- 1XPS, Falconbridge, ON, Canada
- 2Queen’s Facility for Isotope Research, Queen’s University, Kingston, ON, Canada
Activities performed at mineral processing operations are capable of producing significant quantities of dust. To ensure that regulatory compliance is maintained throughout operation, dust levels are monitored by routine analysis of air filter samples. Determining the quantity and type of particulate matter present in dust allows for the operation to identify the sources of dust and where warranted, implement a dust suppressant strategy. Conventional methods of analysis, such as chemical assay, are unable to rigorously differentiate between phases containing the same elements and may result in ambiguity related to the identification of dust sources. By incorporating Quantitative Evaluation of Materials by Scanning Electron Microscope (QEMSCAN) into their routine monitoring programs, the Sudbury INO smelter has greatly improved characterization of the Ni and Co dust emissions generated at the operation. To determine specific activities responsible for emissions, bulk particulate matter was sampled from several key locations at the smelter that regularly produce dust. QEMSCAN was able to distinguish between critical subspecies of Ni and Co and mineralogical, and chemical signatures in the dust that are representative of locational activity were established. An apportionment of elements contributed by each source was calculated based on this information. The results of the characterization study assisted personnel in developing strategies to mitigate dust emissions that originate from the Smelter operations, and have general implications to occupational hygiene and environmental risk assessment.
Introduction
With a mining history spanning nearly two centuries, the Sudbury district serves as a noteworthy example of the success that monitoring initiatives have on environmental restoration and improved industrial hygiene (Gunn, 1995 and Gunn et al., 1995). The region produces a significant portion of the world’s Ni, Cu, and platinum-group elements (PGE). Its unique wealth of mineral deposits is owed to a 1.85 Ga impact event that concentrated chalcophile metals into mineable, footwall embayments along the perimeter of the basin (Dietz, 1964). Sudbury is home to eight active mines, two mills, two smelters, and one refinery, and mining in the area remains prosperous as expansions are targeted through the onset of deeper drilling programs. Although the impacts of early mining have taken an environmental toll, the Sudbury district is well-known for its remarkable re-greening and rehabilitation efforts (Lautenbach et al., 1995; Kramer et al., 2018; Boerchers, et al., 2016). These transformations are, in part, due to advancements in smelting technology to reduce fugitive SO2 emissions (e.g., pyrrhotite rejection to the smelter feed and installation of roasters circuits; Schonewille et al., 2005) and the introduction of aggressive monitoring systems aimed at reducing overall particulate matter (PM) emissions that reach the environment and neighbouring communities (Potvin, 2007). Historical data shows that annual SO2 emissions at the smelters in Sudbury have decreased significantly from >300 to <50 kilotons per year in the last 30 years (Potvin, 2007; Wren, 2012).
The current study described here provides a method for detailed characterization of PM and sources of PM as a means of monitoring and further reducing emissions. Continued monitoring of PM emissions is critical for limiting the environmental impacts of mining in Sudbury, and ensuring the health and safety of workers and local community members. In addition to SO2, PM originating from operations can contain metals or compounds that are toxic (e.g., Ni, Pb, Cd, As), and can be composed of particles that are fine enough to penetrate lower regions of the respiratory tract when inhaled. Fine (<2.5 µm) dust is also easily dispersed, has potential to travel several kilometers from source, and can be deposited amongst various environmental systems where further transport or biological uptake is possible (e.g., soils, rivers, lakes, and community property). Environmental and risk assessment studies performed for the Sudbury area (Wren, 2012) have shown that metal concentrations in soil samples from the Sudbury region increase closer to mining operations due to deposition from airborne PM. Despite the substantial re-greening that has been done in the area, biodiversity and recovery of plant species have not reached full capacity at locations in close proximity to operations.
At mining operations in Sudbury, Ni and its compounds are abundantly present in mined and processed materials, and dust containing these products poses environmental and occupational health risks if not properly contained. Due to increased rates of repository cancers in Ni refinery workers (Grimsrud et al., 2000 and Grimsrud et al., 2002; Sivulka and Seilkop, 2009 and Sivulka et al., 2014), Ni oxide and Ni subsulfide (Ni3S2) have been classified as carcinogenic substances (Nickel Institute, 2008). In addition, Ni processed in Sudbury can be sourced from ore that contains other potentially harmful metals such as cobalt (Co). Although small amounts of Co are essential nutrients for some plants and animals, some Co compounds can introduce adverse health effects when present at elevated levels (Shirakawa et al., 1989; Lauwerys and Lison, 1994; Barceloux and Barceloux, 1999), complicating exposure to Ni.
In compliance with government mandated regulations (EPA, 1990), operations in Sudbury may implement environmental management systems (ISO 14001) to monitor air quality onsite and in areas surrounding facilities. Sampling of particulate matter (PM) is achieved through collection of dust using high-volume air sampling stations to evaluate overall air quality (workplace and ambient), and through personal sampling pumps to assess time-weighted exposure of workers to emissions. Suspended particulates (SP) and total metal contents are routinely assayed according to the recommended analytical procedures described in ISO 14001 and 15202 (determination of particle loading through gravimetric analysis, and total metal content by inductively coupled plasma mass spectrometry/optical emission spectroscopy; ICP-MS/OES) (ISO 2004; ISO 2010; ISO 2015). If high levels of particulates or metals are observed, mitigation strategies are carried-out to avoid reaching exceedances. In cases where the source of the elevated concentrations cannot be identified, mitigation strategies may not be effective and activities will be suspended. To effectively mitigate emissions while maintaining production, it is essential to understand the origins of the dust and the dispersion patterns relative to activities performed at the operation. Commonly this will require speciation of metals through complex methods of analysis not included in standard monitoring programs.
The Sudbury Integrated Nickel Operations (Sudbury INO) Smelter, located in Falconbridge, Ontario, actively participates in routine dust monitoring following the guidelines set in Operations manual for air quality monitoring in Ontario (Ministry of the Environment, Conservation and Parks, 2009). For more in-depth assessment, the operation now incorporates the use of Quantitative Evaluation of Materials by Scanning Electron Microscope (QEMSCAN) for characterizing particulate matter. The system, which uses energy dispersive X-rays to classify dust particulates, is novel to the field industrial monitoring, and has proven to be exceptional for characterizing Ni and Co in solid particulate matter >2.5 µm. Combining QEMSCAN analysis with methods of chemical speciation is advantageous because it provides particle-specific information relating to the composition, grain size, and morphology. Using QEMSCAN to analyze 24-h high-volume air filters stationed at the smelter and in the community has allowed the operation to well-characterize dust emissions and determine which types of Ni and Co consistently enter the surrounding community when near exceedances are recorded (Kelvin and Kormos, 2015). Emissions that have potential to reach the community, if not properly contained, include Ni concentrate and custom feed products which enter the smelting process, intermediate smelted fugitive emissions, and Ni matte (end product of smelting). The compositions of these bulk materials have been thoroughly characterized using QEMSCAN in order to identify PM originating from the materials. However, emissions from activities that produce rapidly changing fugitive emissions (e.g., oxidation of particulates from smelting and pouring of molten matte and slag) cannot be sampled in bulk form, and characteristics of these emissions are not well understood.
The objective of the current study was to determine the specific origins of the Ni and Co dust within the Falconbridge smelter in order to establish the most effective mitigation strategies against fugitive PM emissions. To better understand critical emission points associated with specific activity, a large-scale sampling campaign was executed onsite. For this campaign, stationary personal sampling pumps were assembled at key locations throughout the smelter, rather than collecting bulk samples. This type of sampling is not ideal for assessment of occupational or environmental exposure, but was performed with the objective of determining the characteristic signatures of emission sources. A total of 98 dust samples, representative of 24-h periods, were taken over several days at each location, and the dust was characterized using a combination of QEMSCAN and ICP-MS. The overall study completed by Sudbury INO: 1) provides a good example of the value that quantitative mineralogy can add to dust characterization; 2) serves as an internal sampling model for baseline dust monitoring programs aimed at reducing emissions at large-scale industrial operations; and 3) provides information that can be combined with studies designed to assess long-term impacts of dust exposure on environmental and occupational health.
Smelter Operation and Possible Sources of Dust
For context, a general description of Sudbury INO’s Smelter operations and the materials that were handled onsite during particulate sampling are briefly summarized. Currently, the feed supplied to the smelter includes a mixture of Ni concentrate from Raglan mine, Ni concentrate from the Strathcona mill and a variety of custom feed materials (Schonewille et al., 2005). In both Strathcona and Raglan concentrates, pentlandite is the main Ni-bearing phase and represents 20%–30% of the mass of the concentrates. The remaining phases present in the concentrates include chalcopyrite, pyrrhotite, and a variety of silicate and oxide gangue minerals. The custom feed materials are composed of recycled Ni and Co products and typically contain combinations of metallics and complex Ni- and Co-bearing phases.
The treated concentrates are subjected to high temperature roasting to remove sulfur. The sulfur is collected in the form of SO2 off-gas and directed to the acid plant. Depending on composition, the custom feed materials are either blended with the Ni concentrate slurry prior to roasting, or fed to a calciner to produce a secondary smelting feed. The roasted product (calcine) and some secondary feed is subsequently melted in the electric furnace, resulting in a primary matte containing elevated contents of Fe (furnace matte). To reduce the Fe content and achieve pay metal grade recovery, the furnace matte is passed through a series of converters consisting of two hybrid vessels and two finishing vessels. A portion of the secondary feed bypasses the electric furnace and is directly fed to the converter aisle (Schonewille et al., 2005). From the finishing vessel, the cast matte is passed to matte granulation. The finished matte product is composed of a mixed Ni-Cu sulfide phase (containing low levels of Fe) with minor proportions of heazlewoodite (Ni3S2) and Ni, Fe, Cu alloys.
QEMSCAN analysis of a PM collected at high-volume sampling stations and materials entering the smelting process over several years has indicated that Ni and Co phases present in the dust originating from operations can be grouped in four broad categories:
• Ni concentrate consisting of mostly pentlandite;
• Ni matte (includes Ni-Cu-Fe-S matte, Ni sulfide, minor Ni sulfate and Ni-Cu-Co metals);
• Custom feed materials, which can contain Ni and Co–Cr alloys, Al Co oxide, and other Ni metals;
• Ni and Co oxides containing varying degrees of Ni, Co and Fe that are likely produced through intermediate smelting processes. These phases include Ni-Fe oxide, Ni oxide, Fe (Ni, Co) oxide and Co oxide. These groupings are based on compositions of source materials (matte, concentrate, and custom feed products) determined through QEMSCAN. Nickel and Co oxides are not abundant in typical products handled at the smelter. It is suspected that the oxides are intermediate phases created during the high-temperature smelting process. Airborne molten droplets that originate from the smelting process have the potential to oxidize quickly due to increased surface area. Because this material cannot be sampled directly, the portable stations were assembled near smelting activities to determine which activities are producing Ni and Co oxide dust.
Methodology
Sampling
The main points of activity where samples were collected include the following (see Figure 1): 1) the shuttle conveyor gallery that carries materials into the smelter (shuttle); 2) the calciner; 3) hybrid converters (CA#7/8); 4) finishing converter (CA#5/6); 5) platform adjacent to converter aisles; 6) area above the roasters and converter aisles where transport of dust is expected to occur through convective motion (Roof Fan).
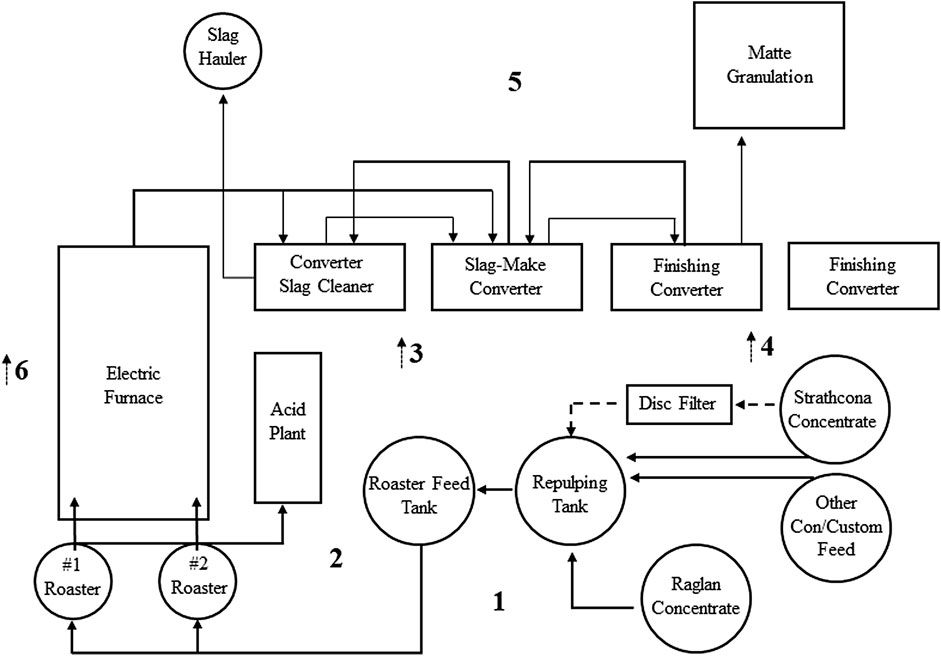
FIGURE 1. Schematic of plant operations at the Sudbury INO Smelter (modified from Schonewille et al., 2005). Numbers represent the sampling points used for the dust monitoring campaign; 1) Shuttle; 2) Calciner; 3) CA #7/8; 4) CA #5/6; 5) Platform (North); 6) Roof Fan (arrow indicates location on upper floor).
Make-shift, portable stations were assembled at each location using size-selective personal monitoring pumps (p. Particulate samples were collected on versapor silica based filters. Samples were taken every 24 h. Two samplers at each location were assembled because chemical assays require digestion of the entire filter sample. The samplers were operated in parallel so that both QEMSCAN and chemical assays could be performed on filter samples from each location. In the shuttle and calciner locations, 22 days of sampling was conducted, and at the remaining locations, 14 days of sampling was conducted.
Analysis
Particle loading (total mass of particulate) was determined gravimetrically by weighing each filter before and after dust collection using a microbalance. Chemical assays were performed on one sample from each sampling day/location using hot-acid digestion (HCl, HNO3, and HF) followed by ICP-MS for total extractable metals. Analysis was completed at the Sudbury INO chemistry lab in Falconbridge, Ontario.
QEMSCAN analysis was completed on the second sample from each sampling day/location. QEMSCAN is an SEM-based automated system that produces particle maps (color coded by mineral) through the collection of rapidly acquired X-rays. QEMSCAN is capable of measuring thousands of particles over a relatively short period, increasing the population size of the dataset. This rapid phase determination is useful for measuring air samples with low concentrations of Ni, although long measurement times are required to achieve the appropriate counting statistics. The system that was used is a Quanta 650 field emission gun (FEG) QEMSCAN instrument and provided the resolution required for detailed characterization of the dust particulates. X-ray spectra are collected at a predefined spacing that can be set to as low as 0.25 μm. The X-ray spectra generated at each point was statistically matched to corresponding groupings compiled in the species identification protocol (SIP); the colour-coded maps were produced through this data. The maps and associated data files quantify modal mineralogy and grain size (Gottlieb et al., 2000). All measurements were completed at the XPS facility in Falconbridge, Ontario.
Sample preparation for the QEMSCAN analysis was kept to a minimum to avoid losing the fine particulates. A section of each filter was placed on a 30 mm epoxy puck using double sided tape and then carbon coated. The measurements were performed directly on the filter without any additional preparation. The operating conditions for measurements included a beam current of 10 nA, spot size of ∼500–600 nm, and an accelerating voltage of 25 kV. The measurements were completed in field image mode using a pixel spacing of 0.5 µm producing a field size of 100 by 100 µm. A SIP that was developed through extensive characterization of the products handled at the Sudbury smelter was used for the measurements. SIP entries were built based on a combination of compositions determined for the materials that are processed through the smelter (Raglan and Strathcona Ni concentrate, Ni matte, and custom feed products), and analysis of particulate matter collected from the Smelter vicinity over several years. The compositions of the bulk materials were determined using a combination of QEMSCAN, Electron Probe Microanlaysis (EPMA) and X-ray Diffraction (XRD).
Because the filter samples are not polished flat, X-ray scatter from neighbouring filter fibers can be detected during measurement of particulates. Interfering X-rays from the filter fibers need to be incorporated into the SIP algorithms. For instance, Si and O are a main components of the filter fibers and the SIP entries for the Ni and Co phases were adjusted to allow the appropriate level of Si and O to the definition. In instances where Si is a chemical constituent of the particulate, the Si level in the particulate is estimated by subtracting the average Si X-ray counts in the filter fibers from the total Si X-ray counts in the particulate. Because O cannot be quantified in a similar manner, discrimination between Ni/Fe/Co oxides and metals is achieved by incorporating the backscatter electron (BSE) intensity into the SIP definition which were set based on intensities measured for metal and oxide standards. Due to the similar chemistry, quartz cannot be reliably distinguished from Si-based filter fibers. Quartz is not included in the overall data set, but is not a focus of this study.
3 Results
Particle Loading and Chemical Assays
In general, the metal content of the particulates increases with increasing particle loading. The shuttle samples contain the highest recorded particle loading, where it reaches 18 mg/filter on day three of sampling (Figure 2, Table 1) and 8 mg/filter on day nine of sampling. The shuttle area also contains the highest levels of Fe, Ni, and Cu reaching 2.8 mg/filter. In the shuttle samples, particle loading occurs with increased Fe and Ni content. The converter aisle samples contain the most consistently elevated levels of particle loading (1–6.5 mg/filter). At this location, particle loading increases with Ni + Cu + Fe + S content (individual metal content reaches 0.5 mg/filter). In the remaining samples, particle loading is < 2 mg/filter and the metal content is < 0.2 mg/filter.
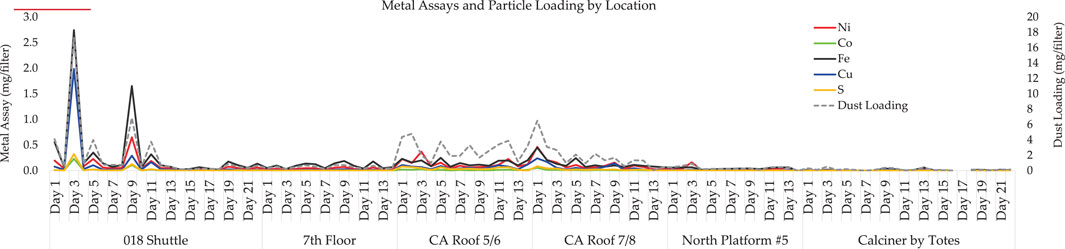
FIGURE 2. Metal assays (left axis) and particle loading (right axis) in PM collected at portable stations throughout the smelter.
QEMSCAN
Because the sampling was designed to purposely collect Ni and Co emissions, the Ni and Co phases contribute to significant proportions of the overall particulate matter in each sample (>50% in some cases). Non-Ni- and Co-bearing PM consist of a variety of Mg, Al, and K silicate minerals (gangue from concentrates or ambient dust such as olivine, serpentine, and orthoclase), gypsum, Fe oxide and Fe silicate minerals (magnetite and fayalite slag), chalcopyrite, pyrrhotite, and other metals and alloys containing Ni, Cu and/or Zn. In some cases, the phases present in the dust are specific to location. In the shuttle area more Ca oxide (likely lime) is present due to the addition of lime to the calciner. In the converter aisle and calciner, more non-Ni and Co metallic phases are present (Zn and Cu alloys and sulfates). Trace amounts of elemental sulfur (or condensed SO2 droplets) were consistently detected.
The types of Ni and Co phases detected include penlandite (from Ni concentrate), finished granulated Ni matte (Ni-Cu-Fe-S), intermediate matte (Ni sulfide/Ni-Cu-Fe-S), Ni and Co oxides and metals (Fe-Ni oxide, Ni-Fe-Co oxide, Co oxide), and custom feed materials (Al-Co oxide, Ni-Co-Cr metals). The type and composition of Ni and Co phases vary depending on location, indicating that the dust created at each location is related to locational and daily activity. In particular, dust created near the calciner is representative of daily custom feed activity. On day 14, custom feed material containing Al-Co oxide was added to the secondary feed, and an increase in Al-Co oxide is observed in the calciner during this period. The samples taken near the roof fan contain higher abundances of Fe-Ni oxide, which may be related to the roasting sequence.
In the shuttle samples that contain elevated particle loading, the dominant particulates are Ni matte (day 3) and pentlandite (Ni concentrate, day 9). The elevated contents on these days are likely due to isolated events. In the converter aisle samples where the particle loading and Ni and Co concentrations are more consistently elevated, the dominant Ni phases include Ni matte and Ni and Co oxides.
To determine the overall contribution of Ni and Co from each source, a Ni and Co deportment was calculated based on the normalized abundance and mass distribution of Ni and Co phases, their known (or assumed) compositions, and the Ni and Co chemical assays (Figure 3 daily, Figure 4 combined filters by location). Overall, the dominant source of Ni is matte (finished or furnace matte originating from the shuttle location, or intermediate matte originating from the converter aisle). The dominant sources of Co are custom feed products Ni and Co oxides. Similar to the particle loading, the most significant contributions of Ni and Co occur as the isolated events of Ni matte and pentlandite emissions near the shuttle, however, the most consistent daily contributions occur at the converter aisle as intermediate Ni matte emissions.
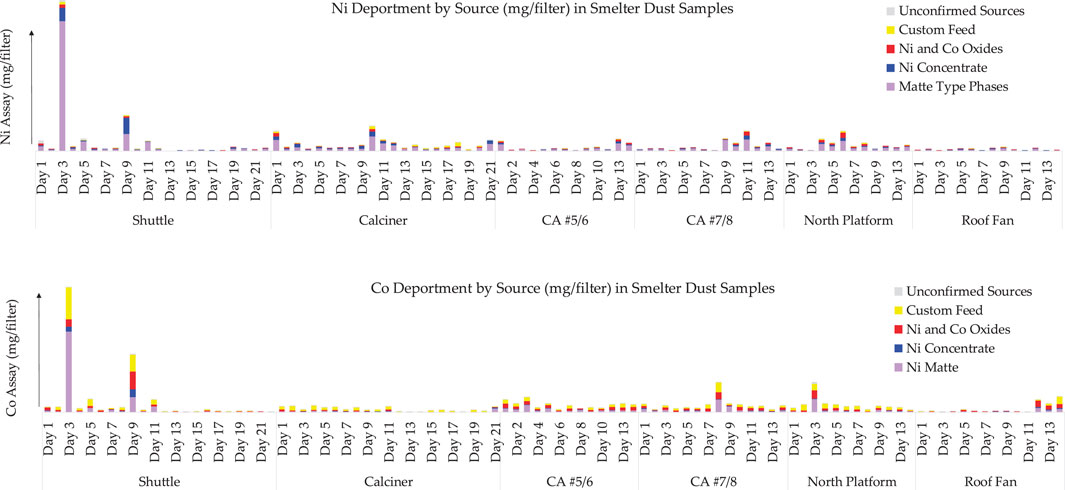
FIGURE 3. Ni and Co deportments in PM (grouped by source). Data is presented as mg of Ni or Co per filter.
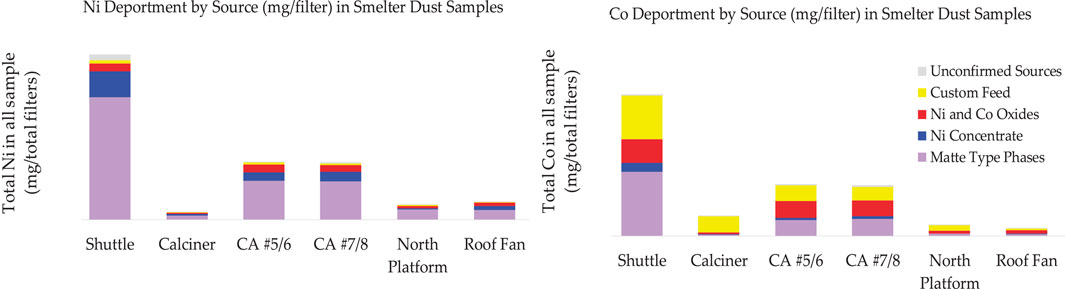
FIGURE 4. Total absolute Ni and Co contribution by source and location (combined Ni and Co mg/filter at each location).
To better demonstrate the correlation between location and dust type, the relative daily abundance (mass distribution) and average abundance of the critical Ni and Co phase are plotted in Figures 5 through 7. In the converter aisle, oxides that contain Ni as a dominant metal, and intermediate matte phases (Ni sulfides and Ni-Co sulfides) occur more commonly. Fe oxides with low levels of Ni and Co occur more commonly in samples collected on the upper floor near the roof fan. Custom feed products occur more commonly in the samples collected near the calciner. Particulate matter containing finished matte (or furnace matte, Ni-Cu-S) and Ni concentrate occur more commonly throughout each location, however, Ni concentrate appears to be relatively low in the samples collected on the platform. Intermediate matte phases, including Ni sulfides and Ni-Co sulfide, occur more commonly in the converter aisle samples. Although the condensed sulfur occurs in trace amounts, it is more commonly present on the upper levels of the facility (above converter aisles and roof fan).
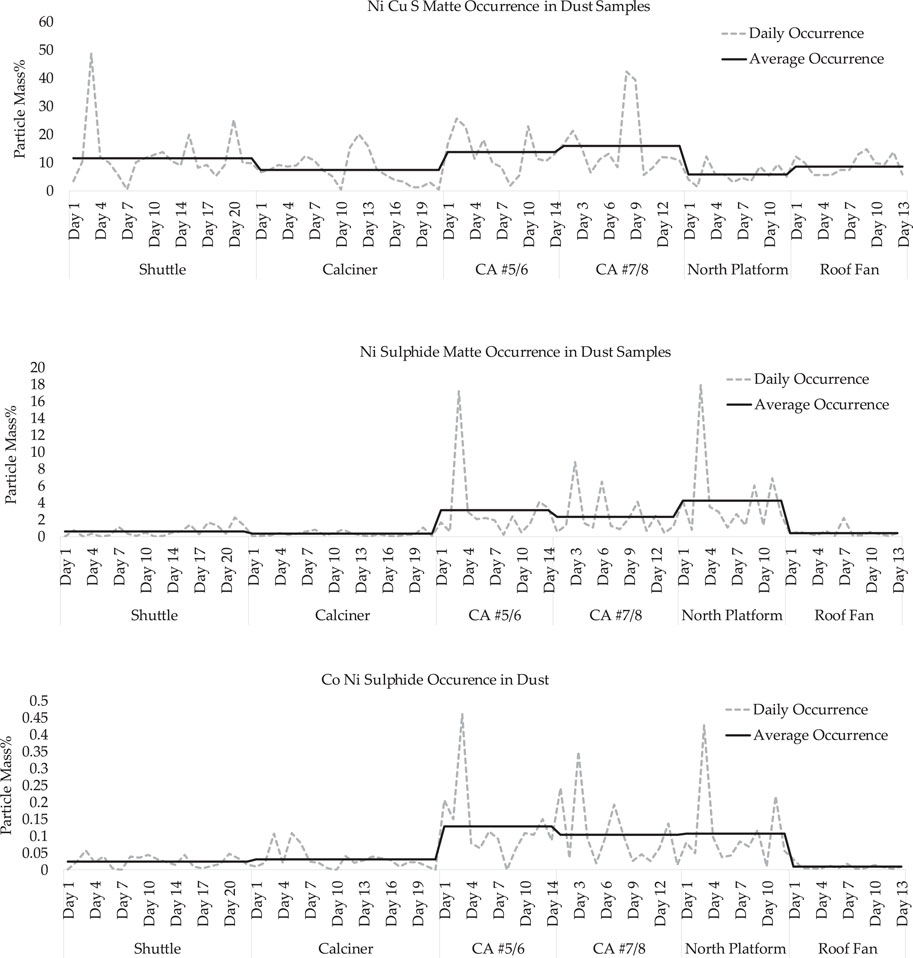
FIGURE 6. Average and daily occurrence of PM by type of Ni matte (Ni sulfide, Ni-Cu-Fe-S, Ni Co sulfide) and custom feed and location.
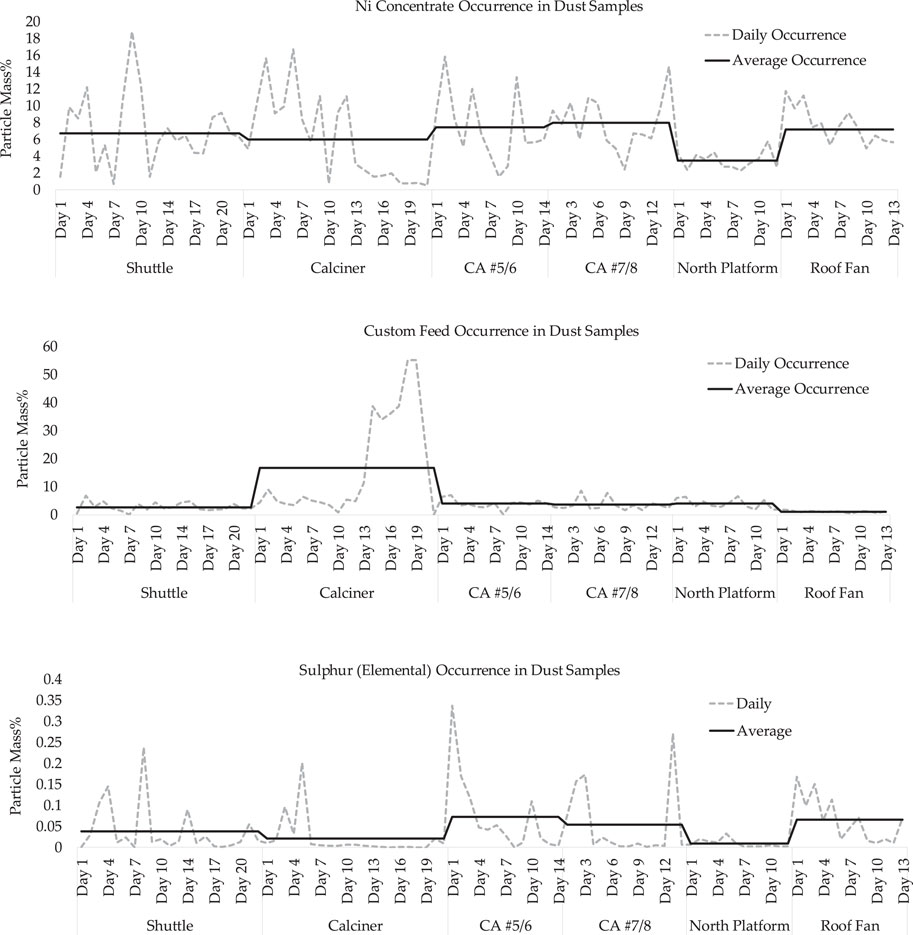
FIGURE 7. Average and daily occurrence of PM of Ni concentrate, custom feed products, and elemental sulfur by location.
Particle Characteristics and Morphology
Upon collection of the QEMSCAN data, selected particles were inspected using scanning electron microscope to confirm the chemistry of the particulates identified by the QEMSCAN SIP and to examine morphology. Examples of selected particles with their corresponding energy dispersive X-ray spectra (EDX) are provided in Figures 8 through 11. The morphology of the particles is consistent with activity. Particulates produced from crushed, bulk materials, such as concentrate, are angular/crystalline (Figure 8), and the oxide phases tend to be rounded, suggestive of emissions from molten material (Figures 9, 10).
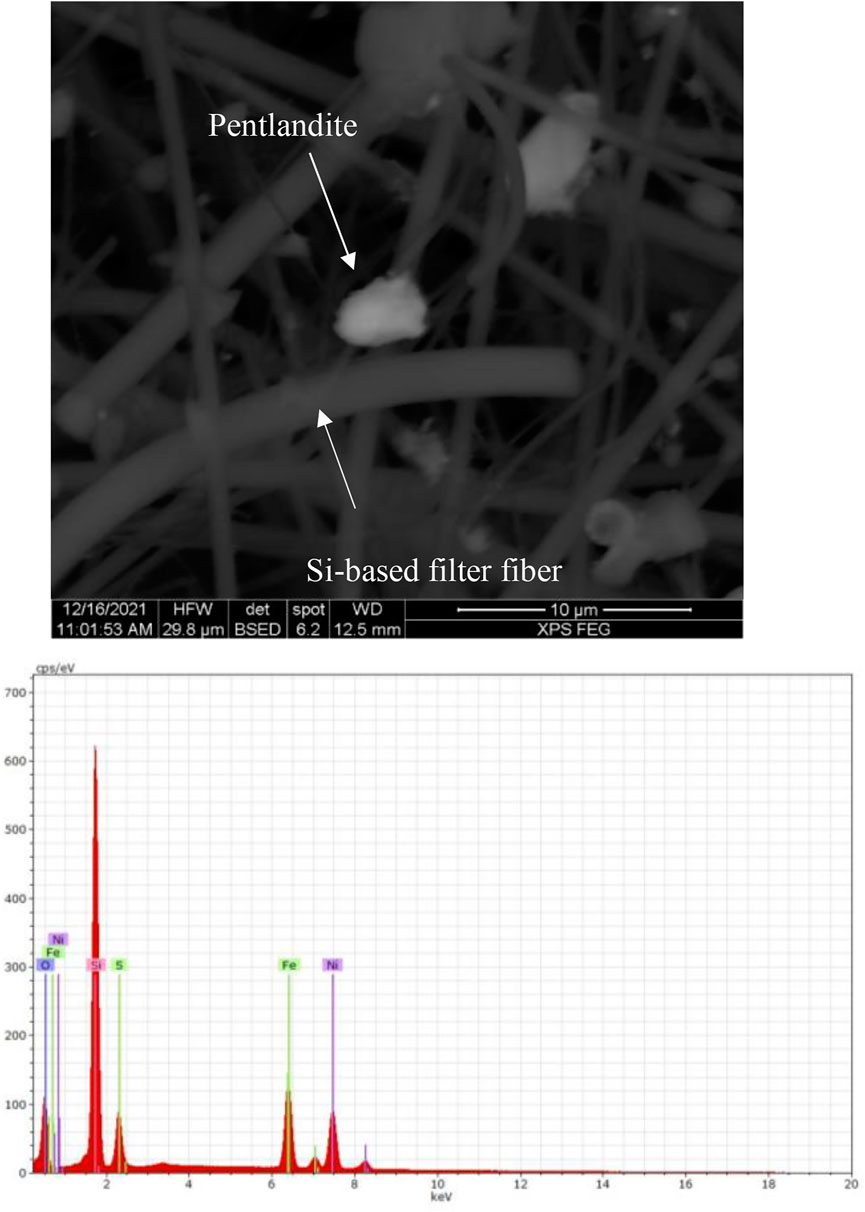
FIGURE 8. Pentlandite particulate (top) and respective Energy Dispersive X-ray spectra (bottom). Silica and O signal detected in the pentlandite grain are interference from the Si-O based filter fiber due to X-ray scatter.
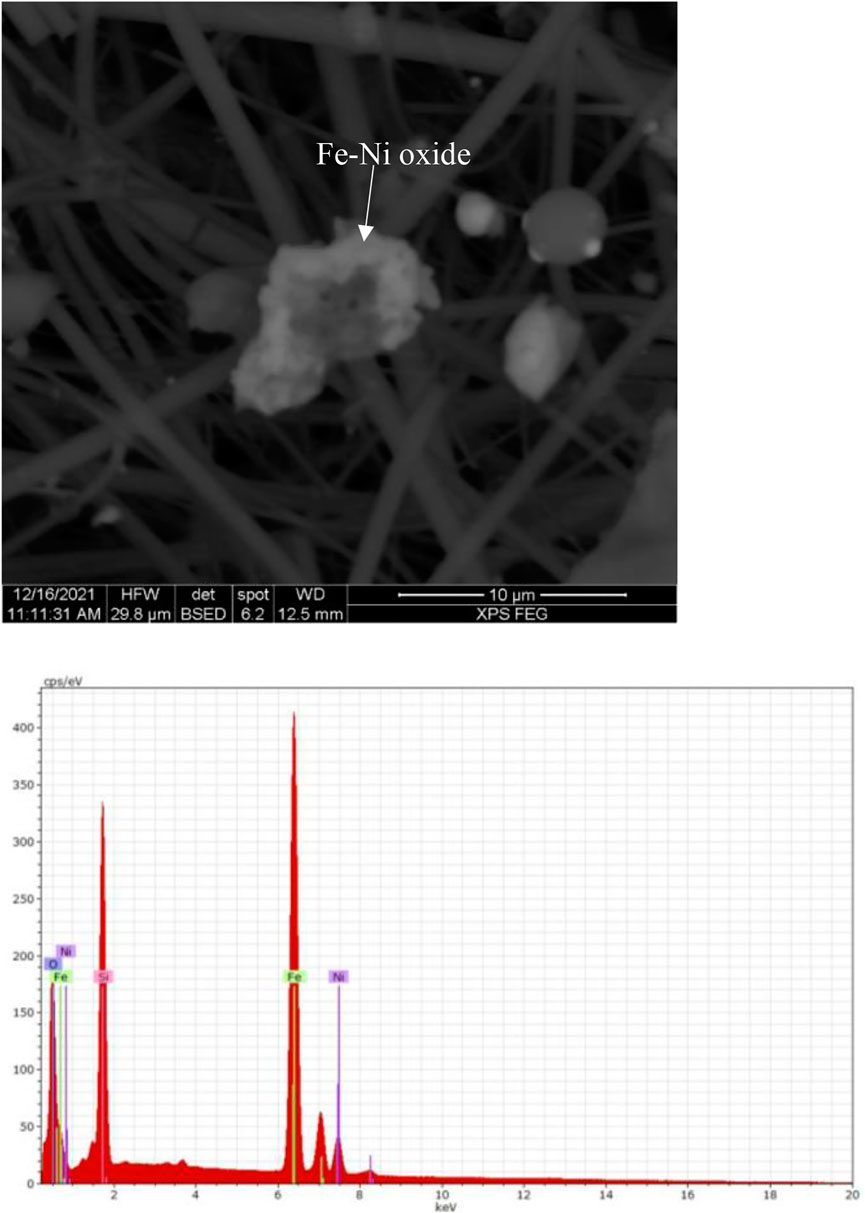
FIGURE 9. Fe-Ni oxide particulate (top) and respective Energy Dispersive X-ray spectra (bottom). The Si signal detected in the Fe-Ni oxide grain are interference from the Si-O based filter fiber due to X-ray scatter.
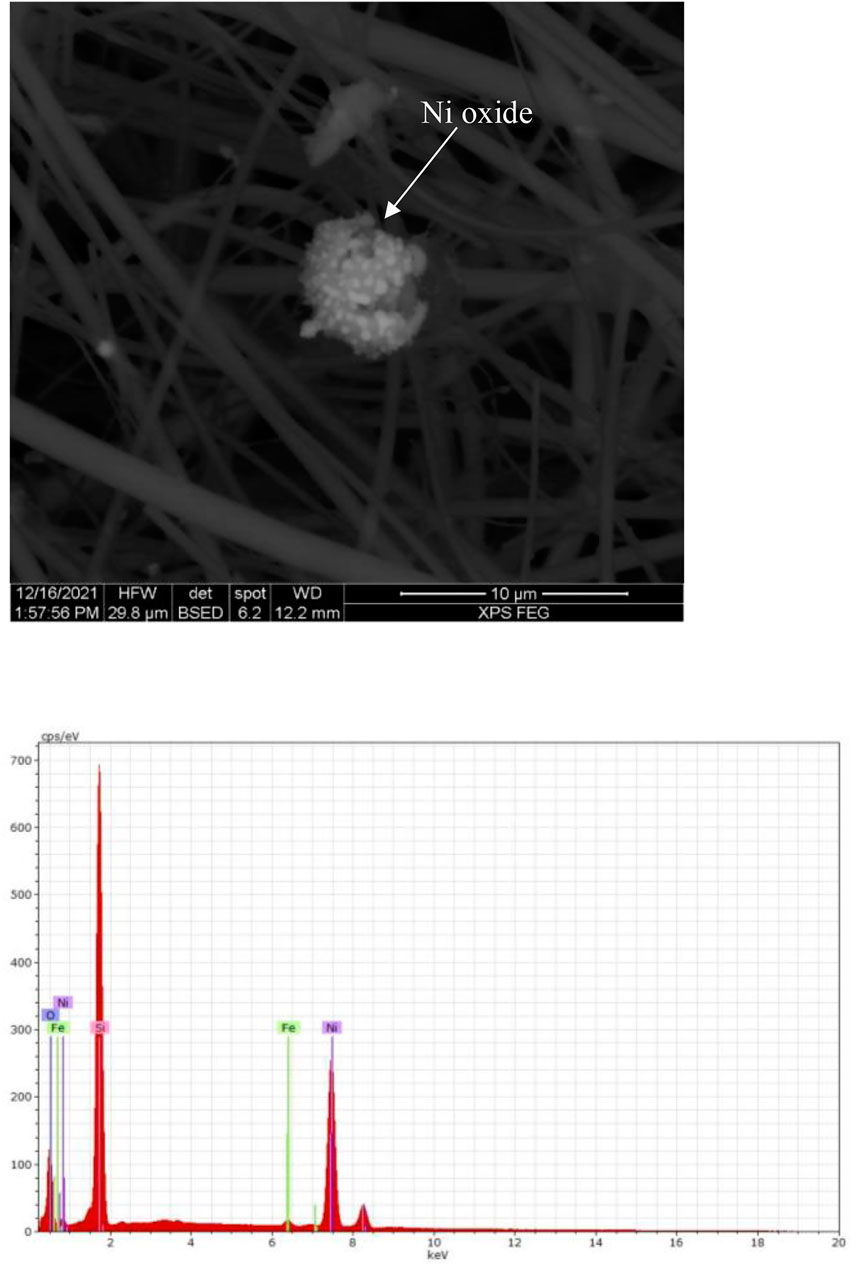
FIGURE 10. Agglomerated Ni oxide particulate (top) and respective Energy Dispersive X-ray spectra (bottom). The Si signal detected in the Ni oxide grain are interference from the Si-O based filter fiber due to X-ray scatter. The BSE intensities of the agglomerated particle suggests a variable Ni content.
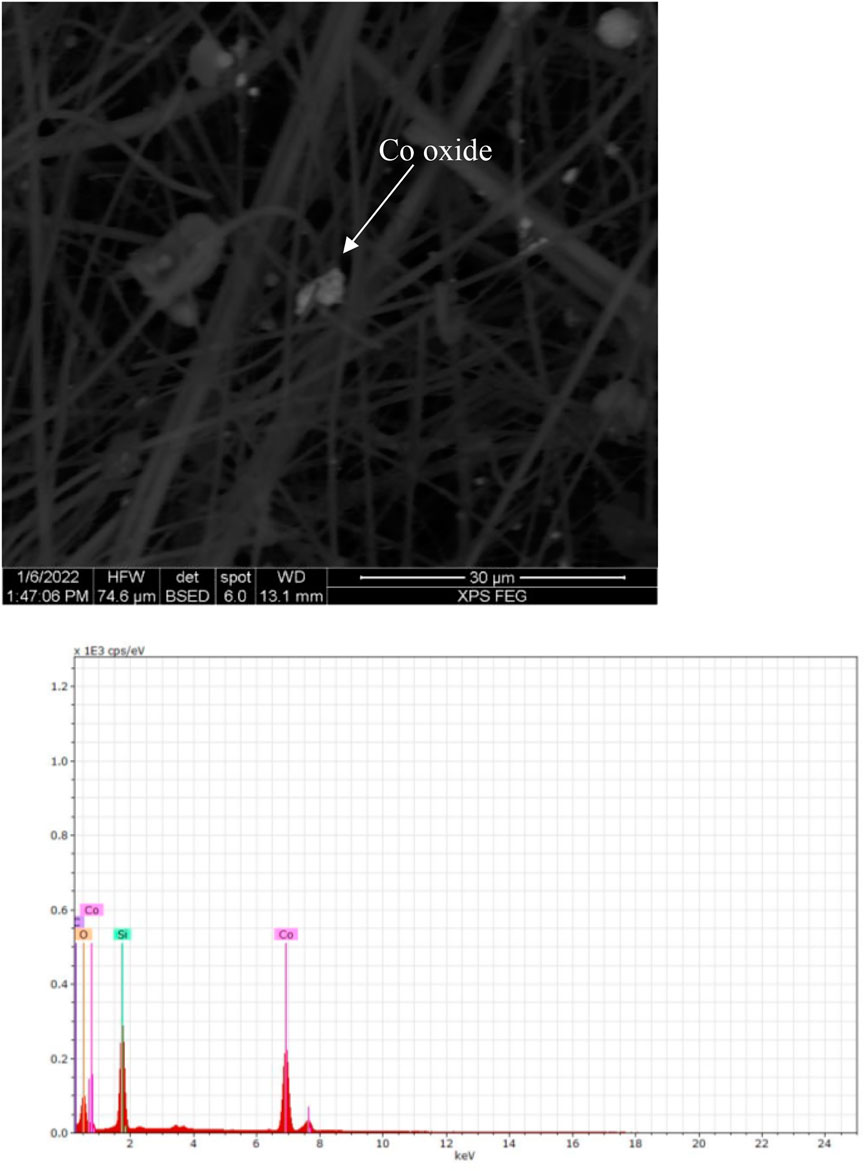
FIGURE 11. Co oxide particulate (top) and respective Energy Dispersive X-ray spectra (bottom). The Si signal detected in the oxide grain are interference from the Si-O based filter fiber due to X-ray scatter.
Data Validation
As part of the data validation process, quantification of compositions in the bulk products was performed over years of sampling using EPMA to determine concentrations of elements of interest (e.g., Ni, Co, S, Fe). Because the dust is too fine to be measured using EPMA, the existing compositions determined for the bulk materials were used as a proxy for the materials identified in dust. In some cases, the emissions are created from molten materials and no bulk materials could be collected, which is a primary focus of this baseline investigation. To ensure that all emissions have been identified as accurately as possible, a reconciliation between measured and calculated particle loading (mass) was performed for each sample. The calculated mass was based on the number of particles measured per area measured (scaled to meet the total area of the filter), the average density of the minerals identified, and the average particle size. Any discrepancy between the measured and calculated values may be due to non-uniform distribution of particles across the filter, or imprecise definitions of mineral density or compositions where these values are assumed.
There is generally good agreement between the calculated and measured particle loading (Figure 12) indicating optimal counting statistics for particles representing the majority of the sample mass.
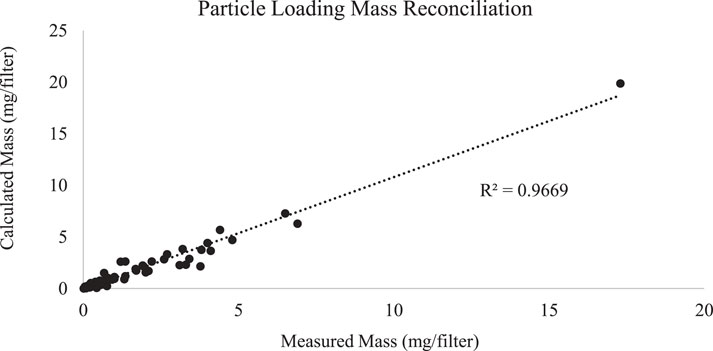
FIGURE 12. Comparison of calculated particle loading (particle mass), and Ni and Co concentration to measured values.
Discussion
The results of the sampling campaign demonstrate that the shuttle area and converter aisle are the most significant sources of emissions. The composition of the Ni and Co dust at these locations can provide indications of the type of activities that have contributed to the emissions. In the shuttle area, the dust is characteristic of finished granulated matte (Ni-Cu-S) or pentlandite, suggesting that emissions are related to transport of bulk materials. This observation is also supported by the inconsistent emission patterns recorded at the shuttle, including erratic metal concentrations and inconsistent sources of dust (isolated events of matte and pentlandite emissions).
In the converter aisle samples, the dust is characteristic of fugitive emissions where it contains intermediate smelting phases (Ni sulfide, Ni-Co sulfide, Ni, Fe, and Co oxides, and condensed sulfur). Emissions in this area can be produced from spray through pouring and tapping molten material. The fine Ni and Co oxide particulates from molten materials may oxidize faster because the increased surface area will promote faster cooling. The emissions patterns at the converter aisle are also more consistent day-to-day, and correlate with the routine activity performed at this location. These fugitive emissions are important to document because matte pouring and tapping are daily activities performed year round at most smelter facilities, and understanding the associated dispersion patterns can be useful for assessing worker safety and mitigating emissions.
Custom feed materials have a stronger contribution to Co emissions than Ni emissions due to their higher content of Co. Emissions from custom feed are most prominent at the calciner, but relatively well contained at the other locations. The abrupt presence of Al-Co oxide suggests that emissions were generated during addition of this material to calciner. Aside from the isolated event in the shuttle area, Ni concentrate (pentlandite) only contributes to low quantities of the total Ni in all samples, but the frequency of abundance of pentlandite suggests that it is consistently present in low amounts throughout each location (except for the platform), and efforts to contain pentlandite dust should focus on widespread mitigation.
Comparison of the data at each location indicates that movement of material in the shuttle area is capable of generating greater net emissions than activities performed in the converter aisle even though the emissions at the shuttle area are localized, isolated events. The materials handled in the shuttle area are generally low moisture content, while the fugitive emissions are molten. Emission with low moisture content are more likely to become airborne and travel greater distances. However, an integrated study combining long-term sampling of year-round activity and air samples collected from areas surrounding the facility are needed to determine the overall dispersion of PM from each location. Samples taken for different size ranges should also be included in future emission studies to evaluate the dispersion and health effects associated with particulate size.
Although a direct assessment of workplace exposure cannot be made through the sampling mechanisms used in this study, general comparisons of relative exposure between locations can be made. Based on increased particle loading, workers performing tasks in the converter aisles and shuttle area are at greatest risk of dust exposure. The Ni matte generated at the Sudbury INO smelter is considered to be a Ni-subsulfide and can have potential carcinogenic effects with repeated, elevated exposures. Animal exposure studies and studies performed on human tissue cells have shown increased rates of adverse health effects with chronic exposure to Ni oxide particulates and nanoparticles (e.g., Dunnick et al., 1995; Capasso et al., 2014). Even though the absolute emissions generated in the shuttle area are higher, the converter aisle emissions contain both Ni-subsulfide and Ni oxides. The health effects associated with combined emissions (Ni-subsulfide + Ni oxide) should be investigated.
Speciation of the Ni and Co phases can add value to environmental assessments studies and remediation efforts in the Sudbury area. Wren (2012) attributed lower vegetation growth rate near active mining operations to increased metal concentrations (i.e., Ni, Cu, As, Se) in soils surrounding these operations. The study suggested that interaction of metals with acidic soils can be toxic to certain forms of vegetation. Understanding the types of metals present in the soils can help determine the best strategies for remediation and predict the time required for full remediation to occur. The physical and chemical properties of metals, such as solubility and ion exchange capacity, will depend on the form they are present in. For example, it is believed that Ni2+ in Ni subsulfide is more mobile compared to other Ni species (Nickel Institute, 2008). Mobility of Ni ions can influence the level of toxicity in soils and impact the ability of the soil to support communities of terrestrial plants. In addition, characterization studies of the particulate matter generated at operations will help reduce overall emissions that reach surrounding communities by implementing mitigation strategies that are specific to source.
The detection of condensed elemental sulfur droplets through QEMSCAN analysis was not expected due to the low density and fluidity of SO2 emissions. Although elemental sulfur did not contribute to significant quantities of the overall PM mass, it increases in abundance in samples collected at upper levels of the smelter, and is relatively depleted on the main floors. This trend is consistent with fugitive emissions of low density, and suggests that QEMSCAN may have capability to characterize sulfur emissions at the particle level. The ability to detect elemental sulfur using QEMSCAN presents opportunity to incorporate quantitative mineralogy into SO2 emission studies related to sustainability and climate change.
A series of action plans for reducing Ni and Co emissions related to handling matte products and Ni concentrates are being commissioned based collectively on the results of the work presented here and several dust characterization studies performed by Sudbury INO. Supplemental hood vents have been recently installed directly above the casting ladles in an effort to reduce emissions produced in the converter aisles. Items for improving Ni and Co particulate emissions from bulk materials include containment of stock materials stored outdoors, frequent inspection of dust collection systems used to maintained roads and buildings on-site, and redesigning Ni concentrate baghouse receiving areas to increase holding capacities. The smelter has also implemented an Online Particulate Monitor early warning system to help predict emissions under certain meteorological conditions. Under unfavourable meteorological conditions, activities that are known to produce dust are suspended (Ransom, 2018).
The study carried-out at the Sudbury INO smelter has shown that QEMSCAN is effective for speciating solid Ni and Co particles in TSP dust. Other common methods of metal speciation include wet-chemical sequential leaching (e.g., Zatka et al., 1992; Conard et al., 2008) and synchrotron X-ray absorption near-edge structure spectroscopy (SR-XANES) (Van Loon et al., 2015). Wet-chemical extraction techniques leach metals into four main categories: soluble, metallic, oxidic, and sulfidic, and SR-XANES identifies metal species based on energy absorption profiles that are specific to ion coordination and bonding chemistry. These methods have shown promise for speciating metals with relatively simple origins, but SR-XANES and sequential leaching cannot produce results with the same level of detail as QEMSCAN (e.g., sub-speciation and observation of particle morphology) or do not have the detection limits necessary for speciating metals in ambient dust. Additionally, due to the limited beam time and long acquisition time, SR-XANES is not a practical method for monitoring dust pollution when rapid turnaround is required. Differentiating between the Ni sub-species was critical for determining which activities at the smelter generated the most significant emissions. Identification of finished/granulated Ni matte versus intermediate matte, and identification of Ni-Fe oxides revealed that transport of dry bulk materials was responsible for significant quantities of the absolute emissions, while matte pouring and tapping were responsible for constant fugitive emission sprays in the converter aisle. This level of speciation could not have been achieved using sequential leaching or SR-XANES.
Physical limitations of QEMSCAN are encountered for fine (<2.5 µm) and ultra-fine (<100 nm) PM where sufficient measurement resolution cannot be obtained due to contributing X-ray volume of material surrounding the particulates. There is a growing need to examine fine and ultra-fine dust because it is easily respirable and has been shown to have altered toxicity compared to larger particulates. This will be considered in a subsequent stage of work and may require a combination of speciation methods and analysis of multiple particle fractions.
Conclusion
To better understand activity specific sources of dust emissions at the Sudbury INO Ni smelter, a total 98 samples of particulate matter (TSP) collected from key locations were characterized using QEMSCAN. QEMSCAN was able to easily differentiate between critical Ni and Co subspecies present in the dust samples, and mineralogical and chemical signatures of emissions produced at each location were established. Subspeciation of particulate matter is unique to QEMSCAN, and cannot be achieved using other common methods of dust analysis such as sequential leaching and SR-XANES.
The overall results indicate that transport of bulk materials with low moisture content releases the greatest net emissions of Ni and Co dust, whereas handling molten matte materials in the converter aisle produces the most consistently elevated emissions. These findings allowed personnel to implement dust suppressant strategies that are specific to location and activity. Strategies included configuration of supplemental hood vents in the converter aisles to better capture fugitive emissions, and increasing storage capacity for bulk materials.
The thorough characterization of emissions produced through the smelting process can provide general information related to environmental risk assessment and reclamation. Reducing dust emissions is more effective when the sources of emissions are well understood. In addition, the toxicity level of metal particulates present in soils surrounding mining operations will depend on its chemical form.
An unexpected result was that condensed elemental sulfur was detected using the QEMSCAN method, with abundance increasing at upper level locations. Although elemental sulfur did not contribute to significant quantities of the particulate matter, the ability to detect sulfur using QEMSCAN presents opportunity to incorporate quantitative mineralogy in environmental assessments related to SO2 emissions (United States Environmental Protection AgencyCentre for Environmental Research, 1999).
Data Availability Statement
The data that supports the findings of this study are available from the corresponding author (MK) upon reasonable request.
Author Contributions
Data analysis and manuscript preparation was completed by MK. Supervision and manuscript review were performed by EW and ML.
Funding
All funding for this work was provided by Sudbury INO.
Conflict of Interest
Author MK is employed by XPS.
The remaining authors declare that the research was conducted in the absence of any commercial or financial relationships that could be construed as a potential conflict of interest.
The handling editor declared a past co-authorship with one of the authors EW.
Publisher’s Note
All claims expressed in this article are solely those of the authors and do not necessarily represent those of their affiliated organizations, or those of the publisher, the editors and the reviewers. Any product that may be evaluated in this article, or claim that may be made by its manufacturer, is not guaranteed or endorsed by the publisher.
Acknowledgments
We wish to thank Sudbury INO for permission to publish this work, and the technical staff at Sudbury INO and XPS for their help with sampling, sample preparation and review of the manuscripts.
References
Barceloux, D. G., and Barceloux, D. (1999). Cobalt. J. Toxicol. Clin. Toxicol. 37, 201–216. doi:10.1081/clt-100102420
Boerchers, M., Fitzpatrick, P., Storie, C., and Hostetler, G. (2016). Reinvention through Regreening: Examining Environmental Change in Sudbury, Ontario. Extr. Industries Soc. 3, 793–801. doi:10.1016/j.exis.2016.03.005
Capasso, L., Camatini, M., and Gualtieri, M. (2014). Nickel Oxide Nanoparticles Induce Inflammation and Genotoxic Effect in Lung Epithelial Cells. Toxicol. Lett. 226 (1), 28–34. doi:10.1016/j.toxlet.2014.01.040
Conard, B. R., Zelding, N., and Bradley, G. T. (2008). Speciation/fractionation of Nickel in Airborne Particulate Matter: Improvements in the Zatka Sequential Leaching Procedure. J. Environ. Monit. 10, 532–540. doi:10.1039/b714884d
Dunnick, J. K., Elwell, M. R., Radovsky, A. E., Benson, J. M., Hahn, F. F., Nikula, K. J., et al. (1995). Comparative Carcinogenic Effects of Nickel Subsulfide, Nickel Oxide, or Nickel Sulfate Hexahydrate Chronic Exposures in the Lung. Cancer Res. 55, 5251–5256. doi:10.1016/0300-483X(88)90087-X
EPA (1990). Environmental Protection Act (Canada), R.S.O 1990, C. E. 19. Available at: https://www.ontario.ca/laws/statute/90e19 (updated 2017, accessed February, 2018).
Gottlieb, P., Wilkie, G., Sutherland, D., Ho-Tun, E., Suthers, S., Perera, K., et al. (2000). Using Quantitative Electron Microscopy for Process Mineralogy Applications. J. Min. Met. Mat. Soc. 52, 25. doi:10.1007/s11837-000-0126-9
Grimsrud, T. K., Berge, S., Resmann, F., Norseth, T., Andersen, A., and Andersen, A. (2000). Assessment of Historical Exposures in a Nickel Refinery in Norway. Scand. J. Work Environ. Health 26, 338–345. doi:10.5271/sjweh.551
Grimsrud, T. K., Berge, S., Haldorsen, T., and Andersen, A. (2002). Exposure to Different Forms of Nickel and Risk of Lung Cancer. Am. J. Epidemiol. 156, 1123–1132. doi:10.1093/aje/kwf165
Gunn, J., Keller, W., Negusanti, J., Potvin, R., Beckett, P., and Winterhalder, K. (1995). Ecosystem Recovery after Emission Reductions: Sudbury, Canada. Water Air Soil Pollut. 85, 1783–1788. doi:10.1007/bf00477238
ISO (2004). Workplace Air—Determination of Metals and Metalloids in Airborne Particulate Matter by Inductively Coupled Plasma Atomic Emission Spectrometry—Part 3: Analysis, ISO 15202-3. Geneva, Switzerland: International Organization for Standardization.
ISO (2010). Workplace Air—Determination of Metals and Metalloids in Airborne Particulate Matter by Inductively Coupled Plasma Mass Spectrometry ISO 30011. Geneva, Switzerland: Internation Organization for Standardization.
J. M. Gunn (Editor) (1995). Restoration and Recovery of an Industrial Region (New York: Springer-Verlag).
Kelvin, M., and Kormos, L. (2015). QEMSCAN Analysis of Hivol Air Filters to Determine Sources of Dust Particulates at the Sudbury INO Smelter – Oral Presentation and Abstract 2015 mining and Environment Conference. Sudbury, Ontario.
Kramer, D. M., Haynes, E., Lightfoot, N., and Holness, D. L. (2018). Dimensions of Community Change: How the Community of Sudbury Responded to Industrial Exposures and Cleaned up its Environment. J. Community Engagem. Scholarsh. 10, 9. doi:10.54656/ruom4253
Lautenbach, W. E., Miller, J., Beckett, P. J., Negusanti, J. J., and Winterhalder, K. (1995). “Municipal Land Restoration Program: The Regreening Process,” in Restoration and Recovery of an Industrial Region. Editor J. M. Gunn (New York, NY: Springer Series on Environmental Management. Springer), 109–122. doi:10.1007/978-1-4612-2520-1_8
Lauwerys, R., and Lison, D. (1994). Health Risks Associated with Cobalt Exposure - an Overview. Sci. Total Environ. 150, 1–6. doi:10.1016/0048-9697(94)90125-2
Ministry of the Environment, Conservation and Parks (2009). Operations Manual for Air Quality Monitoring in Ontario. Available at: https://www.publications.gov.on.ca/operations-manual-for-air-quality-monitoring-in-ontario (Date accessed: November, 2021).
Nickel Institute (2008). Safe Use of Nickel in the Workplace: A Guide for Health Maintenance of Workers Exposed to Nickel, its Compounds and Alloys. Brussels, Belgium: Nickel Institute.
Potvin, R. (2007). “Air Quality Trends in the Sudbury Area 1953-2002,” in Presented at the Mining and Environment Conference (Sudbury, Ontario, 19–27.
Ransom, C. (2018). “Environmental Management System & Community Engagement Report 2017,” in Report Submitted to the Ministry of the Environment and Climate Change March 31st, 2018 on Behalf of Sudbury Integrated Nickel Operations (Sudbury: Smelter).
Schonewille, R., Boissoneault, M., Ducharme, D., and Chenier, J. (2005) Update on Falconbridge’s Sudbury Nickel Smelter. Met Soc, Nickel and Cobalt, 2005: Challenges in Extraction and Production, 44th Annual Conference of Metallurgist of CIM, Calgary, Alberta, Canada (ed by J. Donald, and R. Schonewille)
Shirakawa, T., Kusaka, Y., Fujimura, N., Goto, S., Kato, M., Heki, S., et al. (1989). Occupational Asthma from Cobalt Sensitivity in Workers Exposed to Hard Metal Dust. Chest 95, 29–37. doi:10.1378/chest.95.1.29
Sivulka, D. J., Seilkop, S. K., Lascelles, K., Conard, B. R., Jones, S. F., and Collinson, E. C. (2014). Reconstruction of Historical Exposures at a Welsh Nickel Refinery (1953-2000). Ann. Occup. Hyg. 58, 739–760. doi:10.1093/annhyg/meu022
Sivulka, D. J., and Seilkop, S. K. (2009). Reconstruction of Historical Exposures in the US Nickel Alloy Industry and the Implications for Carcinogenic Hazard and Risk Assessments. Regul. Toxicol. Pharmacol. 53, 174–185. doi:10.1016/j.yrtph.2009.01.006
United States Environmental Protection Agency, Centre for Environmental Research (1999). Compendium of Methods for the Determination of Inorganic Compounds in Ambient Air. Washington, DC: EPA/625/SR-96/010a.
Van Loon, L. L., Throssell, C., and Dutton, M. D. (2015). Comparison of Nickel Speciation in Workplace Aerosol Samples Using Sequential Extraction Analysis and X-Ray Absorption Near-Edge Structure Spectroscopy. Environ. Sci. Process. Impacts 17, 922–931. doi:10.1039/c4em00603h
Wren, C. (2012). Risk Assessment and Environmental Management: A Case Study in Sudbury, Ontario, Canada. Leiden: Maralte B.V.
Keywords: dust moniroting, QEMSCAN, PM and S emissions, nickel characterization, apportionment
Citation: Kelvin M, Whiteman E and Leybourne M (2022) Characterization of Particulate Matter Generated at a Nickel Smelter Using Quantitative Mineralogy. Front. Earth Sci. 10:817759. doi: 10.3389/feart.2022.817759
Received: 18 November 2021; Accepted: 12 April 2022;
Published: 04 July 2022.
Edited by:
Alan R. Butcher, Geological Survey of Finland, FinlandReviewed by:
Ajka Šorša, Croatian Geological Survey, CroatiaFabrizio Bardelli, Italian National Research Council, Italy
Copyright © 2022 Kelvin, Whiteman and Leybourne. This is an open-access article distributed under the terms of the Creative Commons Attribution License (CC BY). The use, distribution or reproduction in other forums is permitted, provided the original author(s) and the copyright owner(s) are credited and that the original publication in this journal is cited, in accordance with accepted academic practice. No use, distribution or reproduction is permitted which does not comply with these terms.
*Correspondence: Michelle Kelvin, bS5rZWx2aW5AcXVlZW5zdS5jYQ==