- 1Wuhan Center, China Geological Survey (Central South China Innovation Center for Geosciences), Wuhan, China
- 2Faculty of Engineering, China University of Geosciences, Wuhan, China
- 3China Institute of Geo-Environment Monitoring, Beijing, China
- 4Hydrogeology and Engineering Geology Team of Chongqing Bureau of Geology and Minerals Exploration, Chongqing, China
The reactivation mechanism of old landslides has been extensively studied from building load, erosion of the slope toe, heavy rainfall, and slope cutting for existing research. However, previous research on the reactivation of old landslides pays little attention to the combined action of engineering disturbance and heavy rainfall is rarely studied. This paper describes an old landslide in Wushan County, Chongqing, China, that was reactivated in August 2019 due to engineering disturbance and heavy rainfall. The deformation of the old landslide was first observed in 2007 and 2008, resulting from excavation and rainfall, respectively, and remained stable for about 11 years after treatment. In August 2019, the landslide was reactivated by slope cutting and damaged anti-sliding piles at the toe, and entered a state of imminent sliding due to the concentrated heavy rainfall events that occurred from October 4 to 22, 2019. In order to reveal the deformation features and reactivation mechanism of the landslide, field investigations, drilling activities and monitoring were performed. The results showed that tectonic effects and the stratigraphic lithology were the main reasons for the formation of the old Dashuitian landslide. The cut slope and damaged anti-sliding piles at the toe of the landslide provided the sliding space and reduced the anti-sliding force, and therefore resulted in the reactivation of the landslide. Continuous intense rainfall increased the weight of the landslide, decreased the mechanical properties and increased the pore water pressure of the weak interlayer, which accelerated the deformation rate. Therefore, 1.5 million m3 of rock and soil masses slid along the weak interlayer under the action of gravity, threatening the safety of Wuliu Road, Ring Road, National Road G42 and the Wu-Da Expressway. Our research provides a theoretical basis for reducing the hazard of similar engineering projects involving slopes.
1 Introduction
In recent years, hundreds of old landslides in China have been reactivated under a combination of internal and external geological dynamics and have resulted in major property damage and severe casualties (Yin et al., 2010; Guo et al., 2019). Examples include the Huangtupo landslide in Badong Town (Fourniadis et al., 2007), the Sanmendong landslide in Zigui County (Sun et al., 2016; Huo et al., 2019), the Liangshuijing landslide in Yunyang County (Xiao et al., 2013; Yin et al., 2016; Li et al., 2020) and the Baiyangwan landslide in Wushan Town (Zhang et al., 2020).
The reactivation of an old landslide is usually the result of multiple factors, among which heavy rainfall and disturbance from engineering projects are considered to be the most important (Cruden and Varnes 1996; Chen et al., 2014). Many old landslides have reactivated under the combined action of human engineering activities and concentrated rainfall. Zhang et al. (2020) reported that the old Baiyangwan landslide showed long-term creep due to the combined effects of building load, open excavation and heavy rainfall. By analyzing the deformation features of the old Outang landslide, Dai et al. (2016) reported that erosion of the slope toe by the Yangtze River, concentrated rainfall and reservoir impoundment were the main inducing factors leading to the reactivation of the landslide. Huang et al. (2020) investigated the reactivation mechanism of the old Dalixi landslide and found that excavation and rainfall together promoted the continuous deformation of the landslide. Due to seasonal heavy rainfall, overloading and slope cutting, the old Jiaju landslide in Danba county was reactivated and has undergone progressive retrograde failure since July 1992 (Li et al., 2008; Yin et al., 2010). With respect to old landslides, due to the existence of the old sliding zone and old cracks, the disturbance caused by excavation and heavy rainfall is more obvious and more likely to lead to landslide instability (Zhang et al., 2020). But how the combined action of the two factors affects the stability of an old landslide is little known.
The Dashuitian landslide, occurred in Wushan County, Chongqing, China, provided an opportunity to study reactivation mechanism of an old landslide induced by excavation and rainfall. Due to excavation that occurred in August 2019, the landslide reactivated and began to slowly slide, and professional monitoring was initiated on 30 August 2019. In October 2019, continuous and concentrated rainfall events promoted the deformation rate, and the landslide entered a state of imminent sliding. The total volume of the activated zone was about 150 m3 × 104 m3, the average thickness was about 25 m, and the main sliding direction was about 7°. From August to December 2019, the total accumulated displacement of the Dashuitian landslide was about 150 mm. The landslide directly threatened the safety of Wuliu Road, Ring Road, G42 and the Wu-Da Expressway. The potential economic losses exceed 100 million CNY.
Based on detailed field investigations, drilling activities and field monitoring data, this study presents the particular characteristics of the Dashuitian landslide and its deformation features. The triggering factors were explored, and the reactivation mechanism was preliminarily analyzed. The effects of slope cutting and continuous intense rainfall on the reactivation of the old landslide were also examined. Considering that a large number of old landslides in China have been triggered by engineering disturbances and concentrated rainfall, we believe this study will provide useful guidelines and suggestions for the prevention and control of similar landslide events.
2 The Dashuitian landslide
2.1 General description
The Dashuitian landslide is located in Wushan County, Chongqing, China, which has undergone rapid development. The severe deformation of the landslide occurred in August 2019 (Figures 1A–C ). The topography of the landslide area is high in the south and low in the north. The rear part of the Dashuitian landslide has an elevation of 540 m compared to 460 m at the leading edge, which is a height difference of 80 m. The angle of the slope is approximately 20°–25°. The G42 Expressway is located at approximately 80 m outside the northern boundary of the landslide. The leading edge of the landslide is a multistage stepped slope with an average angle of about 35°, which was excavated during the Wu-Da Expressway Interchange construction (Figure 1C). Ring Road is located at the middle-rear part of the landslide (Figure 1B). The rear edge of the landslide is bounded by Wuliu Road (Figure 1A). In addition, a gully has developed along the western edge of the landslide (Figures 1A, 2).
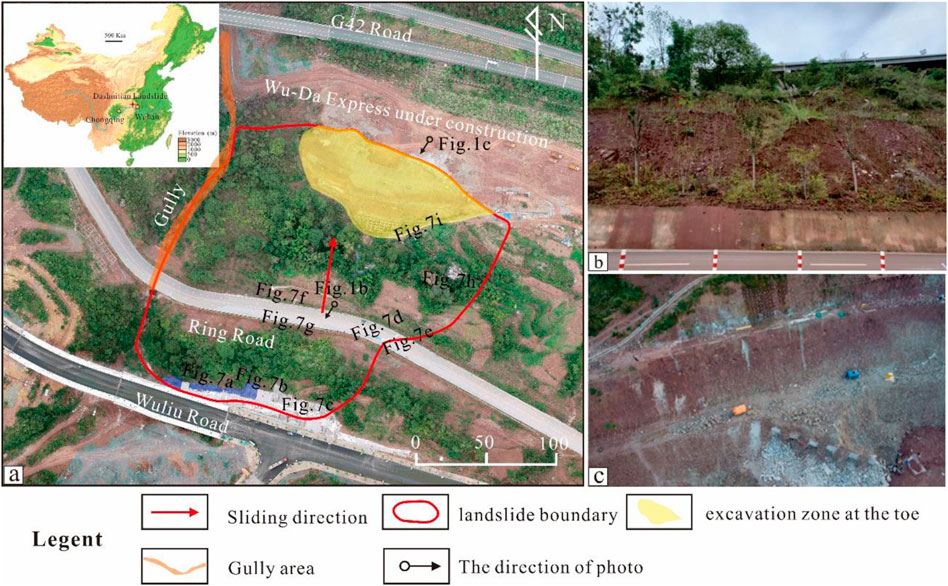
FIGURE 1. Photos of (A)—location and overview of the Dashuitian landslide, (B)—sliding body at the middle-rear part of the landslide, and (C)—excavation zone and broken anti-sliding piles at the toe of the landslide damaged during excavation.
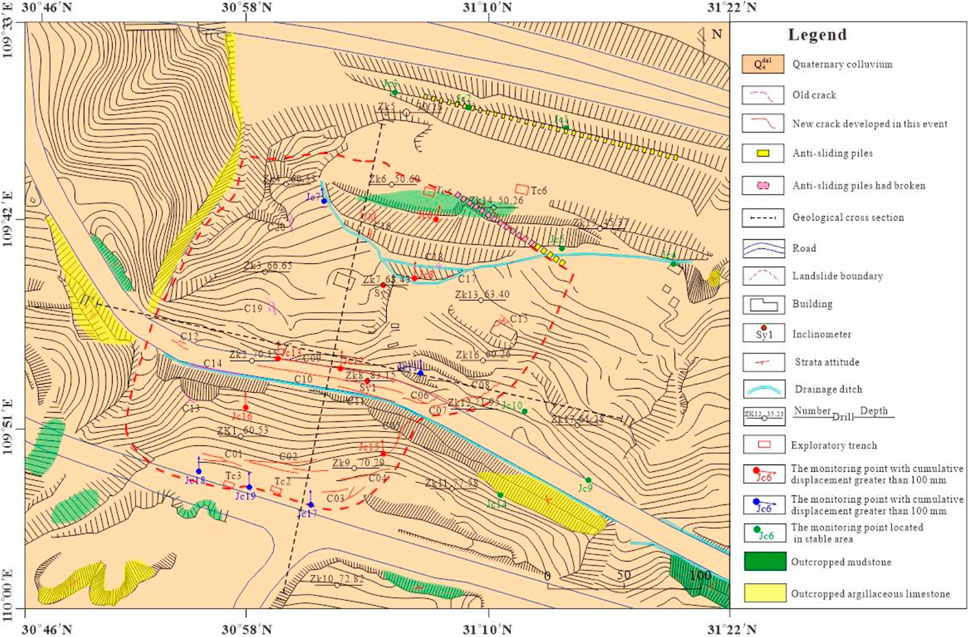
FIGURE 2. Layout map of the landslide area, showing the stratigraphic boundaries, landslide boundary and the location of boreholes, monitoring points, inclinometers, exploratory trenches and cracks. C13, C14, C18, C19, and C20 are old cracks induced by previous failure events, and the others are new cracks caused by this failure event.
The landslide area is located at the south east wing of the Baishuihe syncline, which is the secondary fold of the Wushan syncline. The strata in the study area are monoclinal in structure with a northward dip, and the geological units, from top to bottom, include Quaternary landslide deposits (Q4del), and argillaceous limestone and mudstone of the Middle Triassic Badong Formation (T2b2) (Figure 3). The study area has a monsoon-influenced warm and humid subtropical climate with an average annual precipitation of 1049.5 mm. The rainy season mainly occurs from May to September, while the dry season occurs from December to February.
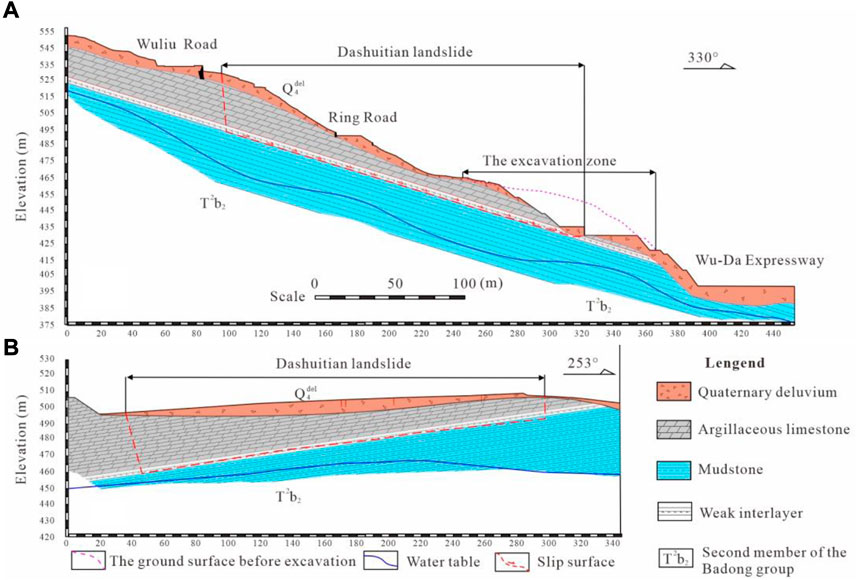
FIGURE 3. Cross sections (sections shown in Figure 2) of the Dashuitian landslide. (A)—Geological I-I′ cross-section; (B)—Geological II-II′ cross-section. The blue lines are the groundwater levels.
2.2 Hydrogeological conditions
The study area is a sloping terrain with good drainage conditions, and there is no surface water in the area. Based on the occurrence and migration conditions, the groundwater can be divided into pore water of loose rock and fissure water of bedrock, and mainly flows from the southeast to the northwest. According to the investigation and drilling data, groundwater in the flat areas of the south and north boundaries of the Dashuitian landslide is abundant, while in the landslide area the groundwater is deep with no evident springs (Figures 2, 3). The pumping test results of the Zk6 and Zk10 boreholes showed that the permeability coefficients of the rock and soil masses range between 0.145 and 0.167 m/d, corresponding to a medium permeability layer.
3 Material composition
3.1 Sliding mass
The sliding mass consists of Quaternary landslide deposits (Q4del) and argillaceous limestone of the Middle Triassic Badong Formation (T2b2). The composition of the sliding mass varies greatly in the vertical direction. The upper part is a thin layer of gravel soil, and the lower part is a thick layer of broken limestone (Figure 3). Based on remote sensing images (Figure 1), the horizontal length of failed material is about 250 m, the maximum width is about 240 m, and the plane distribution area is about 6 × 104 m2. In addition, the field investigation and drilling data revealed that the sliding direction of the landslide is 7°, the average thickness of the sliding body is about 25 m, and the unstable volume is about 150 m3 × 104 m3.
3.2 Sliding zone
The sliding zone occurs at depths of 7.5 (Zk6) to 32.9 m (Zk1) and is the contact zone between the argillaceous limestone and mudstone of the Middle Triassic Badong Formation (T2b2) with a thickness of about 0.8–1.3 m (Figures 4A–C). The dip angle of the sliding surface is about 17–25° and exhibits characteristics of deep burial depth in the southwest and shallow burial depth in the northeast. There is a weak interlayer in the sliding zone, which is composed of broken argillaceous limestone and mudstone clasts (Figures 3, 4B,C). Many polished surfaces, scratch, and slickensides were observed in the sliding zone (Figures 4D–G). Based on the results of direct shear tests, the mechanical properties of the sliding zone materials are poor, especially in the saturated state (Table 1).
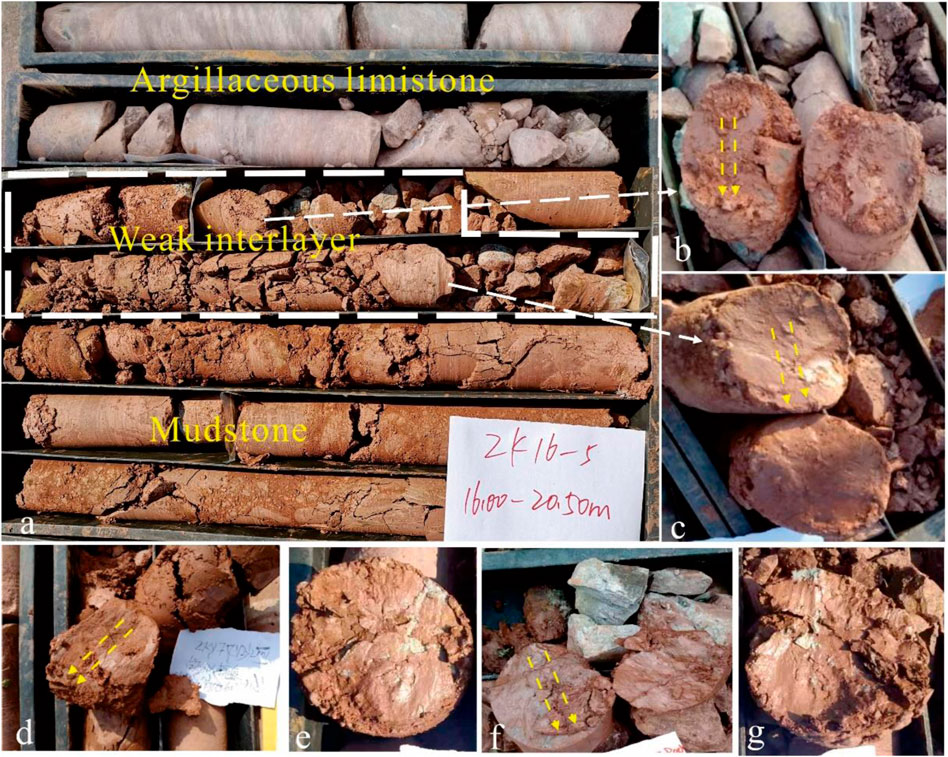
FIGURE 4. (A) is a weak interlayer in the sliding zone composed of broken mudstone and argillaceous limestone, which is revealed in Zk16, located at 17.5–18.6 m with a thickness of 1.1 m; (B,C) are argillaceous limestone and weathered mudstone, as revealed in Zk16, with respective depths of 17.7 m and 18.1 m; and (D–G) are revealed in Zk3, Zk7, Zk8 and Zk9, respectively, and contain many polished surfaces and slickensides.
3.3 Sliding bed
The sliding bed is composed of the mudstone, and the attitude of the strata is 330° with a dip of 25°. The mudstone is purple, features a medium-thick layered structure and appears relatively intact compared with the overlying argillaceous limestone. However, the mudstone near the sliding zone is highly weathered due to softening by groundwater (Figure 5).
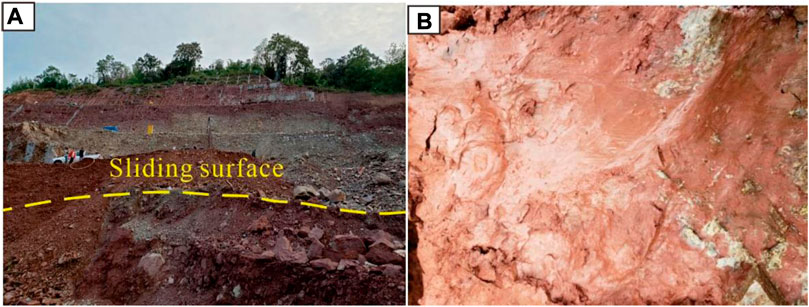
FIGURE 5. (A) is the sliding zone revealed by the excavation; (B) is the softened sliding zone exposed by TC5 (Figure 2) at a depth of 16 m.
4 Human engineering activities
In the past 20 years, with rapid economic development, many human engineering disturbances have taken place in the landslide area, including along Wuliu Road at the rear part of the landslide, Ring Road at the middle–rear part, the Wu-Da Expressway at the toe and the G42 Expressway at the leading edge. Both Wuliu Road and Ring Road cross the sliding body, forming subgrade slopes with lengths of 450 and 480 m, and heights of 1–12 and 15–30 m, respectively (Figure 1). The G42 Expressway is located at approximately 80 m outside the northern boundary of the landslide and forms a subgrade slope with a length of 450 m, a height between 25 and 35 m and an average slope angle of 50°. 18 anti-sliding piles, with a length of 28–30 m, a cross section of 2 m×3 m and a buried depth of 15–25 m, were established at the landslide toe to prevent its deformation caused by heavy rainfall in August 2008. In August 2019, 30 m3 × 104 m3 of rock and soil masses was excavated, and 13 anti-sliding piles were destroyed at the toe of the landslide during the construction of the Wu-Da Expressway. As a result of the excavations, three high-steep slopes were formed at the toe with heights between 25 and 35 m and a slope angle of 35° (Figure 1C).
5 Landslide deformation characteristics
5.1 Field investigation
Five typical old cracks (C13, C14, C18, C19, C20) were developed prior to this event and were revealed during a detailed field investigation (Figures 2, 6). C13 is a tension crack of about 30 m in length, 5–20 cm in width and 50 cm in depth, and is located at the rear part of the Dashuitian landslide (Figure 6). C14 is also a tension crack that developed at the middle-rear part of the landslide with a length of 2 m and width of 2–4 cm (Figure 6). C18 is a shear crack located at the leading part of the landslide (Figure 6). The investigation indicated that these cracks are historical cracks that were induced by the excavation of the G42 Expressway in 2007 and 2008.
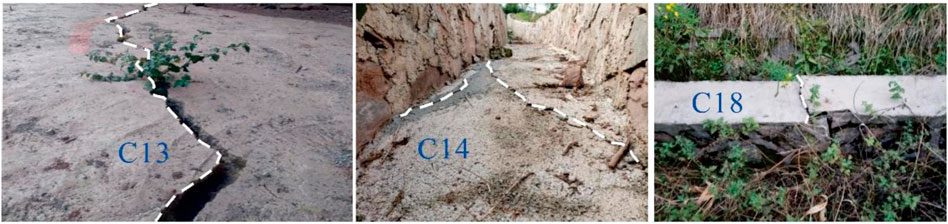
FIGURE 6. The typical old cracks developed in 2007 and 2008 due to deformation of the old Dashuitian landslide.
The new deformation signs of the Dashuitian landslide that were identified during the field investigation mainly include cracks on the roads and ground surface (Figure 7). Deformation signs became evident after the heavy rainfall event of October 4 to 22, 2019, when about 228 mm of precipitation fell (Figure 8). The new deformation signs mainly developed at the leading part, rear edge, the middle-rear part and eastern edge of the landslide. C15, C16 and C17 developed at the leading part of the landslide, reaching a length and width of 7–10 m and 1–2 cm, respectively. The deformation signs at the rear edge developed along Wuliu Road and consist of several transverse tension cracks, such as C01 and C02. The length of these is 20–50 m, the width is 1–40 cm and the visible depth is 20–200 cm. C09–C12 are large tension cracks along Ring Road at the middle-rear part of the landslide with a length of 8–120 m. C03–C08 are feathery shear cracks, located along the eastern edge of the landslide, with a length of 8–40 m, width of 1–25 cm and depth of 1–50 cm. Overall, the greatest deformation mainly occurs at the middle-rear part of the Dashuitian landslide, while the deformation in the leading part is relatively minor (Figure 2).
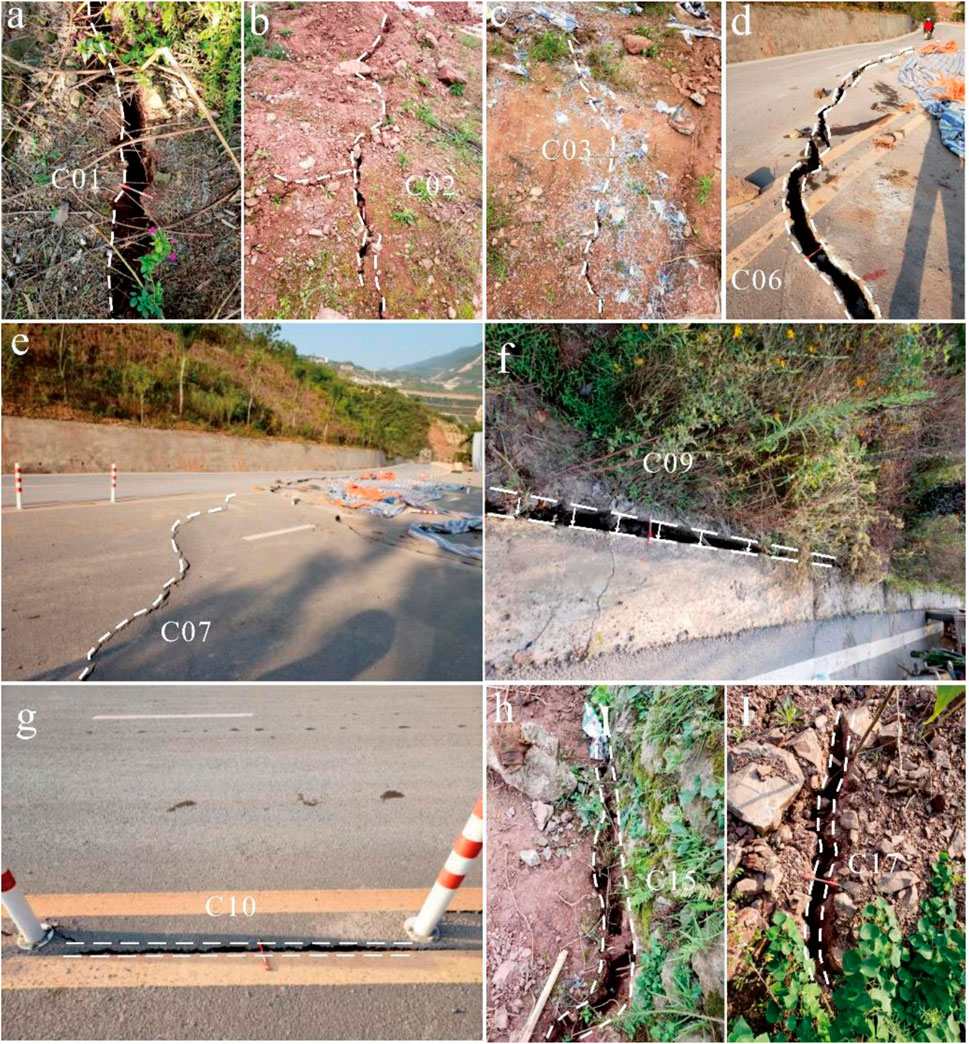
FIGURE 7. New deformation signs of the Dashuitian landslide. (A,B) are tensile cracks located at the rear part; (C–E) are shear cracks located at the eastern edge; (F,G) are large tensile cracks develop along Ring Road at the middle-rear part; (H,I) are tensile cracks located at the leading part.
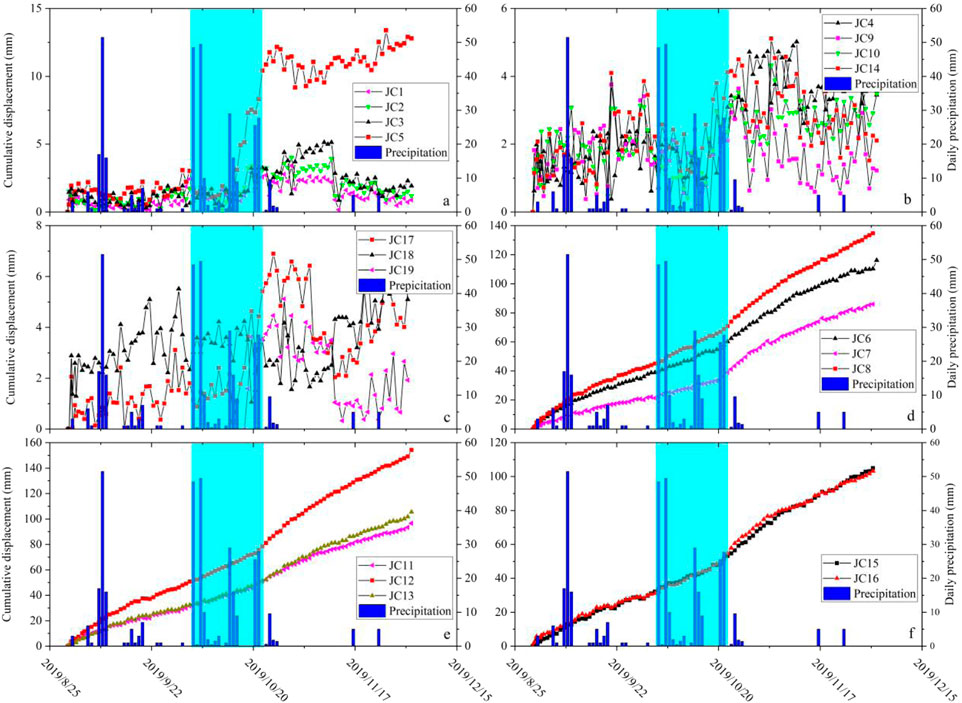
FIGURE 8. Monitoring results of the cumulative displacement and daily precipitation from August 30 to 2 December 2019. (A–C) are boundary control points; (D) is located at the open excavation zone in the leading part of the landslide; (E) is located along Ring Road; and (F) has been installed at the rear part of the landslide. The cyan section corresponds to the period from October 4 to 22, 2019, during which a continuous heavy rainfall event occurred, with a total precipitation of 228 mm.
5.2 GNSS-based monitoring data
After 30 August 2019, we installed GNSS (Global Navigation Satellite Systems) in the study area to monitor the ground deformation, as shown in Figure 2. Based on the results of the field investigation and the geomorphological features of the study area, the gully located at the western edge of the landslide was determined as the western boundary. Moreover, in order to further determine the northern, southern and eastern boundaries of the landslide, monitoring points JC1, JC2, JC3, and JC5 were installed on the northern side, JC17, JC18, and JC19 were developed at the southern edge, and JC4, JC9, JC10, and JC14 were situated along the eastern side. The monitoring results showed that the cumulative displacement of all 11 monitoring points was about 2–10 mm, and the deformation rate was only 0.02–0.09 mm/d from August 30 to 2 December 2019, indicating that the positions of the monitoring points are relatively stable (Figures 8A–C).
Regarding the activated zone, two typical stages in the deformation curves are shown in Figures 8D–F, which correspond perfectly with the concentrated rainfall events from October 4 to 22, 2019, with a total precipitation of 228 mm. Monitoring points JC6, JC7, and JC8, which are located at the open excavation zone in the leading part of the landslide, had a maximum deformation rate of 1.29 mm/day before the rainfall event and 1.61 mm/day after the rainfall event. The highest deformation rate of monitoring points JC11, JC12 and JC13, located at Ring Road in the middle part of the landslide, reached approximately 1.43 mm/day and 1.87 mm/day before and after the rainfall event, respectively. The highest deformation rate of monitoring points JC15 and JC16, located at the rear part of the landslide, was approximately 0.97 mm/day and 1.25 mm/day before and after the rainfall event, respectively. In addition, the total cumulative displacement in the leading (JC6, JC7, and JC8), middle (JC11, JC12, and JC13) and rear (JC15, JC16) parts of the landslide were about 85.9–134.7, 96.5–154.3, and 103.1–105 mm, respectively.
5.3 Subsurface deformation characteristics
Two inclinometers were used to measure the subsurface deformation, and their positions are shown in Figure 2. The range of the inclinometer used in this monitoring was ±15°, and the accuracy was 0.01 mm/500 mm.
The monitoring displacement curve of SY01 indicates that the displacement increased gradually from the bottom to the top (Figure 9). An obvious sliding surface was evident between 17 and 20 m. From October 9 to 2 December 2019, the cumulative horizontal displacement and deformation rate above the sliding surface reached 78 mm and 1.5 mm/day, respectively. Based on data from borehole Zk16 (Figure 4A), the weak interlayer was inferred at a depth of 16–20.5 m, which is the contact zone between the argillaceous limestone and mudstone. Given that scratches were apparent in the borehole core, the contact zone is considered to be a sliding surface.
The monitoring curve of SY02 shows an obvious sliding surface at a depth of 19–21 m, and the unstable rock and soil masses above the sliding surface moved as a whole, suggesting that the Dashuitian landslide has a well-defined deep sliding surface (Figures 3, 9). The cumulative horizontal displacement and deformation rate above the sliding surface reached 48 mm and 1.6 mm/day from October 20 to 2 November 2019. Based on information from borehole Zk7 (Figure 4E), the contact zone between the argillaceous limestone and mudstone is located at 16.7–19.2 m, and the sliding surface is interpreted to be located near this contact zone.
In conclusion, the locations of the sliding surface revealed by the two inclinometers (Figure 9) are nearly consistent with the contact zone between the argillaceous limestone and mudstone revealed by the boreholes (Figure 4).
6 Discussion
6.1 Influencing factors of the Dashuitian landslide
The reactivation mechanism of landslides has been extensively studied from building load (Zhang et al., 2020), erosion of the slope toe by river (Dai et al., 2016), heavy rainfall (Huang et al., 2020) and slope cutting (Li et al., 2008; Yin et al., 2010) for previous research. With respect to old landslides, the disturbance caused by excavation and heavy rainfall is more obvious and more likely to lead to landslide instability due to the existence of the old sliding zone and old cracks (Zhang et al., 2020). However, the combined action of engineering disturbance and heavy rainfall on the reactivation of old landslides is rarely studied. Under such scenarios, we mainly focus on the predisposing factors (including tectonic effect and formation lithology) and triggering factors (including rainfall and human engineering activities) of the Dashuitian landslide to study its reactivation mechanism. Our research may provide a theoretical basis for reducing the hazard of similar engineering projects involving slopes.
6.2 Predisposing factors
6.2.1 Tectonic effect
The study area is located in the northwest wing of the Wushan syncline where the Baishuihe syncline is developed. Due to the effects of regional tectonic action, the rock and soil masses in the landslide area are extremely broken (Figure 4) and easily infiltrated by rainwater and groundwater.
6.2.2 Formation lithology
Broken rock and soil masses compose the Quaternary colluvium layer of the Dashuitian landslide, which is rapidly infiltrated by rainfall and surface water due to its loose structure and good permeability. The underlying layer is argillaceous limestone, where joints and fractures are well developed due to tectonic compression (Chai et al., 2013; Yan et al., 2019). These joints and fractures provide channels for the rapid infiltration of rainwater. The bedrock is mudstone, which is relatively intact and has poor permeability compared with the upper argillaceous limestone. Therefore, rainwater can rapidly infiltrate to the contact surface between argillaceous limestone and mudstone, and form stagnant water in the sliding zone, especially during rainfall. A weak interlayer therefore presents near the contact surface, which is heavily weathered, has poor mechanical properties and is prone to slippage (Zhang et al., 2020).
6.3 Triggering factors
6.3.1 Human engineering activities
During the construction of the Wuliu and Ring roads, the slope body showed no obvious deformation signs. In 2007, due to the construction of the G42 Expressway, a large-scale excavation was carried out at about 80 m outside the toe of the landslide, causing apparent deformation for the first time. In order to control the deformation of the slope, 33 anti-sliding piles were established at the toe (Figure 2). However, under the influence of concentrated rainfall in July and August 2008, new deformation occurred. An additional 18 anti-sliding piles were therefore established at the slope toe (Figure 2). No obvious deformation had occurred since then. In August 2019, 30 m3 × 104 m3 of rock and soil masses was excavated from the toe area during the construction of the Wu-Da Expressway, which provided the necessary conditions for reactivation. In addition, the anti-sliding force of the landslide was reduced due to damage to 13 of the anti-sliding piles at the toe (Figures 1C, 2), which triggered the overall slow deformation of the Dashuitian landslide during August 30 to 22 October 2019 (Figure 8).
6.3.2 Rainfall
Prior to the construction of the Wu-Da Expressway, the maximum daily precipitation recorded in Wushan County was 384.6 mm on 31 August 2014 (Yan et al., 2019), which is much higher than the total precipitation of 228 mm that fell from October 4 to 22, 2019 (Figure 8). The maximum recorded daily precipitation (346.8 mm) did not induce any significant deformation to the Dashuitian landslide. However, following the construction of the Wu-Da Expressway in August 2019, the lower rainfall amount promoted the deformation rate of the landslide, which is supported by the GNSS-based monitoring data collected after 22 October 2019 (Figure 8). Therefore, compared with engineering activities, the effect of rainfall was not a decisive factor that led to the reactivation of the Dashuitian landslide. The concentrated heavy rainfall increased the pore water pressure and reduced the mechanical properties of the weak interlayer, increased the weight of the Dashuitian landslide, and finally promoted the deformation rate. Zhang, 2020.
6.4 Reactivation mechanism
The extensive excavation at the toe of the Dashuitian landslide provided the sliding space for reactivation. Meanwhile, the anti-sliding force of the landslide was significantly reduced due to damage to 13 of the anti-sliding piles located at the toe. Thus, in August 2019, the Dashuitian landslide slid slowly along the weak interlayer. Moreover, the sliding body is composed of loose Quaternary colluvium and argillaceous limestone broken by tectonic compression, which provide channels for the rapid infiltration of rainwater. The old cracks in particular, formed by the deformation of the old Dashuitian landslide, accelerated the infiltrate process. Rainwater can therefore quickly infiltrate the sliding body, increasing the water content of the rock and soil masses above the sliding zone, and increasing the sliding force of the landslide. Most importantly, the bedrock is composed of mudstone, which is relatively intact and has poor permeability compared with the upper argillaceous limestone. Therefore, the weak interlayer remained saturated during the heavy rainfall, which significantly increased its pore water pressure and reduced the shear strength. This dramatically reduced the anti-sliding force on the weak interlayer, thereby promoting the deformation rate of the Dashuitian landslide. Finally, the landslide entered a state of imminent sliding on 22 October 2019.
7 Conclusion
The Dashuitian landslide is an old landslide with a volume of 150 m3 × 104 m3, which reactivated in August 2019, and then entered a state of imminent sliding on 22 October 2019. The area of greatest deformation is mainly located at the middle-rear part of the landslide, while the deformation at the leading part is relatively minor. Based on the field investigation, monitoring data and drilling results, the reactivation mechanism of Dashuitian landslide could be deduced as follows. Tectonic effects and formation lithology are the main reasons for the formation of the old landslide. The slope cutting and the damaged anti-sliding piles at the landslide toe provided the sliding space, reduced the anti-sliding force and therefore resulted in the reactivation of the landslide. Moreover, the concentrated rainfall increased the weight of the Dashuitian landslide, reduced the mechanical properties and increased the pore water pressure of the weak interlayer and finally promoted the landslide to enter a state of imminent sliding. Our research may provide a theoretical basis for reducing the hazard of similar engineering projects involving slopes.
Data availability statement
The original contributions presented in the study are included in the article/supplementary material, further inquiries can be directed to the corresponding author.
Author contributions
All authors listed have made a substantial, direct, and intellectual contribution to the work and approved it for publication.
Funding
The work was supported by funding from National key R&D plan (2018YFC1505401-3), Natural Science Foundation of Hubei Province (Grant No. 2020CFB352), a follow-up of the Geological Disaster Prevention and Control Project in the Three Gorges area (Grant Nos 000121 2019C C60 001 and 000121 2021C C60 001), and Chongqing Natural Science Key program: Key Technology for Treatment of Massive Hydraulic Landslide in Three Gorges Reservoir Area (Grant No. cstc2020jcyj-zdxmX0019).
Acknowledgments
The authors would like to thank Xiannian Jiang, Baibing Jiang, Dan Luo, and Peng Jin from 208 Geological Team, Chongqing, for their great assistance in the field investigation and providing monitoring data.
Conflict of interest
The authors declare that the research was conducted in the absence of any commercial or financial relationships that could be construed as a potential conflict of interest.
Publisher’s note
All claims expressed in this article are solely those of the authors and do not necessarily represent those of their affiliated organizations, or those of the publisher, the editors and the reviewers. Any product that may be evaluated in this article, or claim that may be made by its manufacturer, is not guaranteed or endorsed by the publisher.
References
Chai, B., Yin, K. L., Du, J., and Xiao, L. L. (2013). Correlation between incompetent beds and slope deformation at Badong town in the Three Gorges Reservoir, China. Environ. Earth Sci. 69, 209–223. doi:10.1007/s12665-012-1948-9
Chen, C. L., He, K., and Li, T. L. (2014). Research on the mechanism of the ancient landslide resurrection triggered by slope toe excavation. Northwest. Geol. 47 (1), 255–260. in Chinese.
Cruden, D. M., and Varnes, D. J. (1996). Landslide types and processes, special report, transportation research board. Natl. Acad. Sci. 247, 36–75.
Dai, Z. W., Yin, Y. P., Wei, Y. J., Lu, T., Luo, J. H., and Wang, Y. (2016). Deformation and failure mechanism of outang landslide in three gorges reservoir area. J. Eng. Geol. 24 (1), 44–55. In Chinese. doi:10.13544/j.cnki.jeg.2016.01.006
Fourniadis, I. G., Liu, J. G., and Mason, P. J. (2007). Landslide hazard assessment in the Three Gorges area, China, using aster imagery: Wushan–Badong. Geomorphology 84 (1), 126–144. doi:10.1016/j.geomorph.2006.07.020
Guo, C. B., Zhang, Y. S., Li, X., Ren, S. S., Yang, Z. H., Wu, R. A., et al. (2019). Reactivation of giant jiangdingya ancient landslide in zhouqu county, gansu province, China. Landslides 17, 179–190. doi:10.1007/s10346-019-01266-9
Huang, X. H., Yi, W., Gong, C., Huang, H. F., and Yu, Q. (2020). Reactivation and deformation mechanism of ancient landslides by excavation. Chin. J. Geotechnical Eng. 42 (7), 1276–1285. in Chinese. doi:10.1155/2021/6614180
Huo, Z., Huang, B., Wang, L., Lu, S. Q., and Fan, Y. (2019). Potential surge risk of Sanmendong landslide in three gorges reservoir area. J. Catastrophology 8 (1), 454–461. doi:10.1080/21642583.2020.1788469
Li, M. H., Zheng, W. M., Shi, S. W., and Xie, Z. S. (2008). The revival mechanism and stability analysis to Jiaju landslide of Danba county in Sichuan province. J. Mt. Sci. 26 (5), 577–582. in Chinese. doi:10.21203/rs.3.rs-555768/v1
Li, Y., Utili, S., Milledge, D., Chen, L. X., and Yin, K. L. (2020). Chasing a complete understanding of the failure mechanisms and potential hazards of the slow moving liangshuijing landslide. Eng. Geol. 281, 105977. doi:10.1016/j.enggeo.2020.105977
Sun, G., Zheng, H., Huang, Y., and Li, C. (2016). Parameter inversion and deformation mechanism of Sanmendong landslide in the Three Gorges Reservoir region under the combined effect of reservoir water level fluctuation and rainfall. Eng. Geol. 205, 133–145. doi:10.1016/j.enggeo.2015.10.014
Xiao, S. R., Lu, S. S., Guan, H. F., and Song, G. L. (2013). Study of geomechanical model of liangshuijing landslide in three gorges reservoir area. Rock Soil Mech. 34, 3534–3542. In Chinese.
Yan, G. Q., Yin, Y. P., Huang, B. L., Zhang, Z. H., and Zhu, S. N. (2019). Formation mechanism and characteristics of the jinjiling landslide in wushan in the three gorges reservoir region, China. Landslides 16 (11), 2087–2101. doi:10.1007/s10346-019-01234-3
Yin, Y. P., Huang, B. L., Wang, W. P., Wei, Y. J., Ma, X. H., Ma, F., et al. (2016). Reservoir-induced landslides and risk control in three gorges project on Yangtze River, China. J. Rock Mech. Geotechnical Eng. 8, 577–595. doi:10.1016/j.jrmge.2016.08.001
Yin, Y. P., Zheng, W. M., Liu, Y. P., Zhang, J. L., and Li, X. C. (2010). Integration of GPS with InSAR to monitoring of the Jiaju landslide in sichuan, China. Landslides 7 (3), 359–365. doi:10.1007/s10346-010-0225-9
Zhang, C. Y., Yin, Y. P., Dai, Z. W., Huang, B. L., Zhang, Z. H., Jiang, X. N., et al. (2020). Reactivation mechanism of a large-scale ancient landslide. Landslides 18 (1), 397–407. doi:10.1007/s10346-020-01538-9
Keywords: old landslide, engineering disturbances, rainfall, reactivation mechanism, deformation characteristics
Citation: Dai Z, Yang L, Zhang N, Zhang C, Zhang Z and Wang H (2023) Deformation characteristics and reactivation mechanism of an old landslide induced by combined action of excavation and heavy rainfall. Front. Earth Sci. 10:1009855. doi: 10.3389/feart.2022.1009855
Received: 02 August 2022; Accepted: 03 November 2022;
Published: 17 January 2023.
Edited by:
Wei Zhao, Institute of Mountain Hazards and Environment (CAS), ChinaReviewed by:
Qiang Zou, Institute of Mountain Hazards and Environment (CAS), ChinaWang Lei, Anhui University of Science and Technology, China
Copyright © 2023 Dai, Yang, Zhang, Zhang, Zhang and Wang. This is an open-access article distributed under the terms of the Creative Commons Attribution License (CC BY). The use, distribution or reproduction in other forums is permitted, provided the original author(s) and the copyright owner(s) are credited and that the original publication in this journal is cited, in accordance with accepted academic practice. No use, distribution or reproduction is permitted which does not comply with these terms.
*Correspondence: Long Yang, eWFuZ2xvbmcxOTM4QGN1Zy5lZHUuY24=