- 1Changqing Engineering Design Co., Ltd., Xi’an, China
- 2School of Geology Engineering and Geomatics, Chang’an University, Xi’an, China
In order to reveal the destructive mechanism of loess landslide induced by stagnant water on the combined surface, and to clarify the influence of the main control factors, this paper takes a typical loess landslide in northern Shaanxi as the research object, analyzes the structure of the rock and soil body, and the excavation and filling construction through the geohazard survey, and analyzes the process of traction sliding caused by the stagnant water on the combined surface at the different stages of the project by combining with the calculation of the stability of the slope body. Further the article analyses the process of traction sliding caused by water on the combined area due to construction by means of a discrete element model, and delves into the mechanism of strength reduction of saturated loess. The results show that: 1) the combined surface stagnant water type loess landslide has the characteristics of sudden sliding and rapid evolution, which is highly hazardous and difficult to prevent and control; 2) the slope destabilization is controlled by the engineering geological conditions, and the slope excavation changes the original mechanical equilibrium conditions of the slope, which provides the dynamic conditions for the traction sliding of the slope; 3) the change of the hydrogeological environment results in the obstruction of the natural drainage channel, which leads to the formation of continuous sliding surface due to stagnant water on the combined surface, and the formation of a continuous sliding surface due to stagnant water on the combined surface. Surface stagnant water to form a continuous slippery surface, inducing the overall destabilization of the slope damage; 4) loess strength index with the increase of saturation and the exponential function form of reduction, and when the saturation degree reaches more than 80%, the strength index of the soil body to reach the basic stability. The article expanding the ideas of landslide control and analysis, and the research results will provide a theoretical basis for the design of junction landslide management in the loess areas of northern Shaanxi.
1 Introduction
Northern Shaanxi is not only an important node of the “Belt and Road” strategy, but also an important energy reserve base in China. The region is geologically fragile and has a complex topography, which makes it a geohazard-prone area (Ministry of Natural Resources, 2022; Xu et al., 2015; Xu et al., 2019; Rui et al., 2023). With the gradual advancement of the Western Development Strategy, human engineering activities have increased, further exacerbating the risk of geologic hazards. The geological disaster census in 2016 showed that there were many loess slope geological disasters in Changqing oilfield field stations, and the cost of treatment was as high as 1.19 billion yuan, which was characterized by a large number, concentrated distribution, and difficult to control.
The Loess Plateau is an arid and semi-arid region, and loess bodies have good engineering properties in the unsaturated state, but are susceptible to wet subsidence when exposed to water, leading to a decrease in strength, which triggers landslides (Lu, 2019). During the study and monitoring of many macroscopic physical models of landslides (Fang et al., 2023; Fang et al., 2022; Fang et al., 2024), it can be found that, the difference in physical properties between loess and underlying strata may form a stagnant water layer, further inducing landslides. For the correlation between pore water and loess landslides, scholars at home and abroad have also carried out a lot of research (Rui et al., 2017; Maosheng and Tonglu, 2011). Min et al. (2021) proposed a model of landslide destabilization and damage under the condition of pore water pressure change rate, Leo-tao et al. (2023) studied the scientific setting of landslide warning threshold triggered by precipitation on Loess Plateau, and Shuaihua and Yilei (2018) found that rainfall infiltration in engineering practice will cause changes in the saturation and gravity of slope soil, and used finite element software to calculate the stability of slopes under the condition of rainfall infiltration. Existing studies are mostly concerned with the effect of rainfall infiltration on slope stability, while there are fewer studies on the micro-mechanism of landslides induced by surface stagnation and the effect of changes in the hydrogeological environment.
In addition, the study of loess strength characteristics considering the influence of pore water has a long history, and the existing research results mainly focus on the macroscopic strength characteristics of loess body under different water content and its influencing factors (Zhao et al., 2023) and also put forward the theory of shear strength of unsaturated soils represented by Bishop’s effective stress shear strength formula (Bishop, 1959) and Fredlund’s double-stress state-variable shear strength formula (Fredlund et al., 1978), this allows for loess strength index estimation at different levels of saturation to assess the impact of water on engineering design indexes. However, because the loess body is a structural system composed of a series of particles, pores fissures, etc., on the microscopic level, and its force deformation and damage process is very complex and the influencing factors are variable, the inhomogeneity, discrete nature, and force deformation and damage process of loess are difficult to be analyzed by the conventional continuum medium mechanics method (Xian-li et al., 2014; Gu et al., 2023; Liu et al., 2020). Some studies have focused on the effect of precipitation infiltration on slope stability (Muntohar and Liao, 2010; Huat et al., 2006; Liu et al., 2017), while there are fewer studies on the micro-mechanisms of landslides induced by combined surface’s stagnant water and the effect of changes in the hydrogeological environment. In addition, most of the studies are still in the stage of theoretical analysis, and the analysis of the saturated loess strength reduction mechanism is not very mature, and the research on the influence of stagnant water on the combined surface on the stability of the slope is also still relatively small. There is a lack of comparative analysis of examples and models, especially for landslide disasters in northern Shaanxi, and most of the studies only discuss the statistical relationship between geologic disasters and precipitation in the Loess Plateau region (Li et al., 2009; Shuaihua and Yilei, 2018) but lack the analysis of the mechanism of loess landslides induced by stagnant water on the combined surface.
Given this, this study takes a large loess landslide at an oil field station in the Yan’an area as a case study and uses the particle discrete element method to simulate the loess force deformation and damage process, to explore the effect of water on the mechanical characteristics of loess from a microscopic point of view, and to combine experimental and theoretical analyses to macroscopically validate the effect of the change in the strength of the loess on the evolution of the landslide. This study aims to provide theoretical support for landslide analysis and management design in the Loess Plateau region of northern Shaanxi.
2 Materials and methods
2.1 Primitive geological environment
The selected case study site has been active for a long time under the specific climatic and geological conditions of northern Shaanxi, and has a strong environmental adaptation, as well as representing the general climatic conditions of northern Shaanxi. According to the above criteria, the geological conditions and climatic characteristics of the study site are well representative and valuable, and can provide reference and guidance for landslide studies in northern Shaanxi and even under similar geological and climatic conditions. The specific original geological environment is as follows.
The studied oil field site is located in the southwest region of Yan’an City, which falls within a typical mid-temperate continental semi-arid monsoon climate. This climate features four distinct seasons, with mild temperatures, moderate rainfall, dry and windy springs, frequent thunderstorms in summer, and cool autumns, winters are characterized by cold temperatures and low precipitation, with severe short-term heavy precipitation events typically occurring in July and August. The site belongs to the tectonic low-middle mountainous terrain and Luohe River valley terrace terrain (refer to Figure 1). Located on the first-level terrace of the Luohe River, the site area has higher elevation in the northeast and lower elevation in the southwest, ranging from 1054.1 to 1088.3 m, with an overall slope of 5°–10°. The southwest side of the site area borders the Luo River channel, approximately 50 m away from the site area, with the site itself positioned about 20 m above the riverbed. Natural washes flank the east and west sides of the site area, while the north side is a loess slope, with the base of the slope directly adjacent to the planned site area. As depicted in Figure 1, the construction site’s morphology, along with the mountain backdrop, displays distinct characteristics. Natural gullies flank the slopes, creating a shape reminiscent of a lap chair, where the front is gently sloping and the rear is steep. The profile morphology resembles a “ladder,” while the planar morphology takes the shape of a tongue, indicative of a stable-state ancient landslide morphology.
The site is primarily covered by loess, with the stratigraphy being comprised of five layers arranged from top to bottom as follows: 1) Quaternary Holocene Residual Slope Deposits (Q4dl+el) silty clay, 2) Quaternary Holocene landslide accretion (Q4del) silty clay, 3) Quaternary Upper Pleistocene (Q3al+pl) silty clay and rounded gravel, 4) Quaternary Middle Pleistocene (Q2al+pl) silty clay, 5) Underlying Jurassic Zhiluo Formation (J2z) mudstone interbedded with thin sandstone.
The geological environment of the original site before the construction of the project was relatively stable, and there were no obvious adverse geological phenomena on the original slope. Through the geotechnical engineering investigation before the construction of the station, it was found that there was no obvious concentrated surface water system in the original site, and only temporary water flow appeared on the surface after rainfall, and the rainfall flowed with the inclination of topographic elevation difference, and the natural drainage conditions were better, and there was no groundwater within the depth of exploration at each exploration point during the investigation period.
2.2 Landslide morphology characterization
In July 2021, earthwork construction began due to the site construction needs. Following the construction, a nearly 10-m-high steep cut was formed at the foot of the slope, leading to the “resurrection” of ancient landslides, there were evident signs of deformation and destruction, with large areas of tensile cracks developing in the slope, eventually causing extensive collapse in some parts. The landslide occurred suddenly and evolved rapidly. The deformation and damage pattern of the slope after excavation is shown in Figure 2. According to the on-site investigation, the landslide is about 250 m long from east to west and 240 m long from north to south, and is in the shape of multi-level platform and steep canyon.
As shown in Figure 2, due to the construction of the section of the front edge of the landslide excavation, clearing the ancient landslide leading edge of the slip blocking section of the slide, resulting in the stabilization of the ancient landslide re-sliding, traction landslide deformation and destruction of the signs of obvious, only in the southwest side of the front edge of the slope local area due to the influence of the main body of the landslide pushed, the formation of a small secondary transfer type of landslides, and the formation of ground bulge bulging in the front of the edge of the slope. The entire landslide is generally a “chair” shape, and the perimeter is obvious. The front edge of the slide shear outlet in the artificial excavation surface has continuous distribution, and shows anti-dipping out of the wrong, the back edge of the tension cracking, and the formation of steep cans. The surrounding slip surface is smooth, and the scuff marks are visible.
2.3 Deep displacement monitoring
After the slope is destabilised, the study uses borehole inclinometers to monitor and warn of landslide deformation. Borehole inclinometers are inclinometer tubes with guide channels installed in landslide boreholes, and the deformation of the guide channels is observed to respond to the deformation of the deeper part of the landslide, and at present, there are movable and fixed borehole inclinometers, and four movable inclinometer observation holes are set up for the monitoring of the deeper part of the deformation in this programme.
The location of the deep displacement measurement holes is shown in Figure 2. Representative monitoring results are shown in Figure 3. The deep displacement monitoring about half a year after the landslide occurred (February 2022) shows that the landslide body still has obvious and continuous deformation, and the destabilization damage potential of the slope body is still serious. As can be seen from the figure, the cumulative lateral horizontal displacement curve along the depth change in the measured hole, the lateral displacement at 14.5 m below the ground surface of the lateral displacement occurs a large sudden change, the cumulative lateral horizontal displacement up to 5 mm, the lateral displacement below the place is small, above the displacement is larger, and the maximum cumulative lateral horizontal displacement within 10 days is as high as 16 mm, which indicates that the soil in the location near the combined surface has formed a more obvious sliding surface, which is in line with the engineering geological stratigraphy revealed by the engineering geology.
The deep displacement data in Figure 3 verifies that the landslide surface is located near the soil-rock combined surface, which is consistent with the location of the exposed sliding shear surface around the slope found in the field investigation shown in Figure 2, indicating that the landslide is a traction soil-rock combined surface landslide induced by engineering excavation at the foot of the slope, which is commonly found in the loess area of northern Shaanxi.
2.4 Numerical simulation analysis
Numerical simulation has been widely used as a powerful tool in geotechnical slope engineering research for stability analysis, design and disaster prevention. Numerical simulation allows researchers to vary different parameters and conditions in order to study their effects on slope stability, which is difficult to do in the real world. Numerical simulation can save a great deal of time and cost compared to traditional physical model testing, as it reduces the need for physical models and the reliance on field tests.
However, numerical simulation has some limitations, including high demand for computational resources, uncertainty in model parameters, and dependence on model accuracy. In addition, the interpretation of numerical simulation results requires expertise to ensure the reliability and validity of the simulation results.
Overall, the application of numerical simulation in geotechnical slope engineering provides a powerful platform for research, design and disaster management, although it requires accurate model and parameter inputs as well as careful analysis and validation of results.
3 Results
3.1 Stability analysis
Based on the mechanical mechanism, this study conducted an in-depth analysis of the causes of landslide deformation based on the Design Code for Landslide Prevention and Control (GB/T 38509-2020) (Ministry of Natural Resources.GB/T 38509-2020, 2020) and executed a stability calculation of the landslide body. Using data from the geotechnical investigation report before July 2021, a computational profile model was established based on the test results of 112 in-situ soil samples (see Figure 4). The landslide control project was designated as Class I. Natural working conditions were simulated, and the unbalanced thrust transfer method was used to assess the stability and calculate the thrust (see blue line in Figure 4).
The calculation shows that the stability coefficient of the original slope is 1.55, which meets the stability standard and is consistent with the actual stable state of the slope before construction. However, the excavation caused by the construction of the field station resulted in the stability coefficient dropping to 1.06, and according to the code, the present slope body is in an unstable state and does not meet the safety requirements. In addition, the analysis of the residual sliding force after excavation showed that the actual excavation line was at the critical position where the residual sliding force changed from positive to negative, which was consistent with the stability coefficient critical value near 1.0, indicating that the slope body was close to the critical state of damage after excavation. Further analysis indicates that tensile and shear stresses may be concentrated inside the slope body after excavation, which may lead to the penetration of the potential shear outlet along the maximum shear stress surface, and then trigger the overall instability damage under certain conditions.
As mentioned before, the excavation of the foot of the slope during the construction of the station was the main factor that caused the ancient landslide to retraction slide. However, as shown in Figures 2, 3, the actual deformation and damage state of the landslide, as well as its continuous deformation process, indicate that the true stability of the slope should be an unstable state, so only considering the effect of the foot of the slope excavation has not been able to fully explain the occurrence of the landslide and its scale.
As shown in Figure 5, the field engineering investigation in February 2022 found that there was a saturated soil belt about 1–2 m high in the upper loess layer of the shear exit at the leading edge of the landslide, which was continuously exposed and distributed along the excavation of the foot of the slope. The water content of the soil is large, the soil is almost saturated, and it is a soft-plastic to fluid-plastic state. From the drilling process of the deep displacement borehole in the middle of the slope shown in Figure 3, it can also be found that the soil samples taken near the soil-rock combined surface are also saturated (Figure 4), which indicates that near the soil-rock combined surface of the landslide body, the water content of the soil is abnormal, and there is a saturated soil zone. Therefore, we should consider the possibility of landslides induced by the combined effect of stagnant water on the combined surface and excavation at the foot of the slope after the construction of the project.
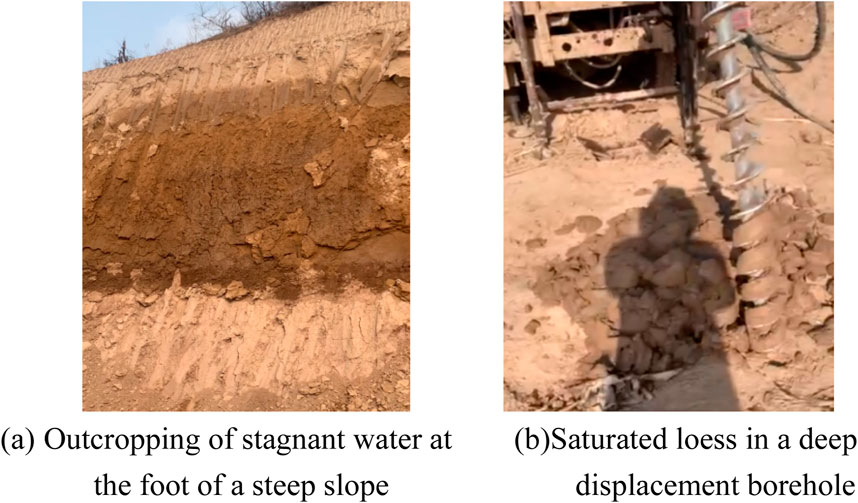
Figure 5. Water stagnation of soil-rock interface (A) Outcropping of stagnant water at the foot of a steep slope (B) Saturated loess in a deep displacement borehole.
3.2 The phenomenon of stagnant water on the combined surface
As can be seen in Figure 4, the stability coefficient of the current slope after excavation is near 1, which is close to the critical state of sliding damage, and the stability of the slope is evaluated as unstable. However, as shown in Figures 2, 3, the actual deformation and damage state of the landslide, and its continuous deformation process, indicate that the real stability of the slope body should be unstable, so only considering the effect of excavation at the foot of the slope cannot fully explain the occurrence of the landslide and its scale.
In February 2022, the soil strength indexes obtained from the consolidation fast shear test of the original soil samples taken from the upper part of the soil-rock combination surface are shown in Figure 4 as the water stagnation state (red curve). From the test results in February 2022, the saturated soil strength index compared to the original design in July 2021 has decreased significantly, this strength index for the stability calculation is shown in Figure 5, with the slope stability coefficient of Fs = 0.67, the slope is in an unstable state, in line with the actual situation of landslides.
Among the factors inducing landslides, poor engineering geological conditions and structural conditions of the geotechnical body are the main internal causes, and the role of water, and human engineering activities are the main external causes (Lu, 2019). From the above analysis, it can be seen that the slope was excavated at the foot of the slope, the slip-resisting section was removed, the slip-resisting force was reduced, and the geotechnical body thrust continued to act, which provided dynamic conditions for the formation of landslides; the engineering construction process changed the original hydrogeological environment of the slope, resulting in stagnant water on the combined surface, which provided material conditions for the formation of landslides.
3.3 Reasons for water stagnation on the combined surface
As shown in Figure 5, after the construction of the project, the original geological environment was altered. There was stagnant water on the upper part of the soil-rock binding surface of the landslide body, which led to the saturation of the soil near the sliding surface and a significant reduction in its strength. This, coupled with the excavation at the foot of the slope, ultimately led to the formation of the landslide. However, a geotechnical engineering investigation conducted before the construction of the station in July 2021 found no obvious concentrated surface water system at the original site. Only temporary water flow appeared on the surface after rainfall, which flowed according to the inclination of the terrain elevation difference. The natural drainage conditions were favorable, and no groundwater was detected within the depth of exploration at each exploration point during the investigation period. Therefore, from a historical perspective, the possibility of the emergence of a long-term stagnant water layer on the slope, as shown in Figure 5, is extremely low. Moreover, the construction time of the station was in the fall and winter, during the dry season in northern Shaanxi. During this period, there was no significant record of precipitation in the field area, and no conditions existed for stagnant water in the upper layer of the slope formed by continuous infiltration of heavy rainfall.
After excluding the possibility of rainwater infiltration and accumulation forming the upper layer of stagnant water in the slide, the reason for stagnant water on the combined surface can be traced to changes in the hydrogeological environment of the site. As depicted in Figure 6, during earthmoving operations in the site area, a substantial amount of earth was deposited in the natural gully on the side of the slope body, creating a large-scale gully filling site and a high filling slope adjacent to the landslide body. While the filling project serves to expand industrial or civil land significantly, it may also alter the hydrogeological conditions within the site area. As shown in Figure 6, on the other side of the natural side slopes within the gully that had not yet been covered by fill soil, there was significant bedrock fissure water outflow within the laminated mudstone or sandstone.
The natural gullies on both sides of the landslide body serve as natural channels for surface water to converge and flow into the Luo River after rainfall, and they are also the primary pathways for groundwater discharge. Under natural conditions, groundwater from the slope body, including water from bedrock fissures, primarily exits through these natural gullies surrounding the slope body. Gully after large-scale filling project, due to the filling soil is mainly clayey silt or clayey soil, after filling compaction of low permeability, hindering the original groundwater drainage channel, resulting in bedrock fissure water accumulation, in the upper loess capillary phenomenon and other roles, seepage into the upper part of the soil layer on the combined surface, the formation of the combined surface stagnant layer shown in Figure 5.
4 Discussion
4.1 Strength theory
The notion of effective stress (Yong-qiang et al., 2024) describes how water affects soil strength as follows:
where σ represents total normal stress, σ’ represents effective stress, and uw represents pore water pressure.
From Equation 1, it can be seen that under the condition of constant total stress σ, with the increase of pore water pressure uw, the effective stress σ’, which represents the strength index of the soil body, will be relatively reduced. In practical engineering, with the groundwater drainage blocked, after the stagnant water in the combined surface occurs, the water in the infiltrated soil body increases, the water content w of the soil body rises, the saturation degree Sr of the soil body increases, and the pore water pressure uw increases accordingly, which usually results in the decrease of the strength index of the soil body.
Although the effective stress principle explains the qualitative result that the increase of loess saturation will lead to a decrease in soil strength (Ping et al., 2013; Mao-hua et al., 2006), it is difficult to determine its mathematical function quantitatively and further explain its correlation. The more mature Fredlund two-stress state variable shear strength formula is used to estimate the strength index of loess under different degrees of saturation. The loess in the model is considered in an unsaturated state, and the formula is shown as follows:
where c’ and φ’ denote the effective stress intensity parameters in the conventional sense; ua is the pore gas pressure; and φb is the inclination of the curve of shear strength increase caused by the increase of matrix suction, which is also a function of matrix suction.
From Equation 2, it can be seen that although the unsaturated soil strength theory tries to establish a quantitative mathematical functional relationship between the strength index τf and the pore water pressure uw, it is still unable to establish a direct relationship between the strength index τf and the water content w or saturation degree Sr. On the other hand, Equation 2 introduces indicators such as φb related to matrix suction, which reflects the influence of water content w or saturation degree Sr from the side. However, it is still difficult to accurately obtain these Equation 2 indicators in engineering. The soils in different regions are extremely different, so the universality of these indicators still needs to be improved.
4.2 Discrete element model
Conventional geotechnical test methods can obtain the geotechnical body’s macroscopic mechanical properties and deformation characteristics. Still, observing the changes in the soil particles and pores is difficult, so it is impossible to explore the deformation mechanism from a fine view. On the microscopic level, the loess body is a structural system composed of a series of particles, pores and fissures, etc., and its force deformation and damage process is very complicated while the influencing factors are variable, so the inhomogeneity, discretization, and force deformation and damage process of loess are difficult to be analyzed by the conventional continuous medium mechanics method.
DEM, on the other hand, is a flexible simulation tool with high adaptability for a wide range of particle system studies and engineering applications. It is able to simulate particle motions and interactions from microscopic to macroscopic scales, and is particularly good at dealing with complex dynamics such as particle flow and collapse. It directly simulates particle contact forces without the need for complex assumptions, which improves accuracy, and the DEM is adaptable to particles of different shapes and materials, and is able to deal with dynamic boundary conditions, which are often consistent with experiments and validate the reliability of the model. Its models can be scaled to large-scale simulations, and although it requires high-performance computational resources, it provides intuitive visualisations to help understand the complexity of granular systems.
In the discrete element method, the geotechnical body model is constructed by stacking and cementing a series of particles with specific mechanical properties. In the most basic linear-elastic model, the particles are assumed to rely on springs to contact each other and generate forces. Based on classical Newtonian mechanics, the particles’ acceleration, velocity, and displacement are calculated by a time-step iterative algorithm. In turn, the simulation of deformation and damage processes of macroscopic rocks and soils is realized (Gen-long et al., 2011; Li-nan et al., 2017). To analyze the strength folding mechanism of saturated loess from the mechanical mechanism, this paper analyzes the interaction between pore water and loess in the consolidation fast shear test process using MatDEM, a three-dimensional discrete element software prepared by MatLab.
The numerical simulation is carried out in strict accordance with the Standard for Geotechnical Test Methods (GB/T 50123-2019) (Ministry of Housing and Urban-Rural Development of the People’s Republic of China. GB/T 50123-2019, 2019). The discrete element model is shown in Figure 7. The height of the specimen is 2 cm, the radius is 3.09 cm, the particle radius is within 0.01 cm, the number of compactions is 2 times, and the gravity is reduced to 1/10 to eliminate the prestressing force; after the stacking model is established, the shear box is set up, the thickness of the edge of the shear box is 0.5 cm, and the distance between the box units is set to 70% of the radius. To make the specimen model study meet the unsaturated soil mechanical analysis, to obtain soil samples with different degrees of saturation at the macroscopic level, based on the matrix suction experiments of typical loess in Northwest China to obtain the function relationship between the water content of loess in the natural state and matrix suction (Wang and Tang, 2016; Ming-Jing et al., 2019), and to adjust the physical parameters under the corresponding particles to make quantitative changes to set up the effect of different degrees of saturation on the soil body structure. The model building and consolidation fast shear process includes original model stacking, shear box modeling, and shear completion. The data units in Figure 7 are all standard.
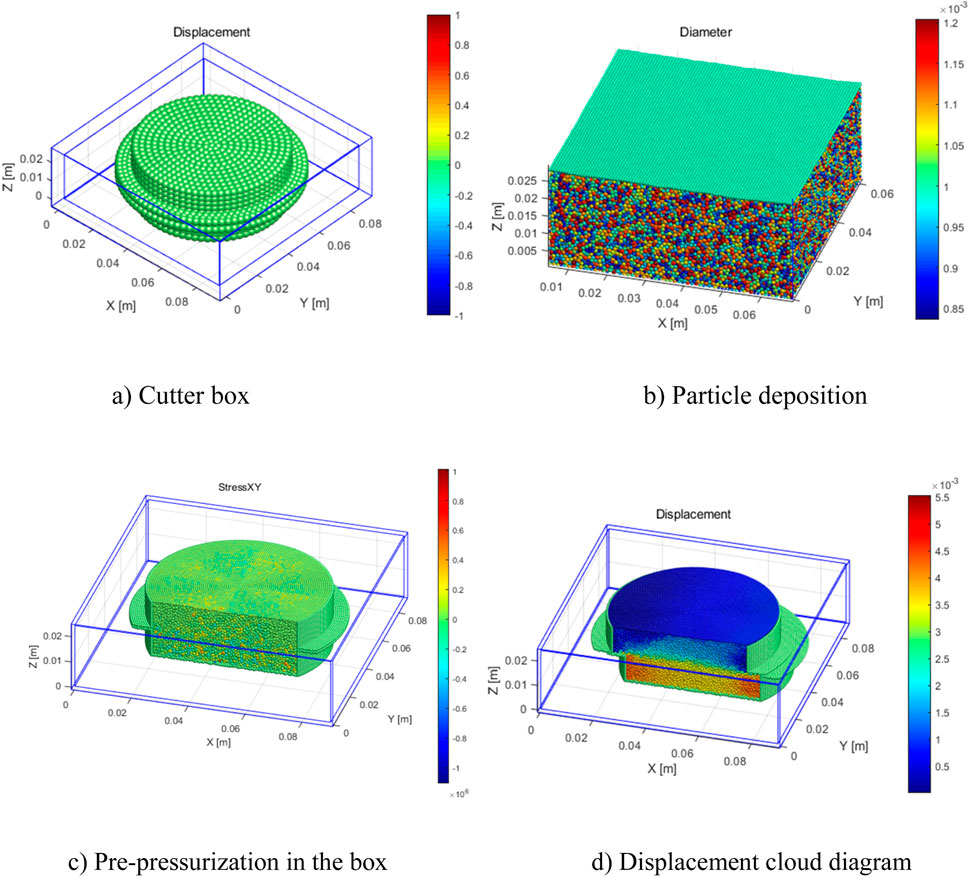
Figure 7. Discrete element simulation (A) Cutter box (B) Particle deposition (C) Pre-pressurization in the box (D) Displacement cloud diagram.
4.3 Analysis of soil strength
Due to computational resource constraints, simplifying the model may reduce the number of particles or change the scale of the model, which may affect the simulation of the macroscopic behaviour of the material. And the model may use homogeneous material properties, whereas the actual material may be inhomogeneous or anisotropic. Therefore the results of simplified models need to be validated by experimental data to ensure their validity and applicability. In this paper, laboratory consolidation fast shear results are used as a control.
Through the discrete element model shown in Figure 7, the simulation results of consolidation fast shear of loess with different degrees of saturation shown in Figure 8 can be obtained, which are basically consistent with the results of consolidation fast shear in the test laboratory.
As shown in Figure 8, the soil body’s cohesiveness decrease in the shape of an exponential function as the saturation degree increases, and the soil body’s strength index reaches fundamental stabilization when the saturation degree exceeds 80%. In the actual project, the impact of water stagnation on the combined surface of the slope body would enhance the soil body’s saturation level. According to the modeling results, this rise will result in a drop in the soil body’s strength index, which would eventually cause macro-landslides. Compared with the traditional strength theory, as shown in Equations 1, 2, the discrete element model not only quantitatively explains the result of the decrease of soil strength due to the increase of loess saturation but also inverts the evolution of soil deformation and damage from a fine view, and explores the interaction characteristics between the particles and its deformation and damage mechanism, which is of universal significance in related research.
When analysed in conjunction with the interrelationship between water and particles, it can be assumed that water infiltration alters the interactions between soil particles, leading to a decrease in cohesion. Secondly, as water increases, soil particles may undergo rearrangement, which can alter the microstructure of the soil and consequently affect its macroscopic mechanical properties. In addition, an increase in soil saturation alters pore water pressure, which reduces the shear strength of the soil. Finally, under waterlogged conditions, the water pressure in the soil pore space increases, which can further affect the soil’s carrying capacity. Together, these factors lead to a decrease in the overall stability of the soil under waterlogged conditions.
4.4 Evolution characteristics of combined surface’s stagnant water type landslide
The use of FLAC3D in stability analysis requires high requirements for the establishment of complex models and the assignment of parameters, while the accuracy of the computational results is highly dependent on the accuracy of the models and the reasonableness of the parameters, which needs to be supported by field investigations and experimental data. However, its explicit finite difference method gives FLAC3D a certain advantage in computational efficiency, which is especially suitable for the simulation of dynamic problems and large deformation problems.
To analyze the landslide initiation mechanism and evolution process from the mechanical mechanism, based on the above analysis, the FLAC3D computational model was established after the excavation of the foot of the slope, as shown in Figure 9A. The simulation results found that the resistance of the leading edge of the slope body was relatively reduced after excavation, and local tensile stress and shear stress concentration phenomena appeared in the interior of the slope body due to traction sliding. As shown in the shear strain cloud diagram in Figure 9B, with the development of water stagnation in the bond surface, under this shear-tension stress environment, the maximum shear stress surface gradually forms a potential shear outlet along the bond surface, which will produce overall instability damage under certain conditions.
Adopting the strength index of soil body under different saturation degrees shown in Figure 8 as the calculation parameter, the stability safety coefficient of slope body under different working conditions shown in Figure 10 is calculated by strength discount method, which is basically the same as the specification calculation results shown in Figure 4. From the simulation results, after the excavation of the foot of the slope, there is no stagnant water on the combined surface, and the stability coefficient of the slope body is slightly larger than 1, which is close to the critical sliding state. With the beginning of the filling project, the original groundwater drainage environment of the site is changed. The water content of the soil near the combined surface begins to rise gradually. The saturation degree increases, resulting in the slope stability coefficient dropping rapidly to the critical state point of 1, and the slope body appears along the combined surface with a shear rupture surface. With the continuous development of the stagnant water on the combined surface, the combined surface forms a through-slip surface, the stability coefficient of the slope body continues to drop rapidly, and the slope body destroys rapidly. By analyzing the results of actual investigation and numerical modeling, it is found that the numerical simulation of FLAC3D can show the effect of water stagnation on the combined surface in a more graphic way, and confirms the mechanism of water stagnation on the combined surface inducing the damage of loess landslides.
The formation of a landslide by stagnant water on the combined surface is a specific type of landslide that involves the reduction of the shear strength of the bond surface on a slope (usually a weak rock or soil layer) due to the action of stagnant water, ultimately leading to the occurrence of a landslide. The formation mechanism of this type of landslide is unique compared to other landslide formation mechanisms. The main triggering factor of a combined surface stagnant water landslide is stagnant water, i.e., water that accumulates in one of the weak layers or contact surfaces of the slope, increasing the pore water pressure and reducing the shear strength of the material. It is not necessary to have a well-defined weak layer in the geological structure of other types of landslides. Also the sliding surface of combined surface stagnant water landslides usually develops along a pre-existing weak layer or contact surface. The sliding surfaces of other landslides may be new rupture surfaces or develop along structural surfaces such as joints and laminations in the rock. This also demonstrates the critical role of water in bonded surface stagnant landslides, as it directly affects the stability of the weak layer. Whereas in other types of landslides, it may be a factor, but not the only or main factor.
4.5 Prevention and control of combined surface’s stagnant water type landslide
The main points of prevention and control of landslides formed by stagnant water on combined surfaces focus on controlling and reducing the negative impact of stagnant water on slope stability. Areas with the potential to cause such landslides should be investigated in advance in detail for the hydrogeological conditions of the slopes, including groundwater levels, direction of flow, rate of flow, and association with the landslide. Assess the impact of stagnant water on slope stability and identify critical drainage areas. Based on this, the weak layers or contact surfaces can be reinforced by using grouting, shotcrete or setting anchors to increase their shear strength. In addition topographic modifications can be made to increase drainage paths on slopes and reduce the accumulation of surface and rainwater on slopes. Drainage trenches, drainage holes and underground drainage pipes can also be installed to facilitate rapid removal of water from slopes. For the setting up of a monitoring system, monitoring equipment, such as displacement gauges, pressure gauges and water level gauges, should be installed to monitor in real time the deformation of slopes and changes in water pressure, especially the degree of soil saturation in the weak layer or contact surface. On this basis, an early warning system should be established so that emergency measures can be taken immediately once abnormal data are monitored.
5 Conclusion
(1) Poor engineering geological conditions and structural conditions of the geotechnical body are the main internal causes of landslides. In contrast, the role of water, human engineering activities, and so on are the main external causes. The combined surface stagnation effect caused by several factors induces the mechanism of landslide damage, which is included in engineering safety considerations.
(2) In order to avoid additional impacts of human activities on the formation of landslides, attention should be paid to the drainage design when carrying out large-scale filling projects, so as to avoid blocking the natural drainage paths and changing the hydrogeological environment.
(3) In the simulation study, the strength index of loess decreases in the form of an exponential function with the increase of saturation, and it reaches basic stability when the saturation reaches more than 80%.
(4) Loess landslides of the combined surface stagnant water type are characterized by their rapid evolution, high hazard, and difficulty in prevention and control. Consequently, it is imperative that we engage in geotechnical investigation work, accurately predict the potential hazards of disasters, and investigate the deformation and destruction mechanisms to guarantee the safety of the geological environment.
Data availability statement
The raw data supporting the conclusions of this article will be made available by the authors, without undue reservation.
Author contributions
DH: Methodology, Writing–original draft. FZ: Investigation, Writing–review and editing, Conceptualization. JD: Software, Writing–review and editing, Visualization. HW: Writing–review and editing, Data curation. RX: Project administration, Validation, Investigation, Writing–review and editing.
Funding
The author(s) declare that financial support was received for the research, authorship, and/or publication of this article. National Natural Science Foundation of China funded project (No. 41572261); Changqing Oilfield Loess Slope Management Design Key Technology Research Project (No. 2023DJ0701); Project of Science and Technology Research and Development Fund of Mechanical Industry Survey and Design Research Institute Limited (No. 11740903120220021); 2024 Key Science and Technology Project of Northwest Survey and Design Research Institute of China Electric Construction Group (No. XBY-KJ-2024-55).
Conflict of interest
Authors DH and FZ were employed by Changqing Engineering Design Co., Ltd.
The remaining authors declare that the research was conducted in the absence of any commercial or financial relationships that could be construed as a potential conflict of interest.
Publisher’s note
All claims expressed in this article are solely those of the authors and do not necessarily represent those of their affiliated organizations, or those of the publisher, the editors and the reviewers. Any product that may be evaluated in this article, or claim that may be made by its manufacturer, is not guaranteed or endorsed by the publisher.
References
Fang, K., Miao, M., Tang, H., Jia, S., Dong, A., Pengju, A., et al. (2022). Insights into the deformation and failure characteristic of a slope due to excavation through multi-field monitoring: a model test. Acta Geotech. 18 (2), 1001–1024. doi:10.1007/s11440-022-01627-0
Fang, K., Tang, H., Changdong, L., Su, X., Pengju, A., and Sun, S. (2023). Centrifuge modelling of landslides and landslide hazard mitigation: a review. Geosci. Front. 1. doi:10.1016/j.gsf.2022.101493
Fang, K., Dong, A, Tang, H., An, P., Wang, Q., Jia, S., et al. (2024). Development of an easy-assembly and low-cost multismartphone photogrammetric monitoring system for rock slope hazards. Int. J. Rock Mech. Min. Science 174, 105655. doi:10.1016/j.ijrmms.2024.105655
Fredlund, D. G., Morgenstern, N. R., and Widger, R. A. (1978). The shear strength of unsaturated soils. Can. Geotechnical J. 15 (3), 313–321. doi:10.1139/t78-029
Gen-long, W., Mao-sheng, Z., Tian-ming, S. U., and Qing-ming, Z. (2011). Collapse failure modes and dem numerical simulation for loess slopes. J. Eng. Geol. 19 (04), 541–549. doi:10.3969/j.issn.1004-9665.2011.04.015
Gu, K., Xiang, F., Liu, C., Shi, B., and Zheng, X. (2023). Insight into the mechanical coupling behavior of loose sediment and embedded fiber-optic cable using discrete element method. Eng. Geol. 312, 106948. doi:10.1016/j.enggeo.2022.106948
Huat, B. B. K., Ali, F. H., and Low, T. H. (2006). Water infiltration characteristics of unsaturated soil slope and its effect on suction and stability. Geotechnical and Geol. Eng. 24 (5), 1293–1306. doi:10.1007/s10706-005-1881-8
Leo-tao, F., Wei-jiang, W., Xing-rong, L., Xing, S., and Zhao-dong, W. (2023). Study on precipitation infiltration mode and triggered landslides in the Loess Plateau--A case study in the Loess region of Gansu. Sci. Technol. Eng. 23 (14), 5937–5945. doi:10.3969/j.issn.1671-1815.2023.14.010
Li, M., Du, J.-S., and Gao, W.-Y. (2009). Study on the relationship between geological disasters and precipitation in the Loess Plateau in north Shaanxi province: study on the relationship between geological disasters and precipitation in the Loess Plateau in north Shaanxi province. Arid Zone Res. 26 (4), 599–606. doi:10.3724/sp.j.1148.2009.00599
Li-nan, L., Shou-ding, L., Yea, J., Yaheng, B., and Yu, L. (2017). Failure mechanism of loess landslides due to saturated-unsaturated seepage-case study of gallente landslide in ili, xinjiang. J. Eng. Geol. 25 (5), 1230–1237. doi:10.13544/j.cnki.jeg.2017.05.00
Liu, W., Luo, X., Huang, F., and Fu, M. (2017). Uncertainty of the SoilWater characteristic curve and its effects on slope seepage and stability analysis under conditions of rainfall using the Markov chain Monte Carlo method. Water 10, 758. doi:10.3390/w9100758
Liu, C., Le, T.-C., and Shi, B. (2020). S discussion on three major problems of engineering application of the particle discrete element method. Chin. J. Rock Mech. Eng. 39 (06), 1142–1152.
Maosheng, Z., and Tonglu, L. (2011). Triggering factors and forming mechanism of loess landslides. J. Eng. Geol. 19 (4), 530–540. doi:10.3969/j.issn.1004-9665.2011.04.014
Min, S., Yuting, L., Chan, D., and Fen, L. (2021). Analysis of damage mechanism of Yanguan landslide in three gorges reservoir area based on pore water pressure change. J. Eng. Geol. 29 (3), 734–741. doi:10.13544/j.cnki.jeg.2020-040
Ming-Jing, J., Hao-ze, Z., Tao, L., and Peng, Z. (2019). DEM analysis of isotropic compression tests on remolded and structured unsaturated loess. Chin. J. Geotechnical Eng. 41 (S2), 121–124. doi:10.11779/CJGE2019S2031
Ministry of Housing and Urban-Rural Development of the People’s Republic of China. GB/T 50123-2019 (2019). Standard for geotechnical test methods. Beijing: China Standard Publication.
Ministry of Natural Resources (2022). The 14th FYP of the People’s Republic of China for preventing and combating geological hazards. Bei Jing: Ministry of Natural Resources.
Ministry of Natural Resources.GB/T 38509-2020 (2020). Design code for landslide prevention and control. Beijing: China Standard Press.
Mao-hua, Z., Yong-li, X., Bao-jian, L., et al. (2006). Analysis of shear strength characteristics of loess during moistening process. Rock Soil Mech. (07), 1195–1200. doi:10.3969/j.issn.1000-7598.2006.07.036
Muntohar, A. S., and Liao, H. J. (2010). Rainfall infiltration: infinite slope model for landslides triggering by rainstorm. Nat. Hazards 54 (3), 967–984. doi:10.1007/s11069-010-9518-5
Ping, L., Tong-Lu, L., Hong, W., and Yan, L. (2013). Soil-water characteristic curve and permeability perdiction on childs and collis-geroge model of unsaturated loess. Rock Soil Mech. 34 (S2), 184–189. doi:10.16285/j.rsm.2013.s2.067
Rui, X., Hui, C., Xun-chang, L., and An-feng, M. (2017). Research on deformation mechanism and urgent treatment of unstable slope induced by construction. COAL Geol. and Explor. 45 (3), 107–111. doi:10.3969/j.issn.1001-1986.2017.03.020
Rui, X., Guang-lu, W., Guo-chen, S., Xun-chang, L., and Wei, Y. (2023). Erosion evolution of loess excavated slope in yan’an area. J. Railw. Eng. Soc. 40 (7), 1–8. doi:10.3969/j.issn.1006-2106.2023.07.001
Shuaihua, Y., and Yilei, S. (2018). Stability analysis of multilevel loess high slopes under rainfall infiltration conditions. J. Eng. Geol. 26 (6), 1648–1656. doi:10.13544/j.cnki.jeg.2017-552
Wang, N.-Q., and Tang, L.-C. (2016). Motion mechanism and numerical simulation of the loess collapse. J. Water Resour. Archit. Eng. 14 (02), 152–156. doi:10.3969/j.issn.1672-1144.2016.02.030
Xian-li, X., Tong-lu, L., Ping, L., Yu-kai, F., and Yu, X. (2014). Variation regularities of loess shear strength with the moisture content. Hydrogeology Eng. Geol. 41 (03), 53–59+97. doi:10.16030/j.cnki.issn.1000-3665.2014.03.019
Xu, R., Guo, L., Li, X., and Dong, Q. (2015). Research on the growth characteristics and slope protection of artemisia ordosica in yan’ an area. J. Railw. Eng. Soc. 32 (9), 19–24. doi:10.3969/j.issn.1006-2106.2015.09.004
Xu, R., Li, X., Yang, W., Jiang, C., and Rabiei, M. (2019). Use of local plants for ecological restoration and slope stability: a possible application in Yan’an, Loess Plateau, China. Nat. Hazards Risk 10 (1), 2106–2128. doi:10.1080/19475705.2019.1679891
Yong-qiang, L., Yan, X., Bo, C., and Bin-yu, L. (2024). Correlation mechanism between effective stress variation mode induced by back pressure and soil strength. Rock Soil Mech. 41, 1–13. doi:10.16285/j.rsm.2023.1488
Keywords: loess, traction landslide, soil-rock interface, stagnant water, discrete element
Citation: Hou D, Zeng F, Deng J, Wei H and Xu R (2024) Failure mechanism of loess landslide induced by water stagnation on the combined surface. Front. Earth Sci. 12:1467209. doi: 10.3389/feart.2024.1467209
Received: 19 July 2024; Accepted: 18 September 2024;
Published: 30 September 2024.
Edited by:
Hans-Balder Havenith, University of Liège, BelgiumReviewed by:
Kun Fang, Hong Kong University of Science and Technology, Hong Kong SAR, ChinaPaul Sestras, Technical University of Cluj-Napoca, Romania
Copyright © 2024 Hou, Zeng, Deng, Wei and Xu. This is an open-access article distributed under the terms of the Creative Commons Attribution License (CC BY). The use, distribution or reproduction in other forums is permitted, provided the original author(s) and the copyright owner(s) are credited and that the original publication in this journal is cited, in accordance with accepted academic practice. No use, distribution or reproduction is permitted which does not comply with these terms.
*Correspondence: Rui Xu, firewoodxu@chd.edu.cn