- 1Railway Engineering Research Institute, China Academy of Railway Sciences Corporation Limited, Beijing, China
- 2School of Architecture and Engineering, Shangqiu Normal University, Shangqiu, China
- 3School of Energy and Mining Engineering, China University of Mining and Technology, Beiing, China
- 4School of Engineering, University of Warwick, Coventry, United Kingdom
Due to the frequent occurrence of defects of slope protection facilities on existing lines causing the potential major geological hazards (e.g., landslides), it is necessary to promote the inspection and detection efficiency of various defects of slope protection facilities for carrying out early warning and evaluation, as well as, putting forward relevant control measures for slope disasters. Based on the case studies of hundreds of protective facilities on the existing railway line, the typical defect types, characteristics and specific causes of retaining wall, slope protection, anti-slide pile and anchorage engineering structure are firstly determined. Subsequently, a new comprehensive method combining unmanned air vehicle (UAV) and intelligent detection of portable radar (IDPR) are proposed. Among of them, UAV can effective and efficient identify the defect situations of slope protection facilities, and the water accumulation and cracks of retaining wall through performing a periodic inspection of the surrounding environment of protective facilities. In addition, IDPR can detect the filling situations behind the protective facilities to prevent the collapse danger. Through comparison with the drilling core results of tested areas, it is found that the detection results of the IDPR are more reliable and accurate, which is worthy of popularization and application in the field.
1 Introduction
Railways are the main mode of transportation in the world, while the railway slope hazards can be caused by the deformation, movement and destruction of surrounding geological body under the action of various factors. Many slope disasters of railways may be occurred in the construction and operation period, especially for old railways due to the lack of experiences and weak technologies (Dong et al., 2013). Moreover, the slope disasters can cause serious safety accidents and have huge impacts in economic and social aspects. Therefore, it is necessary to carry out efficient inspection and detection to the protection facilities of the existing railway lines for reducing the geological hazards.
The construction and maintenance of slope protection facilities have been paid more attention in the recent years. Collins (2008) studied the cracks and small steep slopes of fill slopes, mapped the potential debris flow paths and carried out risk assessment. Control measures were proposed to prevent and reduce losses. Beben et al. (2013) applied ground penetrating radar (GPR) technology in the diagnosis of bedrock and retaining walls, and obtained data required for geotechnical and civil engineering. Shinoda et al. (2013) developed a detection method for quantitative evaluation of existing railway retaining walls based on model tests, which provided an effective means for evaluating the health status of retaining wall. Turner et al. (2015) established the digital surface models of landslides and used the COSI-Corr image correlation algorithm to track and quantify the movement of landslides. The algorithm allowed the UAV to map and monitor the dynamics of landslides on a relatively long time series. Atzeni et al. (2015) discussed the main characteristics of modern SAR (Synthetic Aperture Radar) slope monitoring technology, which can be used for early warning and monitoring of natural and engineering slopes, improving the understanding of slope failure mechanism. Dixon et al. (2015) conducted a field monitoring test on a railway slope in the UK based on the acoustic emission monitoring system, which was consistent with the continuous deformation measurement results. The system can provide continuous displacement rate information and send out early warning through short message. Huang et al. (2022) established a safety risk assessment model based on BP neural network algorithm to evaluate the safety risk in the construction of high cutting slope, and put forward the control measures of safety risk, which can provide experience and reference for the safety management of high cutting slope construction. Kromer et al. (2015) used lidar to monitor a rock slope around a railway in Canada. It can better identify the precursors of slope failure and obtain the area with the highest probability of slope failure, which provided a basis for the hazard management of rock slopes around the railway. Dabbiru et al. (2016) detected slopes around the Mississippi River based on Synthetic Aperture Radar technology, which can identify problem areas along the embankment and avoid catastrophic damage. Nolesini et al. (2016) used remote sensing technology and ground radar to monitor the displacement of a mountain landslide in Italy, providing technical support for managers. Lin et al. (2017) analyzed the seismic performance of gravity retaining wall of steep rock slope reinforced by anchored frame beam, and considered that the nonlinear behavior of anchored frame beam was more obvious than that of gravity retaining wall. Xu (2017) proposed a damage alarm index based on the finite element model of the retaining wall, which can be used as an alarm for the damage stability of the wall. Rossi et al. (2018) developed a new type of UAV equipped with an optical camera to draw and describe the characteristics of landslides. It can detect slope areas that were prone to failure and evaluate the area and volume of landslides involved. Xu et al. (2018) revealed the anchoring mechanism of the adaptive anchor cable (AAC) system and the dynamic characteristics of the AAC system-anchor slope model based on the Xiangjiapo anchored rock slope test, which provided a basis for the design and seismic measures of slopes in seismically active areas. Galli and Bassani (2018) proposed a design method of slope stability system based on field displacement data, which provided a reliable and safe solution for slope defects. Carlà et al. (2018) deeply understood the behavior and kinematics of the landslide by monitoring the displacement of the retaining wall. Chen et al. (2019) proposed a composite unit anti-slide pile model, and analyzed the influence of different design parameters on the slope reinforcement effect and potential failure modes of anti-slide piles, which has certain guiding significance for the design of anti-slide piles. Ye et al. (2019) established the ground motion analysis model of slope reinforced by prestressed anchor frame, revealed the dynamic response characteristics of reinforced slope under horizontal seismic action, and provided the basis for seismic analysis and design of anchorage structure. Wang et al. (2020) established a digital elevation model of the slope based on the UAV technology, and used the DEM model to calculate the displacement of the monitoring area, which has high monitoring efficiency. Lin et al. (2020) established a numerical model of three-stage soil slope supported by anchor frame structure, revealed the dynamic response characteristics of three-stage soil slope and anchor frame structure, and provided basic information for the seismic design of three-stage soil slope and anchor frame structure. Ishak et al. (2020) developed a DSM based on UAV technology, which can provide researchers with important parameters and information about the topography of the study area. Based on the large-scale shaking table test, Zhang et al. (2020) analyzed the interaction between the slope and the anchor cable and lattice beam, which provided a reference for the seismic design of the slope and the optimization of the supporting structure. Macciotta and Hendry (2021) identified large-scale active landslides in Canada based on remote sensing technology, and redefined the landslide range and its deformation mechanism. Vemulapalli and Mesapam (2021) established a DEM of the slope based on UAV technology, which was used to analyze the stability of the slope of the open-pit mine and evaluate the influencing factors leading to the slope failure. Based on UAV technology, Zolkepli et al. (2021) mapped several slopes around Pahang Matriculation College in Malaysia, determined the important information of the slope, and then identified potential slope hazards. It is helpful for engineers and researchers to carry out early prevention to prevent property losses and casualties. Qu et al. (2021), Qu et al. (2022) revealed the seismic failure mechanism of the geogrid reinforced gravity retaining wall, and illustrated the failure development process of the reinforced subgrade slope. It was considered that the geogrid can reduce the displacement and rotation of the retaining wall, and the energy of the ground motion can be absorbed by the surrounding soil. Sestras et al. (2022) analyzed the stability of a slope in Romania using methods such as topological geodesy, UAV surface displacement monitoring, and GPR surveys. Valuable information on landslide and erosion dynamics was obtained, which can predict the future movement of landslides. Zhang et al. (2022) constructed a comprehensive monitoring and early warning platform for high and steep slopes based on health monitoring and safety evaluation, which can provide comprehensive early warning information, evaluation indicators, monitoring elements and other key data, and realize the intelligence, automation and visualization of monitoring data. Zhong et al. (2024) proposed a simple method to identify the hidden dangers of landslide geological disasters, and carried out comprehensive remote sensing identification and field verification of landslide disasters in Longde County, China. Hao et al. (2023) analyzed the slope stability under normal conditions and rainfall conditions, and revealed the dynamic characteristics of soil slope caused by blasting effect. On this basis, a slope mitigation method was proposed, and it was considered that the slope was unstable under the coupling condition of rainfall and blasting. Shehadeh et al. (2024) proposed a new slope displacement inspection and management algorithm, which can provide an automated decision support tool for site managers.
Throughout the literature review, the application of remote sensing, displacement and acoustic emission and artificial intelligence technology in slope monitoring and early warning system has been significantly mature, providing effective means for field technicians (Song et al., 2023). In addition, the technology related to UAV and geological radar was adopted in the defect inspection and detection of protective facilities. However, geological radar was relatively difficult to be obtained and there needs cost long time to process data. Therefore, this study proposes a new comprehensive method combing UAV and intelligent detection of portable radar (IDPR) to reduce the geological hazards in the existing railway lines. Among of them, the surrounding environment of protective facilities and retailing wall can be periodic inspected and compared by UAV, and the internal quality of retaining wall and slope protection can be detected by IDPR. The results were compared with the statistical results of borehole drilling. In addition, hundreds of protective facilities on existing lines in different regions of China were investigated, the typical defect types, characteristics and specific causes of retaining wall, slope protection, anti-slide pile and anchorage engineering structure were determined. The aims of the current research are to provide new ideas for the on-site inspection and detection of protective facilities and provide support for the risk assessment of slope supporting structure.
2 Typical defects and their routine inspection and detection methods
2.1 Typical defect types and causes
Through the investigation of hundreds of protective facilities on existing lines under the jurisdiction of Zhengzhou and Nanning, the typical defect types, characteristics and causes of retaining wall, slope protection, anti-slide pile and anchorage engineering structure of existing railway lines are analyzed.
Retaining wall refers to the supporting roadbed filling or hillside soil, to prevent filling or soil deformation instability structures. With the continuous deterioration of geological conditions, under the influence of temperature, humidity and atmospheric rainfall, the retaining wall along the railway is prone to bulging, cracking, cavity, falling off, water leakage, mud and vertical and horizontal cracks of the wall, which has a negative impact on the stability of the subgrade slope and seriously endangers the safe operation of the railway. The main defect types of the retaining wall structure can be divided into four categories: quality problems, insufficient bearing capacity, drainage system failure and external environmental changes. Typical cases are shown in Figure 1. Among them, the causes of quality problems include insufficient geometric size of the wall, unqualified wall materials, voids in the wall, and insufficient bonding of the wall. The causes of insufficient bearing capacity include foundation sliding failure, failure to rotate around the toe of the wall, uneven subsidence of the foundation, shear failure of the wall body, and sliding along the fracture surface of the weak layer. The causes of the failure of the drainage system include the aging of the drainage hole structure, the destruction of the filter layer, the overflow of the ditch, the cultivation and irrigation, and the domestic sewage discharge. The causes of external environmental changes include wall top farming, wall top loading, and adjacent engineering disturbances.

Figure 1. Typical defects of retaining wall. (A) Insufficient wall thickness; (B) Foundation sliding failure; (C) Drainage hole structure aging; (D) Wall top stack load.
Slope protection is a general term for all kinds of paving and planting on the slope in order to prevent the slope from being scoured. Mortar rubble revetment is a kind of masonry structure made of mortar and rubble, which often occurs slope cracking, aging damage and poor drainage. The main defect types of slope protection structure can be divided into four categories: quality problems, unreasonable setting, drainage system failure and external environmental changes. Typical cases are shown in Figure 2. Among them, the causes of quality problems are the slope protection cavity, slope protection bulging and mortar rubble aging and damage, etc. The causes of unreasonable slope protection setting are the slope protection cracking and slope protection outward collapse, etc. The causes of drainage system failure are aging of drainage hole structure, filling of rock and soil mass, change of groundwater flow path, etc. The causes of external environment change are the slope top farming, slope top surcharge, adjacent engineering disturbance.

Figure 2. Typical defects of slope protection. (A) Partial cavity; (B) Collapse; (C) Drainage ditch cracking; (D) Slope top stack load.
Anti-slide pile is a new type of retaining structure, but due to the quality of the project and the defects in operation, it is easy to lead to the destruction of anti-slide piles. According to relevant statistics, the probability of various defects in the pile body is about 15%–20%. If it is not found and treated in time, it will cause hidden dangers to the normal use of geotechnical structures and buildings. The main defect types of anti-slide pile structure can be divided into three categories: anti-slide pile defects, embedded rock mass defects, and deformation body defects. Typical cases are shown in Figure 3. Among them, the causes of anti-slide pile defects include insufficient bending resistance of pile body, insufficient shear resistance of pile body and excessive horizontal displacement of pile top. The causes of embedded rock mass defects include insufficient resistance of soil in front of pile and insufficient depth of embedded section. The causes of deformation body defects include overtopping failure and soil arching effect failure between piles.
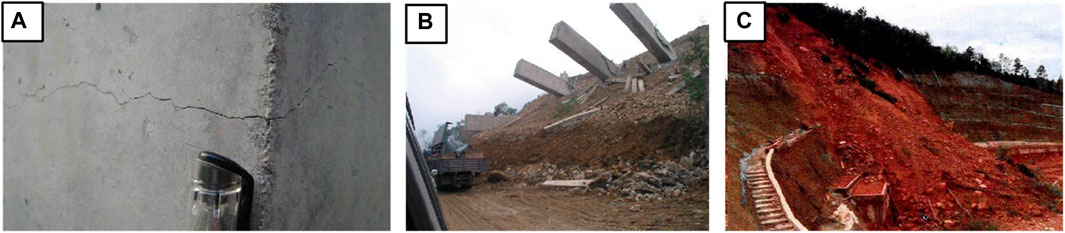
Figure 3. Typical defects of anti-slide piles. (A) Pile cracking; (B) Pile toppling; (C) Soil over the top of pile.
Anchorage technology refers to the use of bonding medium or a locking mechanism to fix one end of the metal rod in a more stable rock and soil layer, and the other end is connected to the engineering structure, thereby controlling the deformation of the rock and soil. Due to the pre-applied prestress, the anchor frame structure can change the overall stress of the slope, thus effectively controlling the displacement of the slope. During the operation period, due to the effect of long-term high prestress, the change of geological and hydrological conditions, the corrosion of the structure, etc., the working performance of the anchorage structure will continue to deteriorate, which constitutes a potential safety hazard of the anchorage slope. The main defect types of anchorage engineering structures can be divided into four categories: anchorage defects, frame defects, slope foundation defects, and slope protection measures defects. Typical cases are shown in Figure 4. Among them, the causes of anchoring defect defects are insufficient anchoring force and insufficient strength of anchor rod (cable). The causes of frame defect defects are insufficient bending resistance of lattice beam, insufficient shear resistance of lattice beam, over-limit crack width of lattice beam, etc. The causes of slope foundation defect defects are frame suspension and insufficient bearing capacity of frame base. The causes of slope protection measures defect defects are destruction of slope protection measures and local collapse of slope interior. Through the above analysis, it is found that there are many kinds of defects in the existing railway line protection facilities, which seriously endanger the operation safety of the railway. It is necessary to carry out efficient inspection and detection to prevent accidents in time. The specific types of typical defects are shown in Table 1.

Figure 4. Typical defects of anti-slide piles. (A) Anchor failure; (B) Damage of frame beams; (C) The frame is suspended; (D) Damage of protective measure.
2.2 Conventional inspection and detection methods
The current protective facilities in existing railway lines were mainly inspected manually, requiring video recorders, cameras, engineering drills, steel rulers and other self-made equipment, which can clearly identify common defects such as cracking, cavity, bulging, collapse and extroversion, structural aging, and poor drainage. However, the safety pressure faced by the engineering department increased and the simple routine inspection cannot meet the current requirements due to the increase of the service life of protective structures, the gradual deterioration of itself, and the change of the external environment and hydrological conditions. Therefore, a new technology for periodic inspection of the surrounding environment of the protective facilities based on UAV was proposed.
The detection methods of retaining wall facilities include non-destructive detection and destructive detection. Non-destructive detection cannot cause any damage to the structure of concrete, and can judge the uniformity, compactness, strength, defects and other information of the retaining wall. The destructive detection results are reliable, while it is not convenient for sampling and testing, especially it will cause a certain degree of damage. The slope protection detection method is artificial visual and drilling core sampling. Artificial visual has certain limitations for hidden parts, and on-site drilling core sampling is easy to cause a certain degree of damage to the slope protection structure. The detection methods of anti-slide pile facilities are low strain method and acoustic wave transmission method. The low strain method uses the reflected wave method to process and analyze the waveform with pile quality information during the propagation of stress wave along the pile. The acoustic transmission method is used to identify the location of the pile foundation defects and estimate the size of the defects by analyzing the changes in acoustic parameters such as sound speed, frequency and wave attenuation of sound waves propagating in the concrete medium. The detection method of anchoring engineering facilities is mainly acoustic reflection method. By analyzing the reflected wave signal in time domain and frequency domain, the working parameters such as the effective length of anchor rod (cable) and anchoring quality are obtained.
At present, there are still some problems in the detection of protective facilities, such as inconsistent detection schemes, multiple detection equipment components, complex operation, and long internal and external industry cycles. After the accident, the detection team needs to rush from all over to the scene, which is time-consuming and laborious. Therefore, a new portable intelligent detection technology was proposed.
3 New inspection and detection methods of protective facilities in existing railway lines
3.1 UAV inspection method
3.1.1 Introduction of UAV
Unmanned aerial vehicle (UAV) can take topography from the air to obtain an overlooking map. Due to its unique advantages, it has been widely used in railway inspection and safety assessment (Wu et al., 2023; Qin et al., 2024).
After investigation and comparison, the Ddajiang series UAV was selected to compare and analyze the aerial photography cycle of protective facilities because it was portable and powerful, with higher-definition, smoother thermal imaging sensors and higher-pixel visible light sensors, etc. The precise positioning can complete the refined automatic flight inspection operation in complex scenes. This type of UAV has been widely used in fire rescue, emergency search and rescue, police law enforcement, environmental protection, power inspection and other fields. Figure 5 is UAV equipment and aerial photography, respectively.
3.1.2 Period comparison analysis technology
In general, the creep rate of geological disasters is very small and stable. When it suddenly increases, it means the disaster coming. According to the specific working conditions of different points, different cycles of aerial photography can be carried out. The aerial photography cycle is generally quarterly, semi-annual and annual. Periodic comparison analysis can interpret unknown images or understand the changes of ground objects based on known images, including time-phase dynamic comparison method and spatial comparison analysis method. The time-phase dynamic comparison method is an interpretation method to understand the dynamic changes of the same target by analyzing the remote sensing images of the same area at different times. The spatial comparison analysis method analyzed the two images of similar regions or applies different types of remote sensing images to the selected area. The above methods can effectively identify the defect information of protective facilities, which is more accurate and effective than manual visual inspection. This technology is our application innovation on the basis of UAV aerial photography technology and has been widely promoted.
3.2 Intelligent detection of portable radar (IDPR)
Based on the shortcomings of traditional detection equipment, it is urgent to develop a portable intelligent detection equipment. As shown in Figure 6, the equipment has small size and the ability of waterproof, dustproof and shockproof. It is simple to operate and can display the collected images in real time, realize wireless transmission, network transmission, and realize the synchronous interrogation between the background and the foreground. One to two inspectors can complete the data collection of the rescue task.
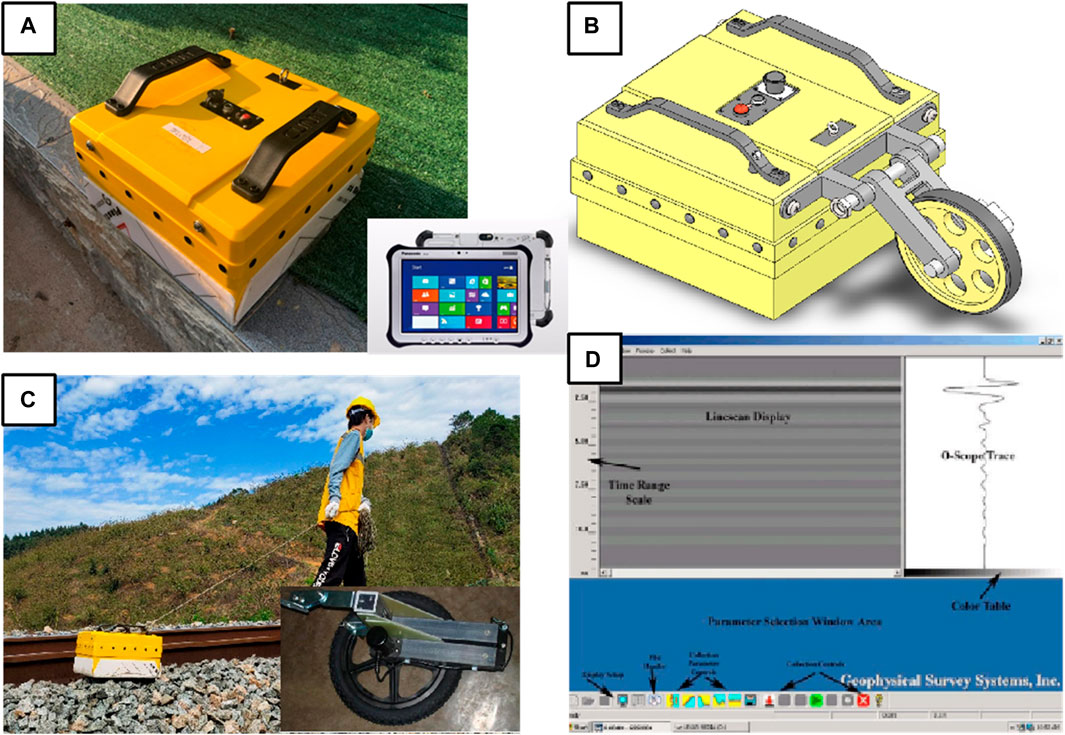
Figure 6. IDPR. (A) Instrument; (B) Portable wheel; (C) Field detection; (D) Detection result display window.
The new equipment can detect the internal filling of the retaining facilities, such as water content, compactness, cavity, etc. In addition, the quality detection of tunnel lining and subgrade structure can also be realized. The specific performance indicators are shown in Table 2.
3.3 Inspection and detection content and process of new technology
For high protective facilities and cliffs that cannot be reached by manpower, new technologies such as UAV aerial photography and SRS (satellite remote sensing) images should be used for periodic comparison analysis. The inspection of the key road base section shall not be less than once a quarter. The flood season inspection can be divided into rain, after rain and monthly inspection. In case of heavy rain, rainstorm and continuous rainfall, the number of inspections should be increased as appropriate, and the problems found in the inspection should be reported in time. In case of emergency, safety measures should be taken decisively. In order to grasp the changes in the surrounding environment of the retaining facilities, new technologies such as UAV aerial photography and SRS images should be used for periodic comparison analysis. The key areas should be inspected every 6 months. In the flood season, the number of inspections should be increased as appropriate, and the problems found in the inspection should be reported in time.
For conventional protective facilities, AVO (artificial visual observation) and IDPR are often used. AVO should set a reasonable observation path to ensure that common defects such as cracking, cavity, bulging, collapse and extroversion, structural aging and poor drainage of retaining facilities can be clearly identified. In order to avoid the subjective problem of manual visual inspection, IDPR can be used to detect the protective facilities in special areas. For defects that are difficult to accurately judge, core drilling method and pull-out method can be used. The specific inspection and detection process is shown in Figure 7.
4 Case study
4.1 Application of UAV technology
As shown in Figure 8, the time-phase dynamic comparison method is used to compare and analyze the UAV cycle aerial images of project section Ⅰ in Hunan and Guangxi. It can be seen that the change of the retaining wall during and after the flood season. The structure of the retaining wall is relatively complete before the flood season. During the flood season, the rear part of the retaining wall is seriously scoured by rainwater and is damaged to a certain extent. Therefore, it is easy to produce the defect of water accumulation, which can be verified by the comparative analysis of aerial images in the next year.
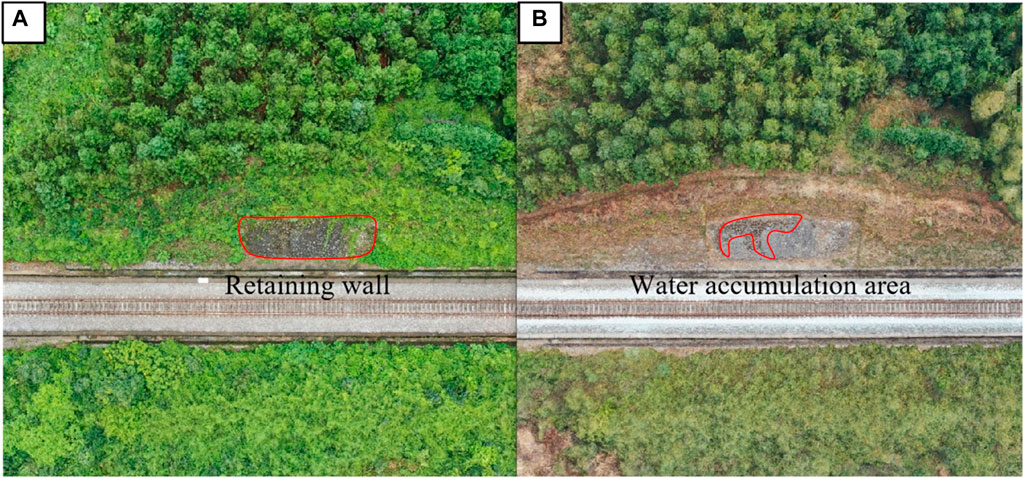
Figure 8. UAV aerial image of retaining wall of project section Ⅰ in Hunan and Guangxi. (A) Aerial model in July; (B) Aerial model in February of the next year.
As shown in Figure 9, by comparing the images of the mortar rubble retaining wall in a certain section of Xianggui in different periods, it can be seen that due to the influence of flood season and its own quality, there are defects such as water accumulation and cracks in the middle of the retaining wall.
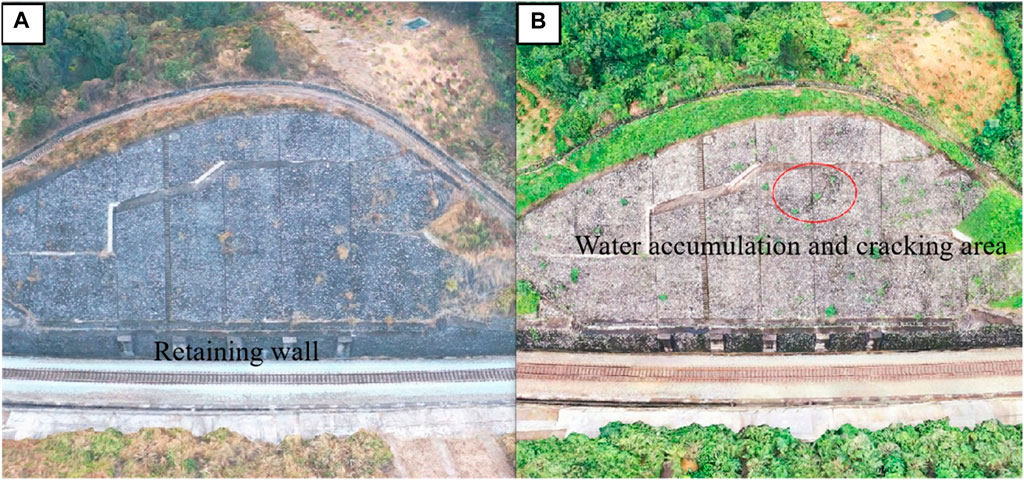
Figure 9. UAV aerial image of retaining wall of project section Ⅱ in Hunan and Guangxi. (A) aerial model in June; (B) Aerial model in February of the next year.
The Digital Surface Model (DSM) is established for the slope protection of a project section in Hunan and Guangxi by using the time phase dynamic comparison method and the spatial comparison analysis method. As shown in Figure 10, by comparing and analyzing the UAV aerial images and DSM images, it can be seen that the slope protection is relatively stable in June. Affected by the flood season, there are obvious sliding and accumulation defects on the left surface of the slope protection in February of the following year.
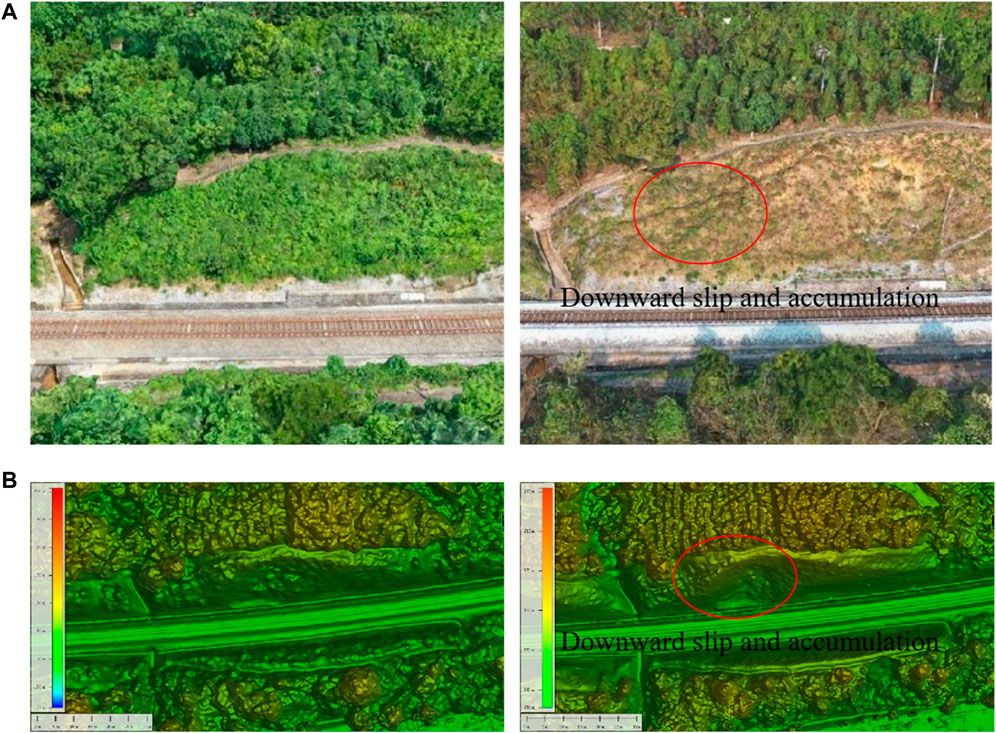
Figure 10. Slope protection cycle image of a project section in Hunan and Guangxi. (A) UAV images for June and February of the following year; (B) DSM images for June and February of the following year.
From the above cycle comparative analysis technology application cases, it can be seen that UAV remote sensing images contain three types of information with space, spectrum and texture. Using image data at different times can monitor the change of the target, and using different types of images can assist in identifying the change of the target, so as to obtain more geographic information of the target. Compared with the traditional AVO, the UAV aerial photography technology has a large inspection range, high efficiency, and clear discrimination criteria, which can be used as a necessary supplement to the existing inspection methods.
4.2 Application of IDPR
4.2.1 The downward left retaining wall of K1 and K2 sections of Taijiao line
This section is a road cut, and the line is excavated in the middle of the mountain from southwest to northeast in the form of double lines. The small mileage is higher than the large mileage, and the left side is slightly higher than the right side. The vegetation of the hillside is strong, and the gradient of the slope protection is gentle.
Figures 11,12 are the results of geological radar profile and drilling core diagram, respectively. From the geological radar detection map, it can be seen that the retaining wall has second-level cavity in the K1 section and the retaining wall has second-level not dense in the K2 section. The statistical results of borehole coring in K1 section show that 0–46 cm limestone is intact, 46–55 cm cavity, 55–79 cm limestone is intact, 79–90 cm soil (hard plastic). The statistical results of borehole coring in K2 section show that 0–15 cm limestone is intact, 15–30 cm mortar is not dense, 30–65 cm limestone is intact, 65–74 cm cavity (including gravel is not dense), 74–80 cm soil. This result is consistent with the geological radar detection results. The analysis results can provide sufficient basis for identifying the types of retaining wall defects and proposing corresponding prevention measures. The relevant drilling coring parameters are shown in Table 3.
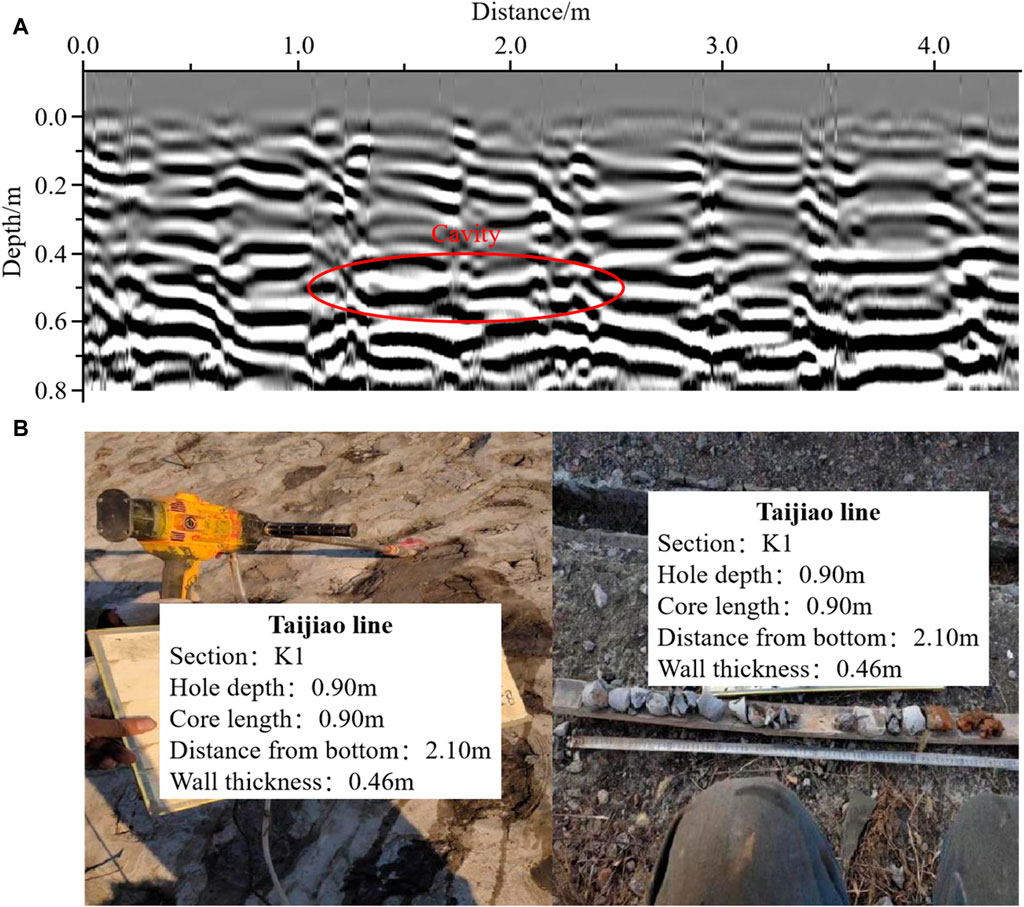
Figure 11. Comparison of detection results of downward left retaining wall in K1 section of Taijiao line. (A) Geological radar profile; (B) Core drilling profile.
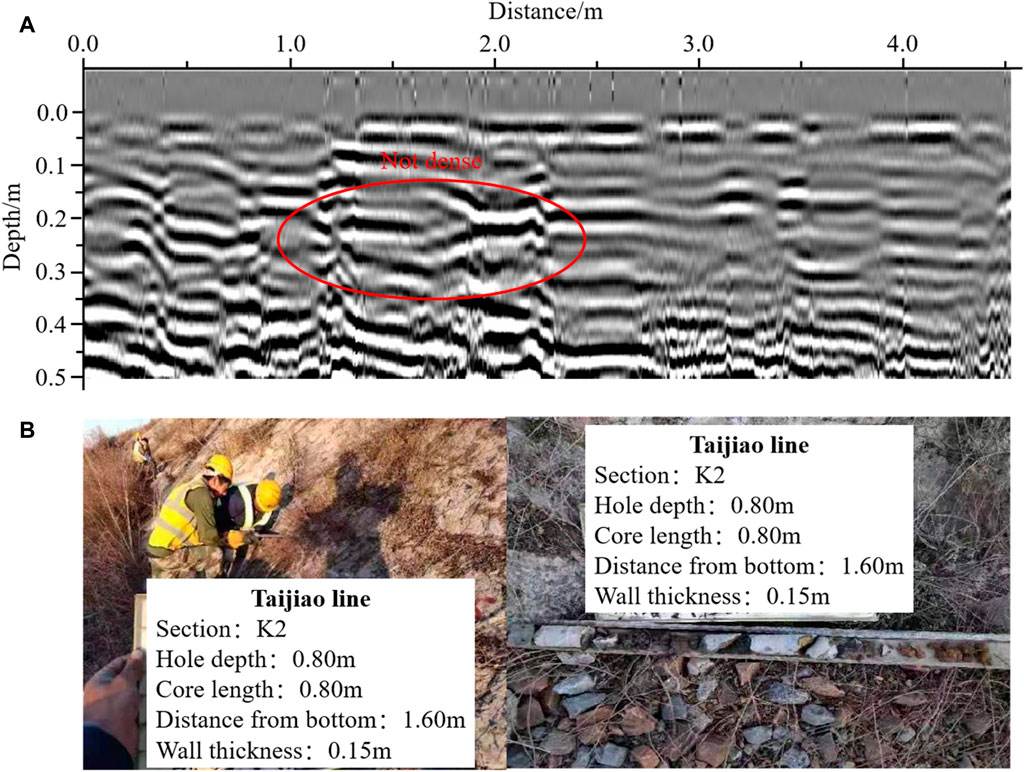
Figure 12. Comparison of detection results of downward left retaining wall in K2 section of Taijiao line. (A) Geological radar profile; (B) Core drilling profile.
4.2.2 The upward left slope protection of K1 and K2 sections of Houyue line
The terrain of this section is high, the right side is the embankment, the left side is the road cut, and the starting and ending of the slope are both the road cut. The line passes from south to north, the right side is the special line for railway freight yard, and the slope has a curvilinear appearance.
Figures 13,14 are the results of geological radar profile and drilling core diagram, respectively. From the geological radar detection map, it can be seen that the slope protection has cavity in the K1 section and the slope protection has not dense in the K2 section. The statistical results of borehole coring in K1 section show that 0–21 cm limestone is intact, 21–30 cm mortar, 30–60 cm limestone is intact, 60–66 cm cavity, 66–73 cm limestone is broken, 73–81 cm limestone is intact, 81–92 cm cavity, 92–100 cm limestone is intact. The statistical results of borehole coring in K2 section show that 0–21 cm limestone is intact, 21–28 cm mortar, 28–50 cm limestone is intact, 50–60 cm is not dense, 60–80 cm limestone is intact. This result is consistent with the geological radar detection results. The analysis results can provide sufficient basis for identifying the types of slope protection defects and proposing corresponding prevention measures. The relevant drilling coring parameters are shown in Table 4.
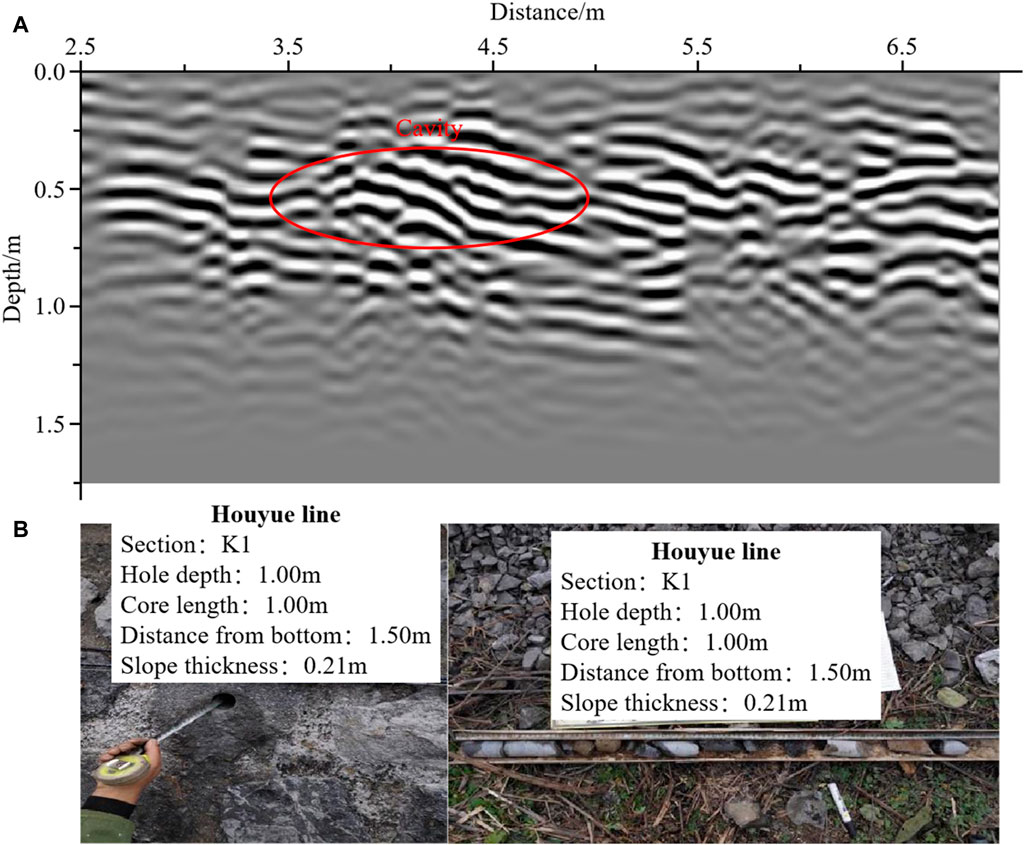
Figure 13. Comparison of detection results of downward left slope protection in K1 section of Houyue line. (A) Geological radar profile; (B) Core drilling profile.
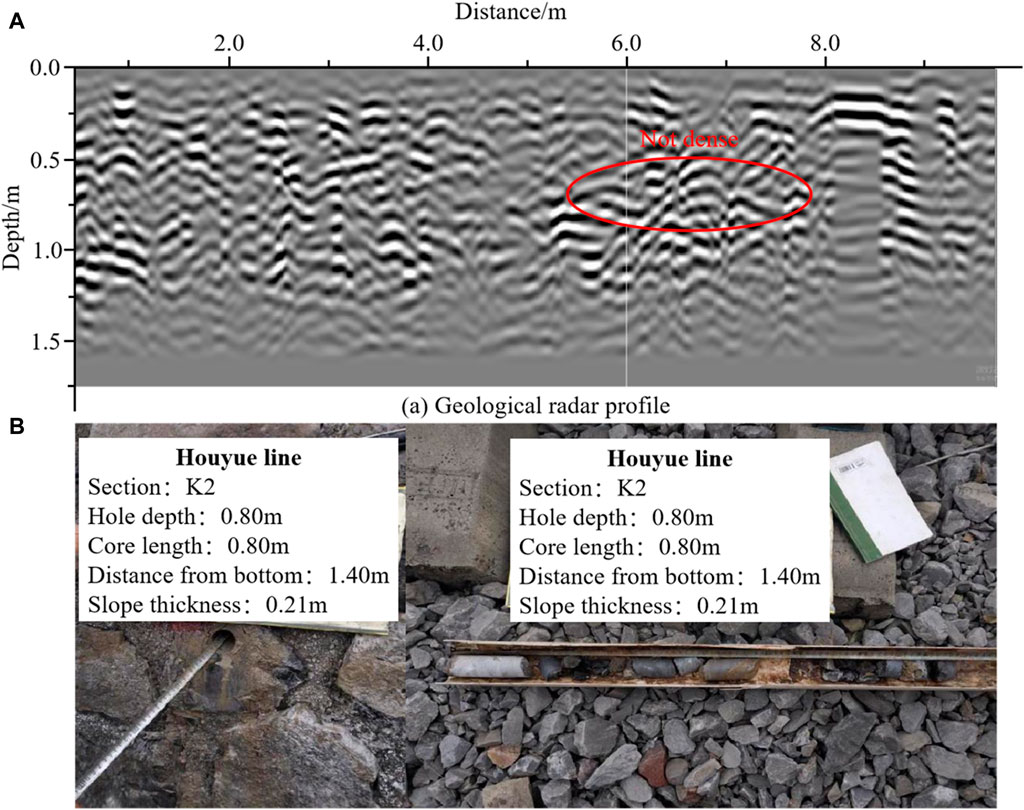
Figure 14. Comparison of detection results of downward left slope protection in K2 section of Houyue line. (A) Geological radar profile; (B) Core drilling profile.
4.2.3 Comparison of detection accuracy
Figure 15 is the image of the same geological site detected by the self-developed portable structure detector and the SIR series products of GSSI (Geophysical Survey Systems, Inc.) company. Through comparative analysis, it is found that the image waveforms obtained by the two devices have good correspondence. The self-developed equipment has obvious layered display, more prominent recognition of the detailed structure, and strong software post-processing functions, which can be promoted and applied in the field.
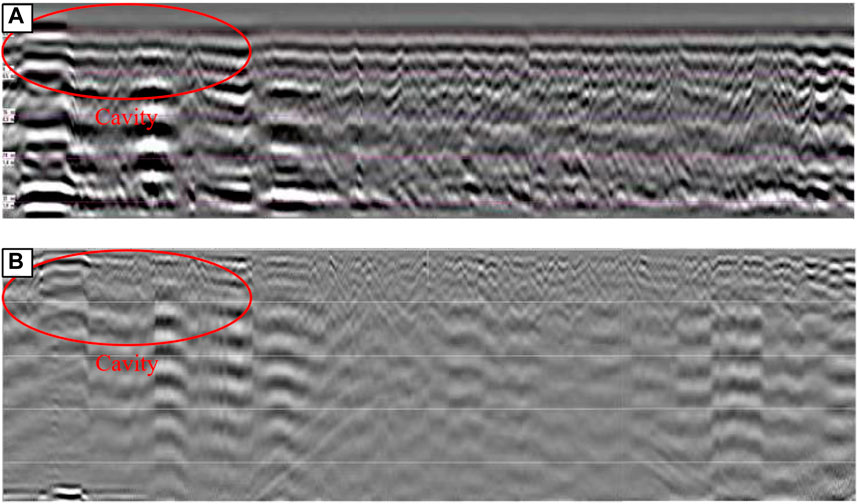
Figure 15. Comparison of detection results of the same geological site. (A) detection results of portable structure detector; (B) detection results of SIR series products.
5 Conclusion
In view of the large number of retaining facilities on both sides of the existing railway, which have a long service life and are prone to deterioration and defects, the types and characteristics of typical defects are summarized from the perspective of engineering inspection, and their causes are analyzed. The existing defect inspection and detection methods for old retaining facilities of existing railways have various problems with small inspection scope, low efficiency, unobvious contrast effect, low accuracy of detection technology, and inconsistent standards. Therefore, on this basis, a new technology of periodic inspection of surrounding environment of retaining facilities based on UAV and a new technology of portable intelligent detection are proposed and applied in the field. The main conclusions are drawn as follows.
(1) The causes of the defect of the mortar rubble retaining wall include the quality problem of the retaining wall, the insufficient bearing capacity of the retaining wall, the failure of the drainage system of the retaining wall, and the change of the external environment. According to the manifestation of the defect, it can be divided into 13 categories. The causes of slope protection defects include quality problems of slope protection, unreasonable setting of slope protection, slope protection drainage system failure and external environment changing. According to the manifestations of defects, they are divided into nine categories. The causes of anti-slide pile defects include anti-slide pile defects, embedded rock mass defects, and deformable body defects. According to the manifestations of the defects, they are divided into six categories. Meanwhile, the causes of anchorage engineering defects are anchorage defects, frame defects, slope foundation defects and slope protection measures defects. According to the manifestations of the defects, they are divided into nine categories.
(2) The time-phase dynamic comparison method is used to compare and analyze the UAV periodic aerial images of the first and second sections of a project in Hunan and Guangxi. It can be found that the back of the retaining wall in the flood season is prone to water accumulation and cracks in the middle of the retaining wall. According to the UAV aerial image of a third section of a project in Hunan and Guangxi and combined with DSM, the defect of downward slip and accumulation on the slope surface can be identified. From the above application cases, it can be seen that the new technology of periodic inspection of the surrounding environment of the retaining facilities based on UAV has obvious advantages.
(3) The application of new portable intelligent detection technology in multiple lines shows that the holes and non-compactness in the borehole coring results are consistent with the detection results of the self-developed portable radar, which proves the reliability of the developed portable intelligent detection equipment. The comparison results of detection accuracy show that the detection results of self-developed portable radar equipment have obvious stratification and clear detail recognition, which can be popularized and applied in the field.
The UAV and IDPR can bring a large number of images of slope protective facilities, and these images can be provided valuable data for current popular deep learning models. For ongoing and future research, convolutional neural network (CNN)-based deep learning models, such as hybrid attention convolutional neural network (Pan et al., 2020; Zhao et al., 2023a; Zhao et al., 2023b), can be further developed to automatically and accurately to detect defects of slope protective facilities from the UAV images and IDPR images.
Data availability statement
The original contributions presented in the study are included in the article/Supplementary material, further inquiries can be directed to the corresponding author.
Author contributions
JL: Methodology, Project administration, Software, Writing–original draft. XG: Data curation, Funding acquisition, Validation, Writing–original draft. HL: Investigation, Visualization, Writing–review and editing. HG: Formal Analysis, Resources, Writing–original draft. ZW: Formal Analysis, Resources, Writing–original draft. ZC: Formal Analysis, Supervision, Writing–review and editing.
Funding
The author(s) declare that financial support was received for the research, authorship, and/or publication of this article. This research was funded by “Key Fund of China Academy of Railway Sciences Group Co., Ltd., grant number 2023YJ304” and “National Natural Science Foundation of China High-speed Rail Joint Fund, grant number 2023YJ304.”
Conflict of interest
Authors JL and XG were employed by China Academy of Railway Sciences Corporation Limited.
The remaining authors declare that the research was conducted in the absence of any commercial or financial relationships that could be construed as a potential conflict of interest.
Publisher’s note
All claims expressed in this article are solely those of the authors and do not necessarily represent those of their affiliated organizations, or those of the publisher, the editors and the reviewers. Any product that may be evaluated in this article, or claim that may be made by its manufacturer, is not guaranteed or endorsed by the publisher.
References
Atzeni, C., Barla, M., Pieraccini, M., and Antolini, F. (2015). Early warning monitoring of natural and engineered slopes with ground-based synthetic-aperture radar. Rock Mech. Rock Eng. 48 (1), 235–246. doi:10.1007/s00603-014-0554-4
Beben, D., Anigacz, W., and Ukleja, J. (2013). Diagnosis of bedrock course and retaining wall using GPR. NDT E Int. 59, 77–85. doi:10.1016/j.ndteint.2013.05.006
Carlà, T., Macciotta, R., Hendry, M., Martin, D., Edwards, T., Evans, T., et al. (2018). Displacement of a landslide retaining wall and application of an enhanced failure forecasting approach. Landslides 15 (3), 489–505. doi:10.1007/s10346-017-0887-7
Chen, C., Wang, W., and Lv, H. (2019). Stability analysis of slope reinforced with composite anti-slide pile model. Rock Soil Mech. 40 (8), 3207–3217. doi:10.16285/j.rsm.2018.0747
Collins, T. K. (2008). Debris flows caused by failure of fill slopes: early detection, warning, and loss prevention. Landslides 5 (1), 107–120. doi:10.1007/s10346-007-0107-y
Dabbiru, L., Aanstoos, J. V., and Younan, N. H. (2016). Earthen levee slide detection via automated analysis of synthetic aperture radar imagery. Landslides 13 (4), 643–652. doi:10.1007/s10346-015-0599-9
Dixon, N., Smith, A., Spriggs, M., Ridley, A., Meldrum, P., and Haslam, E. (2015). Stability monitoring of a rail slope using acoustic emission. Proc. Inst. Civ. Eng.-Geotec. Eng. 168 (5), 373–384. doi:10.1680/jgeen.14.00152
Dong, J., Song, X., and Xu, Z. (2013). Study on analysis method for stability of bedding cutting slope along railway. J. Rail. Eng. Soc. 30 (3), 19–23. doi:10.3969/j.issn.1006-2106.2013.03.005
Galli, A., and Bassani, A. (2018). Innovative performance-based design of slope stabilizing piles for a railway embankment. Eur. J. Environ. Civ. Eng. 22 (1), 99–121. doi:10.1080/19648189.2016.1179681
Hao, Y., Liu, C., Zhang, W., Liu, X., and Liu, G. (2023). Landslide risk evaluation: rainfall and blast-induced potential soil landsides in an expressway area underneath a railway tunnel, Guangzhou, China. Bul. Eng. Geol. Environ. 82 (11), 420. doi:10.1007/S10064-023-03449-0
Huang, J., Zeng, X., Fu, J., Han, Y., and Chen, H. (2022). Safety risk assessment using a BP neural network of high cutting slope construction in high-speed railway. Buildings 12 (5), 598. doi:10.3390/BUILDINGS12050598
Ishak, M. F., Zolkepli, M. F., and Muhammad, N. (2020). Effectiveness of unmanned aerial vehicle (UAV) for digital slope mapping. Int. J. Eng.g Tech. Sci. 7, 119–136. doi:10.15282/ijets.7.2.2020.1010
Kromer, R. A., Hutchinson, D. J., Lato, M. J., Gauthier, D., and Edwards, T. (2015). Identifying rock slope failure precursors using LiDAR for transportation corridor hazard management. Eng. Geol. 195, 93–103. doi:10.1016/j.enggeo.2015.05.012
Lin, Y. L., Li, Y. X., Zhao, L. H., and Yang, T. Y. (2020). Investigation on seismic response of a three-stage soil slope supported by anchor frame structure. J. Cent. South Univ. 27, 1290–1305. doi:10.1007/s11771-020-4367-1
Lin, Y. L., Yang, G. L., Yang, X., Zhao, L. H., Shen, Q., and Qiu, M. M. (2017). Response of gravity retaining wall with anchoring frame beam supporting a steep rock slope subjected to earthquake loading. Soil Dyn. Earthq. Eng. 92, 633–649. doi:10.1016/j.soildyn.2016.11.002
Macciotta, R., and Hendry, M. T. (2021). Remote sensing applications for landslide monitoring and investigation in western Canada. Remote Sens. 13 (3), 366. doi:10.3390/RS13030366
Nolesini, T., Frodella, W., Bianchini, S., and Casagli, N. (2016). Detecting slope and urban potential unstable areas by means of multi-platform remote sensing techniques: the Volterra (Italy) case study. Remote Sens. 8 (9), 746. doi:10.3390/rs8090746
Pan, Y., Zhang, G., and Zhang, L. (2020). A spatial-channel hierarchical deep learning network for pixel-level automated crack detection. Automation Constr. 119, 103357. doi:10.1016/j.autcon.2020.103357
Qin, X., Zhang, Z., Wang, H., Yang, F., and Jing, G. (2024). Analysis of the application status of railway inspection UAV at home and abroad (I). Railw. Qual. Control 52 (1), 48–51+55.
Qu, H., Huang, X., Gao, Y., Deng, Y., and Li, B. (2021). HHT-based seismic damage analysis of a subgrade slope reinforced by a gravity retaining wall. Soil Mech. Found. Eng. 58 (5), 425–432. doi:10.1007/S11204-021-09761-9
Qu, H., Huang, X., Gao, Y., Zhang, Z., and Wang, C. (2022). Shaking table test of subgrade slope reinforced by gravity retaining wall with geogrids. Earth. Eng. Eng. Vib. 21 (3), 715–727. doi:10.1007/s11803-022-2108-1
Rossi, G., Tanteri, L., Tofani, V., Vannocci, P., Moretti, S., and Casagli, N. (2018). Multitemporal UAV surveys for landslide mapping and characterization. Landslides 15 (5), 1045–1052. doi:10.1007/s10346-018-0978-0
Sestras, P., Bilașco, Ș., Roșca, S., Ilies, N., Hysa, A., Spalević, V., et al. (2022). Multi-instrumental approach to slope failure monitoring in a landslide susceptible newly built-up area: topo-Geodetic survey, UAV 3D modelling and ground-penetrating radar. Remote Sens. 14 (22), 5822. doi:10.3390/RS14225822
Shehadeh, A., Alshboul, O., and Almasabha, G. (2024). Slope displacement detection in construction: an automated management algorithm for disaster prevention. Expert Syst. Appl. 237 (PB), 121505. doi:10.1016/J.ESWA.2023.121505
Shinoda, M., Nakajima, S., Abe, K., Ehara, T., and Kubota, Y. (2013). Stability inspection method for existing retaining walls. Quart. Rep. RTRI 54 (3), 159–165. doi:10.2219/rtriqr.54.159
Song, Z., Li, X., Huo, R., and Liu, L. (2023). Intelligent early-warning platform for open-pit mining: current status and prospects. Rock Mech. Bul. 3 (1), 100098. doi:10.1016/J.ROCKMB.2023.100098
Turner, D., Lucieer, A., and De Jong, S. M. (2015). Time series analysis of landslide dynamics using an unmanned aerial vehicle (UAV). Remote Sens. 7, 1736–1757. doi:10.3390/rs70201736
Vemulapalli, S. C., and Mesapam, S. (2021). Slope stability analysis for mine hazard assessment using uav. J. Indian Soc. Remote Sens. 49 (7), 1483–1491. doi:10.1007/S12524-020-01239-9
Wang, S., Zhang, Z., Ren, Y., and Zhu, C. (2020). UAV photogrammetry and AFSA-Elman neural network in slopes displacement monitoring and forecasting. KSCE J. Civ. Eng. 24 (8), 19–29. doi:10.1007/s12205-020-1697-3
Wu, Y., Meng, F., Qin, Y., Qian, Y., Xu, F., and Jia, L. (2023). UAV imagery based potential safety hazard evaluation for high-speed railroad using Real-time instance segmentation. Adv. Eng. Inf. 55, 101819. doi:10.1016/J.AEI.2022.101819
Xu, M., Tang, Y., Liu, X., Yang, H., and Luo, B. (2018). A shaking table model test on a rock slope anchored with adaptive anchor cables. Int. J. Rock Mech. Min. Sci. 112, 201–208. doi:10.1016/j.ijrmms.2018.10.021
Xu, Q. (2017). Investigation of stability alarming for retaining wall structures with damage. Shock Vib. 2017, 1–12. doi:10.1155/2017/4691947
Ye, S., Fang, G., and Zhu, Y. (2019). Model establishment and response analysis of slope reinforced by frame with prestressed anchors under seismic considering the prestress. Soil Dyn. Earthq. Eng. 122, 228–234. doi:10.1016/j.soildyn.2019.03.034
Zhang, J. J., Niu, J. Y., Fu, X., Cao, L. C., and Xie, Q. (2020). Shaking table test of seismic responses of anchor cable and lattice beam reinforced slope. J. Mt. Sci. 17 (5), 1251–1268. doi:10.1007/s11629-019-5712-4
Zhang, L., Qiu, Y., Yu, H., Yin, L., Zhang, F., and Wu, T. (2022). Development and application of an integrated monitoring and early warning platform for land transportation infrastructure under special engineering conditions. Geofluids 2022, 1429011. doi:10.1155/2022/1429011
Zhao, S., Tan, D., Lin, S., Yin, Z., and Yin, J. (2023a). A deep learning-based approach with anti-noise ability for identification of rock microcracks using distributed fibre optic sensing data. Int. J. Rock Mech. Min. Sci. 170, 105525. doi:10.1016/j.ijrmms.2023.105525
Zhao, S., Zhang, G., Zhang, D., Tan, D., and Huang, H. (2023b). A hybrid attention deep learning network for refined segmentation of cracks from shield tunnel lining images. J. Rock Mech. Geotechnical Eng. 15 (12), 3105–3117. doi:10.1016/j.jrmge.2023.02.025
Zhong, J., Li, Q., Zhang, J., Luo, P., and Zhu, W. (2024). Risk assessment of geological landslide hazards using D-InSAR and remote sensing. Remote Sens. 16 (2), 345. doi:10.3390/RS16020345
Keywords: slope protective facilities, geological hazards, UAV, intelligent detection of portable radar, inspection and detection methods
Citation: Li J, Gao X, Lv H, Guo H, Wang Z and Cheng Z (2024) A new method for inspection and detection of typical defects of protective facilities in existing railways and its application. Front. Earth Sci. 12:1416233. doi: 10.3389/feart.2024.1416233
Received: 12 April 2024; Accepted: 17 June 2024;
Published: 16 July 2024.
Edited by:
Bin Gong, Brunel University London, United KingdomReviewed by:
Zhanping Song, Xi’an University of Architecture and Technology, ChinaDefeng Wang, Freiberg University of Mining and Technology, Germany
Shuai Zhao, Hong Kong Polytechnic University, Hong Kong SAR, China
Copyright © 2024 Li, Gao, Lv, Guo, Wang and Cheng. This is an open-access article distributed under the terms of the Creative Commons Attribution License (CC BY). The use, distribution or reproduction in other forums is permitted, provided the original author(s) and the copyright owner(s) are credited and that the original publication in this journal is cited, in accordance with accepted academic practice. No use, distribution or reproduction is permitted which does not comply with these terms.
*Correspondence: Zhanbo Cheng, emhhbmJvLmNoZW5nQG50dS5lZHUuc2c=