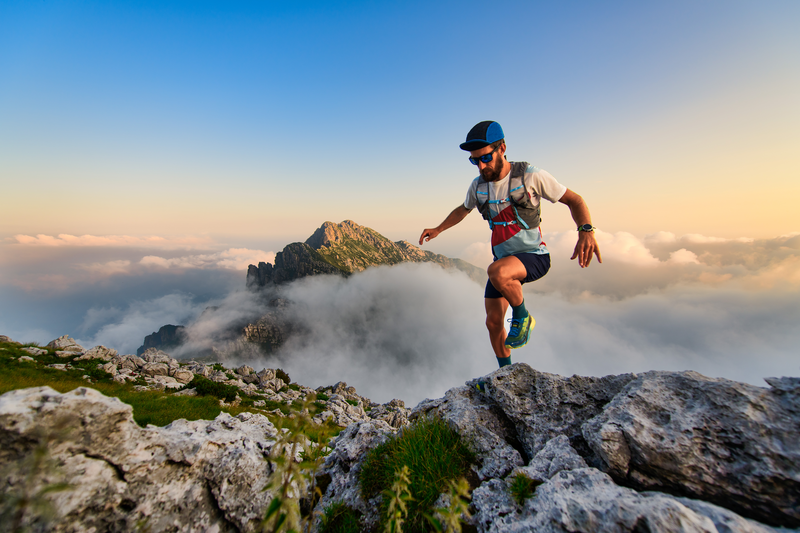
95% of researchers rate our articles as excellent or good
Learn more about the work of our research integrity team to safeguard the quality of each article we publish.
Find out more
ORIGINAL RESEARCH article
Front. Ecol. Evol. , 24 February 2022
Sec. Biogeography and Macroecology
Volume 10 - 2022 | https://doi.org/10.3389/fevo.2022.723286
This article is part of the Research Topic Temporal and Large-Scale Spatial Patterns of Plant Diversity and Diversification View all 10 articles
The Brazilian Caatinga is considered the richest nucleus of the Seasonally Dry Tropical Forests (SDTF) in the Neotropics, also exhibiting high levels of endemism, but the timing of origin and the evolutionary causes of its plant diversification are still poorly understood. In this study, we integrate comprehensive sampled dated molecular phylogenies of multiple flowering plant groups and estimations of ancestral areas to elucidate the forces driving diversification and historical assembly in the Caatinga flowering plants. Our results show a pervasive floristic exchange between Caatinga and other neotropical regions, particularly those adjacent. While some Caatinga lineages arose in the Eocene/Oligocene, most dry-adapted endemic plant lineages found in region emerged from the middle to late Miocene until the Pleistocene, indicating that only during this period the Caatinga started to coalesce into a SDTF like we see today. Our findings are temporally congruent with global and regional aridification events and extensive denudation of thick layers of sediments in Northeast (NE) Brazil. We hypothesize that global aridification processes have played important role in the ancient plant assembly and long-term Caatinga SDTF biome stability, whereas climate-induced vegetation shifts, as well as the newly opened habitats have largely contributed as drivers of in situ diversification in the region. Patterns of phylogenetic relatedness of Caatinga endemic clades revealed that much modern species diversity has originated in situ and likely evolved via recent (Pliocene/Pleistocene) ecological specialization triggered by increased environmental heterogeneity and the exhumation of edaphically disparate substrates. The continuous assembly of dry-adapted flora of the Caatinga has been complex, adding to growing evidence that the origins and historical assembly of the distinct SDTF patches are idiosyncratic across the Neotropics, driven not just by continental-scale processes but also by unique features of regional-scale geological history.
The Brazilian Caatinga is recognized as the largest and most species rich nucleus of the Seasonally Dry Tropical Forest (SDTF) biome in the New World (Pennington et al., 2000; Banda et al., 2016; Queiroz et al., 2017; Fernandes et al., 2020), yet it is one of the least studied neotropical biogeographic regions (Fiaschi and Pirani, 2009). The highly heterogeneous gradients of topography, geology, soils, and the spatial and temporal distribution of rainfall support a wide variety of dry-adapted plant communities in the region (Velloso et al., 2002; Silva and Souza, 2018). In particular, the nature of geological parent material composing the soils is suggested to be the most important constraint to plant diversity and endemism, producing an impressive turnover in the distribution of species and, consequently defining the arrangement of the floristic sets (Gomes et al., 2006; Cardoso and Queiroz, 2007; Santos et al., 2012; Moro et al., 2016; Queiroz et al., 2017). Caatinga plant communities have been classically divided into: (i) Crystalline Caatinga, established over fertile-soils primarily derived from Precambrian crystalline basement of the Depressão Sertaneja, ranging from woodlands (Caatinga s.s.) on driest sites to tall forests (Arboreal Caatinga) on moister areas; (ii) Sedimentary Caatinga, open scrub occupying the patchily distributed nutrient-poor sandy soils of ancient sedimentary basins and continental sand dunes and; (iii) the vegetation established over the karst deposits of the Bambuí and Jandaíra groups (Figures 1A–F; Queiroz, 2006; Moro et al., 2016; Queiroz et al., 2017).
Figure 1. Vegetation structure variation throughout the three main geological types of the Caatinga seasonally dry forests. The small side map shows the distribution of the Caatinga in Northeast Brazil in the context of the Seasonally Dry Tropical Forest biome (SDTF) in the Neotropics (Queiroz et al., 2017). The Depressão Sertaneja (red; A,B) is the most dominant and continuously distributed formation, while the Sedimentary Caatinga (pale yellow; C,D) and Karst Caatinga (gray; E,F) are patchily distributed. Representative images highlight the structurally heterogenous vegetation, with exposed crystalline rocks, white-sand soil, or limestones, from across these main geological formations at: (A) Parnamirim, Bahia, showing the Arboreal Caatinga with a leafless large tree of the Brazilian baobab Cavanillesia umbellata (Malvaceae); (B) Sobral, Ceará, showing a more open Crystalline Caatinga woodland with an individual of the dominant legume tree Luetzelburgia auriculata and the Caatinga endemic Pilosocereus chrysostele (Cactaceae); (C) Morro do Chapéu, Bahia, showing a Sedimentary Caatinga with the yellow-flowered shrub Allamanda puberula; (D) Barra, Bahia, showing a sand dune of the mid São Francisco River valley with the endemic opuntioid cactus Tacinga inamoena; (E) Parque Nacional Carvernas do Peruaçu, Minas Gerais, showing the exposed razor-sharp limestone outcrops, dominated by individuals of the Bromeliaceae Encholirium luxor and the karst endemic Allamanda calcicola; (F) Morro do Chapéu, Bahia, with individuals of the barrel Cactaceae Melocactus pachyacanthus, and the Caatinga endemic Encholirium spectabile and the columnar Cactaceae Xiquexique gounellei. All photos by Domingos Cardoso.
The development of the Caatinga’s diverse geomorphological systems has been mostly the result of large-scale regional denudation from the Miocene onward (Figure 2; Ab’Sáber, 1974; Peulvast and Claudino-Sales, 2004; Valadão, 2009; Japsen et al., 2012), caused by neotectonic reactivation (Oliveira and Medeiros, 2012; Rodríguez-Tribaldos et al., 2017; Klöckinga et al., 2020) and a series of global and regional events that exacerbated aridity (Zachos et al., 2001). For example, the rise of the Borborema Plateau at the eastern limits of the Caatinga formed an orographic barrier to air masses entering from the Atlantic Ocean, creating a rain shadow effect that drastically altered temperature and precipitation regimes in the region (Gottsberger and Silberbauer-Gottsberger, 2006; Hoorn et al., 2014). Ultimately, increased aridity resulted in massive erosion of a thick sedimentary cover accumulated during wetter and warmer climatic conditions of the Early Cenozoic (Figure 2A). This process culminated in the exhumation of the Paleo-Mesozoic sediments and the formation of the large-scale, lower-level peneplain of the Depressão Sertaneja (Figure 2B). Yet, post-Miocene uplift within the Araripe Plateau led to widespread fluvial incision (Peulvast et al., 2008) and dramatic changes in the course of the São Francisco River (once flowing toward equatorial Atlantic Ocean), creating an endorheic system (Figure 2C; Mabesoone, 1994). Later, in the Pleistocene, when the river acquired its current course toward eastern Atlantic, large amounts of sand accumulated in this “paleo-lake” were reshaped by wind, forming the modern São Francisco River dune system (Figure 2D; Barreto and Suguio, 1993; Mabesoone, 1994; Barreto, 1996). Also in the Pleistocene, the large karst systems belonging to Bambuí and Jandaíra groups were exhumed (Silva et al., 2017), adding to the increased environmental heterogeneity in the Caatinga (Figure 2D).
Figure 2. Paleogeographic maps of the transition from the widespread paleo-sedimentary surface to modern heterogeneous Caatinga landscapes. (A) The sedimentary cover accumulated during previous times is maintained in the Caatinga due to the widespread dominance of tropical rainforests over the region at Early Cenozoic (Fine and Ree, 2006; Jaramillo and Cardenas, 2012). (B) Global climate changes and increased uplift and erosion at the Miocene (Rodríguez-Tribaldos et al., 2017; Klöckinga et al., 2020) exposed the Paleo-Mesozoic sediments accumulated due to post-rift sedimentation–after fragmentation of Gondwana supercontinent (Magnavita et al., 1994; Maia et al., 2010; Bezerra et al., 2011; Gurgel et al., 2013) and generated a new, low-level peneplain, known as Depressão Sertaneja. (C) Pliocene tectonic reactivation within the Araripe Plateau changed the course of São Francisco (SF) River, which had a paleo-course flowing toward the equatorial Atlantic; this event created an endorheic drainage at the central region of the Caatinga (Mabesoone, 1994). (D) In the Pleistocene, the lacustrine sediments, which remained after the São Francisco River acquired its current watercourse, were remodeled by wind and formed a system of continental dunes that is currently renowned by a highly endemic biota (Rocha et al., 2004; Queiroz et al., 2017). Also at this period, most of the karst systems were also exhumed (Silva et al., 2017).
While major geological transformations have been relatively well characterized in the Caatinga, little is known about the origins and evolution of the Caatinga biota, particularly how the chronology of major geological and climatic events impacted its diverse and endemic plant assemblages. The Caatinga flora was long supposed to be composed of a minor subset of drought-tolerant elements from surrounding biomes (see Rizzini, 1979; Andrade-Lima, 1981). Other authorities interpreted it as derived from lineages occupying other nuclei of the neotropical SDTF (Sarmiento, 1975; Prado and Gibbs, 1993; Pennington et al., 2000) or the Chaco of southern South America (Andrade-Lima, 1982). An alternative, more integrative hypothesis that took into account the complex geological evolution of the Brazilian Northeast (NE) region was proposed by Queiroz (2006), which suggested that fragmentation of the previously continuous sedimentary cover would have promoted vicariance of isolated populations during the Miocene. Such fragmentation exposed the fertile-soils of the Depressão Sertaneja, allowing massive migration of dry-adapted elements that originated in other neotropical SDTF. A major issue with Queiroz’s (2006) propositions, however, is that he considered peneplanation (long-term erosion that generated Caatinga stepped relief) as a single, temporally defined (Miocene) event, greatly contrasting with subsequent geological evidence of multiple events of massive erosion taking place over discontinuous periods (e.g., Valadão, 2009; Japsen et al., 2012). This more complex geological history suggests that Queiroz’s (2006) hypothesis about the timing of origin and historical processes that shaped modern Caatinga’s plant diversity deserves new scrutiny using rigorous phylogenetic testing.
Improved understanding of the timing and forces underlying species assembly and diversification in the Caatinga is not just limited and incomplete but has also been challenging because only few Caatinga plant lineages had DNA sequence data available or were included in molecular phylogenetic studies. Over the last decade, new densely sampled phylogenies of key Caatinga lineages have been published (e.g., Queiroz et al., 2010; Simon et al., 2011; Cardoso et al., 2013; Carvalho-Sobrinho et al., 2016; Almeida et al., 2018; Maya-Lastra and Steinmann, 2019), all of which now provide an excellent opportunity to evaluate the impact of the complex geological and climatic history on the Caatinga biodiversity and to unveil the spatial and temporal dynamics underlying floristic exchanges between the Caatinga SDTF and other neotropical regions. By exploring and comparing the biogeographical history of multiple, unrelated taxonomic groups during the complex landscape evolution of the Caatinga (Figure 2), here we also address whether (i) the Caatinga plant lineages constitute a subset of the surrounding flora (Rizzini, 1979; Andrade-Lima, 1981, 1982) and (ii) endemic lineages have emerged by Miocene vicariance (Queiroz, 2006). By analysing plant lineage diversification in a geological-evolutionary perspective that takes into account the synergistic effect of landscape and biological evolution, we expect to provide a series of more focused pictures of the historical assembly of the Caatinga SDTF.
We used the updated checklist of flowering plants of the Caatinga SDTF (Fernandes et al., 2020) to identify a set of lineages that are taxonomically and ecologically important in the dry formations of the region, i.e., representative plant clades in terms of species richness, endemism, or ecological dominance. We then compiled from the literature a set of densely sampled species-level phylogenies from the target lineages. Our final dataset encompasses 20 monophyletic groups, which include a total of 150 Caatinga-inhabiting species, from which 95 are endemics. By sampling widely across several families of flowering plants and a wide range of life forms (e.g., herbs and trees), we maximize the inclusion of lineages with contrasting biogeographical histories that may have colonized the Caatinga by different routes and at different times, providing a multi-taxon picture of plant evolution in that region.
We retrieved complete alignments or individual DNA sequences for each selected lineage from TreeBASE1 (Piel et al., 2009) and GenBank2 (Benson et al., 2013), respectively (see Supplementary Table 1 for details on study groups, genes, and sources and Supplementary Table 2 for GenBank accessions). For downstream dating analyses, the originally retrieved TreeBASE alignments were not modified, whereas sequences of each individual region retrieved from GenBank were first aligned automatically using MAFFT (Katoh and Standley, 2013) with default settings and then, manually adjusted in Geneious (Drummond et al., 2012a).
Phylogenetic relationships and divergence times were estimated using a Bayesian approach in BEAST v1.8.4 (Drummond et al., 2012b) as implemented in the CIPRES Science Gateway (Miller et al., 2010). We ran analyses using the Yule speciation model and uncorrelated relaxed molecular clock with a lognormal prior distribution. We estimated the best-fit nucleotide substitution models using AIC (Akaike Information Criterion) in jModelTest2 (Darriba et al., 2012). Depending on the availability of reliable fossil records for each group, we used primary (fossil) or secondary (in the absence of reliable fossils) calibration points, with lognormal and normal prior distributions, respectively (Supplementary Table 3 details the calibration points). We performed a minimum of two independent runs of 107 generations of MCMC (Markov chain Monte Carlo), sampling parameters every 104 generations. Convergence of the runs was evaluated in Tracer v1.6 (Rambaut and Drummond, 2013) to ensure that effective sample sizes (ESS) reached values higher than 200 for all parameters. After the exclusion of 25% of the initial trees (burn-in) of each independent run, we combined tree files in LogCombiner v.1.8.2 (Drummond et al., 2012b) and used TreeAnnotator v1.8.4 (Drummond et al., 2012b) to summarize results into maximum clade credibility (MCC) trees. Prior to ancestral biome reconstruction analyses all outgroups were pruned from phylogenies.
Defining biogeographical units for ancestral area reconstruction is a difficult task. Because they are highly dependent on the lineages under study, there is no universal way to define them. There are also multiple limitations associated with choosing a reasonable number of operational units to be used in the analyses, leading to highly polymorphic states (occurrence in multiple areas). Taking this into account, we aimed to define sets of regions that are biologically meaningful for all lineages under analysis, reflecting geography and the geological history.
We produced a classification for the New World based on previous regionalization proposed by Morrone (2014). Our classification is composed of nine regions (Figure 3), and includes: two regions dominated by tropical rainforests (Amazon, Atlantic Forest); one dominated by grass-rich and fire-prone savannas (Cerrado + Pampas, which here also includes the higher elevations of the Chapada Diamantina mountain range, in the core of the Caatinga dry matrix and also the southern grasslands); the Caatinga, characterized by fire-sensitive, succulent-rich formations associated with the global SDTF biome; the Chaco, a region with great temperature seasonality that experiences regular frost, and potentially represents a distinct biome with more temperate affinities (Prado and Gibbs, 1993; Banda et al., 2016; Miranda et al., 2018); the other four regions (Andes, Caribbean Islands, North America, and Northern South America) are composed by a mix of distinct biome types. Since our aim is to assess the evolution of the dry-adapted flora, we considered Caatinga in its narrow sense (sensu Fernandes et al., 2020), including only vegetation types connected to the SDTF biome, which encompasses distinct formations that mostly occur on areas of low altitude (below 1,000 m) in the region. In this context, species occurring at the rainforest enclaves (locally known as brejos de altitude) were coded as Atlantic Domain, and those recorded for cerrado enclaves and campos rupestres, mostly occurring on the Chapada Diamantina were coded as Cerrado. Despite the disjunct distribution of the Cerrado and the Pampas (phytogeographic region located in Southern Brazil and Uruguay), both regions are characterized by fire-prone grasslands, and their lineages exhibit evident evolutionary affinities (see Simon et al. (2011)), which justifies their clustering into a single region.
Figure 3. Distribution of major areas used in our ancestral reconstructions based on reclassification of Morrone (2014) biogeographical regionalization of Neotropics and the floristic interchange among the Caatinga and other regions as estimated from DEC + j reconstructions for our sample composed of 20 lineages. Colors of the map and the chord-graph coincide and indicate the areas referred in the section “Materials and Methods.” Shapefile from Löwenberg-Neto (2014).
The current distribution of each species within the areas and biomes were obtained primarily from specialized literature (taxonomic monographic works and floras), but we also used information available in Flora do Brasil 2020.3 We coded all species in our phylogenies as present or absent within these regions.
We estimated ancestral areas through a likelihood approach as implemented in the R (R Core Team, 2020) package BioGeoBEARS (BioGeography with Bayesian, and Likelihood, Evolutionary Analysis in R Scripts - Matzke, 2013). We performed analyses using Dispersal-Extinction-Cladogenesis (DEC) model and the DEC + j model, which incorporates the jump parameter (j) that allows for a descendent lineage to occupy a new area that is different from its parental lineage by a founder event. We restricted the maximum number of areas of each analysis to the same as that the most widespread species in the phylogeny were distributed. As a rule, DEC + j reconstructions gave the highest likelihood values and, because of this, they will be discussed in this text. To quantify the number of transitions from all regions into Caatinga and from the Caatinga to all other regions, we counted the biogeographical events as inferred by our DEC + j reconstructions.
We identified a total of 175 events involving the Caatinga across all lineages. Approximately 65% (114 events) of this total represent biogeographical shifts, whereas the 35% (61 events) are related to in situ speciation (Supplementary Table 4). The majority of biogeographical shifts (73 events, or 64% of total shifts) represent transitions into the Caatinga and, only 41 events (36%) represent shifts from the Caatinga into other regions (Figure 3). Atlantic Forest and Cerrado + Pampas were the most important regions in terms of plant lineage exchange. Together, they account for 61% of all shifts into the Caatinga and 81% of total shifts from the Caatinga. The Amazon and North America exhibited moderate floristic exchange with the Caatinga, whereas the Andean region, Caribbean Islands, Chaco, and Northern South America were involved in few exchange events (Figure 3; see Supplementary Figure 1 and Supplementary Table 4 for further details on the types, areas, and ages involved in each of these biogeographical events as well as the ancestral area estimations). These numbers clearly demonstrate multiple independent origins for Caatinga plant lineages, and also show asymmetrical connectivity dynamics, in a way that Caatinga has received more lineages than being a source of plant diversity to other regions.
Temporal patterns show that colonization of the Caatinga by lineages from other regions precede events of in situ speciation (Figure 4A). They also show that the relevance of cladogenetic shifts (those generating endemic species through isolation of lineages in the Caatinga) from distinct regions changed markedly over evolutionary time (Figures 4B,C). Early divergence that formed the initial species pool of elements adapted to the seasonally dry conditions of the Caatinga started as early as the Eocene-Oligocene (estimated mean age of 41.9 Ma for the oldest transition in Bombacoids), from ancestors primarily associated with regions largely characterized by the dominance of rainforests (Amazon and Atlantic Forest). However, these events are rare and, only after the mid-Miocene, particularly in the last 10 Ma, we found a general increase in the relative number of events isolating lineages in the Caatinga (Figure 4C). This increased Miocene lineage immigration that continues up to the Pleistocene came from regions that harbor major areas of seasonally dry and/or open biomes (Cerrado + Pampas and North America). We also identified a significant number of transitions from the Atlantic Forest to the Caatinga during the Miocene-Pleistocene periods. However, many of these cases are lineages (e.g., Amorimia, Luetzelburgia, and Pereskia) that are largely confined to the drier formations within the Atlantic Forest region and so they perhaps are better characterized as phylogenetic biome conservatism than evolutionary biome-shift events.
Figure 4. Temporal patterns of biogeographical events generating Caatinga endemic lineages. Boxplots summarize ages for nodes with (A) highest probabilities of cladogenetic shifts into the Caatinga and in situ speciation and; (B) cladogenetic shifts from regions that are important providers of lineages to the Caatinga. (C) Node ages and confidence intervals of cladogenetic shifts (both dispersal and vicariance) leading to Caatinga crown clades as estimated by a DEC + j model. Ages are in decreasing order and grouped by inferred ancestral areas. Colors correspond to the same shown in Figure 3. Vertical dotted red line represents the onset of uplift and erosion at 18–15 Ma according to Japsen et al. (2012). Vertical dotted black lines indicate the Pleistocene period.
Though significant, the number of independent colonization events of the Caatinga cannot fully account for its present-day plant diversity and our results (Supplementary Table 4) show that in situ speciation also played a key role in the evolution of the Caatinga flora, with most events having occurred within the last few million years (Figure 4A). In situ speciation events began in the late Miocene, with an increase at the Pliocene and peaking in the Pleistocene. From the Pliocene onward, in situ diversification became the dominant mode of species accumulation in the Caatinga (Supplementary Table 4), exceeding rates of transition from other regions. A common pattern that emerges from our analyses is that Caatinga endemic lineages generally represent small radiations of clades composed by a few endemic species, which are often restricted to distinct geological substrates (Figure 5). Therefore, after the early colonization and establishment of plant lineages in the Caatinga, many of them have diversified in situ, showing that relatively recent diversification has generated a great portion of endemic plant species that inhabit the region today.
Figure 5. BEAST-dated phylogenies showing examples of Caatinga endemic clades within the mostly SDTF endemic legume clades Coursetia (Queiroz and Lavin, 2011) and the Vataireoids (Cardoso et al., 2013; Cruz et al., 2018). Red dots indicate the nodes where isolation of lineages within the Caatinga occurs. Red lines indicate Caatinga endemic clades. Distinct name colors denote the type of geological substrate where the corresponding species is endemic: Arboreal Tall Caatinga (dark red); more open Crystalline Caatinga (light red); Sedimentary Caatinga (pale yellow); and Karst Caatinga (gray). Note that species do not group by geological substrate. Photos of Coursetia rostrata and Luetzelburgia neurocarpa by Domingos Cardoso.
The recruitment of dry-adapted Caatinga plant lineages was partly a by-product of complex dynamics of colonization from geographically adjacent areas. On one hand, our results reinforce a scenario of high biotic interchange among neotropical biogeographical regions and biomes (Queiroz et al., 2017; Antonelli et al., 2018). Thus, they partially support previous propositions (Rizzini, 1979; Andrade-Lima, 1981) that the Caatinga’s plant diversity originated from a subset of drought-tolerant elements coming from the surroundings, particularly the Atlantic Forest and the Cerrado. On the other hand, the small proportion of events involving the Caribbean (in its broadest sense, including both the Caribbean Islands and the northern coast of South America) and the Chaco indicates that floristic exchanges with these regions have been less significant than previously thought (e.g., Sarmiento, 1975; Andrade-Lima, 1982). The large size of the Caatinga, bordered by the ecologically distinct, species-rich formations of Amazonia, Atlantic Forests, and the Cerrado have likely impacted the high levels of their historical biotic exchange. The highly heterogeneous environments found within the Caatinga region, including scattered rainforest and savanna enclaves more biogeographically related to the Atlantic Forest and the Cerrado (Ratter et al., 2006; Queiroz et al., 2017; Silveira et al., 2019), facilitates exchange with these neighboring biomes. This likely accounts for high biological exchange, allowing the successful establishment of ecologically labile species from other regions within the Caatinga.
The environmental differences and physiological tolerances of lineages inhabiting distinct biomes may account for differences recovered by our ancestral biome reconstructions (Figure 3). Despite the long-term presence of tropical rainforests in South America (Burnham and Johnson, 2004; Wing et al., 2009), and hence time for lineages to adapt to emerging dry climatic conditions of Northeastern Brazil, shifting events from the Amazon into the Caatinga drylands have been infrequent. Even arrivals from the Atlantic Forest often derived from ancestors that occupied SDTF enclaves there (e.g., Amorimia and the genus Luetzelburgia within the Vataireoids), not the rainforest biome that largely characterizes the region. This suggests that rainforest species are not able to easily overcome the barriers imposed by such a seasonally dry ecology. Indeed, rainforest species are highly constrained by low water-use efficiency, which means susceptibility to drought-induced mortality (McDowell et al., 2008). Many savanna lineages, on the other hand, may already possess the eco-physiological adaptations needed to cope with a seasonally dry climate, which may explain the greater amount of immigration into the Caatinga of lineages that previously inhabited the Cerrado + Pampas region. Such an active role for climatic similarity is also reflected in mechanisms regulating community-level diversity patterns and greater floristic similarity between SDTF and savannas (Dexter et al., 2015; Fernandes et al., 2020; Segovia et al., 2020).
Our results indicate that the dry-adapted species that inhabit present-day Caatinga began to emerge during the Eocene-Oligocene, but the low proportion of elements with such an old age (only three were recorded for this period; Figure 4C) suggest that a SDTF community resembling a modern one may not have existed at that time. It is possible that such early dry-adapted species remained restricted to suitable niches scattered across the rainforest matrix that dominated most of South America (Fine and Ree, 2006; Jaramillo and Cardenas, 2012). The early presence of rainforest immigrants (from the Amazon and the Atlantic Forest) that formed the initial species pool of modern dry-adapted Caatinga plant lineages suggests a gradual replacement of rainforests by SDTF in the region. These lineages could have gained advantage from an early presence that allowed adaptation to developing more seasonal, arid climates. The more frequent transitions into the Caatinga after the mid-Miocene (Figure 4C) are temporally congruent with the establishment of semi-arid climate conditions in Northeastern South America (∼13 Ma; Harris and Mix, 2002) and the extensive process of geological denudation that culminated in the formation of the low-lying Depressão Sertaneja (Ab’Sáber, 1974; Japsen et al., 2012). The timing of diversification of SDTF plant lineages is dated to Miocene as evidenced from time-calibrated phylogenies (Arakaki et al., 2011) and from the fossil record (Burnham and Carranco, 2004). The high fraction of migrations into the Caatinga by lineages nested within clades largely restricted to SDTF (e.g., Amorimia, Coursetia, and Leuenbegeria) suggests niche conservatism as an important process in the historical assembly of the Caatinga endemic flora, as also observed at various spatial scales and ecological settings (Donoghue and Smith, 2004; Wiens and Donoghue, 2004; Ricklefs et al., 2006; Donoghue, 2008; Crisp et al., 2009), particularly in the SDTF (Schrire et al., 2005; Lavin, 2006; Pennington et al., 2006, 2009; Govindarajulu et al., 2011; Oliveira-Filho et al., 2013; Gagnon et al., 2019; Ringelberg et al., 2020). As argued by Queiroz (2006), the exposure of the nutrient rich soils that characterizes the Depressão Sertaneja, and are preferred by many SDTF species (Pennington et al., 2000, 2004), opened the way for greater migration and successful establishment of dry-adapted lineages with a SDTF ecology that originated elsewhere. Toward the Pliocene, as drier seasonal conditions became more pronounced in South America (Fedorov et al., 2013; Jaramillo et al., 2020) and suitable edaphic conditions more widespread, Caatinga probably coalesced into a seasonally dry community physiognomically similar to the present-day vegetation. This pre-Pleistocene immigration of SDTF elements into the Caatinga adds to growing evidence that historical exchanges between different fragments of the SDTF biome have been primarily driven by long-distance dispersal (Mayle, 2004; Pennington et al., 2004), rather than by the fragmentation of a wider historical formation due to Pleistocene climatic fluctuations (Prado and Gibbs, 1993; Pennington et al., 2000; Prado, 2000).
The observed evolutionary transitions during the Pleistocene involving lineages that inhabit a wide array of different ecologies (e.g., Bombacoids, Cereus, Ficus, and Manihot) indicate that climate-induced vegetational shifts in the recent past may have provided opportunities for evolutionary biome shifts. Fossil evidence (e.g., Oliveira et al., 1999) and paleoecological reconstructions (e.g., Auler et al., 2004; Wang et al., 2004; Arruda et al., 2017) for the Caatinga indicate large shifts in vegetation during this period, which potentially created a complex mosaic of vegetation types representing distinct biomes. Biome-shift events are critically dependent on the spatial adjacency and dimensions of boundaries between biomes over time (Donoghue and Edwards, 2014). The historical climatic instability within the Caatinga might have promoted vegetation shifts that favored the exchange of lineages with other regions, resulting in the relatively high levels of transitions for particular plant clades, and thus facilitating the mixing of lineages with distinct geographical distributions and ecological preferences.
In the Caatinga, the influence of geology is not only reflected in the impressive species turnover even at local scale, but also on the disproportional contribution of species that are specialist to particular edaphic conditions to regional plant diversity and endemism (Queiroz, 2006; Moro et al., 2016; Queiroz et al., 2017; Fernandes et al., 2020). Such an influence of geological features on plant distribution patterns led Queiroz (2006) to postulate that a great proportion of Caatinga endemics had been generated by allopatric (vicariance) speciation due to Miocene fragmentation of the previously continuous sedimentary surface. This hypothesis clearly implies that pairs of sandy-soil species should occupy disjunct sedimentary basins, and their split should be dated within the Miocene.
General patterns that emerged from our dated phylogenies (Figure 5) largely rejected Queiroz’s (2006) expectations. Rather, they suggest that parallel in situ diversification of plant lineages has probably evolved by fine-scale niche differentiation, reflecting adaptations of the regional flora to different conditions over evolutionary timescales. The time span of in situ speciation events coincides with changes in the geology of the Caatinga. Post-Miocene uplifting of the Araripe plateau, for example, induced dramatic changes in the course of the São Francisco River and the formation of an endorheic drainage (with no outflow to the ocean) in its middle course (Mabesoone, 1994; Maia et al., 2010; Barros-Corrêa et al., 2019). As a result of sedimentation of this ancient endorheic drainage and posterior action of wind, a system of continental sand dunes was formed later in the Pleistocene (Barreto and Suguio, 1993; Mabesoone, 1994; Barreto, 1996). It was also during the Pleistocene that most of the Caatinga karst systems, formed by previous marine incursions into the continent, were exhumed (Silva et al., 2017). The plant communities on both sand dunes and karstic rocks are physiognomically distinctive and rich in endemism (e.g., Rocha, 1995; Rocha et al., 2004; Queiroz et al., 2017; Fernandes et al., 2020).
Although greater topographic heterogeneity caused by continuation of regional denudation (Figures 2A–D) might have played a role in diversification of Caatinga endemics, the disproportional contribution of edaphic specialization to regional plant diversity suggests that the formation of soils with contrasting textures and fertility, derived from distinct parental material, have more likely acted as the trigger of Caatinga’s plant diversification through ecological speciation. The emergence of completely new environments created ecological opportunities for divergence of lineages of the regional species-pool within these edaphic islands. Indeed, edaphic specialization has been previously designated as an important driver of diversification, endemism, and spatial genetic structure of plants (e.g., Fine et al., 2005, 2013; DRYFLOR et al., 2016; Cacho and Strauss, 2014), happening relatively rapidly in some lineages (Rajakaruna, 2017). Factors other than the chemical and textural nature of the substrate, however, may be responsible for the diversification, and the nature of the substrate may be only a surrogate for yet unmeasured factors. For example, the water retention capacity within the Depressão Sertaneja is critically impacted by the features of its rocky shallow soils, derived from non-porous crystalline basement rocks, a characteristic that is not present on more sandy soils or even on the deeper soils of mountains slopes (Queiroz et al., 2017).
Relatively few studies, most of which are lineage-specific, have investigated plant evolution in the isolated patches of the SDTF biome. Despite their important biogeographical insights, we lacked basic understanding on the timing of origin and the evolutionary causes that shaped the high levels of diversity and endemism in the key SDTF nucleus of the Caatinga. Here, we used a multi-taxon phylogenetic approach to provide a comprehensive assessment of the evolutionary dynamics shaping current patterns of plant diversity in the Caatinga. We showed that the historical assembly of plant lineages in this region has been more complex than previously proposed (e.g., Sarmiento, 1975; Rizzini, 1979; Andrade-Lima, 1981, 1982; Queiroz, 2006). The dry-adapted Caatinga plant communities resulted from a mosaic of apparently idiosyncratic evolutionary histories, involving pervasive, accumulating immigration from nearby biogeographic regions, and relatively recent in situ speciation events. Transitions into the Caatinga have been facilitated by both geographic accessibility (reflected in the large number of floristic exchanges with surrounding regions) and niche conservatism (reflected in the large number of Caatinga lineages nested within clades largely restricted to the SDTF). In contrast to what had been hypothesized by Queiroz (2006), our results show that vicariance processes did not drive allopatric speciation of Caatinga endemics but that the high levels of plant species endemism are more likely a by-product of ecological speciation. We hypothesize that global aridification processes may explain the antiquity of some Caatinga SDTF lineages, whereas the newly opened habitats in the region, as well as Pleistocene climate-induced biome reconfigurations and vegetation shifts, have jointly contributed to more recent in situ plant diversification.
The model of geological evolution for the Caatinga region summarized here (Figure 2) provides a starting point for research aimed at understanding the mechanisms underlying the assembly of many other organisms. For example, the Caatinga also harbors high diversity and endemism of aquatic plants (Queiroz et al., 2017) adapted to temporary lakes and flooded areas on the margins of rivers or lowered terrains (e.g., Moro et al., 2014; The Brazil Flora Group, 2015; Queiroz et al., 2017). Investigating whether the Pliocene São Francisco “paleo-lake” has influenced the evolution of these lineages, just like the Pebas system may have contributed to the evolution of aquatic plants in the Amazon basin (e.g., Hoorn et al., 2010), is an important future question. Investigations should also be extended to beyond plants, for example to the seasonal killifishes (Rivulideae) which are highly diverse in the Caatinga, with impressive rates of endemism in the middle São Francisco River basin (Costa et al., 2013).
While our comparative approach across multiple lineages of ecologically and geographically important Caatinga plant families has revealed a more complete picture of the evolutionary history in the Caatinga SDTF, many underlying evolutionary processes require further study. For example, most Sedimentary Caatinga endemic lineages in our sampling are restricted to the São Francisco Dune System and examples need expanding to the endemic flora of the Paleo-Mesozoic sedimentary basins. The inclusion of such species will be particularly important to determine the differential effect of geological history over the disjunctly distributed sedimentary plant communities.
Future studies should also focus on the remarkable cases of transitions into Caatinga without subsequent diversification, which generally encompass relatively old endemic genera (Pennington et al., 2006). For example, Holoregmia and Tabaroa were included in our sampling, but 29 endemic genera have already been recorded for the Caatinga (Queiroz et al., 2017; Fernandes et al., 2020). Species occurring in multiple Caatinga environments (e.g., crystalline, sedimentary, and arboreal) or disjunct nuclei of the same environment (e.g., sedimentary species occurring on Tucano-Jatobá and Araripe sedimentary basins) should also be focus of finer-scale studies on incipient and cryptic speciation, a pattern that seem to be relatively common in other neotropical SDTF (e.g., Särkinen et al., 2011). Detailed phylogenetic studies, including robust sampling with multiple accessions of individual species may reveal how frequent cryptic speciation is, shedding light on the processes driving high genetic divergence despite little morphological variation.
The datasets presented in this study can be found in online repositories. The names of the repository/repositories and accession number(s) can be found in the article/Supplementary Material.
MF and LQ designed the original project. MF performed the analyses, interpreted the results, and drafted the manuscript. LQ, RP, and DC supervised the study and revised the manuscript. All authors conceived the main underlying ideas, presentation of the study, and approved the final manuscript.
This work was part of MF Ph.D. research. MF acknowledges the Conselho Nacional de Desenvolvimento Científico e Tecnológico (CNPq) for a doctorate fellowship (#141560/2015-0) and the Coordenação de Aperfeiçoamento de Pessoal de Nível Superior (CAPES) for funding a “sandwich” scholarship (PDSE 88881.133545/2016-01) at the Royal Botanic Garden Edinburgh. DC and LQ acknowledge their research productivity fellowships (Grant Nos. 306736/2015-2 and 308244/2018-4, respectively) from CNPq. DC’s research in plant biodiversity and evolution was also supported by the Royal Society (Newton Advanced Fellowship Grant No. NAF/R1/180331), CNPq (Universal Grant No. 422325/2018), and Fundação de Amparo à Pesquisa do Estado da Bahia (FAPESB, Universal Grant No. APP0037/2016). All authors acknowledge support of the NERC and FAPESP grant “NORDESTE” (NE/N012550/1, #2015/50488-5).
The authors declare that the research was conducted in the absence of any commercial or financial relationships that could be construed as a potential conflict of interest.
All claims expressed in this article are solely those of the authors and do not necessarily represent those of their affiliated organizations, or those of the publisher, the editors and the reviewers. Any product that may be evaluated in this article, or claim that may be made by its manufacturer, is not guaranteed or endorsed by the publisher.
We thank Lucas Cardoso (Universidade Federal do Maranhão) for helping with edition of figures, Cássia Bitencourt (Royal Botanic Gardens Kew) for assistance with edition of sequences, and to Alessandro Rapini (Universidade Estadual de Feira de Santana), Danilo Neves (Universidade Federal de Minas Gerais), Henrique Batalha-Filho (Universidade Federal da Bahia), Lígia Silveira Funch (Universidade Estadual de Feira de Santana), Rubson Pinheiro Maia (Universidade Federal do Ceará), Carlos Jaramillo, and an anonymous reviewer for insightful suggestions. We also thank Danilo Neves for inviting us to join the special issue “Temporal and large-scale spatial patterns of plant diversity and diversification.”
The Supplementary Material for this article can be found online at: https://www.frontiersin.org/articles/10.3389/fevo.2022.723286/full#supplementary-material
Ab’Sáber, N. A. (1974). O domínio morfoclimático semiárido das Caatingas brasileiras. Geomorfologia 43, 1–39.
Almeida, R. F., Amorim, A. M. A., and van den Berg, C. (2018). Timing the origin and past connections between Andean and Atlantic Seasonally Dry Tropical Forests in South America: insights from the biogeographical history of Amorimia (Malpighiaceae). Taxon 67, 739–751. doi: 10.12705/674.4
Andrade-Lima, D. (1982). “Present day forest refuges in Northeastern Brazil,” in Biological Diversification in the Tropics, ed. G. T. Prance (New York, NY: Columbia University Press), 245–251.
Antonelli, A., Zizka, A., Carvalho, F. A., Scharn, R., Bacon, C. D., Silvestro, D., et al. (2018). Amazonia is the primary source of Neotropical biodiversity. Proc. Natl. Acad. Sci. U.S.A. 115, 6034–6039. doi: 10.1073/pnas.1713819115
Arakaki, M., Christin, P.-A., Nyffeler, R., Lendel, A., Eggli, U., Ogburn, R. M., et al. (2011). Contemporaneous and recent radiations of the world’s major succulent plant lineages. Proc. Natl. Acad. Sci. U.S.A. 108, 8379–8384. doi: 10.1073/pnas.1100628108
Arruda, D. M., Schaefer, C. E. G. R., Fonseca, R. S., Solar, R. R. C., and Fernandes-Filho, E. I. (2017). Vegetation cover of Brazil in the last 21 ka: new insights into the Amazonian refugia and Pleistocenic arc hypotheses. Glob. Ecol. Biogeogr. 27, 47–56.
Auler, A. S., Wang, X., and Edwards, R. L. (2004). Quaternary ecological and geomorphic changes associated with rainfall events in presently semi-arid north-eastern Brazil. J. Quat. Sci. 19, 693–701.
Banda, K., Delgado, A., Dexter, K., Linares, R., Oliveira, A., Prado, D., et al. (2016). Plant diversity patterns in neotropical dry forests and their conservation implications. Science 353, 1383–1387. doi: 10.1126/science.aaf5080
Barreto, A. M. F. (1996). Interpretação Paleoambiental do Sistema de Dunas Fixadas do Médio Rio São Francisco, Bahia. São Paulo :SP: Universidade de São Paulo. [PhD thesis].
Barreto, A. M. F., and Suguio, K. (1993). Considerações Sobre a Idade e a Paleogeografia das Paleodunas do Médio Rio São Francisco, Bahia. Congresso da Associação Brasileira de Estudos do Quaternário. São Paulo: ABEQUA, 11–11.
Barros-Corrêa, A. C., Tavares, B. A. C., Lira, D. R., Mutzenberg, D. S., and Cavalcanti, L. C. S. (2019). “The semi-arid domain of the northeast of brazil,” in The Physical Geography of Brazil. Geography of the Physical Environment, eds A. Salgado, L. Santos, and J. J. Paisani (Cham: Springer International Publishing AG), 119–150. doi: 10.1007/978-3-030-04333-9_7
Benson, D. A., Cavanaugh, M., Clark, K., Karsch-Mizrachi, I., Lipman, D. J., Ostell, J., et al. (2013). GenBank. Nucleic Acids Res. 41, D36–D42.
Bezerra, F. H. R., Nascimento, A. F., Ferreira, J. M., Nogueira, F. C., Fuck, R. A., Neves, B. B. B., et al. (2011). Review of active faults in the Borborema Province, intraplate South America integration of seismological and paleoseismological data. Tectonophysics 510, 269–290. doi: 10.1016/j.tecto.2011.08.005
The Brazil Flora Group [BFG] (2015). Growing knowledge: an overview of seed plant diversity in Brazil. Rodriguesia 66, 1085–1113.
Burnham, R. J., and Carranco, N. L. (2004). Miocene winged fruits of Loxopterygium (Anacardiaceae) from the Ecuadorian Andes. Am. J. Bot. 91, 1767–1773. doi: 10.3732/ajb.91.11.1767
Burnham, R. J., and Johnson, K. R. (2004). South American paleobotany and the origins of Neotropical rainforests. Philos. Trans. R. Soc. Lond. B Biol. Sci. 359, 1595–1610. doi: 10.1098/rstb.2004.1531
Cacho, N. I., and Strauss, S. Y. (2014). Occupation of bare habitats, an evolutionary precursor to soil specialization in plants. Proc. Natl. Acad. Sci. U.S.A. 111, 15132–15137. doi: 10.1073/pnas.1409242111
Cardoso, D., Queiroz, L. P., Lima, H. C., Suganuma, E., van den Berg, C., and Lavin, M. (2013). A molecular phylogeny of the vataireoid legumes underscores floral evolvability that is general to many early-branching papilionoid lineages. Am. J. Bot. 100, 403–421. doi: 10.3732/ajb.1200276
Cardoso, D. B. O. S., and Queiroz, L. P. (2007). Diversidade de Leguminosae nas Caatingas de Tucano, Bahia: implicações para a fitogeografia do semiárido do Nordeste do Brasil. Rodriguesia 58, 379–391.
Carvalho-Sobrinho, J. G., Alverson, W. S., Alcantara, S., Queiroz, L. P., Mota, A. C., and Baum, D. A. (2016). Revisiting the phylogeny of Bombacoideae (Malvaceae): novel relationships, morphologically cohesive clades, and a new tribal classification based on multilocus phylogenetic analyses. Mol. Phylogenet. Evol. 101, 56–74. doi: 10.1016/j.ympev.2016.05.006
Costa, W. J. E. M., Amorim, P. F., and Bragança, P. H. N. (2013). Species limits and phylogenetic relationships of red-finned cryptic species of the seasonal killifish genus Hypsolebias from the Brazilian semi-arid Caatinga (Teleostei: Cyprinodontiformes: Rivulidae). J. Zool. Syst. Evol. Res. 52, 52–58.
Crisp, M., Arroyo, M. T., Cook, L. G., Gandolfo, M. A., Jordan, G. J., McGlone, M. S., et al. (2009). Phylogenetic biome conservatism on a global scale. Nature 458, 754–758. doi: 10.1038/nature07764
Cruz, D. T., Idárraga, A., Banda, K., Cogollo, A., van den Berg, C., Queiroz, L. P., et al. (2018). Ancient speciation of the papilionoid legume Luetzelburgia jacana, a newly discovered species in an inter-Andean seasonally dry valley of Colombia. Taxon 67, 931–943. doi: 10.12705/675.6
Darriba, D., Taboada, G., Doallo, R., and Posada, D. (2012). jModelTest2: more models, new heuristics and parallel computing. Nat. Methods 9:772. doi: 10.1038/nmeth.2109
Dexter, K. G., Smart, B., Baldauf, C., Baker, T. R., Balinga, M. P. B., Brienen, R. J. W., et al. (2015). Floristics and biogeography of vegetation in seasonally dry tropical regions. Int. For. Rev. 17, 10–32. doi: 10.1016/j.pld.2016.11.004
Donoghue, M. (2008). A phylogenetic perspective on the distribution of plant diversity. Proc. Natl. Acad. Sci. U.S.A. 105, 11549–11555. doi: 10.1073/pnas.0801962105
Donoghue, M. J., and Edwards, E. J. (2014). Biome shifts and niche evolution in plants. Annu. Rev. Ecol. Evol. Syst. 45, 547–572. doi: 10.1146/annurev-ecolsys-120213-091905
Donoghue, M. J., and Smith, S. A. (2004). Patterns of the assembly of temperate forests around the northern hemisphere. Philos. Trans. R. Soc. Lond. B Biol. Sci. 359, 1633–1644. doi: 10.1098/rstb.2004.1538
Drummond, A. J., Ashton, B., Cheung, M., Heled, J., Kearse, M., Moir, R., et al. (2012a). Geneious Version 6.1.6. http://www.genious.com (accessed February 17, 2021).
Drummond, A. J., Suchard, M. A., Xie, D., and Rambaut, A. (2012b). Bayesian phylogenetics with BEAUti and the BEAST 1.7. Mol. Biol. Evol. 29, 1969–1973. doi: 10.1093/molbev/mss075
DRYFLOR Karina Banda, R., Dexter, K. G., Linares-Palomino, R., Oliveira-Filho, A., Prado, D., et al. (2016). Plant diversity patterns in neotropical dry forests and their conservation implications. 353, 1383–1387. doi: 10.1371/journal.pone.0062639
Fedorov, A. V., Brierley, C. M., Lawrence, K. T., Liu, Z., Dekens, P. S., and Ravelo, A. C. (2013). Patterns and mechanisms of early Pliocene warmth. Nature 496, 43–49. doi: 10.1038/nature12003
Fernandes, M. F., Cardoso, D., and Queiroz, L. P. (2020). An updated plant checklist of the Brazilian Caatinga seasonally dry forests and woodlands reveals high species richness and endemism. J. Arid Environ. 174:104079. doi: 10.1016/j.jaridenv.2019.104079
Fiaschi, P., and Pirani, J. R. (2009). Review of plant biogeographic studies in Brazil. J. Syst. Evol. 47, 477–496.
Fine, P. V. A., Daly, D. C., Muñoz, G. V., Mesones, I., and Cameron, K. (2005). The contribution of edaphic heterogeneity to the evolution and diversity of Burseraceae trees in the Western Amazon. Evolution 59, 1464–1478. doi: 10.1554/04-745
Fine, P. V. A., and Ree, R. H. (2006). Evidence for a time-integrated species-area effect on the latitudinal gradient in tree diversity. Am. Nat. 168, 796–804. doi: 10.1086/508635
Fine, P. V. A., Zapata, F., Daly, D. C., Mesones, I., Misiewicz, T. M., Cooper, H. F., et al. (2013). The importance of environmental heterogeneity and spatial distance in generating phylogeographic structure in edaphic specialist and generalist tree species of Protium (Burseraceae) across the Amazon Basin. J. Biogeogr. 40, 646–661.
Gagnon, E., Ringelberg, J. J., Bruneau, A., Lewis, G. P., and Hughes, C. E. (2019). Global Succulent Biome phylogenetic conservatism across the pantropical Caesalpinia Group (Leguminosae). New Phytol. 222, 1994–2008. doi: 10.1111/nph.15633
Gomes, A. P. S., Rodal, M. J. N., and Melo, A. L. (2006). Florística e fitogeografia da vegetação arbustiva subcaducifólia da Chapada de São José. Buíque PE Brasil Acta Bot. Bras. 20, 37–48.
Gottsberger, G., and Silberbauer-Gottsberger, I. (2006). Life in the Cerrado: a South American tropical seasonal ecosystem. Germany: Reta Verlag.
Govindarajulu, R., Hughes, C. E., and Bailey, C. D. (2011). Phylogenetic and population genetic analyses of diploid Leucaena (Leguminosae) reveal cryptic species diversity and patterns of allopatric divergent speciation. Am. J. Bot. 98, 2049–2063. doi: 10.3732/ajb.1100259
Gurgel, S. P. P., Bezerra, F. H. R., Corrêa, A. C. B., Marques, F. O., and Maia, R. P. (2013). Cenozoic uplift and erosion of structural landforms in NE Brazil. Geomorphology 186, 68–84.
Harris, S. E., and Mix, A. C. (2002). Climate and tectonics drive continental erosion of tropical South America, 0-13 Ma. Geology 30, 447–450.
Hoorn, C., Bernardes-de-Oliveira, M. E. C., Dino, R., Garcia, M. J., Antonioli, L., Casado, F. C., et al. (2014). “Neogene climate evolution in Amazonia and the Brazilian Northeast,” in Paleontologia: Cenários de vida - Paleoclimas, eds I. S. Carvalho, M. J. Garcia, O. Strohschoen, and C. C. Lana (Rio de Janeiro, RJ: Editora Interciência), 277–310.
Hoorn, C., Wesselingh, F. P., Ter Steege, H., Bermudez, M. A., Mora, A., Sevink, J., et al. (2010). Amazonia through time: Andean uplift, climate change, landscape evolution, and biodiversity. Science 330, 927–931. doi: 10.1126/science.1194585
Japsen, P., Bonow, J. M., Green, P. F., Cobbold, P. R., Chiossi, D., Lilletveit, R., et al. (2012). Episodic burial and exhumation in NE Brazil after opening of the South Atlantic. Geol. Soc. Am. Bull. 124, 800–816. doi: 10.1130/b30515.1
Jaramillo, C., and Cardenas, A. (2012). Global warming and Neotropical rainforests, a historical perspective. Annu. Rev. Earth Planet. Sci. 41, 741–766.
Jaramillo, C., Sepulchre, P., Cardenas, D., Correa-Metrio, A., Moreno, J. E., Trejos, R., et al. (2020). Drastic Vegetation Change in the Guajira Peninsula (Colombia) During the Neogene. Paleoceanogr. Paleoclimatol 35:e2020PA003933.
Katoh, K., and Standley, D. M. (2013). MAFFT Multiple Sequence Alignment Software Version 7: Improvements in performance and usability. Mol. Biol. Evol. 30, 772–780. doi: 10.1093/molbev/mst010
Klöckinga, M., Hoggard, M. J., Rodríguez-Tribaldos, V., Richards, F. D., Guimarães, A. R., Maclennan, J., et al. (2020). A tale of two domes: Neogene to recent volcanism and dynamic uplift of northeast Brazil and southwest Africa. Earth Planet. Sci. Lett. 547:116464. doi: 10.1016/j.epsl.2020.116464
Lavin, M. (2006). “Floristic and geographical stability of discontinuous seasonally dry tropical forests explains patterns of plant phylogeny and endemism,” in Neotropical savannas and seasonally dry forests: plant diversity, biogeography, and conservation, eds G. P. Lewis and J. A. Ratter (London, UK: CRC Press), 433–447.
Löwenberg-Neto, P. (2014). Neotropical region: a shapefile of Morrone’s (2014) biogeographical regionalisation. Zootaxa 3802:300. doi: 10.11646/zootaxa.3802.2.12
Mabesoone, J. M. (1994). Sedimentary Basins of Northeast Brazil. Recife: Editora Universitária UFPE.
Magnavita, L. P., Davison, I., and Kusznir, N. J. (1994). Rifting, erosion and uplift history of the Reconcavo–Tucano–Jatoba Rift, northeast Brazil. Tectonics 13, 367–388. doi: 10.1029/93tc02941
Maia, R. P., Bezerra, F. H., and Sales, V. C. (2010). Geomorfologia do Nordeste: concepções clássicas e atuais acerca das superfícies de aplainamento. Rev. Bras. Geogr. 27, 6–19.
Matzke, N. J. (2013). BioGeoBEARS: Biogeography with Bayesian (and likelihood) Evolutionary Analysis in R Scripts. Berkeley, CA: University of California.
Maya-Lastra, C. A., and Steinmann, V. W. (2019). Evolution of the untouchables: Phylogenetics and classification of Cnidoscolus (Euphorbiaceae). Taxon 68, 692–713.
Mayle, F. E. (2004). Assessment of the Neotropical dry forest refugia hypothesis in the light of palaeoecological data and vegetation model simulations. J. Quat. Sci. 19, 713–720.
McDowell, N., Pockman, W. T., Allen, C. D., Breshears, D. D., Cobb, N., Kolb, T., et al. (2008). Mechanisms of plant survival and mortality during drought: why do some plants survive while others succumb to drought? New Phytol. 178, 719–739. doi: 10.1111/j.1469-8137.2008.02436.x
Miller, M. A., Pfeiffer, W., and Schwartz, T. (2010). Creating the CIPRES Science Gateway for Inference of Large Phylogenetic Trees. Piscataway, NJ: IEEE, 1–8.
Miranda, P. L. S., Oliveira-Filho, A. T., Pennington, R. T., Neves, D. M., Baker, T. R., and Dexter, K. G. (2018). Using tree species inventories to map biomes and assess their climatic overlaps in lowland tropical South America. Glob. Ecol. Biogeogr. 27, 899–912. doi: 10.1111/geb.12749
Moro, M. F., Nic Lughadha, E., de Araújo, F. S., and Martins, F. R. (2016). A phytogeographical metaanalysis of the semiarid Caatinga Domain in Brazil. Bot. Rev. 82, 91–148. doi: 10.1007/s12229-016-9164-z
Moro, M. F., Sousa, D. J. L., and Matias, L. (2014). Rarefaction, richness estimation and extrapolation methods in the evaluation of unseen plant diversity in aquatic ecosystems. Aquat. Bot. 117, 48–55. doi: 10.1016/j.aquabot.2014.04.006
Morrone, J. J. (2014). Biogeographical regionalisation of the Neotropical region. Zootaxa 3782, 1–110. doi: 10.11646/zootaxa.3782.1.1
Oliveira, P. E., Barreto, A. M. F., and Suguio, K. (1999). Late Pleistocene-Holocene climatic and vegetational history of the Brazilian Caatinga: the fossil dunes of the middle São Francisco River. Palaeogeogr. Palaeoclimatol. Palaeoecol. 152, 319–337.
Oliveira, R. G., and Medeiros, W. E. (2012). Evidences of buried loads in the base of the crust of Borborema Plateau (NE Brazil) from Bouguer admittance estimates. J. South Am. Earth Sci. 37, 60–76.
Oliveira-Filho, A. T., Cardoso, D., Schrire, D., Lewis, G. P., Pennington, R. T., Brummer, T. J., et al. (2013). Stability structures tropical woody plant diversity more than seasonality: Insights into the ecology of high legume-succulent-plant biodiversity. S. Afr. J. Bot. 89, 42–57.
Pennington, R. T., Lavin, M., and Oliveira-Filho, A. T. (2009). Woody plant diversity, evolution and ecology in the tropics: perspectives from seasonally dry tropical forests. Ann. Rev. Ecol. Evol. Syst. 40, 437–457.
Pennington, R. T., Lavin, M., Prado, D. E., Pendry, C. A., Pell, S. K., and Butterworth, C. A. (2004). Historical climate change and speciation: neotropical seasonally dry forest plants show patterns of both tertiary and quaternary diversification. Philos. Trans. R. Soc. Lond. B Biol. Sci. 359, 515–538. doi: 10.1098/rstb.2003.1435
Pennington, R. T., Lewis, G. P., and Ratter, J. A. (2006). “An overview of the plant diversity, biogeography and conservation of Neotropical savannas and seasonally dry forests,” in Neotropical Savannas and Seasonally Dry Forests: plant Diversity, Biogeography, and Conservation, eds R. T. Pennington, G. P. Lewis, and J. A. Ratter (London, UK: CRC Press), 193–211.
Pennington, R. T., Prado, D. E., and Pendry, C. A. (2000). Neotropical seasonally dry forests and Pleistocene vegetation changes. J. Biogeogr. 27, 261–273.
Peulvast, J. P., and Claudino-Sales, V. (2004). Stepped surfaces and paleolandforms in the Northern Brazilian “Nordeste”: constraints on models of morphotectonic evolution. Geomorphology 3, 89–122. doi: 10.1016/j.geomorph.2004.02.006
Peulvast, J. P., Claudino-Sales, V., Bétard, F., and Gunnell, Y. (2008). Low post-Cenomanian denudation depths across the Brazilian Northeast: implications for long-term landscape evolution at a transform continental margin. Glob. Planet. Change 62, 39–60. doi: 10.1016/j.gloplacha.2007.11.005
Piel, W. H., Chan, L., Dominus, M. J., Ruan, J., Vos, R. A., and Tannen, V. (2009). v. 2: a database of phylogenetic knowledge. In: e-BioSphere 2009. Available online at: https://treebase.org/treebase-web/reference.html (accessed February 17, 2021).
Prado, D. E. (2000). Seasonally dry forests of tropical South America: from forgotten ecosystems to a new phytogeographic unit. Edinb. J. Bot. 57, 437–461. doi: 10.1017/s096042860000041x
Prado, D. E., and Gibbs, P. E. (1993). Patterns of species distributions in the dry seasonal forests of South America. Ann. Mo. Bot. Gard. 80, 902–927.
Queiroz, L. P. (2006). “The Brazilian Caatinga: phytogeographical patterns inferred from distribution data of the Leguminosae,” in Neotropical Savannas and Seasonally Dry Forests: plant Diversity, Biogeography, and Conservation, eds R. T. Pennington, G. P. Lewis, and J. A. Ratter (London, UK: CRC Press), 121–158.
Queiroz, L. P., Cardoso, D., Fernandes, M. F., and Moro, M. F. (2017). “Diversity and evolution of flowering plants of the Caatinga Domain,” in Caatinga: the Largest Tropical Dry Forest Region in South America, eds J. M. C. Silva, I. R. Leal, and M. Tabarelli (Cham, CH: Springer International Publishing AG), 23–63. doi: 10.1099/ijsem.0.004193
Queiroz, L. P., and Lavin, M. (2011). Coursetia (Leguminosae) from eastern Brazil: nuclear ribosomal and chloroplast DNA sequence analysis reveal the monophyly of three caatinga-inhabiting species. Syst. Bot. 36, 69–79.
Queiroz, L. P., Lewis, G. P., and Wojciechowski, M. F. (2010). Tabaroa, a new genus of Leguminosae tribe Brongniartieae from Brazil. Kew Bull. 65, 189–203. doi: 10.1007/s12225-010-9202-7
R Core Team (2020). R: a Language and Environment for Statistical Computing. Vienna, AUS: R Foundation for Statistical Computing.
Rajakaruna, N. (2017). Lessons on evolution from the study of edaphic specialization. Bot. Rev. 84, 1–40.
Rambaut, A., and Drummond, A. J. (2013). Tracer version 1.6. Computer Program and Documentation Distributed by the Author. http://beast.bio.ed.ac.uk/Tracer (accessed February 17, 2021).
Ratter, J. A., Bridgewater, S., and Ribeiro, J. F. (2006). “Biodiversity patterns of the woody vegetation of the Brazilian Cerrados,” in Neotropical Savannas and Seasonally Dry Forests: Plant Diversity, Biogeography and Conservation, eds R. T. Pennington, J. A. Ratter, and G. P. Lewis (London, UK: CRC Press), 31–66. doi: 10.1201/9781420004496.ch2
Ricklefs, R. E., Schwarzbach, A. E., and Renner, S. S. (2006). Rate of lineage origin explains the diversity anomaly in the world’s mangrove vegetation. Am. Nat. 168, 805–810. doi: 10.1086/508711
Ringelberg, J. J., Zimmermann, N. E., Weeks, A., Lavin, M., and Hughes, C. E. (2020). Biomes as evolutionary arenas: convergence and conservatism in the trans-continental succulent biome. Glob. Ecol. Biogeogr. 29, 1100–1113.
Rocha, P. L. (1995). Proechimys yonenagae, a new species of spiny rat (Rodentia: Echimyidae) from fossil sand dunes in Brazilian Caatinga. Mammalia 59, 537–550.
Rocha, P. L., Queiroz, L. P., and Pirani, J. R. (2004). Plant species and habitat structure in a sand dune field in the Brazilian Caatinga: a homogeneous habitat harbouring an endemic biota. Rev. Bras. Bot. 27, 739–755.
Rodríguez-Tribaldos, V., White, N. J., Roberts, G. G., and Hoggard, M. J. (2017). Spatial and temporal uplift history of South America from calibrated drainage analysis. Geochem. Geophys. Geosyst. 18, 2321–2353. doi: 10.1002/2017gc006909
Santos, R. M., Oliveira-Filho, A. T., Eisenlohr, P. V., Queiroz, L. P., Cardoso, D. B. O. S., and Rodal, M. J. N. (2012). Identity and relationships of the arboreal Caatinga among other floristic units of seasonally dry tropical forests (SDTFs) of north-eastern and Central Brazil. Ecol. Evol. 2, 409–428. doi: 10.1002/ece3.91
Särkinen, T., Marcelo-Peña, J. L., Yomona, A. D., Simon, M. F., and Pennington, R. T. (2011). Underestimated endemic species diversity in the dry inter-Andean valley of the Río Marañón, northern Peru: an example from Mimosa (Leguminosae. Mimosoideae). Taxon 60, 139–150.
Sarmiento, G. (1975). The dry plant formations of South America and their floristic connections. J. Biogeogr. 2, 233–251. doi: 10.1371/journal.pone.0233729
Schrire, B. D., Lavin, M., and Lewis, G. P. (2005). Global distribution patterns of the Leguminosae: insights from recent phylogenies. Biol. Skr. 55, 375–386.
Segovia, R. A., Pennington, R. T., Baker, T. R., Souza, F. C., Neves, D. M., Davis, C. C., et al. (2020). Freezing and water availability structure the evolutionary diversity of trees across the Americas. Sci. Adv. 6:eaaz5373. doi: 10.1126/sciadv.aaz5373
Silva, A. C., and Souza, A. F. (2018). Aridity drives plant biogeographical subregions in the Caatinga, the largest tropical dry forest and woodland block in South America. PLoS One 13:e0196130. doi: 10.1371/journal.pone.0196130
Silva, O. L., Bezerra, F. H. R., Maia, R. P., and Cazarin, C. L. (2017). Karst landforms revealed at various scales using LiDAR and UAV in semi-arid Brazil: Consideration on karstification processes and methodological constraints. Geomorphology 295, 611–630.
Silveira, M. H. B., Mascarenhas, R., Cardoso, D., and Batalha-Filho, H. (2019). Pleistocene climatic instability drove the historical distribution of forest islands in the northeastern Brazilian Atlantic Forest. Palaeogeog. Palaeoclimatol. Palaeoecol. 527, 67–76. doi: 10.1016/j.palaeo.2019.04.028
Simon, M. F., Grether, R., Queiroz, L. P., Särkinen, T. E., Dutra, V. F., and Hughes, C. E. (2011). The Evolutionary history of Mimosa (Leguminosae): toward a phylogeny of the sensitive plants. Am. J. Bot. 98, 1201–1221. doi: 10.3732/ajb.1000520
Valadão, R. C. (2009). Geodinâmica de superfícies de aplanamento, desnudação continental e tectônica ativa como condicionantes da megageomorfologia do Brasil oriental. Braz. J. Geol. 10, 77–90.
Velloso, A. L., Sampaio, E. V. S. B., Giulietti, A. M., Barbosa, M. R. V., Castro, A. A. J. F., Queiroz, L. P., et al. (2002). Ecorregiões: propostas Para o Bioma Caatinga. Recife: Associação Plantas do Nordeste and The Nature Conservancy do Brasil.
Wang, X., Auler, A. S., Edwards, R. L., Cheng, H., Cristalli, P. S., Smart, P. L., et al. (2004). Wet periods in Northeastern Brazil over the past 210 ka linked to distant climate anomalies. Nature 432, 740–743. doi: 10.1038/nature03067
Wiens, J. J., and Donoghue, M. J. (2004). Historical biogeography, ecology and species richness. Trends Ecol. Evol. 19, 639–644. doi: 10.1016/j.tree.2004.09.011
Wing, S. L., Herrera, F., Jaramillo, C. A., Gomez-Navarro, C., Wilf, P., and Labandeira, C. C. (2009). Late Paleocene fossils from the Cerrejón Formation, Colombia, are the earliest record of Neotropical rainforest. Proc. Natl. Acad. Sci. U.S.A. 106, 18627–18632. doi: 10.1073/pnas.0905130106
Keywords: biogeography, biome evolution, biome reconstruction, landscape evolution, Northeastern South America, semi-arid, SDTF, speciation
Citation: Fernandes MF, Cardoso D, Pennington RT and de Queiroz LP (2022) The Origins and Historical Assembly of the Brazilian Caatinga Seasonally Dry Tropical Forests. Front. Ecol. Evol. 10:723286. doi: 10.3389/fevo.2022.723286
Received: 10 June 2021; Accepted: 27 January 2022;
Published: 24 February 2022.
Edited by:
Danilo M. Neves, Federal University of Minas Gerais, BrazilReviewed by:
Carlos Jaramillo, Smithsonian Tropical Research Institute, PanamaCopyright © 2022 Fernandes, Cardoso, Pennington and de Queiroz. This is an open-access article distributed under the terms of the Creative Commons Attribution License (CC BY). The use, distribution or reproduction in other forums is permitted, provided the original author(s) and the copyright owner(s) are credited and that the original publication in this journal is cited, in accordance with accepted academic practice. No use, distribution or reproduction is permitted which does not comply with these terms.
*Correspondence: Moabe F. Fernandes, bW9hYmVmZmVybmFuZGVzQGdtYWlsLmNvbQ==
Disclaimer: All claims expressed in this article are solely those of the authors and do not necessarily represent those of their affiliated organizations, or those of the publisher, the editors and the reviewers. Any product that may be evaluated in this article or claim that may be made by its manufacturer is not guaranteed or endorsed by the publisher.
Research integrity at Frontiers
Learn more about the work of our research integrity team to safeguard the quality of each article we publish.