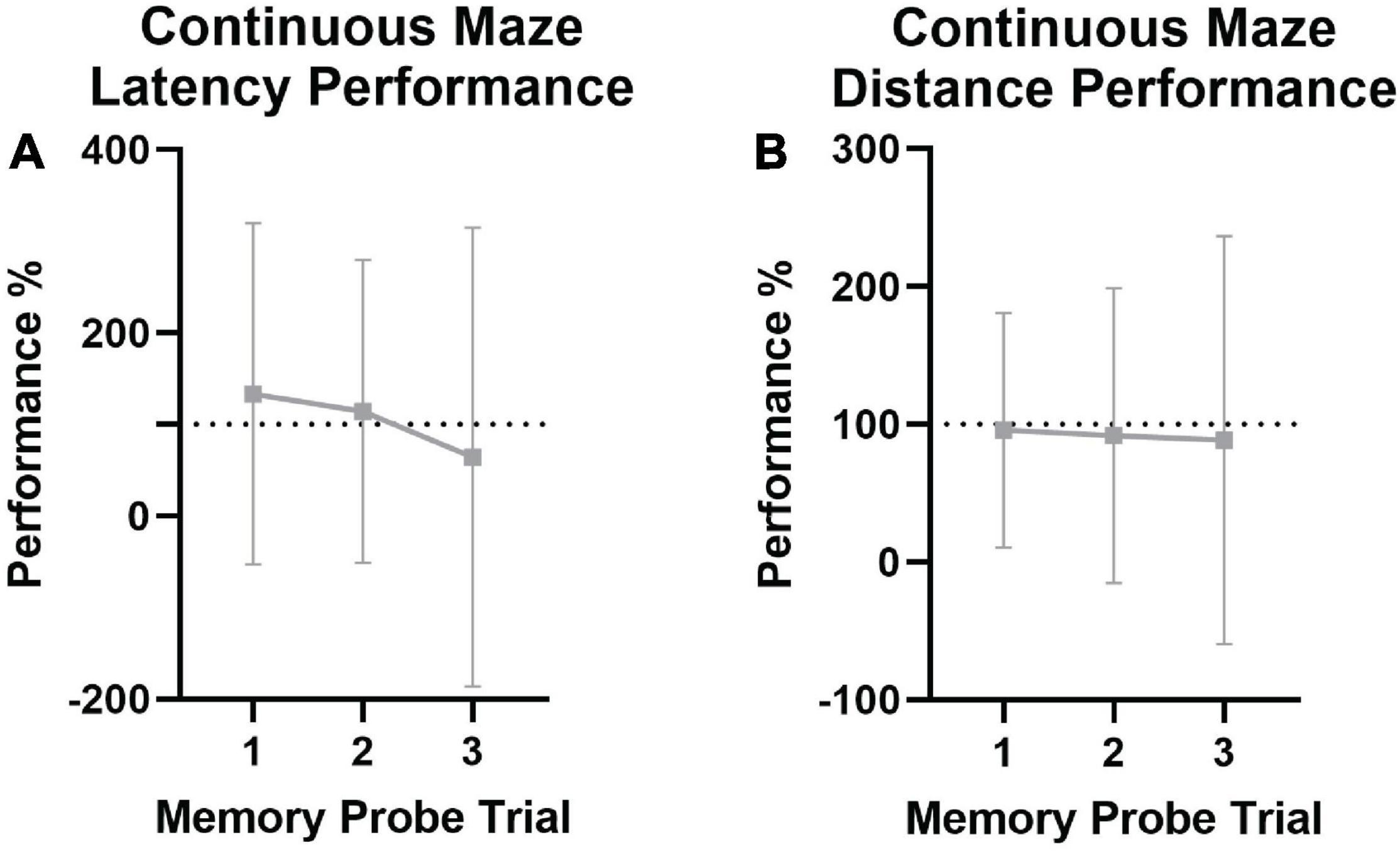
Figure 7. Continuous spatial maze memory performance in naked mole-rats. Animals were tested for spatial memory retention at 1 day, 1 week, and 1 month (memory probe 1, 2, and 3, respectively) following training. Dashed lines indicate the point of equivalence for performance on the last training day. (A) Latency across memory probe trials, with memory probe trial latency performance shown as a percentage of the last trial on the last training day in the learning phase of testing. No significant differences were found, indicating spatial memory retention, although this could be skewed by the high variability present. (B) Total distance traveled across memory probe trials, with distance traveled during the indicated memory probe trial shown as a percentage of the distance traveled on the last trial of the last training day. No significant impairments in performance were noted across the three time points.
Discussion
The present study created a customized and effective maze design for testing spatial learning and memory in naked mole-rats. We predicted that animals would exhibit spatial learning by reaching the reward chamber faster (reduced latency) and more adeptly (shorter total distance traveled, and/or fewer errors committed, indicating optimized maze navigation) across training trials. This would result in steep learning curves and abrupt plateaus upon reaching peak performance. In order to effectively create a maze to evaluate these measures of learning and memory, we made targeted alterations to the Hebb-Williams maze design for use in naked mole-rats based on their unique sensory systems.
Naked mole-rats are functionally blind, with low visual acuity but some remnants of light sensitivity. The olivary pretectal nucleus, a region implicated in light detection, is small but maintains its typical morphology (Crish et al., 2006). Paired with conserved pupillary constriction responses to light, in addition to behavioral responses to light detection (e.g., freezing behaviors with sudden changes in brightness), naked mole-rats retain the ability to detect changes in light intensity (Hetling et al., 2005) but are otherwise visually impaired. Such reductions in visual capabilities are thought to conserve sensory resources in favor of other modalities (e.g., tactile) that are more useful in underground tunnel systems which lack many meaningful visual cues that would facilitate behaviors such as navigation. Because traditional protocols for spatial mazes utilize visual cues, adjustments were necessary to accommodate the visual deficits of naked mole-rats.
The naked mole-rat’s subterranean habitat is also thought to have driven a reduction in auditory capabilities. Naked mole-rats are most sensitive to frequencies between 65 and 12.8 kHz (Heffner and Heffner, 1993; Okanoya et al., 2018). Naked mole-rats demonstrate poor sound localization in addition to having smaller auditory brainstem nuclei related to binaural, high-frequency sound processing (Heffner and Heffner, 1993). Their cochleas are characterized by less extensive coiling (Mason et al., 2016). These features, along with differential variations in hair cell density within the cochlea (Barone et al., 2019), are all possible contributions to the demonstrated poor sound localization abilities in this species (Heffner and Heffner, 1993). Due to the relatively reduced auditory capabilities of naked mole-rats, auditory stimuli were not incorporated into the spatial maze design to help guide the animals to the reward chamber.
In contrast to auditory and visual systems, the somatosensory system of naked mole-rats is well-developed and includes an array of tactile hairs distributed on the entire body and face (Crish et al., 2003; Henry et al., 2006). With approximately 40 tactile hairs on each side of the body, naked mole-rats display robust orienting responses to stimulation of individual hairs (Crish et al., 2003). These sensory hairs are also present in some members of Bathyergidae (Bennett and Jarvis, 2004; Bennett et al., 2009; Walcher et al., 2018), providing sensory information that compensates for visual impairments in subterranean rodents (Jarvis and Bennett, 2017). Based on these sensory specializations, we constructed a spatial maze with tubing that was narrow enough to stimulate tactile hairs and provide spatial cues. Previous studies in the blind mole-rat demonstrated enhanced learning and memory performance when using similarly narrow passageways that provided tactile cues in spatial mazes (Kimchi and Terkel, 2004).
During the preliminary data collection phase of this study, we initially implemented a modified Hebb-Williams maze design that was non-continuous (Figure 1A). However, this maze design proved to be ineffective in eliciting the true spatial learning and memory capabilities of naked mole-rats, and no learning curves were evident when testing animals in the non-continuous maze (Figure 6). This may be due to the confounding distraction of tunnel dead-ends. Naked mole-rats exhibited a strong preference to attempt to dig or bite through the dead-ends of a tunnel rather than seek the reward chamber, which highly affected measures of spatial learning and memory (e.g., latency) in addition to affecting completion of the maze within the allotted time (i.e., Fail Rate, Table 1). These behavioral observations are supported by previous studies that have shown that naked mole-rats are highly motivated to remove any blockages in their tunnel systems (Brett, 1991).
Based on the results of the non-continuous maze, we investigated motivators that would work well paired with the coinciding desires to bite and dig in a tunnel setting. Typically, spatial mazes rely on food rewards to motivate animals to complete the maze (Olton and Samuelson, 1976; Brown and Cook, 1986; du Toit et al., 2012). However, the colony structure of naked mole-rats makes it difficult to food deprive animals without permanently isolating them from their colony, and this in turn is likely to adversely affect behavioral performance. Thus, it was necessary to devise alternative methods of motivation. Naked mole-rats have been classified as poikilotherms—poor regulators of their own body temperature (Yahav and Buffenstein, 1992), but this varies with their activity state and thus they may be more accurately described as heterothermic (Kovalzon et al., 2020, 2021). Therefore, a heat lamp was added to the reward chamber as a motivator (Table 2 and Figure 3). As burrowing rodents, the animals were quick to enter the reward chamber when bedding was present and they immediately began to dig in it and to kick it around the chamber. With both home and foreign colony bedding proving to be effective motivators (Table 2 and Figure 3), we incorporated a combination of home colony bedding and a heat lamp in the reward chamber for subsequent maze testing, supporting previous research showing the desirability of home bedding (O’Riain and Jarvis, 1997; Deacon, 2012; Toor et al., 2015). Foreign colony bedding—though effective at increasing time spent in the reward chamber and decreasing latency to the reward chamber—was determined to be suboptimal and was not used in subsequent maze testing. This decision was based on the territorial nature of naked mole-rats (O’Riain and Jarvis, 1997; Braude et al., 2021) and the risk of an experimental animal being attacked upon reintroduction to the home colony due to smelling like an intruder.
In our re-designed, continuous version of a Hebb-Williams maze, there were no dead-ends that the naked mole-rats could have interpreted as a blockage in their tunnel systems (Figure 1B), resulting in a reduced fail rate (Table 1). In contrast to the non-continuous maze (Figure 4A), learning curves were evident in the continuous maze (Figure 5A). When using a repeated measures MANOVA, both latency and distance across learning trials displayed significant learning curves across trials and days (Figures 5A,B). Thus, the current study demonstrated spatial learning in naked mole-rats using a customized, continuous maze design, with latency to the reward chamber and distance traveled as measurements of learning. Smaller animals demonstrated faster learning (Supplementary Tables 1, 2). There was no multivariate effect of Day by Trial by Age. The overall effects of age on performance may be diminished due to the comparatively small age range tested in the current study (approximately 7 years) compared to the over 30 year life span of captive naked mole-rats (Buffenstein and Craft, 2021; Buffenstein et al., 2022). In a Day by Trial by Sex interaction, neither latency nor distance were significantly different between sexes. One possible explanation for the lack of effects of sex on spatial learning and memory is that these differences would be present in species with sex differences in home ranges (Costanzo et al., 2009), of which our naked mole-rats do not exhibit due to shared burrow systems for the males and females. There was no significant effect of dominance ranking (Supplementary Tables 1, 2) despite previous publications showing an effect of social hierarchy on spatial memory (Costanzo et al., 2009).
Using repeated measures MANOVA analyses to analyze memory retention of spatial learning gains, significant memory performance declines were not evident. This indicates good memory retention for at least 1 month (Table 3 and Figure 7), however, it is important to note that the motivators were present in the reward chamber during memory probe trials and the animals could have been following sensory cues rather than explicitly utilizing spatial memory. These results are supported by previous studies showing that other species of mole-rats didn’t experience decreases in spatial memory performance/retention until 1 month following training – outperforming more distant relatives within Rodentia, the rat and the prairie vole (Kimchi and Terkel, 2001).
Conclusion and Future Directions
The results of this study provide novel insight into the considerations that need to be taken when establishing behavioral tests for atypical animal models. In conducting behavioral testing of members of Bathyergidae, it is important to create tasks suited to their evolutionary adaptations. This includes modifying traditional maze setups to account for sensory capabilities and subterranean specializations. In addition, atypical motivators (e.g., home bedding) and removal of competing and confounding motivators (e.g., tunnel dead-ends) may need to be incorporated in order to optimize a species’ performance in a behavioral task and to elicit a true representation of the species’ spatial learning and memory capabilities. For naked mole-rats specifically, the use of a heat lamp paired with home bedding in the reward chamber effectively replaced typical motivators (food or liquid rewards following food deprivation) which are not ideal for animals within a social colony structure where isolation could prove detrimental. Because H. glaber has many behavioral and anatomical distinctions from other bathyergids, the methods used in the current study would likely require certain modifications in order to optimize spatial learning and memory testing for other members of Bathyergidae.
An apparent floor effect was evident in the steep learning curve associated with continuous maze testing in naked mole-rats, but latency and distance proved to be effective measures of spatial learning (Figure 5). In addition, the acquired spatial learning was retained for at least 1 month (Figure 7). In future studies, the continuous maze design that we developed would benefit from increased complexity in order to better test the extent of spatial learning and memory. Further time points (e.g., 2 months, 6 months, 1 year) and comparing memory probe trial performance with and without motivators present in the reward chamber may help to elucidate the true extent of spatial memory retention in naked mole-rats, in addition to determining factors that may affect memory loss.
This study provides a basis for future behavioral tests to be developed to aid translational research such as the use of naked mole-rats in neurodegenerative research (Markey et al., 2015) or examination of spatial learning and memory impairments following tooth loss (Okamoto et al., 2010; Chen et al., 2018; Lin et al., 2020), in addition to basic science research focused on evolutionary adaptations specific to burrowing rodents (Edrey et al., 2011; Smith et al., 2015; Grimes et al., 2017). Given the unique adaptations of sensory systems in bathyergids, future investigation of their behavioral use of specialized senses such as magnetic orientation (Oliveriusová et al., 2012) could yield valuable information about how these systems contribute to learning and memory performance.
Data Availability Statement
The raw data supporting the conclusions of this article will be made available by the authors, without undue reservation.
Ethics Statement
The animal study was reviewed and approved by the Institutional Animal Care and Use Committee at Southern Illinois University, Carbondale, IL, United States.
Author Contributions
NH and DS contributed to the experimental design. NH and LA collected data for the study. NH and KS analyzed the data. NH, KS, and DS interpreted the data. NH prepared the manuscript figures. NH and DS drafted the manuscript with revisions made by NH, LA, KS, and DS. All authors contributed to the article and approved the submitted version.
Conflict of Interest
The authors declare that the research was conducted in the absence of any commercial or financial relationships that could be construed as a potential conflict of interest.
Publisher’s Note
All claims expressed in this article are solely those of the authors and do not necessarily represent those of their affiliated organizations, or those of the publisher, the editors and the reviewers. Any product that may be evaluated in this article, or claim that may be made by its manufacturer, is not guaranteed or endorsed by the publisher.
Acknowledgments
We would like to thank Gregory Rose for his invaluable input to the design of the study and the reviewers for their helpful feedback which greatly improved this manuscript.
Supplementary Material
The Supplementary Material for this article can be found online at: https://www.frontiersin.org/articles/10.3389/fevo.2022.879989/full#supplementary-material
References
Antinuchi, C. D., and Schleich, C. E. (2003). ‘Spatial memory in a solitary subterranean rodent Ctenomys talarum (rodentia: ctenomyidae)’. Bel. J. Zool. 133, 89–91.
Artwohl, J., Hill, T., Comer, C., and Park, T. (2002). ‘Naked mole-rats: unique opportunities and husbandry challenges.’. Lab Animal 31:32. doi: 10.1038/5000156
Barone, C. M., Douma, S., Reijntjes, D. O. J., Browe, B. M., Köppl, C., Klump, G., et al. (2019). ‘Altered cochlear innervation in developing and mature naked and damaraland mole rats’. J. Comparat. Neurol. 527, 2302–2316. doi: 10.1002/cne.24682
Bennett, N. C., and Jarvis, J. U. M. (2004). ‘Cryptomys damarensis’. Mammal. Species 756:1. doi: 10.1644/756
Bennett, N. C., Faulkes, C. G., Hart, L., and Jarvis, J. U. M. (2009). ‘Bathyergus suillus (rodentia: bathyergidae)’. Mammal. Oxford Univ. 828, 1–7. doi: 10.1644/828.1
Brachetta, V., Schleich, C. E., and Zenuto, R. R. (2014). ‘Effects of acute and chronic exposure to predatory cues on spatial learning capabilities in the subterranean rodent Ctenomys talarum (rodentia: ctenomyidae)’. Ethology 120, 563–576. doi: 10.1111/eth.12230
Braude, S., Hess, J., and Ingram, C. (2021). ‘Inter-colony invasion between wild naked mole-rat colonies’. J. Zool. 313, 37–42. doi: 10.1111/jzo.12834
Brett, R. A. (1991). “The ecology of naked mole-rat colonies: burrowing, food and limiting factors,” in The Biology of the Naked Mole-Rat, eds P. W. Sherman, J. U. M. Jarvis, and R. D. Alexander (New Jersey: Princeton University Press), 137–184.
Brown, M. F., and Cook, R. G. (1986). ‘Within-trial dynamics of radial arm maze performance in rats’. Learn. Motivat. 17, 190–205. doi: 10.1016/0023-9690(86)90010-X
Buffenstein, R., Amoroso, V., Andziak, B., Avdieiev, S., Azpurua, J., Barker, A. J., et al. (2022). ‘The naked truth: a comprehensive clarification and classification of current “myths” in naked mole-rat biology’. Biol. Rev. 97, 115–140. doi: 10.1111/brv.12791
Buffenstein, R., and Craft, W. (2021). ‘The idiosyncratic physiological traits of the naked mole-rat; a resilient animal model of aging, longevity, and healthspan’. Adv. Exp. Med. Biol. United States 1319, 221–254. doi: 10.1007/978-3-030-65943-1_8
Chen, J., Ren, C. J., Wu, L., Xia, L. Y., Shao, J., and Leng, W. D. (2018). ‘Tooth loss is associated with increased risk of dementia and with a dose-response relationship’. Front. Aging Neurosci. 10:1–9. doi: 10.3389/fnagi.2018.00415
Clarke, F. M., and Faulkes, C. G. (1997). ‘Dominance and queen succession in captive colonies of the eusocial naked mole-rat, heterocephalus glaber’. Proc. R. Soc. Biol. Sci. 264, 993–1000. doi: 10.1098/rspb.1997.0137
Clarke, F. M., and Faulkes, C. G. (1998). Hormonal and behavioural correlates of male dominance and reproductive status in captive colonies of the naked mole-rat, Heterocephalus glaber. Biol. Sci. 37, 1391–1399. doi: 10.1098/rspb.1998.0447
Costanzo, M. S., Bennett, N. C., and Lutermann, H. (2009). ‘Spatial learning and memory in african mole-rats: the role of sociality and sex’. Physiol. Behav. 96, 128–134. doi: 10.1016/j.physbeh.2008.09.008
Crish, S. D., Dengler-Crish, C. M., and Catania, K. C. (2006). ‘Central visual system of the naked mole-rat (Heterocephalus glaber)’. Anat. Rec. Part A Dis. Mol. Cell. Evol. Biol. 288, 205–212. doi: 10.1002/ar.a.20288
Crish, S. D., Rice, F. L., Park, T. J., and Comer, C. M. (2003). ‘Somatosensory organization and behavior in naked mole-rats I: vibrissa-like body hairs comprise a sensory array that mediates orientation to tactile stimuli’. Brain Behav. Evolu. 62, 141–151. doi: 10.1159/000072723
Deacon, R. (2012). ‘Assessing burrowing, nest construction, and hoarding in mice’. J. Visu. Exp. 59, 1–10. doi: 10.3791/2607
du Toit, L., Bennett, N. C., Nickless, A., and Whiting, M. J. (2012). ‘Influence of spatial environment on maze learning in an African mole-rat’. Animal Cogn. 15, 797–806. doi: 10.1007/s10071-012-0503-0
Edrey, Y. H., Park, T. J., Kang, H., Biney, A., and Buffenstein, R. (2011). ‘Endocrine function and neurobiology of the longest-living rodent, the naked mole-rat’. Exp. Geront. Elsevier 46, 116–123. doi: 10.1016/j.exger.2010.09.005
Faulkes, C. G., and Bennett, N. C. (2021). ‘Social evolution in african mole-rats − a comparative overview.’. Adv. Exp. Med. Biol. United States 1319, 1–33. doi: 10.1007/978-3-030-65943-1_1
Gilbert, J. D., Rossiter, S. J., and Faulkes, C. G. (2020). ‘The relationship between individual phenotype and the division of labour in naked mole-rats: it’s complicated’. PeerJ 8:e9891. doi: 10.7717/peerj.9891
Grimes, K. M., Barefield, D. Y., Kumar, M., McNamara, J. W., Weintraub, S. T., de Tombe, P. P., et al. (2017). ‘The naked mole-rat exhibits an unusual cardiac myofilament protein profile providing new insights into heart function of this naturally subterranean rodent’. Pflugers Arch. Eur. J. Physiol. Pflügers Arch. Eur. J. Physiol. 469, 1603–1613. doi: 10.1007/s00424-017-2046-3
Heffner, R. S., and Heffner, H. E. (1993). ‘Degenerate hearing and sound localization in naked mole rats (Heterocephalus glaber), with an overview of central auditory structures’. J. Comparat. Neurol. 331, 418–433. doi: 10.1002/cne.903310311
Henry, E. C., Remple, M. S., O’Riain, M. J., and Catania, K. C. (2006). ‘Organization of somatosensory cortical areas in the naked mole-rat (Heterocephalus glaber)’. J. Comparat. Neurol. 495, 434–452. doi: 10.1002/cne.20883
Hetling, J. R., Baig-Silva, M. S., Comer, C. M., Pardue, M. T., Samaan, D. Y., Qtaishat, N. M., et al. (2005). ‘Features of visual function in the naked mole-rat Heterocephalus glaber’. J. Comparat. Physiol. Neuroethol. Sen. Neural Behav. Physiol. 191, 317–330. doi: 10.1007/s00359-004-0584-6
Jarvis, J. U. M., and Bennett, N. C. (2017). “Ecology and behavior of the family bathyergidae,” in The Biology of the Naked Mole-Rat, eds P. W. Sherman, J. U. M. Jarvis, and R. D. Alexander (Princeton University Press), 66–96. doi: 10.1515/9781400887132-006
Kimchi, T., and Terkel, J. (2001). ‘Spatial learning and memory in the blind mole-rat in comparison with the laboratory rat and Levant vole’. Animal Behav. 61, 171–180. doi: 10.1006/anbe.2000.1565
Kimchi, T., and Terkel, J. (2004). ‘Comparison of the role of somatosensory stimuli in maze learning in a blind subterranean rodent and a sighted surface-dwelling rodent’. Behav. Brain Res. 153, 389–395. doi: 10.1016/j.bbr.2003.12.015
Kovalzon, V. M., Averina, O. A., and Vysokikh, M. Y. (2021). ‘Motor activity and “neotenic” sleep in the naked mole rat (Heterocephalus glaber) under isolation’. Doklady Biol. Sci. 496, 25–29. doi: 10.1134/S0012496621010063
Kovalzon, V. M., Averina, O. A., Minkov, V. A., Petrin, A. A., and Yu, M. (2020). Unusual correlation between rest–activity and body temperature rhythms in the naked mole rat (Heterocephalus glaber) as compared to five other mammalian species. J. Evol. Biochem. Physiol. 56, 451–458. doi: 10.1134/s0022093020050087
Lin, C. S., Lin, H. H., Fann, S. W., Lee, W. J., Hsu, M. L., Wang, S. J., et al. (2020). ‘Association between tooth loss and gray matter volume in cognitive impairment’. Brain Imag. Behav. 14, 396–407. doi: 10.1007/s11682-020-00267-w
Markey, P. M., Medina, D. X., Gaczynska, M., Osmulski, P. A., Oddo, S., Caccamo, A., et al. (2015). ‘Amyloid beta and the longest-lived rodent: the naked mole-rat as a model for natural protection from Alzheimer’s disease’. Neurobiol. Aging 6, 300–308. doi: 10.1016/j.neurobiolaging.2013.03.032.Amyloid
Mason, M. J., Cornwall, H. L., and Smith, E. S. J. (2016). ‘Ear structures of the naked mole-rat, Heterocephalus glaber, and its relatives (rodentia: bathyergidae)’. PLoS One 11:e0167079. doi: 10.1371/journal.pone.0167079
Morris, R. (1984). ‘Developments of a water-maze procedure for studying spatial learning in the rat’. J. Neurosci. Methods 11, 47–60. doi: 10.1016/0165-0270(84)90007-4
Neumann, C., and Kulik, L. (2020). ‘Animal Dominance Hierarchies by Elo Rating’, R Package Version 0.46.
O’Riain, M. J., and Jarvis, J. U. M. (1997). ‘Colony member recognition and xenophobia in the naked mole-rat’. Animal Behav. 53, 487–498. doi: 10.1006/anbe.1996.0299
Okamoto, N., Morikawa, M., Okamoto, K., Habu, N., Iwamoto, J., Tomioka, K., et al. (2010). ‘Relationship of tooth loss to mild memory impairment and cognitive impairment: findings from the fujiwara-kyo study’. Behav. Brain Funct. 6, 1–8. doi: 10.1186/1744-9081-6-77
Okanoya, K., Yosida, S., Barone, C. M., Applegate, D. T., Brittan-Powell, E. F., Dooling, R. J., et al. (2018). ‘Auditory-vocal coupling in the naked mole-rat, a mammal with poor auditory thresholds’. J. Comparat. Physiol. Neuroethol. Sen. Neural Behav. Physiol. 204, 905–914. doi: 10.1007/s00359-018-1287-8
Oliveriusová, L., Němec, P., Králová, Z., and Sedláèek, F. (2012). ‘Magnetic compass orientation in two strictly subterranean rodents: learned or species-specific innate directional preference?’. J. Exp. Biol. 215, 3649–3654. doi: 10.1242/jeb.069625
Olton, D. S., and Samuelson, R. J. (1976). ‘Remembrance of places passed: spatial memory in rats’. J. Exp. Psychol. Animal Behav. Proc. 2, 97–116. doi: 10.1037/0097-7403.2.2.97
Oosthuizen, M. K. (2020). Exploratory behaviour, memory and neurogenesis in the social damaraland mole-rat (Fukomys damarensis). J. Exp. Biol. 223:1093. doi: 10.1242/jeb.221093
Oosthuizen, M. K., Scheibler, A. G., Bennett, N. C., and Amrein, I. (2013). ‘Effects of laboratory housing on exploratory behaviour, novelty discrimination and spatial reference memory in a subterranean, solitary rodent, the cape mole-rat (Georychus capensis)’. PLoS One 8:1–8. doi: 10.1371/journal.pone.0075863
R Core Team (2021). R: A Language and Environment for Statistical Computing. Vienna: R Foundation for Statistical Computing.
Rüppell, E. (1842). ‘Säugethiere aus der ordnung der nager, beobachtet im nordöstlichen Africa’. Museum Senckenbergianum: Abhandlungen Aus Dem Gebiete der Beschreibenden Naturgeschichte 3, 99–101.
Schleich, C. E. (2010). ‘Ontogeny of spatial working memory in the subterranean rodent Ctenomys talarum’. Dev. Psychobiol. 52, 592–597. doi: 10.1002/dev.20466
Sherman, P. W., Jarvis, J. U. M., and Alexander, R. D. (1991). The Biology of the Naked Mole-Rat. Princeton, N.J: Princeton University Press.
Smith, E. S. J., Schuhmacher, L.-N., and Husson, Z. (2015). The naked mole-rat as an animal model in biomedical research: current perspectives. Open Access Animal Physiol. 137:50376. doi: 10.2147/oaap.s50376
Smith, M., and Buffenstein, R. (2021). “Managed care of naked mole-rats BT,” in The Extraordinary Biology of the Naked Mole-Rat, eds R. Buffenstein, T. J. Park, and M. M. Holmes (Cham: Springer International Publishing), 381–407. doi: 10.1007/978-3-030-65943-1_16
Toor, I., Clement, D., Carlson, E. N., and Holmes, M. M. (2015). ‘Olfaction and social cognition in eusocial naked mole-rats. Heterocephalus Glaber’, Animal Behav. 107, 175–181. doi: 10.1016/j.anbehav.2015.06.015
Vallianatou, C. A., Alonso, A., Aleman, A., and Genzel, L. (2020). Schema-induced shifts in mice navigational strategies are unveiled by a minimal behavioral model of spatial exploration. bioRxiv [preprint]. doi: 10.1101/2020.12.21.423808
Walcher, J., Ojeda-Alonso, J., Haseleu, J., Oosthuizen, M. K., Rowe, A. H., Bennett, N. C., et al. (2018). ‘Specialized mechanoreceptor systems in rodent glaborous skin’. J. Physiol. 596, 4995–5016.
Wilson, E. O. (1971). The Insect Societies. Cambridge, MA: Belknap Press of Harvard University Press.
Keywords: naked mole-rat, learning, memory, spatial navigation, rodent, reward
Citation: Hite NJ, Sudheimer KD, Anderson L and Sarko DK (2022) Spatial Learning and Memory in the Naked Mole-Rat: Evolutionary Adaptations to a Subterranean Niche. Front. Ecol. Evol. 10:879989. doi: 10.3389/fevo.2022.879989
Received: 20 February 2022; Accepted: 18 May 2022;
Published: 16 June 2022.
Edited by:
Stan Braude, Washington University in St. Louis, United StatesReviewed by:
Melissa Holmes, University of Toronto Mississauga, CanadaVladimir M. Kovalzon, Severtsov Institute of Ecology and Evolution (RAS), Russia
Markus Zöttl, Linnaeus University, Sweden
Copyright © 2022 Hite, Sudheimer, Anderson and Sarko. This is an open-access article distributed under the terms of the Creative Commons Attribution License (CC BY). The use, distribution or reproduction in other forums is permitted, provided the original author(s) and the copyright owner(s) are credited and that the original publication in this journal is cited, in accordance with accepted academic practice. No use, distribution or reproduction is permitted which does not comply with these terms.
*Correspondence: Natalee J. Hite, bmhpdGU0M0BzaXVtZWQuZWR1