- 1School of Resources and Safety Engineering, Henan University of Engineering, Zhengzhou, China
- 2Henan Energy & Chemical Industry Group Co., Ltd., Zhengzhou, China
The overlying strata of the Yima coalfield are ultrathick conglomerate. Aiming at the problem of frequent occurrence of rockburst events in the central Yima coalfield during 2006–2015, the characteristics of rockburst events, microseismic (MS) monitoring, and rockburst event-inducing factors were analyzed through data mining and field investigation methods. The results showed that the rockburst events in roadways mainly occurred during mining of the working face, and they occurred at a large buried depth and were within the influence of mining stress, accompanied by an abrupt energy release. The occurrence of rockburst in roadways was accompanied by a sudden release of energy. The ultrathick strata and the fault nearby were the key influence factors of rockburst events. The stress field of roadway surrounding rocks was changed because of the mining disturbance, roadway repair and maintenance, and blasting, which would change the regional stress fields in the surrounding rocks and induce roadway rockburst events. The characteristics of rockburst events were floor heave, sharp convergence of two side walls, severe damage of the supporting body, and even closure of the roadway. The occurrence of rockburst can be prevented by reducing the mining speed and injecting water into coal seam.
1 Introduction
Rockburst is an instantaneous release of elastic energy of an over-stressed coal and rock mass and has been recognized as one of the most critical dynamic failures in coal mines (Dai et al., 2021; Frith et al., 2020; Keneti and Sainsbury, 2018). The disaster poses a serious threat to the safe production of underground coal mining. It can destroy roadways with hundreds of meters instantaneously, causing injuries or deaths, damage to facilities, and leading to property losses. Complex and various geological conditions during tunneling and mining in coal mines lead to different causes and failure modes of rockbursts (Jiang et al., 2019; Wang et al., 2019). Coal mine safety production in many countries is seriously threatened by rockburst disaster, especially in countries such as South Africa, the United States, Russia, Australia, Czechia, Poland, and China (Ghorbani et al., 2020; Konicek et al., 2013; Małkowski and Niedbalski, 2020; Zhang et al., 2017). In China, because of the high-intensity mining of coal resources for a long time, coal mining is gradually transferred to the deep, and more than 170 coal mines have suffered rockbursts. So rockburst disasters have become a major problem in deep mining (Anderson, 2017; Ranjith et al., 2017).
When the large elastic energy accumulated in the surrounding rock of coal mine roadways is released abruptly, the blast waves produced acts on the surrounding rock, which may cause tensile shear deformation and damage of the surrounding rock, casualties, and equipment damage under the influence of deep high stress and complex geological environments (Mark and Gauna, 2021; Newman and Newman, 2021; Zhou et al., 2021). Such a rockburst is characterized by abruptness, serious damage, and complex inducements, and therefore, it has become one of the main current disasters in coal mines (Jiang et al., 2016; Pan et al., 2021; Wasilewski 2020). At present, the academic community has not formed a uniform theory to explain the occurrence mechanism of rockburst in mines. Through decades of theoretical research and investigation of causes of accidents, a series of classical theories have been proposed (Cao et al., 2019; Dai et al., 2021; Xuelong Li et al., 2021; Pang et al., 2016), such as the energy theory (Cook et al., 1966), strength theory, stiffness theory (Procházka, 2004), rockburst tendency theory (Gong et al., 2021; Tsirel' and Krotov, 2001), instability theory (Zhang 1987), and “three factors” theory (Qi and Dou, 2008). With the introduction of interdisciplinary disciplines such as mathematics and mechanics into this research field, the burst start-up theory (Pan et al., 2012), catastrophe theory, and chaos theory have been formed (Pan and Zhang 1992; Liu 2014). But there are some shortcomings of the corresponding theoretical explanation. For example, the energy theory does not give a good description of the properties of coal rocks in the equilibrium state and the coal rock rupture conditions. The strength theory only gives the sufficient conditions for rockburst but does not point out the actual conditions under which rockburst happens. The stiffness theory is only a necessary condition for the occurrence of rockburst and does not give a clear definition of the mine structure division and its composite systems (Xuelong Li et al., 2021). On the basis of these innovative theoretical results, corresponding technological measures have been formulated to provide guidance for the practical control of rockburst in roadways. However, the aforementioned studies mainly focus on theoretical analysis based on the characteristics of in situ rockburst. This study analyzes the main inducing factors of 108 rockburst events in the Yima coalfield from 2006 to 2015.
Yima coalfield (Yima City, Henan Province, China) is frequently subject to rockbursts in roadways and has ultrathick strata, which is its typical stratigraphic characteristic. On the basis of the elastic theory, Shi and Jiang (2006) analyzed the stress distribution in thick strata using a model of fixed beams considering gravity. The researcher also studied the roof stability and occurrence mechanism of abnormal pressure on an island working face under ultrathick conglomerate in Changcun coal mine, Yima coalfield. They found that thick overlying strata and hard roofs and floors are adverse geological conditions inducing rockburst, and the stress concentration during the mining of working faces will further increase the occurrence probability of rockburst. Therefore, a large number of coal pillars and large areas of suspended roof of goaf should be circumvented in the mining design.
Although coal mines in the Yima coalfield have taken various rockburst prevention measures, rockbursts still occur from time to time in roadways. According to the statistics, five coal mines (Yangcun, Gengcun, Qianqiu, Yuejin, and Changcun coal mines) in the center of the Yima coalfield had been accumulatively subject to 108 rockburst events in roadways from 2006 to 2015. These events have led to different degrees of damage to roadways, more than 11,000 m in length, dozens of casualties, and nearly 100 million yuan of direct economic losses. Therefore, based on the statistical analysis of rockburst in roadways in the Yima coalfield, the research investigated the characteristics and influencing factors of rockburst in roadways in coal seams under such conditions. The research findings can provide guidance for revealing the occurrence mechanism of rockburst in the coalfield.
2 Research Area and Materials
Yima coalfield is located in Mianchi County, Yima City, in the west of Henan Province, in which five operating mines are distributed from west to east, namely, Yangcun, Gengcun, Qianqiu, Yuejin, and Changcun coal mines. As a whole, the Yima coalfield presents an asymmetrical syncline structure, with hidden coal outcrop in the north and F16 thrust fault in the south, and this fault occurs in all of the five coal mines. The overlying conglomerate of the Yima coalfield is tens to hundreds of meters thick, and the ultrathick strata with massive structure is mainly composed of quartzite and quartz sandstone with a gravel size of 2∼500 mm. About 200 m above the coal seam is conglomerate, whose cohesive force and internal friction angle is 13.5 MPa and 29.6°, respectively. The ultrathick strata are likely to store elastic energy, and with the mining of working faces and the enlargement of the goaf, the risk of rockburst in roadways rises correspondingly, which increases the difficulty for rockburst control in roadways (Li et al., 2014; Xu et al., 2015).
The minable seams of the Yima coalfield occur in the Yima Formation of the lower Middle Jurassic series and can be divided into two seams (2-1# and 2-3# coal seams) in the majority of areas, which are merged in the deep part and expressed as 2# coal seam for short. The average thickness of the coal seam is about 25.4 m whose uniaxial compressive strength is 18.7 MPa. The immediate roof and main roof of the 2# coal seam are separately shown as mudstone and ultrathick conglomerate, while the immediate floor is comprised of interbedded mudstone and carbonaceous mudstone. Through decades of mining, the mining activity of the five coal mines in the center of the Yima coalfield has shifted to the deep merged zone of seams, and the maximum mining depth has reached 1,060 m underground. At present, the mining depths are separately 400∼600 m, 600∼800 m, 750∼980 m, 650∼1,060 m, and 600∼800 m in Yangcun, Gengcun, Qianqiu, Yuejin, and Changcun coal mines, respectively. The geological profile of the Yima coalfield is shown in Figure 1.
On the basis of site survey and data collection, the numbers of rockburst events in the roadways of the five coal mines in the center of the Yima coalfield from 2006 to 2015 are summarized in Table 1. As shown in Table 1, Qianqiu coal mine was subject to the most rockburst in roadways, up to 41 times, which accounted for 38.0% of the total number of rockburst events; it was followed by the Yuejin coal mine, which suffered from 34 rockburst events (31.5%); Meanwhile, the numbers of rockburst events in Gengcun and Changcun coal mines are similar. It can also be seen from the table that more rockburst events happened from 2010 to 2012, which was found to be the mining period of the 21,141 working face of the Qianqiu coal mine according to the site survey results and relevant data.
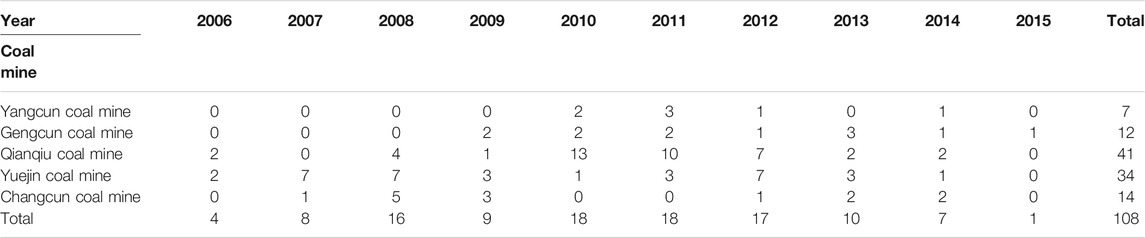
TABLE 1. Number of rockburst events in the five coal mines in the center of the Yima coalfield (2006–2015).
3 Research Methods
3.1 Data Mining of Rockburst Events
In accordance with the above analysis, the mining depths of Qianqiu and Yuejin coal mines are deeper than those of Gengcun and Changcun coal mines, and the Changcun coal mine is mined at a depth slightly deeper than the Gengcun coal mine. Figure 2A shows the statistics of rockburst events in roadways at different burial depths in the five coal mines from 2006 to 2015. As displayed in the figure, roadways at burial depths of 600∼700 m and deeper than 700 m were separately subjected to 46 and 44 rockburst events, which accounted for 42.6% and 40.7% of the total. It cannot be ignored that fewer mining works on deeper levels. It is evident that the burial depth of roadways directly influences the occurrence of rockburst.
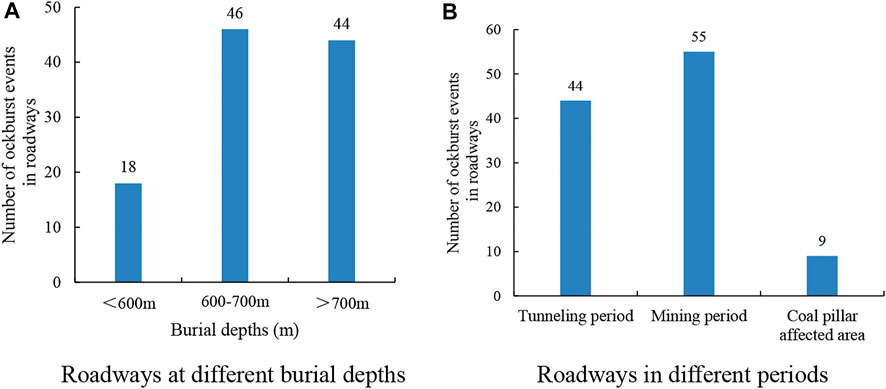
FIGURE 2. Statistics of rockburst events in roadways of the Yima coalfield from 2006 to 2015. (A) Roadways at different burial depths. (B) Roadways in different periods.
Figure 2B illustrates a statistical bar chart of rockburst events in roadways in different periods and regions in the five coal mines from 2006 to 2015. As shown in the figure, the mining period witnessed the largest number of rockburst events (55) in roadways, followed by the tunneling period (44), which separately accounted for 50.9 and 40.7% of the total. Only nine rockburst events happened in the roadways within the coal pillar-affected area, being 8.4% of the total. Therefore, according to the field feedback, a relatively large number of rockburst events happened in the area 300 m ahead of the working face, particularly the region 150 m ahead, which was an area of high occurrence frequency of rockburst. There were also many rockburst events in the area 250 m behind the tunneling face, especially the area 125 m behind, which was also an area of high occurrence frequency of rockburst. Hence, mining disturbance is an important factor inducing rockburst in roadways.
By combining the above analysis, it is revealed that areas of the Yima coalfield under the combined effects of location in the affected zone of a thrust fault, thick conglomerate in the coal seam roof, large mining depth of working faces, and mining disturbances are subject to frequent rockbursts in roadways. Limited by length, three typical rockburst events in the roadway were selected to analyze their characteristics and key inducing factors.
3.2 Field Investigation
Among the rockburst events in the roadways of the Yima coalfield, three caused severe economic losses, namely, those on 3 November 2011, 27 March 2014, and 22 December 2015. The characteristics of these three events are analyzed as follows.
3.2.1 Roadway Tunneling and Fault
The designed length of the 21,221 working face in the Qianqiu coal mine and the length of the open-off cut are separately 1,520 and 180 m. The coal seam with an average thickness of 23 m exhibits a dip angle of 10°∼14°. The working face is the seventh one in the downhill west wing of the No. 21 mining area, with the goaf of 21,181 and 21,201 working faces to the north, coal to the south, mine boundary to the west, and protective coal pillars for the main roadways to the east. The layout plan of the working face is illustrated in Figure 3.
The head entry of the 21,221 working face was tunneled along the coal seam floor and buried at about 760 m underground. The support combining cable anchors, 36U-shaped steel yieldable support, and large-scale props was used in the roadway with a cross-sectional area of 24 m2. Multiple rockburst prevention measures including deep-hole water infusion in coal seams, large-diameter pressure-relief boreholes, and deep-hole pressure-relief blasting for roof, and floor breaking were taken during tunneling.
A rockburst event happened at 19:18 p.m. on 3 November 2011, in the head entry of the 21,221 working face. According to the microseismic (MS) monitoring, 3.5 × 108 J of energy was released (ARAMIS), leading to a seismic magnitude of 4.1 (KZ-301). The event caused not only significant casualties and economic losses but also severe floor heave and deformation of the head entry. In some sections, the roadway height was only 0.5∼0.8 m, and lots of strengthened large-scale props skewed to the upper side, and the two side walls were deformed remarkably. The 36U steel yieldable support was distorted, with the legs of the shed support on the upper side sliding inward to the roadway. The minimum height of the roadway was less than 1.9 m, and the minimum width was only 2.3 m, and the roadway was closed in local sections. A site photograph of the rockburst in the local section of the roadway is shown in Figure 4.
When the rockburst event occurred, the tail entry and the head entry in the 21,221 working face were separately tunneled to 890 and 715 m, which were 382 and 73 m from the location of the rockburst. The rockburst was 98 m from the F16 thrust fault in the south. At the same time, the head entry was repaired and maintained at seven locations. Therefore, the rockburst event was mainly induced by roadway tunneling and roadway repair and maintenance, as well as the fault, which is also one of the possible inducements.
3.2.2 Coal Pillars and Tunneling Disturbance
The uphill return airway in the 21,032 working face of the Qianqiu coal mine had a designed length of 152 m and was tunneled horizontally for 28.5 m from the terminal of the diversion of the underground station. After encountering the floor of the 2# coal seam, it was tunneled upward with an inclination of +22° for a length of 48.3 m. The tunneling was turned on along the roof of the coal seam after exposing the roof.
The uphill return airway in the 21,032 working face was buried 497 m underground and supported by combining shotcreting with wire mesh, 36U-shaped steel yieldable support, and 36U strengthened pillars. The roadway had a cross-sectional area of 16.69 m2, and the slope change points were supported in combination with anchor cables, 36U-shaped steel yieldable support, and door-like scaffolds. The plan sketch of the roadway is shown in Figure 5. Rockburst prevention measures including deep-hole pressure-relief blasting and deep-hole water infusion were taken in the tunneling period.
At 11:18 am on 27 March 2014, a rockburst event happened in the uphill return airway of the 21,032 working face, for which it was measured through MS monitoring to have an energy release of 1.1 × 107 J (ARAMIS) and seismic magnitude of 1.9 (KZ-301). Apart from large casualties and economic losses, the rockburst event also caused distortion of the 36U steel yieldable support, and the two side walls of a 20 m roadway section upward from the lower slope change point were converged to different degrees, along with the floor heave. At the position 50 m from the lower slope change point, the roadway was closed, and there was only a space of 0.8 m around on the lower side. Most 36U strengthened pillars were bent. The two ventilation doors in the underground station were significantly damaged so that the gas concentration was as high as 9%. In addition, the No. 763 inclined belt conveyor roadway, the strong belt head chamber, and the hoist house in the No. 21 mining area nearby were also deformed to different degrees. Figure 6 shows a site photograph of the rockburst in the roadway.
When the rockburst event happened, the uphill return airway of the 21,032 working face was tunneled to a location 85 m from the return airway, and the station of the haulage roadway was tunneled to 45 m, which were separately 20 and 88 m from the location of the rockburst. The rockburst occurred at the roof at the position 80 m from the eastern F3-7 fault. Figure 6 depicts that there were many roadways around the tunneling face of the uphill return airway in the 21,032 working face, which was easily influenced by the stress-concentrated zone in the coal pillars. Besides, the tunneling of the station to the haulage roadway also exerted a certain disturbance on the uphill return airway. Hence, stress concentration around the coal pillars and the tunneling disturbance were the main triggers of the rockburst event.
3.2.3 Mining Disturbance
The 13,230 working face in the Gengcun coal mine extended eastward to its boundary with the Qianqiu coal mine, and it was back-to-back to the goaf of the 21,121 working face in the Qianqiu coal mine. To the north of the working face was the stopped goaf of five working faces including 13,210 and to the east and south was unmined coal, with the average burial depth of 622 m. The layout plan of the working face is shown in Figure 7. The cross section of the head entry of the 13,230 working face was supported by combining anchor cables and a 36U-shaped fully enclosed steel yieldable support, with a net cross-sectional area of 6,200 mm × 4,150 mm (width × height).
To avoid the occurrence of rockburst, many rockburst prevention measures and monitoring and early warning methods were used in the coal mine. Meanwhile, roadway support and hydraulic lift sheds were both used for advanced support of the tail entry and the head entry for 150 and 300 m separately from the working face.
A rockburst event occurred in the head entry during the mining period of the 13,230 working face of the Gengcun coal mine at 10:42 a.m. on 22 December 2015. It caused floor heave and reduction of the cross section of the head entry of 150 m long expected for the emergency exit. In addition, some electromechanical equipment, transportation facilities, and supports in the roadway were damaged or rolled over. The roadway in local areas was only 1.1 m high, and 30 of the total 35 hydraulic lift sheds were damaged. Figure 8 shows a site photograph of the rockburst.
At the moment when the rockburst happened, the 13,230 working face was advanced for 36.0 and 29.7 m in the tail entry and head entry, respectively, and the rockburst occurred within the affected area of the mining-induced stress. Because the working face was in the initial mining stage, the analysis indicates that the rockburst event was induced by factors related to the mining activities. In the geological environment, because of the induction of triggering events such as external disturbances, the regional stress field of the surrounding rock of the head entry of the 13,230 working face will be suddenly changed, and a large amount of elastic energy which is gathered in the body of coal will be suddenly released in the form of vibration, sound, and coal or rock mass burst which stands for an explosive power and seriously destroys the 150 m mining roadway from the working face (Liu et al., 2017).
The aforementioned analysis indicates that the rockburst in the roadways of the Yima coalfield was mainly characterized by remarkable floor heave, convergence of two side walls, and even closure of roadways in some sections. It was also accompanied by the deformation and damage of 36U-shaped steel supports and large-scale props. Factors including mining disturbance, roadway repair and maintenance, roof weighting, and faults may change the regional stress field in the surrounding rock of roadways, thus triggering the rockburst.
4 Microseismic Monitored the Energy Characteristics of Typical Rockburst Events
Rock is an anisotropic and heterogeneous material. During crack initiation, propagation, and coalescence, the energy stored in the rock mass is released in a stress wave form, which then triggers microseismic (MS) events (Konicek and Waclawik, 2018; Wang et al., 2018; Li et al., 2019; Niu et al., 2022). Similar to earthquakes, there is a complex activity process which is accompanied by the formation of microcracks and the release of elastic energy inside the rock mass before a rockburst (Li et al., 2016; Zhang et al., 2019; Huang et al., 2020; Huang et al., 2021). It is possible to capture precursory information by using MS monitoring equipment (Ma et al., 2018; Liang et al., 2020; Xiang Li et al., 2021; Xue et al., 2021).
Regarding to the monitoring and early warning, a local monitoring system consisting of the method of drilling, cuttings, and mine pressure observation was formed in various coal mines of the Yima coalfield. Besides, an electromagnetic radiometer, a KJ550 on-line stress monitoring system, a microseismic (MS) monitoring system, and a KZ-301 mining earthquake monitoring device were also used to capture rockburst information through multiple channels and means. In this way, a three-level early-warning system enabled by an all-around and stereoscopic monitoring network from the perspectives of coal mines, mining areas, and working faces was formed.
Monitoring data shows that when there is no rockburst of the roadway surrounding the rock, the fluctuation range of maximum MS monitoring energy is small, but each rockburst event is accompanied by a sharp increase of the MS monitoring energy. Taking the rockburst on 3 November 2011 in the Qianqiu coal mine as an example, the energy characteristics thereof through MS monitoring were analyzed. Figure 9 shows the MS-monitored energy and total frequency curves 10 days before the occurrence of the rockburst. It can be seen from the figure that before the occurrence of the rockburst, the MS monitored the maximum energy, and the total energy changed slightly, with the maximum energy of 3.5 × 107 J and its total frequency of 13, which happened on 21 October 2011. Then, 10 days before the occurrence of the rockburst, the total frequency curve of the maximum energy was found to have large fluctuations. On the day when the rockburst happened, the maximum energy increased abruptly to 3.5 × 108 J, although the total frequency was only 8.
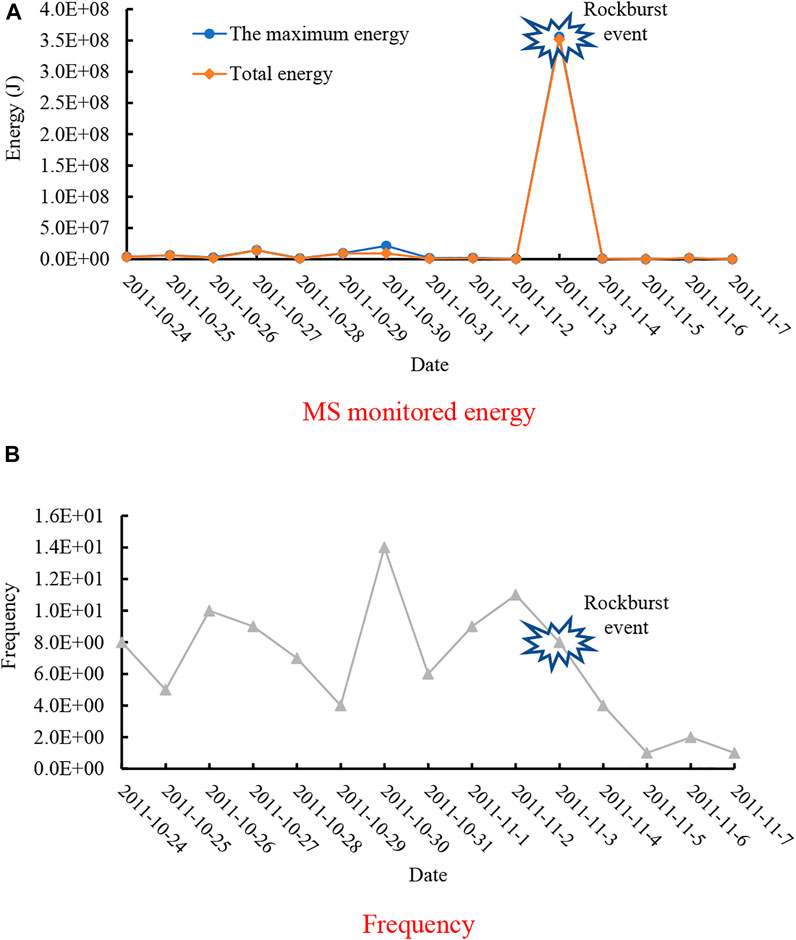
FIGURE 9. Curves of the MS-monitored energy and its frequency. (A) MS-monitored energy. (B) Frequency.
According to the aforementioned research findings, abrupt energy release is induced, and rockburst is caused in a roadway within the affected zone of a fault under the disturbance of roadway tunneling and repair. If only low energy is released abruptly, it is shown as a low-energy event in the MS monitoring, during which the rockburst of the roadway is insignificant. If the energy is released slowly, it is shown as the deformation of the surrounding rock.
5 Discussion
Based on the 108 rockburst events in the Yima coalfield during 2006–2015, the influence of buried depth, roadway driving, and working face mining on rockburst is obtained. There are ultrathick overlying strata in the Yima coalfield. After coal mining, the roof is not easy to collapse and accumulates a lot of elastic energy. Under the action of dynamic load factors such as mining disturbance, roadway repair, blasting within the roadway and so on, the stress field of the roadway surrounding rock will be changed, and the plastic zone of the roadway surrounding rock expands rapidly accompanied by the sudden releasing of accumulated elastic energy (Afraei et al., 2018; Duan et al., 2019; Hosseini et al., 2013). This burst dynamic disaster will lead to severe floor heave, convergence of two side walls, and severe damage of supporting body. Compared to the existing research, the research results of this study illustrate the mechanism of typical rockburst events in the Yima coalfield and provide support for the rockburst theory.
The inducing factors of roadway rockburst are not limited to the mentioned factors, faults, folds, and working face pressure may also induce rockburst (Sainoki and Mitri, 2014; Sainoki and Mitri, 2016; Zhao et al., 2018; He et al., 2022). Under the action of external high stress, coal near the roadway accumulates a large amount of elastic energy. After external disturbance, the regional stress field of the surrounding rock of the roadway changes suddenly, causing the elastic energy in the coal and rock to be released in the form of vibration, sound, and coal and rock throwing, and explosive dynamic failure phenomenon occurs, so the cases of stress rockburst cannot be completely ruled out in mines located in the Yima coalfield. Effective measures should be taken so as to prevent the occurrence of rockburst and promote the safe mining of rockburst mines. Many materials and equipment have been developed and used for the prevention of roadway rockbursts. In addition, to reduce elastic energy accumulation, coal seam properties can be changed by means of water injection and pressure relief by large-diameter boreholes. In order to reduce the disturbance effect, mining and roadway repair are not carried out simultaneously and lower the mining speed. The prevention and control mechanism of rockburst should be researched in the future research.
6 Conclusion
This study aimed to obtain the rockburst characteristics and influence factors of roadways in the Yima coalfield by analyzing the roadway rockburst events in the Yima coalfield during 2006–2015 and microseismic monitoring energy. Compared with the published literature, we mainly obtained the following conclusions:
(1) Under ultrathick strata, the Yima coalfield is affected by the thrust fault so that the roadways are located in a high-stress environment. In the study, factors including mining disturbance, roadway repair and maintenance, and blasting in roadways may change the regional stress fields in the surrounding rock and induce rockburst. The rockburst shows the following characteristics, including serious floor heave, remarkable convergence of two side walls, remarkable damage of support, and even closure of roadways.
(2) Rockburst in roadways mainly occurs in the mining period of working faces and at locations of large burial depths within the affected zone of mining-induced stress, accompanied by abrupt energy release.
Data Availability Statement
The original contributions presented in the study are included in the article/Supplementary Material, further inquiries can be directed to the corresponding author.
Author Contributions
All authors listed have made a substantial, direct, and intellectual contribution to the work and approved it for publication.
Funding
This work was partially supported by the National Natural Science Foundation of China (Grant No. 52104126), the Program for Innovative Research Team (in Science and Technology) in the University of Henan Province (22IRTSTHN009), the Key Scientific Research Project of Higher Education Institutions of Henan Province (Grant No. 21A440001), and the Doctoral Cultivation Fund of Henan University of Engineering (Grant No. DKJ2019003).
Conflict of Interest
Author GZ was employed by the company Henan Energy & Chemical Industry Group Co. Ltd.
The remaining authors declare that the research was conducted in the absence of any commercial or financial relationships that could be construed as a potential conflict of interest.
Publisher’s Note
All claims expressed in this article are solely those of the authors and do not necessarily represent those of their affiliated organizations, or those of the publisher, the editors, and the reviewers. Any product that may be evaluated in this article, or claim that may be made by its manufacturer, is not guaranteed or endorsed by the publisher.
References
Afraei, S., Shahriar, K., and Madani, S. H. (2018). Statistical Assessment of Rock Burst Potential and Contributions of Considered Predictor Variables in the Task. Tunnelling Underground Space Tech. 72 (11), 250–271. doi:10.1016/j.tust.2017.10.009
Anderson, T. L. (2017). Fracture Mechanics Fundamentals and Applications. Fourth Edition. Boca Raton: Boca Raton CRC Press.
Cao, G. M., Hao, Z., Liu, H. T., Guo, L. F., Zhao, X. D., and Zhang, G. H. (2019). Impact Failure Mechanism of Mining Roadway under Ultra-thick Conglomerate. J. Mining Saf. Eng. 36 (2), 290–297. doi:10.13545/j.cnki.jmse.2019.02.010
Cook, N. G. W., Hoek, E. P., Pretorius, J. P. G., Ortlepp, W. D., and Salamon, M. D. G. (1966). Rock Mechanics Applied to the Study of Rockbursts. J. South Afr. Inst. Mining Metall. 66 (10), 435–528.
Dai, L., Pan, Y., Li, Z., Wang, A., Xiao, Y., Liu, F., et al. (2021). Quantitative Mechanism of Roadway Rockbursts in Deep Extra-thick Coal Seams: Theory and Case Histories. Tunnelling Underground Space Tech. 111, 103861. doi:10.1016/j.tust.2021.103861
Duan, K., Ji, Y., Wu, W., and Kwok, C. Y. (2019). Unloading-induced Failure of Brittle Rock and Implications for Excavation-Induced Strain Burst. Tunnelling Underground Space Tech. 84, 495–506. doi:10.1016/j.tust.2018.11.012
Frith, R., Reed, G., and Jones, A. (2020). A Causation Mechanism for Coal Bursts during Roadway Development Based on the Major Horizontal Stress in Coal: Very Specific Structural Geology Causing a Localised Loss of Effective Coal Confinement and Newton's Second Lawfic Structural Geology Causing a Localised Loss of Effective Coal Confinement and Newton’s Second Law. Int. J. Mining Sci. Tech. 30, 39–47. doi:10.1016/j.ijmst.2019.12.018
Ghorbani, M., Shahriar, K., Sharifzadeh, M., and Masoudi, R. (2020). A Critical Review on the Developments of Rock Support Systems in High Stress Ground Conditions. Int. J. Mining Sci. Tech. 30, 555–572. doi:10.1016/j.ijmst.2020.06.002
Gong, F., Wang, Y., Wang, Z., Pan, J., and Luo, S. (2021). A New Criterion of Coal Burst Proneness Based on the Residual Elastic Energy index. Int. J. Mining Sci. Tech. 31, 553–563. doi:10.1016/j.ijmst.2021.04.001
He, Z.-L., Lu, C.-P., Zhang, X.-F., Guo, Y., Wang, C., Zhang, H., et al. (2022). Research on Mechanisms and Precursors of Slip and Fracture of Coal-Rock Parting-Coal Structure. Rock Mech. Rock Eng. doi:10.1007/s00603-021-02724-4
Hosseini, N., Oraee, K., Shahriar, K., and Goshtasbi, K. (2013). Studying the Stress Redistribution Around the Longwall Mining Panel Using Passive Seismic Velocity Tomography and Geostatistical Estimation. Arab J. Geosci. 6 (5), 1407–1416. doi:10.1007/s12517-011-0443-z
Huang, F., Cao, Z., Jiang, S.-H., Zhou, C., Huang, J., and Guo, Z. (2020). Landslide Susceptibility Prediction Based on a Semi-supervised Multiple-Layer Perceptron Model. Landslides 17, 2919–2930. doi:10.1007/s10346-020-01473-9
Huang, F., Tao, S., Chang, Z., Huang, J., Fan, X., Jiang, S.-H., et al. (2021). Efficient and Automatic Extraction of Slope Units Based on Multi-Scale Segmentation Method for Landslide Assessments. Landslides 18, 3715–3731. doi:10.1007/s10346-021-01756-9
Jiang, L., Sainoki, A., Mitri, H. S., Ma, N., Liu, H., and Hao, Z. (2016). Influence of Fracture-Induced Weakening on Coal Mine Gateroad Stability. Int. J. Rock Mech. Mining Sci. 88, 307–317. doi:10.1016/j.ijrmms.2016.04.017
Jiang, L., Wu, Q., Wu, Q., Wang, P., Xue, Y., Kong, P., et al. (2019). Fracture Failure Analysis of Hard and Thick Key Layer and its Dynamic Response Characteristics. Eng. Fail. Anal. 98, 118–130. doi:10.1016/j.engfailanal.2019.01.008
Keneti, A., and Sainsbury, B.-A. (2018). Review of Published Rockburst Events and Their Contributing Factors. Engineering Geology. 246, 361–373. doi:10.1016/j.enggeo.2018.10.005
Konicek, P., Soucek, K., Stas, L., and Singh, R. (2013). Long-hole Destress Blasting for Rockburst Control during Deep Underground Coal Mining. Int. J. Rock Mech. Mining Sci. 61, 141–153. doi:10.1016/j.ijrmms.2013.02.001
Konicek, P., and Waclawik, P. (2018). Stress Changes and Seismicity Monitoring of Hard Coal Longwall Mining in High Rockburst Risk Areas. Tunnelling Underground Space Tech. 81, 237–251. doi:10.1016/j.tust.2018.07.019
Li, B. F., Li, X. J., and Ren, Y. K. (2014). Experimental and Theoretical Study on Rock Burst Inducement by Movement of Super-thick Conglomerate Strata Overlying Working Face. J. China Coal Soc. 39 (S1), 31–37. doi:10.13225/j.cnki.jccs.2013.0050
Li, Z.-l., Dou, L.-m., Cai, W., Wang, G.-f., Ding, Y.-l., and Kong, Y. (2016). Roadway Stagger Layout for Effective Control of Gob-Side Rock Bursts in the Longwall Mining of a Thick Coal Seam. Rock Mech. Rock Eng. 49 (2), 621–629. doi:10.1007/s00603-015-0746-6
Li, P.-X., Feng, X.-T., Feng, G.-L., Xiao, Y.-X., and Chen, B.-R. (2019). Rockburst and Microseismic Characteristics Around Lithological Interfaces under Different Excavation Directions in Deep Tunnels. Eng. Geology. 260, 105209. doi:10.1016/j.enggeo.2019.105209
Liang, Z., Xue, R., Xu, N., and Li, W. (2020). Characterizing Rockbursts and Analysis on Frequency-Spectrum Evolutionary Law of Rockburst Precursor Based on Microseismic Monitoring. Tunnelling Underground Space Tech. 105, 103564. doi:10.1016/j.tust.2020.103564
Liu, H. T., Hao, Z., Wu, X. Y., Zhao, X. D., Guo, L. F., and Ma, Z. Y. (2017). Mechanism of Blast Disaster Induced by Instantaneous Malignant Expansion of Plastic Zone. J. China Coal Soc. 42 (6), 1392–1399. doi:10.13225/j.cnki.jccs.2016.1145
Liu, S. H. (2014). Nonlinear Catastrophy Model and Chaotic Dynamic Mechanism of Compound Coal-Rock Unstable Failure under Coupled Static-Dynamic Loading. J. China Coal Soc. 39 (2), 292–300. doi:10.13225/j.cnki.jccs.2013
Ma, T.-H., Tang, C.-A., Tang, S.-B., Kuang, L., Yu, Q., Kong, D.-Q., et al. (2018). Rockburst Mechanism and Prediction Based on Microseismic Monitoring. Int. J. Rock Mech. Mining Sci. 110, 177–188. doi:10.1016/j.ijrmms.2018.07.016
Małkowski, P., and Niedbalski, Z. (2020). A Comprehensive Geomechanical Method for the Assessment of Rockburst Hazards in Underground Mining. Int. J. Mining Sci. Tech. 30, 345–355. doi:10.1016/j.ijmst.2020.04.009
Mark, C., and Gauna, M. (2021). Pillar Design and Coal Burst Experience in Utah Book Cliffs Longwall Operations. Int. J. Mining Sci. Tech. 31, 33–41. doi:10.1016/j.ijmst.2020.12.008
Newman, C., and Newman, D. (2021). Numerical Analysis for the Prediction of Bump Prone Conditions: A Southern Appalachian Pillar Coal Bump Case Study. Int. J. Mining Sci. Tech. 31, 75–81. doi:10.1016/j.ijmst.2020.12.020
Niu, W., Feng, X.-T., Feng, G., Xiao, Y., Yao, Z., Zhang, W., et al. (2022). Selection and Characterization of Microseismic Information about Rock Mass Failure for Rockburst Warning in a Deep Tunnel. Eng. Fail. Anal. 131, 105910. doi:10.1016/j.engfailanal.2021.105910
Pan, J. F., Ning, Y., Mao, D. B., Lan, H., Du, T. T., and Peng, Y. W. (2012). Theory of Rockburst Start-Up during Coal Mine. Chin. J. Rock Mech. Eng. 31 (3), 586–596.
Pan, Y. S., Dai, L. P., Li, G. Z., and Li, Z. H. (2021). Study on Compound Disaster of Rock Burst and Roof Falling in Coal Mines. J. China Coal Soc. 46 (1), 112–122. doi:10.13225/j.cnki.jccs.2020.0557
Pan, Y. S., and Zhang, M. T. (1992). The Study of Coalburst by Catastrophic Theory. J. Fuxin Mining Inst. 11 (1), 12–18.
Pang, L. L., Xu, X. F., Si, L., Zhang, H., and Li, Z. K. (2016). Analysis of Prevention Mechanism of Upper Protective Seam Mining on Rock Rockburst Induced by Thick Conglomerate. Rock Soil Mech. 37 (S2), 120–128. doi:10.16285/j.rsm.2016.S2.014
Procházka, P. P. (2004). Application of Discrete Element Methods to Fracture Mechanics of Rockbursts. Eng. Fracture Mechanic 71, 601–618.
Qi, Q. X., and Dou, L. M. (2008). Theory and Technology of Rockburst. Xuzhou: China University of Mining and Technology Press.
Ranjith, P. G., Zhao, J., Ju, M., De Silva, R. V. S., Rathnaweera, T. D., and Bandara, A. K. M. S. (2017). Opportunities and Challenges in Deep Mining: A Brief Review. Engineering 3, 546–551. doi:10.1016/j.eng.2017.04.024
Sainoki, A., and Mitri, H. S. (2014). Dynamic Behaviour of Mining-Induced Fault Slip. Int. J. Rock Mech. Mining Sci. 66, 19–29. doi:10.1016/j.ijrmms.2013.12.003
Sainoki, A., and Mitri, H. S. (2016). Dynamic Modelling of Fault Slip Induced by Stress Waves Due to Stope Production Blasts. Rock Mech. Rock Eng. 49 (1), 165–181. doi:10.1007/s00603-015-0721-2
Shi, H., and Jiang, F. X. (2006). Analysis on Rupture of Hard and Massive Overlying Strata in Fully-Mechanized Sublevel Caving Face. Chin. J. Geotechnical Eng. 28 (4), 525–528.
Tsirel', S. V., and Krotov, N. V. (2001). Probability Interpretation of Indirect Risk Criteria and Estimate of Rock-Burst hazard in Mining Anthracite Seams. J. Mining Sci. 37 (3), 240–260. doi:10.1023/a:1013194110443
Wang, Z., Ning, J., and Ren, H. (2018). Frequency Characteristics of the Released Stress Wave by Propagating Cracks in Brittle Materials. Theor. Appl. Fracture Mech. 96, 72–82. doi:10.1016/j.tafmec.2018.04.004
Wang, G., Gong, S., Dou, L., Cai, W., Yuan, X., and Fan, C. (2019). Rockburst Mechanism and Control in Coal Seam with Both Syncline and Hard Strata. Saf. Sci. 115, 320–328. doi:10.1016/j.ssci.2019.02.020
Wasilewski, S. (2020). Gas-dynamic Phenomena Caused by Rock Mass Tremors and Rock Bursts. Int. J. Mining Sci. Tech. 30, 413–420. doi:10.1016/j.ijmst.2020.03.012
Xiang Li, X., Mao, H., Li, B., and Xu, N. (2021). Dynamic Early Warning of Rockburst Using Microseismic Multi-Parameters Based on Bayesian Network. Eng. Sci. Technol. Int. J. 24, 715–727. doi:10.1016/j.jestch.2020.10.002
Xu, S. M., Li, S. Y., Li, D. X., Zhang, W. P., Lian, J., and Wei, Q. D. (2015). Geological Laws of Rock Burst Occurrence in Yima coalfield. J. China Coal Soc. 40 (9), 2015–2020. doi:10.13225/j.cnki.jccs.2015.0715
Xue, R., Liang, Z., and Xu, N. (2021). Rockburst Prediction and Analysis of Activity Characteristics within Surrounding Rock Based on Microseismic Monitoring and Numerical Simulation. Int. J. Rock Mech. Mining Sci. 142, 104750. doi:10.1016/j.ijrmms.2021.104750
Xuelong Li, X., Chen, S., Wang, E., and Li, Z. (2021). Rockburst Mechanism in Coal Rock with Structural Surface and the Microseismic (MS) and Electromagnetic Radiation (EMR) Response. Eng. Fail. Anal. 124, 105396. doi:10.1016/j.engfailanal.2021.105396
Zhang, M. T. (1987). Instability Theory and Mathematical Model for Coal/rock Bursts. Chin. J. Rock Mech. Eng. 6 (3), 197–204.
Zhang, C., Canbulat, I., Hebblewhite, B., and Ward, C. R. (2017). Assessing Coal Burst Phenomena in Mining and Insights into Directions for Future Research. Int. J. Coal Geology. 179, 28–44. doi:10.1016/j.coal.2017.05.011
Zhang, S., Li, Y., Shen, B., Sun, X., and Gao, L. (2019). Effective Evaluation of Pressure Relief Drilling for Reducing Rock Bursts and its Application in Underground Coal Mines. Int. J. Rock Mech. Mining Sci. 114, 7–16. doi:10.1016/j.ijrmms.2018.12.010
Zhao, T.-b., Guo, W.-y., Tan, Y.-l., Yin, Y.-c., Cai, L.-s., and Pan, J.-f. (2018). Case Studies of Rock Bursts under Complicated Geological Conditions during Multi-Seam Mining at a Depth of 800 M. Rock Mech. Rock Eng. 51, 1539–1564. doi:10.1007/s00603-018-1411-7
Keywords: rockburst, energy, microseismic monitoring (MS), mining roadway, coal seam
Citation: Hao Z, Sun G and Zhang G (2022) Mechanism and Inducing Factors of Rockburst Events of Roadways Under Ultrathick Strata. Front. Earth Sci. 10:860929. doi: 10.3389/feart.2022.860929
Received: 24 January 2022; Accepted: 10 February 2022;
Published: 09 March 2022.
Edited by:
Faming Huang, Nanchang University, ChinaReviewed by:
Lukasz Wojtecki, Central Mining Institute, PolandJia Housheng, Henan Polytechnic University, China
Copyright © 2022 Hao, Sun and Zhang. This is an open-access article distributed under the terms of the Creative Commons Attribution License (CC BY). The use, distribution or reproduction in other forums is permitted, provided the original author(s) and the copyright owner(s) are credited and that the original publication in this journal is cited, in accordance with accepted academic practice. No use, distribution or reproduction is permitted which does not comply with these terms.
*Correspondence: Zhen Hao, aGFvemhlbjA5QDE2My5jb20=