- 1Laboratorio de Biología Marina, Departamento de Zoología, Facultad de Biología, Universidad de Sevilla, Seville, Spain
- 2Área de Investigación I+D+i del Acuario de Sevilla, Seville, Spain
- 3Estación de Biología Marina del Estrecho, Ceuta, Spain
- 4Biodiversidad y Ecología Acuática de la Universidad de Sevilla, Departamento de Zoología, Facultad de Biología, Seville, Spain
The present study constitutes the first evaluation of the space colonization strategies performed by Rugulopteryx okamurae when co-occurring with the resident macroalgal community in the introduced areas. Since the first apparition of the nonindigenous macroalga in the Strait of Gibraltar, its high propagation capacity together with its colonization ability has enhanced the establishment success of the species in detriment of the resident biota. In this study, we carried out observational surveys during 2017–2020 in order to assess the coverage levels of R. okamurae on different lighting conditions, surface orientations, and substrata types (artificial and natural). Results revealed that, beyond the high percent coverages already reported at illuminated and semi-illuminated natural rocky habitats, R. okamurae is able to settle on a wide variety of artificial substrata. The settlement performance of the species was also investigated and different mechanisms underlying the space colonization were proposed. Thus, R. okamurae was observed interacting with 43 resident macroalgal species at generally illuminated rocky habitats of the northern Strait coasts. Six colonization mechanisms were proposed for spatial growth scenarios. Overall, results pointed out that, in most of the cases where the invasive species co-occur with the resident community, R. okamurae would be favored as regards spatial growth success. Competitive interactions and environmental factors which influence results obtained must be addressed in order to fully predict impacts on resident communities. Moreover, together with previous scientific works, overall data provided in this study highlight the need to urgent implement management measures focused on habitats susceptible to be invaded, as well as studies on the ecology and dispersal vectors of R. okamurae in the Strait of Gibraltar and adjacent areas.
Introduction
The introduction and spread of nonindigenous marine species (NIS) are within the major threats to global biodiversity, natural resources, and human health and constitute a priority for the protection and management of coastal areas (Bax et al., 2003; Galil et al., 2018). When an introduced NIS acclimates and expresses an aggressive ecological behavior, its status progresses from established NIS to invasive NIS. In such cases, NIS competes with the resident community for resources like space, so the ecosystem and its services can be altered. In the case of macroalgae, the establishment of the introduced species may lead to competitive interactions with the resident macroalgal community through lateral and epiphytic growth, which can be facilitated by a higher thermal tolerance than its competitors and the production of secondary metabolites for allelopathic defense (Tronholm et al., 2012). Secondary metabolites can ease lateral competition but also impede predation by native consumers, thus increasing the ecological competitiveness of the species in the introduced environments (Pereira and Da Gama, 2008). Consequently, NIS may severely affect the marine ecosystems by modifying the local habitats, community structure, and food webs (Viard and Comtet, 2015; Marks et al., 2018). To meet EU regulation on the prevention and management of NIS, it is highly necessary to establish standard methodologies and monitoring plans in sensitive and little-studied areas (Galil et al., 2018).
The Strait of Gibraltar is a biodiversity hotspot located in the convergence of three biogeographic provinces within the Atlantic–Mediterranean subregion (Ekman, 1953). This area is highly sensitive to environmental changes (Coll et al., 2010), and it experiences an intense maritime traffic, which is a major vector for the introduction of NIS (Papacostas et al., 2017). The benthic communities of the Strait of Gibraltar may have already suffered the cumulative impacts from the invasive NIS Asparagopsis armata, Asparagopsis taxiformis, and Caulerpa cylindracea (Boudouresque and Verlaque, 2002; Andreakis et al., 2004; Rivera-Ingraham et al., 2010). In such vulnerable geographical area, the detection and monitoring of potential invasive species can provide valuable information. Mechanisms that can influence the invasion success of marine macroalgae remain misunderstood, even though they are critical for the successful mitigation and conservation of the ecosystems affected (Noè et al., 2018).
In 2015, the brown macroalga Rugulopteryx okamurae, native to the Northwestern Pacific (Hwang, 1994), was detected for the first time on both shores of the Strait of Gibraltar (Altamirano-Jeschke et al., 2016; Ocaña et al., 2016). In the Mediterranean Sea, this species was previously recorded on the coastal lagoon of Thau (French Mediterranean coast), presumably introduced through the commerce of Japanese oysters for aquaculture (Verlaque et al., 2009). Nevertheless, in Thau lagoon, this species did not show a critical invasive behavior as it happened in the Strait coasts (Verlaque et al., 2009; García-Gómez et al., 2018). During 2015–2016, R. okamurae quickly colonized most of the shoreline of this Strait, requiring municipal cleaning machines to remove more than 5,000 tons of detached biomass from the touristic beaches of Ceuta, North Africa (Ocaña et al., 2016; El Aamri et al., 2018). The massive algal wracks detected (Supplementary Figure 1), together with the huge coverages identified when the species is attached to the rocky bottoms (García-Gómez et al., 2018, 2020), suggest that this case is worryingly unusual because of the large amounts of biomass produced in such a small geographical area. Beyond the ecological impacts, social and economic consequences of the invasive behavior of R. okamurae can be also assumed (e.g., the massive biomass released on the coasts threats the tourist inflow and the fishing sector as it becomes entangled in nets, hindering the fish extraction).
On its native distributional range, R. okamurae is abundant throughout the year. The maximum growth and reproductive development occurs above 15°C, while the thallus is reduced to a basal system of perennial rhizoids in winter (Kajimura, 1992; Hwang et al., 2009). Agatsuma et al. (2005) suggests that the biological cycle of the species in the pacific waters is completed in 2 years, but it is still unknown if the species is able to complete its life cycle in the Mediterranean and Atlantic waters (Verlaque et al., 2009; Altamirano-Jeschke et al., 2016, 2017). Despite that sexual reproduction is still unperceived in the introduced habitats, the vegetative and asexual strategies of the established populations (propagules and monospores) have led to the massive occupation of most of the rocky bottoms (Altamirano-Jeschke et al., 2017 and Altamirano et al., 2019), becoming present all-year round in the southern and northern coasts of the Strait of Gibraltar.
The extreme propagation capacity of the species, combined with a high survival capability, would make the impact magnitude high and any secondary spread events worrisome. In fact, shortly after delimiting the distributional range of R. okamurae in 2017 (see García-Gómez et al., 2020), massive wrack deposits of the species were sighted at points beyond its eastern and western limits along the northern coasts of the Strait of Gibraltar, in Granada and Almeria (Altamirano et al., 2019; Figueroa et al., 2020). Moreover, preliminary models predicting suitable areas for the incoming establishment of R. okamurae populations have evidenced the risk of nearby areas along the entire southern and eastern Iberian Peninsula, where protected areas and others of great conservation interest are included (Muñoz et al., 2019).
This study aims to perform a preliminary evaluation of the establishment potential of R. okamurae in the introduced habitats, as well as the vulnerability of the resident benthic macroalgae facing its spatial growth and propagation through the coasts of the Strait of Gibraltar. Specifically, the following objectives were raised (1) to estimate the coverage of R. okamurae on different substrata, orientations, and lighting conditions and (2) to assess the implications on the resident macroalgal community when R. okamurae is established in the same substrata, highlighting apparent colonization strategies underlying the spatial growth of the invasive species.
Materials and Methods
Sampling Locations
Observational studies were performed in 12 surveys (period from June to September, during 2017–2020), comprehending all the ranges of distribution of R. okamurae on the northern coasts of the Strait of Gibraltar and adjacent areas. Each survey corresponded to one sampling site where the invasive species was present and attached to the rocky bottom and located less than 20 km from each other (Figure 1). This area hosts the Marine Protected Area “El Estrecho Natural Park,” included within The Intercontinental Biosphere Reserve of the Mediterranean. The study sites were the following: Barbate, Punta Camarinal, Bolonia, Isla de Tarifa Oeste, Isla de Tarifa Este, Torre del Guadalmesí, Faro de Punta Carnero, La Ballenera, Punta de San García, Crinavis, Puerto de La Alcaidesa, and Playa de La Alcaidesa.
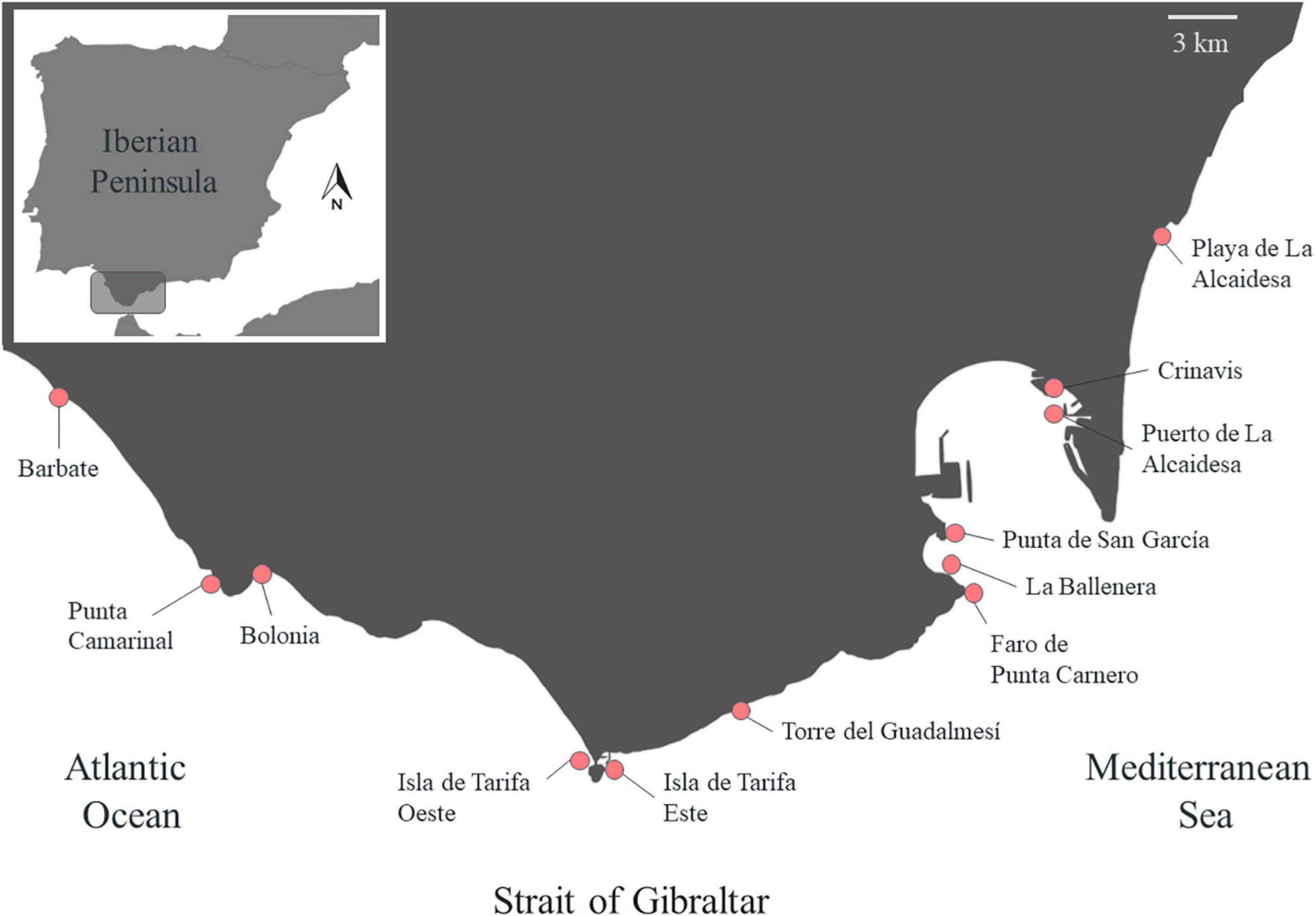
Figure 1. Location of the sites surveyed across the northern coast of the Strait of Gibraltar and adjunct areas: Barbate (36°10′1.45″N, 5°53′28.60″O); Punta Camarinal (36°4′48.64″N, 5°47′58.51″O); Bolonia (36° 5′9.37″N, 5°47′4.56″O); Isla de Tarifa Oeste (36°0′16.72″N, 5°36′44.22″O); Isla de Tarifa Este (36°0′14.25″N, 5°36′20.41″O); Torre del Guadalmesí (36°2′8.30″N, 5°31′15.58″O); Faro de Punta Carnero (36°4′35.93″N, 5°25′29.14″O); La Ballenera (36°5′5.71″N, 5°25′57.41″O); Punta de San García (36°6′16.37″N, 5°25′48.19″O); Crinavis (36°9′52.15″N, 5°22′23.30″O); Puerto de La Alcaidesa (36°9′19.99″N, 5°21′57.90″O); and Playa de La Alcaidesa (36°13′58.02″N, 5°18′53.36″O).
Coverage of R. okamurae on Different Substrata and Lighting Conditions
In each sampled site, an estimation of R. okamurae coverage was carried out by direct visual observations in three 50-m-long and 4-m-wide transects. When necessary, in situ observations were supported by video- and photographic data taken in the same survey. Transects were located parallel to the shoreline between 0 and 10 m depth. In the case of sites where the considered substrata limited the sampling area (i.e., artificial structures at Puerto de La Alcaidesa and Crinavis), transects were located parallel to the shoreline but distributed along the horizontal plane. Thus, the coverage of R. okamurae was assessed considering the following substrata conditions: inclination (i.e., vertical and horizontal), lighting conditions (i.e., highly illuminated, moderately illuminated, poorly illuminated and unlit areas), and substrata nature (i.e., natural and artificial substrata). Natural substrata were represented by maërls and pebbles, limestone, sandstone, slate, and wood. Meanwhile, artificial substrata considered were breakwater boulders near sandy bottoms, cement and concrete, ceramics, breakwater limestone boulders, abandoned fishing nets and ropes, glass bottles, metallic surfaces, metallic surfaces on boats and ships, plastics, and car tires.
The total percent cover of R. okamurae per substratum was estimated as the overall coverage occupied at each abovementioned condition within the 200 square meters of each transect. Because some anomalous artificial substrata are not frequently found while crucial considering space availability in marine habitats, a selective exploration was carried near the limits of the sampling transects when considering components of the marine debris (i.e., plastics, ceramics, abandoned fishing nets and ropes, glass bottles, and car tires). Overall R. okamurae coverage values were included within a 0 to 3 scale attending to different ranges. Thus, if the coverage ranged between 1 and 30% for all aforementioned transects at one specific substratum, the species was classified as “present” (1) for that substratum; percent coverages between 31 and 60% were classified as “abundant” (2) and coverages over 61% as “dominant” (3). Resident macroalgal coverage was not included since competitive scenarios were not contemplated for this objective.
Substrata Types and Colonization Strategies Associated With R. okamurae Establishment
In order to better understand how R. okamurae is spatially growing on the coastal habitats, the presence of the invasive macroalga was examined in, at least, one of all aforementioned sampling sites by direct—in situ—or indirect observation (photographs and/or videos). In surveys, we recorded the spatial growth scenarios where R. okamurae co-occurred with other macroalgal species when established at different recipient habitats. Habitats were differentiated attending to rocky substrata nature (natural and artificial), tide exposition (intertidal areas, tide pools, and subtidal areas), illumination (illuminated and shadow habitats), and bottom composition (sandy bottoms). The resident macroalgal community was identified to the lowest taxonomic level possible, and the percentage of co-occurring scenarios at different habitats was recorded. Because preliminary surveys revealed different coexistence patterns, the spatial colonization strategies by which R. okamurae seems to become established on generally illuminated rocky habitats were proposed.
To synthesize all the information obtained about the spatial growth of R. okamurae, Venn diagrams were created using Venny 2.1 online software (Oliveros, 2007). Venn diagrams allow to visualize and analyze relationships between different data groups by representing all possible combinations and elements contained in each one of them. Identifying the spatial growth strategies described as groups, displayed diagrams allow the identification of shared (overlapped circles) and unique species (independent regions of circles) performing each interaction described and/or their different combinations. No more than four group combinations were displayed in each analysis to comply with the requirements of the exotic geometries of the software (Oliveros, 2007).
Results
Coverage of R. okamurae on Different Substrata and Lighting Conditions
Rugulopteryx okamurae dominated (i.e., 100% coverage) at highly illuminated areas (vertical and horizontal) and moderately illuminated horizontal surfaces (0–10 m depth) (Table 1). The species was abundant (31–60%) at vertical moderately illuminated areas, present (1–30%) at horizontal surfaces of the entrance of caves and crevices, and totally absent (0% coverage) at unlit areas and vertical poorly illuminated habitats. Concerning substrata nature, 100% of coverage was obtained for limestone and sandstone (natural rocky substrata), and limestone rip-rap boulders (artificial rocky substrata). The species was abundant (31–60% coverage) at ceramics, tires, and abandoned fishing nets and ropes, while present (1–30% coverage) on a wide variety of substrata, including breakwater boulders close to sandy bottoms, cement and concrete, glass bottles, metallic surfaces (including those from the upper zone of boats and ship hulls), and plastics.
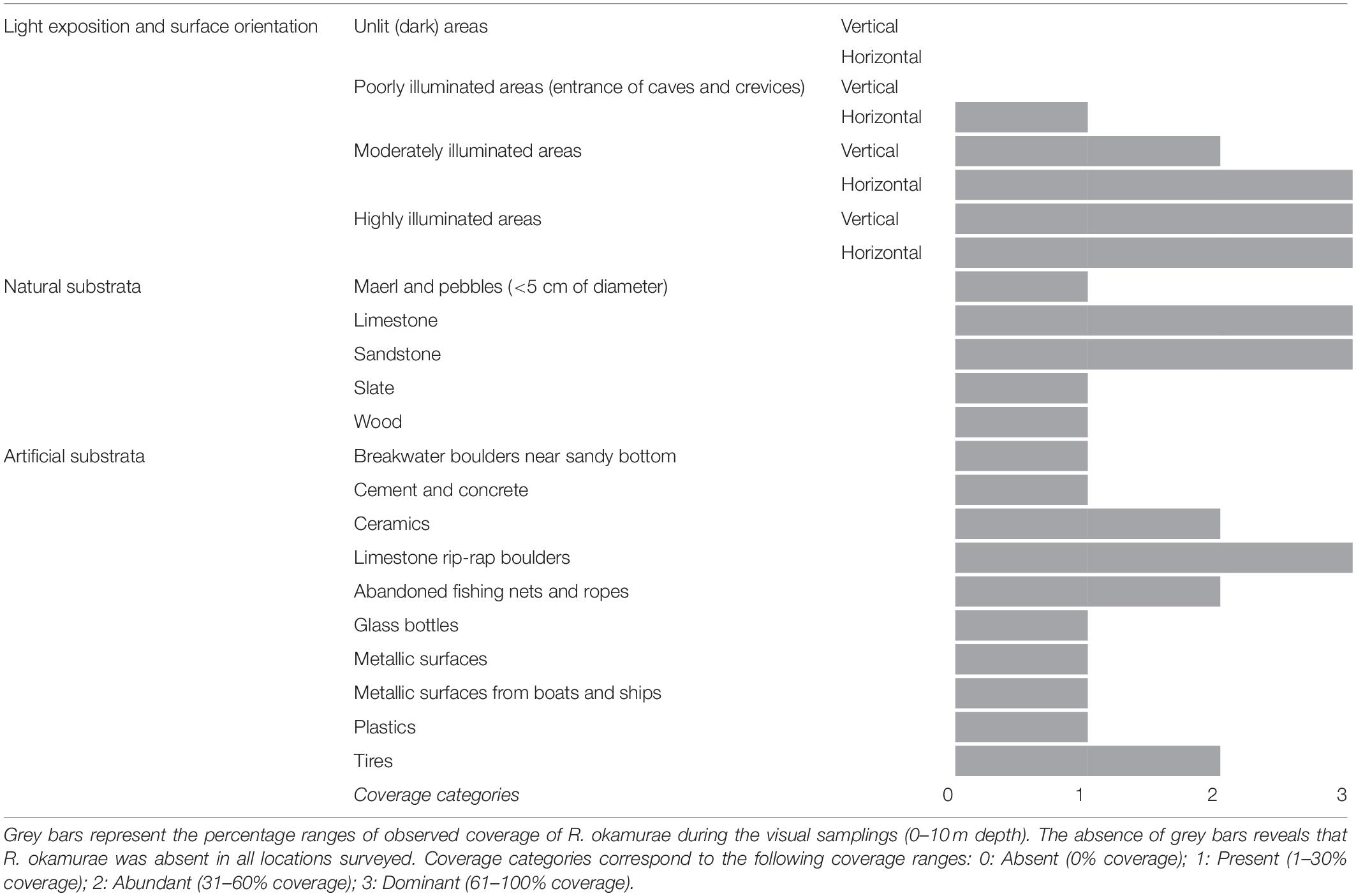
Table 1. Coverage of Rugulopteryx okamurae under different lighting conditions and substrata nature (natural and artificial substrata).
Habitats and Colonization Strategies Underlying R. okamurae Establishment
Forty-three macroalgal species were detected in relation to possible implications inferred by the spatial establishment of R. okamurae, including four NIS (A. armata, A. taxiformis, C. cylindracea, and Dictyota cyanoloma). Species which spatially co-occurred with R. okamurae at each habitat type are listed in Table 2 (left section). In 22 and 23% of the cases, the invasive species co-occurred with the macroalgal community at natural and illuminated habitats, respectively (Figure 2A). Sublittoral habitats harbored 17% of the spatial growth scenarios, while tide pools, artificial structures, and intertidal zones recorded 10, 10, and 9%, respectively. Sandy (5%) and shadow bottoms (4%) were less represented, being the habitats where R. okamurae was rarely observed spatially growing and interacting with the resident community.
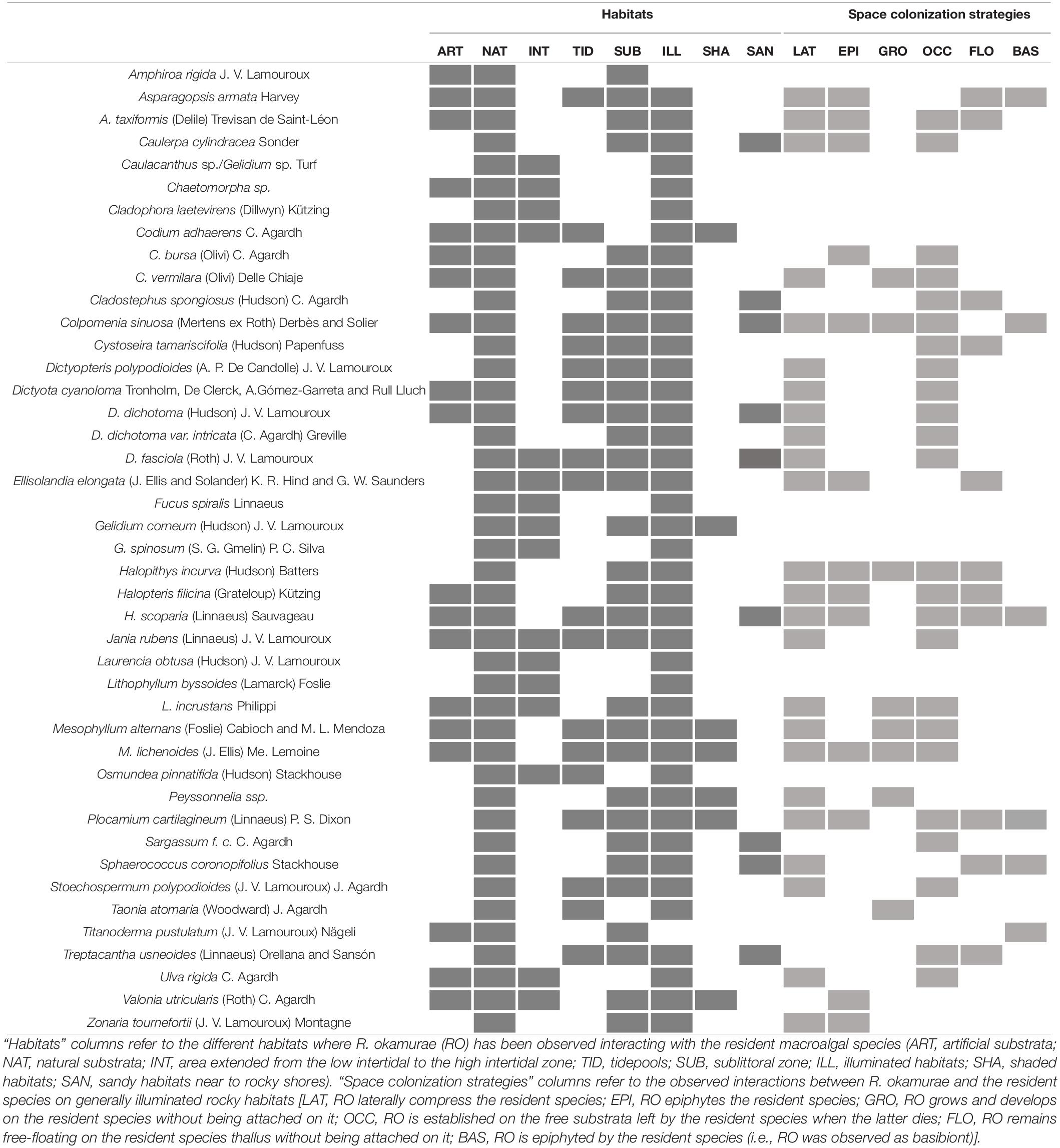
Table 2. Presence (shaded quadrats) of perceived interactions between R. okamurae and resident macroalgal species at introduced habitats.
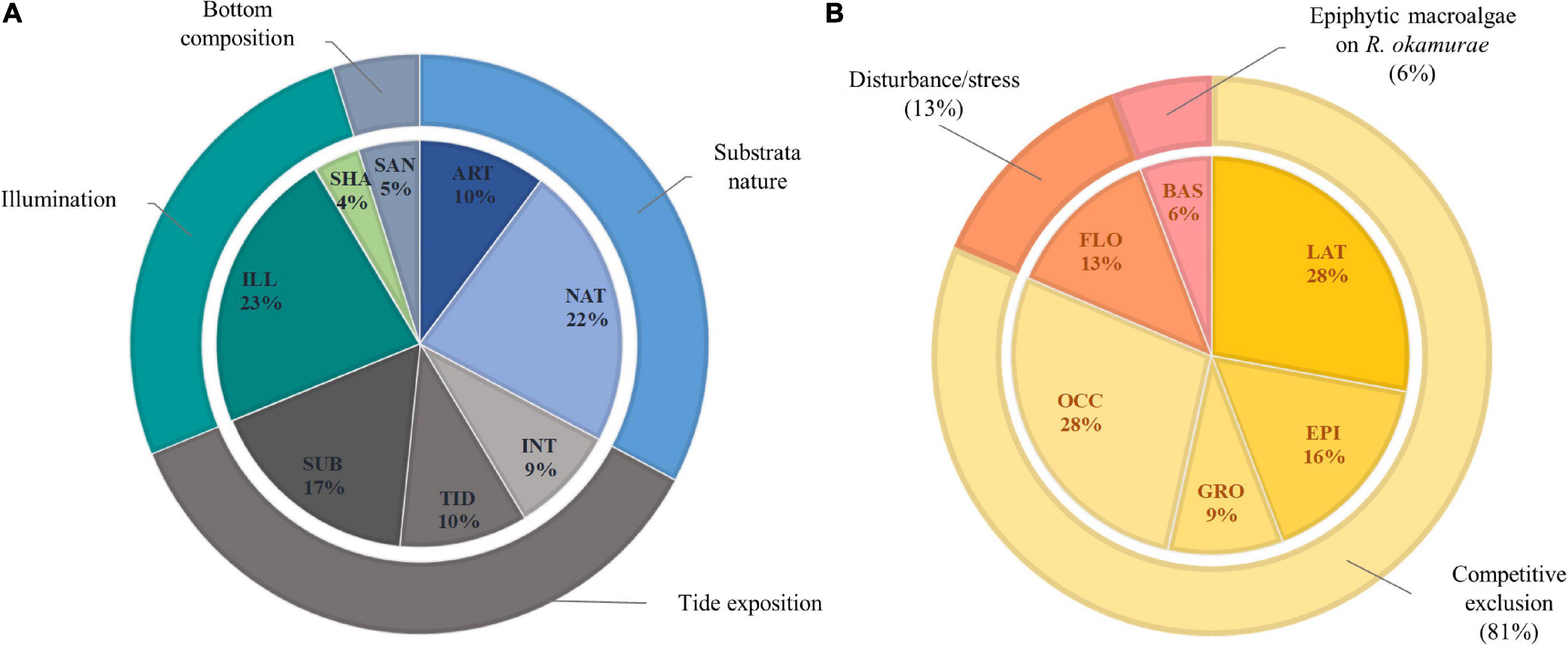
Figure 2. (A) Percentage of total observed interactions between R. okamurae (RO) and the 43 resident macroalgal species present at each habitat surveyed in the study area (ART, artificial substrata; NAT, natural substrata; INT, area extended from the low intertidal to the high intertidal zone; TID, tidepools; SUB, sublittoral zone; ILL, illuminated habitats; SHA, shaded habitats; SAN, sandy habitats near to rocky shores). (B) Percentage of space colonization strategies between RO and the resident macroalgal community [LAT, RO laterally compress the resident species; EPI, RO epiphytes the resident species; GRO, RO grows and develops on the resident species without being attached on it; OCC, RO is established on the free substrata left by the resident species when the latter dies; FLO, RO remains free-floating on the resident species thallus without being attached on it; BAS, RO is epiphyted by the resident species (i.e., RO was observed as basibiont)].
Six spatial growth strategies by which R. okamurae becomes established at generally illuminated rocky habitats were proposed regarding possible effects on the resident macroalgae (right section of Table 2 and Figures 2B, 3). In two of the strategies identified, we suggest the space colonization as a result of the direct interaction with the resident macroalgae. Thus, competition by lateral compression (LAT) and overgrowth as epiphyte (EPI) were proposed for 28% and 16% of the total cases, respectively, where R. okamurae was established on the substrata interacting (i.e., co-occurring) with the resident macroalgal community. Other settlement scenarios did not show signs of direct macroalgal interaction although the final occupancy by the invasive species was expected to occur. Thus, the invasive species was observed to grow and develop on the resident macroalgae without being attached on the adult thalli (GRO) (9%) and, in 28% of the cases, the resident species were surrounded by R. okamurae, which remained abundantly established around the resident macroalgal populations (OCC). In this last strategy, and according to overall observations, R. okamurae became established on the space left by the previously established populations with the resident macroalgae death, as a consequence of its biological cycle or another unidentified factors (e.g., abiotic stressors, predation).
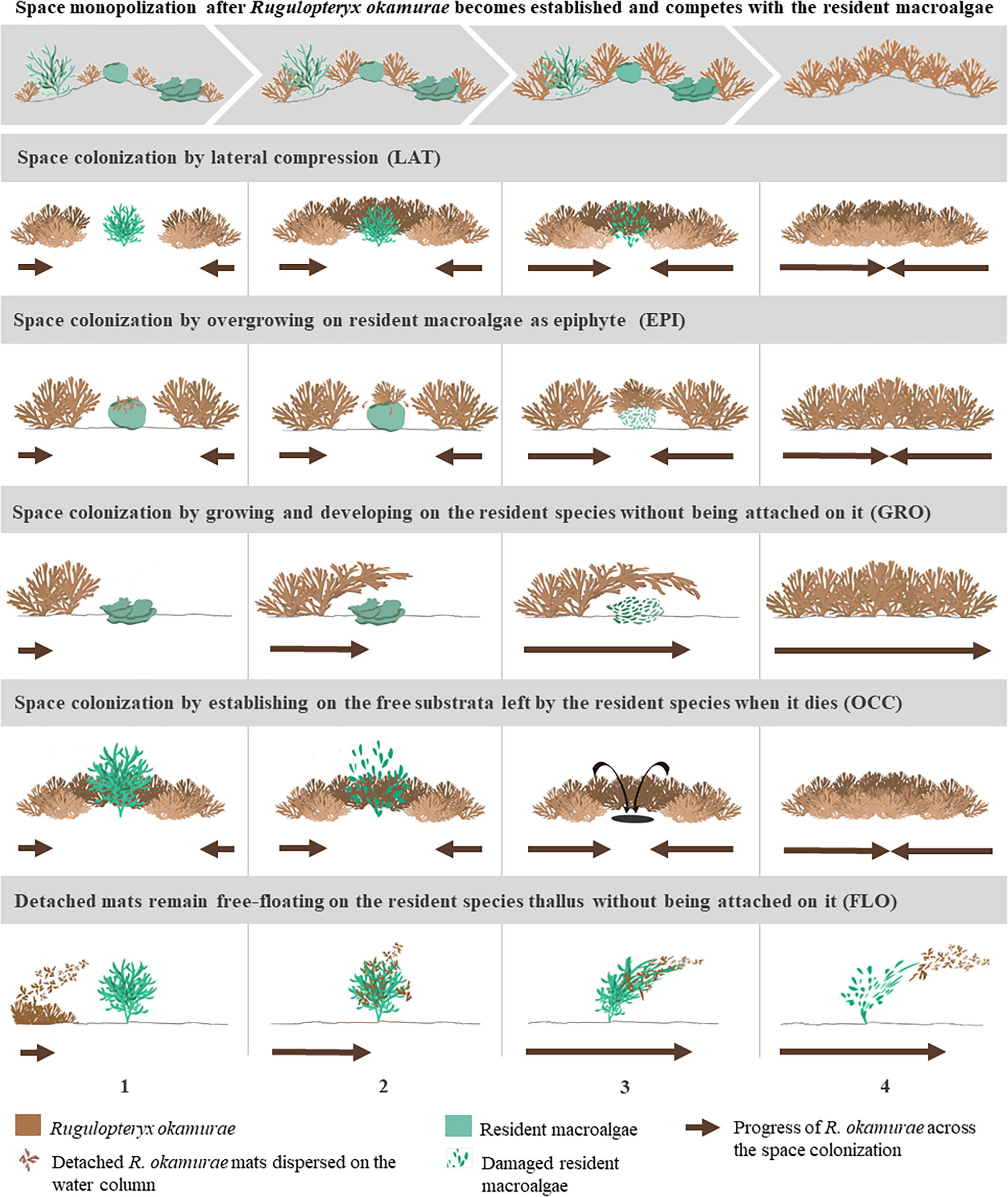
Figure 3. llustrative diagram showing space colonization strategies described between R. okamurae and resident marine macroalgae and subsequent implications in generally illuminated rocky bottoms of the Strait of Gibraltar. Numbers represent the sequential stages of the process.
Surveys revealed other cases where, despite that biological interactions between both macroalgae were apparently perceived, no direct evidences of negative impacts on the resident community were observed. However, it must be taken into account that physicochemical factors, physiological impacts, or other indirect effects from R. okamurae competitiveness have not been examined here, so results obtained for the latter mechanisms must constitute just the preliminary basis to further investigate patterns observed if they happened (impacts). Two main processes needed to be differentiated in this case: on the one hand, we described spatial growth events where disturbance/stress processes may be occurring even though they are not perceived yet. Despite the lack of negative evidences of resident species death or damaged tissues in our surveys, this strategy was assumed to lead to disturbance events in the species involved since indirect implications derived from similar competitive scenarios have been obtained and could be likely to occur in the study area (e.g., indirect implications like shading, chemical alteration, bioturbation). In this regard, only one strategy was proposed for the 13% of the overall co-occurrence cases. It was described for those cases where R. okamurae mats, detached from the rocky bottom and dispersed in the water column, become free-floating on the resident species thallus without being totally attached on them (FLO). On the other hand, we also perceived colonization strategies leaded by the resident macroalgae. Unless R. okamurae was established on the substrata, the resident macroalgae seemed to take advantage from the spatial growth (e.g., epiphytes of the invasive macroalga) in the 6% of the cases surveyed. Hence, R. okamurae was observed as a basibiont (i.e., host to a macroalgal epibiont) (BAS) (Figures 4A–C) of A. armata (Figure 4A), Colpomenia sinuosa, Halopteris scoparia, Plocamium cartilagineum (Figure 4B), Titanoderma pustulatum, and Sphaerococcus coropifolius.
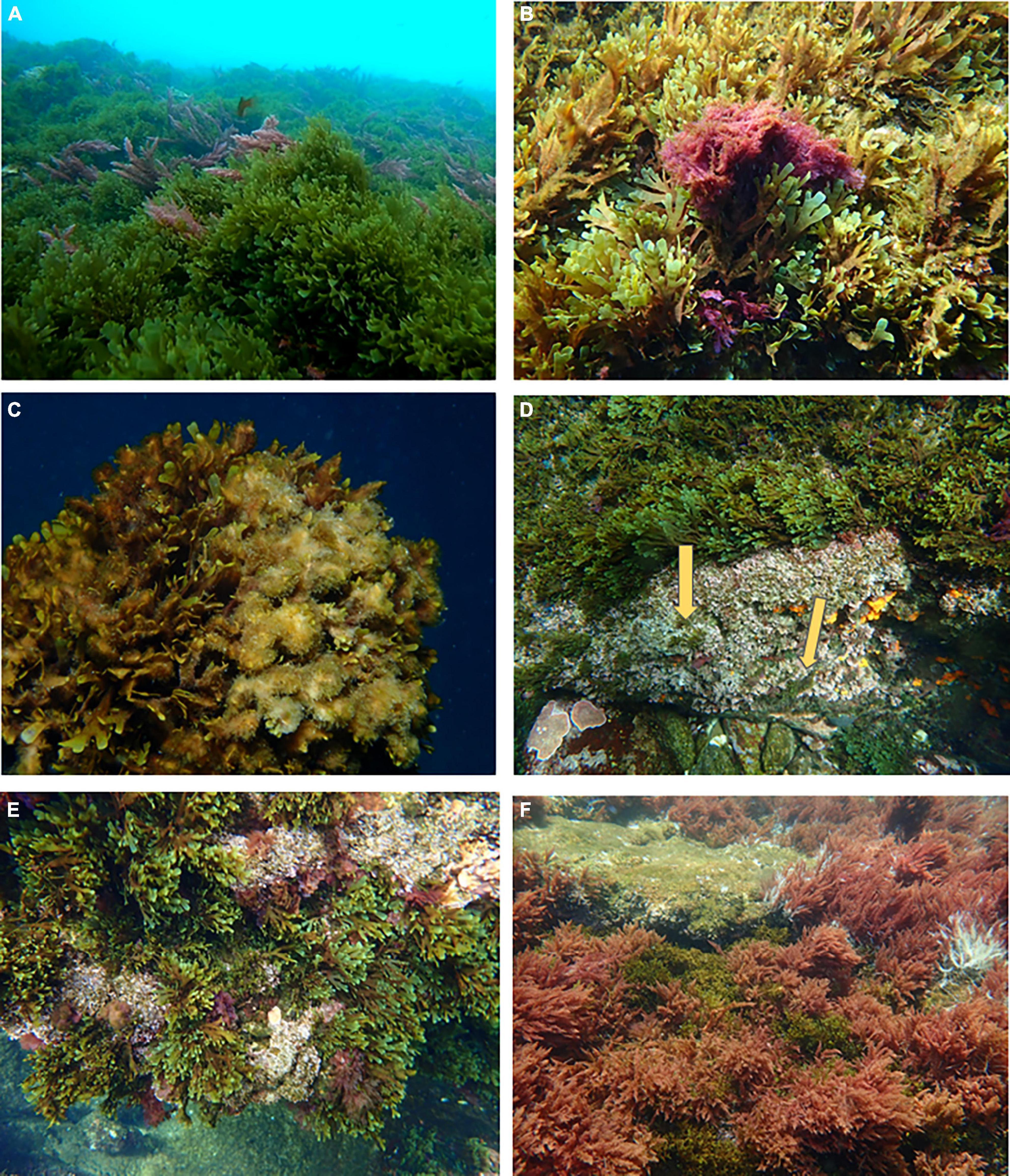
Figure 4. (A) Overgrowth of Asparagopsis armata on Rugulopteryx okamurae in the Strait of Gibraltar (1–5 m depth) after 2017. (B) Overgrowth of Plocamium cartilagineum and (C) generalist algae on R. okamurae. (D) Ellisolandia elongata surrounded by R. okamurae fronds with small specimens already inserted on E. elongata specimens (arrows). (E) Expansion of R. okamurae in detriment of E. elongata in rocky illuminated habitats. (F) Asparagopsis armata laterally compressed by R. okamurae before 2017.
Mechanisms underlying spatial exclusion were those expected to seriously compromise resident species survival, so they were selected to be explored with Venn diagrams. Thus, relations between LAT, EPI, GRO, and OCC were together examined (Figure 5A). Besides, GRO, EPI, FLO, and BAS were independently studied in order to check possible relations in terms of disturbance/stress and overgrowth strategies (Figure 5B). Most of species displaced by LAT were also observed to suffer spatial pressure by OCC (seven common species were involved in LAT and OCC strategies: D. cyanoloma, Dictyota dichotoma, Dictyota dichotoma var. intricata, Dictyota fasciola, Dictyopteris polypodioides, Stoechospermum polypodioides, and Ulva rigida). In fact, despite that LAT was perceived to only affect two of the resident species in an exclusive way, it was the mechanism most combined with remaining interactions. In contrast, OCC was more exclusive in terms of species affected by only one spatial growth strategy of R. okamurae (i.e., five species were displaced only by OCC processes).
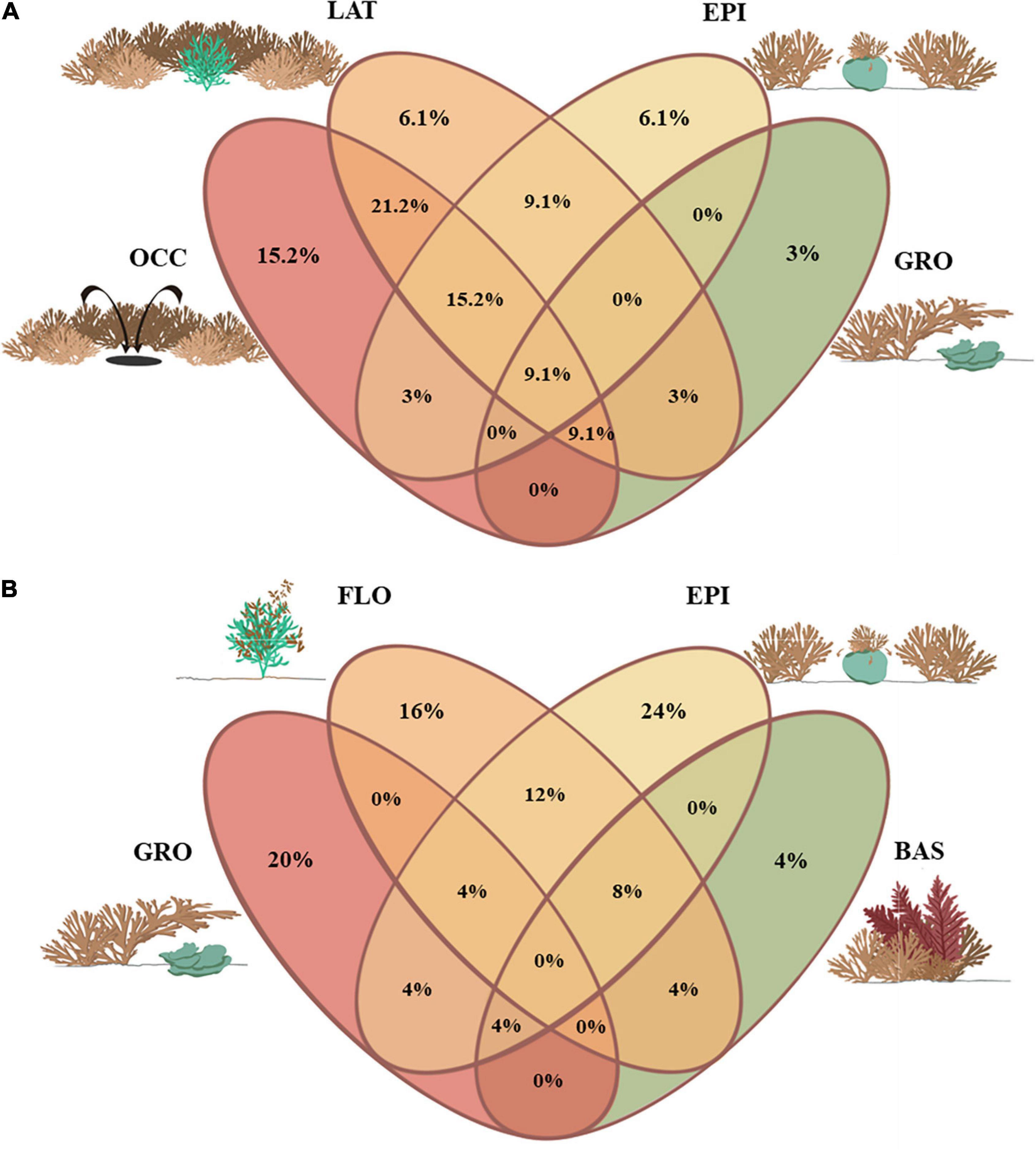
Figure 5. Venn diagrams showing the percentage of resident macroalgal species contained in each possible combination of the colonization strategies described for R. okamurae (RO) and the resident macroalgal commnunity: (A) spatial exclusion interactions and (B) overgrowth interactions. [LAT, RO laterally compress the resident species; EPI, RO epiphytes the resident species; GRO, RO grows and develops on the resident species without being attached on it; OCC, RO is established on the free substrata left by the resident species when the latter dies; FLO, RO remains free-floating on the resident species thallus without being attached on it; BAS, RO is epiphyted by the resident species (i.e., RO was observed as basibiont)].
GRO was also poor combined with other interactions of spatial exclusion. Overall, macroalgae suffering overgrowth interactions by this strategy included the macroalgal species Codium vermilara, C. sinuosa, Lithophyllum incrustans, Mesophyllum alternans, Mesophyllum lichenoides, Halopithys incurva, Peyssonnelia ssp., and Taonia atomaria. Venn diagrams revealed that six species were observed to be affected by R. okamurae establishment only by epiphytic strategies: Amphiroa rigida, C. cylindracea, Codium bursa (Figure 6), Ellisolandia elongata (Figures 4D,E), Valonia utricularis, and Zonaria tournefortii. Species epiphited by R. okamurae did not experience GRO events during the sampling process. Contrarily, spatial occupancy by free-floating mats on resident species thalli (FLO) involved the species Cladostephus spongiosus, E. elongata, A. taxiformis, Halopteris filicina, P. cartilagineum, and Treptacantha usneoides. The latter three species were also observed to be epiphited by R. okamurae. Contrarily, epibiosis on R. okamurae (BAS) was less frequent and relegated to few species: A. armata, C. sinuosa, H. scoparia, S. coronopifolius, and T. pustulatum.
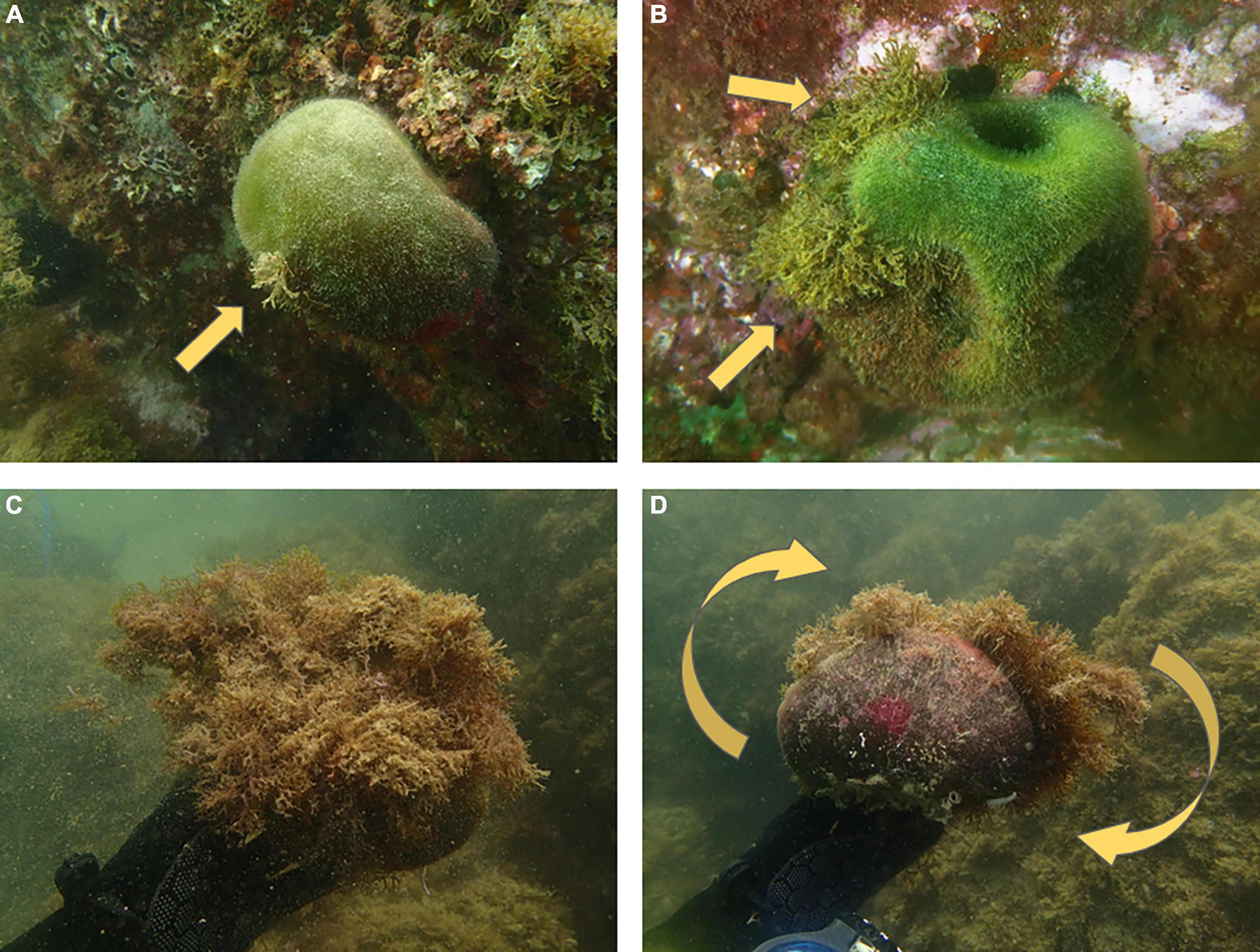
Figure 6. Example of progressive epibiosis of Codium bursa by Rugulopteryx okamurae. (A) Incipient occurrence after R. okamurae establishment. (B) Progress of R. okamurae spatial growth. (C) C. bursa extremely epiphyted by R. okamurae. (D) Opposite side of the same individual of C. bursa showing depigmented tissue in lateral and basal areas.
Discussion
It is crucial to perform observational studies to obtain full ecological characterizations in environmental assessments (Moschella et al., 2005). In this regard, this study evidences the high establishment success and preliminary spatial colonization strategies underlying the invasive potential of R. okamurae for the first time at the northern coasts of the Strait of Gibraltar. Although findings reflect a difficult scenario for mitigation strategies on the invaded areas, information about the invasibility of the resident communities could result useful in the development of early detection and rapid responses in areas not yet invaded (Lodge et al., 2006; Williams and Smith, 2007). In this respect, these preliminary results aim to advance our understanding in marine community ecology, targeting conservation efforts on the present bioinvasion case.
Substrata Conditions and Establishment Success
Results obtained for natural habitats agree with those from previous contributions (see García-Gómez et al., 2020) that well-illuminated hard rocky bottoms may present a major propagule pressure at the local range expansion of R. okamurae in the Strait of Gibraltar. Likewise, shelter conditions from subtidal rocky habitats may also facilitate the presence of introduced species even if detrimental to light availability (Piazzi and Ceccherelli, 2002), as observations performed in this study and the high coverages of R. okamurae at coralligenous (Sempere-Valverde et al., 2020) and precoralligenous habitats reflect (García-Gómez et al., 2020).
Acclimation capacity plays a key role in the success over the native communities, particularly at scenarios of environmental change (Tronholm et al., 2012; Papacostas et al., 2017). In this regard, any knowledge about the type of substrata colonized could help to better develop management actions to prevent potential dispersal vectors and to minimize the range expansion of the invasive species (Checoli-Mantelatto et al., 2020). According to our surveys, R. okamurae was present and even abundant in a number of artificial surfaces, from harbor infrastructures (e.g., breakwaters, limestone rip-rap boulders and metallic surfaces of boats) to marine litter (abandoned fishing nets, ropes, plastics, tires, and glass bottles). The diversity of artificial substrata colonized led to give attention to the potential ability of the species to take advantage to ecosystem degradation, which increases the scale of the impact on recipient communities (Occhipinti-Ambrogi and Savini, 2003). The development and survival through time and distance on different substrata nature must complement results obtained in this work for futures researches. Furthermore, raised awareness must be adopted to those sectors which may be involved in the accidental dispersion of the invasive species (e.g., correct cleaning of fishing and diving equipments). In this regard, beyond the socioeconomic impacts reported in fisheries (see García-Gómez et al., 2018; Altamirano et al., 2019), the spatial growth of R. okamurae on materials related to artificial coastal defense structures, commercial shipping, recreational boats, and even fishing equipment could imply the establishment and dispersion of the species at short (<1 km) and/or long (>1 km) distances (Ruitton et al., 2005; Lord et al., 2015). In the same way, most of materials derived from marine litter also showed to constitute adequate substrata for the invasive species, and thus the permanence or increase in marine litter in the area could become another key donor habitat for secondary spread events (Kiessling et al., 2015).
Habitat Conditions Associated With General R. okamurae–Resident Macroalgae Interactions
Once established on the rocky substrata, R. okamurae seems to be much more effective in competing for the space than most of the resident species (García-Gómez et al., 2018, 2020). Thus, competing with the photophilous community, it usually becomes dominant over other benthic taxa (García-Gómez et al., 2020; Figure 7). Parallel schemes were perceived in this study, since illuminated and natural habitats harbored most of the interactive scenarios between R. okamurae and the resident macroalgae.
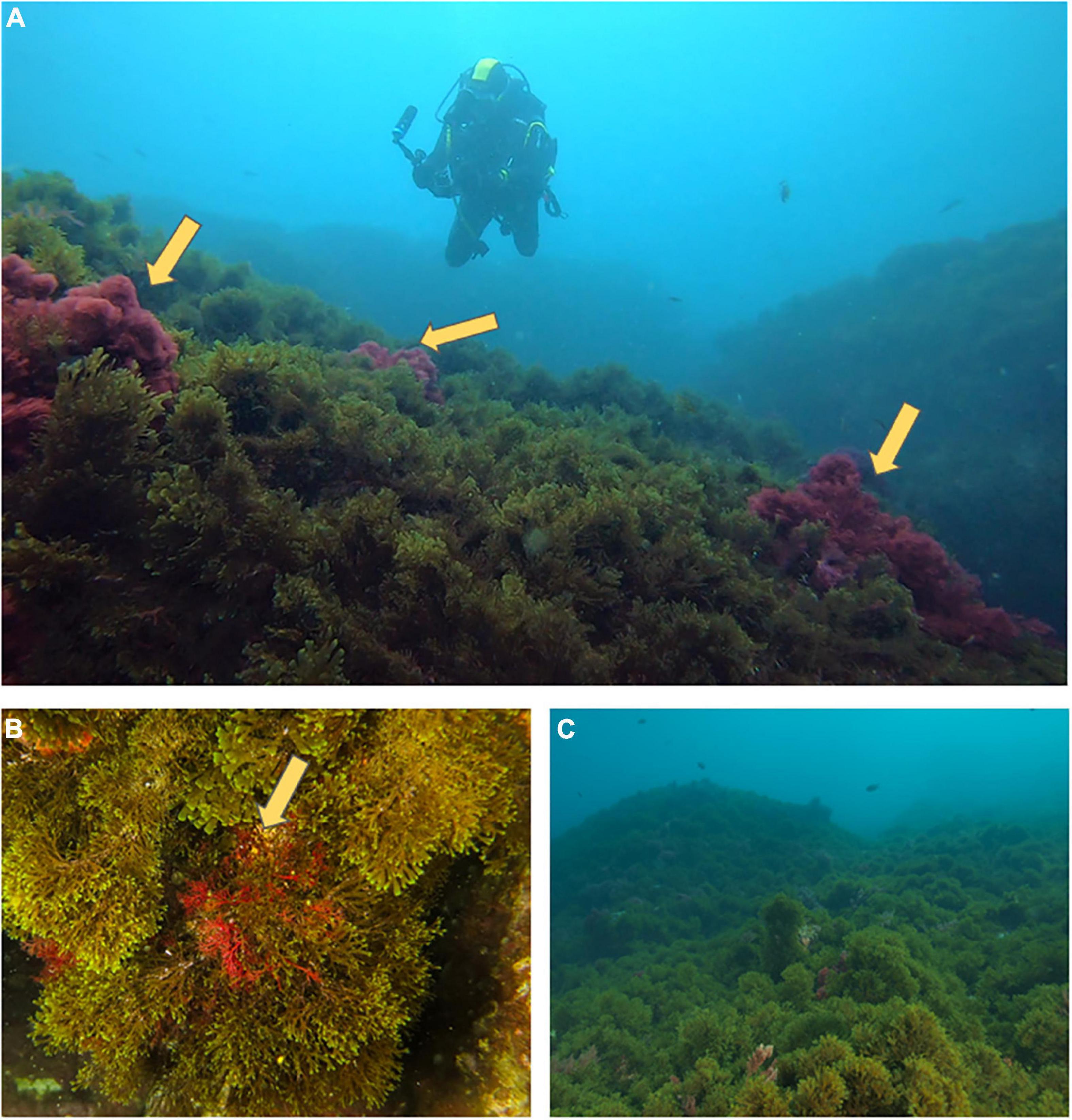
Figure 7. Resident benthic species exclusion and subsequent monopolization of the space by R. okamurae in the rocky bottoms of the Strait of Gibraltar. (A) First scheme of R. okamurae establishment on rocky substrata of the Strait of Gibraltar (–10 m depth) in the presence of resident species, as Sphaeroccocus coronopifolius (arrows). (B) S. coronopifolius expressing signs of degeneration (arrow) after/while interacting with the introduced species. (C) Resident species lost and subsequent monopolization of rocky bottoms by R. okamurae.
However, particular caution is required interpreting spatial competitive interactions and direct consequences on recipient communities, as other factors not considered can influence distribution patterns (McCook et al., 2001). More exposed habitats may compromise the adequate development of macroalgae due to abiotic stressors (i.e., canopy erosion by hydrodynamics and/or sediment abrasion) (Ruitton et al., 2005), which also makes difficult the direct observation of interactions with the recipient biota. Even more, spatial competition due to invasive macroalgae establishment may be enhanced in habitats where resources are limited (light, nutrients, or space) (Ólaffson, 2017). For example, good adaptation to low-light conditions may endow competitive advantages over other resident macroalgae in introduced habitats (e.g., the study case of the invasive Agarophyton vermiculophyllum) (Zi-Min and Lopez-Bautista, 2014). In accordance, co-occurring events detected at shadow environments stress effects already reported on endangered sciaphilic taxa by R. okamurae (Ocaña et al., 2016; García-Gómez et al., 2018; Sempere-Valverde et al., 2020).
Colonization Strategies Underlying R. okamurae Establishment
Despite that the recent loss of resident macroalgal habitats has been associated with the spread of R. okamurae in the Strait coasts to a large extent (Ocaña et al., 2016; García-Gómez et al., 2018, 2020; Altamirano et al., 2019), mechanisms underlying the invasion success of R. okamurae remain misunderstood. In this study, the spatial collision between the invasive species and the resident macroalgae allowed to better understand the spatial colonization strategies at generally illuminated rocky habitats, driving different patterns in the settlement of the introduced species.
Eighty-one percent of the scenarios observed were conceived as negative for the resident community, and thus the total displacement of the resident macroalgae was conceived as the most common consequence of the spatial establishment of R. okamurae. The strategies most addressed for scenarios perceived were the spatial exclusion by LAT and the total occupancy of the space when the resident species dies because of unidentified factors (e.g., natural death). Species exclusion and replacement can become critical in terms of habitat modification in rocky environments, especially when talking about engineered species (Buschbaum et al., 2006; Alomar et al., 2016). Indirect consequences of species replacement are not contemplated here. However, habitat changes derived from R. okamurae displacement could promote cascade effects on benthic ecosystems (e.g., associated fauna and higher trophic levels), even when habitat complexity is not compromised because species competing are morphologically similar (e.g., Navarro-Barranco et al., 2019). Together with impacts already reported on macroalgal communities (e.g., García-Gómez et al., 2018; 2020), potential implications in associated biota enhance the need to encompass the percentage cases of total exclusion found in this study to ecological repercussions under a more holistic view of the impacts at nonnative habitats.
With thermal tolerance, allelopathic defense in macroalgal communities (mainly on tropical areas) constitutes an important factor in the success of marine bioinvasions (Pereira and Da Gama, 2008; Tronholm et al., 2012). Settlement inhibition by chemical defenses does not necessarily imply significant negative impacts at community level when considering faunal taxa, because settling larvae can usually settle on other substrata (Ólaffson, 2017). Otherwise, it could become crucial for the establishment success of sessile or sedentary species dispersing by passive mechanisms (e.g., macroalgal propagules). In this regard, R. okamurae contains bioactive compounds which may limit settlement inhibition from the sesquiterpene family and spatane and seco-spatane skeleton diterpenes (Kurata et al., 1988; Ninomiya et al., 1999; De Paula et al., 2011). Indeed, epibiosis on the invasive species was an event scarcely represented when compared with other strategies of space colonization. However, epibiosis on R. okamurae must also be attributed when considering impacts on the recipient communities, especially taking into account that dense populations of the invasive macroalga have colonized wide surfaces through its bathymetric range of distribution in the northern and southern coasts of the Strait of Gibraltar (e.g., over 90% coverage at 10–20 m depth) (García-Gómez et al., 2020; Sempere-Valverde et al., 2020).
Resident species able to take advantage of the spatial growth of other species can also exist depending on the environmental conditions (Ólaffson, 2017). The established invasive species A. armata has been to overgrow R. okamurae populations in shallow subtidal bottoms after 2018 (1–5 m depth) (Figures 4A,F). It could be expected that certain competitors can limit the invasion success through time (Levine et al., 2004). Otherwise, R. okamurae could be contributing to provide habitat for other invasive species, facilitating the establishment when available space is inaccessible (Olyarnik et al., 2009). Biotic resistance in marine environments is often not strong enough (Papacostas et al., 2017), and scenarios of facilitation between invaders could make this susceptibility to progressively increase by altering the competitive landscape (Simberloff and Von-Holle, 1999). In the Strait of Gibraltar, different notorious invasions by macroalgae have happened in the latter years (e.g., A. armata, A. taxiformis, Lophocladia lallemandii, C. taxifolia, C. cicylindracea) (Boudouresque and Verlaque, 2002; Andreakis et al., 2004; Rilov and Galil, 2009; Rivera-Ingraham et al., 2010). These successive scenarios, together with other drivers of global change (e.g., changing temperature conditions), destabilize not only the current species distribution but also the structure and function of resident communities in the Mediterranean Sea (Occhipinti-Ambrogi, 2007). On the other hand, it has not been tested yet if the reproductive and vegetative behavior of R. okamurae varies throughout time, leading other species to take advantage of those moments of minimum biomass production. As in its native habitats, R. okamurae can be observed all year round in the Strait coasts, but whether the species is able to complete its life stage is still unknown (Verlaque et al., 2009; Altamirano-Jeschke et al., 2016, 2017). Studies carried out during the months of maximum algal expansion (2016–2017) have shown that R. okamurae achieves coverages greater than 30% even in the winter months at 15-m depth (García-Gómez et al., 2020), periods when it would be expected that the low temperatures make the species reduces its maximum biomass. Also, experiences on C. taxifolia by (Glasby et al., 2005a, b) show that even when the total disappearance of the algal populations occur over relatively short periods of time, recoveries in the native biota may not occur. Further studies on mentioned interactions will elucidate the capability of resident species (invasive or native) to buffer against R. okamurae displacement. Meanwhile, according to Piazzi et al. (2001), it is possible that only when resident species are able to be invaded but not displaced can the macroalgal community be said to resist the invasion of the introduced macroalga.
A different pattern is perceived when R. okamurae grows on other species thalli, as direct overgrowth often results in the total displacement of the basibiont. One example is the interaction between R. okamurae and C. bursa, represented in Figure 6. Unlike this growth strategy, the rest of observed overgrowth conditions did not imply the direct attachment of R. okamurae rhizomes or thallus. In this respect, it was noteworthy that the macroalgal species affected by the horizontal elongation of R. okamurae thallus were highly characterized by flattened and encrusting taxa, where crustose macroalgae are included. According with other marine bioinvasion cases, it should be verified whether habitat type (Piazzi et al., 2001; Ceccherelli et al., 2002) and allelopathic substances from coralline macroalgae (Vermeij et al., 2011) influence interactions by which R. okamurae rhizomes remain attached to other species.
Yet the consequences of the introduction of R. okamurae are not necessarily limited to interactions performed when attached to the rocky substrata. Mats of R. okamurae can be released after being established on the bottom becoming free-floating on the water column. It has not been clarified yet if the wrack depositions of the invasive macroalga are due to the thorough water agitation in specific geographic areas (e.g., high tidal coefficient or groundswell), the result after completing the natural life cycle, or the combination of all mentioned processes. Meanwhile, results indicate that disturbances by floating mats trapped on the thallus of resident erect macroalgae could be minimal because, at least in current areas, these mats shed shortly afterward. However, albeit negative impacts were not perceived, consequences on the macroalgal community can be wide and diverse. For example, physical damages can occur since contact can reduce light levels by shading (Kennelly, 1989), abrasion, chemical effects, and the facilitation of direct overgrowth (McCook et al., 2001). Also, overall floating mats may serve as physical barriers for settling larvae (Zi-Min and Lopez-Bautista, 2014) or pelagic organisms, whose behavior can result as modified due to the decreased visibility nearshore (Kirby et al., 2000). Figueroa et al. (2020) have pointed out that R. okamurae mats detached from the rocky bottoms and transported by currents to a certain depth are capable of maintaining high photosynthetic activity and physiologically viable spores. Because the easy dispersion of R. okamurae constitutes a threat facing other European marine areas (Altamirano et al., 2019), the role of released mats needs to be accounted also considering potential secondary spread events.
Conclusion
All the available data clarify that the high propagation capacity of R. okamurae, in combination with its competitive and spatial establishment capacity, enhances the settlement of the species once having entered an area that presents favorable biotic and abiotic factors (Altamirano et al., 2019; García-Gómez et al., 2020). Results obtained in this study reinforces the high ability of R. okamurae to grow on different substrata types, formed by not only abiotic but also biotic compounds, since macroalgal species also constitute suitable substrata and thus they could facilitate the invasion in a similar way than hard bottoms (Olyarnik et al., 2009). The high representation of strategies which implied the spatial exclusion of the resident macroalgae, together with other interactions of potential disturbance, claims the development of major biodiversity assessments in the Strait of Gibraltar. This, combined with the high propagation capability of the species (Altamirano-Jeschke et al., 2017 and Altamirano et al., 2019), makes the dispersion to habitats susceptible to be invaded, an alarming phenomenon that needs to be managed. So far, the life history of R. okamurae remains uncertain in the Mediterranean and Atlantic waters, more change the future of this bioinvasion represents. The spatial establishment capability observed in this work enhances the performance of observational and experimental investigations including environmental factors which influence patterns obtained. This could help to fully understand those elements which favor the establishment success of the species on lit and semi-lit rocky substrata and thus to lend further urgency for management actions focused on R. okamurae impacts.
Data Availability Statement
The original contributions presented in the study are included in the article/Supplementary Material, further inquiries can be directed to the corresponding author/s.
Author Contributions
JG-G: investigation, obtaining data in situ, data analysis, supervision, validation, funding acquisition, and project administration. MF: investigation, data analysis, and data curation. JG-G and MF cowrote the manuscript. All the authors contributed substantially to revisions. LO-P: investigation, obtaining of data in situ, and data curation. JS-V: investigation. CM: investigation and supervision.
Funding
This study was funded by CEPSA Foundation and Red Eléctrica de España (REE). Additional financial support was provided by the Organization of Fish Producers of Almadrabas (OPP-51), ENDESA, ACERINOX, and Diputación Provincial de Cádiz. This project has also benefited from scientific equipment and infrastructures financed by the Autoridad Portuaria (AP) of Seville and the Aquarium of Seville.
Conflict of Interest
The authors declare that the research was conducted in the absence of any commercial or financial relationships that could be construed as a potential conflict of interest.
Acknowledgments
We are deeply grateful to the city of La Línea, particularly to the logistical support provided by Puerto La Alcaidesa, the Yacht Club (RCNLL), and the Maritime Club, as well as by the town halls of La Línea and Tarifa. Special thanks are given to Darren Fa and Stephen for facilitating information from Gibraltar shores. Thanks are also given to Campo de Gibraltar (La Linea) and to the CIES (Algeciras) diving clubs. Finally, we express our gratitude to Antonio Vegara, Juanjo Quintero, Salvador Magariño, Juan Antonio Parada, José Fosela, Isabel Cárdenas, Candela Sánchez, and José Cantera for their continued collaboration.
Supplementary Material
The Supplementary Material for this article can be found online at: https://www.frontiersin.org/articles/10.3389/fevo.2021.631754/full#supplementary-material
References
Agatsuma, Y., Kuwahara, Y., and Taniguchi, K. (2005). Life cycle of Dilophus okamurae (Phaeophyceae) and its associated invertebrate fauna in Onagawa Bay, Japan. Fish. Sci. 71, 1107–1114. doi: 10.1111/j.1444-2906.2005.01070.x
Alomar, C., Deudero, S., Andaloro, F., Castriota, L., Consoli, P., Falautano, M., et al. (2016). Caulerpa cylindracea Sonder invasion modifies trophic niche in infralittoral rocky benthic community. Mar. Environ. Res. 120, 86–92. doi: 10.1016/j.marenvres.2016.07.010
Altamirano, M., De la Rosa, J., Carmona, R., Zanolla, M., and Muñoz, A. R. (2019). Macroalgas invasoras en las costas andaluzas. Algas 55e, 10–13.
Altamirano-Jeschke, M., De la Rosa, J., and Martínez, J. (2016). Arribazones de la especie exótica Rugulopteryx okamurae (E.Y. Dawson) I.K. Hwang, W.J. Lee & H.S. Kim (Dictyotales, Ochrophyta) en el Estrecho de Gibraltar: primera cita para el Atlántico y España. Algas 52:20.
Altamirano-Jeschke, M., De la Rosa Álamos, J., Martínez, F. J. G., and Muñoz, A. R. G. (2017). Prolifera en el Estrecho un alga nunca citada en nuestro litoral de origen asiático “Rugulopteryx okamurae” ocupa ya una gran extensión. Quercus 364, 32–33.
Andreakis, N., Procaccini, G., and Kooistra, W. H. (2004). Asparagopsis taxiformis and Asparagopsis armata (Bonnemaisoniales, Rhodophyta): genetic and morphological identification of Mediterranean populations. Eur. J. Phycol. 39, 273–283. doi: 10.1080/0967026042000236436
Bax, N., Williamson, A., Agüero, M., Gonzalez, E., and Geeves, W. (2003). Marine invasive alien species: a threat to global biodiversity. Mar. Policy 27, 313–323. doi: 10.1016/S0308-597X(03)00041-1
Boudouresque, C. F., and Verlaque, M. (2002). Biological pollution in the Mediterranean Sea: invasive versus introduced macrophytes. Mar. Poll. Bull. 44, 32–38. doi: 10.1016/S0025-326X(01)00150-3
Buschbaum, C., Chapman, A. S., and Saier, B. (2006). How an introduced seaweed can affect epibiota diversity in different coastal systems. Mar. Biol. 148, 743–754. doi: 10.1007/s00227-005-0128-9
Ceccherelli, G., Piazzi, L., and Balata, D. (2002). Spread of introduced Caulerpa species in macroalgal habitats. J. Exp. Mar. Biol. Ecol. 280, 1–11. doi: 10.1016/S0022-0981(02)00336-2
Checoli-Mantelatto, M., Alves-Póvoa, A., Felipe-Skinner, L., Vieira de Araujo, F., and Christopher-Creed, J. (2020). Marine litter and wood debris as habitat and vector for the range expansion of invasive corals (Tubastraea spp.). Mar. Pollut. Bull. 160:111659. doi: 10.1016/j.marpolbul.2020.111659
Coll, M., Piroddi, C., Steenbeek, J., Kaschner, K., Lasram, F. B. R., Aguzzi, J., et al. (2010). The biodiversity of the mediterranean sea: estimates, patterns, and threats. PLoS One 5:e11842. doi: 10.1371/journal.pone.0011842
De Paula, J. C., Vallim, M. A., and Teixeira, V. L. (2011). What are and where are the bioactive terpenoids metabolites from Dictyotaceae (Phaeophyceae). Rev. Bras. Farmacogn. 21, 216–228. doi: 10.1590/S0102-695X2011005000079
Ekman, S. (1953). Zoogeography of the Sea. London: Sidgwick and Jackson, doi: 10.1080/00222935308654417
El Aamri, F., Idhalla, M., and Tamsouri, M. N. (2018). Occurrence of the invasive brown seaweed Rugulopteryx okamurae (EY Dawson) IK Hwang, WJ Lee & HS Kim (Dictyotales, Phaeophyta) in Morocco (Mediterranean Sea). MedFAR 1, 92–96.
Figueroa, F. L., Vega, J., Gómez-Valderrama, M., Korbee, N., Mercado, J. M., Bañares, E., et al. (2020). Invasión de la especie exótica Rugulopteryx okamurae en Andalucía I: estudios preliminares de la actividad fotosintética. Algas 56:35.
Galil, B. S., Marchini, A., and Occhipinti-Ambrogi, A. (2018). East is east and West is west? Management of marine bioinvasions in the Mediterranean Sea. Estuar. Coast. Shelf. Sci. 201, 7–16. doi: 10.1016/j.ecss.2015.12.021
García-Gómez, J. C., Sempere-Valverde, J., González, A. R., Martínez-Chacón, M., Olaya-Ponzone, L., Sánchez-Moyano, E., et al. (2020). From exotic to invasive in record time: the extreme impact of Rugulopteryx okamurae (Dictyotales, Ochrophyta) in the strait of Gibraltar. Sci. Total Environ. 704:135408. doi: 10.1016/j.scitotenv.2019.135408
García-Gómez, J. C., Sempere-Valverde, J., Ostalé-Valriberas, E., Martínez, M., Olaya-Ponzone, L., González, A. R., et al. (2018). Rugulopteryx okamurae (E.Y. Dawson) I.K. Hwang, W.J. Lee & H.S. Kim (Dictyotales, Ochrophyta), alga exótica “explosiva” en el estrecho de Gibraltar. Observaciones preliminares de su distribución e impacto. Almoraima 48, 97–113.
Glasby, T. M., Creese, R. G., and Gibson, P. T. (2005a). Experimental use of salt to control the invasive marine alga Caulerpa taxifolia in New South Wales, Australia. Biol. Conserv. 122, 573–580.
Glasby, T. M., Gibson, P. T., and Kay, S. (2005b). Tolerance of the invasive marine alga Caulerpa taxifolia to burial by sediment. Aquat. Bot. 82, 71–81.
Hwang, I.-K., Lee, W. J., Kim, H. S., and De Clerck, O. (2009). Taxonomic reappraisal of Dilophus okamurae (Dictyotales, Phaeophyta) from the western Pacific Ocean. Phycologia 48, 1–12. doi: 10.2216/07-68.1
Hwang, Z. G. (1994). Marine Species and Their Distributions in China’s Seas, Vol. 48. Beijing: China Ocean Press, 1–12. doi: 10.2216/07-68.1
Kajimura, M. (1992). Vegetative propagation in Dilophus okamurae and Zonaria flabellata (Dictyotaceae, Phaeophyta). Mem. Fac. Sci. Shimane Univ. 26, 96–106.
Kennelly, S. (1989). Effects of kelp canopies on understorey species due to shade and scour. Mar. Ecol. Prog. Ser. 50, 215–224. doi: 10.3354/meps050215
Kiessling, T., Gutow, L., and Thiel, M. (2015). “Marine litter as habitat and dispersal vector,” in Marine Anthropogenic Litter, eds M. Bergmann, L. Gutow, and M. Klages, (Springer: Cham), doi: 10.1007/978-3-319-16510-3_6
Kirby, D. S., and Fiksen, Ø, and Hart, P. J. (2000). A dynamic optimisation model for the behaviour of tunas at ocean fronts. Fish. Oceanogr. 9, 328–342. doi: 10.1046/j.1365-2419.2000.00144.x
Kurata, K., Suzuki, M., Shiraishi, K., and Taniguchi, K. (1988). Spatane-type diterpenes with biological activity from the brown alga Dilophus okamurae. Phytochemistry 27, 1321–1324. doi: 10.1016/0031-9422(88)80185-7
Levine, J. M., Adler, P. B., and Yelenik, S. G. (2004). A meta-analysis of biotic resistance to exotic plant invasions. Ecol. Lett. 7, 975–989. doi: 10.1111/j.1461-0248.2004.00657.x
Lodge, D. M., Williams, S., MacIsaac, H. J., Hayes, K. R., Leung, B., Reichard, S., et al. (2006). Biological invasions: recommendations for U.S. policy and management. Ecol. Appl. 16, 2035–2054.
Lord, J. P., Calini, J. M., and Whitlatch, R. B. (2015). Influence of seawater temperature and shipping on the spread and establishment of marine fouling species. Mar. Biol. 162, 2481–2492. doi: 10.1007/s00227-015-2737-2
Marks, L. M., Reed, D. C., and Holbrook, S. J. (2018). Life history traits of the invasive seaweed Sargassum horneri at Santa Catalina Island, California. Aquat. Invas. 13, 339–350. doi: 10.3391/AI.2018.13.3.03
McCook, L. J., Jompa, J., and Diaz-Pulido, G. (2001). Competition between corals and algae on coral reefs: a review of evidence and mechanisms. Coral Reefs 19, 400–417. doi: 10.1007/s003380000129
Moschella, P. S., Abbiati, M., Åberg, P., Airoldi, L., Anderson, J. M., Bacchiocchi, F., et al. (2005). Low-crested coastal defence structures as artificial habitats for marine life: using ecological criteria in design. Coast. Eng. 52, 1053–1071. doi: 10.1016/j.coastaleng.2005.09.014
Muñoz, A. R., Martín-Taboada, A., De la Rosa, J., Carmona, R., Zanolla, M., and Altamirano, M. (2019). La modelización de la distribución de especies como herramienta en la gestión de invasiones biológicas en el medio marino: el caso de Rugulopteryx okamurae (Dictyotaceae, Ochrophyta) en el Mediterráneo. Algas 55:37.
Navarro-Barranco, C., Muñoz-Gómez, B., Saiz, D., Ros, M., Guerra-García, J. M., Altamirano, M., et al. (2019). Can invasive habitat-forming species play the same role as native ones? The case of the exotic marine macroalga Rugulopteryx okamurae in the Strait of Gibraltar. Biol. Invas. 21, 3319–3334. doi: 10.1007/s10530-019-02049-y
Ninomiya, M., Hirohara, H., Onishi, J. I., and Kusumi, T. (1999). Chemical study and absolute configuration of a new marine secospatane from the brown alga Dilophus okamurae. J. Org. Chem. 64, 5436–5440. doi: 10.1021/jo9902190
Noè, S., Badalamenti, F., Bonaviri, C., Musco, L., Fernández, T. V., Vizzini, S., et al. (2018). Food selection of a generalist herbivore exposed to native and alien seaweeds. Mar. Pollut. Bull. 129, 469–473. doi: 10.1016/j.marpolbul.2017.10.015
Ocaña, O., Afonso-Carrillo, J., and Ballesteros, E. (2016). Massive proliferation of a dictyotalean species (Phaeophyceae, Ochrophyta) through the Strait of Gibraltar (Research note). Rev. Acad. Canar. Cienc. 28, 165–170.
Occhipinti-Ambrogi, A. (2007). Global change and marine communities: alien species and climate change. Mar. Poll. Bull. 55, 342–352. doi: 10.1016/j.marpolbul.2006.11.014
Occhipinti-Ambrogi, A., and Savini, D. (2003). Biological invasions as a component of global change in stressed marine ecosystems. Mar. Poll. Bull. 46, 542–551. doi: 10.1016/S0025-326X(02)00363-6
Oliveros, J. C. (2007). Venny. An Interactive Tool for Comparing Lists with Venn’s Diagrams. Available online at: https://bioinfogp.cnb.csic.es/tools/venny/index.html (accessed October 26, 2020).
Olyarnik, S. N., Bracken, M. E. S., Byrnes, J. E., Hughes, A. R., Hultgren, K. M., and Stachowicz, J. J. (2009). “Ecological factors affecting community invasibility,” in Biological Invasions in Marine Ecosystems. Ecological, Management, and Geographic Perspectives, eds G. Rilov and J. A. Crooks, (New York, NY: Springer), 215.
Papacostas, K. J., Rielly-Carroll, E. W., Georgian, S. E., Long, D. J., Princiotta, S. D., Quattrini, A. M., et al. (2017). Biological mechanisms of marine invasions. Mar. Ecol. Prog. Ser. 565, 251–268. doi: 10.3354/meps12001
Pereira, R. C., and Da Gama, B. A. P. (2008). “Macroalgal chemical defenses and their roles in structuring tropical marine communities,” in Algal Chemical Ecology, ed. C. D. Amsler, (Berlin: Springer), 25–55.
Piazzi, L., and Ceccherelli, G. (2002). Effects of competition between two introduced Caulerpa. Mar. Ecol. Prog. Ser. 225, 189–195. doi: 10.3354/meps225189
Piazzi, L., Ceccherelli, G., and Cinelli, F. (2001). Threat to macroalgal diversity: Effects of the introduced green alga Caulerpa racemosa in the Mediterranean. Mar. Ecol. Prog. Ser. 210, 149–159. doi: 10.3354/meps210149
Rilov, G., and Galil, B. (2009). “Marine bioinvasions in the mediterranean sea – history, distribution and ecology,” in Biological Invasions in Marine Ecosystems. Ecological, Management, and Geographic Perspectives, eds G. Rilov and J. A. Crooks, (New York, NY: Springer), 549.
Rivera-Ingraham, G. A., García-Gómez, J. C., and Espinosa, F. (2010). Presence of Caulerpa racemosa (Forsskål) J. Agardh in Ceuta (Northern Africa, Gibraltar Area). Biol. Invasions. 12, 1465–1466. doi: 10.1007/s10530-009-9576-3
Ruitton, S., Javel, F., Culioli, J. M., Meinesz, A., Pergent, G., and Verlaque, M. (2005). First assessment of the Caulerpa racemose (Caulerpales, Chlorophyta) invasion along the French Mediterranean coast. Mar. Poll. Bull. 50, 1061–1068. doi: 10.1016/j.marpolbul.2005.04.009
Sempere-Valverde, J., Ostal, E., Maestre, M., and González, R. (2020). Impacts of the non-indigenous seaweed Rugulopteryx okamurae on a Mediterranean coralligenous community (Strait of Gibraltar): the role of long-term monitoring. Ecol. Indic. 121:107135. doi: 10.1016/j.ecolind.2020.107135
Simberloff, D., and Von-Holle, B. (1999). Positive interactions of non-indigenous species: invasional meltdown? Biol. Invas. 1, 21–32.
Tronholm, A., Leliaert, F., Sansón, M., Alfonso-Carrillo, J., Tyberghein, L., Verbruggen, H., et al. (2012). Contrasting geographical distributions as a result of thermal tolerance and long-distance dispersal in two allegedly widespread tropical brown algae. PLoS One 7:e30813. doi: 10.1371/journal.pone.0030813
Verlaque, M., Steen, F., and De Clerck, O. (2009). Rugulopteryx (Dictyotales, Phaeophyceae), a genus recently introduced to the Mediterranean. Phycologia 48, 536–542. doi: 10.2216/08-103.1
Vermeij, M. J. A., Dailer, M. L., and Smith, C. M. (2011). Crustose coralline algae can suppress macroalgal growth and recruitment on Hawaiian coral reefs. Mar. Ecol. Prog. Ser. 422, 1–7. doi: 10.3354/MEPS08964
Viard, F., and Comtet, T. (2015). “18. Applications of DNA-based methods for the study of biological invasions,” in Biological Invasions in Changing Ecosystems, ed. J. Canning-Clode, (Berlin: De Gruyter), 410–435. doi: 10.1515/9783110438666-025
Williams, S. L., and Smith, J. E. (2007). A global review of the distribution, taxonomy, and impacts of introduced seaweeds. Ann. Rev. Ecol. Evol. Syst. 38:36. doi: 10.1146/annurev.ecolsys.38.091206.095543
Keywords: Rugulopteryx okamurae, macroalgae, biological invasions, invasive macroalga, space colonization, Strait of Gibraltar
Citation: García-Gómez JC, Florido M, Olaya-Ponzone L, Sempere-Valverde J and Megina C (2021) The Invasive Macroalga Rugulopteryx okamurae: Substrata Plasticity and Spatial Colonization Pressure on Resident Macroalgae. Front. Ecol. Evol. 9:631754. doi: 10.3389/fevo.2021.631754
Received: 20 November 2020; Accepted: 09 April 2021;
Published: 08 June 2021.
Edited by:
Ana Sofia Vaz, University of Granada, SpainReviewed by:
Marcos Rubal, University of Porto, PortugalJoana Raquel Vicente, Centro de Investigacao em Biodiversidade e Recursos Geneticos (CIBIO-InBIO), Portugal
Copyright © 2021 García-Gómez, Florido, Olaya-Ponzone, Sempere-Valverde and Megina. This is an open-access article distributed under the terms of the Creative Commons Attribution License (CC BY). The use, distribution or reproduction in other forums is permitted, provided the original author(s) and the copyright owner(s) are credited and that the original publication in this journal is cited, in accordance with accepted academic practice. No use, distribution or reproduction is permitted which does not comply with these terms.
*Correspondence: Marta Florido, bWZsb3JpZG8uY0BnbWFpbC5jb20=
†These authors have contributed equally to this work