- 1School of Chinese Materia Medica, Beijing University of Chinese Medicine, Beijing, China
- 2Zhejiang Yangshengtang Institute of Natural Medication Co., Ltd., Hangzhou, China
- 3Yangshengtang Pharmaceutical Co., Ltd., Hangzhou, China
Heosemys grandis, a species of Asian water turtle, that has a wide range of applications in the food and pharmaceutical industries. Since some processed products cannot be identified exclusively by morphological and microscopic identification, a reliable and quick approach to guarantee authenticity is critical. Thus, we fostered an effective and stable molecular identification system to identify Heosemys grandis based on DNA mini-barcoding and species-specific primers PCR technology. A total of 48 turtle samples from 16 different species were collected. To distinguish Heosemys grandis from its counterfeits, DNA mini-barcoding and a pair of species-specific primers were designed and verified by PCR after analyzing the COI sequences of samples. The results showed that only Heosemys grandis samples could generate a single clear band following amplification using species-specific primers. Employing DNA mini-barcoding to amplify samples can verify authenticity by sequence alignment. These findings indicated that species-specific primers PCR technology combined with DNA mini-barcoding could accurately detect the authenticity of Heosemys grandis. This technology broadens the application of molecular biology techniques in the food and pharmaceutical industries. It provides a reliable and convenient method for identifying raw materials to standardize the market and protect customers’ rights and interests.
Introduction
Heosemys grandis, a turtle of Geoemydidae Heosemys, is the largest species of hard-shelled and semiaquatic Asian water turtles. They are mainly distributed in Cambodia, Vietnam, Myanmar, Thailand, and Malaysia (Lintner et al., 2012). In the 1980s, Heosemys grandis was introduced to China. Then, breeding populations gradually shaped and primarily distributed in Zhejiang, Shandong, Jiangsu, Hunan, Hubei, Henan, Jiangxi, Guangdong, Guangxi, and Hainan (Zhou and Huang, 2007). Heosemys grandis is both palatable and restorative. Since 2002, many Heosemys grandis specimens have been sold in Haikou markets, with the majority of them being consumed as food to nourish the body (Wang et al., 2004). What’s more, Heosemys grandis is sold as a medicinal material in Chengdu, Anhui, and Hebei (Lan et al., 2012).
Market fraud, such as species substitution, is a concern in the food and drug industries (Lv et al., 2014; Tan et al., 2021). Low-cost and readily available materials are utilized to replace rare and expensive raw materials for profit (Silva et al., 2020). It has the potential to produce severe economic concerns and negative consequences on public health (Pinheiro et al., 2020; Silva et al., 2020). As a health product, the Guibie pill is a health supplement that benefits the kidney, replenishes the vital essence and the blood, and improves body immunity (Fan and Lv, 1999). Heosemys grandis is the raw material of Guibie pills. Due to the increase in demand for Heosemys grandis, various adulterated materials, such as Mauremys mutica, Trachemys scripta, Mauremys sinensis, and Mauremys reevesii, have surfaced on the market. These items are inexpensive and easy to obtain. As a result, there are many counterfeit Guibie pills on the market. Thus, consumers’ rights and interests cannot be guaranteed essentially, and the business volume of genuine products declined (Yu and Xia, 2004, 2000). For inexperienced researchers, it is hard to distinguish between authentic and counterfeit products through traditional methods, such as appearance and microscopic identification. Therefore, it is necessary to establish a simple and accurate identification method to distinguish Heosemys grandis from adulterations.
DNA barcode technology is currently used as an effective tool to identify species (Natonek-Wisniewska and Slota, 2008; An et al., 2012). DNA barcode based on sequencing and analyzing a standard region of cytochrome c oxidase subunit I (COI) provides a convenient method for identifying animal species (Che et al., 2012; Chen et al., 2021). Factors like high temperature, high pressure, repeated freezing and thawing in raw material processing can cause DNA degradation (Huang et al., 2021). Standard DNA barcode sequences are difficult to obtain for samples with degraded DNA (Su et al., 2020). Therefore, some scholars have proposed DNA mini-barcoding, a DNA sequence with a length of 100∼200 bp (Shokralla et al., 2015; Chen et al., 2018). Compared with traditional DNA barcodes, DNA mini-barcoding is easier to amplify sequences from degraded samples than standard DNA barcodes and has greater efficiency. It has become increasingly crucial for identification in the food and pharmaceutical industries (Hellberg et al., 2017; Chen et al., 2018). In addition, species-specific primers PCR technology is also a commonly applied method for identification (Gargouri et al., 2021). It obviates the sequencing process and has the advantage of simplicity and quickness. As a result, it has been widely applied in species identification research (Agee and Barthet, 2021; Ma et al., 2021).
This study designed a DNA mini-barcoding and species-specific primers to distinguish Heosemys grandis from other species. And the identification success rates of the two identification systems have been verified through experiments. It provides an effective and low-cost method for identifying authentic Heosemys grandis. This technology fosters the integration of molecular biology and pharmacognosy research and expands the use of molecular biology techniques in food and medicine development. Besides, this study offers an effective tool for the market supervision of Heosemys grandis transactions, which is critical for enhancing the safety and effectiveness of food and medicine related to Heosemys grandis.
Materials and Methods
Materials
Forty-eight turtle samples belonging to 16 species were collected from the markets of five provinces in China (Hubei, Zhejiang, Guangxi, Hainan, Yunnan) in October-November 2015 and June-July 2019 (Table 1). All of the samples come from turtles that are lawfully kept in captivity. Two treatments were given to each turtle. One method was to cut the skin of the turtle leg and extract muscle tissue with a scalpel. The muscle tissue was then tagged and kept at −80°C. This portion of the material was used to test the COI universal primer PCR. The other was to simulate the raw material processing process. After removing viscera, turtle oil, and a tiny portion of muscle tissue, the remaining part was dried at a temperature of 70∼80°C, frozen and crushed in liquid nitrogen, and passed through an 80-mesh sieve before being labeled and stored at −80°C. This portion of the sample was utilized to test COI universal primers, DNA mini-barcoding, and species-specific primers. All the samples were labeled according to the individual. The information was shown in Table 1, and the turtle species labeled in the marketplace matched the sequenced samples.
The identification primers were synthesized by Sangon Biotech (Shanghai) Co., Ltd., (2OD per tube), centrifuged at 8,000 r min–1 for 5 min, 1 mL of ddH2O was added, and the primers were vortexed for 1 min before being aliquoted, and then kept at −20°C for subsequent use. Other reagents were purchased locally.
DNA Extraction
A mortar was used to smash the tissue sample. Approximately 30 mg of tissue sample/powder was used for DNA extraction according to the instructions of the tissue/cell genomic DNA extraction kit (Biomed, China). To increase the DNA concentration, three adsorption columns were eluted with ddH2O (50 μL).
Establishment of COI Barcode Sequence Database
The extracted genomic DNA was amplified using COI primers (Table 2). PCR was carried out in a 30 μL reaction volume (Table 3). The PCR conditions included predenaturation for 5 min at 94°C followed by 35 cycles of denaturing (94°C, 1 min), annealing (46°C, 1 min) and extension (72°C, 1 min), and extension at 72°C for 10 min finally. The electrophoresis process was performed on a 1.0% agarose gel with Golden View Stain using 5 μL of PCR product. The results were visualized by a gel imaging system, and the PCR products were delivered to Sangon Biotech (Shanghai) for bidirectional sequencing.
To acquire the DNA sequence, Contig Express software was used to splice the two-way sequencing peak map and remove the weak or overlapped peak regions at both ends. DNAMAN software was used to align the sequences obtained and analyze the relatively specific sites and relatively conserved regions of Heosemys grandis. MEGA software was used to construct an N-J tree based on standard parameters with bootstrap testing of 1,000 replicates. Because the samples originated from separate suborders, Deinagkistrodon acutus (KP403586.1) was chosen as an outgroup since it belongs to the same class as the samples and is in a different order.
Establishment of DNA Mini-Barcoding Identification System
According to the COI sequence analysis results, DNA mini-barcoding primers HG (Table 2) were designed using Primer-BLAST1. The amplification system was consistent with Table 3. The thermal cycling conditions were 94°C for 5 min, followed by 35 cycles of 94°C for 45 s, 45°C for 45 s, and 72°C for 45 s, and a final extension at 72°C for 10 min. An agarose gel electrophoresis was utilized with a 1.0% gel concentration and the nucleic acid gel stain Golden View to observe the amplification results. The samples and DNA marker were loaded in a 5 μL volume. The PCR products and PCR amplification primers were sent to Sangon for DNA sequencing.
After using Contig Express software to splice the peak map, DNAMAN software was used to align the sequences. An N-J tree using GenBank sequence (KP403586.1) as outgroups was produced by MEGA software based on standard parameters with bootstrap testing of 1,000 replicates.
Establishment of a Species-Specific Primers PCR Identification System
Based on the COI sequence analysis results, Primer-BLAST (see text footnote 1) was used to design species-specific primers HGTY (Table 3). The PCR amplification conditions were initially set up as 94°C for 5 min, followed by 35 cycles of 94°C for 45 s, 60°C for 45 s, and 72°C for 45 s, and a final extension at 72°C for 10 min. Since the Table 2 system’s amplified band was weak, the experiment was repeated with the rTaq enzyme instead. The reaction system is shown in Table 4.
Results
Analysis of COI Barcode Sequence
The results of amplifying all of the samples are shown in Figure 1. As shown in the diagram, the processed products cannot amplify bands. It could be due to DNA degradation induced by drying samples at 70 ∼ 80°C and frequent freezing and thawing samples during transportation. As a result of this finding, we used processed products to test species-specific primers and DNA mini-barcoding.
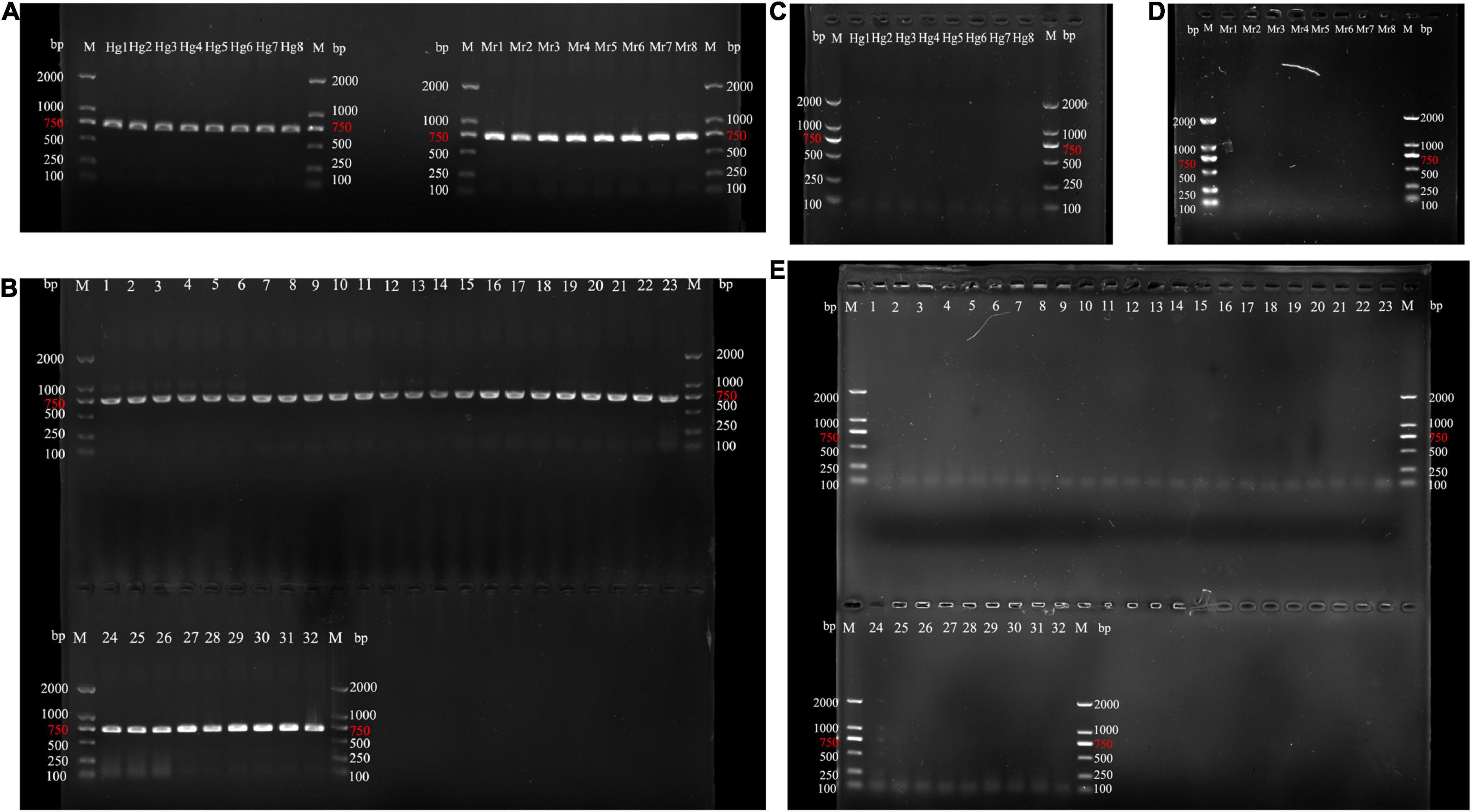
Figure 1. PCR product agarose gel results for amplifying COI sequences using optimal conditions. (A,B) Agarose gel electrophoresis of unprocessed samples. (C–E) Agarose gel electrophoresis of processed samples. Genuine samples: Hg1-Hg8; Mr1-Mr8:M. reevesii samples; 1–4: C. serpentina; 5–6: C. galbinifrons; 7–8: I. elongata; 9–10: P. megacephalum; 11–14: T. scripta; 15–16: P. subrufa; 17–18: C. amboinensis; 19–20: M. sinensis; 21–22: C. mouhotii; 23–24: C. dentata; 25–26: G. spengleri; 27–28: M. mutica; 29–30: M. annamensis; 31–32: P. unifilis). M, DNA ladders.
The COI sequence obtained after removing the primer region was 572 bp in total. Supplementary File 1 contains the full-length COI barcode sequences (572 bp) of 48 sequences. Among the turtle species studied in this experiment, there were 21 relatively specific sites (Table 5) and 5 segments of relatively conserved regions (∼22 bp) in the Heosemys grandis sequence (Table 6). These sequence characteristics were used to design primers in subsequent experiments.
The results of the N-J tree (Figure 2) revealed that samples from various species were clustered in one branch based on the species. Genetic distances were calculated by MEGA software. Three species (T. scripta, M. mutica, and P. unifilis) have an intraspecific distance of 0.0018, one (C. serpentina) has an intraspecific distance of 0.0012, and the remainder have an intraspecific distance of 0. The maximum interspecific distance was 0.4302, while the minimum interspecific distance was 0.0133. This result suggested that the COI universal barcode can discriminate between samples from different turtle species with undegraded DNA.
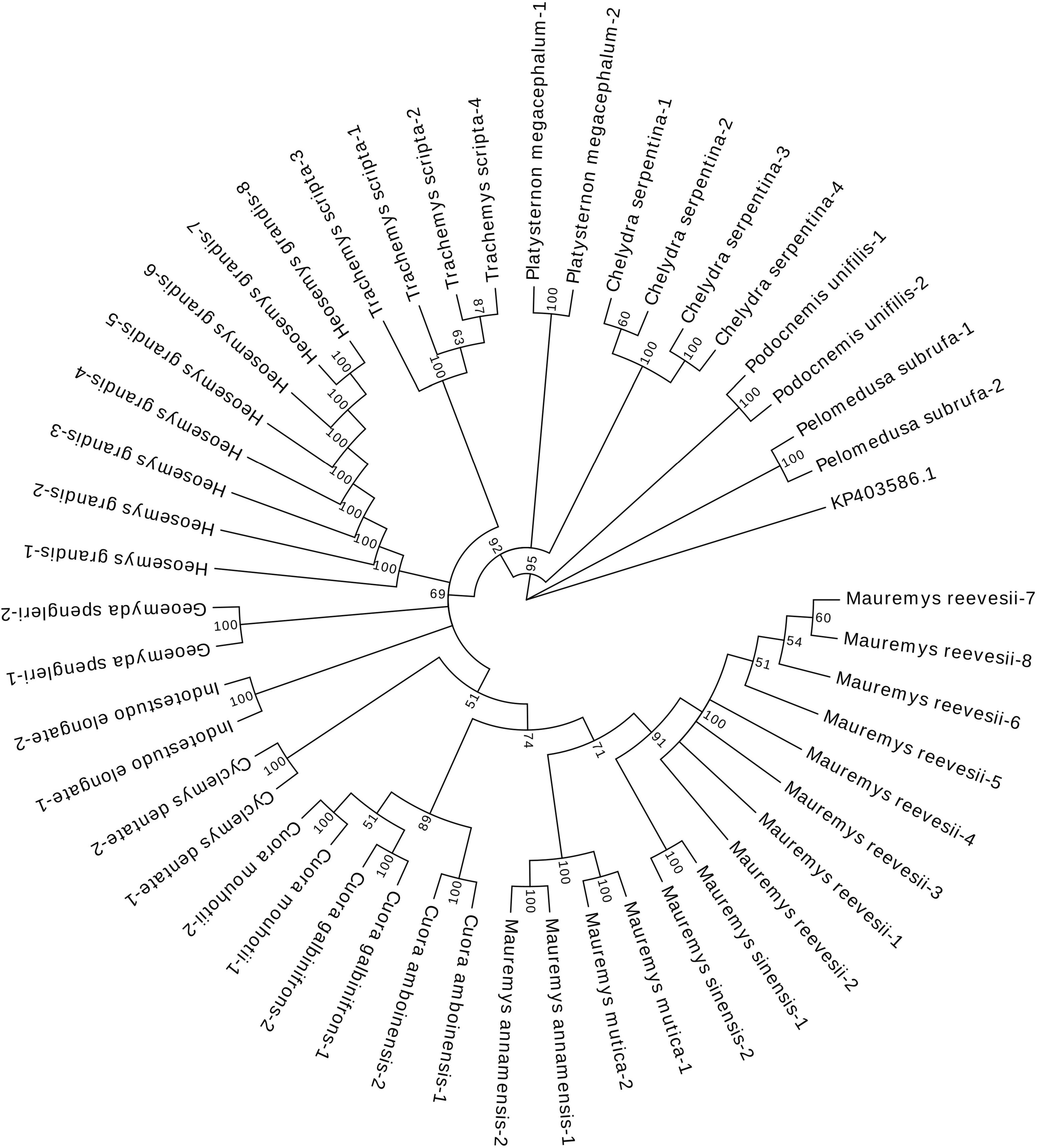
Figure 2. Results of the neighbor-joining tree based on COI sequences of the 48 turtle samples. The value represented the percentage of these branches in the bootstrap test, indicating whether the data adequately support their associated partitions. The closer to 100, the higher the credibility.
Verification of the Identification Efficiency of DNA Mini-Barcoding
According to agarose gel electrophoresis and sequencing experiments, HG had a 100% identification success rate and could produce a bright band around 170 bp (Figure 3) for subsequent gel-cut sequencing. The sequencing results of the verification experiment confirmed that HG could effectively identify Heosemys grandis and counterfeit species. The overall length of the HG sequence was 168 bp. All HG sequences are presented in Supplementary File 2. The sequence has been registered in GenBank2.
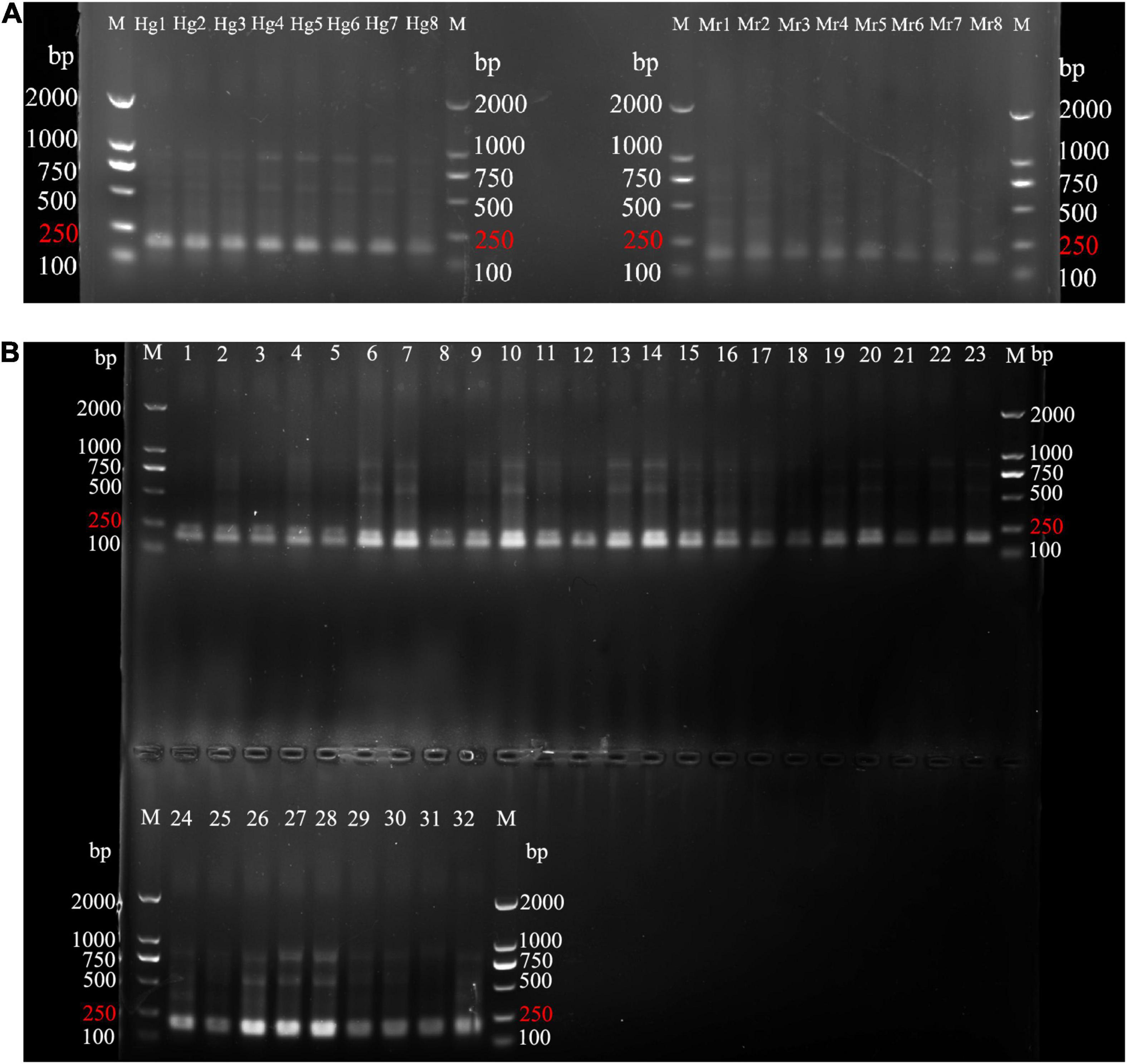
Figure 3. PCR product agarose gel results for 8 genuine and 40 counterfeit samples using HG. (A) Agarose gel electrophoresis of 8 genuine samples (Hg1-Hg8) and 8 M. reevesii samples (Mr1-Mr8). (B) Agarose gel electrophoresis of HG4 with 32 counterfeit species samples (1–4: C. serpentina; 5–6: C. galbinifrons; 7–8: I. elongata; 9–10: P. megacephalum; 11–14: T. scripta; 15–16: P. subrufa; 17–18: C. amboinensis; 19–20: M. sinensis; 21–22: C. mouhotii; 23–24: C. dentata; 25–26: G. spengleri; 27–28: M. mutica; 29–30: M. annamensis;31–32: P. unifilis). M, DNA ladders.
The N-J tree (Figure 4) presented that the samples of different species were aggregated in one branch according to the species. Genetic distances were calculated using MEGA software. Except for T. scripta, which had an intraspecific distance of 0.0045, all other species had an intraspecific distance of 0. The maximum interspecific distance was 0.2426, while the minimum interspecific distance was 0.0137. This result demonstrated that HG could be used for molecular identification between processed turtle samples.
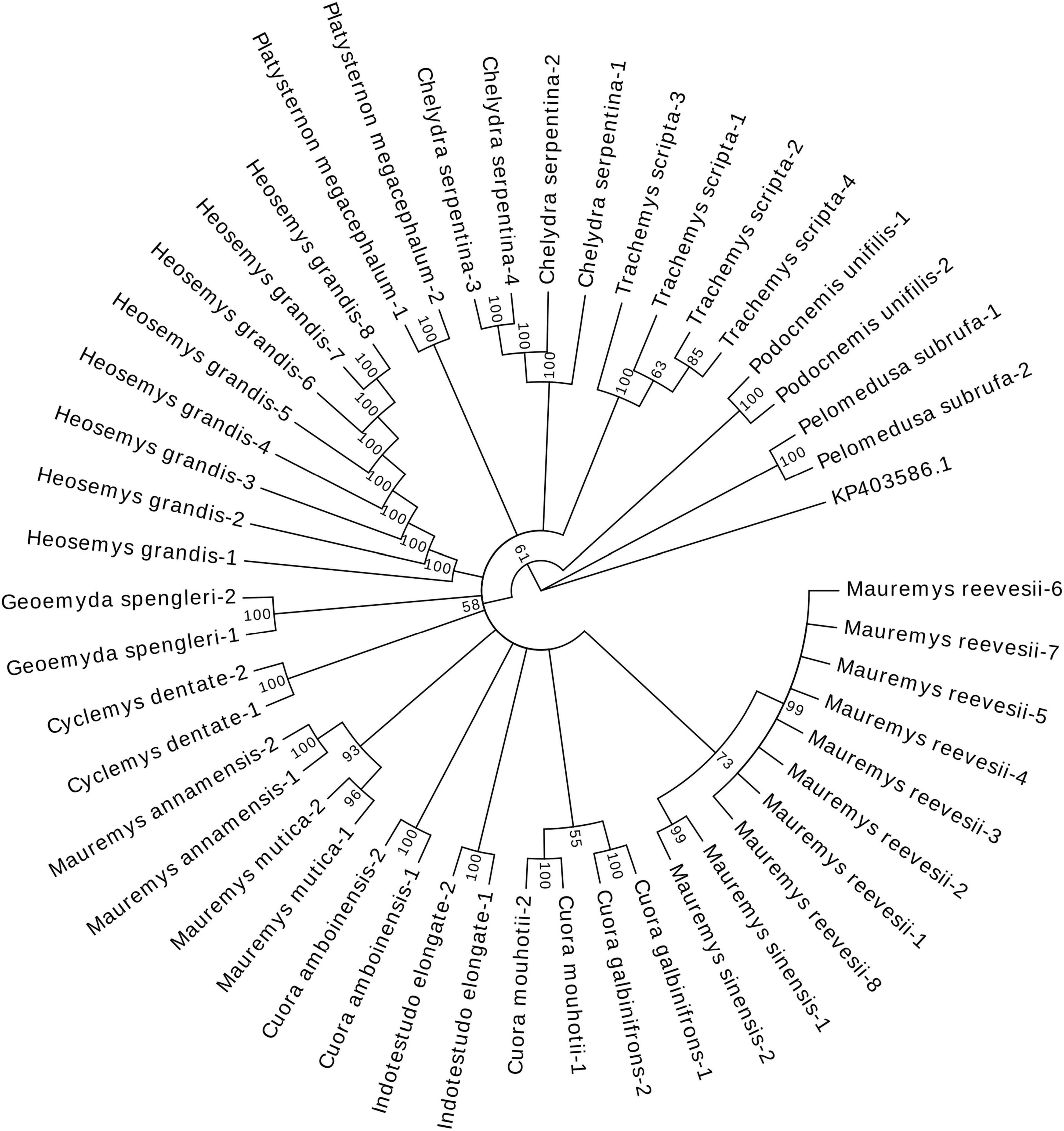
Figure 4. Results of the neighbor-joining tree based on HG sequences of the 48 turtle samples. The value represented the percentage of these branches in the bootstrap test, indicating whether the data adequately support their associated partitions. The closer to 100, the higher the credibility.
Verification of the Identification Efficiency of the Species-Specific Primers
Experiments on 8 genuine and 40 counterfeit samples confirmed that the species-specific primers HGTY had a 100% identification success rate and efficiency. Figure 5 depicted the outcome. The genuine product showed a single bright band at approximately 250 bp, while the bands of counterfeit species were faint and messy. Since the gel result of Heosemys grandis was a single band with no non-specific amplification, they could be used as a positive control to see if the sample is genuine. In summary, the identification system based on the species-specific primers HGTY effectively distinguished Heosemys grandis from other turtle species.
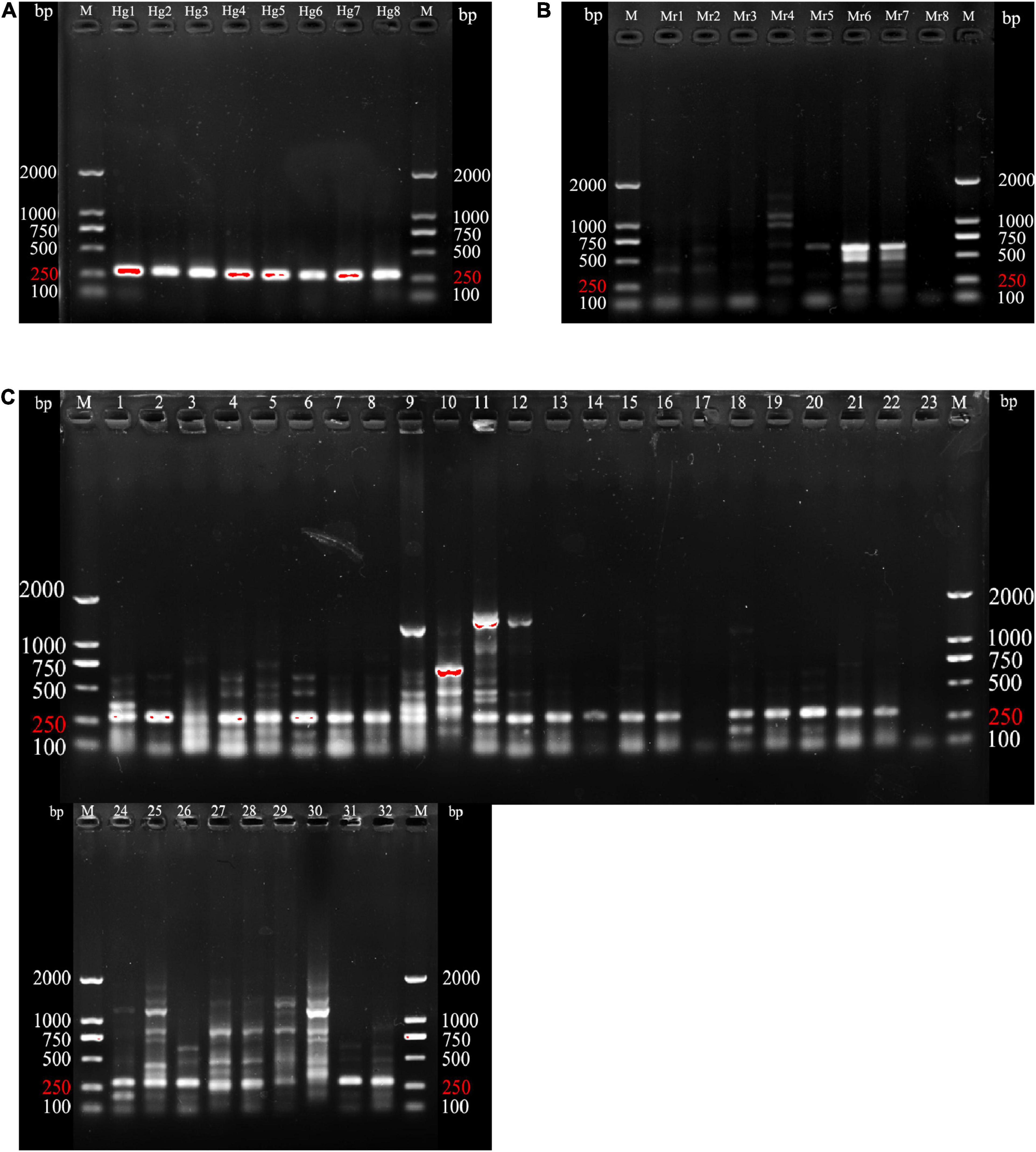
Figure 5. PCR product agarose gel results for 8 genuine and 40 counterfeit samples using HGTY. (A) Agarose gel electrophoresis of 8 genuine samples (Hg1-Hg8). (B) Agarose gel electrophoresis of 8 M. reevesii samples (Mr1-Mr8) species-specific primers HGTY1. (C) Agarose gel electrophoresis of the species-specific primers HGTY1 with 32 counterfeit species samples (1–4: C. serpentina; 5–6: C. galbinifrons; 7–8: I. elongata; 9–10: P. megacephalum; 11–14: T. scripta; 15–16: P. subrufa; 17–18: C. amboinensis; 19–20: M. sinensis; 21–22: C. mouhotii; 23–24: C. dentata; 25–26: G. spengleri; 27–28: M. mutica; 29–30: M. annamensis; 31–32: P. unifilis). M, DNA ladders.
Discussion
Because Heosemys grandis can be eaten and used as medicine, it has a significant market potential (Wang et al., 2004; Lan et al., 2012). As a result, market demand has increased, and various counterfeits have appeared, potentially disrupting the market, reducing clinic effectiveness, and affecting public safety. This research proposed combining DNA mini-barcoding and species-specific primers PCR technology to address the issues above. Common turtle species from East and Southeast Asia markets were used as test subjects. These turtles have a wide range of distribution, including Southeast Asia, America, and Africa. The study’s species breadth was enough for practical application. In a word, the identification system could serve as an effective instrument for market surveillance of Heosemys grandis.
Both DNA mini-barcoding identification and species-specific primers identification approaches have certain advantages and limitations. Through DNA sequencing, the DNA mini-barcoding identification method can obtain the DNA sequence of a specific location in a sample and properly identify the species of an unknown source sample (Chen et al., 2021). However, the DNA sequencing experiment cycle is long (over 36 h) and requires an additional expenditure (Shokralla et al., 2015). It displays a lack of economy and timeliness. Through the gel results of agarose gel electrophoresis, the species-specific primers directly identify whether the sample is a genuine species. Still, source information of the sample cannot be retrieved. The experiment can be carried out in a general molecular biology laboratory. Species-specific primers have superior timeliness and cost-effectiveness, but it lacks the accuracy of the DNA barcode identification approach. Although species-specific primers can meet most identification requirements, it isn’t easy to totally eliminate false-positive and false-negative findings. Therefore, we recommend that species-specific primers be used as the primary method in practical applications, with DNA mini-barcoding as a supplementation. When the gel image results are hazy or crucial samples are being used, it is preferable to employ the DNA mini-barcoding identification method to determine the sample’s accurate species source.
The complementarity of primers and sequences was a major consideration when designing primers (Supplementary Table 1). The complementarity of species-specific primers and genuine products should, on average, be better than that of counterfeit products. In addition to complementarity, amplification conditions are also related to the presence or absence of non-specific amplification. Therefore, it is necessary to optimize the amplification conditions before evaluating the primers. In particular, species-specific primers must be tested simultaneously with genuine and counterfeit products to eliminate external interference such as instruments and optimize amplification conditions where only authentic products can produce a single bright band.
The ideal DNA barcode sequence should have significant interspecific and sufficiently small intraspecific variations, and its minimum variation between species should exceed the maximum variation within species (Chase et al., 2007). The lowest interspecific distances of COI and HG were bigger than the intraspecific genetic distances. There are no areas where intraspecific and interspecific genetic distances overlap. This result suggested that both COI and HG are ideal DNA barcodes.
In the food and medicine industries, identifying the correct species source has become a critical problem. It’s difficult for untrained persons to identify samples using traditional methods, and amplifying DNA from processed products is challenging. This study clarified developing a reliable and rapid method for identifying Heosemys grandis based on COI. We designed species-specific primers to quickly identify Heosemys grandis to lower identification costs, save time, and enhance identification efficiency. Simultaneously, we created a DNA mini-barcoding as a complement to avoid the occurrence of false results. This method combines DNA mini-barcoding and species-specific primers PCR technology, broadens the breadth of molecular biology technology’s application in pharmacognosy, and provides an effective way for food and medication identification and quality monitoring. Meanwhile, the technology aids in regulating and controlling raw materials and processed products and provides an effective tool for protecting consumer health and reducing market fraud.
Data Availability Statement
The datasets presented in this study can be found in online repositories. The names of the repository/repositories and accession number(s) can be found below: NCBI (accessions: OL678057-OL678072, OL678459-OL678474, OM937962-OM937993, and OM945813-OM945844).
Ethics Statement
This study was approved by the Ethics Committee of Experimental Animals of Beijing University of Chinese Medicine.
Author Contributions
CL and LH designed the study. PL and DL performed the experiments and wrote the manuscript. YH collected samples. MC and XZ provided technical assistance. All authors read and approved the final manuscript.
Funding
This work was supported by the Development of Identification Method of Heosemys grandis (2020071720328), a project of Beijing University of Chinese Medicine-Zhejiang Yangshengtang Institute of Natural Medication Co., Ltd.
Conflict of Interest
DL, XZ, and LH were employed by Zhejiang Yangshengtang Institute of Natural Medication Co., Ltd. YH was employed by Yangshengtang Pharmaceutical Co., Ltd.
The remaining authors declare that the research was conducted in the absence of any commercial or financial relationships that could be construed as a potential conflict of interest.
The reviewer LX declared a shared affiliation with several of the authors PL, MC, and CL at the time of review.
Publisher’s Note
All claims expressed in this article are solely those of the authors and do not necessarily represent those of their affiliated organizations, or those of the publisher, the editors and the reviewers. Any product that may be evaluated in this article, or claim that may be made by its manufacturer, is not guaranteed or endorsed by the publisher.
Acknowledgments
We would like to thank CL of Beijing University of Chinese Medicine for the species identification. We would also like to thank American Journal Experts (AJE) for its linguistic assistance during the preparation of this manuscript.
Supplementary Material
The Supplementary Material for this article can be found online at: https://www.frontiersin.org/articles/10.3389/fevo.2022.822871/full#supplementary-material
Footnotes
References
Agee, A. F., and Barthet, M. M. (2021). Development of species-specific primers for rapid identification by PCR of the ecologically important pathogen Fusarium keratoplasticum from isolated and environmental samples. Lett. Appl. Microbiol. 73, 607–615. doi: 10.1111/lam.13545
An, H. S., Kim, M. J., Lee, J. W., and Lee, W. O. (2012). Molecular identification of Korean catfish (Siluriformes) based on two genetic markers. Genes Genomics 34, 695–702.
Chase, M. W., Cowan, R. S., Hollingsworth, P. M., van den Berg, C., Madrinan, S., Petersen, G., et al. (2007). A proposal for a standardised protocol to barcode all land plants. Taxon 56, 295–299. doi: 10.1371/journal.pone.0034089
Che, J., Chen, H. M., Yang, J. X., Jin, J. Q., Jiang, K., Yuan, Z. Y., et al. (2012). Universal COI primers for DNA barcoding amphibians. Mol. Ecol. Res. 12, 247–258. doi: 10.1111/j.1755-0998.2011.03090.x
Chen, C. T., Ding, Y. F., Jiang, Z. H., Jiang, H., Lu, C. Z., Zhang, L. L., et al. (2021). DNA barcoding of yellow croakers (Larimichthys spp.) and morphologically similar fish species for authentication. Food Control 127:108087.
Chen, M. Y., Han, X., Ma, J., Liu, X. Y., Li, M., Lv, X. N., et al. (2018). Identification of Plastrum Testudinis used in traditional medicine with DNA mini-barcodes. Rev. Bras. Farmacogn. 28, 267–272.
Fan, C., and Lv, G. (1999). Study on the immune effect of Guibie Pills on experimental mice. Chin. Tradit. Pat. Med. 21:4.
Gargouri, H., Moalla, N., and Kacem, H. H. (2021). PCR-RFLP and species-specific PCR efficiency for the identification of adulteries in meat and meat products. Eur. Food Res. Technol. 247, 2183–2192.
Hellberg, R. S., Hernandez, B. C., and Hernandez, E. L. (2017). Identification of meat and poultry species in food products using DNA barcoding. Food Control 80, 23–28.
Huang, M., Xiong, X., Yuan, F., Cao, M., Lu, L., and Xiong, X. (2021). Application of mini DNA barcoding in species identification of aquatic products. Chin. J. Bioprocess Eng. 19:8.
Lan, Z.-Q., Xian-Ming, L., Gui-Hua, J., and Th, H. (2012). Prelimilary Market Research Report of Guijia in Sichuan. Anhui. Hebei. Jiangxi in China. J. Chengdu Univ. TCM 35, 34–36.
Lintner, M., Weissenbacher, A., and Heiss, E. (2012). The Oropharyngeal morphology in the semiaquatic giant Asian Pond Turtle, Heosemys grandis, and its evolutionary implications. PLoS One 7:e46344. doi: 10.1371/journal.pone.0046344
Lv, Y. C., Zheng, R., Zuo, T., Wang, Y. M., Li, Z. J., Xue, Y., et al. (2014). Identification of five sea cucumber species through PCR-RFLP Analysis. J. Ocean Univ. China 13, 825–829.
Ma, H. T., Gao, H. M., Zhang, Y., Qin, Y. P., Xiang, Z. M., Li, J., et al. (2021). Multiplex species-specific PCR identification of native giant clams in the South China Sea: a useful tool for application in giant clam stock management and forensic identification. Aquaculture 531:735991. doi: 10.1016/j.aquaculture.2020.735991
Natonek-Wisniewska, M., and Slota, E. (2008). Polymorphism of the cytB gene as a marker for species identification of mammalian mtDNA. Ann. Anim. Sci. 8, 243–247.
Pinheiro, R. S. B., Ramos, P. R. R., de O Roça, R., Bezerra, L. R., Francisco, C. L., and Oliveira, R. L. (2020). Differences between cattle and buffalo in the water-soluble proteins of the Longissimus muscle as shown by electrophoretic techniques. Anim. Prod. Sci. 60, 1759–1768. doi: 10.1071/an19239
Shokralla, S., Hellberg, R. S., Handy, S. M., King, I., and Hajibabaei, M. (2015). A DNA Mini-Barcoding system for authentication of processed fish products. Sci. Rep. 5:15894. doi: 10.1038/srep15894
Silva, A. J., Kawalek, M., Williams-Hill, D. M., and Hellberg, R. S. (2020). PCR cloning combined With DNA barcoding enables partial identification of fish species in a mixed-species product. Front. Ecol. Evol. 8:28. doi: 10.3389/fevo.2020.00028
Su, Y., Ding, D., Yao, M., Wu, L., Dong, G., Zhang, D., et al. (2020). Specific DNA mini-barcoding for identification of Gekko gecko and its products. Chin. Med. 15:103. doi: 10.1186/s13020-020-00382-2
Tan, C. K., Selamat, J., Jambari, N. N., Sukor, R., Murugesu, S., and Khatib, A. (2021). Muscle and serum metabolomics for different chicken breeds under commercial conditions by GC-MS. Foods 10:2174. doi: 10.3390/foods10092174
Wang, Z., Gong, S., Shi, H., Zhang, Y., and Xu, J. (2004). Survey on turtle trade in haikou. hainan province. China. Sichuan J. Zool. 24, 414–417.
Yu, H., and Xia, X. (2000). Severely crack down on the production and sale of fake and shoddy commodities to protect the legitimate rights and interests of consumers. Mark. Regul. 2, 37–38.
Yu, H., and Xia, X. (2004). Multidimensional analysis of the existence of counterfeit and shoddy goods. J. Hubei Univ. 31:6.
Keywords: Heosemys grandis, COI, DNA mini-barcoding, species-specific primers, identification
Citation: Li P, Li D, Hong Y, Chen M, Zhang X, Hu L and Liu C (2022) Combining DNA Mini-Barcoding and Species-Specific Primers PCR Technology for Identification of Heosemys grandis. Front. Ecol. Evol. 10:822871. doi: 10.3389/fevo.2022.822871
Received: 26 November 2021; Accepted: 18 February 2022;
Published: 05 April 2022.
Edited by:
Claudio Oliveira, São Paulo State University, BrazilReviewed by:
Anthony Joseph Silva, Chapman University, United StatesZhi Chao, Southern Medical University, China
Li Xiang, China Academy of Chinese Medical Sciences, China
Panagiotis Madesis, University of Thessaly, Greece
Jing Jia, Affiliated Hospital of Shandong University of Traditional Chinese Medicine, China
Copyright © 2022 Li, Li, Hong, Chen, Zhang, Hu and Liu. This is an open-access article distributed under the terms of the Creative Commons Attribution License (CC BY). The use, distribution or reproduction in other forums is permitted, provided the original author(s) and the copyright owner(s) are credited and that the original publication in this journal is cited, in accordance with accepted academic practice. No use, distribution or reproduction is permitted which does not comply with these terms.
*Correspondence: Liu Hu, lhu@mail.yst.com.cn; Chunsheng Liu, max_liucs@263.net
†These authors have contributed equally to this work and share first authorship