- 1Laboratorio de Biología Marina, Departamento de Zoología, Facultad de Biología, Universidad de Sevilla, Seville, Spain
- 2Área de Investigación I+D+i del Acuario de Sevilla, Seville, Spain
- 3Estación de Biología Marina del Estrecho, Ceuta, Spain
- 4Estudios Geológicos Marinos (Esgemar S.A.), Málaga, Spain
- 5Citizen Science linked to Laboratorio de Biología Marina, Departamento de Zoología, Facultad de Biología, Universidad de Sevilla, Seville, Spain
- 6Biodiversidad y Ecología Acuática de la Universidad de Sevilla, Departamento de Zoología, Facultad de Biología, Seville, Spain
The invasive macroalga Rugulopteryx okamurae represents an unprecedented case of bioinvasion by marine macroalgae facing the European coasts. Since the first apparition of the species in the Strait of Gibraltar in 2015, its fast dispersion along the introduced habitats constitutes a real challenge to develop monitoring strategies that ahead of its impacts. The present study uses three different approaches to address impacts on the benthic ecosystems, at the same time offers relevant data for future management actions in El Estrecho Natural Park (PNE). Information obtained by monitoring permanent sentinel stations revealed a significant loss in resident species coverage after the moment of maximum growth in 2017. Thus, despite coverage of R. okamurae did not strongly varied in the latter years, impacts generated remain high in the habitats studied. Estimations of the invasive species coverage by combining cartographic image analysis and in situ data predicted a major occupation (over 85% coverage) between 10 and 30 m, coinciding with the maximum rocky surface areas (m2) mapped on the PNE. Furthermore, a Citizen Science research collaboration evidenced impacts on the benthic seascape through an ad hoc exploration of images that allowed a “before” and “after” comparison of the invasion process in the same geographic locations. This has made it possible to graphically demonstrate severe changes in the underwater seascape and, therefore, the general impact of this new biological invasion. The spatial colonization estimations combined with the impacts reported by both scientific [Sessile Bioindicators in Permanent Quadrats (SBPQ) sentinel stations] and civilian (Citizen Science) monitoring methodologies claim the urgent development of further studies that allow the design of monitoring strategies against R. okamurae expansion across the Mediterranean and Atlantic waters.
Introduction
The Strait of Gibraltar is a hot spot area for marine biodiversity in the Atlantic-Mediterranean waters with great biogeographic importance due the coalesce of the Lusitanian, Mediterranean and Mauritanian regions (Ekman, 1953; Briggs, 1974; García-Gómez, 2002). Together with the Alboran Sea, the Strait coasts harbor species from the northern Atlantic, subtropical waters of the northwestern of Africa and the Mediterranean Sea (Templado, 2011; Mannino et al., 2017; Urra et al., 2017). Its species richness and habitat diversity is influenced by the littoral physiographic complexity, which has led to the co-occurrence and dominance of different biological strategies and thus affecting the composition of benthic communities (Zabala and Ballesteros, 1989). The biodiversity of this area has been largely assessed by a list of references in terms of foundation species [macroalgae (Flores-Moya et al., 1995a, b; Conde et al., 1996; Adama et al., 2021) and sessile macroinvertebrates as ascidians (Carballo et al., 1997) or molluscs (García-Gómez, 2002; Gofas et al., 2011)] and associated fauna (e.g., García-Raso, 1993; Conradi et al., 2000; Castello and Carballo, 2001; Guerra-García et al., 2009; García-Raso et al., 2011).
However, the benthic systems have changed over time according to different biotic and abiotic factors (see Gallardo et al., 2021). The biodiversity and species richness have been threatened by several anthropogenic pressures for many years, mainly due to the proximity to pollution resources (e.g., chemical industries, thermal plants, oil slick) (Morales, 2007; Soussi et al., 2020), artificial infrastructures (Sánchez-Moyano et al., 2000; Sedano et al., 2020) or maritime shipping and human activities (Bianchi et al., 2013; Nachite et al., 2020). Indeed, the Strait of Gibraltar supports the highest density of maritime traffic in the Western Mediterranean (Abdulla and Linden, 2008; Endrina et al., 2018). This implies a threat due to marine bioinvasions since ballast waters and boat hulls fouling are recognized as potential transport vectors for non-indigenous species (NIS) (Ribera-Siguan, 2003).
Favorable environmental conditions increase the settlement success of invaders (Villèle and Verlaque, 1995), which in turn compromise the survival of surface-dependent organisms by changing the physical characteristics and available substrata (Mannino et al., 2017). Macrophytes (macroalgae and seagrases) constitute the dominant group of invasive species in the Western Mediterranean (Zenetos et al., 2010, 2012). In the recent years, a number of studies have been performed on invasive macroalgae that have interacted the resident sessile communities promoting also cascading influence on associated fauna in the Strait waters. Some examples are Asparagopsis armata Harvey (Boudouresque and Verlaque, 2002; Guerra-García et al., 2012), Asparagopsis taxiformis (Delile) Trevisan de Saint-Léon (Altamirano et al., 2008; Navarro-Barranco et al., 2018), Caulerpa cylindracea Sonder (Altamirano et al., 2014); Womersleyella setacea (Hollenberg) R. E. Norris (Bedini et al., 2015), or Lophocladia lallemandii (Montagne) F. Schmitz (Ballesteros et al., 2007). Despite positive and neutral effects may also occur [e.g., Caulerpa racemosa (Forsskål) J. Agardh may maintain caprellid populations in shallow Mediterranean habitats (Vázquez-Luis et al., 2009)], these species have displayed pronounced and drastic effects on the underwater seascape making it difficult the implement success of strategies to mitigate their impacts (Anderson, 2007; Klein and Verlaque, 2008).
The last notorious case of macroalga colonizing the Western Mediterranean littorals is the species Rugulopteryx okamurae (E. Y. Dawson) I.K. Hwang, W.J. Lee, and H.S. Kim. This brown macroalga, originated from the northwest of Asia (Hwang et al., 2009), has been systematically reported on the coasts of the Strait of Gibraltar since 2015 (see Altamirano-Jeschke et al., 2016), when more than 5,000 tons of wracks were extracted from the beaches of its south coasts, in the city of Ceuta (North-Africa) (Ocaña et al., 2016). The rocky bottoms of The Jbel Moussa Site of Biological and Ecological Interest (SIBE) (El Aamri et al., 2018) and the eastern littoral of El Estrecho Natural Park (PNE) (García-Gómez et al., 2018) firstly represented the northern and southern scenarios of R. okamurae expansion in the Strait of Gibraltar. Until date, these areas constitute the most intensely affected by the brown alga, which continues its westward and eastward directionality of expansion (Altamirano et al., 2019; Figueroa et al., 2020) with trend to monopolize the sea rocky bottom in detriment of the photophilous resident biota (García-Gómez et al., 2020b). In the introduced areas, the species is present throughout the year and is dispersed mainly due to asexual and vegetative strategies by propagules and monospores (Altamirano-Jeschke et al., 2017; Altamirano et al., 2019). Although it has not been possible to assess if the species is able to complete its entire life cycle in the Atlantic and Mediterranean waters (Verlaque et al., 2009; Altamirano-Jeschke et al., 2016, 2017), the fast expansion and massive occupation potential since its first detection in 2015 reflects that this bioinvasion case is one of the most serious and threatening caused by marine macroalgae in the European waters (García-Gómez et al., 2018, 2020b).
It is urgent to carry out studies on the distribution, ecology and impacts of R. okamurae in the Mediterranean and the Atlantic coasts, as well as the implementation of management measures. However, few studies have been carried out on R. okamurae distribution (e.g., Altamirano-Jeschke et al., 2016; Ocaña et al., 2016; El Aamri et al., 2018; Altamirano et al., 2019) and its derived impacts on the recipient sessile (García-Gómez et al., 2018, 2020b; Sempere-Valverde et al., 2020) and mobile associated biota (Navarro-Barranco et al., 2019). In this regard, there is only one published study monitoring the temporal dynamic of the invasion since the first apparition of the species in the Strait waters (see García-Gómez et al., 2020b) by the utilization of Sessile Bioindicators in Permanent Quadrats (SBPQ). The SBPQ is part of a monitoring of sessile sentinel species which has been carried out since 2013 to detect early impacts on the littoral environment, including changes in the benthic system related to global warming (García-Gómez, 2015). Thus, this methodology has allowed to document not only the establishment of R. okamurae but also the disappearance of target species which become displaced in the absence of environmental stability.
Long-term monitorings, as observational studies that obtain full ecological characterizations in environmental evaluations (Moschella et al., 2005), are essential for the management of invasive species, their early detection and the implementation of a rapid response (Lodge et al., 2006; Williams and Smith, 2007; García-Gómez, 2015). In this sense, Citizen Science is a research collaboration strategy involving members of the public in scientific research projects to address real-world problems (Wiggins and Crowston, 2011). This constitutes an emerging movement of citizen participation given the breakdown or reduction of barriers caused by advances in communication technologies. Although there are challenges to effectively use citizen-generated data to monitor invasive species on a global scale (Earp and Liconti, 2020; Johnson et al., 2020), it can be a useful tool to detect and monitoring the bioinvasion processes (present study). In this sense, special emphasis has been placed on the underwater seascape, as highlighted in previous works performed in other research fields (Pittman et al., 2011; Gobert et al., 2014; Cheminée et al., 2016; Schejter et al., 2017; Ceraulo et al., 2018). The monitoring of an area by comparative images reflecting “before” and “after” scenarios due to bioinvasion processes as a proxy of BACI analyses (Before After Control Impact) (Underwood, 1994; Montefalcone et al., 2008; Conner et al., 2015; Donázar-Aramendía et al., 2018, 2020), may offer key information on the degree of affection that the local ecosystem has suffered and the risk of its prevalence in the area. Among other aspects, the comparative analysis of images can allow inferences regarding the behavior of biota and ecological connectivity, since the underwater seascape can have a great influence on it (Grober-Dunsmore et al., 2009).
The overall aim of this study was to advance our knowledge on the R. okamurae bioinvasion case targeting efforts on different monitoring approaches. Thus, objectives were threefold: (1) to provide updated data about the evolution of R. okamurae and key resident taxa in pre-coralligenous habitats and to test better designs for future monitoring programs; (2) to estimate the coverage of the invasive species on the rocky surface areas of the PNE, providing mapping areas of suitable rocky substrata to host the invasive species; and (3) to offer graphical evidences of radical changes before and after the spatial establishment of R. okamurae along the PNE littoral through Citizen Science collaborations.
Materials and Methods
Study Area
The present study is focused on the northern coastline of the Strait of Gibraltar. Concretely, the PNE, which is located in the southern Iberian Peninsula and included within the Marine Protected Areas (MPAs) of the Andalusian Intercontinental Biosphere Reserve (Spain-Morocco). Situated between the Atlantic Ocean (western coasts) and the Mediterranean Sea (eastern coasts), the PNE extends from southern Spain to northern Morocco, separating Europe and Africa by 14 km distance. The study area includes the eastern littoral of the PNE coastline, between Tarifa Island (36°05′20.38″N, 5°48′45.34″O) and Punta Carnero (Algeciras) (36°4′30.18″N, 5°25′11.16″O) (Figure 1).
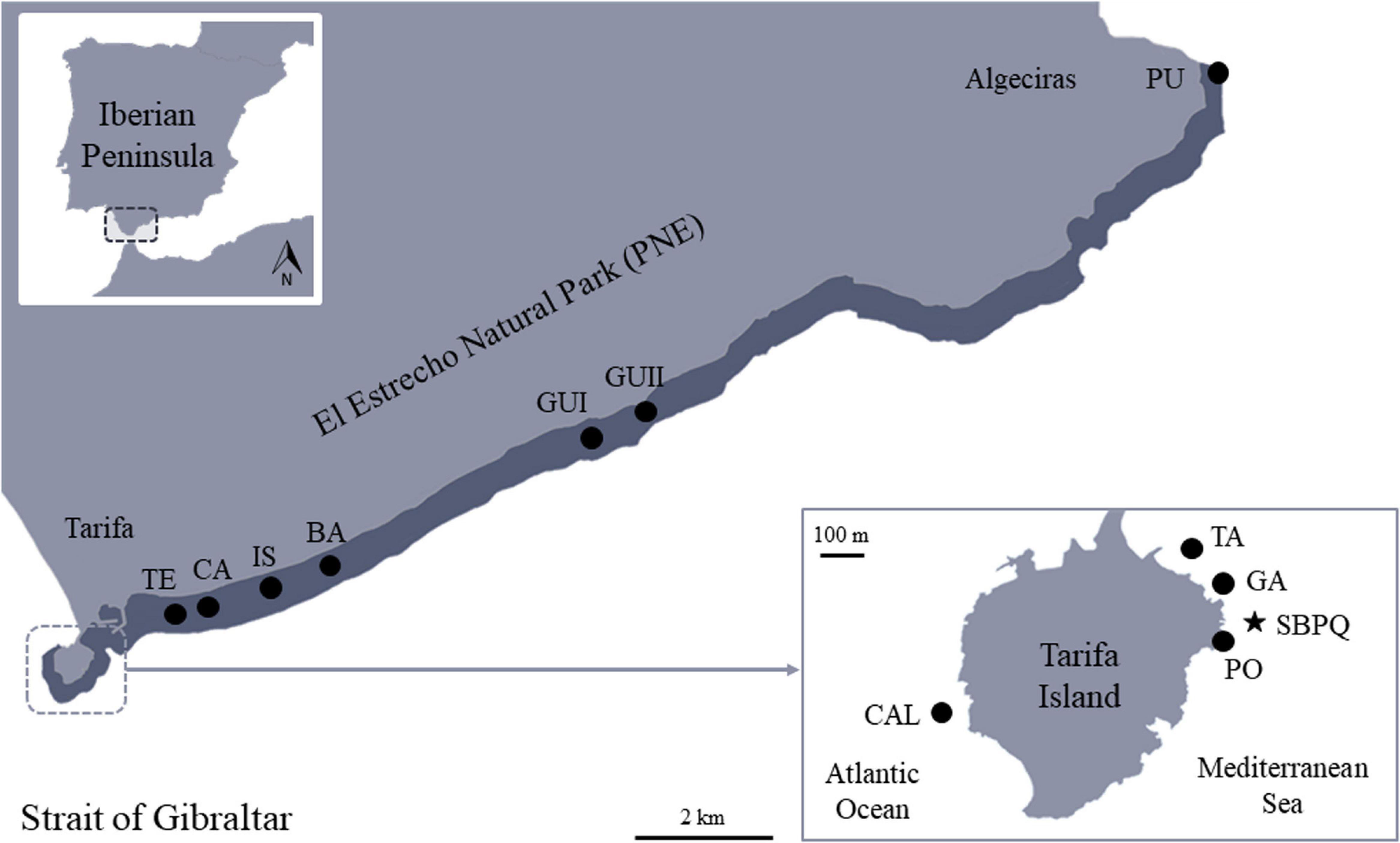
Figure 1. Location of the study area in the Strait of Gibraltar showing the different sampling stations for each research objective. Star: location of the SBPQ station in the Tarifa Island; Dark band: eastern littoral of El Estrecho Natural Park (PNE) subjected to estimations of R. okamurae coverage-affections; Black points: sampling stations for Citizen Science collaborations. CAL = Caldera Profunda; TA = Tanque Gasoil; GA = La Garita; PO = Pozas Intermareales; TE = El Tejar; CA = La Caleta; IS = La Isleta; BA = Barranco Hondo; GUI = Guadalmesí I; GUII = Guadalmesí II; PU = Punta Carnero.
Monitoring of the Submarine SBPQ Station of Tarifa Island
The sessile community at the SBPQ station of Tarifa Island (Figure 1) was seasonally sampled on partially shaded vertical walls of the pre-coralligenous habitats (15 m depth) during 2013–2017 by García-Gómez et al. (2020b). The SBPQ methodology is specifically designed to assess impacts on sensitive species characterized by long-life cycles, thus providing evidences in any period of the year. This methodology has been designed as a simple, non-invasive, underwater environmental alert tool for the potential early detection of environmental impacts of anthropic origin in the sublittoral system: in the short term (local alterations derived from pollutants, coastal dredging or civil engineering works, early detection of exotic species with invasive potential, etc.), and in the medium or long term (global warming) (García-Gómez, 2015; García-Gómez et al., 2020a). Because R. okamurae is present all-year round in the Strait of Gibraltar (pers. obs.) and most of target species from the sentinel station of Tarifa showed at least 10% of coverage in all seasons (see García-Gómez et al., 2020b), it could be expected that this long-term technique based on permanent quadrats will allow the detection of changes in the coverage of the invasive and the target species only considering one sampling season. In this study, the monitoring activities started in 2013 were temporally continued until 2020 and only considering winters (no data are available from 2018, when sampling was missed because of technical issues).
The monitoring station of Tarifa Island is composed of three 1 × 1 m fixed quadrats separated 5–10 m from each other. Within each fixed quadrat, four photo-quadrats of 50 × 50 cm were collected using in situ photographs via scuba diving. Following the methodology proposed by García-Gómez (2015), García-Gómez et al. (2020a) and the monitoring study performed for 2013–2017 (García-Gómez et al., 2020b), species coverage (percent) was obtained by superposing 10 × 10 grid over each photo-quadrat obtained for each fixed quadrat. Since the methodology overestimates the species-cover, the total percentage coverage in each photo-quadrat exceeded 100%.
To visualize the spatial distribution of replicates (quadrats) among years, a non-metric multidimensional scaling (nmMDS) based on a Bray–Curtis similarity matrix was performed on the square root transformed coverage data considering all the data pool since 2013. Differences in the sessile community structure over the sampling years were analyzed using PERMANOVA analysis under a nested design (sampling times were defined as random factor, “Season,” nested within “Year,” fixed factor with seven levels: 2013–2020). P-values were obtained through a Monte Carlo test when small number of unique permutations were obtained (Anderson et al., 2008). Also, the homogeneity of the data dispersion among samples was tested for the fixed factor “Year,” using a permutational analysis of multivariate dispersions (PERMDISP) (Anderson, 2006). The contribution of each species to the Bray–Curtis similarity was obtained using a SIMPER (SiMilarity PERcentages) analysis. After performing analyses considering the whole data base, we wanted to test if the methodology was functional only sampling once a year considering the community present in this sentinel station. We considered winters as the season of the year most appropriate to test whether the SBPQ methodology is minimally robust, since it is the time when it is expected (for middle latitudes) that the development and growth of the invasive macroalga is lower (this has been supported by pers. obs. and growth data for R. okamurae in Japan (Agatsuma et al., 2005), with similar north latitude to Spain: between 20° and 40°). Thus, the sensitivity of the SBPQ methodology was tested repeating all the analyses but only considering the data from the winter of each year (i.e., factor “Year,” with seven levels: 2013–2020). Overall multivariate analyses were carried out using PRIMER-e v6 PERMANOVA + software (Clarke and Gorley, 2006; Anderson et al., 2008).
Estimations of Rugulopteryx okamurae Coverage in the PNE
The sublittoral physical cartography of the Strait of Gibraltar (0–1 nautical mile zone) (García-Gómez et al., 2003; see also CMAyOT Consejería de Medio Ambiente y Ordenacion del Teritorio, 2008; MITECO Ministerio para la Transición Ecológica, 2012) was used to estimate the geographical and bathymetric expansion of R. okamurae along the sublittoral coastline of the PNE (Figure 1). Thus, R. okamurae coverage data were estimated and applied to bathymetric intervals of rocky sublittoral habitats (0–5, 5–10, 10–20, 20–30, and 30–40 m) by averaging coverage data obtained at the horizontal illuminated rocky surfaces of the southern coasts of the Strait (summer 2018, at 14 km from the study area; data previously published in García-Gómez et al., 2020b). Thus, the degree of coverage of the rocky bottoms were mapped using a polygon methodology for each bathymetric interval considered as follow: (1) A digital bathymetry model (DTM) was made in the PNE area and a 3-m orthogonal mesh was generated (surfer application from Golden Software, LLC was used). (2) The polygons of the outcropping rocks were extracted from the GIS database and (3) an intersection operation was performed between the DTM and the outcropping rock polygons. For this, the geo-processing tools of the Qgis application with the GNU general public license was used. (4) Once the DTM of the bathymetry was obtained, surface of the outcropping rock in each bathymetric interval was automatically calculated using a basic scripting language code from Golden Software, LLC (Contarea2.bas, 2000). The planar area (projected surface in the horizontal plane) and the real surface were calculated. (5). Finally, to generate a mapping of the rocky areas, the bathymetric DTM was divided in the ranges described above, so percentage coverages per bathymetric interval considered were displayed.
“Before-After” Underwater Seascape Approximation by Citizen Science Initiative
With the aid of local scuba divers and citizen scientists, the research group of this study often obtains useful information in environmental assessments on the Strait coasts and Algeciras bay. In this regard, occasional observational surveys of the benthic communities have been performing on the subtidal coastline of the PNE for more than 10 years, providing valuable evidences of the underwater seascape dynamic over time. In this study, 11 video graphically monitored sites between Tarifa and Punta Carnero (Algeciras) (Figure 1) have been selected to compare environmental scenarios before and after the first establishment of R. okamurae on the northern Strait of Gibraltar (2015). Citizen participants were constituted by experienced local divers, that carefully recorded the underwater seascape every time they performed their activities in the sites indicated. With the attempt to combine minimal diving efforts with the widest relevant information obtained (and thus, an optimal collaboration), data was recorded by video graphic instead of photographic methods (Cabatain et al., 2006). Thus, citizen and scientific collaborators provided a large number of video graphic data, of which individual video frames were selected and analyzed in the laboratory.
Data from videos recorded between Tarifa Island and Punta Carnero (i.e., El Tejar, La Caleta, La Isleta, Barranco Hondo, Guadalmesí I, Guadalmesí II and Punta Carnero) yielded information on the whole natural rocky area between 3 and 10 m depth, while videos from Tarifa Island offered data on artificial substrata (i.e., Tanque Gasoil and Caldera Profunda; 7–10 m depth) and natural sublittoral (i.e., La Garita; 10–14 m depth) and intertidal habitats (i.e., Pozas Intermareales). Images taken before and after the first citation of R. okamurae in the Strait coasts (2015) were then selected and compared providing different scenarios of the underwater seascape and bioinvasion consequences.
Results
Sessile Communities at the SBPQ Monitoring Station of Tarifa (2013–2020)
Results obtained showed that the percentage coverage of the sessile species at the SBPQ sentinel station located on vertical surfaces of shady pre-coralligenous habitats in Tarifa Island suffered a decrement in terms of species percent coverage until 2020, excepting R. okamurae and the coral Astroides calycularis (Pallas, 1766), which slightly increased after 2019 (Figure 2). PERMANOVA results revealed no evidences of significant differences in the community structure between 2019 and 2020. However, it significantly differed from previous years (2013–2017) (Table 1A and Figure 3A). The same pattern was observed when the community from 2017 was compared with the rest of sampling years. SIMPER analysis revealed that dissimilarities with previous years were mainly given by R. okamurae mean coverage values, which highly increased until 2017 (Supplementary Table 1). Instead, R. okamurae coverage decreased after 2017, and only contributed to differences with most recent data (2019–2020) in 13 and 15%, respectively. In this case, it was the native community which mainly contributed to dissimilarities, with an accumulative contribution up to 60% in both cases and lower average values.
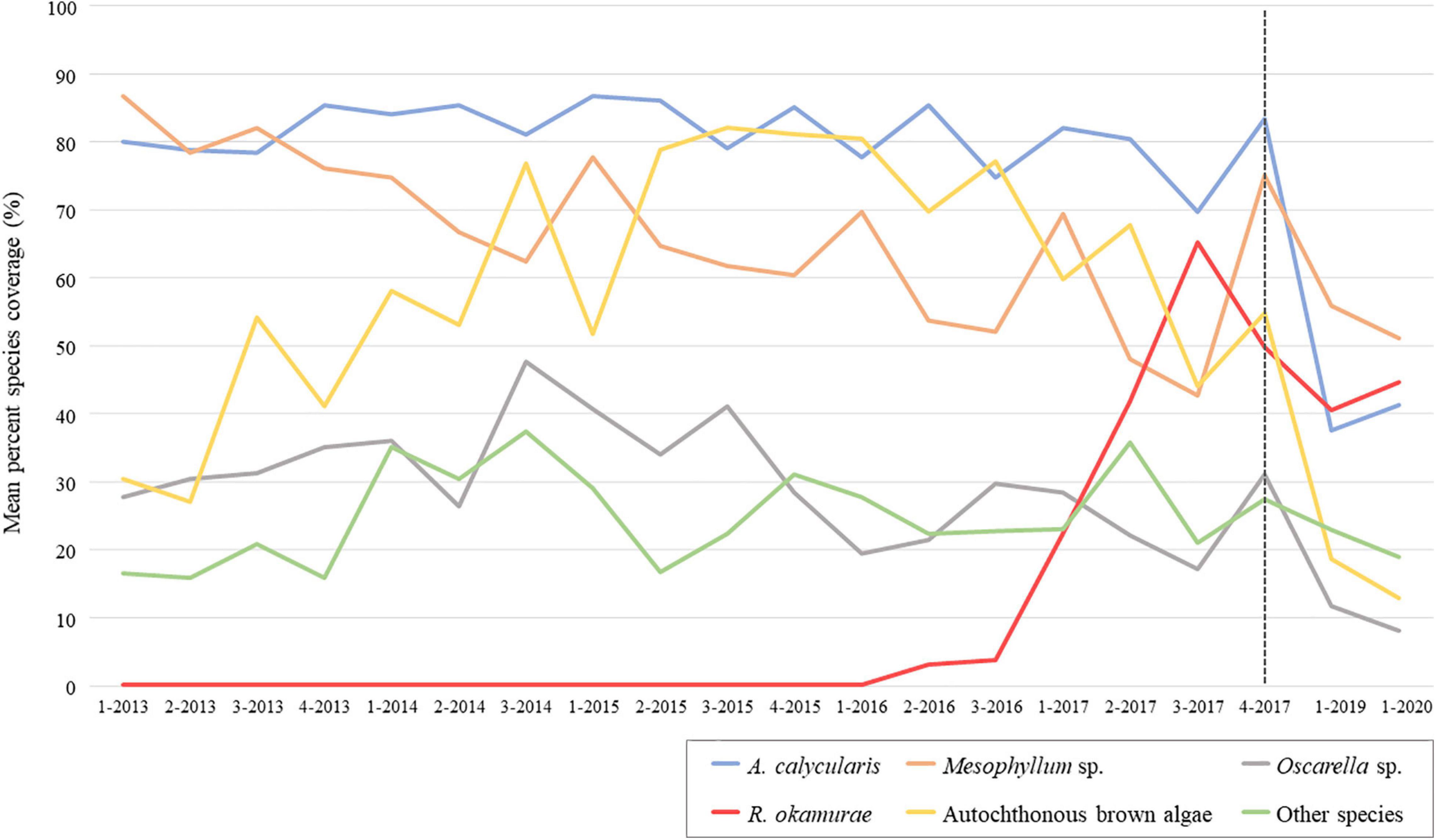
Figure 2. Temporal variation of benthic mean species percent coverage at the SBPQ station in Tarifa Island from years 2013 to 2020 (2018 data is missed). The dotted line locates, at the right side of the graph, the species fluctuations recorded after the last monitoring in 2017. Species with less than 10% coverage (Alcyonium sp., Aplidium sp., A. armata, Crambe sp., Ircinia sp., and Polycitor adriaticum) were grouped under “Other species.” 1 = winter; 2 = spring; 3 = summer; 4 = autumn.
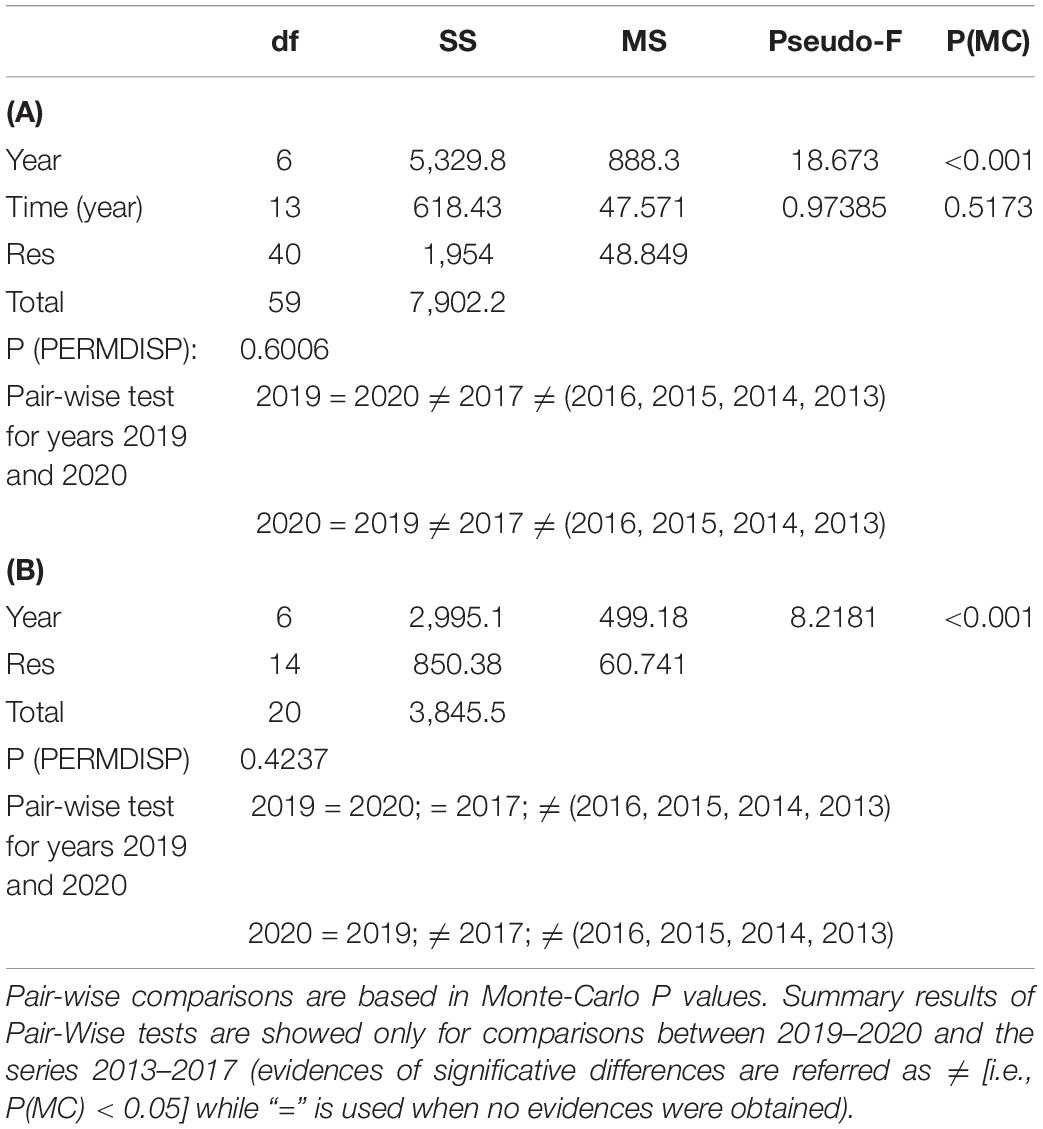
Table 1. PERMANOVA and PERMDISP (for the factor “Year”) results for SBPQ station coverage data (2013–2020) (A) considering intra-annual variability (i.e., all seasons) and (B) only winter seasons.
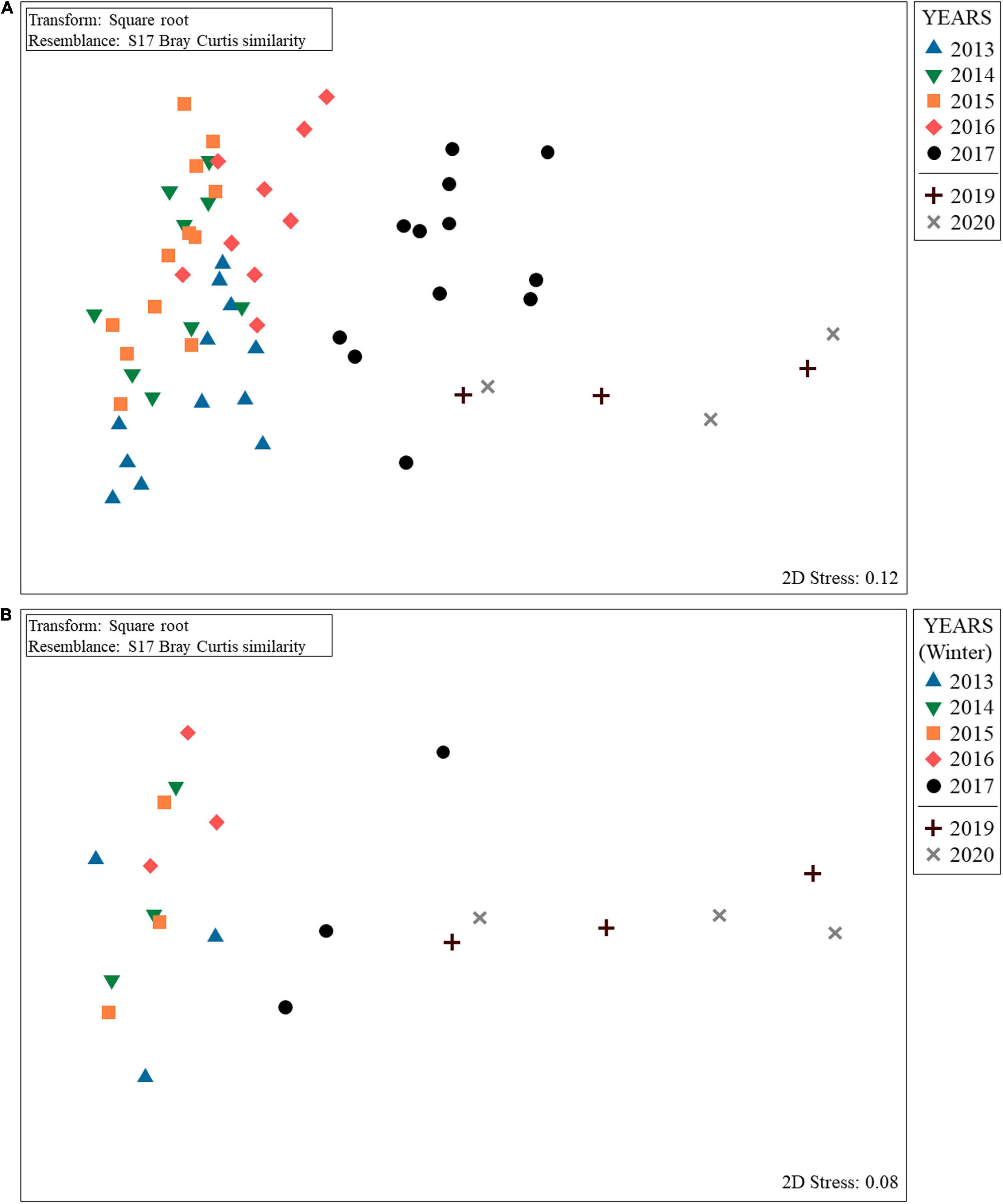
Figure 3. nmMDS ordination for Tarifa Island SBPQ station showing the evolution of sessile communities from 2013 to 2020 (2018 data is missed) taking into account (A) intra-annual variability (i.e., seasons within each year) until 2017 and (B) only winter seasons.
Results obtained only considering samplings from winters are shown in Supplementary Figure 1 and Table 1B. Comparisons revealed a hindrance of the exponential growth of R. okamurae from 2016 (Supplementary Figure 1). In 2019 and 2020, a slightly coverage enhancement was observed, but these values only contributed to dissimilarities against previous winters in a 16% (2019) and 17% (2020) (Supplementary Table 2). Overall, species coverage did not significantly differ between 2019 and 2020 (Table 1B). In fact, ordination analyses (nmMDS) revealed similar patterns to those obtained when including all the seasons form the period 2013–2017 (Figure 3B). Communities in 2020 significatively differed from winters before 2017 (included) and 2019 significantly differed from winters before 2016 (included). Despite the high coverages reached in 2019 and 2020 contributed to main differences against 2016 in both cases, when comparing with 2017, the loss of autochthonous brown algae and A. calycularis contributed up to 50% of dissimilarities in both cases.
Rugulopteryx okamurae Coverage Estimations on the Sublittoral Areas of the PNE
Coverage values registered at the bathymetric range of distribution of R. okamurae in 2018 on horizontal illuminated surfaces (García-Gómez et al., 2020b) (Figure 4A) allowed the estimation of the species coverage for the different bathymetric intervals (Figure 4B). R. okamurae percentage coverages were mapped for all the PNE coastline (Figure 5) and rocky surface areas and corresponding estimated percent coverages were obtained for the eastern littoral of the PNE (Figures 6, 7). The highest percentage coverages (85–96%) were obtained between 5 and 30 m depth. Over 10 m, these values coincided with the less extensive (565,469 m2 between 5 and 10 m) and the most extensive rocky surfaces registered along the PNE (more than 3,000,000 m2) (Figure 7). Thus, 96% of total coverage values were reached at the bathymetric interval of 10–20 m depth, estimated for a total rocky surface of 3,141,476 m2. Estimated percentages of R. okamurae cover remained high (85%) until – 30 m. The lowest percentage coverages (42 and 45%) occupied the deepest and the shallowest habitats (30–40 m and 0–5 m, respectively). No coverage data deeper than 40 m were obtained since no measurements were performed at such depth in studies performed in 2018 (see García-Gómez et al., 2020b).
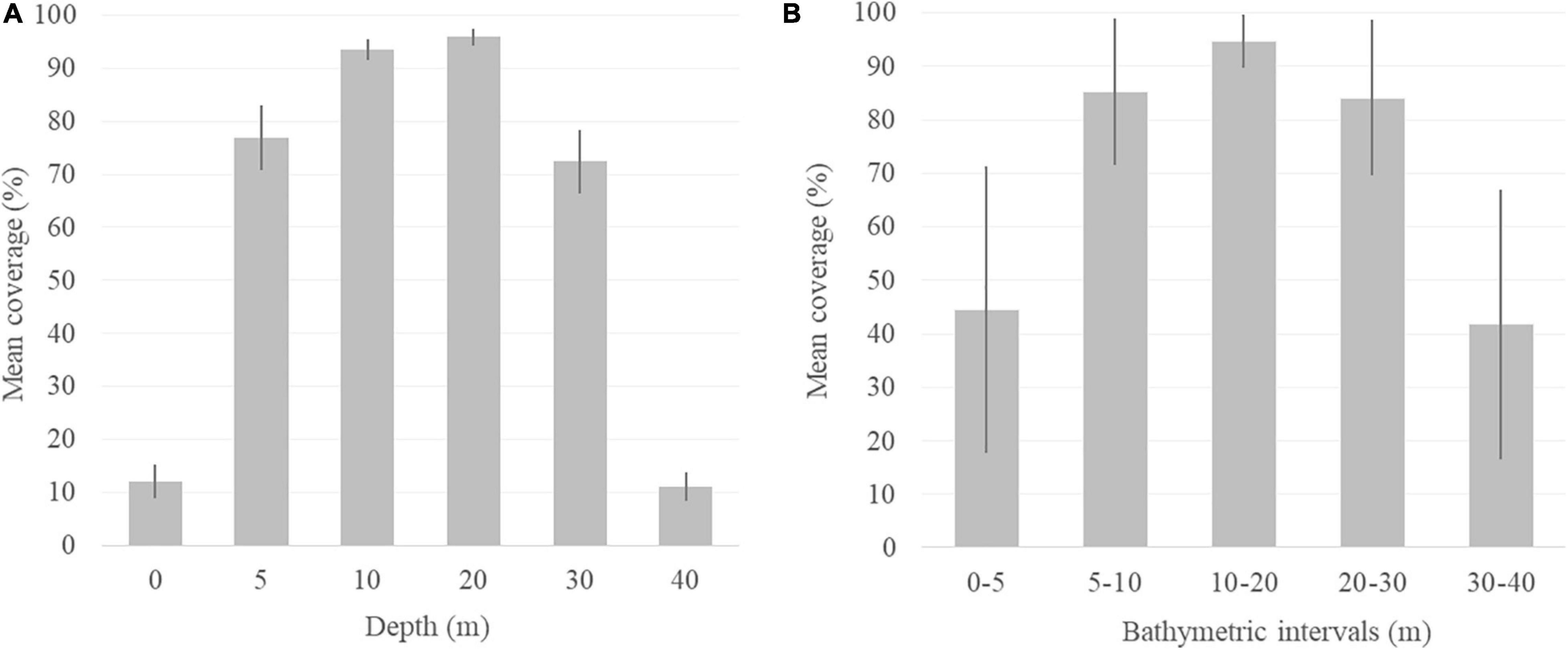
Figure 4. Mean percentage coverage of R. okamurae in the (A) bathymetric range obtained from the southern coasts of the Strait of Gibraltar (Ceuta) in summer 2018 (García-Gómez et al., 2020b) and (B) average mean percent coverage estimated for the bathymetric intervals of the sublittoral PNE rocky areas. Standard deviation error bars are included in both cases.
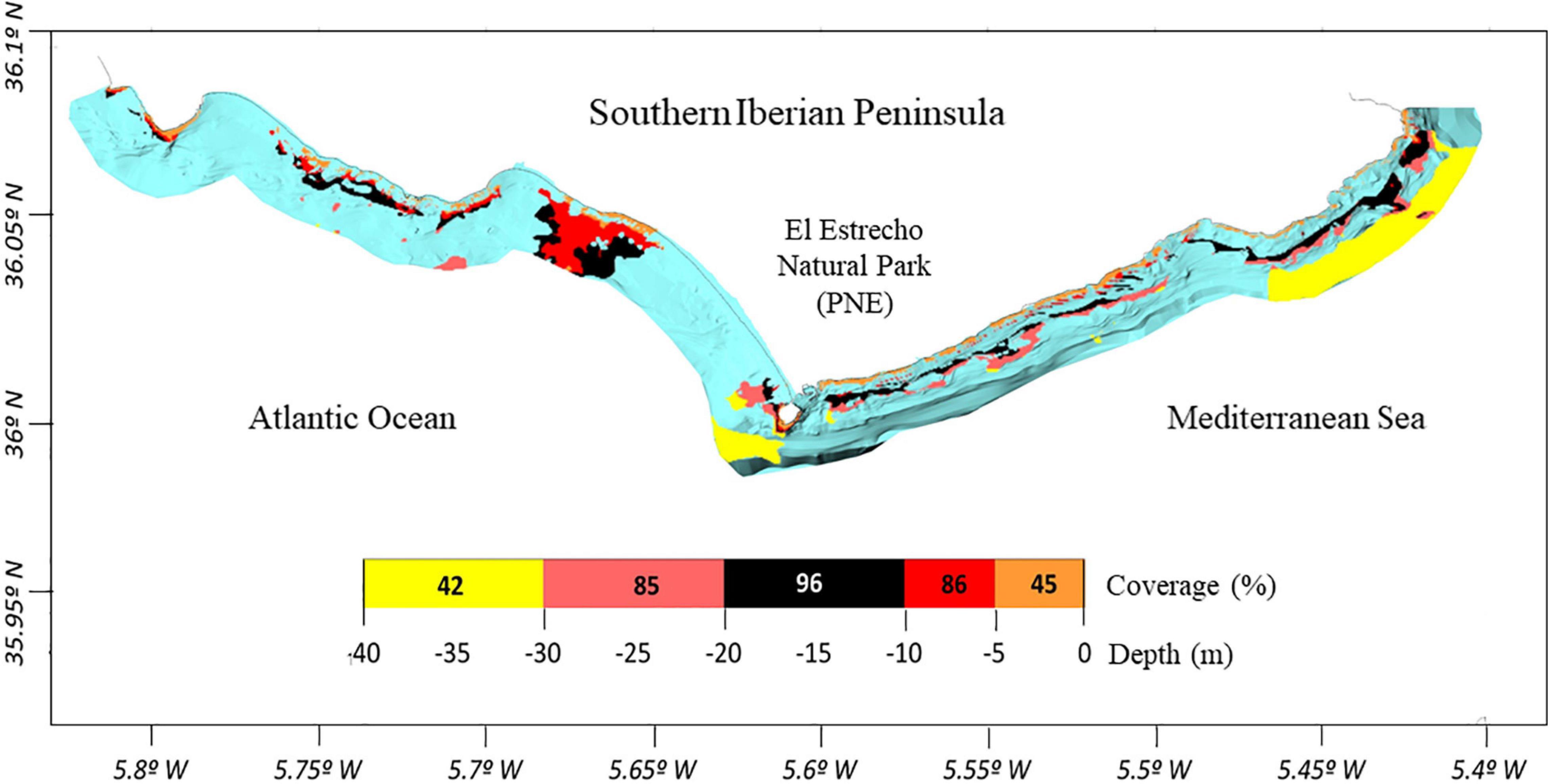
Figure 5. Distribution map of estimated R. okamurae coverage along the rocky coastline of the PNE. Color scale represents coverage percentages per bathymetric intervals. Blue colors are referred to the total area considered within the cartography (i.e., 1 mile from the coast).
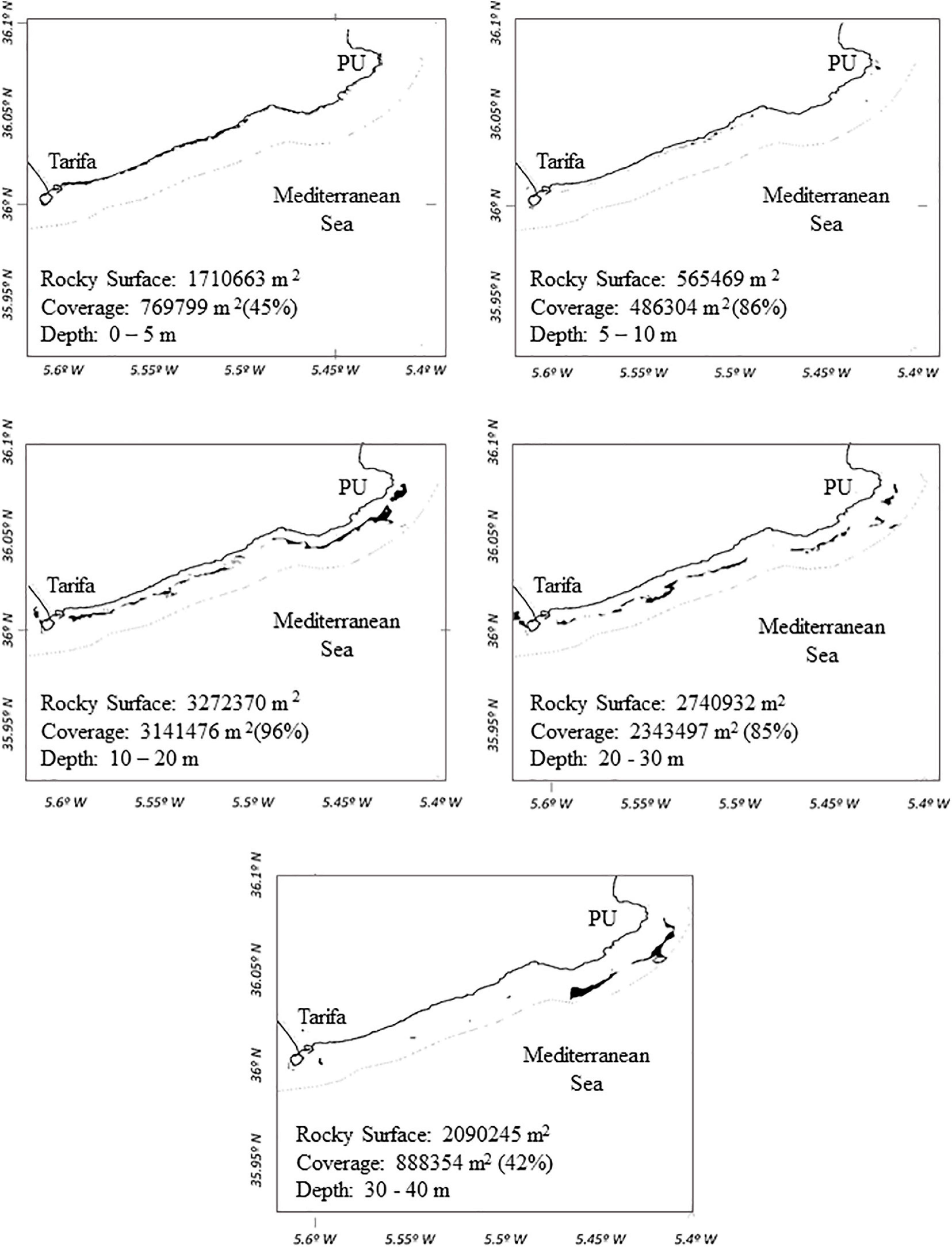
Figure 6. Rocky surface areas (m2) (black patches) mapped for the eastern sublittoral of the PNE (1 mile from the coast). Percentage coverages of R. okamurae at the different bathymetric intervals are represented (0–5, 5–10, 10–20, 20–30, and 30–40 m). PU = Punta Carnero.
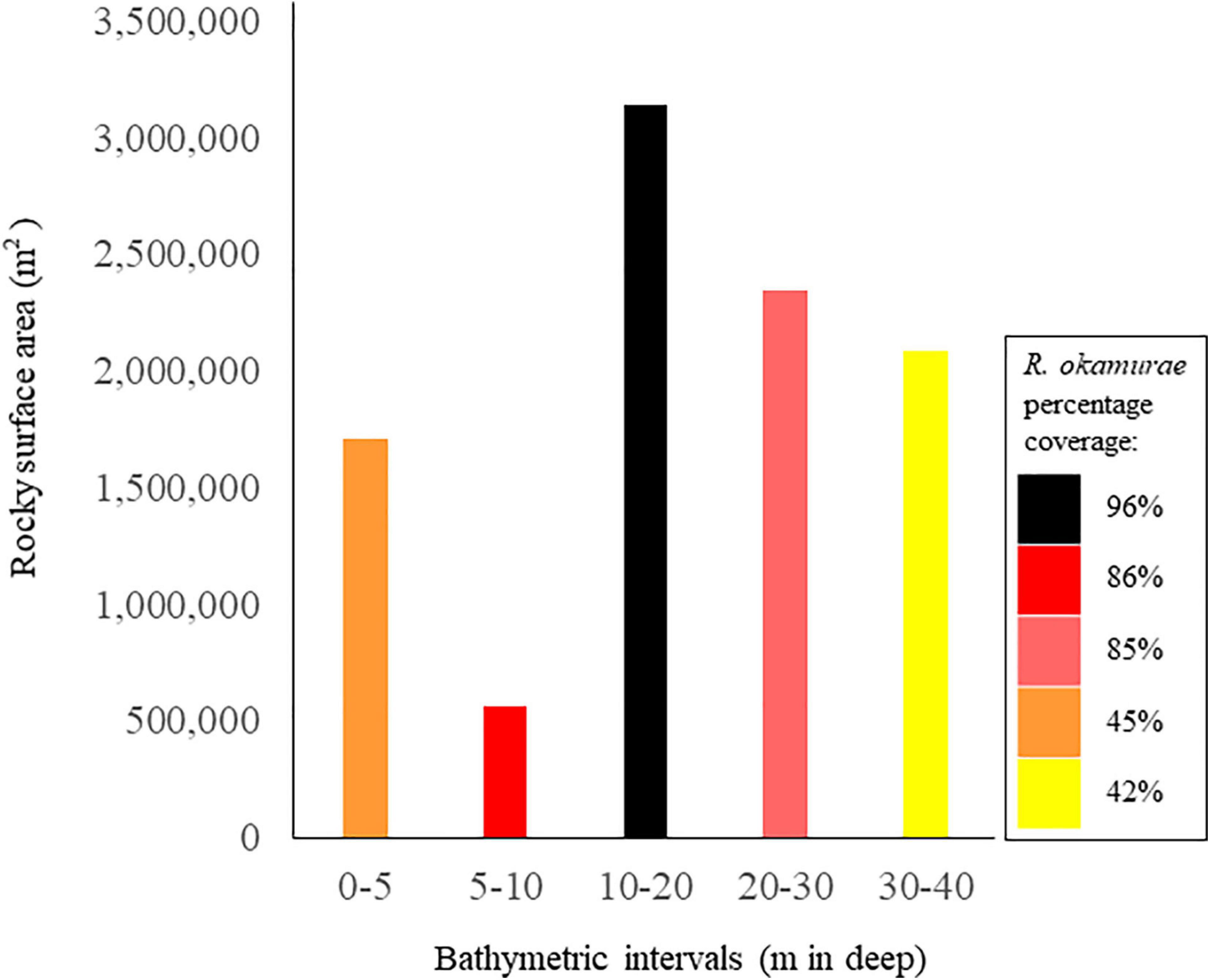
Figure 7. Rocky surface areas (m2) obtained for each bathymetrical interval (m) of the sublittoral coastline of the PNE. Colors legend represent the different percentage coverage values obtained for each bathymetrical interval mapped for the northern Strait of Gibraltar coastline (see Figure 5).
“Before-After” Underwater Seascape Approximation by Citizen Science Initiatives
The monitoring activities performed under the Citizen Science approach leaded to identify radical changes in the underwater seascape along the PNE littoral. Graphical data before and after the first establishment of R. okamurae revealed visual changes in the benthic community composition where the invasive species was represented as a dominant component by most of image data taken after 2015. The selection of visual data and concerning results at each sampling station and time is presented in the Table 2.
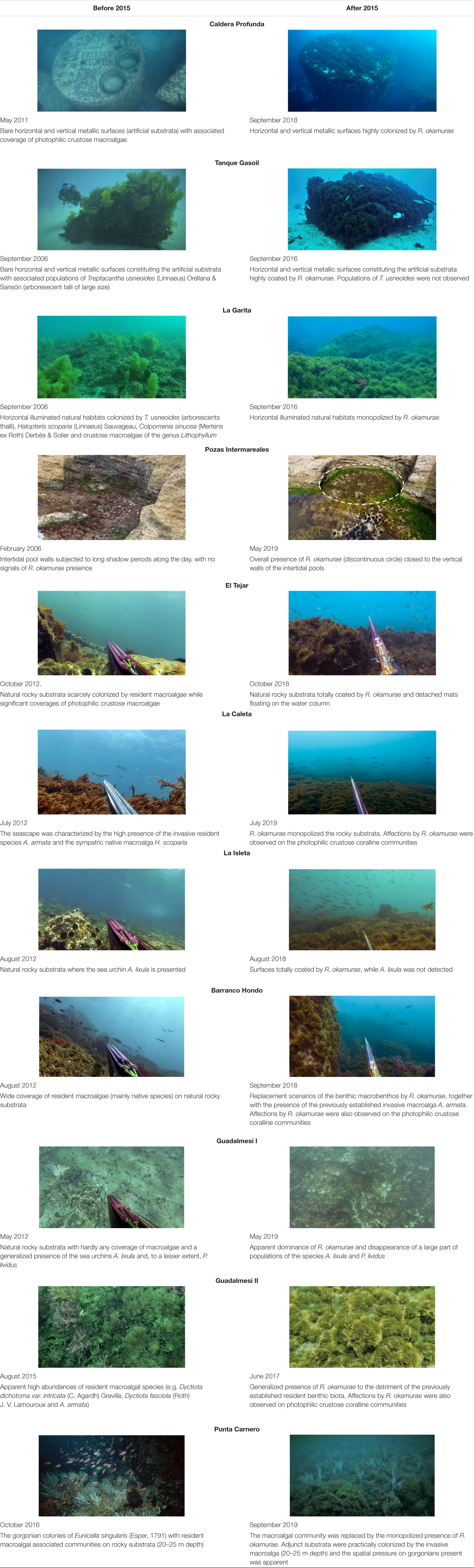
Table 2. Comparisons before and after R. okamurae first apparition in the Strait of Gibraltar coasts (2015) in locations surveyed in the study area.
Discussion
Results obtained by the different objectives prospected in this study evidenced the extreme effects of R. okamurae on the benthic ecosystems of the northern coasts of the Strait of Gibraltar, both by scientific (SBPQ alert method) and citizen (Citizen Science) monitoring methodologies. This, together with the high coverage levels estimated along the PNE coastline, illustrates the potential risk that the species represents to the benthic ecosystems in the Strait waters.
Updated Fluctuations of Rugulopteryx okamurae by Monitoring Sessile Bioindicators in Permanent Quadrats
The application of the SBPQ methodology has allowed the continuity of monitoring activities from 2017 to 2020 at the shady vertical surfaces of the pre-coralligenous habitats from the submarine sentinel station of Tarifa Island. Results showed that the benthic community structure differed between 2016 and 2017 due the high coverage levels of R. okamurae reached in that year. This pattern reflects the high spatial growth of the invasive species and, potentially, the ability to efficient using resources in the recipient habitats (Vaz-Pinto et al., 2014), which could results in the stronger and faster growth in detriment of the resident community, as is observed later in 2019 and 2020.
Until our study, few data have been offered about dominance-dynamics of R. okamurae after the huge coverages reached in 2017. Using the same methodology, Sempere-Valverde et al. (2020) registered coverage increments of the invasive species in most of monitored sites of coralligenous habitats after 2017. Our results showed a decrease in percent coverage, slightly increasing in 2020. Changes in shape and size of the macroalgal beds can be modified by a variety of abiotic factors as temperature, salinity or turbidity (Glasby et al., 2005a). In fact, according to bloom-bust dynamic theories, a drastic decline in the invasive populations can occur after an initial rapid increase of the abundances, without implying recoveries in the macroalgal resident communities [e.g., Caulerpa taxifolia (M.Vahl) C.Agardh (Glasby et al., 2005a, b)]. This has been further combined with other works that assume that impacts on the resident biota cannot be assessed only considering species gain and loss without including mechanisms involved (Chapin et al., 2000). According to our results, differences in the community structure between the last 2 years and 2017 were due to an effective loss of resident biota, instead of fluctuations in R. okamurae presence. This is in line with results from Sempere-Valverde et al. (2020), who also found changes in the community structure and the regression of bioindicator species. Thus, despite certain coverage stabilization could have been raised in the last years, impacts generated by R. okamurae remains high in the habitat studied, and therefore it can be assumed that no signs of decline in its invasive potential have been perceived. However, longer-scale monitorings are needed in order to totally stablish the strength of the bioinvasion, so monitoring efforts should be continued in the future to full characterize dynamics observed here.
Sessile Bioindicators in Permanent Quadrats focuses on monitoring information in substrata colonized by colonial species with long life cycle [i.e., pre-corallingenous (García-Gómez et al., 2020a) and coralligenous rocky bottoms (Sempere-Valverde et al., 2020)], so changes due to long established species can be easily perceived. In this study, the data available in winter revealed similar patterns while more progressive changes across years than when considering the whole intra-annual variability from 2013 to 2017. Thus, although the larger information obtained the higher characterization of the species dynamic can be ensured, the high similarity between ordination analyses performed in this study, reinforce the utility of the SBPQ methodology even with one sampling per year. Moreover, taking into account that at least in introduced habitats the species is also present in winter, cold seasons could be especially interesting because smaller coverages of the species may allow to reflect competitive processes missed in those periods where huge biomass led the substrata overgrowth.
Implications of Coverage Estimations of Rugulopteryx okamurae Within the PNE
Coverage results obtained in 2018 by García-Gómez et al. (2020b) on horizontal illuminated rocky surfaces, extrapolated here for a larger-scale approximation, suggest a high impact on sublittoral habitats due to the massive occupation of the generalized conquest of rocky areas by R. okamurae. In general, the coverage data obtained were very high, especially if compared with the biomass data observed in native habitats (Japan) by Agatsuma et al. (2005) (with similar north latitude to Spain, between 20° and 40°). Results obtained for coverage extrapolation coincide with those obtained in 2019–2020 by CAGPyDS (2020) for the bathymetric intervals between 0 and 20 m (80–100% R. okamurae coverage). However, the same results were not found for the intervals between 20 and 30 m (10–50%), so it could be interpreted a possible decline between 2018 and 2020 in the depth ranges where the illumination exposition is lower, so the competitive interactions with the resident macrobiota have not been able to be maintained after the initial stages of strong expansion after 2016.
Following the favorability models (Real et al., 2006), R. okamurae could proliferate within the ecological environments within the Atlantic coasts of Andalusia and Morocco, the Mediterranean Sea and the Black Sea (Muñoz et al., 2019). In fact, it has been confirmed the fast progress of the species toward the Mediterranean and Atlantic waters, and the recent arrival by wrack deposits at the nearby coasts of Granada and Almería (Altamirano et al., 2019; CAGPyDS, 2020; Figueroa et al., 2020). The nature of the ecosystem implications by R. okamurae establishment in the recipient ecosystems have been previously exposed in García-Gómez et al. (2018); Altamirano et al. (2019) and García-Gómez et al. (2020b). Results obtained in these experiences and the spread dynamic of the species alarm the rate of recorded invasions by marine macroalgae that have taken the place of target resident species becoming dominant in the last years (Boudouresque and Verlaque, 2002). This makes the geographic expansion along the rocky surfaces of the PNE littoral worrying and stress the detriment of the benthic biota already attributed to the establishment of R. okamurae in the area. In the southern coasts of the Strait, habitat changes derived from R. okamurae establishment have proved to have implications in endangered coralligenous species (Sempere-Valverde et al., 2020) and associated fauna to resident macroalgae (Navarro-Barranco et al., 2019), so it could be also expected that effects on sessile communities can be also translated to other ecosystem components. As Levine et al. (2004) propose, recognizing that biotic containment can occur through species interactions, it could be expected that ecosystem components interacting with R. okamurae could regulate the invasive populations dominance. In this sense, more efforts are needed studying interspecific interactions involving the invasive species and the incipient role of co-occurring specific resident taxa taking advantage of the spatial colonization [e.g., A. armata has been observed on dense populations of R. okamurae (unpublished data)].
Moreover, the high coverage estimations challenge the ecosystem and socio-economic services in the area. The PNE is an integral part of the MediterraneanIntercontinental Biosphere Reserve. It is frequented by tourists and scuba divers, while receives a high influence from both commercial and recreational traffic from areas as Algeciras Bay (Bermejo et al., 2014). Although impacts on socio-economic services have not being investigated, the excess of biomass shortly after R. okamurae establishment reported substantial impacts in the area, both in tourism and fisheries (e.g., the trap of Tarifa) (García-Gómez et al., 2018; Altamirano et al., 2019). If we consider the ability of the species to easily remain attached on nets and other artificial materials (García-Gómez et al., 2018), results obtained for estimations at 1 mile from the coastline enhance the attention on potential impacts in practices developed in the area. Moreover, taking into account that the species was inadvertently introduced via marine aquaculture within the European waters (Thau Lagoon) (Verlaque et al., 2009), preventive strategies for secondary spread pathways across the Mediterranean and the Atlantic waters must be strongly considered.
Facing with the arduous challenge to strategies applied in advanced invasion stages, mitigation efforts could be essential to protect similar areas not yet impacted but susceptible to be invaded. It is as this point that monitor/modeling techniques play a key role, since estimations based on cartographies can result useful to identificate areas not yet colonized. Moreover, existing precedents of successful actions against invasive marine macroalgae establishment and propagation have revealed that monitoring strategies are not effective unless applied in early stages of colonization, when the species has a limited spatial distribution (Anderson, 2007). In fact, according to Ojaveer et al. (2015), if the species has already managed to establish in large areas, eradication is unlikely. In this sense, it is worth highlighting the case of C. taxifolia as a precedent of marine bioinvasion which invasive process resulted impossible to be stopped by control efforts (Ruesink and Collado-Vides, 2006), becoming the most widespread invasive macroalga in the Mediterranean waters, occupying 20,000 Ha of sublittoral areas (Anderson, 2007).
Before-After Seascape Scenarios Reflecting Extreme Changes Due Rugulopteryx okamurae Invasiveness
The comparative analyses of images can make it possible to obtain inferences about the behavior of the biota and the ecological connectivity, since the underwater seascape can have a great influence (Grober-Dunsmore et al., 2009). In this regard, the Citizen Science initiative developed in this study allowed a large spatial and temporal dataset to visualize impacts related with R. okamurae establishment, providing an accurate underwater seascape of the bioinvasion consequences. The seascape sampling provided an adequate approach for monitoring developments and it was useful for describing and categorizing some benthic communities that interact with the brown macroalga in the PNE littoral. Comparisons before and after R. okamurae establishment revealed an overall substitution of the benthic seascapes by the invasive species, but also effectively evidenced negative impacts on particular resident species. For example, the disappearance of sea urchin species [Arbacia lixula (Linnaeus, 1758) and Paracentrotus lividus (Lamarck, 1816)] from different shallow rocky bottoms could be inferred by comparing pairs of images examined. Indeed, in most of the cases, signs of total colonization were observed only 3 years after its establishment in the Strait of Gibraltar and thus, impacts by the generalized substitution of the resident macroalgae at illuminated and shaded habitats (i.e., native and invasive species already established in the area, as those from the genus Asparagopsis) were particularly visible.
In situ observational data also increased the quantity of R. okamurae observations available for ecological researches (as it has previously been pointed out by Crall et al., 2015). Image data from 2016 at Tarifa Island revealed that R. okamurae monopolized more than 80% of highly illuminated horizontal surfaces at hard bottoms between 5 and 10 m depth, which contrast with results obtained at partially shaded vertical substrata sampled in the SBPQ station in the same year, where <10% mean coverage was estimated by photoquadrat analyses. In the latter habitats, R. okamurae coverage increased later, in 2017 (<60% coverage) (García-Gómez et al., 2020b), and thus revealing habitat-dependent patterns not previously perceived.
Conclusion
The present study determined that impacts derived from R. okamurae establishment remain high in the rocky habitats studied of the PNE. Monitoring studies on the SBPQ station of Tarifa Island revealed a high spatial establishment of the invasive species since its first detection in 2015 and an efficient loss in the sessile resident biota in the latter years, even in periods of minimum growth. In view of the ecosystem implications, coverage values estimated for rocky habitats (over 85% between 10 and 30 m depth) claim monitoring efforts focused in threatened habitats not yet colonized a remain step ahead of the drastic scenarios observed. In this regard, Citizen Science collaborations for the detection, evaluation and monitoring of impacts from R. okamurae resulted a useful and promising tool for further studies. We consider that these monitoring initiatives would be even more successful if combined with periodic monitoring methodologies under specific designs. Thus, monitoring stations located in areas coinciding with those where contributors act (e.g., SBPQ station in Tarifa Island), could allow citizen collaboration through the applicability of non-invasive tools for image analysis procedures easy to understand and apply. Only if these tools are promoted within local networks (i.e., high anthropic pressure areas), they could help to early detect and monitor (e.g., “before-after” approximations) local (e.g., urban discharges, oil slicks) or global environmental impacts (e.g., global change), which would facilitate to act in time in the face of bioinvasion schemes.
Data Availability Statement
The original contributions presented in the study are included in the article/Supplementary Material, further inquiries can be directed to the corresponding author.
Author Contributions
JG-G involved in investigation, writing – original draft, analysis data, supervision, validation, funding acquisition, and project administration. MF involved in investigation, writing – original draft, analysis data, and data curation. LO-P involved in investigation, special collaboration writing – original draft, and data curation. JRDdR involved in investigation and collaboration in cartography of coverage. ID-A involved in investigation, analysis data and special collaboration writing – original draft. MC, JQ, and SM collaboration underwater images “before-after” impact (Citizen Science). CM involved in investigation and supervision; JG-G, MF, LO-P, ID-A, and CM contributed substantially to revisions of manuscript. All authors contributed to the article and approved the submitted version.
Funding
This study was funded by the CEPSA Foundation and Red Eléctrica de España (REE). Additional financial support was provided by the Organization of Fish Producers of Almadrabas (OPP-51), ENDESA, ACERINOX, and Diputación Provincial de Cádiz. This project has also benefited from scientific equipment and infrastructures financed by the AP of Seville and the Aquarium of Seville and by the VI Plan Propio Universidad de Sevilla via a post-doctoral grant (ID-A).
Conflict of Interest
JRDdR was employed by the company Esgemar S.A.
The remaining authors declare that the research was conducted in the absence of any commercial or financial relationships that could be construed as a potential conflict of interest.
Acknowledgments
We are deeply grateful to Manuel Martínez Chacón, Juanjo Quintero, and Salvador Magariño for their selfless collaboration “Citizen Science” in the search, obtaining underwater images, and selection of graphic information, especially from documentary archives prior to 2015. We are also grateful to Red Eléctrica de España (REE) and CEPSA Foundation for mean financial support to scientific research related to the environmental impact of the invasive species Rugulopteryx okamurae on the marine autochthonous biodiversity of the Bay of Algeciras, Strait of Gibraltar, and nearby areas, and to Organization of Fish Producers of Almadrabas (OPP-51), ENDESA, ACERINOX, and Diputación Provincial de Cádiz for additional financial support, related with the presence of invasive algae in their artisanal fishing or industrial port infrastructures. We thank the Port Authority of Seville (APS) and Seville Aquarium for scientific infrastructure acquired in project number 1889 (FIUS), related to the estuary of the Guadalquivir River implied in environmental monitoring campaigns and controls in the areas near the mouth of the mentioned river. We thank ESGEMAR, S.A., for providing cartographic support and map delimitation and CIES (Algeciras) and Campo de Gibraltar (La Linea) diving clubs. We also thank Isabel Cárdenas, Curro Sánchez, Rocío Espada, Juan Miguel Recio, Antonio Vegara, José Fosela, and Candela Sánchez for their continued collaboration. This paper has been benefited in the city of La Línea by the logistical support of Puerto La Alcaidesa, the Yacht Club (RCNLL), and the Maritime Club, as well as by the town halls of this city and Tarifa.
Supplementary Material
The Supplementary Material for this article can be found online at: https://www.frontiersin.org/articles/10.3389/fevo.2021.639161/full#supplementary-material
References
Abdulla, A., and Linden, O. (2008). Maritime Traffic Effects on Biodiversity in the Mediterranean Sea: Review of Impacts, Priority Areas and Mitigation Measures. Malaga: IUCN Centre for Mediterranean Cooperation, 184.
Adama, D., Mohammed, A., Maroua, H., Mohammed, E., Essalmani, H., and Mouna, D. (2021). Distribution and biomass assessment of macroalgae from moroccan strait of gibraltar. Acta Ecol. Sin. 1872–2032. doi: 10.1016/j.chnaes.2021.01.004 [Epub ahead of print].
Agatsuma, Y., Kuwahara, Y., and Taniguchi, K. (2005). Life cycle of Dilophus okamurae (Phaeophyceae) and its associated invertebrate fauna in Onagawa Bay. Japan. Fish. Sci. 71, 1107–1114. doi: 10.1111/j.1444-2906.2005.01070.x
Altamirano, M., Andreakis, N., Souza-Egips, V., Zanolla1, M., and De la Rosa, J. (2014). First record of Caulerpa cylindracea (Caulerpaceae, Chlorophyta) in Andalusia (Southern Spain). Anales Jard. Bot. Madrid 71:e007. doi: 10.3989/ajbm
Altamirano, M., Muñoz, A. R., De la Rosa, J., Barrajón-Minguez, A., Barrajón-Domenech, A., Moreno-Robledo, C., et al. (2008). The invasive species Asparagopsis taxiformis (Bonnemaisoniales, Rhodophyta) on Andalusian coasts (Southern Spain): reproductive stages, new records and invaded communities. Acta Bot. Malac 33, 5–15. doi: 10.24310/abm.v33i0.6963
Altamirano, M., De la Rosa, J. D., Carmona, R., Zanolla, M., and Muñoz, A. R. (2019). Macroalgas invasoras en las costas andaluzas. Algas 55e.
Altamirano-Jeschke, M., De la Rosa, J., and Martínez, J. (2016). Arribazones de la especie exótica Rugulopteryx okamurae (E.Y. Dawson) I.K. Hwang, W.J. Lee & H.S. Kim (Dictyotales, Ochrophyta) en el Estrecho de Gibraltar: primera cita para el Atlántico y España. Algas 52:20.
Altamirano-Jeschke, M., De la Rosa Álamos, J., Martínez, F. J. G., and Muñoz, A. R. G. (2017). Prolifera en el Estrecho un alga nunca citada en nuestro litoral de origen asiático “Rugulopteryx okamurae” ocupa ya una gran extensión. Quercus 364, 32–33.
Anderson, L. W. J. (2007). Control of invasive seaweeds. Bot. Mar. 50, 418–437. doi: 10.1515/BOT.2007.045
Anderson, M. J. (2006). Distance-based tests for homogeneity of multivariate dispersions. Biometrics 62, 245–253. doi: 10.1111/j.1541-0420.2005.00
Anderson, M. J., Gorley, R. N., and Clarke, K. R. (2008). PERMANOVA for PRIMER: Guide to Software and Statistical Methods. Plymouth: PRIMER-E, 214.
Ballesteros, E., Cebrian, E., and Alcoverro, T. (2007). Mortality of shoots of Posidonia oceanica following meadow invasion by the red alga Lophocladia lallemandii. Bot. Mar. 50, 8–13. doi: 10.1515/BOT.2007.002
Bedini, R., Bedini, M., Bonechi, L., and Piazzi, L. (2015). Effects of non-native turf-forming Rhodophyta on mobile macro-invertebrate assemblages in the north-western. Mar. Biol. Res. 4, 430–437. doi: 10.1080/17451000.2014.952310
Bermejo, R., Mangialajo, L., Vergara, J. J., and Hernández, I. (2014). Comparison of two indices based on macrophyte assemblages to assess the ecological status of coastal waters in the transition between the Atlantic and Mediterranean eco-regions. J. Appl. Phycol. 26, 1899–1909. doi: 10.1007/s10811-013-0226-x
Bianchi, C. N., Boudouresque, C. F., Francour, P., Morri, C., Par-Ravicini, V., Templado, J., et al. (2013). The changing biogeography of the Mediterranean Sea: from the old frontiers to the new gradients. Boll. Mus. Ist. Biol. Univ. Genova 75, 81–84.
Boudouresque, C. F., and Verlaque, M. (2002). Biological pollution in the Mediterranean Sea: invasive versus introduced macrophytes. Mar. Poll. Bull. 44, 32–38. doi: 10.1016/S0025-326X(01)00150-3
Cabatain, P. C., Licuanan, W. Y., and Gomez, E. D. (2006). Comparison between videographic and photographic methods assessing coral reef benthic communities. Sci. Diliman. 19, 7–13.
CAGPyDS (2020). Resultados de los Trabajos con Rugulopteryx Okamurae en la ZEC y PN del Estrecho en el Marco del Convenio Suscrito Entre Amaya, Agapa, y Ocean Cleaner Technology S.L. Consejería de Agricultura, Ganadería, Pesca y Desarrollo Sostenible de la Junta de Andalucía. Seville: Agencia de Medio Ambiente y Agua de Andalucía.
Carballo, J. L., Naranjo, S., and García-Gómez, J. C. (1997). Where does the Mediterranean Sea begin? Zoogeographical affinities of the littoral sponges of the Strait of Gibraltar. J. Biogeogr. 24, 223–232. doi: 10.1046/j.1365-2699.1997.00082.x
Castello, J., and Carballo, J. L. (2001). Isopod fauna, excluding Epicaridea, from the Strait of Gibraltar and nearby areas (Southern Iberian Peninsula). Sci. Mar. 65, 221–241. doi: 10.3989/scimar
Ceraulo, M., Papale, E., Caruso, F., Filiciotto, F., Grammauta, R., Parisi, I., et al. (2018). Acoustic comparison of a patchy Mediterranean shallow water seascape: Posidonia oceanica meadow and sandy bottom habitats. Ecol. Indic. 85, 1030–1043. doi: 10.1016/j.ecolind.2017.08.066
Chapin, F. S., Zavaleta, E. S., Eviner, V. T., Naylor, R. L., Vitousek, P. M., Reynolds, H. L., et al. (2000). Consequences of changing biodiversity. Nature 405, 234–242. doi: 10.1038/35012241
Cheminée, A., Merigot, B., Vanderklift, M. A., and Francour, P. (2016). Does habitat complexity influence fish recruitment? Mediterr. Mar. Sci. 17, 39–46. doi: 10.12681/mms.1231
Clarke, K. R., and Gorley, R. N. (2006). Plymouth: Primer-E. PRIMER v6: User Manual/Tutorial. Plymouth: PRIMER-E.
CMAyOT Consejería de Medio Ambiente y Ordenacion del Teritorio (2008). Memoria Técnica Descriptiva Referente a las Unidades de las Comunidades Biológicas Marinas del Futuro Espacio Protegido “Frente Litoral Algeciras -Tarifa”. Criterios Tecnicos para la Ordenación y Gestión de los Recursos Naturales del frente Litoral Algeciras Tarifa. Informe Cartografía Temática. Cadiz: Universidad de Sevilla-Universidad de Cádiz.
Conde, F., Flores-Moya, A., Soto, J., Altamirano, M., and Sánchez, A. (1996). Check-list of Andalusia (S Spain) seaweeds. III. Rhodophyceae. Acta Bot. Malacitana 21, 7–33. doi: 10.24310/abm.v21i0.8664
Conner, M. M., Saunders, W. C., Bouwes, N., and Jordan, C. (2015). Evaluating impacts using a BACI design, ratios, and a Bayesian approach with a focus on restoration. Environ. Monit. Assess. 188, 1–14. doi: 10.1007/s10661-016-5526-6
Conradi, M., López-González, P. J., Cervera, J. L., and García-Gómez, J. C. (2000). Seasonality and spatial distribution of peracarids associated with the bryozoan Bugula neritina in Algeciras. Bay. J. Crust. Biol. 20, 334–349. doi: 10.1651/0278-0372(2000)020[0334:sasdop]2.0.co;2
Crall, A. W., Jarnevich, C. S., Young, N. E., Panke, B. J., Renz, M., and Stohlgren, T. J. (2015). Citizen science contributes to our knowledge of invasive plant species distributions. Biol. Invasions. 17, 2415–2427. doi: 10.1007/s10530-015-0885-4
Donázar-Aramendía, I., Sánchez-Moyano, J. E., García-Asencio, I., Miró, J. M., Megina, C., and García-Gómez, J. C. (2018). Maintenance dredging impacts on a highly stressed estuary (Guadalquivir estuary): a BACI approach through oligohaline and polyhaline habitats. Mar. Environ. Res. 140, 455–467. doi: 10.1016/j.marenvres.2018.07.012
Donázar-Aramendía, I., Sánchez-Moyano, J. E., García-Asencio, I., Miró, J. M., Megina, C., and García-Gómez, J. C. (2020). Environmental consequences of dredged-material disposal in a recurrent marine dumping area near to Guadalquivir estuary. Spain. Mar. Pollut. Bull. 161:111736. doi: 10.1016/j.marpolbul.2020.111736
Earp, H. S., and Liconti, A. (2020). “Science for the future: the use of citizen science in marine research and conservation,” in YOUMARES 9 - The Oceans: Our Research, Our Future, eds S. Jungblut, V. Liebich, and M. Bode-Dalby (Cham: Springer), doi: 10.1007/978-3-030-20389-4_1
El Aamri, F., Idhalla, M., and Tamsouri, M. N. (2018). Occurrence of the invasive brown seaweed Rugulopteryx okamurae (E.Y.Dawson) I.K.Hwang, W.J. Lee & H.S. Kim (Dictyotales, Phaeophyta) in Morocco (Mediterranean Sea). MedFAR 1, 92–96.
Endrina, N., Rasero, J. C., and Konovessis, D. (2018). Risk analysis for RoPax vessels: a case of study for the Strait of Gibraltar. Ocean Eng. 151, 141–151. doi: 10.1016/j.oceaneng.2018.01.038
Figueroa, F. L., Vega, J., Gómez-Valderrama, M., Korbee, N., Mercado, J. M., Bañares, E., et al. (2020). Invasión de la especie exótica Rugulopteryx okamurae en Andalucía I: estudios preliminares de la actividad fotosintética. Algas 56:35.
Flores-Moya, A., Soto, J., Sánchez, A., Altamirano, M., Reyes, G., and Conde, F. (1995a). Check-list of Andalusia (S Spain) seaweeds. I. Phaeophyceae. Acta Bot Malacitana 20, 5–18.
Flores-Moya, A., Soto, J., Sánchez, A., Altamirano, M., Reyes, G., and Conde, F. (1995b). Check-list of Andalusia (S Spain) seaweeds. II. Chlorophyceae. Acta Bot Malacitana 20, 19–26.
Gallardo, D., Oliva, F., and Ballesteros, M. (2021). Marine invertebrate epibionts on photophilic seaweeds: importance of algal architecture. Mar. Biodiv. 51:116. doi: 10.1007/s12526-020-01151-y
García-Gómez, J. C. (2002). Paradigmas de una Fauna Insólita. Los Moluscos Opistobranquios del Estrecho de Gibraltar. Thesis, Instituto de Estudios Campogibraltareños, Cádiz, 397.
García-Gómez, J. C. (2015). A Guide on Environmental Monitoring of Rocky Seabeds in Mediterranean Marine Protected Areas and Surrounding Zones. Tunis: RAC/SPA-MedMPAnet Project. 1-491. Available online at: http://biblioteca.oapn.es/cgi-bin/koha/opac-detail.pl?biblionumber=57497 (accessed December 6, 2020).
García-Gómez, J. C., Corzo, J. R., López-Fe, C. M., Sánchez-Moyano, J. E., Corzo, M., Rey, J., et al. (2003). Metodología cartográfica submarina orientada a la gestión y conservación del medio litoral: mapa de las comunidades bentónicas del frente litoral norte del estrecho de Gibraltar. Bol. Inst. Esp. Oceanogr. 19, 149–163.
García-Gómez, J. C., González, A. R., Maestre, M. J., and Espinosa, F. (2020a). Detect coastal disturbances and climate change effects in coralligenous community through sentinel stations. PLoS One 15:e0231641. doi: 10.1371/journal
García-Gómez, J. C., Sempere-Valverde, J., González, A. R., Martínez-Chacón, M., Olaya-Ponzone, L., Sánchez-Moyano, E., et al. (2020b). From exotic to invasive in record time: the extreme impact of Rugulopteryx okamurae (Dictyotales, Ochrophyta) in the strait of Gibraltar. Sci. Total Environ. 704:135408. doi: 10.1016/j.scitotenv.2019.135408
García-Gómez, J. C., Sempere-Valverde, J., Ostalé-Valriberas, E., Martínez, M., Olaya-Ponzone, L., González, A. R., et al. (2018). Rugulopteryx okamurae (E.Y. Dawson) I.K. Hwang, W.J. Lee & H.S. Kim (Dictyotales, Ochrophyta), alga exótica “explosiva” en el estrecho de Gibraltar. Observaciones preliminares de su distribución e impacto. Almoraima 48, 97–113.
García-Raso, J. E. (1993). New record of other African species of Crustacea Decapoda, Cycloes cristata (Brulle), from European and Mediterranean waters. Bios 1, 215–221.
García-Raso, J. E., Marina, P., and Baro, J. (2011). Bythocaris cosmetops (Decapoda: Caridea: Hippolytidae) in the western Mediterranean Sea. Mar. Biodivers. Rec. 4:e52. doi: 10.1017/S1755267211000467
Glasby, T. M., Creese, R. G., and Gibson, P. T. (2005a). Experimental use of salt to control the invasive marine alga Caulerpa taxifolia in New South Wales, Australia. Biol. Conserv. 122, 573–580. doi: 10.1016/j.biocon.2004.09.012
Glasby, T. M., Gibson, P. T., and Kay, S. (2005b). Tolerance of the invasive marine alga Caulerpa taxifolia to burial by sediment. Aquat. Bot. 82, 71–81. doi: 10.1016/j.aquabot.2005.02.004
Gobert, S., Chéry, A., Volpon, A., Pelaprat, C., and Lejeune, P. (2014). “The seascape as an indicator of environmental interest and quality of the mediterranean benthos: the in situ development of a description index: the LIMA,” in Underwater Seascapes: From Geographical to Ecological Perspectives, eds O. Musard, L. Le Dû-Blayo, P. Francour, J.-P. Beurier, E. Feunteun, and L. Talassinos (Berlin: Springer International Publishing), 277–291. doi: 10.1007/978-3-319-03440-9_18
Gofas, S., Moreno, D., and Salas, C. (2011). Moluscos Marinos de Andalucía. Servicio de Publicaciones e Intercambio Científico. Málaga: Universidad de Málaga.
Grober-Dunsmore, R., Pittman, S. J., Caldow, C., Kendall, M. S., and Frazer, T. K. (2009). “A landscape ecology approach for the study of ecological connectivity across tropical marine seascapes,” in Ecological Connectivity among Tropical Coastal Ecosystems, ed. I. Nagelkerken (Netherlands: Springer), 493–530. doi: 10.1007/978-90-481-2406-0_14
Guerra-García, J. M., Cabezas, P., Baeza-Rojano, E., Espinosa, F., and García-Gómez, J. C. (2009). Is the north side of the Strait of Gibraltar more diverse than the south side? A case study using the intertidal peracarids (Crustacea: Malacostraca) associated to the seaweed Corallina elongate. J. Mar. Biolog. 89, 387–397. doi: 10.1017/S0025315409002938
Guerra-García, J. M., Ros, M., Izquierdo, D., and Soler-Hurtado, M. M. (2012). The invasive Asparagopsis armata versus the native Corallina elongata: differences in associated peracarid assemblages J. Exp. Mar. Biol. Ecol. 41, 121–128. doi: 10.1016/j.jembe.2012.02.018
Hwang, I. K., Wook, J. L., Kim, H. S., and De Clerck, O. (2009). Taxonomic reappraisal of Dilophus okamurae (Dictyotales, Phaeophyta) from the western Pacific Ocean. Phycologia 48, 1–12. doi: 10.2216/07-68.1
Johnson, B. A., Mader, A. D., Dasgupta, R., and Kumar, P. (2020). Citizen science and invasive alien species: an analysis of citizen science initiatives using information and communications technology (ICT) to collect invasive alien species observations. Glob. Ecol. Conserv. 21:e00812. doi: 10.1016/j.gecco.2019.e00812
Klein, J., and Verlaque, M. (2008). The Caulerpa racemosa invasion: a critical review. Mar. Poll. Bull. 56, 205–225. doi: 10.1016/j.marpolbul.2007.09.043
Levine, J. M., Adler, P. B., and Yelenik, S. G. (2004). A meta-analysis of biotic resistance to exotic plant invasions. Ecol. Lett. 7, 975–989. doi: 10.1111/j.1461-0248.2004.00657.x
Lodge, D. M., Williams, S., MacIsaac, H. J., Hayes, K. R., Leung, B., Reichard, S., et al. (2006). Biological invasions: recommendations for U.S. policy and management. Ecol. Appl. 16, 2035–2054.
Mannino, A. M., Balistreri, P., and Deidun, A. (2017). “The marine biodiversity of the mediterranean sea in a changing climate: the impact of biological invasions,” in Mediterranean Identities - Environment, Society, Culture, ed. B. Fuerst-Bjelis (London: INTECH), 101–127.
MITECO Ministerio para la Transición Ecológica (2012). Ecocartografia del litoral Español. Available online at: https://www.miteco.gob.es/es/costas/temas/proteccion-costa/ecocartografias/ecocartografia-cadiz.aspx (accessed November 1, 2020).
Montefalcone, M., Chiantore, M., Lanzone, A., Morri, C., Albertelli, G., and Bianchi, C. N. (2008). BACI design reveals the decline of the seagrass Posidonia oceanica induced by anchoring. Mar. Pollut. Bull. 56, 1637–1645. doi: 10.1016/j.marpolbul.2008.05.013
Morales, C. (2007). Caracterización de la Calidad de Sedimentos Afectados por Vertidos de Petróleo: Comparación Entre Casos de Vertidos Accidentales (impacto agudo) Frente a Derrames Continuos (impacto crónico). Thesis, Universidad de Cádiz, Cadiz.
Moschella, P. S., Abbiati, M., Åberg, P., Airoldi, L., Anderson, J. M., Bacchiocchi, F., et al. (2005). Low-crested coastal defence structures as artificial habitats for marine life: using ecological criteria in design. Coast. Eng. 52, 1053–1071. doi: 10.1016/j.coastaleng.2005.09.014
Muñoz, A. R., Martín-Taboada, A., De la Rosa, J., Carmona, R., Zanolla, M., and Altamirano, M. (2019). La modelación de la distribución de especies como herramienta en la gestión de invasiones biológicas en el medio marino: el caso de Rugulopteryx okamurae (Dictyotaceae, Ochrophyta) en el Mediterráneo. Algas Sef 55e, 37–40.
Nachite, D., Del Estal Domínguez, N., El M’rini, A., and Anfuso, G. (2020).). Environmental Sensitivity Index Maps in a high maritime transit area: the Moroccan coast of the Gibraltar Strait study case. J. Afr. Earth Sci. 163:103750. doi: 10.1016/j.jafrearsci.2020.103750
Navarro-Barranco, C., Florido, M., Ros, M., González-Romero, P., and Guerra-García, J. M. (2018). Impoverished mobile epifaunal assemblages associated with the invasive macroalga Asparagopsis taxiformis in the Mediterranean Sea. Mar Environ Res. 141, 44–52. doi: 10.1016/j.marenvres.2018.07.016
Navarro-Barranco, C., Muñoz-Gómez, B., Saiz, D., Ros, M., Guerra-García, J. M., Altamirano, M., et al. (2019). Can invasive habitat-forming species play the same role as native ones? The case of the exotic marine macroalga Rugulopteryx okamurae in the Strait of Gibraltar. Biol. Invasions. 21, 3319–3334. doi: 10.1007/s10530-019-02049-y
Ocaña, O., Afonso-Carrillo, J., and Ballesteros, E. (2016). Massive proliferation of a dictyotalean species (Phaeophyceae, Ochrophyta) through the Strait of Gibraltar (Research note). Rev. Acad. Canar. Cienc. 28, 165–170.
Ojaveer, H., Galil, B. S., Campbell, M. L., Carlton, J. T., Canning-Clode, J., Cook, E. J., et al. (2015). Classification of non-indigenous species based on their impacts: considerations for application in marine management. PLoS Biolo. 13:e1002130. doi: 10.1371/journal.pbio.1002130
Pittman, S. J., Costa, B., Jeffrey, C. F. G., and Caldow, C. (2011). Importance of seascape complexity for resilient fish habitat and sustainable fisheries. Proc, Gulf Caribbean Fish. Inst. 63, 420–426.
Real, R., Barbosa, A. M., and Vargas, J. M. (2006). Obtaining environmental favourability functions from logistic regression. Environ. Ecol. Stat. 13, 237–245. doi: 10.1007/s10651-005-0003-3
Ribera-Siguan, M. A. (2003). “Pathways of biological invasions of marine plants,” in Invasive Species: Vectors and Management Strategies, eds G. M. Ruiz and J. T. Carlton (Washington: Island Press), 183–226. doi: 10.1111/j.1366-9516.2004.00120.x
Ruesink, J. L., and Collado-Vides, L. (2006). Modeling the increase and control of Caulerpa taxifolia, an invasive marine macroalga. Biol. Invasions 8, 309–325. doi: 10.1007/s10530-004-8060-3
Sánchez-Moyano, J. E., Estacio, F. J., García-Adiego, E. M., and García-Gómez, J. C. (2000). The molluscan epifauna of the alga Halopterix scoparia in southern Spain as a bioindicator of coastal environmental conditions. J. Molluscan Stud. 66, 431–448. doi: 10.1093/mollus/66.4.431
Schejter, L., Martín de Nascimento, J., and Lovrich, G. A. (2017). Unveiling the submarine landscape of the Namuncurá Marine Protected Area, Burdwood Bank, SW Atlantic Ocean. Pan-Am. J. Aquat. Sci. 12, 248–253.
Sedano, F., Navarro-Barranco, C., Guerra-García, J. M., and Espinosa, F. (2020). From sessile to vagile: understanding the importance of epifauna to assess the environmental impacts of coastal defence structures. Estuar. Coast. Shelf Sci. 235:106616. doi: 10.1016/j.ecss.2020.106616
Sempere-Valverde, J., Ostalé, E., Maestre, M., González, R., Bazairi, H., and Espinosa, F. (2020). Impacts of the non-indigenous seaweed Rugulopteryx okamurae on a Mediterranean coralligenous community (Strait of Gibraltar): the role of long-term monitoring. Ecol. Indic. 121:107135. doi: 10.1016/j.ecolind.2020.107135
Soussi, A., Bersani, C., Sacile, R., Bouchta, D., El Amarti, A., Seghiouer, H., et al. (2020). Coastal risk modelling for oil in the mediterranean sea. Adv. Sci. Technol. Eng. Syst. 5, 273–286. doi: 10.25046/aj0504234
Templado, J. (2011). “La diversidad marina en España,” in Biodiversidad: Aproximación a la Diversidad Botánica y Zoológica en ESPAÑA, ed. J. L. Viejo (Madrid: Real Sociedad Española de Historia Natural, Segunda época), 343–362.
Underwood, A. J. (1994). On beyond BACI: sampling designs that might reliably detect environmental disturbances. Ecol. Appl. 4, 3–15. doi: 10.2307/1942110
Urra, J., Gofas, S., Rueda, J. L., Marina, P., Mateo-Ramírez, A., Antit, M., et al. (2017). Biodiversity and biogeographical patterns of molluscan assemblages in vegetated and unvegetated habitats in the northern Alboran Sea (W Mediterranean Sea). Mar. Biodiv. 47, 187–201. doi: 10.1007/s12526-016-0468-3
Vaz-Pinto, F., Rodil, I. F., Mineur, F., Olabarria, C., and Arenas, F. (2014). “Understanding biological invasions by seaweeds,” in Marine Algae: Biodiversity, Taxonomy, Environmental Assessment, and Biotechnology, eds L. Pereira and J. M. Neto (Boca Raton, FL: CRC Press), 140–177. doi: 10.1201/b17540-5
Vázquez-Luis, M., Guerra-García, J. M., Sanchez-Jerez, P., and Bayle-Sempere, J. T. (2009). Caprellid assemblages (Crustacea: Amphipoda) in shallow waters invaded by Caulerpa racemosa var. cylindracea from southeastern Spain. Helgol. Mar. Res. 63, 107–117. doi: 10.1007/s10152-008-0129-4
Verlaque, M., Steen, F., and De Clerck, O. (2009). Rugulopteryx (Dictyotales, Phaeophyceae), a genus recently introduced to the Mediterranean. Phycologia 48, 536–542. doi: 10.2216/08-103.1
Villèle, X., and Verlaque, M. (1995). Changes and degradation in a Posidonia oceanica bed invaded by the introduced tropical alga Caulerpa taxifolia in the north western Mediterranean. Bot. Mar. 38, 79–87.
Wiggins, A., and Crowston, K. (2011). “From conservation to crowdsourcing: a typology of citizen science,” in Proceedings of the Annual Hawaii International Conference on System Sciences, Kauai, HI, doi: 10.1109/HICSS.2011.207
Williams, S. L., and Smith, J. E. (2007). A global review of the distribution, taxonomy, and impacts of introduced seaweeds. Annu. Rev. Ecol. Evol. Syst. 38, 327–359. doi: 10.1146/annurev.ecolsys.38.091206.095543
Zabala, M., and Ballesteros, E. (1989). Surface-dependent strategies and energy flux in benthic marine communities or, why corals do not exist in the Mediterranean. Scient. Mar. 53, 3–17.
Zenetos, A., Gofas, S., Morri, C., Rosso, A., Violanti, D., García-Raso, E., et al. (2012). Alien species in the Mediterranean Sea by 2012. A contribution to the application of European Union’s Marine Strategy Framework Directive (MSFD). Part II. Introduction trends and pathways. Mediterr. Mar. Sci. 13, 328–352. doi: 10.12681/mms.327
Zenetos, A., Gofas, S., Verlaque, M., Cinar, M. E., García-Raso, E., Bianchi, C. N., et al. (2010). Alien species in the Mediterranean Sea by 2010. A contribution to the application of European Union’s Marine Strategy Framework Directive (MSFD). Part I. Spatial distribution. Mediterr. Mar. Sci. 11:381. doi: 10.12681/mms.87
Keywords: Rugulopteryx okamurae, Citizen Science, benthic biota, monitoring, invasive species, invasive macroalgae, El Estrecho Natural Park, Strait of Gibraltar
Citation: García-Gómez JC, Florido M, Olaya-Ponzone L, Rey Díaz de Rada J, Donázar-Aramendía I, Chacón M, Quintero JJ, Magariño S and Megina C (2021) Monitoring Extreme Impacts of Rugulopteryx okamurae (Dictyotales, Ochrophyta) in El Estrecho Natural Park (Biosphere Reserve). Showing Radical Changes in the Underwater Seascape. Front. Ecol. Evol. 9:639161. doi: 10.3389/fevo.2021.639161
Received: 08 December 2020; Accepted: 12 March 2021;
Published: 15 April 2021.
Edited by:
Ana Sofia Vaz, University of Granada, SpainReviewed by:
Alessandro Buosi, Ca’ Foscari University of Venice, ItalyAntonella Petrocelli, National Research Council (CNR), Italy
Copyright © 2021 García-Gómez, Florido, Olaya-Ponzone, Rey Díaz de Rada, Donázar-Aramendía, Chacón, Quintero, Magariño and Megina. This is an open-access article distributed under the terms of the Creative Commons Attribution License (CC BY). The use, distribution or reproduction in other forums is permitted, provided the original author(s) and the copyright owner(s) are credited and that the original publication in this journal is cited, in accordance with accepted academic practice. No use, distribution or reproduction is permitted which does not comply with these terms.
*Correspondence: Marta Florido, bWZsb3JpZG8uY0BnbWFpbC5jb20=
†These authors share first authorship