- 1Department of Biological Sciences, Universidade Estadual de Santa Cruz/UESC, Ilhéus, Brazil
- 2Department of Biological Sciences, Universidade Federal Rural de Pernambuco/UFRPE, Recife, Brazil
- 3Non-Governmental Organization (NGO) Ecoassociados, Ipojuca, Brazil
- 4Instituto Tartarugas do Delta - ITD, Serviço Social do Comércio (SESC) Praia, Luis Correia, Brazil
- 5Associação Guajiru, Ciência, Educação e Meio Ambiente, Cabedelo, Brazil
- 6Instituto Biota de Conservação, Maceió, Brazil
- 7Projeto Amiga Tartaruga - PAT Ecosmar, Porto Seguro, Brazil
- 8Herpetology Section, Zoologisches Forschungsmuseum Alexander Koenig, Bonn, Germany
Marine turtle nesting areas are characterized by receiving several females every year. The species Eretmochelys imbricata, known as hawksbill turtle is listed as Critically Endangered by the IUCN, and has been the target of studies on genetic structure and population diversity in nesting areas. Therefore, this study aimed to analyze the genetic diversity and haplotype composition of populations sampled in nesting areas from the coast of northeastern Brazil based on the mtDNA D-loop region. We used genetic information and compared it with data from feeding areas available in public databases. We recorded a total of six exclusive haplotypes in the nesting areas and 27 exclusive haplotypes for the feeding areas. The H_1 haplotype was shared in all nesting areas. The turtles of these regions had a low diversity and a genetic structure composed of five divergent groups separating the reproductive areas from the ones of feeding areas. Positive and significant geographical distance relationships were also recorded with FST values (r = 0.2302, p = 0.007). Our results revealed that hawksbill turtles from reproductive areas comprise a single population that needs management strategies to protect the threatened species, in addition to providing information that contributes to future actions for the species conservation.
Introduction
Five species of sea turtles are distributed along the Brazilian coastline and all of them have been categorized under some level of extinction threat (Santos et al., 2013). Among them, the hawksbill sea turtle Eretmochelys imbricata (Linnaeus, 1766) is classified as Critically Endangered (IUCN, 2008). The species E. imbricata has a circumglobally distribution in tropical and subtropical waters in the Atlantic, Indian and Pacific Oceans (Pritchard and Mortimer, 1999; LeRoux et al., 2012). Previous research revealed that the populations of E. imbricata are concentrated mainly in the Eastern Atlantic Ocean and the Caribbean (Marcovaldi et al., 2007; Vilaça et al., 2013). Mexico, for example, is considered the country hosting the largest nesting population of this species in the Atlantic, in addition to several areas along the coast that are used as feeding areas (Garduño-Andrade et al., 1999; Labastida-Estrada et al., 2019). Hawksbill turtles feed preferentially on sponges, and like other sea turtles, they have a life cycle with a migratory habit between rookeries and feeding areas and show philopatric behavior (Wallace et al., 2010; Putman et al., 2014).
Philopatry, defined as the tendency of females to return to natal areas around the beaches they were hatched, supposes that nesting areas have specific genetic information of maternal inheritance, derived from mitochondrial DNA (mtDNA) (Bowen and Karl, 2007), resulting in a genetically structured population that can be considered as a Management Unit (Moritz, 1994). In general, molecular studies are being increasingly applied in analyses of genetic diversity and structure in the areas of reproduction, phylogenetic relationships, migratory behavior and composition in the feeding areas and, in philopatry of sea turtles worldwide (Bolten et al., 1998; Bowen et al., 2005; Bjorndal and Bolten, 2008; Joseph et al., 2016; Vargas et al., 2016). In this context, the use of molecular markers such as mtDNA (control/D-loop region) stands out for being efficient in assessing the genetic structure among reproductive areas (Jensen et al., 2013; López-Barrera et al., 2016; Reis and Goldberg, 2017). Most studies on mtDNA used few bases for sequencing (∼300 bp), but in view of the need to obtain more detailed information about the detection of variation and population structure, longer sequences are being applied for this species (Troëng et al., 2005; LeRoux et al., 2012; Nishizawa et al., 2016; Arantes et al., 2020).
Many research has recorded important nesting and feeding areas for E. imbricata in Brazil. However, few genetic analyses have been carried out with mtDNA in these areas, with only a few studies for nesting areas in the states of Bahia, Sergipe, Rio Grande do Norte, and Ceará. For feeding areas genetic studies are restricted to São Pedro and São Paulo Archipelago, Fernando de Noronha Archipelago, Abrolhos National Marine Park, and some regions of southern Brazil (Bass et al., 1996; Bass, 1999; Lara-Ruiz et al., 2006; Vilaça et al., 2013; Proietti et al., 2014).
Although such research on the Brazilian coast exists, the genetic characterization of nesting areas of E. imbricata will be essential to understand the distribution and dynamics of the Brazilian haplotypes, in addition to contributing additional information on the genetic profile of nesting areas in the country (Proietti et al., 2014). In addition, reproductive records for the species are increasing in areas not considered as conservation priorities and such information can expand knowledge about the biology of these animals for conservation purposes (Vilaça et al., 2013). Thus, aiming to increase the knowledge, this study used mtDNA to analyze the genetic diversity of E. imbricata their nesting beaches (states of Piauí, Rio Grande do Norte, Paraíba, Pernambuco, Alagoas, and southern Bahia), in addition to evaluating the genetic structure and comparing haplotype sequences between the nesting and feeding areas of this species.
Materials and Methods
Study Area and Data Collection
The study was carried out on beaches located on the coast of northeastern Brazil, which stand out for having sea turtle nesting activity, including E. imbricata nests. This coastline is characterized by the presence of sand strips, formation of inlets, some have coral reefs and sandstones, and the vast majority are urban beaches that are under the influence of tourism and anthropic activities (Santana et al., 2009; Moura et al., 2012; Costa et al., 2016; Melo, 2016).
Six nesting areas of E. imbricata located in the states of Piauí (PI_BRA), Alagoas (Al_BRA), Bahia (BA_BRA), Rio Grande do Norte (RN_BRA), Pernambuco (PE_BRA), and Paraíba (PB_BRA) (Figure 1 and Supplementary Table 1) were sampled between 2017 and 2018. Daily monitoring was conducted in each area to record nesting by E. imbricata females, and to preserve all nests during the incubation period until emergence of the hatchlings. After emergence, random tissue samples were collected for DNA extraction from 3 to 5 dead hatchlings/embryos from approximately 20 nests. Tissue fragments measuring approximately 3 mm were obtained with a disposable scalpel from dead hatchlings/embryos from each nest. As it was not possible to tag all females that laid the sampled nests, we only collected tissue samples from nests that hatched in a period of 10 days to avoid double sampling nesting females, as E. imbricata has an internesting period between 12 and 15 days (Santos et al., 2013). Subsequently the material was placed in microtubes containing absolute alcohol (Proietti et al., 2014), and then transferred to the laboratory and stored at −20∘C until molecular analyses were performed. Samples were taken under license SISBIO/ICMBIO n° 56974 and were conducted in accordance with the Ethical Principles of Animal Experimentation adopted by the Ethics Committee on the Use of Animals—CEUA/UESC under n° 038/16.
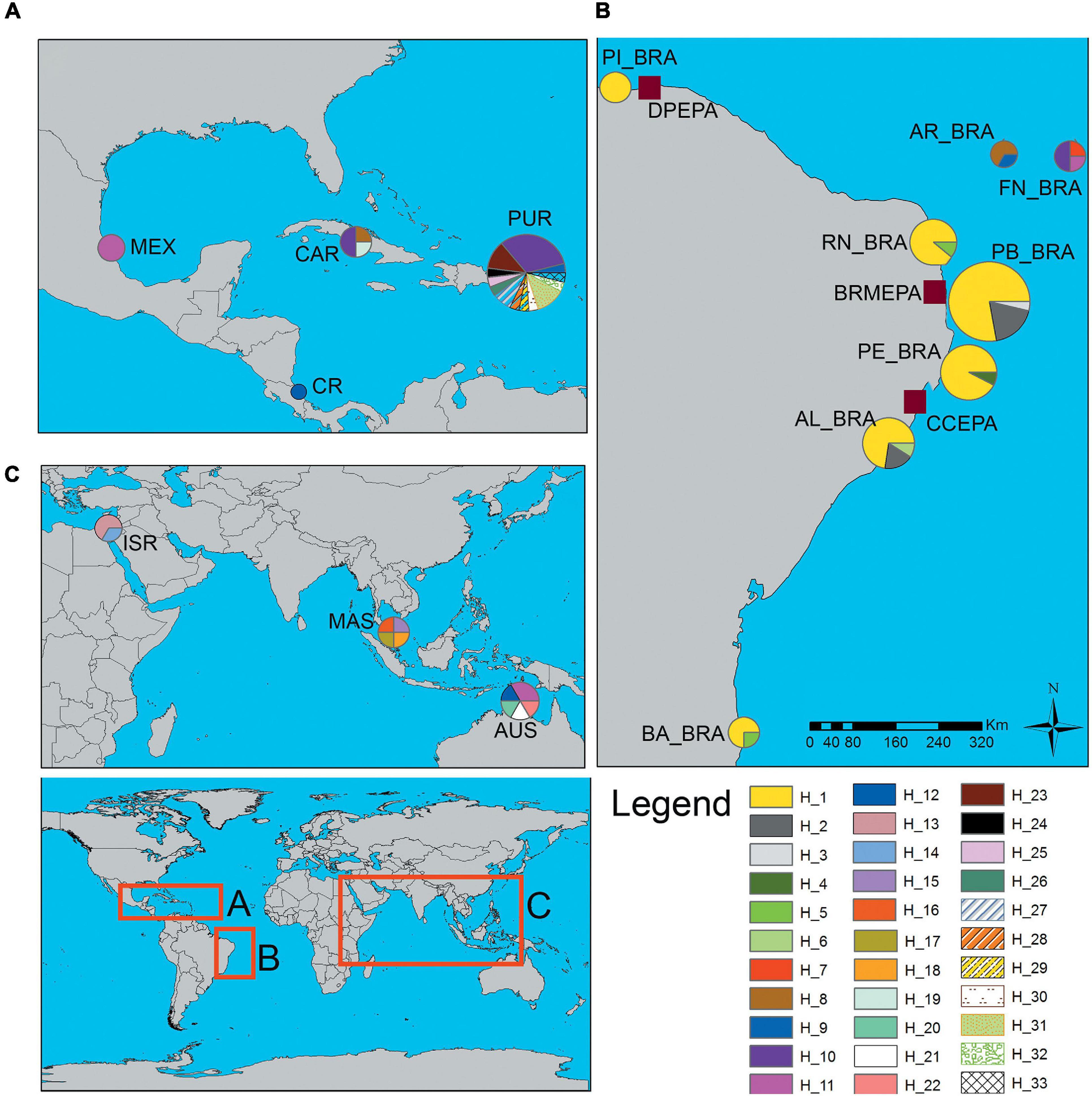
Figure 1. Map showing the location of the 15 populations of Eretmochelys imbricata evaluated and the spatial distribution of the 33 haplotypes recorded in the study. Each color represents a haplotype, the thickness being proportional to its frequency in each population. (A) Costa Rica—CR; Mexico—MEX; Caribbean—CAR; Puerto Rico—PUR. (B) Bahia—BA_BRA; Alagoas—AL_BRA; Pernambuco—PE_BRA; Paraíba—PB_BRA; Rio Grande do Norte—RN_BRA; Piaui—PI_BRA; Atol das Rocas—AR_BRA; Fernando de Noronha—FN_BRA. Location of Protection Areas: Costa dos Corais Environmental Protection Area (CCEPA); Barra do Rio Mamanguape Environmental Protection Area (BRMEPA); Delta Parnaíba Environmental Protection Area (DPEPA). (C) Australia—AUS; Malaysia—MAS; Israel—ISR.
Subsequently, we conducted a research of mtDNA haplotype sequences for E. imbricata in foraging areas in the GenBank database. Nine areas were included in this study (Atol das Rocas—AR_BRA; Fernando de Noronha—FN_BRA; Costa Rica—CR; Mexico—MEX; Malaysia—MAS; Caribbean—CAR; Australia—AUS; Puerto Rico—PUR; Israel—ISR) (Figure 1 and Supplementary Tables 2, 3). The criteria used to select the DNA sequences of the species in the Genbank database were restricted to feeding areas only, to haplotype sequencing exclusively in the D-Loop region of mtDNA and to those that had above 700 bp (Supplementary Table 3). Data that did not meet these requirements were not included in our study due to non-compatibility with survey data for statistical analyses.
Sample Processing
The obtained samples were digested with proteinase K (20 mg ml) in a lysis buffer and incubated overnight at 37∘C until complete digestion (Proietti et al., 2014). DNA extraction was performed using the Easy-DNA Kit (Invitrogen) following the manufacturer’s protocol. The ∼830 bp fragments of the mtDNA D-loop region were amplified by PCR using primers H950 (5′-GTCTCGGATTTAGGGGGTTTG-3′) and LCM15382 (5′-GCTTAACCCTAAAGCATTGG-3′) (Abreu-Grobois et al., 2006). Amplification was performed in a total volume of 13 μl, containing 5–15 ng of DNA, 10x buffer, 2,25 mM of each deoxynucleotide triphosphate (dNTP), 50 mM of MgCl2, 10 μM of each primer, 0.2 U Taq polymerase. The PCR reactions followed the conditions described by Tabib et al. (2011). Then, the PCR product was purified using ExoSAP-IT®, following the manufacturer’s protocol. Sequencing was performed by ABI PRISM® 3500 capillary electrophoresis, using the reverse and forward primers. The final sequences were analyzed and aligned using the BIOEDIT 7.0.9.0 software (Hall, 1999).
Research with endangered species is occasionally more restricted in relation to the collection of biological samples from live animals, so after DNA extraction and purification some samples had to be discarded, as they were degraded since they were obtained from dead hatchlings of E. imbricata.
Data Analysis
To estimate the genetic diversity from nesting and feeding areas, nucleotide diversity (π), haplotypic diversity (h) and the number of haplotypes were calculated using the DNAsp v5 program (Librado and Rozas, 2009). The relationships between haplotypes and how they are spatially distributed were analyzed by a haplotype network using the median-joining method implemented in the Network 4.613 program (Bandelt et al., 1999). For the genetic structure between populations, the FST (fixation index) was calculated using the DNAsp v5 program (Librado and Rozas, 2009). The FST values were used in the heatmap analysis with the populations under study and the number of groups in the heatmap was established using the hierarchical clustering algorithm with Euclidean distance and the average method using the heatmaply package (Galili et al., 2017) in R software (R Core Team, 2020). The “heatmap” is a graphical representation of the data. However the software enabled us to graphically represent the data frequency associated with the cluster analysis at the same time (for more details, see Galili et al., 2017). Then, to assess whether the geographical distance (km) influenced the FST values obtained among the populations, a Mantel test was performed (Mantel, 1967), using the ecodista package (Goslee and Urban, 2007) in R software (R Core Team, 2020).
Results
The 15 areas analyzed (six reproductive and nine feeding areas) revealed a total of 33 haplotypes for E. imbricata, highlighting the largest number (13) found in Puerto Rico (PUR), these were also registered in the literature for beaches in Mexico, Brazil, Florida, Caribbean and Cuba (Blumenthal et al., 2009; Richardson et al., 2009; Vilaça et al., 2013; Wood et al., 2013; Gorham et al., 2014; Proietti et al., 2014; Cazabon-Mannette et al., 2016; Pérez-Bermúdez et al., 2017; Labastida-Estrada et al., 2019). Of the 33 haplotypes, six are exclusive to the nesting areas and the other 27 exclusive to the feeding areas (Figures 1, 2 and Supplementary Table 1). The most frequent haplotypes in the nesting areas were H_1 (82.35%) followed by H_2 (10.29%), whereas in the feeding areas it were haplotypes H_10 (22.64%) and H_11 (11.32%) (Figure 1 and Supplementary Table 1).
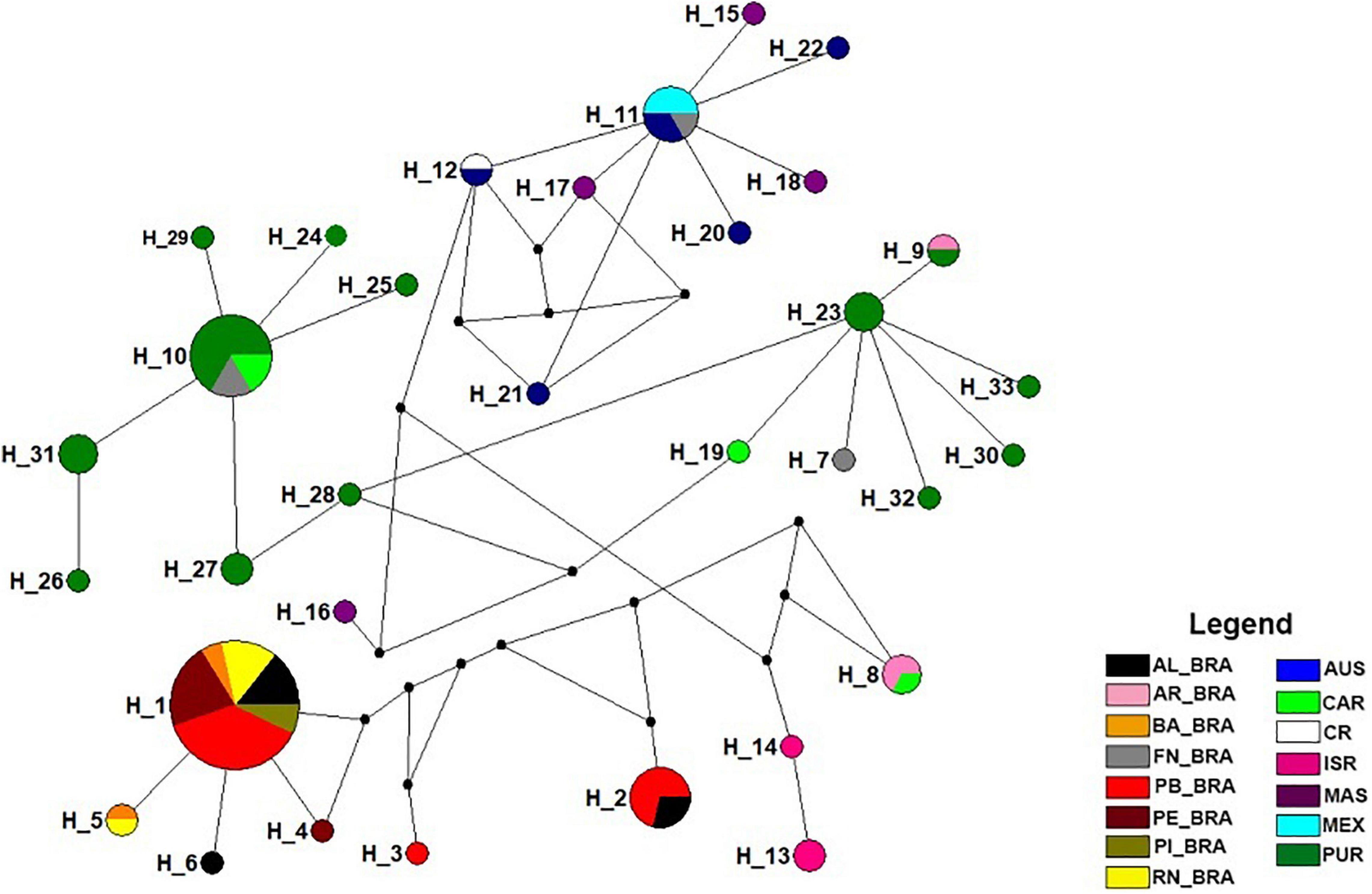
Figure 2. Haplotype network showing the relationships between the 33 haplotypes recorded in the 15 populations of Eretmochelys imbricata. Each circle corresponds to a haplotype, the size being proportional to its frequency. The colors represent the occurrence of haplotypes in the evaluated populations and the small black circles represent the mutational steps. Nesting áreas: Piauí—PI_BRA; Alagoas—AL_BRA; Bahia—BA_BRA; Rio Grande do Norte—RN_BRA; Pernambuco—PE_BRA; Paraíba—PB_BRA. Feeding áreas: Atol das Rocas—AR_BRA; Fernando de Noronha—FN_BRA; Costa Rica—CR; Mexico—MEX; Malaysia—MAS; Caribbean—CAR; Australia—AUS; Puerto Rico—PUR; Israel—ISR.
As for haplotypic diversity (h), the nesting areas had a variation from h = 0.00 (PI_BRA) to h = 0.50 (BA_BRA), and the nucleotide diversity (π) varied from π = 0.00 (PI_BRA) to π = 0.279 (PE_BRA). In feeding areas, MEX showed the lowest diversity (h = 0.00) while the highest indexes were registered in MAS and AUS (h = 1.00 and h = 0.933, respectively), the nucleotide diversity varied between π = 0.00 (MEX) and π = 0.037 (AR_BRA) (Table 1).
Regarding the spatial distribution of haplotypes (H), H_1 was shared between all nesting areas, similar to the EiA01 haplotype recorded for Brazil (Vilaça et al., 2013; Arantes et al., 2020). H_10 (FN_BRA, CAR, PUR) and H_11 (FN_BRA, MEX, AUS) were shared by three areas. Our data also showed that haplotypes H_2 (PB_BRA and AL_BRA), H_5 (BA_BRA and RN_BRA), H_8 (AR_BRA and CAR), H_9 (AR_BRA and PUR), and H_12 (CR, AUS) were shared by two areas simultaneously (Figures 1, 2). Exclusive haplotypes were registered in nine of the 15 sampled areas, with emphasis on PUR with 11 exclusive haplotypes (Figures 1, 2). This location mainly hosts haplotypes EiA01, EiA09, and EiA11 (Velez-Zuazo et al., 2008). Thus, the haplotype network showed that E. imbricata haplotypes in the nesting areas were more related to each other than the ones on feeding areas (Figure 2).
Regarding the genetic structure, the heatmap analysis based on the FST values showed that 15 sampled areas formed five divergent groups (Figure 3 and Supplementary Figure 1). The first group was formed by the nesting areas (BA_BRA; AL_BRA; PE_BRA; PB_BRA; RN_BRA; PI_BRA) and the other groups were restricted to the species’ feeding areas, from which we can highlight two main groups: The first gathering the FN_BRA, CAR, and AR_BRA, and the second by MAS; AUS and MEX (Figure 3). Subsequently, a positive and significant relationship between geographic distance and FST values was demonstrated through the Mantel test (r = 0.2302, p = 0.007) (Figure 4).
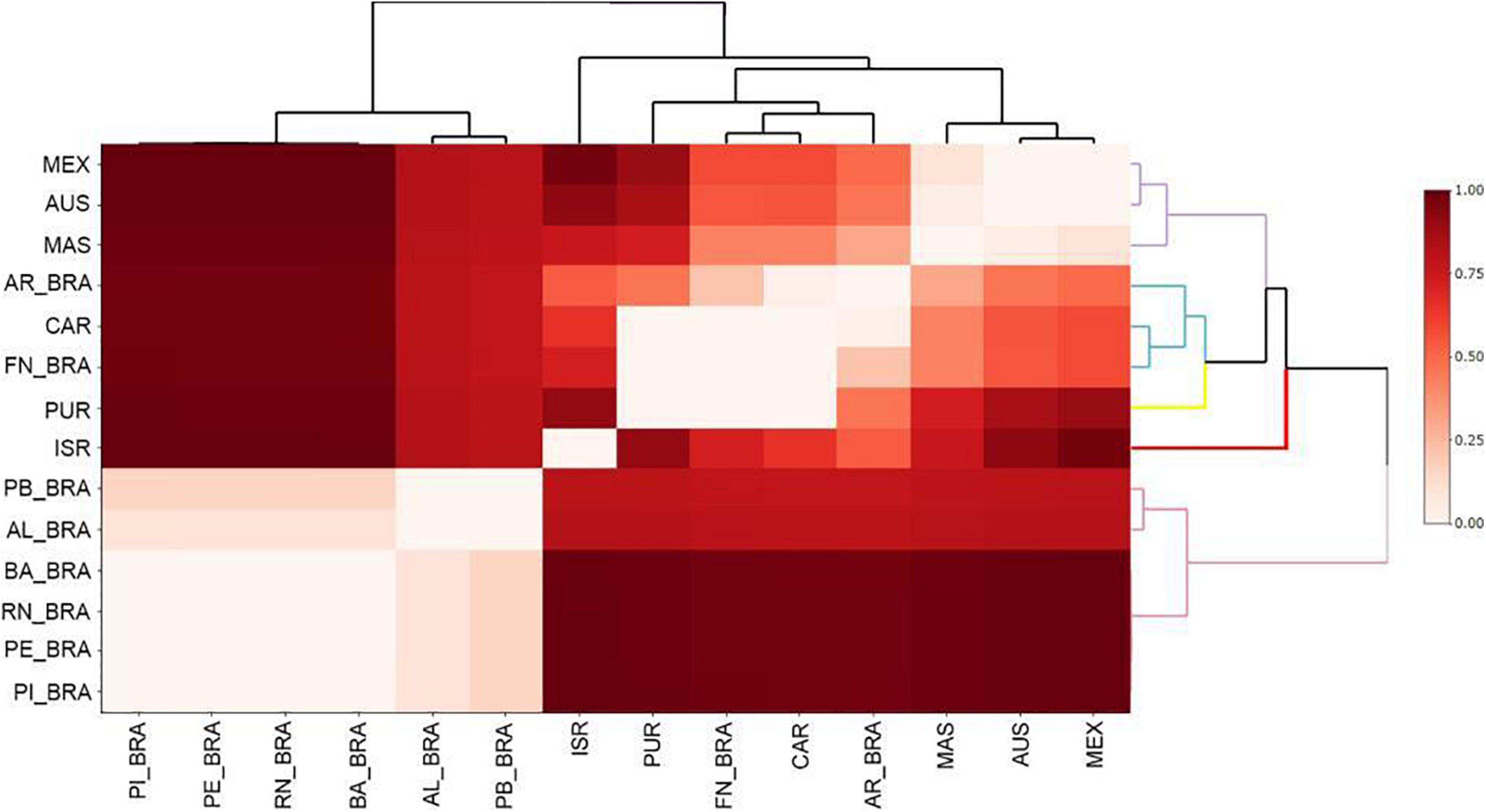
Figure 3. Heatmap of the 14 sampling areas of Eretmochelys imbricata with cluster analysis with Euclidean distance and cluster average method based on FST values. The lines with different colors in the cluster, indicate the five groups identified. Piauí—PI_BRA; Alagoas—AL_BRA; Bahia—BA_BRA; Rio Grande do Norte—RN_BRA; Pernambuco—PE_BRA; Paraíba—PB_BRA; Atol das Rocas—AR_BRA; Fernando de Noronha—FN_BRA; Costa Rica—CR; Mexico—MEX; Malaysia—MAS; Caribbean—CAR; Australia—AUS; Puerto Rico—PUR; Israel—ISR.
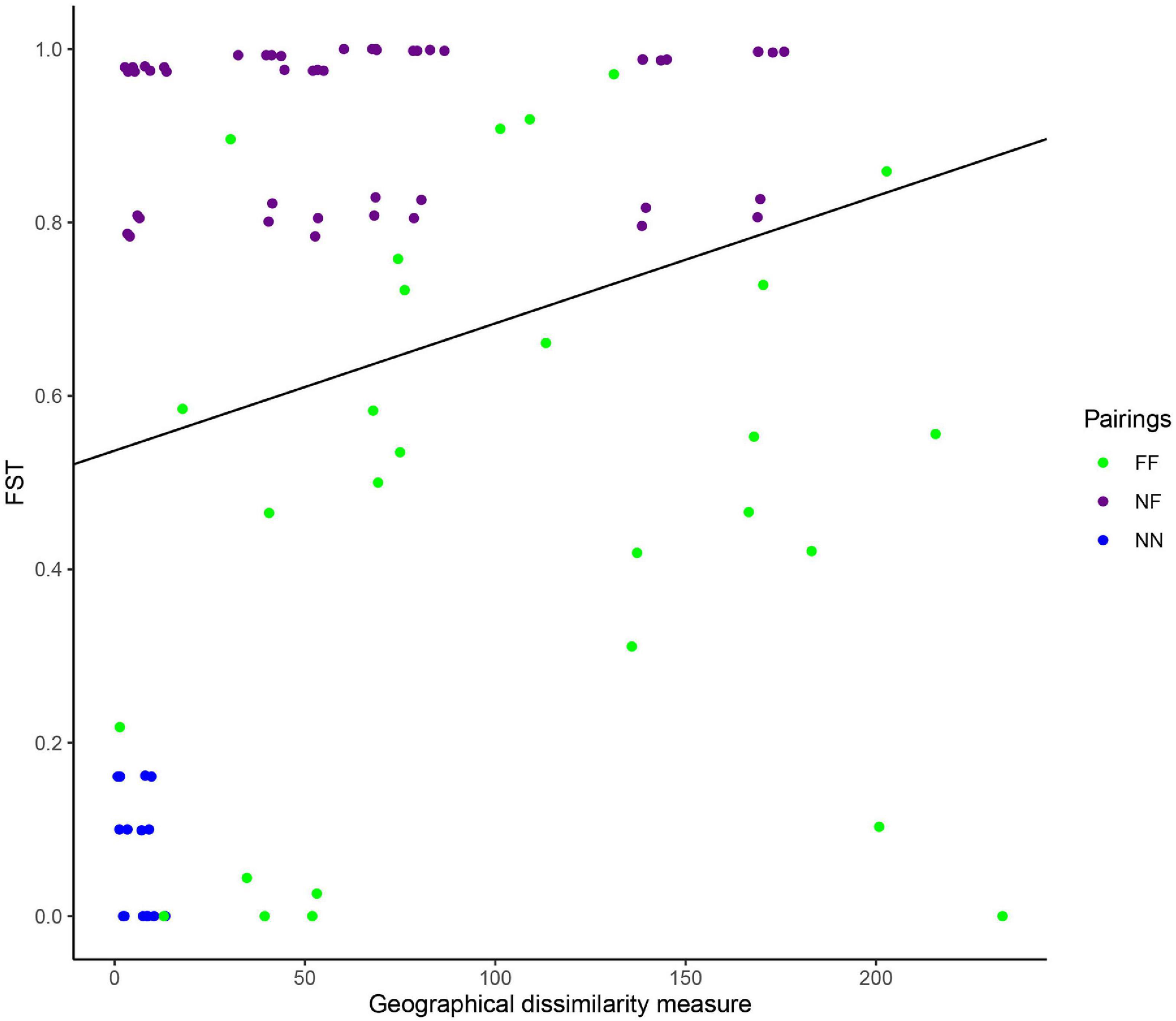
Figure 4. FST values obtained and geographical dissimilarity measure between the 14 populations of Eretmochelys imbricata, with Mantel test (r = 0.2302, p = 0.007). FF, Feeding area X Feeding area; NF, Feeding area X Nesting area; NN, Nesting area X Nesting area.
Discussion
Our work revealed that nesting areas of E. imbricata along the Brazilian coast show low haplotypic diversity, with the presence of exclusive haplotypes. In addition, our data revealed that nesting areas were formed by only a single genetic group, differing from the other genetic groups in feeding areas. Thus, we demonstrated that the evaluated rookeries were made up of only six haplotypes, all of which were registered exclusively for these areas. Thus, these areas are genetically unique, highlighting their importance for preserving the genetic pool of the endangered species (Santos et al., 2013; Vilaça et al., 2013; Arantes et al., 2020).
We registered six exclusive haplotypes of E. imbricata for nesting areas and 27 exclusive haplotypes found in the feeding areas. The predominance of exclusive haplotypes is not uncommon for the hawksbill sea turtle, as Reece et al. (2005) and Labastida-Estrada et al. (2019) also reported restricted haplotypes in Mexico and the Caribbean, as well as Vilaça et al. (2013) recorded haplotype EiA01 being exclusive to the states of Rio Grande do Norte, Ceará, Sergipe, and Bahia, highlighting that this fact may be related to the high degree of female philopatry, that is, fidelity to the places of origin to nidificate (natal homing) (Almeida et al., 2011; Lohmann et al., 2013). Although the presence of exclusive haplotypes is common for the species, it is worth mentioning that the nesting areas consisted of only six haplotypes and that all were exclusive, showing the importance of these areas for the species.
The nesting areas of hawksbill sea turtles were characterized with low haplotypic diversity (ranging from h = 0.00 to h = 0.50), when compared to other reproductive areas in northeastern Brazil, such as Arembepe (h = 0.725), Praia do Forte (h = 0.649) and Sauípe (h = 0.643), as well as being low compared to Islands in the Persian Gulf (h = 0.770 and h = 0.640), the Caribbean (h = 0.642), and the Malaysian (h = 0.3072 and h = 0.679) (Lara-Ruiz et al., 2006; Tabib et al., 2011; Revuelta et al., 2012; Nishizawa et al., 2016). However, there were similarities to the indices previously found for the species in Mexico (h = 0.225 and h = 0.454) (Labastida-Estrada et al., 2019). Although caution is required in interpreting our results, given the small number of individuals sampled inherent to the limitations of working with a critically endangered species, our results show a pattern similar to other results reported in the literature that used larger samples (Tabib et al., 2011; Revuelta et al., 2012; Proietti et al., 2014; Labastida-Estrada et al., 2019). In addition, when we consider the diversity of nucleotides, we observe a pattern similar to that found by Labastida-Estrada et al. (2019) (ranging from π = 0.0005 to π = 0.0006) in nesting areas in Mexico. In general, these data emphasize that the haplotypic and nucleotide diversity recorded in our study is low for the nesting areas of the species, but is within the ranges reported for some studies in the literature. Thus, considering that genetic diversity is essential for species persistence over time (Vilaça et al., 2013), as well as the Brazilian nesting areas, especially the large colonies of Bahia and Rio Grande do Norte, which strongly contribute to the formation of populations of E. imbricata (Vilaça et al., 2013), allied to the fact that it is an endangered species, which suffers great anthropic pressures such as egg collection, illegal fishing, loss of habitat due to disorderly constructions on beaches, vehicular traffic, and garbage ingestion (da Silva et al., 2015), we highlight the importance of these reproduction areas for species maintenance from a genetic perspective. Therefore, we emphasize the demand, to increase protected areas, especially the nesting and hatchling regions of these animals, since these areas showed low genetic diversity. One suggestion would be to expand the protected areas that are close to Conservation Units (UCs), such as nesting areas in the state of Pernambuco that are not part of the Costa dos Corais Environmental Protection Area (APA) and in the state of Paraíba with the APA of Barra do Rio Mamanguape. Most APAs cover relatively small stretches of beach, and according to the nesting data obtained in this study it is evident that a large part is beyond the limits of protection. In addition, it is noteworthy that the studied areas, although they do not present many nests compared to the large reproductive areas, demonstrated to be closely related to other populations of E. imbricata in Brazil (Vilaça et al., 2013; Proietti et al., 2014). Thus, with the expansion of the APAs, beaches where reproduction takes place will be better protected disciplining the fishing activity and disorderly occupation and ensuring the sustainability of the resources that are important for the ecosystem and survival of the species. Greater restrictions are required for those nesting areas that are already part of UCs, mainly during the reproduction of these animals, as in the APA of Delta do Parnaíba/PI and APA Costa dos Corais in Alagoas (Figure 1).
The Haplotypic (h) and nucleotidic (π) diversity of the analyzed feeding areas showed high values following the patterns described in the literature (Lara-Ruiz et al., 2006; Monzón-Arguello et al., 2010; Proietti et al., 2014). Previous studies suggest that sea turtle populations close to warm ocean currents have greater diversity of haplotypes (Bass et al., 2006), since the dispersion of these animals can occur through those currents (Luschi et al., 2003). An example of this is the Fernando de Noronha Archipelago, which due to the influence of the South Equatorial current (north and south of Brazil) has a higher genetic diversity (Vilaça et al., 2013; Proietti et al., 2014). In this context, ocean currents which occur in the Atol das Rocas (AR_BRA) archipelago could be considered a possible explanation for the high genetic diversity in this area. At the same time, Caribbean currents with temporal variations can also contribute to the high diversity in Puerto Rico (PUR) and the Caribbean (CAR) (Blumenthal et al., 2009). As for the diversity of Australia (AUS) and Malaysia (MAS), we believe that they are receiving contributions from the Monsoon Drift and south equatorial currents, however, future studies are needed to test this hypothesis.
When evaluating the spatial distribution of the haplotypes, we observed the sharing of some haplotypes between the nesting areas, such as, for example, H_1 and H_2. This result indicates that, although the sea turtles demonstrate an affinity to reproductive areas, there is a possibility of migrating to nearby areas for nesting (Bowen and Karl, 2007). Furthermore, the proximity and the strong relationship between haplotypes suggest that these areas behave as a single population from the genetic perspective, as demonstrated in the cluster analysis. In addition, it is important to highlight, the fact that there is no sharing of haplotypes between nesting and feeding areas. On the other hand, it is noteworthy that the feeding areas share haplotypes with different frequencies. This pattern may reflect the migration of juvenile individuals from different reproductive places or, due to the migration of individuals after reproduction (Reis et al., 2010; Jensen et al., 2013). It is also important to emphasize that the migration process between the different feeding areas may be under the influence of ocean currents (Luschi et al., 2003; Prosdocimi et al., 2015). Colonization of Atlantic feeding areas happened due to ocean currents from north to south (Encalada et al., 1998; Prosdocimi et al., 2015), and we suggest that the same might have happened for Fernando de Noronha (FN_BRA), CAR, MEX, and PUR, which represented the highest frequencies of the H_10 and H_11 haplotypes in the haplotypic network being similar to the EiA01, EiA02, EiA11, EiA41, and EiA43 haplotypes that have also been reported with high frequencies in these countries (Velez-Zuazo et al., 2008; Vilaça et al., 2013; Cazabon-Mannette et al., 2016; Labastida-Estrada et al., 2019; Arantes et al., 2020). It is also worth noting that changes in ocean conditions, such as water temperature, geographic distance, and physical barriers can influence the dispersal of these animals (Bowen and Karl, 2007; Boyle et al., 2009; Prosdocimi et al., 2015). However, future studies of turtles in feeding areas close to the analyzed nesting areas are necessary to better understand this relationship.
Barriers such as the Amazon Barrier that separates Brazil from the Caribbean, the Central Atlantic Barrier that separates the Americas from Africa and the Benguela Barrier located in the south of the Atlantic (separating the Atlantic Ocean from the Indian Ocean) (Rocha et al., 2005, 2007; Hahn, 2011), may be the reason for the isolation of one group in South America, another in Central America and three more in the Indian Ocean. Such events do not seem to be rare for the species, as according to Reece et al. (2005) the turtles of Mexico were isolated from the Caribbean after historical processes during the Pleistocene. In this sense, although there is a pattern of genetic differentiation by geographical distance, as revealed by the Mantel test, it is important to note that these aforementioned factors can also influence the genetic structure of the species. Besides that, it is important to consider that this pattern of genetic structure may also be associated with other factors, such as changes in ocean conditions; water temperature and ocean currents (Bowen and Karl, 2007; Boyle et al., 2009; Prosdocimi et al., 2015).
Our results provide novel genetic information for some nesting areas of E. imbricata in Brazil. Although there are studies of the species in the country that highlight two important reproduction areas (Vilaça et al., 2013), our data is complementary and reveals that the areas analyzed are composed of a single population (gene pool) with low genetic diversity and exclusive to these locations. Such information can be considered an advance in knowledge about the genetic structure of E. imbricata not yet obtained for Brazil, in addition to contributing to conservation purposes worldwide. Furthermore, the genetic structure of these populations may be a response to the presence of some geographic isolations. Our research suggests that the nesting areas make up a Management Unit that requires a specific management plan for the maintenance of the species. Anthropic factors caused by the development of these locations and local tourism are likely to be threatening the habitat of hawksbill sea turtle populations. Our results also provide unprecedented genetic data relevant to the actions for the conservation of sea turtles.
Data Availability Statement
The datasets presented in this study can be found in online repositories GenBank (ID: MZ615457-MZ615523).
Ethics Statement
The animal study was reviewed and approved by the Ethics Committee on the Use of Animals—CEUA/UESC, the Chico Mendes Institute for Biodiversity Conservation—ICMBio and the Ministry of the Environment—MMA for the consent of the research licenses.
Author Contributions
TS, ES, AS, FG, MC, and MS contributed with contextualization, funding acquisition, research, and methodology and writing. EA contributed to the methodology and writing of the manuscript. VN, WM, RM, WB, and JN contributed to the collection of biological material and contextualization of the manuscript. All authors contributed to the article and approved the submitted version.
Funding
We were grateful to the Rufford Foundation (ID 21566-1) and the State University of Santa Cruz (UESC) for funding the research, to institutions such as Instituto Tartarugas do Delta, Projeto Tamar, NGO Guajiru, NGO Ecoassociados, Instituto Conservation Biota and NGO Pat Ecosmar for their contribution and support to the development of the project.
Conflict of Interest
The authors declare that the research was conducted in the absence of any commercial or financial relationships that could be construed as a potential conflict of interest.
Publisher’s Note
All claims expressed in this article are solely those of the authors and do not necessarily represent those of their affiliated organizations, or those of the publisher, the editors and the reviewers. Any product that may be evaluated in this article, or claim that may be made by its manufacturer, is not guaranteed or endorsed by the publisher.
Acknowledgments
We thank the Bahia Research Foundation (FAPESB) for the scholarship and the Ethics Committee on the Use of Animals—CEUA/UESC, the Chico Mendes Institute for Biodiversity Conservation—ICMBio and the Ministry of the Environment—MMA for the consent of the research licenses. MS thanks Alexander von Humboldt-Stiftung/Coordenação de Aperfeiçoamento de Pessoal de Nível Superior for a scholarship (BEX 0585/16-5) and CNPq for a Research Productivity Grant to MS (309365/2019-8) and to FG (306160/2017-0). We also thank Coordenação de Aperfeiçoamento de Pessoal de nível Superior (CAPES) for providing the AAS postdoctoral fellowship (PNPD 464941/2019-00). We are grateful to Daniela Pareja Mejía for corrections and careful language editing.
Supplementary Material
The Supplementary Material for this article can be found online at: https://www.frontiersin.org/articles/10.3389/fevo.2021.704838/full#supplementary-material
References
Abreu-Grobois, F. A., Horrocks, J., Formia, A., LeRoux, R., Velez-Zuazo, X., Dutton, P., et al. (2006). “New mtDNA Dloop primers which work for a variety of marine turtle species may increase the resolution capacity of mixed stock analysis,” in Proceedings of the 26th Annual Symposium on Sea Turtle Biology and Conservation, eds M. Frick, A. Panagopoulou, A. F. Rees, and K. Williams (Crete), 179.
Almeida, A. P., Santos, A. J. B., Thomé, J. C. A., Belini, C., Baptistotte, C., Marcovaldi, M. A., et al. (2011). Avaliação do estado de conservação da tartaruga marinha Chelonia mydas (Linnaeus, 1758) no Brasil. Biodiversidade Brasileira 1, 12–19. doi: 10.37002/biobrasil.v1i1.87
Arantes, L. S., Vargas, S. M., and Santos, F. R. (2020). Global phylogeography of the critically endangered hawksbill turtle (Eretmochelys imbricata). Genet. Mol. Biol. 43:e20190264. doi: 10.1590/1678-4685-GMB-2019-0264
Bandelt, H. J., Forster, P., and Röhl, A. (1999). Median-joining networks for inferring intraspecific phylogenies. Mol. Biol. Evol. 16, 37–48. doi: 10.1093/oxfordjournals.molbev.a026036
Bass, A. L. (1999). Genetic analysis to elucidate the natural history and behavior of hawksbill turtles (Eretmochelys imbricata) in the wider Caribbean: a review and re-Analysis. Chelonian Conserv. Biol. 3, 195–199.
Bass, A. L., Epperly, S. P., and Braun-McNeill, J. (2006). Green turtle (Chelonia mydas) foraging and nesting aggregations in the Caribbean and Atlantic: impact of currents and behavior on dispersal. J. Hered. 97, 346–354. doi: 10.1093/jhered/esl004
Bass, A. L., Good, D. A., Bjorndal, K. A., Richardson, J. I., Hillis, Z. M., Horrocks, J. A., et al. (1996). Testing models of female reproductive migratory behaviour and population structure in the Caribbean hawksbill turtle, Eretmochelys imbricata, with mtDNA sequences. Mol. Ecol. 5, 321–328. doi: 10.1111/j.1365-294x.1996.tb00323.x
Bell, I., and Jensen, M. P. (2018). Multinational genetic connectivity identified in western Pacific E. imbricata turtles. Wildl. Res. 45, 307–315. doi: 10.1071/WR17089
Bjorndal, K. A., and Bolten, A. B. (2008). Annual variation in source contributions to a mixed stock: implications for quantifying connectivity. Mol. Ecol. 17, 2185–2193. doi: 10.1111/j.1365-294X.2008.03752.x
Blumenthal, J. M., Abreu-Grobois, F. A., Austin, T. J., Broderick, A. C., Bruford, M. W., Coyne, M. S., et al. (2009). Turtle groups or turtle soup: dispersal patterns of hawksbill turtles in the Caribbean. Mol. Ecol. 18, 4841–4853. doi: 10.1111/j.1365-294X.2009.04403.x
Bolten, A. B., Bjorndal, K. A., Martins, H. R., Dellinger, T., Biscoito, M. J., Encalada, S. E., et al. (1998). Transatlantic developmental migrations of loggerhead sea turtles demonstrated by mtDNA sequence analysis. Ecol. Appl. 8, 1–7. doi: 10.1890/1051-0761(1998)008[0001:tdmols]2.0.co;2
Bowen, B. W., and Karl, S. A. (2007). Population genetics and phylogeography of sea turtles. Mol. Ecol. 16, 4886–4907. doi: 10.1111/j.1365-294X.2007.03542.x
Bowen, B. W., Bass, A. L., Soares, L., and Toonen, R. J. (2005). Conservation implications of complex population structure: lessons from the loggerhead turtle (Caretta caretta). Mol. Ecol. 14, 2389–2402. doi: 10.1111/j.1365-294X.2005.02598.x
Boyle, M. C., FitzSimmons, N. N., Limpus, C. J., Kelez, S., Velez- Zuazo, X., and Waycott, M. (2009). Evidence for transoceanic migrations by loggerhead sea turtles in the southern Pacific Ocean. Proc. R. Soc. Lond. B. Biol. Sci. 276, 1993–1999. doi: 10.1098/rspb.2008.1931
Cazabon-Mannette, M., Browne, D., Austin, N., Hailey, A., and Horrocks, J. (2016). Genetic structure of the hawksbill turtle rookery and foraging aggregation in Tobago, West Indies. J. Exp. Mar. Biol. Ecol. 485, 94–101. doi: 10.1016/j.jembe.2016.09.002
Costa, S. A. G. L., Silva, F. J. L., Farias, D. S. D., Fragoso, A. B. L., Costa, T. E. B., Alencar, A. E. B., et al. (2016). “Pesquisa e Conservação de tartarugas marinhas na bacia potiguar, Rio Grande do Norte, Nordeste do Brasil,” in Conservação de Tartarugas Marinhas no Nordeste do Brasil: Pesquisas, Desafios e Perspectivas, eds S. M. J. Correia, E. M. Santos, and G. J. B. Moura (Recife- PE: Universitária da UFRPE), 15–50.
da Silva, V. R. F., Mitraud, S. F., Ferraz, M. L. C. P., Lima, E. H. S. M., Melo, M. T. D., Santos, A. J. B., et al. (2015). Adaptive threat management framework: integrating people and turtles. Environ. Dev. Sustain. 18, 1541–1558. doi: 10.1007/s10668-015-9716-0
Encalada, S. E., Bjorndal, K. A., Bolten, A. B., Zurita, J. C., Schroeder, B., Possardt, E., et al. (1998). Population structure of loggerhead turtle (Caretta caretta) nesting colonies in the Atlantic and Mediterranean as inferred from mitochondrial DNA control region sequences. Mar. Biol. 130, 567–575. doi: 10.1007/s002270050278
Galili, T., O’Callaghan, A., Sidi, J., and Sievert, C. (2017). Heatmaply: an R package for creating interactive cluster heatmaps for online publishing. Bioinformatics 34, 1600–1602. doi: 10.1093/bioinformatics/btx657
Gaos, A. R., Lewison, R. L., Jensen, M. P., Liles, M. J., Henriquez, S., Chavarria, S., et al. (2018). Rookery contributions, movements and conservation needs of hawksbill turtles at foraging grounds in the eastern Pacific Ocean. Mar. Ecol. Prog. Ser. 586, 203–216. doi: 10.3354/meps12391
Gaos, A. R., Lewison, R. L., Liles, M. J., Gadea, V., Altamirano, E., Henríquez, A. V., et al. (2016). Hawksbill turtle terra incognita: conservation genetics of eastern Pacific rookeries. Ecol. Evol. 6, 1251–1264. doi: 10.1002/ece3.1897
Garduño-Andrade, M., Guzmán, V., Miranda, E., Briseño-Dueñas, R., and Abreu-Grobois, F. A. (1999). Increases in hawksbill turtle (Eretmochelys imbricata) nesting in the Yucatan Peninsula, Mexico, 1977–1996: data in support of successful conservation? Chelonian Conserv. Biol. 3, 286–295.
Gorham, J. C., Clark, D. R., Bresette, M. J., Bagley, D. A., Keske, C. L., Traxler, S. L., et al. (2014). Characterization of a subtropical hawksbill sea turtle (Eretmochelys imbricata) assemblage utilizing shallow water natural and artificial habitats in the Florida Keys. PLoS One 9:e114171. doi: 10.1371/journal.pone.0114171
Goslee, S. C., and Urban, D. L. (2007). The ecodist package for dissimilarity-based analysis of ecological data. J. Stat. Softw. 22, 1–19. doi: 10.18637/jss.v022.i07
Hahn, A. T. (2011). Filogeografia global da Tartaruga oliva (Lepidochelys olivacea) (Tese de Doutorado). Porto Alegre, BR: Pontifícia Universidade Católica do Rio Grande do Sul Faculdade de Biociências.
Hall, T. A. (1999). BioEdit: a user-friendly biological sequence alignment editor and analysis program for Windows. Nucleic Acids Symp. Ser. 41, 95–98.
Jensen, P. M., FitzSimmons, N., and Dutton, P. H. (2013). “Molecular genetics of sea turtles,” in The Biology of Sea Turtles, eds J. Wyneken, K. J. Lohmann, and J. A. Musick (Boca Raton, FL: CRC Press), 135–161.
Joseph, J., Nishizawa, H., Arshaad, W. M., Kadir, S. A. S., Jaamana, S. A., Bali, J., et al. (2016). Genetic stock compositions and natal origin of green turtle (Chelonia mydas) foraging at Brunei Bay. Glob. Ecol. Conserv. 6, 16–24. doi: 10.1016/j.gecco.2016.01.003
Labastida-Estrada, E., Machkour-M’Rabet, S., Díaz-Jaimes, P., Cedeño-Vázquez, J. R., and Hénaut, Y. (2019). Genetic structure, origin, and connectivity between nesting and foraging areas of hawksbill turtles of the Yucatan Peninsula: a study for conservation and management. Aquatic. Conserv. Mar. Freshw. Ecosyst. 29, 211–222. doi: 10.1002/aqc.2999
Lara-Ruiz, P., Lopez, G. G., Santos, F. R., and Soares, L. S. (2006). Extensive hybridization in hawksbill turtles (Eretmochelys imbricata) nesting in Brazil revealed by mtDNA analyses. Conserv. Genet. 7, 773–781. doi: 10.1007/s10592-005-9102-9
LeRoux, R. A., Dutton, P. H., Abreu-Grobois, F. A., Lagueux, C. J., Campbell, C. L., Delcroix, E., et al. (2012). Re-examination of population structure and phylogeography of hawksbill turtles in the wider Caribbean using longer mtDNA sequences. J. Hered. 103, 806–820. doi: 10.1093/jhered/ess055
Librado, P., and Rozas, J. (2009). DnaSP v5: a software for comprehensive analysis of DNA polymorphism data. Bioinformatics 25, 1451–1452. doi: 10.1093/bioinformatics/btp187
Lohmann, K. J., Lohmann, C. M. F., Brothers, J. R., and Putman, N. F. (2013). “Natal homing and imprinting in sea turtles,” in The Biology of Sea Turtles, III. Edn, eds J. Wyneken, K. J. Lohmann, and J. A. Musick (Boca Raton, FL: CRC Press), 59–77. doi: 10.1201/b13895-4
López-Barrera, E. A., Reis, E. C., Vilaça, S. T., and Vargas, S. M. (2016). “Genetics and conservation of sea turtles,” in Advances in Research Techniques for the Study of Sea Turtles, 1st Edn, eds J. M. Rguez-Baron, M. M. Lara-Uc, and R. Riosmeena-Rodriguez (New York, NY: Nova Publishers), 119–163.
Luschi, P., Hays, G. C., and Papi, F. (2003). A review of long-distance movements by marine turtles, and the possible role of ocean currents. Oikos 103, 293–302. doi: 10.1034/j.1600-0706.2003.12123.x
Mantel, N. (1967). The detection of disease clustering and a generalized regression approach. Cancer Res. 27, 209–220.
Marcovaldi, M. A., Lopez, G. G., Soares, L. S., Santos, A. J. B., Bellini, C., and Barata, P. C. R. (2007). Fifteen years of hawksbill sea turtle (Eretmochelys imbricata) nesting in northern Brazil. Chelonian Conserv. Biol. 6, 223–228. doi: 10.2744/1071-8443(2007)6[223:fyohst]2.0.co;2
Melo, R. F. (2016). Distribuição Espacial e Temporal de Ninhos de Eretmochelys Imbricata no Litoral Sul do Rio Grande do Norte, Brasil (Monograph Thesis). Rio Grande do Norte RN: Universidade Federal do Rio Grande do Norte.
Monzón-Arguello, C., Rico, C., Marco, A., López, P., and López-Jurado, L. F. (2010). Genetic characterization of eastern Atlantic hawksbill turtles at a foraging group indicates major undiscovered nesting populations in the region. J. Exp. Mar. Biol. Ecol. 387, 9–14. doi: 10.1016/j.jembe.2010.03.004
Moritz, C. (1994). Defining ‘evolutionary significant units’ for conservation. Trends Ecol. Evol. 9, 373–375. doi: 10.1016/0169-5347(94)90057-4
Moura, C. C., de, M., Guimarães, E. D. A. S., Moura, G. J. B., de Amaral, G. J. A., and do Silva, A. C. (2012). Distribuição espaço-temporal e sucesso reprodutivo de Eretmochelys imbricata nas praias do Ipojuca, Pernambuco, Brasil. Iheringia Ser. Zool. 102, 254–260. doi: 10.1590/s0073-47212012005000003
Nishizawa, H., Joseph, J., and Chong, Y. K. (2016). Spatio-temporal patterns of mitochondrial DNA variation in hawksbill turtles (Eretmochelys imbricata) in Southeast Asia. J. Exp. Mar. Biol. Ecol. 474, 164–170. doi: 10.1016/j.jembe.2015.10.015
Pérez-Bermúdez, E., Urquiola, A. R., Rebordinos-González, L., Espinosa-López, G., Lee-González, I., and Petric, B. L. J. (2017). A new approach to mixed stock analysis that informs on the management and conservation of hawksbill in the wider Caribbean: the case of the legal fishery in Jardines del Rey, Cuba. Int J. Mar. Sci. 7, 316–343. doi: 10.5376/ijms.2017.07.0033
Pritchard, P. C. H., and Mortimer, J. A. (1999). “Taxonomy, external morphology, and species identification,” in Research and Management Techniques for the Conservation of Sea Turtles. IUCN/SSC Marine Turtle Specialist Group Publication. No. 4, eds K. L. Eckert, K. A. Bjorndal, F. A. Abreu-Grobois, and M. Donnelly (Gland: IUCN), 21–38.
Proietti, M. C., Reisser, J., Marins, L. F., Rodriguez-Zarate, C., Marcovaldi, M. A., Monteiro, D. S., et al. (2014). Genetic structure and natal origins of immature hawksbill turtles (Eretmochelys imbricata) in Brazilian waters. PLoS One 9:e88746. doi: 10.1371/journal.pone.0088746
Prosdocimi, L., Bugoni, L., Albareda, D., and Remis, M. I. (2015). Are stocks of immature loggerhead sea turtles always mixed? J. Exp. Mar. Biol. Ecol. 466, 85–91. doi: 10.1016/j.jembe.2015.02.006
Putman, N. F., Abreu-Grobois, F. A., Broderick, A. C., Ciofi, C., Formia, A., Godley, B. J., et al. (2014). Numerical dispersal simulations and genetics help explain the origin of hawksbill sea turtles in Ascension Island. J. Exp. Mar Biol. Ecol. 450, 98–108. doi: 10.1016/j.jembe.2013.10.026
R Core Team (2020). R: A Language and Environment for Statistical Computing. Vienna: R Foundation for Statistical Computing.
Reece, J. S., Castoe, T. A., and Parkinson, C. L. (2005). Historical perspectives on population genetics and conservation of three marine turtle species. Conserv. Genet. 6, 235–251. doi: 10.1007/s10592-004-7821-y
Reis, E. C., and Goldberg, D. W. (2017). “Pesquisa e conservação de tartarugas marinhas no Brasil e as recentes contribuições da telemetria e da genética,” in Mamíferos, Quelônios e Aves: Caracterização Ambiental Regional da Bacia de Campos, Atlântico Sudoeste, eds E. C. Reis and M. P. Curbelo-Fernandez (Rio de Janeiro, BR: Elsevier Habitats), 91–120. doi: 10.1016/b978-85-352-7661-9.50005-1
Reis, E. C., Soares, L. S., Vargas, S. M., Santos, F. R., Young, R. J., Bjorndal, K. A., et al. (2010). Genetic composition, population structure and phylogeography of the loggerhead sea turtle: colonization hypothesis for the Brazilian rookeries. Conserv. Genet. 11, 1467–1477. doi: 10.1007/s10592-009-9975-0
Revuelta, O., León, Y. M., Feliz, P., Godley, B. J., Raga, J. A., and Tomás, J. (2012). Protected areas host important remnants of marine turtle nesting stocks in the Dominican Republic. Oryx 46, 348–358. doi: 10.1017/S0030605311001529
Richardson, P. B., Bruford, M. W., Calosso, M. C., Campbell, L. M., Clerveaux, W., Formia, A., et al. (2009). Marine turtles in the Turks and Caicos Islands: remnant rookeries, regionally significant foraging stocks, and a major turtle fishery. Chelonian Conserv. Biol. 8, 192–207. doi: 10.2744/CCB-0871.1
Rocha, L. A., Craig, M. T., and Bowen, B. W. (2007). Phylogeography and the conservation of coral reef fishes. Coral Reefs 26, 501–512. doi: 10.1007/s00338-007-0261-7
Rocha, L. A., Robertson, D. R., Rocha, C. R., Tassel, J. L. V., Craig, M. T., and Bowen, B. W. (2005). Recent invasion of the tropical Atlantic by an Indo-Pacific coral reef fish. Mol. Ecol. 14, 3921–3928. doi: 10.1111/j.1365-294X.2005.02698.x
Santana, W. M., Silva-Leite, R. R., Silva, K. P., and Machado, R. A. (2009). Primeiro registro de nidificação de tartarugas marinhas das espécies Eretmochelys imbricata (Linnaeus, 1766) e Lepidochelys olivacea (Eschscholtz, 1829), na região da Área de Proteção Ambiental Delta do Parnaíba, Piauí, Brasil. Panam. J. Aquat. Sci. 3, 369–371.
Santos, A. J. B., Bellini, C., Vieira, D. H. G., Neto, L. D., and Corso, G. (2013). Northeast Brazil shows highest hawksbill turtle nesting density in the South Atlantic. Endanger. Species Res. 21, 25–32. doi: 10.3354/esr00505
Tabib, M., Zolgharnein, H., Mohammadi, M., Salari-Aliabadi, M. A., Qasemi, A., Roshani, S., et al. (2011). mtDNA variation of the critically endangered hawksbill turtle (Eretmochelys imbricata) nesting on Iranian islands of the Persian Gulf. Genet. Mol. Res. 10, 1499–1503. doi: 10.4238/vol10-3gmr1148
Troëng, S., Dutton, P. H., and Evans, D. (2005). Migration of hawksbill turtles Eretmochelys imbricata from Tortuguero, Costa Rica. Ecography 28, 394–402. doi: 10.1111/j.0906-7590.2005.04110.x
Trujillo-Arias, N., Amorocho, D. F., López-Álvarez, D., and MejíaLadino, L. M. (2014). Phylogeographic relations of some feeding and nesting of hawksbill turtle rookeries (Eretmochelys imbricata) in the Caribbean and Pacific of Colombia. Bulll. Mar. Coast. Res. 43, 159–182. doi: 10.3354/esr003159
Vargas, S. M., Jensen, M. P., Ho, S. Y. W., Mobaraki, A., Broderick, D., Mortimer, J. A., et al. (2016). Phylogeography, genetic diversity, and management units of hawksbill turtles in the Indo-Pacific. J. Hered. 107, 199–213. doi: 10.1093/jhered/esv091
Velez-Zuazo, X., Ramos, W. D., Van Dam, R. P., Diez, C. E., AbreuGrobois, A., and McMillan, W. O. (2008). Dispersal, recruitment and migratory behaviour in a hawksbill sea turtle aggregation. Mol. Ecol. 17, 839–853. doi: 10.1111/j.1365-294x.2007.03635.x
Vilaça, S. T., Lara-Ruiz, P., Marcovaldi, M. A., Soares, L. S., and Santos, F. R. (2013). Population origin and historical demography in hawksbill (Eretmochelys imbricata) feeding and nesting aggregates from Brazil. J. Exp. Mar Biol. Ecol. 446, 334–344. doi: 10.1016/j.jembe.2013.06.004
Wallace, B. P., Di Matteo, A. D., Hurley, B. J., Finkbeiner, E. M., Bolten, A. B., Chaloupka, M. Y., et al. (2010). Regional management units for marine turtles: a novel framework for prioritizing conservation and research across multiple scales. PLoS One 5:e15465. doi: 10.1371/journal.pone.0015465
Keywords: hawksbill turtle, mtDNA, haplotypes, population structure, Brazil, Eretmochelys imbricata
Citation: Simões TN, Santos EMd, Santos AS, Gaiotto FA, Costa MA, Assis ETCdM, Neves VCdS, Magalhães WMdS, Mascarenhas R, Bonfim WAG, Neves JN and Solé M (2021) Low Diversity and Strong Genetic Structure Between Feeding and Nesting Areas in Brazil for the Critically Endangered Hawksbill Sea Turtle. Front. Ecol. Evol. 9:704838. doi: 10.3389/fevo.2021.704838
Received: 12 May 2021; Accepted: 15 July 2021;
Published: 04 August 2021.
Edited by:
Claudio Oliveira, São Paulo State University, BrazilReviewed by:
Julian Walcott, The University of the West Indies, Cave Hill, BarbadosEduardo Cuevas, CONACYT-Universidad Autónoma del Carmen, Mexico
Copyright © 2021 Simões, Santos, Santos, Gaiotto, Costa, Assis, Neves, Magalhães, Mascarenhas, Bonfim, Neves and Solé. This is an open-access article distributed under the terms of the Creative Commons Attribution License (CC BY). The use, distribution or reproduction in other forums is permitted, provided the original author(s) and the copyright owner(s) are credited and that the original publication in this journal is cited, in accordance with accepted academic practice. No use, distribution or reproduction is permitted which does not comply with these terms.
*Correspondence: Thyara Noely Simões, thyara.noely@gmail.com
†These authors have contributed equally to this work and share first authorship