- 1Department of Physiology, University of Southern Illinois Carbondale, Carbondale, IL, United States
- 2Department of Physiology, University of Southern Illinois School of Medicine, Carbondale, IL, United States
Treatment options for chronically aggressive individuals remain limited despite recent medical advances. Traditional pharmacological agents used to treat aggression, such as atypical antipsychotics, have limited efficacy and are often replete with dangerous side effects. The non-competitive NMDAR antagonists ketamine and memantine are promising alternatives, but their effects appear to be highly dependent on dosage, context, and personal experience. Importantly, these drugs can increase aggression when combined with substances of abuse or during periods of heightened stress. This is likely due to mechanistic differences operating at specific synapses under different contexts. Previous findings from our lab and others have shown that early life stress, substance abuse, and attack experience promote aggression through NMDAR-dependent synaptic plasticity within aggression-related brain circuits. Ketamine and memantine affect these types of aggression in opposite ways. This has led us to propose that ketamine and memantine oppositely affect aggression brought on by early life stress, substance abuse, or attack experience through opposite effects on NMDAR-dependent synaptic plasticity. This would account for the persistent effects of these drugs on aggression and suggest they could be leveraged as a more long-lasting treatment option. However, a more thorough examination of the effects of ketamine and memantine on cellular and synaptic function will be necessary for responsible administration. Additionally, because the effects of ketamine and memantine are highly dependent on prior drug use, traumatic stress, or a history of aggressive behavior, we propose a more thorough medical evaluation and psychiatric assessment will be necessary to avoid possible adverse interactions with these drugs.
Introduction
Recurring violent aggression is a major societal concern with few effective treatment options. Currently, the standard of treatment for aggression is antipsychotics, benzodiazepines, or a combination of the two. Unfortunately, these drugs induce numerous side effects, including loss of appetite, fatigue, sleep disturbances, nausea, vomiting, diarrhea, weight gain, increased risk of respiratory depression, and oxygen desaturation (Delbello et al., 2006; Haas et al., 2009; Redden et al., 2009; Geller et al., 2012; Pandina et al., 2012; Pringsheim et al., 2015; Amerio et al., 2018; Pisano et al., 2019; Solmi et al., 2020). In addition, many patients will need to take these drugs for life, increasing their dosage with time as tolerance develops. As a result, current research is devoted to finding a more effective, quick-acting, long-lasting, and well-tolerated alternative.
N-methyl-d-aspartate receptor (NMDAR) antagonists are a promising pharmacological alternative for treating aggressive behavior and aggression-related disorders. NMDARs are members of the ionotropic glutamate binding receptor family (Willard and Koochekpour, 2013). They are composed of five non-identical subunits (GluN1, GluN2A-D, and GluN3A-B) that form a central pore through which cations are conducted upon the binding of glutamate. NMDARs are unique among the ionotropic glutamate binding receptors because of their voltage-gated properties, coincidence detection, and role in synaptic plasticity (Squire and Kandel, 2009; Willard and Koochekpour, 2013). Antagonists for these receptors have been shown to interfere with these properties.
We propose in this review that NMDAR antagonists are favorable in treating aggression over traditional methods due to their quicker onset of action, fewer observed side effects, and potential as a long-lasting treatment option due to their effects on synaptic plasticity (Roberts and Geeting, 2001; Cummings et al., 2008; Wilcock et al., 2008; Hopper et al., 2015; Cole et al., 2016; Riddell et al., 2017; Tran and Mierzwinski-Urban, 2019; Barbic et al., 2021; Nordman et al., 2022). Though there are multiple clinically available NMDAR antagonists, such as the non-competitive antagonists dextromethorphan and amantadine (Hewitt, 2000) and the non-selective, NMDAR-binding opioids methadone, dextropropoxyphene, and ketobemidone (Sang, 2000), we will focus on the non-competitive antagonists ketamine and memantine, as they are the most widely used and successful NMDAR antagonists on the market.
Ketamine and Memantine in Treating Human Aggression
Ketamine is a non-competitive NMDAR antagonist that has been successfully used to treat aggression in humans. Ketamine was first developed in the United States in the 1960s as an alternative anesthetic to phencyclidine (PCP; Domino et al., 1965). It was found to have a quicker onset of action (less than 5 min) and induce fewer negative emergence symptoms than PCP (Dillon et al., 2003; Tran and Mierzwinski-Urban, 2019), though both produce psychotic-like symptoms in schizophrenia patients (Lahti et al., 2001; Beck et al., 2020). Ketamine gained popularity as a party drug in the 1980s, with sub-anesthetic doses sending users into the colloquially named “K-hole”—a dissociative state commonly accompanied by out-of-body experiences, a sensation of weightlessness, and distortions of time (Dillon et al., 2003). The FDA has approved ketamine for the induction and maintenance of general anesthesia, but there are also many off-label uses of ketamine, including as a local anesthetic, in procedural sedation, pain management, asthma, and depression (Papolos et al., 2013; Burger et al., 2016; Dwyer et al., 2017; Cullen et al., 2018; Tran and Mierzwinski-Urban, 2019; Zarrinnegar et al., 2019; Barbic et al., 2021; Kim et al., 2021; Solano et al., 2021).
Many studies support the use of ketamine to treat violent aggression, agitation, psychosis, self-harm, and suicidal ideation (Burger et al., 2016; Dwyer et al., 2017; Cullen et al., 2018; Zarrinnegar et al., 2019). In the majority of these cases, clinicians take advantage of ketamine’s sedative properties to control aggressive outbursts in hospital and pre-hospital settings (Roberts and Geeting, 2001; Melamed et al., 2007; Le Cong et al., 2012; Scheppke et al., 2014; Riddell et al., 2017; Barbic et al., 2021; Kent et al., 2022). Sedative doses of ketamine fall in the 1–2 mg/kg range if given intravenously, and 3–5 mg/kg if given intramuscularly. These doses are necessary to sedate acutely aggressive individuals for the safety of the patient and staff but are also associated with higher rates of intubation, emergence delirium, and other adverse effects (Woods and Almvik, 2002; Cole et al., 2016; Chang et al., 2019). Because of these risks, a low-dose treatment protocol should be followed when possible.
Sub-sedative doses of ketamine (0.2–0.5 mg/kg IV, 30–120 mg intranasal) have been successful in treating aggression in psychiatric patients (Papolos et al., 2013; Burger et al., 2016; Dwyer et al., 2017; Zarrinnegar et al., 2019). For instance, intranasal ketamine significantly reduced aggression in children and adolescents with pediatric bipolar disorder (Papolos et al., 2013). Additionally, military patients presenting to the hospital with depression and suicidal ideations experienced a rapid improvement of symptoms within 40 min of administration of ketamine (Burger et al., 2016). Numerous studies support ketamine’s rapid-acting antidepressant properties when given at low doses (Papolos et al., 2013; Burger et al., 2016; Dwyer et al., 2017; Zarrinnegar et al., 2019). Interestingly, chronic ketamine use has been found to be more addictive at these sub-sedative doses (Morgan et al., 2004; Bonnet, 2015; Schak et al., 2016). Therefore, ketamine treatment for aggression symptoms related to psychiatric disease must be carried out in a regulated, controlled fashion. These studies are discussed in the Synaptic Plasticity section below. A summary of our review findings on ketamine in aggression is outlined in Table 1.
Memantine is a non-competitive NMDAR antagonist, with low to moderate affinity, which is approved for treating moderate to severe Alzheimer’s disease and dementia (Huey et al., 2005; Robinson and Keating, 2006; Thomas and Grossberg, 2009). It has been reported that 5%–10% of Alzheimer’s patients and 96% of dementia patients exhibit aggressive behavior over the course of their illness (Keene et al., 1999; Jaclson and Mallory, 2009). Atypical antipsychotics are among the most common treatments for agitation and aggression in Alzheimer’s and dementia patients (Ballard et al., 2006, 2008; Cerejeira et al., 2012), but there is a significant risk of mortality, stroke, hallucination, and relapse after continued use, especially during the first 30 days of treatment (Kales et al., 2012; Calsolaro et al., 2019).
A key benefit of memantine is that, due to its long biological half-life, it is not used recreationally, is not habit-forming, and is well tolerated. Notably, memantine at a dose of 10–20 mg/day has been effective in reducing aggression and agitation associated with Alzheimer’s disease and dementia patients without the accompanying risks of atypical antipsychotics (Cummings et al., 2008; Wilcock et al., 2008). However, some patients experience treatment-induced agitation with memantine use (Da Re et al., 2015), suggesting that a more careful clinical examination is key to proper treatment. A summary of our review findings on memantine in aggression is outlined in Table 1.
The future of ketamine and memantine is promising. Still, our limited knowledge of the mechanisms behind NMDARs and aggression, paired with the diverging effects observed in animal models warrants more research, which we discuss below.
NMDARs, Ketamine, and Memantine in Animal Aggression
NMDARs are highly expressed in regions associated with attack behavior, such as the amygdala, prefrontal cortex (PFC), hippocampus, nucleus accumbens, hypothalamus, striatum, and brain stem (Shaikh and Siegel, 1994; Shaikh et al., 1994; Petralia et al., 2007; Peregud et al., 2012; Takahashi et al., 2015; Bacq et al., 2018; Chen and Hong, 2018; Newman et al., 2018; Zoicas and Kornhuber, 2019; Falkner et al., 2020; Figure 1A). The NMDARs, particularly the GluN2 subunits, have a nuanced and varied role in species-typical and excessive aggression, as demonstrated by rodent studies. For example, decreased GluN2B expression in the lateral amygdala is associated with naturally occurring and social isolation-induced aggression (Bacq et al., 2018). However, increased GluN2A and GluN2B expression and GluN2B-dependent NMDAR currents in the hippocampus and frontal cortex are associated with social isolation-induced aggression and morphine-induced aggression, respectively (Meyer et al., 2004; Zhao et al., 2009; Chang et al., 2015; Chang and Gean, 2019). Interestingly, in the mPFC, increases in GluN2D, but not GluN2A or GluN2B, may mediate alcohol-induced aggression (Newman et al., 2018).
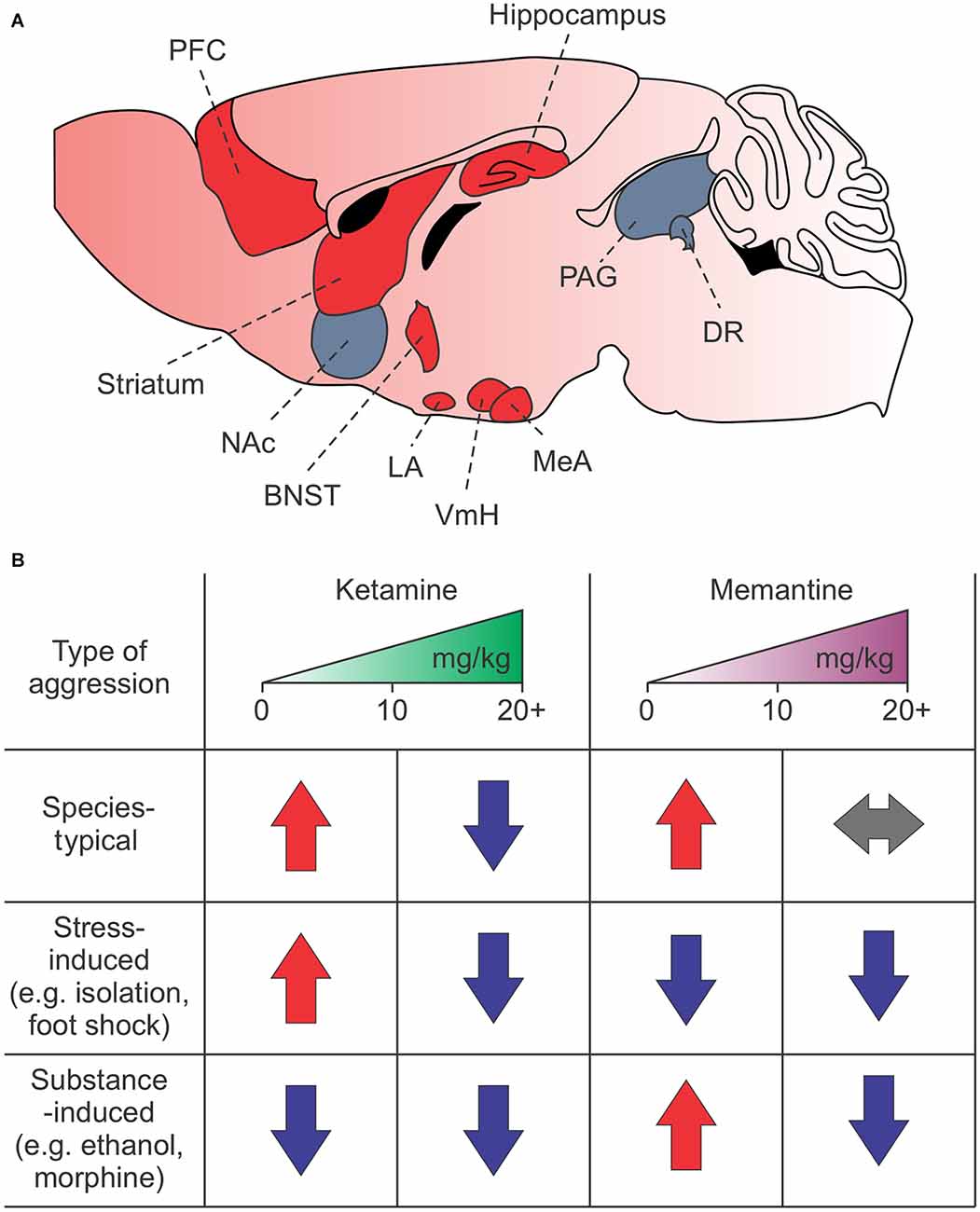
Figure 1. Summary of NMDAR-dependent aggression in mice. (A) Brain regions associated with attack behavior are enriched in and regulated by NMDARs. Red regions indicate known areas involved in NMDAR-dependent synaptic plasticity-induced aggression. (B) Effects of low or high doses of ketamine and memantine on species-typical, stress-induced, and drug or alcohol-induced aggression. Red arrows—increased aggression; blue arrows—decreased aggression; gray arrows—no change in aggression. The PFC, prefrontal cortex; NAc, nucleus accumbens; BNST, bed nucleus of the stria terminalis; LA, lateral amygdala; VmH, ventromedial hypothalamus; MeA, medial amygdala; PAG, periacqeductal gray; DR, dorsal raphe.
Unsurprisingly, ketamine and memantine also have a nuanced role in aggression that depends on dosage, context, experience, and species (Table 2). For example, in mice and zebrafish, high doses of ketamine suppress territorial aggression while low doses increase it (Newman et al., 2012; Michelotti et al., 2018). In rats, low doses, but not high doses, of ketamine suppress muricide behavior (Takahashi et al., 1984). Memantine, by contrast, has no effect on species-typical aggression (Sukhotina and Bespalov, 2000; Newman et al., 2012, 2018).
As with humans, substance use plays a significant role in the effects of ketamine and memantine on aggression. In alcohol-challenged mice, ketamine reduces territorial aggression at high doses and reduces motivated aggression at low doses (Newman et al., 2012; Covington et al., 2018). Memantine increases alcohol-heightened aggression at low doses but has no effect at high doses (Newman et al., 2012, 2018). By contrast, high doses of memantine, but not low doses, decrease morphine withdrawal-facilitated aggressive behavior in mice (Sukhotina and Bespalov, 2000).
Stress also appears to be a strong factor. For example, ketamine increases aggression in sleep-deprived rats and socially isolated mice (Takahashi et al., 1984). In adolescent mice, ketamine decreases aggression induced by neonatal maternal separation (Shin et al., 2019) but increases aggression induced by chronic social isolation followed by acute non-contingent foot shock (Nordman et al., 2022). Interestingly, memantine suppresses aggression induced by chronic social isolation stress followed by acute non-contingent foot shock, similar to what has been seen with the non-competitive NMDAR antagonist MK-801 (Chang et al., 2015; Nordman et al., 2022).
While these findings clearly demonstrate a role for NMDARs in aggression and NMDAR antagonists as a potential treatment option, dosage, context, experience, and brain region all need to be considered (Figure 1B). Furthermore, the effects of ketamine and memantine suggest they operate through distinct mechanisms, which we discuss in the “Mechanistic Differences” section below.
Synaptic Plasticity
NMDARs are one of the primary sources of synaptic plasticity in the brain (Squire and Kandel, 2009; Willard and Koochekpour, 2013). When bound by glycine and glutamate at certain voltage thresholds, NMDARs conduct large amounts of the second messenger cation calcium into the synapse. This sudden, large increase in calcium concentration activates a series of kinases and phosphatases that promote the insertion or removal of another type of ionotropic glutamate binding receptor, the AMPA receptor. AMPA receptors are not voltage-gated, so the more AMPA receptors there are in the synapse, the more the membrane will be depolarized when AMPARs are bound by glutamate, thus increasing the likelihood that a neuron will fire an action potential. Conversely, the fewer AMPA receptors there are in the synapse, the less the membrane will be depolarized when AMPARs are bound by glutamate, thus decreasing the likelihood that a neuron will fire an action potential when activated. Insertion and removal of AMPA receptors by NMDARs is the principal mechanism for synaptic plasticity at excitatory synapses in the brain.
Excessive chronic aggression brought on by early life stress and social isolation is mediated by the persistent effects of NMDAR-dependent synaptic plasticity, which can be suppressed using NMDAR antagonists (Chang et al., 2015, 2018; Nordman et al., 2020a, b). Therefore, NMDAR antagonists possess a feature more popular mood-stabilizing and aggression-suppressing drugs lack: they can inhibit certain persistent forms of maladaptive social behaviors such as excessive and recurring aggression from ever forming, likely through their effects on synaptic plasticity. This is best seen in animal models, where a single dose can inhibit depression-like, anxiety-like, or aggressive behavior when administered before a potentiating event (da Silva et al., 2010; Ma et al., 2013; Yang et al., 2016; Chang et al., 2018; Nordman et al., 2020a, b) or reverse maladaptive behaviors when administered after the potentiating event (Maeng et al., 2008; Moda-Sava et al., 2019).
In a recent study, we showed that NMDAR antagonism could alter aggression induced by early life stress after a single dose (Nordman et al., 2022). Our early life stress paradigm involves social isolation during early adolescence followed by acute physical stress in the form of non-contingent foot shock during late adolescence. Combining these stressors produces prolonged increases in excessive aggression when measured seven days later. When we systemically injected a single dose of the non-competitive NMDAR antagonists MK-801 or memantine immediately before foot shock in our early life-stress paradigm, no increase in aggression was found when measured 7 days later. However, when we administered a single intraperitoneal (IP) injection of ketamine before foot shock, surprisingly, we observed a significant increase in aggression when measured 7 days later. These results indicate that different NMDAR antagonists have distinct effects on early life stress-induced aggression and suggest that memantine and ketamine are mechanistically distinct, possibly through their opposing effects on synaptic plasticity.
In support of this, in another recent study, we showed that NMDARs regulate aggression priming through NMDAR-dependent synaptic plasticity (Nordman et al., 2020a). Attack priming is a phenomenon where previous attack experience increases the likelihood and severity of a future attack within a narrow time window (~30–60 min). Notably, in that study, we found that attack experience, high-frequency optogenetic stimulation of aggression brain pathways, and early life stress heightened aggression by synaptically potentiating glutamatergic synapses within the aggression circuit. Specifically, in vivo electrophysiological recordings of optically evoked excitatory postsynaptic potentials indicate that both attack experience and acute footshock after social isolation potentiate glutamatergic synapses between the posterior ventral segment of the medial amygdala (MeApv) and its downstream synaptic partners the ventrolateral aspect of the ventromedial hypothalamus (MeApv-VmHvl) and the medial aspect of the bed nucleus of the stria terminalis (MeApv-BNSTm; Nordman et al., 2020a, b). A single systemic injection of the non-competitive NMDAR antagonist MK-801 30 min before aggression testing suppressed both aggression priming and synaptic potentiation at these synapses, indicating that both are NMDAR-dependent.
Since (1) aggression priming and early life stress-induced aggression both involve synaptic plasticity within the same glutamatergic MeApv pathways; and (2) aggression priming is NMDAR-dependent, it stands to reason that early life stress-induced aggression is NMDAR-dependent as well. However, the distinct effects of MK-801, memantine, and ketamine on aggression suggest these drugs have opposing effects on synaptic plasticity within MeApv pathways. Future studies should explore this possibility.
Nevertheless, NMDAR antagonists are an exciting treatment option for excessive aggression and aggression associated with early life stress because it bypasses one of the great challenges of traditional pharmacological methods: daily and lifelong administration of a dangerous drug that only diminishes in its efficacy over time. In support of this, it has been found that in some psychiatric patients, ketamine can suppress aggressive behavior long past the time it takes for the body to metabolize the drug (plasma half-life is 79 +/– 8 min; Hirota and Lambert, 1996; Donoghue et al., 2015; Dwyer et al., 2017; Zarrinnegar et al., 2019). For example, a single sedating dose (10 mg) of ketamine successfully suppressed aggressive behavior for 13 days in a 7-year-old child diagnosed with PTSD displaying reactive attachment disorder and disruptive behavior disorder (Donoghue et al., 2015). Three months later, the same sedating dose of ketamine reduced aggression and increased the patient’s receptivity to psychiatric care for another 8 days, showing that ketamine can be effectively readministered while retaining its persistent effects on aggression. Similarly, patients receiving ketamine treatment for depression showed significant improvements in aggression symptoms (e.g., psychosis, self-harm, and suicidality) that lasted for weeks to months afterward (Dwyer et al., 2017; Zarrinnegar et al., 2019). Synaptic plasticity could explain the vast difference between the time it takes to metabolize ketamine and the sustained decrease in aggressive symptoms seen in these patients.
Therefore, in this review, we argue that NMDAR antagonists like ketamine and memantine could reduce the frequency of taking, or even alleviate the patient’s need to remain on, increasingly tolerant and dangerous drugs like antipsychotics, benzodiazepines, lithium, or anticonvulsants for the treatment of aggression. In addition, we argue that NMDAR antagonists could be used to suppress aggression induced by a potentiating event like stress. Of course, it is difficult to predict when a potentiating event might occur. However, NMDAR antagonists could suppress the susceptibility of individuals with psychiatric diseases to develop aggressive behavior during periods of heightened stress or substance abuse, a significant advantage over current options. We note though that the distinct effects of ketamine and memantine on aggression associated with early life stress in our animal model suggest the need for great care when prescribing NMDAR drugs to treat excessive aggression.
Mechanistic Differences
We highlight ketamine and memantine in this review as they are the most common and successful NMDAR-drugs for treating excessive aggression in humans and are potent synaptic plasticity blockers. While both drugs are similar in their effects on channel function (Johnson et al., 2015), important differences remain. Perhaps the most important differences are the receptor types each drug targets.
For example, ketamine is non-selective for the NMDAR, binding to muscarinic, monoaminergic, and opioid receptors, among others (Hirota and Lambert, 1996). It has been hypothesized that these interactions mediate the psychotomimetic effects many patients experience and may account for the more persistent effects of ketamine on pain and as an antidepressant (Sleigh et al., 2014; Zorumski et al., 2016). This would suggest that the effects of ketamine on aggression-related disorders are not exclusively mediated through NMDARs.
Ketamine and memantine also differ in their NMDAR dissociation rates, with ketamine binding to the NMDAR for longer periods of time than memantine (Johnson et al., 2015; Glasgow et al., 2017). This has been used to explain ketamine’s high and memantine’s low sedative and psychotomimetic effects (Lanthorn et al., 2000; Bolshakov et al., 2003; Kotermanski and Johnson, 2009; Kitanaka et al., 2018).
The location of NMDARs may also explain the differences between these drugs. There is evidence that memantine binds more readily to extrasynaptic NMDARs than ketamine (Zhao et al., 2006; Leveille et al., 2008; Okamoto et al., 2009; Milnerwood et al., 2010; Johnson et al., 2015), though these results have been disputed (Wroge et al., 2012; Emnett et al., 2013; Zhou et al., 2013). Extrasynaptic NMDARs have been associated with mood and anxiety disorders related to chronic social defeat stress (Tse et al., 2019). Ketamine, however, likely does not distinguish between synaptic and extrasynaptic NMDARs (Autry et al., 2011; Emnett et al., 2013; Nosyreva et al., 2013; Gideons et al., 2014; Miller et al., 2014).
It is also possible that memantine and ketamine bind to overlapping but distinct NMDAR subpopulations. For example, it was recently shown that memantine and ketamine target different NMDAR subtypes, with memantine preferentially binding to and desensitizing the GluN1/2A subtype and ketamine binding to and desensitizing the GluN1/2B subtype (Glasgow et al., 2017).
Another possibility is that the enhanced effects of ketamine are due to the disinhibition of excitatory signaling in different areas of the brain. Ketamine has been shown to disinhibit pyramidal neurons in the medial prefrontal cortex (mPFC) and the CA1 region of the hippocampus by inhibiting GABAergic interneurons in both areas (Moghaddam et al., 1997; Widman and McMahon, 2018; Ali et al., 2020; Gerhard et al., 2020). This is hypothesized to occur because of ketamine’s higher affinity for GluN2B-containing NMDARs expressed in PFC somatostatin interneurons (Ali et al., 2020; Gerhard et al., 2020) and GluN2D-containing NMDARs preferentially expressed in CA1 hippocampal interneurons (Perszyk et al., 2016; Zanos and Gould, 2018). It is interesting to consider that a similar mechanism may be operating at MeApv-VmHvl and MeApv-BNSTm synapses, enhancing synaptic potentiation that drives early life stress-induced aggression.
Finally, it is worth noting that ketamine is a chiral compound, with most ketamine available today being a racemic mixture of the two optical enantiomers (R) and (S) (Kohrs and Durieux, 1998; Paul et al., 2009; Hashimoto, 2019). (R)-ketamine and (S)-ketamine have differing effects in many human and rodent studies (Paul et al., 2009; Hashimoto, 2019; Rafalo-Ulinska et al., 2022). It was initially suggested that (S)-ketamine was a better candidate as an antidepressant than (R)-ketamine because of its higher affinity for the NMDAR (Singh et al., 2016). And in fact, the FDA recently approved (S)-ketamine in the form of a nasal spray for treatment-resistant depression in adults (Hashimoto, 2019). However, in recent studies, (R)-ketamine was found to have greater potency and fewer side effects at lower doses than (S)-ketamine, suggesting it might be a safer alternative (Chang et al., 2019; Hashimoto, 2019; Beck et al., 2020; Rafalo-Ulinska et al., 2022). In a meta-analysis of 36 studies, racemic and (S)-ketamine were associated with a statistically significant increase in transient psychopathology in healthy and schizophrenia patients (Beck et al., 2020). Prescribing the lowest dose of ketamine necessary is essential due to the high risk of abuse associated with ketamine. Ketamine users quickly develop tolerance to the drug, and typical withdrawal effects include cravings, anxiety, sweating, and shaking (Kokane et al., 2020).
These differences in synaptic location, NMDAR subtype, chirality, and tolerance may explain why memantine and ketamine have such diverging clinical and experimental effects and provide a mechanism for the unique effects of these drugs on excessive and stress-induced aggression.
Clinical Assessment
Our studies and others have demonstrated that different NMDAR antagonists can promote or exacerbate aggressive behavior depending on a prior history of substance abuse, traumatic stress, or aggressive behavior. A proper assessment would decrease the incidence of aggression by ruling out those NMDAR antagonists as treatment options. Therefore, a complete evaluation of the patient’s history of psychiatric and physical illness and an assessment of past substance use should be performed before administering and prescribing these drugs. Fortunately, there are standard methods for assessing whether an individual has a history of traumatic stress or aggressive behavior or is currently using or abusing drugs or alcohol that would exclude them from being prescribed specific NMDAR antagonists.
Tests that evaluate a history of traumatic stress include The Primary Care PTSD Screen for DSM-5 (Prins et al., 2016), The Short Post-Traumatic Stress Disorder Rating Interview (Connor and Davidson, 2001), and the Trauma Screening Questionnaire (Brewin et al., 2002). Tests for individuals with a history of aggressive behavior or who are in an actively aggressive state include the Dynamic Appraisal of Situational Aggression (DASA; Ogloff and Daffern, 2006) and the Brøset Violence Checklist (BVC; Woods and Almvik, 2002), which assess the likelihood that a patient will become aggressive through factors such as physical or verbal threats, negative attitudes, and impulsivity. Finally, tests like the Tobacco, Alcohol, Prescription Medication, and Other Substance Use (TAPS) Tool (McNeely et al., 2016) and the Brief Screener for Alcohol, Tobacco, and Other Drugs (BSTAD) tool (Kelly et al., 2014) are used to evaluate drug and alcohol abuse in adults and adolescents, respectively. Importantly, these assessment tools can be implemented in the hospital setting, significantly reducing the likelihood of unintended aggressive behavior by NMDAR antagonists, and paving the way for more nuanced administration.
Conclusion
This review highlights some of the promises and pitfalls of the non-competitive NMDAR antagonists, and in particular ketamine and memantine, in treating excessive and recurring violent aggression. On the one hand, NMDAR antagonists are clearly powerful clinical tools in managing violent aggression. They possess a quicker onset of action and fewer observed side effects than current alternatives. NMDARs also have great potential as a long-lasting treatment option due to their effects on synaptic plasticity (Roberts and Geeting, 2001; Cummings et al., 2008; Hopper et al., 2015; Cole et al., 2016; Riddell et al., 2017; Barbic et al., 2021), where these drugs can induce persistent changes in synaptic function, neural firing, and animal behavior, in some cases, even after a single dose (Wilcock et al., 2008; Moda-Sava et al., 2019; Tran and Mierzwinski-Urban, 2019; Nordman et al., 2022).
While the potential of these drugs to treat excessive aggression related to changes in synaptic plasticity is enormous, care is needed. Numerous reports show that NMDAR antagonists have highly varied effects on aggression depending on dose, context, and experience (Nordman, 2021), outlined in Figure 1B. While many of these drugs can lessen or even suppress aggression, they can also heighten aggression when used improperly (Sukhotina and Bespalov, 2000; Newman et al., 2012, 2018; Covington et al., 2018; Nordman et al., 2022). Evidence from our lab and others suggests these differences may depend on changes in synaptic plasticity within limbic circuits (Figure 1A). Future studies should be aimed at investigating this intriguing possibility.
Data Availability Statement
The original contributions presented in the study are included in the article, further inquiries can be directed to the corresponding author.
Author Contributions
CB and JN wrote the manuscript. All authors contributed to the article and approved the submitted version.
Funding
This work was supported by School of Medicine, Southern Illinois University.
Conflict of Interest
The authors declare that the research was conducted in the absence of any commercial or financial relationships that could be construed as a potential conflict of interest.
Publisher’s Note
All claims expressed in this article are solely those of the authors and do not necessarily represent those of their affiliated organizations, or those of the publisher, the editors and the reviewers. Any product that may be evaluated in this article, or claim that may be made by its manufacturer, is not guaranteed or endorsed by the publisher.
References
Ali, F., Gerhard, D. M., Sweasy, K., Pothula, S., Pittenger, C., Duman, R. S., et al. (2020). Ketamine disinhibits dendrites and enhances calcium signals in prefrontal dendritic spines. Nat. Commun. 11:72. doi: 10.1038/s41467-019-13809-8
Amerio, A., Ossola, P., Scagnelli, F., Odone, A., Allinovi, M., Cavalli, A., et al. (2018). Safety and efficacy of lithium in children and adolescents: a systematic review in bipolar illness. Eur. Psychiatry 54, 85–97. doi: 10.1016/j.eurpsy.2018.07.012
Autry, A. E., Adachi, M., Nosyreva, E., Na, E. S., Los, M. F., Cheng, P. F., et al. (2011). NMDA receptor blockade at rest triggers rapid behavioural antidepressant responses. Nature 475, 91–95. doi: 10.1038/nature10130
Bacq, A., Astori, S., Gebara, E., Tang, W., Silva, B. A., Sanchez-Mut, J., et al. (2018). Amygdala GluN2B-NMDAR dysfunction is critical in abnormal aggression of neurodevelopmental origin induced by St8sia2 deficiency. Mol. Psychiatry 25, 2144–2161. doi: 10.1038/s41380-018-0132-3
Ballard, C., Day, S., Sharp, S., Wing, G., and Sorensen, S. (2008). Neuropsychiatric symptoms in dementia: importance and treatment considerations. Int. Rev. Psychiatry 20, 396–404. doi: 10.1080/09540260802099968
Ballard, C. G., Waite, J., and Birks, J. (2006). Atypical antipsychotics for aggression and psychosis in Alzheimer’s disease. Cochrane Database Syst. Rev. doi: 10.1002/14651858.CD003476.pub2
Barbic, D., Andolfatto, G., Grunau, B., Scheuermeyer, F. X., Macewan, B., Qian, H., et al. (2021). Rapid agitation control with ketamine in the emergency department: a blinded, randomized controlled trial. Ann. Emerg. Med. 78, 788–795. doi: 10.1016/j.annemergmed.2021.05.023
Beck, K., Hindley, G., Borgan, F., Ginestet, C., McCutcheon, R., Brugger, S., et al. (2020). Association of ketamine with psychiatric symptoms and implications for its therapeutic use and for understanding schizophrenia: a systematic review and meta-analysis. JAMA Netw. Open 3:e204693. doi: 10.1001/jamanetworkopen.2020.4693
Bolshakov, K. V., Gmiro, V. E., Tikhonov, D. B., and Magazanik, L. G. (2003). Determinants of trapping block of N-methyl-d-aspartate receptor channels. J. Neurochem. 87, 56–65. doi: 10.1046/j.1471-4159.2003.01956.x
Bonnet, U. (2015). Long-term ketamine self-injections in major depressive disorder: focus on tolerance in Ketamine’s antidepressant response and the development of ketamine addiction. J. Psychoactive Drugs 47, 276–285. doi: 10.1080/02791072.2015.1072653
Brewin, C. R., Rose, S., Andrews, B., Green, J., Tata, P., McEvedy, C., et al. (2002). Brief screening instrument for post-traumatic stress disorder. Br. J. Psychiatry 181, 158–162. doi: 10.1017/s0007125000161896
Burger, J., Capobianco, M., Lovern, R., Boche, B., Ross, E., Darracq, M. A., et al. (2016). A double-blinded, randomized, placebo-controlled sub-dissociative dose ketamine pilot study in the treatment of acute depression and suicidality in a military emergency department setting. Mil. Med. 181, 1195–1199. doi: 10.7205/MILMED-D-15-00431
Calsolaro, V., Antognoli, R., Okoye, C., and Monzani, F. (2019). The use of antipsychotic drugs for treating behavioral symptoms in Alzheimer’s disease. Front. Pharmacol. 10:1465. doi: 10.3389/fphar.2019.01465
Cerejeira, J., Lagarto, L., and Mukaetova-Ladinska, E. B. (2012). Behavioral and psychological symptoms of dementia. Front. Neurol. 3:73. doi: 10.3389/fneur.2012.00073
Chang, C. H., and Gean, P. W. (2019). The ventral hippocampus controls stress-provoked impulsive aggression through the ventromedial hypothalamus in post-weaning social isolation mice. Cell Rep. 28, 1195–1205.e3. doi: 10.1016/j.celrep.2019.07.005
Chang, C. H., Hsiao, Y. H., Chen, Y. W., Yu, Y. J., and Gean, P. W. (2015). Social isolation-induced increase in NMDA receptors in the hippocampus exacerbates emotional dysregulation in mice. Hippocampus 25, 474–485. doi: 10.1002/hipo.22384
Chang, C. H., Su, C. L., and Gean, P. W. (2018). Mechanism underlying NMDA blockade-induced inhibition of aggression in post-weaning socially isolated mice. Neuropharmacology 143, 95–105. doi: 10.1016/j.neuropharm.2018.09.019
Chang, L., Zhang, K., Pu, Y., Qu, Y., Wang, S. M., Xiong, Z., et al. (2019). Comparison of antidepressant and side effects in mice after intranasal administration of (R,S)-ketamine, (R)-ketamine and (S)-ketamine. Pharmacol. Biochem. Behav. 181, 53–59. doi: 10.1016/j.pbb.2019.04.008
Chen, P., and Hong, W. (2018). Neural circuit mechanisms of social behavior. Neuron 98, 16–30. doi: 10.1016/j.neuron.2018.02.026
Cole, J. B., Moore, J. C., Nystrom, P. C., Orozco, B. S., Stellpflug, S. J., Kornas, R. L., et al. (2016). A prospective study of ketamine versus haloperidol for severe prehospital agitation. Clin. Toxicol. 54, 556–562. doi: 10.1080/15563650.2016.1177652
Connor, K. M., and Davidson, J. R. (2001). SPRINT: a brief global assessment of post-traumatic stress disorder. Int. Clin. Psychopharmacol. 16, 279–284. doi: 10.1097/00004850-200109000-00005
Covington, H. E., Newman, E. L. 3rd, Tran, S., Walton, L., Hayek, W., Leonard, M. Z., et al. (2018). The urge to fight: persistent escalation by alcohol and role of NMDA receptors in mice. Front. Behav. Neurosci. 12:206. doi: 10.3389/fnbeh.2018.00206
Cullen, K. R., Amatya, P., Roback, M. G., Albott, C. S., Westlund Schreiner, M., Ren, Y., et al. (2018). Intravenous ketamine for adolescents with treatment-resistant depression: an open-label study. J. Child Adolesc. Psychopharmacol. 28, 437–444. doi: 10.1089/cap.2018.0030
Cummings, J. L., Mackell, J., and Kaufer, D. (2008). Behavioral effects of current Alzheimer’s disease treatments: a descriptive review. Alzheimers Dement. 4, 49–60. doi: 10.1016/j.jalz.2007.10.011
Da Re, F., Rucci, F., and Isella, V. (2015). Retrospective study on agitation provoked by memantine in dementia. J. Neuropsychiatry Clin. Neurosci. 27, e10–e13. doi: 10.1176/appi.neuropsych.13100226
da Silva, F. C., do Carmo de Oliveira Cito, M., da Silva, M. I., Moura, B. A., de Aquino Neto, M. R., Feitosa, M. L., et al. (2010). Behavioral alterations and pro-oxidant effect of a single ketamine administration to mice. Brain Res. Bull. 83, 9–15. doi: 10.1016/j.brainresbull.2010.05.011
Delbello, M. P., Kowatch, R. A., Adler, C. M., Stanford, K. E., Welge, J. A., Barzman, D. H., et al. (2006). A double-blind randomized pilot study comparing quetiapine and divalproex for adolescent mania. J. Am. Acad. Child Adolesc. Psychiatry 45, 305–313. doi: 10.1097/01.chi.0000194567.63289.97
Dillon, P., Copeland, J., and Jansen, K. (2003). Patterns of use and harms associated with non-medical ketamine use. Drug Alcohol Depend. 69, 23–28. doi: 10.1016/s0376-8716(02)00243-0
Domino, E. F., Chodoff, P., and Corssen, G. (1965). Pharmacologic effects of Ci-581, a new dissociative anesthetic, in man. Clin. Pharmacol. Ther. 6, 279–291. doi: 10.1002/cpt196563279
Donoghue, A. C., Roback, M. G., and Cullen, K. R. (2015). Remission from behavioral dysregulation in a child with PTSD after receiving procedural ketamine. Pediatrics 136, e694–e696. doi: 10.1542/peds.2014-4152
Dwyer, J. B., Beyer, C., Wilkinson, S. T., Ostroff, R. B., Qayyum, Z., Bloch, M. H., et al. (2017). Ketamine as a treatment for adolescent depression: a case report. J. Am. Acad. Child Adolesc. Psychiatry 56, 352–354. doi: 10.1016/j.jaac.2017.01.006
Emnett, C. M., Eisenman, L. N., Taylor, A. M., Izumi, Y., Zorumski, C. F., Mennerick, S., et al. (2013). Indistinguishable synaptic pharmacodynamics of the N-methyl-D-aspartate receptor channel blockers memantine and ketamine. Mol. Pharmacol. 84, 935–947. doi: 10.1124/mol.113.089334
Falkner, A. L., Wei, D., Song, A., Watsek, L. W., Chen, I., Chen, P., et al. (2020). Hierarchical representations of aggression in a hypothalamic-midbrain circuit. Neuron 106, 637–648.e6. doi: 10.1016/j.neuron.2020.02.014
Fox, C., Crugel, M., Maidment, I., Auestad, B. H., Coulton, S., Treloar, A., et al. (2012). Efficacy of memantine for agitation in Alzheimer’s dementia: a randomised double-blind placebo controlled trial. PLoS One 7:e35185. doi: 10.1371/journal.pone.0035185
Gauthier, S., Loft, H., and Cummings, J. (2008). Improvement in behavioural symptoms in patients with moderate to severe Alzheimer’s disease by memantine: a pooled data analysis. Int. J. Geriatr. Psychiatry 23, 537–545. doi: 10.1002/gps.1949
Geller, B., Luby, J. L., Joshi, P., Wagner, K. D., Emslie, G., Walkup, J. T., et al. (2012). A randomized controlled trial of risperidone, lithium, or divalproex sodium for initial treatment of bipolar I disorder, manic or mixed phase, in children and adolescents. Arch. Gen. Psychiatry 69, 515–528. doi: 10.1001/archgenpsychiatry.2011.1508
Gerhard, D. M., Pothula, S., Liu, R. J., Wu, M., Li, X. Y., Girgenti, M. J., et al. (2020). GABA interneurons are the cellular trigger for ketamine’s rapid antidepressant actions. J. Clin. Invest. 130, 1336–1349. doi: 10.1172/JCI130808
Gideons, E. S., Kavalali, E. T., and Monteggia, L. M. (2014). Mechanisms underlying differential effectiveness of memantine and ketamine in rapid antidepressant responses. Proc. Natl. Acad. Sci. U S A 111, 8649–8654. doi: 10.1073/pnas.1323920111
Glasgow, N. G., Povysheva, N. V., Azofeifa, A. M., and Johnson, J. W. (2017). Memantine and ketamine differentially alter NMDA receptor desensitization. J. Neurosci. 37, 9686–9704. doi: 10.1523/JNEUROSCI.1173-17.2017
Haas, M., Unis, A. S., Armenteros, J., Copenhaver, M. D., Quiroz, J. A., Kushner, S. F., et al. (2009). A 6-week, randomized, double-blind, placebo-controlled study of the efficacy and safety of risperidone in adolescents with schizophrenia. J. Child Adolesc. Psychopharmacol. 19, 611–621. doi: 10.1089/cap.2008.0144
Hashimoto, K. (2019). Rapid-acting antidepressant ketamine, its metabolites and other candidates: a historical overview and future perspective. Psychiatry Clin. Neurosci. 73, 613–627. doi: 10.1111/pcn.12902
Herrmann, N., Li, A., and Lanctot, K. (2011). Memantine in dementia: a review of the current evidence. Expert Opin. Pharmacother. 12, 787–800. doi: 10.1517/14656566.2011.558006
Hewitt, D. J. (2000). The use of NMDA-receptor antagonists in the treatment of chronic pain. Clin. J. Pain 16, S73–S79. doi: 10.1097/00002508-200006001-00013
Heydari, F., Gholamian, A., Zamani, M., and Majidinejad, S. (2018). Effect of intramuscular ketamine versus haloperidol on short-term control of severe agitated patients in emergency department; a randomized clinical trial. Bull. Emerg. Trauma 6, 292–299. doi: 10.29252/beat-060404
Hirota, K., and Lambert, D. G. (1996). Ketamine: its mechanism(s) of action and unusual clinical uses. Br. J. Anaesth. 77, 441–444. doi: 10.1093/bja/77.4.441
Hopper, A. B., Vilke, G. M., Castillo, E. M., Campillo, A., Davie, T., Wilson, M. P., et al. (2015). Ketamine use for acute agitation in the emergency department. J. Emerg. Med. 48, 712–719. doi: 10.1016/j.jemermed.2015.02.019
Huey, E. D., Dustin, I. H., Overman, G. P., Mirza, N., and Sunderland, T. (2005). Development of subtle psychotic symptoms with memantine: a case report. J. Clin. Psychiatry 66, 658–659. doi: 10.4088/jcp.v66n0519f
Ichinose, M., Miura, I., Horikoshi, S., Matsumoto, J., Osakabe, Y., Yabe, H., et al. (2021). Memantine for behavioral symptoms of hepatic encephalopathy associated with alcoholic cirrhosis: a case report. J. Clin. Psychopharmacol. 41, 85–86. doi: 10.1097/JCP.0000000000001326
Jaclson, J. L., and Mallory, R. (2009). Aggression and violence among elderly patients, a growing health problem. J. Gen. Intern. Med. 24, 1167–1168. doi: 10.1007/s11606-009-1099-1
Johnson, J. W., Glasgow, N. G., and Povysheva, N. V. (2015). Recent insights into the mode of action of memantine and ketamine. Curr. Opin. Pharmacol. 20, 54–63. doi: 10.1016/j.coph.2014.11.006
Kales, H. C., Kim, H. M., Zivin, K., Valenstein, M., Seyfried, L. S., Chiang, C., et al. (2012). Risk of mortality among individual antipsychotics in patients with dementia. Am. J. Psychiatry 169, 71–79. doi: 10.1176/appi.ajp.2011.11030347
Keene, J., Hope, T., Fairburn, C. G., Jacoby, R., Gedling, K., Ware, C. J., et al. (1999). Natural history of aggressive behaviour in dementia. Int. J. Geriatr. Psychiatry 14, 541–548. doi: 10.1002/(sici)1099-1166(199907)14:7<541::aid-gps961<3.0.co;2-p
Kelly, S. M., Gryczynski, J., Mitchell, S. G., Kirk, A., O’Grady, K. E., Schwartz, R. P., et al. (2014). Validity of brief screening instrument for adolescent tobacco, alcohol and drug use. Pediatrics 133, 819–826. doi: 10.1542/peds.2013-2346
Kent, J., Austin, E., and Nolan, B. (2022). Does ketamine provide a shorter time to sedation when compared to haloperidol and midazolam in the agitated ED patient? CJEM 24, 133–134. doi: 10.1007/s43678-021-00249-x
Kim, S., Rush, B. S., and Rice, T. R. (2021). A systematic review of therapeutic ketamine use in children and adolescents with treatment-resistant mood disorders. Eur. Child Adolesc. Psychiatry 30, 1485–1501. doi: 10.1007/s00787-020-01542-3
Kishi, T., Matsunaga, S., and Iwata, N. (2017). The effects of memantine on behavioral disturbances in patients with Alzheimer’s disease: a meta-analysis. Neuropsychiatr. Dis. Treat 13, 1909–1928. doi: 10.2147/NDT.S142839
Kitanaka, N., Kitanaka, J., Hall, F. S., Kubota, Y., Mimura, Y., Ogura, S., et al. (2018). Psychotomimetic-like behavioral effects of memantine in the mouse. Biomed. Pharmacother. 100, 116–123. doi: 10.1016/j.biopha.2018.01.160
Kohrs, R., and Durieux, M. E. (1998). Ketamine: teaching an old drug new tricks. Anesth. Analg. 87, 1186–1193. doi: 10.1097/00000539-199811000-00039
Kokane, S. S., Armant, R. J., Bolanos-Guzman, C. A., and Perrotti, L. I. (2020). Overlap in the neural circuitry and molecular mechanisms underlying ketamine abuse and its use as an antidepressant. Behav. Brain Res. 384:112548. doi: 10.1016/j.bbr.2020.112548
Kotermanski, S. E., and Johnson, J. W. (2009). Mg2+ imparts NMDA receptor subtype selectivity to the Alzheimer’s drug memantine. J. Neurosci. 29, 2774–2779. doi: 10.1523/JNEUROSCI.3703-08.2009
Lahti, A. C., Weiler, M. A., Tamara Michaelidis, B. A., Parwani, A., and Tamminga, C. A. (2001). Effects of ketamine in normal and schizophrenic volunteers. Neuropsychopharmacology 25, 455–467. doi: 10.1016/S0893-133X(01)00243-3
Lanthorn, T. H., Mealing, G. A., and Morley, P. (2000). Differences in degree of trapping between AR-R15896 and other uncompetitive NMDA receptor antagonists. Amino Acids 19, 173–175. doi: 10.1007/s007260070046
Le Cong, M., Gynther, B., Hunter, E., and Schuller, P. (2012). Ketamine sedation for patients with acute agitation and psychiatric illness requiring aeromedical retrieval. Emerg. Med. J. 29, 335–337. doi: 10.1136/emj.2010.107946
Leveille, F., El Gaamouch, F., Gouix, E., Lecocq, M., Lobner, D., Nicole, O., et al. (2008). Neuronal viability is controlled by a functional relation between synaptic and extrasynaptic NMDA receptors. FASEB J. 22, 4258–4271. doi: 10.1096/fj.08-107268
Ma, X. C., Dang, Y. H., Jia, M., Ma, R., Wang, F., Wu, J., et al. (2013). Long-lasting antidepressant action of ketamine, but not glycogen synthase kinase-3 inhibitor SB216763, in the chronic mild stress model of mice. PLoS One 8:e56053. doi: 10.1371/journal.pone.0056053
Maeng, S., Zarate, C. A., Du, J. Jr., Schloesser, R. J., McCammon, J., G. Chen, G., et al. (2008). Cellular mechanisms underlying the antidepressant effects of ketamine: role of alpha-amino-3-hydroxy-5-methylisoxazole-4-propionic acid receptors. Biol. Psychiatry 63, 349–352. doi: 10.1016/j.biopsych.2007.05.028
Mankowitz, S. L., Regenberg, P., Kaldan, J., and Cole, J. B. (2018). Ketamine for rapid sedation of agitated patients in the prehospital and emergency department settings: a systematic review and proportional meta-analysis. J. Emerg. Med. 55, 670–681. doi: 10.1016/j.jemermed.2018.07.017
McNeely, J., Wu, L. T., Subramaniam, G., Sharma, G., Cathers, L. A., Svikis, D., et al. (2016). Performance of the tobacco, alcohol, prescription medication and other substance use (TAPS) tool for substance use screening in primary care patients. Ann. Intern. Med. 165, 690–699. doi: 10.7326/M16-0317
Melamed, E., Oron, Y., Ben-Avraham, R., Blumenfeld, A., and Lin, G. (2007). The combative multitrauma patient: a protocol for prehospital management. Eur. J. Emerg. Med. 14, 265–268. doi: 10.1097/MEJ.0b013e32823a3c9b
Meyer, W. N., Keifer, J., Korzan, W. J., and Summers, C. H. (2004). Social stress and corticosterone regionally upregulate limbic N-methyl-D-aspartatereceptor (NR) subunit type NR2A and NR2B in the lizard Anolis carolinensis. Neuroscience 128, 675–684. doi: 10.1016/j.neuroscience.2004.06.084
Michelotti, P., Quadros, V. A., Pereira, M. E., and Rosemberg, D. B. (2018). Ketamine modulates aggressive behavior in adult zebrafish. Neurosci. Lett. 684, 164–168. doi: 10.1016/j.neulet.2018.08.009
Miller, O. H., Yang, L., Wang, C. C., Hargroder, E. A., Zhang, Y., Delpire, E., et al. (2014). GluN2B-containing NMDA receptors regulate depression-like behavior and are critical for the rapid antidepressant actions of ketamine. eLife 3:e03581. doi: 10.7554/eLife.03581
Milnerwood, A. J., Gladding, C. M., Pouladi, M. A., Kaufman, A. M., Hines, R. M., Boyd, J. D., et al. (2010). Early increase in extrasynaptic NMDA receptor signaling and expression contributes to phenotype onset in Huntington’s disease mice. Neuron 65, 178–190. doi: 10.1016/j.neuron.2010.01.008
Moda-Sava, R. N., Murdock, M. H., Parekh, P. K., Fetcho, R. N., Huang, B. S., Huynh, T. N., et al. (2019). Sustained rescue of prefrontal circuit dysfunction by antidepressant-induced spine formation. Science 364:eaat8078. doi: 10.1126/science.aat8078
Moghaddam, B., Adams, B., Verma, A., and Daly, D. (1997). Activation of glutamatergic neurotransmission by ketamine: a novel step in the pathway from NMDA receptor blockade to dopaminergic and cognitive disruptions associated with the prefrontal cortex. J. Neurosci. 17, 2921–2927. doi: 10.1523/JNEUROSCI.17-08-02921.1997
Morgan, C. J., Mofeez, A., Brandner, B., Bromley, L., and Curran, H. V. (2004). Acute effects of ketamine on memory systems and psychotic symptoms in healthy volunteers. Neuropsychopharmacology 29, 208–218. doi: 10.1038/sj.npp.1300342
Newman, E. L., Chu, A., Bahamon, B., Takahashi, A., Debold, J. F., Miczek, K. A., et al. (2012). NMDA receptor antagonism: escalation of aggressive behavior in alcohol-drinking mice. Psychopharmacology (Berl) 224, 167–177. doi: 10.1007/s00213-012-2734-9
Newman, E. L., Terunuma, M., Wang, T. L., Hewage, N., Bicakci, M. B., Moss, S. J., et al. (2018). A role for prefrontal cortical NMDA receptors in murine alcohol-heightened aggression. Neuropsychopharmacology 43, 1224–1234. doi: 10.1038/npp.2017.253
Nordman, J. C. (2021). Anger management: mechanisms of glutamate receptor-mediated synaptic plasticity underlying animal aggression. Int. J. Biochem. Cell Biol. 142:106120. doi: 10.1016/j.biocel.2021.106120
Nordman, J. C., Ma, X., Gu, Q., Potegal, M., Li, H., Kravitz, A. V., et al. (2020a). Potentiation of divergent medial amygdala pathways drives experience-dependent aggression escalation. J. Neurosci. 40, 4858–4880. doi: 10.1523/JNEUROSCI.0370-20.2020
Nordman, J. C., Ma, X., and Li, Z. (2020b). Traumatic stress induces prolonged aggression increase through synaptic potentiation in the medial amygdala circuits. eNeuro 7:ENEURO.0147-20.2020. doi: 10.1523/ENEURO.0147-20.2020
Nordman, J. C., Bartsch, C. J., and Li, Z. (2022). Opposing effects of NMDA receptor antagonists on early life stress-induced aggression in mice. Aggress. Behav. 48, 365–373. doi: 10.1002/ab.22022
Nosyreva, E., Szabla, K., Autry, A. E., Ryazanov, A. G., Monteggia, L. M., Kavalali, E. T., et al. (2013). Acute suppression of spontaneous neurotransmission drives synaptic potentiation. J. Neurosci. 33, 6990–7002. doi: 10.1523/JNEUROSCI.4998-12.2013
O’Connor, L., Rebesco, M., Robinson, C., Gross, K., Castellana, A., O’Connor, M. J., et al. (2019). Outcomes of prehospital chemical sedation with ketamine versus haloperidol and benzodiazepine or physical restraint only. Prehosp. Emerg. Care 23, 201–209. doi: 10.1080/10903127.2018.1501445
Ogloff, J. R., and Daffern, M. (2006). The dynamic appraisal of situational aggression: an instrument to assess risk for imminent aggression in psychiatric inpatients. Behav. Sci. Law 24, 799–813. doi: 10.1002/bsl.741
Okamoto, S., Pouladi, M. A., Talantova, M., Yao, D., Xia, P., Ehrnhoefer, D. E., et al. (2009). Balance between synaptic versus extrasynaptic NMDA receptor activity influences inclusions and neurotoxicity of mutant huntingtin. Nat. Med. 15, 1407–1413. doi: 10.1038/nm.2056
Olives, T. D., Nystrom, P. C., Cole, J. B., Dodd, K. W., and Ho, J. D. (2016). Intubation of profoundly agitated patients treated with prehospital ketamine. Prehosp. Disaster Med. 31, 593–602. doi: 10.1017/S1049023X16000819
Pandina, G., Kushner, S., Karcher, K., and Haas, M. (2012). An open-label, multicenter evaluation of the long-term safety and efficacy of risperidone in adolescents with schizophrenia. Child Adolesc. Psychiatry Ment. Health 6:23. doi: 10.1186/1753-2000-6-23
Papolos, D. F., Teicher, M. H., Faedda, G. L., Murphy, P., and Mattis, S. (2013). Clinical experience using intranasal ketamine in the treatment of pediatric bipolar disorder/fear of harm phenotype. J. Affect. Disord. 147, 431–436. doi: 10.1016/j.jad.2012.08.040
Paul, R., Schaaff, N., Padberg, F., Moller, H. J., and Frodl, T. (2009). Comparison of racemic ketamine and S-ketamine in treatment-resistant major depression: report of two cases. World J. Biol. Psychiatry 10, 241–244. doi: 10.1080/15622970701714370
Peregud, D. I., Yakovlev, A. A., Stepanichev, M. Y., Onufriev, M. V., Panchenko, L. F., Gulyaeva, N. V., et al. (2012). Content of mRNA for NMDA glutamate receptor subunits in the frontal cortex and striatum of rats after morphine withdrawal is related to the degree of abstinence. Bull. Exp. Biol. Med. 153, 835–838. doi: 10.1007/s10517-012-1838-x
Perszyk, R. E., DiRaddo, J. O., Strong, K. L., Low, C. M., Ogden, K. K., Khatri, A., et al. (2016). GluN2D-containing N-methyl-d-aspartate receptors mediate synaptic transmission in hippocampal interneurons and regulate interneuron activity. Mol. Pharmacol. 90, 689–702. doi: 10.1124/mol.116.105130
Petralia, S. M., DeBold, J. F., and Frye, C. A. (2007). MK-801 infusions to the ventral tegmental area and ventromedial hypothalamus produce opposite effects on lordosis of hormone-primed rats. Pharmacol. Biochem. Behav. 86, 377–385. doi: 10.1016/j.pbb.2007.01.005
Pisano, S., Pozzi, M., Catone, G., Scrinzi, G., Clementi, E., Coppola, G., et al. (2019). Putative mechanisms of action and clinical use of lithium in children and adolescents: a critical review. Curr. Neuropharmacol. 17, 318–341. doi: 10.2174/1570159X16666171219142120
Pringsheim, T., Hirsch, L., Gardner, D., and Gorman, D. A. (2015). The pharmacological management of oppositional behaviour, conduct problems and aggression in children and adolescents with attention-deficit hyperactivity disorder, oppositional defiant disorder and conduct disorder: a systematic review and meta-analysis. Part 1: psychostimulants, alpha-2 agonists and atomoxetine. Can. J. Psychiatry 60, 42–51. doi: 10.1177/070674371506000202
Prins, A., Bovin, M. J., Smolenski, D. J., Marx, B. P., Kimerling, R., Jenkins-Guarnieri, M. A., et al. (2016). The primary care PTSD Screen for DSM-5 (PC-PTSD-5): development and evaluation within a veteran primary care sample. J. Gen. Intern. Med. 31, 1206–1211. doi: 10.1007/s11606-016-3703-5
Rafalo-Ulinska, A., Branski, P., and Palucha-Poniewiera, A. (2022). Combined administration of (R)-ketamine and the mGlu2/3 receptor antagonist LY341495 induces rapid and sustained effects in the CUMS model of depression via a TrkB/BDNF-dependent mechanism. Pharmaceuticals (Basel) 15:125. doi: 10.3390/ph15020125
Redden, L., DelBello, M., Wagner, K. D., Wilens, T. E., Malhotra, S., Wozniak, P., et al. (2009). Long-term safety of divalproex sodium extended-release in children and adolescents with bipolar I disorder. J. Child Adolesc. Psychopharmacol. 19, 83–89. doi: 10.1089/cap.2008.0106
Riddell, J., Tran, A., Bengiamin, R., Hendey, G. W., and Armenian, P. (2017). Ketamine as a first-line treatment for severely agitated emergency department patients. Am. J. Emerg. Med. 35, 1000–1004. doi: 10.1016/j.ajem.2017.02.026
Roberts, J. R., and Geeting, G. K. (2001). Intramuscular ketamine for the rapid tranquilization of the uncontrollable, violent and dangerous adult patient. J. Trauma 51, 1008–1010. doi: 10.1097/00005373-200111000-00031
Robinson, D. M., and Keating, G. M. (2006). Memantine: a review of its use in Alzheimer’s disease. Drugs 66, 1515–1534. doi: 10.2165/00003495-200666110-00015
Sang, C. N. (2000). NMDA-receptor antagonists in neuropathic pain: experimental methods to clinical trials. J. Pain Symptom Manage. 19, S21–S25. doi: 10.1016/s0885-3924(99)00125-6
Schak, K. M., Vande Voort, J. L., Johnson, E. K., Kung, S., Leung, J. G., Rasmussen, K. G., et al. (2016). Potential risks of poorly monitored ketamine use in depression treatment. Am. J. Psychiatry 173, 215–218. doi: 10.1176/appi.ajp.2015.15081082
Scheppke, K. A., Braghiroli, J., Shalaby, M., and Chait, R. (2014). Prehospital use of i.m. ketamine for sedation of violent and agitated patients. West J. Emerg. Med. 15, 736–741. doi: 10.5811/westjem.2014.9.23229
Shaikh, M. B., Schubert, K., and Siegel, A. (1994). Basal amygdaloid facilitation of midbrain periaqueductal gray elicited defensive rage behavior in the cat is mediated through NMDA receptors. Brain Res. 635, 187–195. doi: 10.1016/0006-8993(94)91438-9
Shaikh, M. B., and Siegel, A. (1994). Neuroanatomical and neurochemical mechanisms underlying amygdaloid control of defensive rage behavior in the cat. Braz. J. Med. Biol. Res. 27, 2759–2779.
Shin, S. Y., Baek, N. J., Han, S. H., and Min, S. S. (2019). Chronic administration of ketamine ameliorates the anxiety- and aggressive-like behavior in adolescent mice induced by neonatal maternal separation. Korean J. Physiol. Pharmacol. 23, 81–87. doi: 10.4196/kjpp.2019.23.1.81
Singh, J. B., Fedgchin, M., Daly, E., Xi, L., Melman, C., De Bruecker, G., et al. (2016). Intravenous esketamine in adult treatment-resistant depression: a double-blind, double-randomization, placebo-controlled study. Biol. Psychiatry 80, 424–431. doi: 10.1016/j.biopsych.2015.10.018
Sleigh, J., Harvey, M., Voss, L., and Denny, B. (2014). Ketamine - More mechanisms of action than just NMDA blockade. Trends Anaesth. Critical Care 4, 76–81. doi: 10.1016/j.tacc.2014.03.002
Solano, J. J., Clayton, L. M., Parks, D. J., Polley, S. E., Hughes, P. G., Hennekens, C. H., et al. (2021). Prehospital ketamine administration for excited delirium with illicit substance co-ingestion and subsequent intubation in the emergency department. Prehosp. Disaster Med. 36, 697–701. doi: 10.1017/S1049023X21000935
Solmi, M., Fornaro, M., Ostinelli, E. G., Zangani, C., Croatto, G., Monaco, F., et al. (2020). Safety of 80 antidepressants, antipsychotics, anti-attention-deficit/hyperactivity medications and mood stabilizers in children and adolescents with psychiatric disorders: a large scale systematic meta-review of 78 adverse effects. World Psychiatry 19, 214–232. doi: 10.1002/wps.20765
Squire, L. R., and Kandel, E. R. (2009). Memory: From Mind to Molecules. Greenwood Village, CO: Roberts and Co.
Sukhotina, I. A., and Bespalov, A. Y. (2000). Effects of the NMDA receptor channel blockers memantine and MRZ 2/579 on morphine withdrawal-facilitated aggression in mice. Psychopharmacology (Berl) 149, 345–350. doi: 10.1007/s002130000386
Takahashi, A., Lee, R. X., Iwasato, T., Itohara, S., Arima, H., Bettler, B., et al. (2015). Glutamate input in the dorsal raphe nucleus as a determinant of escalated aggression in male mice. J. Neurosci. 35, 6452–6463. doi: 10.1523/JNEUROSCI.2450-14.2015
Takahashi, R. N., Morato, G. S., and Monteiro-de-Lima, T. C. (1984). Effects of ketamine on experimental animal models of aggression. Braz. J. Med. Biol. Res. 17, 171–178.
Thomas, S. J., and Grossberg, G. T. (2009). Memantine: a review of studies into its safety and efficacy in treating Alzheimer’s disease and other dementias. Clin. Interv. Aging 4, 367–377. doi: 10.2147/cia.s6666
Tran, K., and Mierzwinski-Urban, M. (2019). Ketamine for Pharmacological Management of Aggression and Agitation in Pre-Hospital Settings: A Review of Comparative Clinical Effectiveness, Safety and Guidelines [Internet]. Ottawa ON: Canadian Agency for Drugs and Technologies in Health.
Tse, Y. C., Lopez, J., Moquin, A., Wong, S. A., Maysinger, D., Wong, T. P., et al. (2019). The susceptibility to chronic social defeat stress is related to low hippocampal extrasynaptic NMDA receptor function. Neuropsychopharmacology 44, 1310–1318. doi: 10.1038/s41386-019-0325-8
Widman, A. J., and McMahon, L. L. (2018). Disinhibition of CA1 pyramidal cells by low-dose ketamine and other antagonists with rapid antidepressant efficacy. Proc. Natl. Acad. Sci. U S A 115, E3007–E3016. doi: 10.1073/pnas.1718883115
Wilcock, G. K., Ballard, C. G., and Cooper, J. A., and Loft, H. (2008). Memantine for agitation/aggression and psychosis in moderately severe to severe Alzheimer’s disease: a pooled analysis of 3 studies. J. Clin. Psychiatry 69, 341–348. doi: 10.4088/jcp.v69n0302
Willard, S. S., and Koochekpour, S. (2013). Glutamate, glutamate receptors and downstream signaling pathways. Int. J. Biol. Sci. 9, 948–959. doi: 10.7150/ijbs.6426
Woods, P., and Almvik, R. (2002). The Broset violence checklist (BVC). Acta Psychiatr. Scand. 106, 103–105. doi: 10.1034/j.1600-0447.106.s412.22.x
Wroge, C. M., Hogins, J., Eisenman, L., and Mennerick, S. (2012). Synaptic NMDA receptors mediate hypoxic excitotoxic death. J. Neurosci. 32, 6732–6742. doi: 10.1523/JNEUROSCI.6371-11.2012
Yang, B., Ren, Q., Ma, M., Chen, Q. X., and Hashimoto, K. (2016). Antidepressant Effects of (+)-MK-801 and (-)-MK-801 in the social defeat stress model. Int. J. Neuropsychopharmacol. 19:pyw080. doi: 10.1093/ijnp/pyw080
Zanos, P., and Gould, T. D. (2018). Mechanisms of ketamine action as an antidepressant. Mol. Psychiatry 23, 801–811. doi: 10.1038/mp.2017.255
Zarrinnegar, P., Kothari, J., and Cheng, K. (2019). Successful use of ketamine for the treatment of psychotic depression in a teenager. J. Child Adolesc. Psychopharmacol. 29, 472–473. doi: 10.1089/cap.2019.0028
Zhao, X., Marszalec, W., Toth, P. T., Huang, J., Yeh, J. Z., and Narahashi, T. (2006). in vitro galantamine-memantine co-application: mechanism of beneficial action. Neuropharmacology 51, 1181–1191. doi: 10.1016/j.neuropharm.2006.08.007
Zhao, X., Sun, L., Jia, H., Meng, Q., Wu, S., Li, N., et al. (2009). Isolation rearing induces social and emotional function abnormalities and alters glutamate and neurodevelopment-related gene expression in rats. Prog. Neuropsychopharmacol. Biol. Psychiatry 33, 1173–1177. doi: 10.1016/j.pnpbp.2009.06.016
Zhou, X., Ding, Q., Chen, Z., Yun, H., and Wang, H. (2013). Involvement of the GluN2A and GluN2B subunits in synaptic and extrasynaptic N-methyl-D-aspartate receptor function and neuronal excitotoxicity. J. Biol. Chem. 288, 24151–24159. doi: 10.1074/jbc.M113.482000
Zoicas, I., and Kornhuber, J. (2019). The role of the N-Methyl-D-aspartate receptors in social behavior in rodents. Int. J. Mol. Sci. 20:5599. doi: 10.3390/ijms20225599
Keywords: aggression, early life stress, NMDA receptor, medial amygdala, synaptic plasticity, ketamine, memantine
Citation: Bartsch CJ and Nordman JC (2022) Promises and Pitfalls of NMDA Receptor Antagonists in Treating Violent Aggression. Front. Behav. Neurosci. 16:938044. doi: 10.3389/fnbeh.2022.938044
Received: 06 May 2022; Accepted: 25 May 2022;
Published: 21 June 2022.
Edited by:
Patricio Huerta, Feinstein Institute for Medical Research, United StatesReviewed by:
Eric H. Chang, Feinstein Institute for Medical Research, United StatesAki Takahashi, University of Tsukuba, Japan
Copyright © 2022 Bartsch and Nordman. This is an open-access article distributed under the terms of the Creative Commons Attribution License (CC BY). The use, distribution or reproduction in other forums is permitted, provided the original author(s) and the copyright owner(s) are credited and that the original publication in this journal is cited, in accordance with accepted academic practice. No use, distribution or reproduction is permitted which does not comply with these terms.
*Correspondence: Jacob C. Nordman, am5vcmRtYW43OEBzaXVtZWQuZWR1
†ORCID: Caitlyn J. Bartsch, orcid.org/0000-0003-4432-9257; Jacob C. Nordman, orcid.org/0000-0003-2513-7831s
‡These authors have contributed equally to this work