- 1Department of Marine Zoology, Senckenberg Research Institute, Frankfurt, Germany
- 2Departamento de Estratigrafía y Paleontología, Universidad de Granada, Granada, Spain
Bioturbation can influence petrophysical properties (e.g., porosity, permeability) of sedimentary rocks and, in consequence, reservoir quality. The impact can be positive, negative, or neutral, requiring detailed ichnological analysis. Ophiomorpha, a branched cylindrical burrow with diagnostic peloidal wall, may be present in bioturbated reservoirs that exhibit properties of both super-permeability and reduced porosity/permeability. However, no mechanistic understanding of how Ophiomorpha positively or negatively impacts petrophysical properties has yet been established. This study presents high-resolution X-ray microtomographic analysis of how the features of Ophiomorpha (i.e., peloidal wall vs. burrow fill) influence porosity distribution in deep-water deposits (Neogene Tabernas Basin, SE Spain). The results show that the peloidal burrow wall exhibits the lowest porosity (up to six orders of magnitude lower than burrow fill or host sediment), while surrounding sediment shows variable values. Abrupt porosity changes within the fill material likely relate to burrow-associated diagenesis. A refined understanding of the features of Ophiomorpha and their associated porosity distribution help to constrain understanding of their diverse impacts on reservoir properties.
Introduction
Applied ichnology is used in tandem with sedimentologic, stratigraphic, diagenetic, and reservoir petrophysical characterization of sedimentary successions considered to have petroleum potential, interest for carbon capture, or representing valuable water resources (e.g., Dawson et al., 1977; MacEachern et al., 2007; Knaust and Bromley, 2012; Hose and Stumpp, 2019). Advances arise from the interest of the petroleum industry and water providers in understanding how bioturbation controls petrophysical properties (e.g., porosity and permeability). Studies have evaluated bioturbation’s impacts on porosity and permeability in reservoir characterization (e.g., McDowell et al., 2001; Pemberton and Gingras, 2005, Cunningham et al., 2009, 2012; Gingras et al., 2012; Knaust, 2013, Knaust et al., 2020; Baniak et al., 2014, 2015, 2022; Bednarz and McIlroy, 2015; Eltom et al., 2019; Dorador et al., 2021; Oliveira de Araújo et al., 2021; Rodríguez-Tovar et al., 2021; Miguez-Salas et al., 2022), showing that bioturbation can impart both positive or negative impacts on reservoir character. Usually, bioturbation lowers porosity and permeability since tracemakers tend to reduce sorting or introduce clay (e.g., Gingras et al., 2012; Alqubalee et al., 2022), but several studies have shown that bioturbation can enhance porosity and permeability (Pemberton and Gingras, 2005; Cunningham et al., 2009; Tonkin et al., 2010; Gingras et al., 2012; Baniak et al., 2022 for a recent review) or may exert no influence at all (Miguez-Salas et al., 2022). Ichnological features such as burrow morphology, architecture, and fill, and tracemaker behavior can combine with post-depositional chemical processes (cementation/dissolution) to modify pore structure and distribution and ultimately affect lateral and vertical fluid flow (Gingras et al., 2012; Knaust, 2013; Miguez-Salas et al., 2022).
Given their uncertain influence on reservoir character, Knaust (2013), Knaust (2014) proposed a morphological classification of burrows related to bioturbation-influenced porosity based on particular ichnogenera. While this predictive model may provide accurate results, countless ichnogenera (e.g., Macaronichnus) have ambiguous effects on void space and results may depend on reservoir facies or some morphological trace-fossil features. These caveats hold true for Ophiomorpha, one of the commonest and most studied ichnogenera in potential reservoir strata. Gingras et al. (2012) stated that, “in the light of their branching morphology, deeply penetrative nature and passive fill, Thalassinoides and Ophiomorpha are likely the most important permeability-enhancing trace fossils.” The positive effects appear clearly in several prominent examples. The Biscayne aquifer of southeastern Florida (United States) hosts a dense Ophiomorpha assemblage that establishes zones of superpermeability (Cunningham et al., 2009; Cunningham et al., 2012). The Upper Jurassic Ula Formation (Norwegian Central Graben) consists of an Ophiomorpha-dominated sandstone with enhanced permeability (Baniak et al., 2014; Baniak et al., 2015). However, Ophiomorpha-dominated strata can also show reduced porosity and permeability, and these clearly diminish reservoir quality (e.g., Tonkin et al., 2010; Knaust, 2013; La Croix et al., 2017; Alqubalee et al., 2022).
Thus, the influence of Ophiomorpha on porosity and permeability remains ambiguous. The diverse, three-dimensional morphology of Ophiomorpha and its pore distribution also remain poorly understood. This study reports a detailed, high-resolution analysis of pore distribution in samples hosting Ophiomorpha specimens, from deep-water deposits (Neogene Tabernas Basin, SE Spain), a facies associated with petroleum potential. The study uses digital X-ray axial microtomographic (Micro-CT) analysis to interpret pore density and distribution in terms of 1) burrow fill, 2) peloidal wall, and 3) host sediment. The analysis seeks to determine how Ophiomorpha burrow features control porosity distribution and burrow-associated diagenesis to ultimately influence reservoir petrophysical properties.
Regional geology, material and methods
The Neogene Tabernas Basin (Almeria, SE Spain) represents an elongate intramontane basin developed during and after an Alpine-aged collision between Iberia and North Africa (Sanz de Galdeano and Vera, 1992; Haughton, 2000). During the late Miocene, sediment gravity-flow deposits came to dominate the basin fill, which consists of muddy to sandy, high-density turbidites (Kleverlaan, 1989; Haughton, 2000; Postma et al., 2014). These deposits have been studied from different perspectives as sedimentology or tectonic (e.g., Kleverlaan, 1989; Hodgson and Haughton, 2004), but bioturbation has not been analyzed in detail and there are just a few contributions where it is even mentioned (e.g., Doyle, 1996; Hodgson, 2002). The ichnological assemblage in the turbiditic deposits from the study area is characterized by pre- and post-depositional associations. In general, the pre-depositional assemblage is mainly composed of graphoglyptids (e.g., Paleodyction, Helminthoraphe), and the post-depositional association is dominated by structures such as Thalassinoides, Scolicia and especially Ophiomorpha (De Matteis et al., 2016; Cabrera-Ortiz et al., 2022, research in progress). Ophiomorpha samples from sandy, clastic turbidites were collected based on their ichnological features (e.g., length, diameter, shape, wall). Then, over 40 Ophiomorpha specimens were collected from the freshest available material and studied in the laboratory through polished sections; special attention was paid to the fill, constructional lining, pellet shape, surrounding sediment, and internal textures (e.g., diagenetic halos), among other features. Some other architectural features, such as branching or orientation, that are usually considered in evaluation of reservoir quality of bioturbated deposits (Knaust, 2017 for a detailed review) were not considered, because they mainly affect permeability in a reservoir, instead of porosity. Micro-CT analysis was performed on 17 representative specimens of Ophiomorpha with well-preserved peloidal walls.
Tomographic data were obtained using X-ray computerized axial microtomography (Micro-CT, Zeiss Xradia 510 Versa) conducted at the Center for Scientific Instrumentation (CIC) of the University of Granada. Instrumental settings for sample analysis were ×0.4 magnification and image bins of 1–2,334 images. Scanning parameter were adjusted for every single sample to get the best results. Voltages ranged from 80 to 140 kV, amperage from 72 to 87 µA and time from 4 to 50 s. Image reconstruction was performed using Reconstructor Scout and Scan™ software (Zeiss), a 0.5 Recon filter, and 3,201 projections. Dragonfly Pro™ (Object Research System) software was used for advanced post-processing analysis of 3D image porosity data. Processing of CT files by Dragonfly Pro™ allows generation of a porosity mesh (pore abundance visualization) in which higher color intensity (red) indicates higher pore density (see Miguez-Salas et al., 2022 for a detailed explanation). Quantitative pore analysis characterization was conducted using binary black and white CT still images with the open software Fiji (Schindelin et al., 2012). Each CT image was converted to an 8-bit grayscale image with a threshold defined to establish an appropriate differentiation range for black and white pixels, wherein black pixels indicate void space (Miguez-Salas et al., 2019). For each Ophiomorpha sample, at least 20 still images were generated and processed. Within the same image, multiple, random porosity measurements were conducted to evaluate the peloidal wall, fill, and host sediment. In total 1,626 values were considered, more than 500 measurements were obtained for each of these three parts (567 for the host sediment, 512 from walls and 547 values from burrow fill). Statistical analysis comparison of porosity data in the three parts included one-way ANOVA testing (alpha level of 0.05), and then t-Test: Two-Sample (assuming equal variances) was performed to address which parts had differences in means at the 0.05 confidence level.
Results
Ophiomorpha specimens exhibit cylindrical burrows, which appear rectilinear or gently curved, and muddy peloidal walls. These burrows, whose diameters range from 1 to 3 cm, are part of tridimensional branching networks composed of horizontal mazes and vertical shafts. The peloidal walls consist of cylindrical or conical pellets with rounded edges (Figure 1). Pellet diameter typically spans ∼0.5 cm but can reach 1 cm. Burrows show occasional branching (Figure 1A). No predominant orientation to the bedding planes is observed. Polished sections of the burrows reveal ferruginized halos in the boundary between the peloidal wall and the fill material for some samples. Fill is passive, commonly structureless but sometimes exhibiting textural heterogeneities (e.g., halos, lamination) reflecting differential cementation and/or mineral replacement.
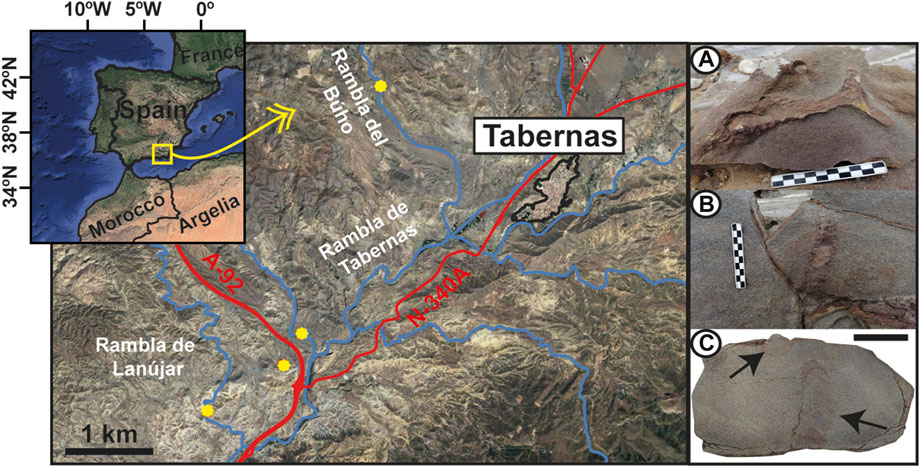
FIGURE 1. Location of the study area (sampled outcrops = yellow points). (A,B) Outcrop examples of Ophiomorpha specimens. (C) Polished section with Ophiomorpha specimens (black arrows) (specimen Op-7), scale bar = 2 cm.
Micro-CT analysis clearly shows different porosity intensities (pore abundance) for the three studied parts (i.e., host rock, peloidal wall and Ophiomorpha fill; Figures 2–4). Peloidal walls exhibit a low abundance of pores and poor connectivity between them, which evidently prevented network porosity (Figures 2, 3). Host sediment confers different degrees of porosity or give varying mesh estimates depending on the particular sample (Figures 2, 3). In some cases, even the same sample can give differing pore intensities that reflect heterogeneity in diagenetic cementation (Figures 2A, 3A). Different Ophiomorpha fill also give varied estimates of porosity and porosity mesh. In some cases, structureless fill without diagenetic textural heterogeneities give low to moderate intensity porosity mesh with a high degree of pore isolation (Figures 2B, 3B). In other cases, diagenetic precipitation (e.g., ferruginized halos; Figure 2A) and dissolution features generate zones with differing degrees of cementation (Figure 2C), yielding a range of different porosity mesh estimates according to diagenetic heterogeneities (Figures 2A,C).
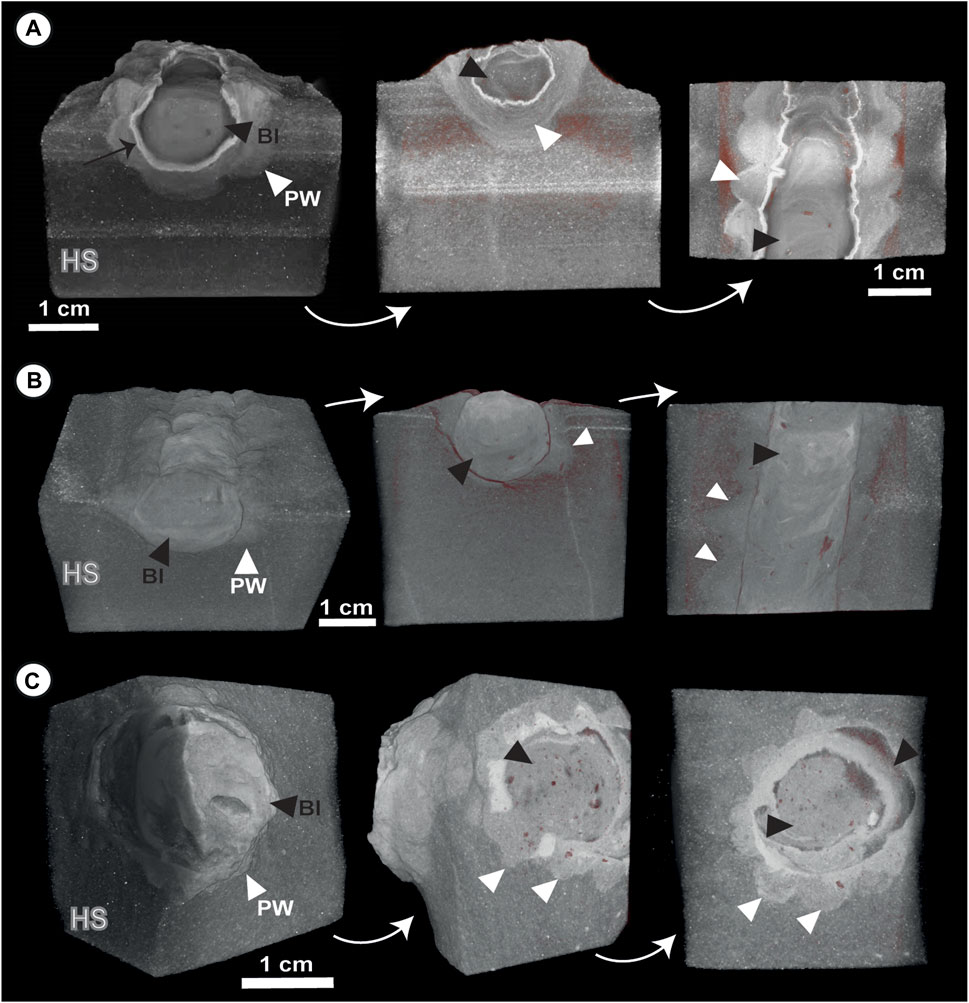
FIGURE 2. (A) Micro-CT images of Ophiomorpha with a ferruginized halo (black arrow) surrounding the fill (specimen Op-15). Note that the peloidal wall (white triangles) lacks porosity, while the fill exhibits (black triangles) porosity zonation (being higher in proximity to the ferruginized halo). (B) Micro-CT captions of Ophiomorpha with structureless and low porosity fill. Peloidal wall again shows near absence of porosity (specimen Op-24). (C) Micro-CT captions of Ophiomorpha with (specimen Op-17A). Note that the fill has areas of high porosity due to differing degrees of cementation. PW, Peloidal wall; BI, Burrow fill; HS, Host sediment.
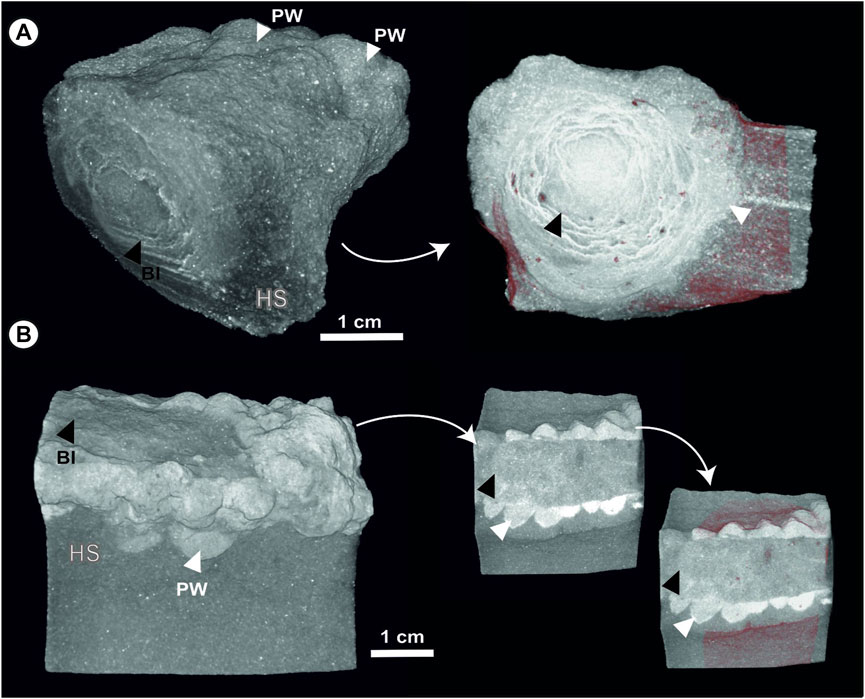
FIGURE 3. (A) Micro-CT images of Ophiomorpha with concentrical burrow-associated diagenesis in the fill (specimen Op-22). Peloidal wall and host sediment show near absence of porosity. (B) Micro-CT captions of Ophiomorpha with structureless and low porosity fill (specimen Op-18). Note that the peloidal wall lacks porosity. PW, Peloidal wall; BI, Burrow fill; HS, Host sediment.
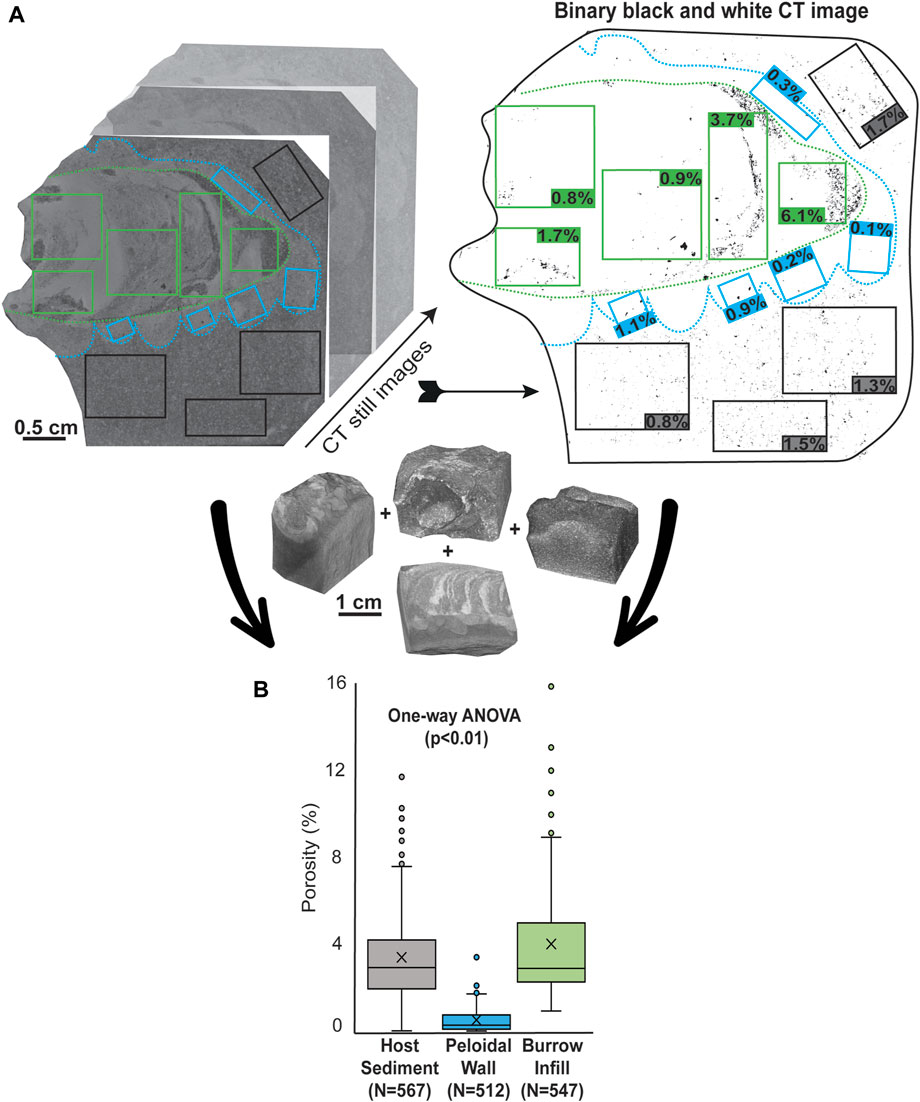
FIGURE 4. (A) Example of Micro-CT still images (left) with associated binary black and white image (right) from one Ophiomorpha sample (specimen Op-18). Note the Micro-CT binary image with random rectangles from which the porosity measurements were obtained for host sediment (grey rectangles), peloidal wall (blue rectangles), and burrow fill (green rectangles). (B) Box plots representing porosity values from the three measured parts of all Ophiomorpha specimens (17 specimens). Note the low porosity values for the peloidal wall and the highly variable porosity for the host sediment and burrow fill.
Tomographic still images confirm that peloidal walls are associated with the lowest observed porosity values. Porosity shows reductions of up to six orders of magnitude in peloidal walls relative to values for fill and host sediment (Figures 4A,B). Peloidal walls shows an average porosity value of 0.54% while the fill and host sediment show 3.39% and 3.20% respectively. The median porosity values are 2.85% for the host sediment, 0.27% for the peloidal wall and 2.56% for the fill.
Binary images indicate differing degrees of diagenetic cementation within the fill of some specimens and show zones of notable porosity contrast (see right and left areas of the Ophiomorpha fill in Figure 4A). Statistical analysis demonstrates that fill and host sediment are associated with a wide range of porosity values (Figure 4B). One-way ANOVA tests showed that the three types of features gave significantly different porosity values [F(2,1623) = (313.4), p < 0.01]. Two sample t-Tests found that burrow fill/peloidal wall and host sediment/peloidal wall porosity values differed significantly (p < 0.01). However, the burrow fill/host sediment t-Test values gave t(1,112) = −1.23, p=0.1 indicating non-unique porosity distributions.
Discussion and implications
Burrowing organisms modify the sediment in several ways. Modification of the original sedimentary fabric and the subsequent influence on diagenetic processes can especially affect void space (Gingras et al., 2012). The tracemakers that create Ophiomorpha, probably callianassids (thalassinidean shrimp), usually create galleries that occupy more than the 50% of the rock volume (Gingras et al., 2012) and modify the host sediment fabric by incorporating constructional pellets into their burrow walls (e.g., Leaman et al., 2015; Netto et al., 2017). The embedment of pellets into burrow walls compacts the surrounding host sediment. Thus, as suggested by previous studies (Tonkin et al., 2010; Baniak et al., 2014; Quaye et al., 2019), peloidal material should show different porosity values than the host sediment. However, these studies were conducted in a limited number of samples and few quantitative measurements were done. Tonkin et al. (2010) based on blue epoxy resin methodology, showed that Ophiomorpha burrow walls create localized zones of porosity reduction, but no quantitative porosity data in relation to the burrow wall was reported. Then, Baniak et al. (2014) based on Micro-CT analysis revealed that greater X-ray attenuation occurred within the Ophiomorpha burrow wall linings, without quantitative porosity data. Recently, Quaye et al. (2019) based on a pressure decay porosimeter (point-counting mesh) made one quantitative porosity measurement of Ophiomopha peloidal walls, showing similar values for host sediment and burrow walls; this is not unexpected since this methodology offers limited results when addressing small features such as burrow walls (<1 cm). Thus, it has not yet been quantitatively verified how Ophiomorpha features may control porosity distribution.
Our research evidence (after doing more than 500 measurements on the peloidal wall and ANOVA One-way p < 0.01) that the peloidal wall can show a porosity reduction of up to six orders of magnitude (Figure 4B). These results carry significant implications for the reservoir potential of sediment hosting dense Ophiomorpha assemblages. The degree of contact between peloidal walls (i.e., the contact between different Ophiomorpha specimens) and the total volume of the peloidal wall with respect to the burrow fill volume can also influence reservoir quality.
Burrow-associated diagenesis, mainly dissolution and cementation processes, can affect petrophysical properties in both ways, but commonly results in improved porosity and permeability (Gingras et al., 2004). Strata hosting dense Ophiomorpha assemblages exhibit biogenic, vuggy macropores which have become superpermeability zones due to fabric-selective carbonate dissolution (Cunningham et al., 2012). However, trace fossils more commonly influence porosity by acting as loci of cementation and dissolution processes, which usually act in tandem during diagenesis (Gingras et al., 2012). Thus, biogenic modification and diagenesis can generate a range of sedimentary fabrics of varying petrophysical properties. Knaust (2017) reports that the nature of Ophiomorpha burrows can guide fluid flow and thereby localize precipitation of early diagenetic minerals. Afterward, Ophiomorpha fill can generate diagenetic heterogeneities through fluid dynamics and recrystallization. Our results support the idea that Ophiomorpha may have experienced differential fluid flow with respect to the host sediment. Comparison of porosity between burrow fill and host sediment, and assuming the absence of primary porosity in peloidal walls, reveals similar but unequal values (p = 0.052). Varying porosity distributions between fill and host sediment may arise from fluid flow through Ophiomorpha, which results in contrasting dissolution and precipitation in fill zones. The diagenetic histories of the Ophiomorpha specimens analyzed here appears highly variable. The varying degrees of precipitation/dissolution observed suggests that a relatively limited volume of sediment with burrow structures can become a complex mosaic of cement and void space (Figures 2C, 3, 4).
The analysis presented here identifies several features of Ophiomorpha that appear to influence reservoir porosity strongly. These include the thickness of the peloidal wall, which usually decrease porosity, and the relative volume occupied by every burrow part (i.e., fill and wall), as the fill usually presents higher porosity values. Overlapping between burrows can also influence porosity. The balance between dissolution and precipitation that occurs as part of burrow-associated diagenesis of the fill also determines the structure and volume of void space. The results reported here demonstrate the importance of burrow-associated diagenesis which, in association with other burrow features (i.e., orientation, architecture, shape, etc.), can guide or hinder fluid flow. In sum, primary sediment fabric, sediment composition, tracemaker behavior, burrow morphology, and diagenetic processes can all significantly influence porosity and permeability. Thus, each of the aforementioned parameters requires rigorous and systematic analysis at different scales when evaluating their impact on reservoir quality. Future research in applied ichnology should seek to further establish precise and accurate paradigms of how bioturbation impacts reservoir properties.
Data availability statement
The original contributions presented in the study are included in the article/Supplementary Material, further inquiries can be directed to the corresponding author.
Author contributions
All the authors performed fieldwork and sampling. OM-S coordinated the project, and conducted the Micro-CT processing and quantitative analysis. All the authors discussed the results and collaborated in writing the manuscript.
Funding
This research was funded by Grants PID 2019-104625RB-100 by MCIN/AEI/10.13039/501100011033, by FEDER/Junta de Andalucía-Consejería de Economía y Conocimiento/Projects P18-RT- 4074, projects B-RNM-072-UGR18 and A-RNM-368-UGR20 (FEDER Andalucía) and PPJIA2021-07 (University of Granada), Research Group RNM-178 (Junta de Andalucía), and Scientific Excellence Unit UCE-2016-05 (Universidad de Granada). The research of OM-S is funded through a postdoctoral grant from the University of Granada (Ayuda Puente Doctores) and the Humboldt Postdoctoral Fellowship. The research by JD was funded through the Juan de la Cierva Program (IJC 2019-038866-I) by the Spanish Ministry of Science and Innovation.
Acknowledgments
We thank Fátima Linares Ordoñez for her help during X-ray microtomography analysis. We thank the two reviewers, whose comments contributed to improve this manuscript.
Conflict of interest
The authors declare that the research was conducted in the absence of any commercial or financial relationships that could be construed as a potential conflict of interest.
Publisher’s note
All claims expressed in this article are solely those of the authors and do not necessarily represent those of their affiliated organizations, or those of the publisher, the editors and the reviewers. Any product that may be evaluated in this article, or claim that may be made by its manufacturer, is not guaranteed or endorsed by the publisher.
Supplementary material
The Supplementary Material for this article can be found online at: https://www.frontiersin.org/articles/10.3389/feart.2022.1010729/full#supplementary-material
References
Alqubalee, A., Muharrag, J., Salisu, A. M., and Eltom, H. (2022). The negative impact of Ophiomorpha on reservoir quality of channelized deposits in mixed carbonate siliciclastic setting: The case study of the dam formation, Saudi arabia. Mar. Petroleum Geol. 140, 105666. doi:10.1016/j.marpetgeo.2022.105666
Baniak, G. M., Gingras, M. K., Burns, B. A., and Pemberton, S. G. (2015). Petrophysical characterization of bioturbated sandstone reservoir facies in the upper Jurassic Ula formation, Norwegian North Sea, Europe. J. Sediment. Res. 85 (1), 62–81. doi:10.2110/jsr.2015.05
Baniak, G. M., La Croix, A. D., and Gingras, M. K. (2022). Recent advancements in characterizing permeability and porosity distributions in bioturbated flow media. Earth-Science Rev. 232, 104162. doi:10.1016/j.earscirev.2022.104162
Baniak, G. M., La Croix, A. D., Polo, C. A., Playter, T. L., Pemberton, S. G., and Gingras, M. K. (2014). Associating X-ray microtomography with permeability contrasts in bioturbated media. Ichnos 21 (4), 234–250. doi:10.1080/10420940.2014.958224
Cabrera-Ortiz, J. F., Rodríguez-Tovar, F. J., and Dorador, J. (August 2022). .Ichnological analysis of the Rambla de Tabernas section (Tabernas Basin, SE Spain), a preliminary approach to improve characterization of a turbiditic system, Proceedings of the 21st International Sedimentological Congress. Beijing, China, Beijing: Abstract Book, 580,,
Cunningham, K. J., Sukop, M. C., Huang, H., Alvarez, P. F., Curran, H. A., Renken, R. A., et al. (2009). Prominence of ichnologically influenced macroporosity in the karst Biscayne aquifer: Stratiform “super-K” zones. Geol. Soc. Am. Bull. 121 (1-2), 1–180. doi:10.1130/B26392.1
Cunningham, K. J., Sukop, M. C., and Curran, H. A. (2012). “Carbonate aquifers,” in Trace fossils as indicators of sedimentary environments. Editors D. Knaust, and R. G. Bromley (Elsevier), Amsterdam, Netherlands, 869–896. Developments in Sedimentology 64. doi:10.1016/B978-0-444-53813-0.00028-9
Dawson, W. C., Reaser, D. F., and Richardson, J. D. (1977). Trace fossils from the pecan gap formation (upper cretaceous), northeastern Texas. Tex. J. Sci. 29, 175–186.
De Matteis, M., Belaústegui, Z., Arbués, P., Granado, P., Cabello, P., Demko, T., et al. (May 2016). Ichnological analysis of the turbidite solitary channel complex from drillcore data (Miocene, Tabernas Basin, SE, Spain). Proceedings of the Ichnia 4th. Portugal, Lisbon, Portugal Idanha a Nova, 78–79.
Dorador, J., Rodríguez-Tovar, F. J., and Miguez-Salas, O. (2021). The complex case of Macaronichnus trace fossil affecting rock porosity. Sci. Rep. 11 (1), 1975–1977. doi:10.1038/s41598-021-81687-6
Doyle, P., Mather, A. E., Bennett, M. R., and Bussell, M. A. (1996). Miocene barnacle assemblages from southern Spain and their palaeoenvironmental significance. Lethaia 29, 267–274. doi:10.1111/j.1502-3931.1996.tb01659.x
Eltom, H. A., Rankey, E. C., Hasiotis, S. T., and Barai, R. (2019). Effect of bioturbation on petrophysical properties: Insights from geostatistical and flow simulation modeling. Mar. Petroleum Geol. 104, 259–269. doi:10.1016/j.marpetgeo.2019.03.019
Gingras, M. K., Baniak, G., Gordon, J., Hovikoski, J., Konhauser, K. O., La Croix, A., et al. (2012). “Porosity and permeability in bioturbated sediments,” in Trace fossils as indicators of sedimentary environments. Editors D. Knaust, and R. G. Bromley (Elsevier), Amsterdam, Netherlands, 837–868. Developments in Sedimentology 64. doi:10.1016/B978-0-444-53813-0.00027-7
Gingras, M. K., Pemberton, S. G., Muehlenbachs, K., and Machel, H. (2004). Conceptual models for burrow-related, selective dolomitization with textural and isotopic evidence from the Tyndall Stone, Canada. Geobiology 2, 21–30. doi:10.1111/j.1472-4677.2004.00022.x
Haughton, P. D. W. (2000). Evolving turbidite systems on a deforming basin floor, Tabernas, SE Spain. Sedimentology 47, 497–518. doi:10.1046/j.1365-3091.2000.00293.x
Hodgson, D. M., and Haughton, P. D. W. (2004). Impact of syndepositional faulting on gravity current behaviour and deep-water stratigraphy: Tabernas-Sorbas Basin, SE Spain. Geol. Soc. Lond. Spec. Publ. 222, 135–158. doi:10.1144/GSL.SP.2004.222.01.08
Hodgson, D. M. (2002). Tectono-stratigraphic evolution of a Neogene oblique extensional orogenic basin, southeast Spain. London, UK: Ph.D. thesis). University of London, 478.
Hose, G. C., and Stumpp, C. (2019). Architects of the underworld: Bioturbation by groundwater invertebrates influences aquifer hydraulic properties. Aquat. Sci. 81, 20–29. doi:10.1007/s00027-018-0613-0
Kleverlaan, K. (1989). Three distinctive feeder-lobe systems within one time slice of the Tortonian Tabernas fan, SE Spain. Sedimentology 36, 25–45. doi:10.1111/j.1365-3091.1989.tb00818.x
Knaust, D. (2017). Atlas of trace fossils in well core. Springer International Publishing. New York, NY, USA,
Knaust, D. (May 2013). “Bioturbation and reservoir quality: Towards a genetic approach,” in Proceedings of the AAPG Annual Convention and Exhibition, Search and Discovery. 50900, Pittsburgh, PA, USA,
Knaust, D. (2014). Classification of bioturbation-related reservoir quality in the khuff formation (Middle East): Towards a genetic approach. Permo-triassic sequence of the arabian plate, EAGE, The Hague, Netherlands, 247–267,
Knaust, D., Dorador, J., and Rodríguez-Tovar, F. J. (2020). Burrowed matrix powering dual porosity systems–a case study from the Maastrichtian chalk of the Gullfaks Field, Norwegian North Sea. Mar. Petroleum Geol. 113, 104158. doi:10.1016/j.marpetgeo.2019.104158
La Croix, A. D., MacEachern, J. A., Ayranci, K., Hsieh, A., and Dashtgard, S. E. (2017). An ichnological-assemblage approach to reservoir heterogeneity assessment in bioturbated strata: Insights from the lower cretaceous viking formation, alberta, Canada. Mar. Petroleum Geol. 86, 636–654. doi:10.1016/j.marpetgeo.2017.06.024
Leaman, M., McIlroy, D., Herringshaw, L. G., Boyd, C., and Callow, R. H. T. (2015). What does Ophiomorpha irregulaire really look like? Palaeogeogr. Palaeoclimatol. Palaeoecol. 439, 38–49. doi:10.1016/j.palaeo.2015.01.022
MacEachern, J. A., Pemberton, S. G., Gingras, M. K., and Bann, K. (2007). Applied ichnology. SEPM short course notes 52. Cambridge University Press, Cambridge, UK,
Miguez-Salas, O., Dorador, J., and Rodríguez-Tovar, F. J. (2019). Introducing Fiji and ICY image processing techniques in ichnological research as a tool for sedimentary basin analysis. Mar. Geol. 413, 1–9. doi:10.1016/j.margeo.2019.03.013
Miguez-Salas, O., Dorador, J., Rodríguez-Tovar, F. J., and Linares, F. (2022). X-Ray microtomography analysis to approach bioturbation's influence on minor-scale porosity distribution: A novel approach in contourite deposits. J. Petroleum Sci. Eng. 208, 109251. doi:10.1016/j.petrol.2021.109251
Netto, R. G., Curran, H. A., Belaústegui, Z., and Tognoli, F. M. (2017). Solving a cold case: New occurrences reinforce juvenile callianassids as the Ophiomorpha puerilis tracemakers. Palaeogeogr. Palaeoclimatol. Palaeoecol. 475, 93–105. doi:10.1016/j.palaeo.2017.03.013
Oliveira de Araújo, O. M., Aguilera, O., Coletti, G., Valencia, F. L., Buatois, L. A., and Lopes, R. (2021). X-Ray micro-computed tomography of burrow-related porosity and permeability in shallow-marine equatorial carbonates: A case study from the Miocene pirabas formation, Brazil. Mar. Petroleum Geol. 127, 104966. doi:10.1016/j.marpetgeo.2021.104966
Pemberton, S. G., and Gingras, M. K. (2005). Classification and characterizations of biogenically enhanced permeability. Am. Assoc. Pet. Geol. Bull. 89, 1493–1517. doi:10.1306/07050504121
Postma, G., Kleverlaan, K., and Cartigny, M. J. (2014). Recognition of cyclic steps in sandy and gravelly turbidite sequences, and consequences for the Bouma facies model. Sedimentology 61 (7), 2268–2290. doi:10.1111/sed.12135
Quaye, J. A., Jiang, Z., and Zhou, X. (2019). Bioturbation influence on reservoir rock quality: A case study of well bian-5 from the second member paleocene funing formation in the jinhu sag, subei basin, China. J. Petroleum Sci. Eng. 172, 1165–1173. doi:10.1016/j.petrol.2018.09.026
Rodríguez-Tovar, F. J., Míguez Salas, O., and Dorador, J. (2021). Mercury intrusion porosimetry to evaluate the incidence of bioturbation on porosity of contourites. Riv. Ital. Paleontol. Stratigr. 127, 149–161. doi:10.13130/2039-4942/15208
Sanz de Galdeano, C., and Vera, J. A. (1992). Stratigraphic record and palaeogeographical context of the Neogene basins in the Betic Cordillera, Spain. Basin Res. 4, 21–36. doi:10.1111/j.1365-2117.1992.tb00040.x
Schindelin, J., Arganda-Carreras, I., Frise, E., Kaynig, V., Longair, M., Pietzsch, T., et al. (2012). Fiji: An open source platform for biological-image analysis. Nat. Methods 9 (7), 676–682. doi:10.1038/nmeth.2019
Keywords: bioturbation, petrophysical properties, Micro-CT, reservoir quality, diagenesis
Citation: Miguez-Salas O, Cabrera Ortiz J, Dorador J and Rodríguez-Tovar FJ (2022) The puzzling influence of Ophiomorpha (trace fossil) on reservoir porosity: X-ray microtomography analysis. Front. Earth Sci. 10:1010729. doi: 10.3389/feart.2022.1010729
Received: 03 August 2022; Accepted: 07 November 2022;
Published: 17 November 2022.
Edited by:
Linda Ivany, Syracuse University, United StatesReviewed by:
Andrew K. Rindsberg, University of West Alabama, United StatesFranek Hasiuk, University of Kansas, United States
Copyright © 2022 Miguez-Salas, Cabrera Ortiz, Dorador and Rodríguez-Tovar. This is an open-access article distributed under the terms of the Creative Commons Attribution License (CC BY). The use, distribution or reproduction in other forums is permitted, provided the original author(s) and the copyright owner(s) are credited and that the original publication in this journal is cited, in accordance with accepted academic practice. No use, distribution or reproduction is permitted which does not comply with these terms.
*Correspondence: Javier Dorador,amF2aWRyQHVnci5lcw==