- 1Key Laboratory of Comprehensive and Highly Efficient Utilization of Salt Lake Resources, Qinghai Institute of Salt Lakes, Chinese Academy of Science, Xining, China
- 2Qinghai Provincial Key Laboratory of Geology and Environment of Salt Lakes, Xining, China
- 3University of Chinese Academy of Sciences, Beijing, China
- 4Department of Earth Sciences, Karakoram International University, Gilgit-Baltistan, Pakistan
- 5State Key Laboratory of Geological Processes and Mineral Resources, China University of Geosciences, Wuhan, China
- 6Institute of Geology, University of Azad Jammu and Kashmir, Muzaffarabad, Pakistan
- 7State Key Laboratory of Biogeology and Environmental Geology, China University of Geosciences, Wuhan, China
- 8GEOTOP/Earth and Atmosphere Sciences Department, UQAM, Montréal, QC, Canada
The Kohat Basin (KB) lies on the Himalayan Foothills and is of scientific importance as it directly recorded the closure of the Tethys Sea and the Himalayan collision between India, Asia, and a number of other small plates. During the Eocene, after the collision between the Indian and Eurasian plates terminated the Tethys Sea, thick-bedded marine evaporite sequences developed in the KB. In this study, we combined mineralogy, geochemistry, fluid inclusion and chlorine stable isotope compositions to discuss the origin and evolution of the KB Eocene halite deposits with the ultimate objective of defining the paleoclimate that was prevailing in Asia during the Eocene. Our results showed that halite samples were SO42− rich (225–370.103 ppm) and Br− poor (<3 ppm). Cl−, B+, Mg2+, K+, SO42− and very low Br concentrations as well as the (Br/Cl) ratios indicated that halite resulted of a mixture of solutions with variable compositions and that dissolution, recrystallization and a progressive decrease in dolomitization were the mechanisms leading to the formation of these evaporites. A Br/Cl vs Cl plot revealed that the end members involved were: seawater (sw), saline waters and/or freshwaters. The recrystallization process prevented identifying the primary structures and primary fluid-inclusions. Most of Cl isotope compositions (−0.54‰<δ37Cl < 0.83‰) were within the usual range (0 ± 0.5‰) associated to seawater as the initial source for the halite. The higher isotope compositions (δ37Cl ≥ 0.83‰) comforted the hypothesis of the genesis by mixing of solutions of different origins as well as the involvement of recrystallization. Based on our results, we are proposing the following to explain the regional paleoclimate sequence: 1) shallow water conditions; 2) halite precipitation induced by evaporation, 3) unstable paleoclimatic conditions that resulted in the morphing from an evaporite basin into a terrestrial foreland basin. All these events were controlled by regional tectonic and linked to both the overall uplift times of the NW Indian Craton and the Eocene thermal maximum one during the Eocene-Oligocene period.
Introduction
The collision tectonic events leading to the final closure of the Tethys Sea during the Cenozoic formed mountain chains that prolong from the Mediterranean region to Asia (Potter and Szatmari, 2009). These events were recorded by the Paleocene–Pleistocene sedimentary rocks present in India, Nepal and Pakistan (Najman et al., 2017). These events most probably debuted after the Indian plate broke away from Gondwanaland, began moving north towards Eurasia, and ended with the subduction of the Tethys Sea (Khan et al., 1987; Ahmad et al., 2002). The continent-continent collision between the Indo-Pakistan and the Eurasian plates not only led to the Himalayan orogeny but also provided enough marine sediments and source material for the development of thick-bedded evaporites in Pakistan (Pivnik and Wells, 1996). The major rock salts deposits in Pakistan are located in the Salt Range area and in the Kohat basin (KB) (e.g. Hussain et al., 2019; Hussain et al., 2020a; Hussain et al., 2020b; Hussain et al., 2021). The Precambrian Salt Range is considered as the main and oldest salt deposits in the sub-continent. The younger salt deposits (Eocene age) are found in the northwestern KB with the main deposits located in the central part of the basin. Deposits contain halite along with variable amounts of gypsum dolomite and clays, and limited layers of potash and magnesium salts. In comparison, the KB salts are thin compared to the Salt Range Formation and can be observed at different locations (Jatta, Karak and Bahadurkhel) of the KB (Figure 1). Just like the SR Fm. the base of the KB is not exposed but its thickness is variable, with a maximum of 700 m (Asrarullah, 1963; Pivnik and Wells., 1996). Records of marine sedimentation ranging from the Paleocene to younger ages can be observed at the Kohat Plateau (KP; NW Pakistan), also known as the Kohat Fold and Thrust Belt (KFTB) (Hussain and Zhang, 2018). These sedimentary rocks are mainly constituted of complex groups of sulfate, evaporite, shale/sandstone, and conglomerates (Meissner et al., 1975). The KP lies within 100 km of the western Himalayan Foothills (Pivnik and Wells, 1996) and is assumed to be the main Himalayan depocenter at the time of the collision between the Indian and Asian plates (Gardezi, 2016). The KP is restricted by the Main Boundary Thrust (MBT) on its northern part and by the Trans-Indus Salt Ranges and the Bannu Plain on its southern border. The Indus River and the Kurram Fault mark (Figure 1) its eastern and western boundaries (Kazmi and Rana, 1982).
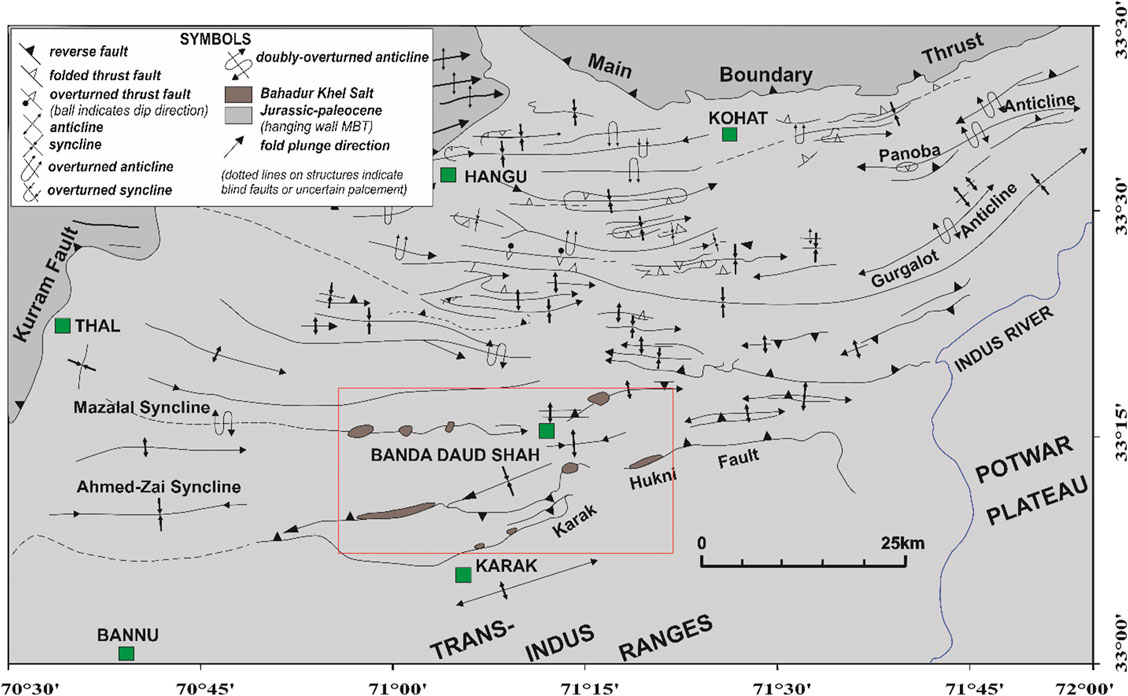
FIGURE 1. Structural map of the Kohat Plateau (modified after Pivnik and Wells, 1996). The map indicates the major structural boundaries as well as the locations of the thick-bedded salt rock deposits.
The KP encompasses Cenozoic sedimentary rocks and previous studies have shown that during the Paleocene (early Eocene) the formation of thick-bedded evaporites in the region was facilitated by the submergence of the NW Indian continental shelf that separated from both the Tethys Sea and the Persian Gulf (e.g. Pivnik and Wells, 1996). Thick-bedded evaporites and particularly brines are common geological features worldwide (Warren, 2006; Guo et al., 2017) that have been vastly investigated (e.g. Gee, 1938; Krishnan, 1966; Hsü et al., 1973; Hsü and Bernoulli, 1978; Alsop, 1996; Hardie and Lowenstein, 2004). Studies have particularly focused on evaporites strictly related to source rocks in sedimentary environments (e.g. Hussain and Warren, 1991; Warren, 2010; Hussain et al., 2021). However, due the lack of modern equivalents, the origin, evolution and the depositional background of these evaporites is still subject to debate (e.g. Guo et al., 2017; Hussain et al., 2020a). Classical chemistry and isotope systematics have already demonstrated their added value to better constrain the sources and processes leading to the formation of these evaporites (Han et al., 2019; Hussain et al., 2020a; Hussain et al., 2021). For example, the Br/Cl ratio and Na-Cl-Br concentrations measured in evaporites can be used to characterize the formation and evolution, including recrystallizsation, dissolution and redeposition processes, of basin fluids (e.g. Walter et al., 1990). The Cl/Br ratio is a common proxy used to study changes during the late phases of halite deposition (e.g. Eggenkamp et al., 2019a; Hussain et al., 2021), the origin of saline waters (e.g. Alcala and Custodio, 2004; Alcala and Custodio, 2008; Katz et al., 2011) as well as dissolved mineral components in deep fluids (Heinrich et al., 1993; Sonney et al., 2010) and paleoenvironment of evaporites (Ercan et al., 2019). On the other hand, the use of Cl stable isotope compositions (noted δ37Cl) has proved to be a reliable tracer for ancient evaporites (Eggenkamp et al., 2018; Eggenkamp et al., 2019a; Eggenkamp et al., 2019b), to identify the origin of modern brines (Eggenkamp, 2014; Luo et al., 2014), and to characterize the geochemical development of connate waters in sedimentary basins (Tomascak et al., 2016; Hanlon et al., 2017). δ37Cl is good at fingerprinting the origin of mixed magmatic–basinal brines and it can differentiate between marine and non-marine environment and depositional conditions (Chiaradia et al., 2006). Recently it has also been proved that δ37Cl can be used to evaluate the evaporation rate at the time of the salt formation (Luo et al., 2014, Luo et al., 2016; Hussain et al., 2019).
The rock salt deposits in Pakistan were early characterized (e.g. Wynne 1875, Wynne 1878, Wynne 1880). Gee (1945) created two inches to one-mile geological maps. Asrarullah, 1962; Asrarullah, 1963 and Alam and Asrarullah (1973) estimated the volumes of Pakistan rock salt resources. While most of this research was focusing on understanding the stratigraphy and geology of the KB (Eames, 1952; Meissner et al., 1974; Meissner et al., 1975; Shah, 1977; Wells, 1984; Pivnik and Sercombe 1993a; Pivnik and Wells 1996), the vast deposits of halite, sulfate and other salts present in the Eocene Kohat Fold and Thrust Belt and their corresponding sources and evolution have never been investigated using chemistry and isotope systematics due to a complex assemblage of these evaporites. The objectives of this study were: 1) to geochemically characterize the Kohat salt (also known as the Bahadur Kel salt) deposits 2) to define the origin and evolution of the ancient halites and ultimately to discuss the paleoclimate prevailing during their deposition. To fulfill these objectives, we decided to couple the classical geochemical, mineralogical and lithological approach with the use of the (Br/Cl) ratios and δ37Cl isotope compositions. To our knowledge, this represents the first study using Cl isotopes to better understand the regional geology, and lays a basis for future isotope investigations of the KFTB.
Geology and Stratigraphy
The Great Himalaya emerged because of a N-S crustal shortening and thickening, resulting from the junction between the Indian and Eurasian Plates and their later impaction (Gansser, 1964; Le Fort, 1975). In the Early Miocene, these tectonic movements formed the Tibetan Plateau (TP) as well as thrust structures such as the KP, which are parts of the Indo-Gangetic foreland (Ahmad et al., 2006), comprising Tertiary sedimentary rocks. Previous studies (Gee 1938; Meissner et al., 1974; Meissner et al., 1975; Shah, 1977; Wells, 1984; Pivnik and Sercombe 1993b; Pivnik and Wells 1996) indicated that the surface rocks are mainly from the Eocene and present an unconformable relationship to the overlying Miocene or younger rocks (Gee, 1945; Fatmi, 1973; Shah, 2009). The strata deposited under restricted marine conditions and represents the first record of the Himalayan collision (Pivnik and Wells 1996). The KFTB was cut off from the Tethys Sea and developed a complex assemblage of evaporites, carbonates and clastic rocks generated by tectonic forces during the later stages of the Himalayan orogeny.
Structurally, the KP represents both compressional and transpression tectonics (Pivnik and Sercombe, 1993b); many features are linked to imbricated thrusts; and associated folds are linked to the oblique convergence and subsequent formation of an hybrid terrain (Paracha, 2004). Seismic data from the Northern Potowar Deformation Zone (NPDZ) and KP revealed that both regions shares parallel structure histories (Pivnik and Sercombe, 1993a). Pop-ups, wide synclines, narrow faults, and evaporite anticlines are the most common structural features of the KFBT. They indicate a high-level translation of a large thrust mass along the Eocene evaporites (Hussain and Zhang, 2018) resulting from the northward clockwise tectonic movement of the Indian plate (Gardezi, 2016).
Figure 2 reports the detailed stratigraphy of the KFBT studied and shows that the Paleozoic and Mesozoic rocks deposited on the north Indian passive continental margin, which facilitated a southern shoreline to the Tethys sea (Pivnik and Wells 1996). The oldest exposed rocks are of Eocene age and consist of evaporites (halite and gypsum) and shales. Drill hole data from hydrocarbon exploration suggest that early Jurassic rocks are the unexposed oldest rocks (Gardezi, 2016). In this study, we are focusing on the Eocene halite deposit that was developed between the NW Indian continental margin, the southern Asian margin, and at least one or more microplates that now constitute western Pakistan and eastern Afghanistan.
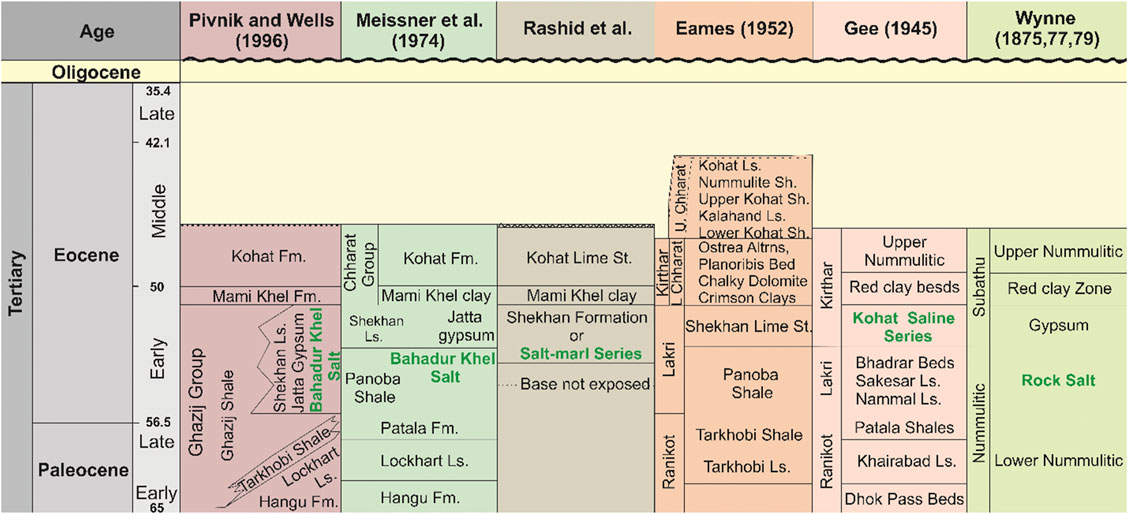
FIGURE 2. Stratigraphic column of the Kohat Plateau (KP), showing major formations and corresponding ages.
The Eocene halite in the KP is referred as the Kohat Saline Series (Gee, 1945) or the Bahadur Khel Salt (Meissner et al., 1974). It is exposed along anticlines with a general EW-trend and crops out in the central part of the KP near the Manzalai anticline and along the Karak-Hukni fault. The Jatta Gypsum confirmly lays on top of the Bahadur Khel Salt (BKS) but correlating its age with other units (e.g. the Ghazij Group) is rendered difficult due to the lack of local age control (Pivnik and Wells, 1996). The real thickness of the BKS is unknown as its base is not exposed. However, while Meissner et al. (1974) reported an exposed thickness of about 100 m, a hole drilled in the core of an anticline (in the Shakarkara village) yielded a thickness of about 700 m (Meissner et al., 1974). Regional geology and stratigraphy indicate that at the time of deposition the brine was most likely warm and deposited on sabkhas and in lagoons in its northeastern part and in a shallow water environment in its southern part (Pivnik and Wells, 1996). The Eocene regression forged the final small and limited basins.
Materials and Methods
Sampling and Elemental Concentrations
In total, we collected 24 representative halite samples in 2018 from three distinct deposits (details are given in Table 1): nine samples from the Jatta deposit, seven from the Karrak one and eight from the Bahadur Khel one. For each halite sample, we measured the K+, Na+, Ca2+, B3+, Mg2+, Li3+, SO42−, Br−, Cl− concentrations and corresponding Cl stable isotope compositions (noted δ37Cl). K+, Ca2+, Mg2+, and B3+ concentrations were measured by ICP-OES (ICAP6500DUO, United States) with a precision better than 5%. Br− and SO42− were analyzed by Ion Chromatography (IC-5000+, Thermo Fisher, United States) and Cl− was measured by chemical mercurimetry, with an accuracy higher than 0.3% (ISL, CAS, 1988). δ37Cl was analyzed by Positive Thermal Ionization Mass Spectrometry (TIMS-TRITON, United States). All measurements were made at the Salt Lakes Analytical and Testing Department, Qinghai Institute of Salt Lakes, Chinese Academy of Sciences.
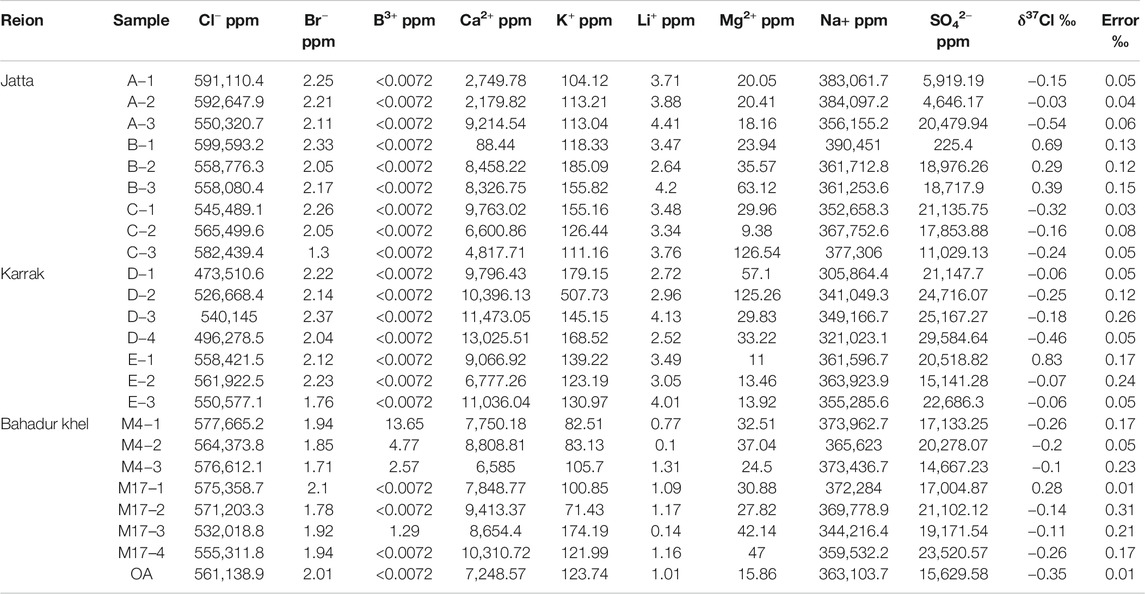
TABLE 1. δ37Cl and ion compositions of the salt deposits from the Kohat basin: Jatta, Karrak, and Bahadur Khel deposits.
Isotope Sample Preparation and Analysis
Each halite sample was first dissolved in highly deionized water and then purified using the following two-step protocol (samples were purified to ultimately contain 5 g/L of Cl): 1) dissolved samples first passed through a polyethylene ion-exchange column (∼0.5 cm diameter) filled with ∼2 cm of Dowex 50WX8 H-cation exchange resin, and subsequently through 2) a second polyethylene ion-exchange column (∼0.5 cm diameter) filled with ∼1.6 cm of Cs-cation exchange resin. The pH of the solution was kept <7 throughout the procedure. Finally, the eluant was collected for further analysis by TIMS. As the halite samples presented low SO42−/Cl− and NO3−/Cl− ratios, interferences by SO42− and NO3− were considered negligible and therefore no additional purification step was needed (for details see Hussain et al., 2020b). A tantalum (Ta) filament was heated under vacuum for 1 h using a current of 2–3A, before being covered with 2.5 µl of a graphite slurry that contained 80% of ethanol and 80 µg of graphite. About 2 µl of the sample solution, having no less than 10 µg of Cl as CsCl, was deposited onto the filament. The filament was then dried using a current of 1 A for ∼2.5 min and inserted into the TIMS (pressure at ∼2.5 × 10–7 mbars). The Cs2Cl+ ion current generated was maintained at 4 × 10–12 A by adjusting the source current. Data were instantaneously collected on Faraday cup “C” and “H1” by using the ion flows from mass numbers 301 (133Cs235Cl+) and 303 (133Cs237Cl+). The average 37Cl/35Cl ratio we obtained for the IAEA ISL-354 NaCl standard was 0.319031 ± 0.000066 (n = 12), in agreement with the value of 0.31964 ± 0.00092 reported by Xiao et al., 1997. The corresponding δ37Cl value was calculated as (error ≤ 0.09‰):
Results and Discussion
Halite Mineralogical Characteristics
The δ37Cl and elemental compositions of the halite samples are given in Table 1. Results show that Na+ and Cl− are the major ions, followed by SO42− and Ca2+. Trace elements, such as B3+ and Br−, are usually negligible. δ37Cl in our halite samples range from −0.54‰ −0.83‰ with an average isotope composition of −0.06 ± 0.33‰.
The main salt deposits in the KB can be observed at three localities: Jatta, Karak and Bahadur Khel (Figure 1). All deposits in the KB are stratigraphically considered as the Bahadur Khel Salt (Meissner et al., 1974; Pivnik and Wells, 1996). The general trend follows a NS direction and extends for up to 40 km. The KB salts are white to grayish in color, the later resulting of the intermixing of black bitumen/shale with rock salt, which in turn affects its purity. The halite thickness in the KB varies from one to hundreds of meters (Meissner et al., 1974). The exposed mineralization is massive and translucent for most of the deposits. Few thin layers are transparent and crystals vary between euhedral and anhedral. Primary textures were absent or difficult to detect and only secondary textures with secondary fluid inclusions were observed under microscopy (Figure 3).
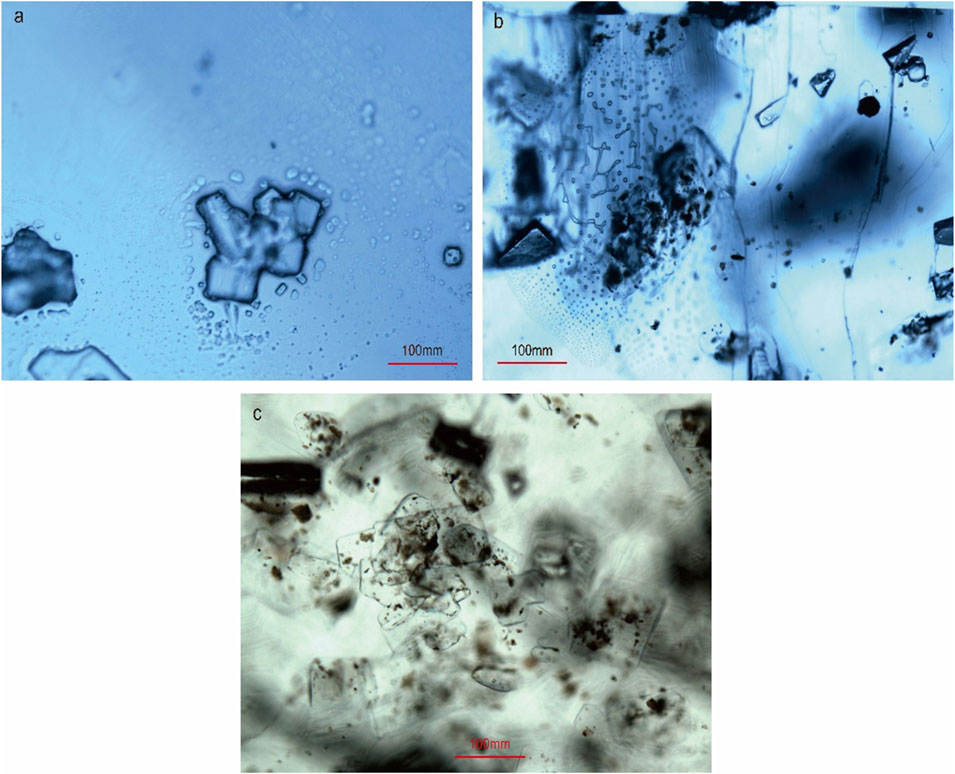
FIGURE 3. Microscopy pictures of the halite samples: (A) random crystal of halite covered by secondary fluids, (B) washout structures, and (C) halite random crystals covered by (black) bituminous materials.
Primary structures in halite, e.g. chevron and hopper structures, are generally interpreted as reflecting shallow lake brines (Salvany et al., 2007) deposited under stable environmental conditions (Warren 2006; Ercan et al., 2019). Although previous studies concluded that the KB brines were warm, shallow and developed within a passive continental margin (e.g. Pivnik and Wells, 1996; Pivnik and Wells, 1996), finding corresponding primary structures was not possible due to the recycling of the ancient halite. On the other hand, the recrystallization and dissolution processes during flooding (Shearman, 1970) and the alteration of the mineral morphologies by burial effects or by active capillary activities around older mineralization develop common secondary features (Ercan et al., 2019). Thus, this indicates that the KB halite either developed or evolved under variable conditions.
Halite Geochemistry
For all halite samples Mg2+, Ca2+ and SO42− ions yielded large variations in their concentrations (Table 1). Li+ Br−, Ca2+, K+, and B+ concentrations varied among the different sites, and even within each sampling site (Table 1): the K+ and Ca2+ contents in the Jatta and Bahadur Khel samples ranged from 104 to 185 ppm, from 88 to 9,763 ppm, from 71 to 174 ppm and from 6,585 to 10,310 ppm, respectively. The Karrak samples gave the highest Ca2+ (6,777–13,025 ppm) and K+ (123–507 ppm) concentrations. Mg2+ concentrations ranged between 15 and 47 ppm for the Bahadur Khel site, between 11 and 125 ppm for the Jatta site and from 9 to 126 ppm for the Jatta site. Li+ and Br− contents varied between 0.09 and 4.4 ppm and between 1.2 and 2.3 ppm, respectively. B+ concentrations were very low (≤0.007 ppm) in most of the halite samples. SO42− content were high in most samples with values between 225 and 29,584 ppm. The highest SO42− concentrations were observed at the Karrak site.
During the late Eocene (37–34 Ma), seawater was Mg2+ and SO42− rich (Holland, 2005), due to a progressive decrease in dolomitization. Between 93.5 and 36 Ma the SO42− concentration was higher than that of Ca2+ (Timofeeff et al., 2006). Figure 4 shows that for the KB SO42− > Ca2+, which indicates that the parent waters were SO42− rich. While the K+ concentration of Tertiary marine halite was constant at about 400 ppm (Holland, 1984), in our samples, it varied between 84 and 9,413 ppm, with an average value around 100 ppm (Figure 4A). As the Mami Khel clays overlay the Kohat salts these lower K+ concentrations may thus be explained by the absorption of K+ onto clay minerals. The Mg2+ content of the Eocene SW was lower (874 ppm) compared to the 1,336 ppm in present day SW (Brennan et al., 2013). In the KB halite the Mg content varied between 1 and 126 ppm (Figure 4B). The low Mg2+ are probably caused by permeable zones. The study area is highly faulted, with the Kurram Thrust Fault covering the western and northern parts of the area and overlaying the Mami Khel clays. It may form impermissible zones that prevent any water input. Brennan et al. (2013) reported that the Ca2+ content in present-day SW (264 ppm) is lower than during the Eocene (384 ppm), giving a negative relationship between Ca2+ and SO42− (Figure 4C). In our study, the relationship between these two ions was linear with a positive slope (Figure 4C) that indicated that the controlling process is a global decrease in the dolomitization process (Holland and Zimmermann, 2000; Holland, 2005).
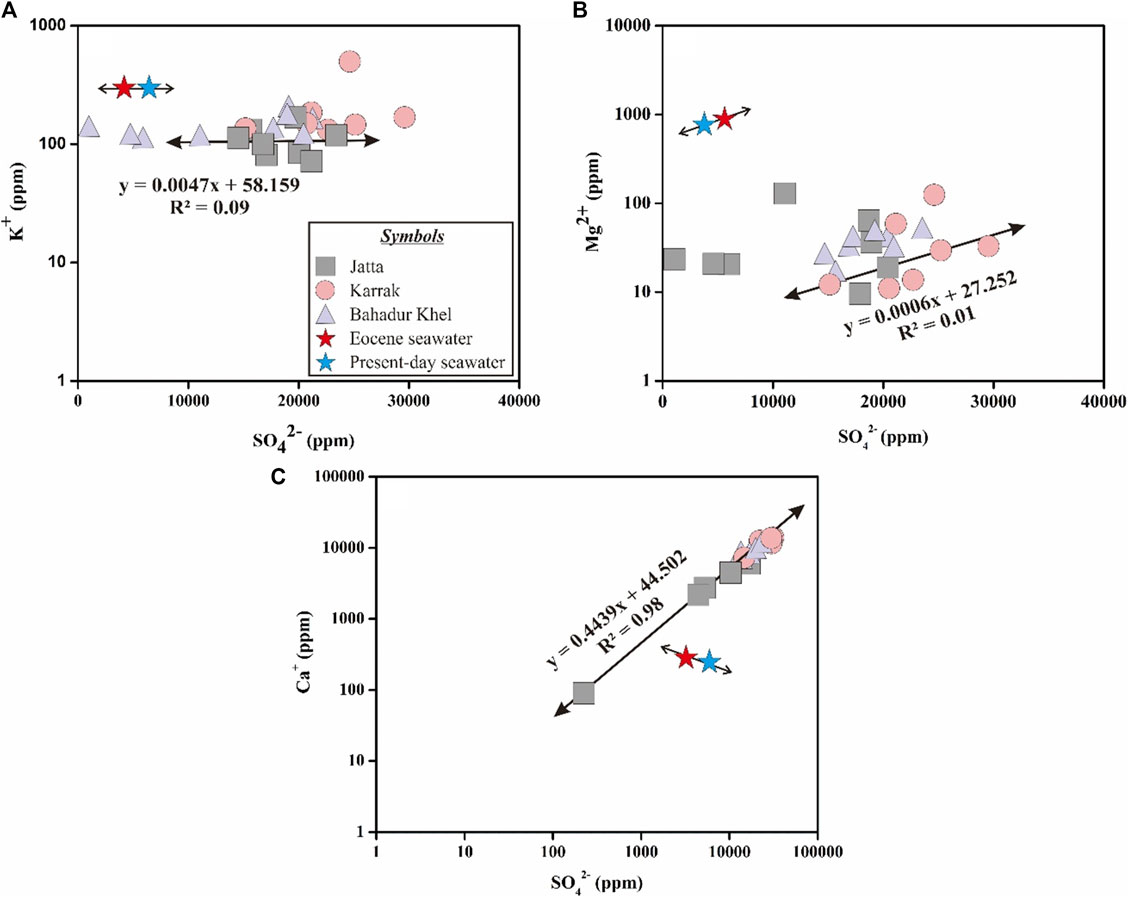
FIGURE 4. Ion relationships observed in the Bahadur Khel salts: (A) SO42−vs K+, (B) SO42−vs Mg2+ and (C) SO42−vs Ca2+. For each diagram the corresponding characteristics of the Eocene (data from Brennan et al., 2013) and present-day seawaters are given (data from Holland, 1984).
The Br− concentration in the first halite precipitated from SW is ∼75 ppm (Holser, 1979) and its value reaches up to 270 ppm in the advanced concentration stages, at the beginning of the Mg sulfate deposition (Kovalevych et al., 2006). The calculated Br− residence time is ∼100 million years (Holland, 1984). Therefore, the Br− concentration in halite precipitated from the evaporation of Tertiary seawater must be about 75–270 ppm (Ercan et al., 2019) but later changes (e.g. recrystallization, dissolution) can affect these concentrations (Eggenkamp et al., 2019a). For example, the Miocene halite of the Mediterranean region yields Br− concentrations up to 40 ppm (Bloch, 1953) but also as low as ∼5 ppm (e.g. Ercan et al., 2019). Brennan et al. (2013) reported Br− concentrations of 11 ppm for halites from the Catalan sub-basin, whereas Moretto (1986) reported Br− concentrations of 10 ppm for the Bresse Basin. The Eocene salts from Bhadur Khel have lower Br− concentrations compared to the other Paleogene and Neogene basins (1.3–2.37 ppm) that indicate an entry of non-marine water (Shouakar-Stash, 2008) or the dissolution or recycling of ancient marine halite (Brennan et al., 2013; Han et al., 2019; Eggenkamp et al., 2019b). The study of the Cl/Br ratio has proved to be one of the most effective and sensitive indexes when defining the geological environment, brine concentrations and the depositional stages associated to the brine evaporation process (e.g. Han et al., 2019; Hussain et al., 2019). Experiments have demonstrated that this Cl/Br ratio is related to the brine and salt evolution (Braitsch, 1962): the more advanced brine solutions produce lower Cl/Br ratios in the precipitates (Eggenkamp et al., 2019a; Eggenkamp et al., 2019b). The Cl/Br ratio in SW (∼300) is lower than at any halite stage as evaporites preferentially incorporate Cl− compared to Br− (Hussain et al., 2020b). Figure 5 shows that our halite samples Br− concentrations were low, yielding high Cl/Br ratios. This indicates that they have been dissolved by saline waters/non-marine waters as evaporites forming in basins having no or limited connections with the open sea are expected to episodically get waters and solutes from non-marine sources (Holland et al., 1984). Geology demonstrates that halite in the KB has a marine origin (Wells, 1984; Pivnik and Wells, 1996), resulting from the precipitation of the ancient Tethys Sea (Pivnik and Wells, 1996; Hussain and Zhang, 2018). Thus, the low Br− concentrations can only be explained by multiple inputs of non-marine water.
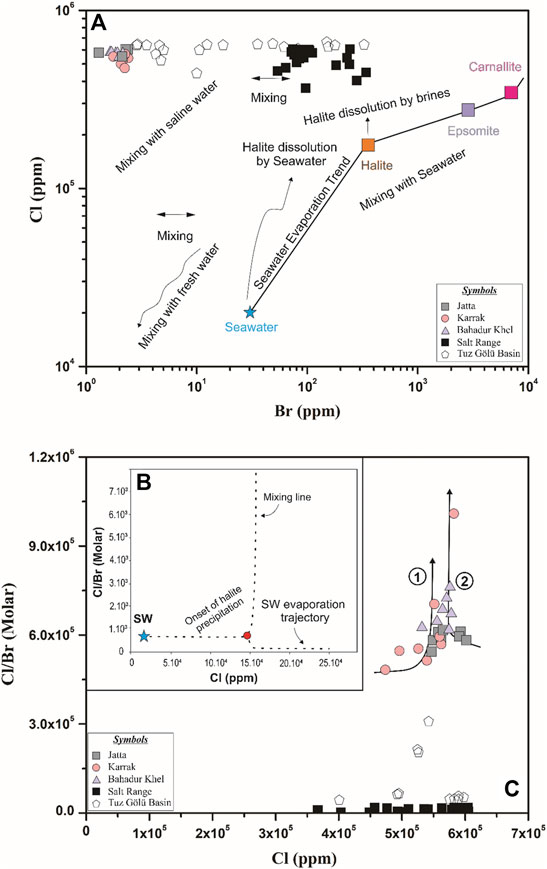
FIGURE 5. Simplified models explaining the Cl and Br behaviors in halite from the Kohat Basin. (A) Cl vs Br ratios. Data are compared to those of the Salt Range (Pakistan) and Tuz Golu (Turkey) Basins. Precipitation data of variable evaporite minerals are from Matray, 1988, the seawater evaporation line is from Shouakar-Stash (2008) and Ercan et al. (2019). (B) Mixing model adapted from Grandia et al. (2003) and Ercan et al. (2019) where the dashed line represents the addition of highly evaporated brine that underwent dilution by a factor of seven to a fluid-dissolving halite. (C) Cl/Br ratio vs. Cl. Trends one and two represent variable evaporation trends.
The Cl/Br vs Cl diagram (Figure 5) shows that in our study area brines of different origins were mixed. The halite samples from our three locations plot along two distinct trends: 1) Most of the samples from the Karrak mine plot along trend 1 in Figure 5 and indicated that they precipitated from two mixed Cl-rich salt waters that have dissimilar compositions, percentages or origins (e.g., SW, saline water or meteoric water). 2) Samples from Bahadur Khel and Jatta lay along trend 2 and agreed with the recrystallization of ancient halite (i.e. WRI; water-rock interaction). We also compared our values with those of two other salt basins (Figure 5): the Salt Range (Pakistan; Hussain et al., 2020a) and the Tuz Golu (Turkey; Ercan et al., 2019) basins. While the KB showed a trend similar (but with lower Br concentrations) to the Tuz Golu basin, the nearest Salt Range yielded significantly lower and homogenous Cl/Br ratios that may be explained by active tectonic and multiple halite dissolutions.
Cl Isotopes in Halite: Implications for Paleoclimate
δ37Cl isotope compositions have been extensively studied in evaporites in order to explain the Cl stable isotope fractionations induced by the evaporation process (e.g. Vengosh et al., 1989; Xiao et al., 1997; Eggenkamp et al., 1995; Eastoe et al., 1999; Ying-Kai et al., 2000; Eastoe et al., 2001; Eastoe et al., 2007; Luo et al., 2012; Luo et al., 2014; Eastoe, 2016; Luo et al., 2016; Eggenkamp et al., 2019a; Eggenkamp et al., 2019b). Salt precipitation from marine brines follows the classic Rayleigh distillation law (Eggenkamp et al., 1995), inducing a decrease in the δ37Cl coupled to an increase in the corresponding Br/Cl ratio (Eggenkamp et al., 2019a). Figure 6 shows that the Bahadurkhel and Karrak samples are mostly characterized by rather constant δ37Cl ∼−0.2‰ but varying Br/Cl ratios, whereas the Jatta samples display somewhat positive δ37Cl values with considerably constant Br/Cl ratios. This may be explained by frequent and multiple dissolution and precipitation processes as Br contents decline with successive recycling processes (Eggenkamp et al., 2019b; Han et al., 2019). It also indicated that the basin was disconnected from the main water reservoir (i.e. sea), allowing for multiple dissolution/precipitation and mixing to occur. Ultimately, this resulted in the halite showing various origins, in agreement with the findings of Holland, 1984.
Previous studies have shown that halite is generally enriched in the heavier 37Cl isotope and that its final δ37Cl is controlled by the corresponding deposition conditions (e.g. Luo et al., 2016). The study of Cl stable isotope fractionations during salt precipitation (e.g. Eggenkamp et al., 1995; Luo et al., 2012; Luo et al., 2014; Eggenkamp et al., 2015; Luo et al., 2016; Eggenkamp et al., 2016) showed that the most negative δ37Cl appear when bischofite (MgCl2•6H2O) starts precipitating (Eggenkamp et al., 2018). Luo et al. (2014) also showed that δ37Cl keep decreasing continuously with the precipitation of K-Mg salts. Thus, Cl isotopes are good proxies for characterizing the evaporation process: the continuous impoverishment in 37Cl can be linked to the evaporation cycles (Luo et al., 2014; Eggenkamp et al., 2019a). Cl− in the Kohat Basin results of the ancient marine water (Pivnik and Wells, 1996; Hussain and Zhang, 2018) that may be mixed with non-marine or meteoric waters, as demonstrated by the δ37Cl values of its halite centered around 0‰ (Eastoe et al., 2001; Eastoe et al., 2007). The δ37Cl for the KB Eocene marine halite ranged from −0.54‰ to 0.83‰, and most of these isotope compositions are within 0 ± 0.5‰, indicating that variable climatic conditions prevailed during deposition as well as the alternation of wet and dry periods climate. Under wetter conditions, dissolution of the already deposited halite is the main source of Cl. This dissolution is accompanied by higher δ37Cl (Luo et al., 2016) compared to lake water. Under dry spell conditions, a limited quantity of Cl enters the basin and, coupled to the evaporation process, this continuously decreases δ37Cl in the salt lakes. Consequently, lake waters during flooding have higher δ37Cl compared to dry conditions. These higher δ37Cl indicate thus both the state of the halite precipitation from the saline lake but also reveal a larger volume of lake water. For the KB, the highest δ37Cl was 0.83‰, suggesting a sudden high-depth lake and thus important amounts of water entering the basin. On the other hand, as most of the δ37Cl values were negative (Figure 6; lowest δ37Cl = −0.54‰), this suggests that long dry and hot climatic conditions prevailed during the deposition of the halite.
Origin of the Eocene Halite in the Kohat Basin
Due to the events associated to the “Eocene thermal maximum 1(ETM1)" (also known as the “Paleocene–Eocene Thermal Maximum; PTEM”), Eocene was an evolving period. The PTEM was characterized by an extreme increase (5–8°C) of the global average temperature (Mcinerney and Wing, 2011). The ETM1 occurred about 56 Ma ago (Bowen et al., 2015) and this hot period remained for at least 200,000 years (Mcinerney and Wing, 2011). The ETM1 effect was recorded worldwide and the mid-latitude areas saw average temperatures of ∼6°C (Hansen et al., 2013). Variations in the δ37Cl and ion compositions of the Kohat Salts may have thus been controlled by the global sea level fluctuations caused by these climate variations during ETM1. The effects of the PTEM are more observable in the marine sedimentary section across the Paleocene/Eocene (P/E) margin in the adjacent Indus basin (Hanif et al., 2014). In this basin, the onset of the carbon and oxygen stable isotope excursion has proved that it’s associated with the PETM (Hanif, 2011). Besides isotopic evidences, paleontological ones also support that the PTEM impacted the area neighboring the Salt Range (Afzal et al., 2011; Hanif et al., 2020) and indicate that the environment fluctuated (bathyal - shallow carbonate platform; Hanif et al., 2020). Still, to our knowledge, the studies about the PTEM effects in this region are still scarce, therefore arising the need for further investigations based on petrographic (e.g. halite homogenized temperature) and isotope approaches. Figure 7 presents a simplified model detailing the successive events that led to the deposition of the KB Eocene halite. At the end of the Early Eocene, an extensive but short-time regression period occurred in the KB, evidenced by the presence of the Jatta gypsum and of the association of the gypsiferous shale with halite (Qadri, 1995). During the Middle Eocene, a widespread transgression formed marshes covering the whole KB. This is evidenced by the Mami Khel Formation that overlays the Chorgali Formation, that was deposited in a coastal or tidal saline/brackish water environment during a transgression event (Paracha, 2004). It comprises red to brownish fluvial and/or alluvial claystone and shale intervals which demonstrate the existence of a highly dry environment for a long period in the KB. The deposition of the Kohat Formation indicated transitional marine conditions about 50 Ma ago (Paracha, 2004). This ultimately established a foredeep basin between the Indian and Asian Plates. Later on, it morphed into a confined evaporite basin, followed by an early terrestrial foreland basin and an open marine basin. And lastly, during the Pliocene-Pleistocene times, it developed into an entirely terrestrial foreland basin (Pivnik and Khan, 1996; Paracha 2004). All these events were controlled by regional tectonic, i.e. the overall rise of the NW Indian Craton between the Eocene and Oligocene periods that formed an evaporite basin (Figure 7).
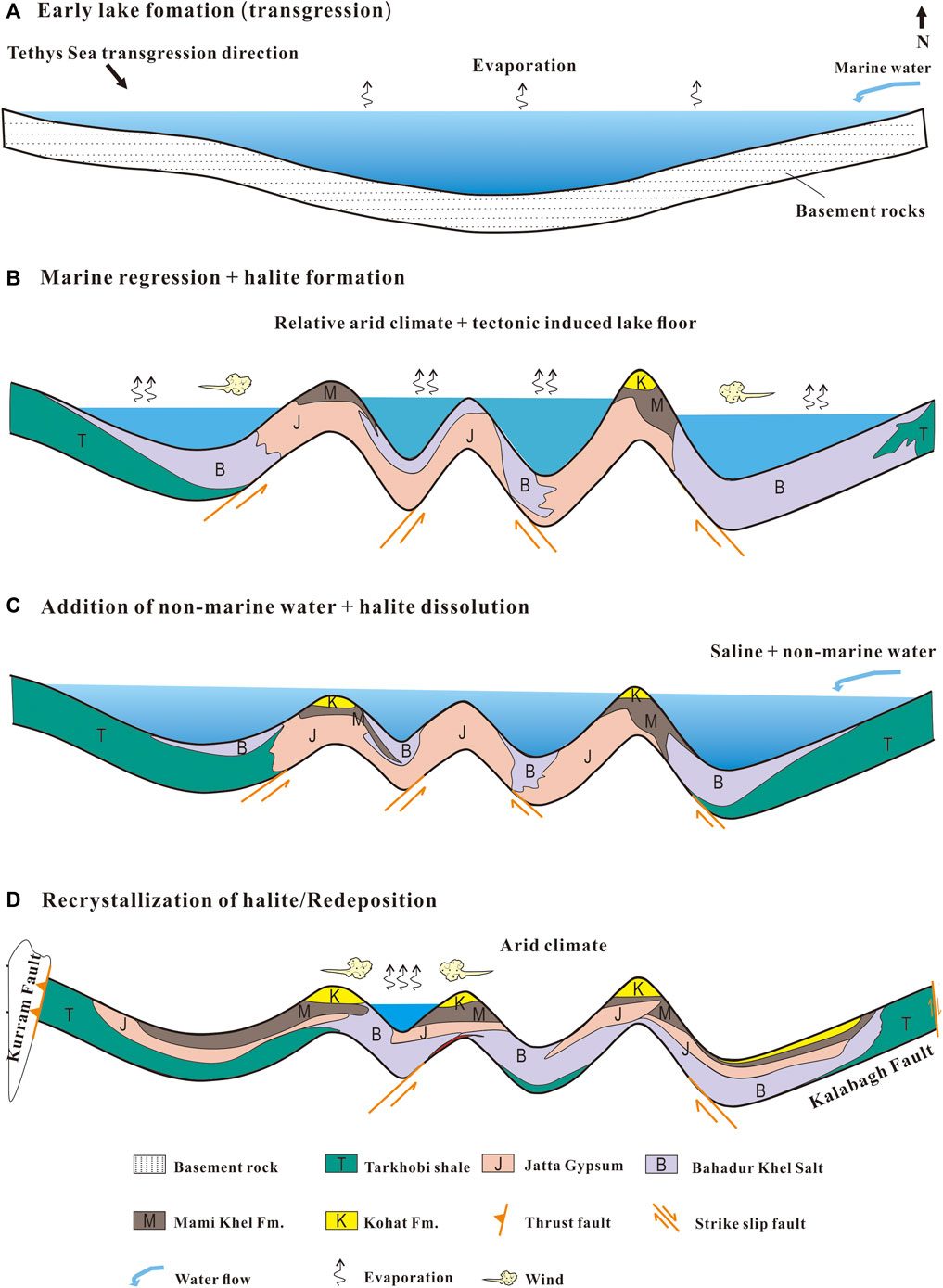
FIGURE 7. Simplified conceptual lake model showing the origin and evolution of the Eocene halite in the Kohat Basin. (A) Lake formation during regression of Tethys sea, (B) marine transgression and halite precipitation, (C) saline and non-marine water input in the lake, and (D) gives recrystallization of halite.
Conclusion
In this study, we used the mineralogy, geochemistry and isotope characteristics of Eocene halite samples from the Kohat Basin in northern Pakistan to discuss the origin and evolution of the evaporites and associated regional paleoclimate. Our results suggested that the source of the ancient halites is not only marine water but also included a combination of solutions having different compositions (e.g. seawater or saline water and meteoric water). Ion compositions (Br−; <3 ppm) and stable high δ37Cl values (+0.83‰) evidenced a non-marine water entry and/or multiple time dissolution/recrystallization by different waters. All samples yielded higher SO42− concentrations compared to Ca2+, which confirmed that halite precipitated from SO42−-type waters. The high Mg2+ contents in the halite samples compared to the Eocene seawater supported a global decrease in the dolomitization process. The absence of primary fluid inclusion indicated that highly unstable regional paleoclimate prevailed during the different stages of the KB formation: 1) during the initial stages shallow seawater existed, which favored the establishment of an evaporite basin, 2) later on, this evaporite basin turned into a terrestrial foreland basin, followed by 3) an open marine basin, and ultimately 4) settled as a terrestrial foreland basin. All this basin sequence was controlled by an active tectonic system that was linked to the Himalayan orogeny and to the global sea-level fluctuations that were triggered by ETM1.
Data Availability Statement
The original contributions presented in the study are included in the article/Supplementary Material, further inquiries can be directed to the corresponding authors.
Author Contributions
Credit author statement: Conceptualization, SH; validation, FH; Software, AH and MM; Formal analysis and investigation, MZ, HJ and SH; Data curation, AA; writing—original draft preparation, SH; writing—review and editing, DW; supervision, FH, DW.
Funding
This scientific work was supported by the Qinghai Natural Science Foundation (grant No. 2019-ZJ-911), Natural Science Foundation of China (No. 42007169), the Fourth Batch of Qinghai Province “Thousand Talents Program for High-end Innovative Talents” (2020000051) and Funds for the Qinghai Province (No. 2020-ZJ-932Q; 2020-ZJ-732).
Conflict of Interest
The authors declare that the research was conducted in the absence of any commercial or financial relationships that could be construed as a potential conflict of interest.
Acknowledgments
We especially thank Ahsan-Ulla Khan & Engineer Shah Burhan-Uddin (Project Manager: PMDC, KPK site, Pakistan) for their help during sampling. We are also thankful to Zhang-Kuang Peng & Prof. Ma Yunqi for their help in the Cl isotope analysis, and the anonymous reviewers for their helpful comments.
References
Afzal, J., Williams, M., Leng, M. J., Aldridge, R. J., and Stephenson, M. H. (2011). Evolution of Paleocene to early eocene larger benthic foraminifer assemblages of the Indus basin, Pakistan. Lethaia 44, 299–320. doi:10.1111/j.1502-3931.2010.00247.x
Ahmad, M., Akram, W., Ahmad, N., Tasneem, M. A., Rafiq, M., and Latif, Z. (2002). Assessment of reservoir temperatures of thermal springs of the northern areas of Pakistan by chemical and isotope geothermometry. Geothermics 31, 613–631. doi:10.1016/s0375-6505(02)00009-3
Ahmad, S., Ali, F., Khan, M. I., and Khan, A. A. (2006). Structural transect of the western Kohat Fold and thrust Belt between hangu and basia Khel, NWFP, Pakistan. Pakistan J. Hydrocarbon Res. 16, 22–35.
Alam, G. S., and Asrarullah, (1973). Potash deposits of salt mine, Khewra, Jhelum District, Punjab, Pakistan. Director General, Geological Survey of Pakistan.
Alcalá, F. J., and Custodio, E. (2004). La deposición atmosférica de cloruro al terreno en España. Boletín Geológico y Minero 115 (5), 319–330.
Alcalá, F. J., and Custodio, E. (2008). Using the Cl/Br ratio as a tracer to identify the origin of salinity in aquifers in Spain and Portugal. J. Hydrol. 359, 189–207. doi:10.1016/j.jhydrol.2008.06.028
Alsop, G. I. (1996). Physical modelling of fold and fracture geometries associated with salt diapirism. Geol. Soc. Lond. Spec. Publications. 100, 227–241. doi:10.1144/gsl.sp.1996.100.01.14
Bloch, M. (1953). On the Cl‐/Br‐ ratio and the distribution of Br ions in liquids and solids during evaporation of bromide-containing chloride solutions. Bull. Res. Counc. Isr.
Bowen, G. J., Maibauer, B. J., Kraus, M. J., Röhl, U., Westerhold, T., Steimke, A., et al. (2015). Two massive, rapid releases of carbon during the onset of the Palaeocene-Eocene thermal maximum. Nat. Geosci. 8, 44–47. doi:10.1038/ngeo2316
Brennan, S. T., Lowenstein, T. K., and Cendón, D. I. (2013). The major-ion composition of Cenozoic seawater: the past 36 million years from fluid inclusions in marine halite. Am. J. Sci. 313, 713–775. doi:10.2475/08.2013.01
Eames, F. (1952). A contribution to the study of Eocene in western Pakistan and western India, Part A. The geology of standard sections in the western Punjab and in the kobat district. Geol.
Eastoe, C. (2016). Stable chlorine isotopes in arid non-marine basins: Instances and possible fractionation mechanisms. Appl. Geochem. 74, 1–12.
Eastoe, C. J., Long, A., and Knauth, L. P. (1999). Stable chlorine isotopes in the Palo Duro Basin, Texas: evidence for preservation of Permian evaporite brines. Geochimica et. Cosmochimica Acta. 63, 1375–1382. doi:10.1016/s0016-7037(99)00186-6
Eastoe, C. J., Long, A., Land, L. S., and Kyle, J. R. (2001). Stable chlorine isotopes in halite and brine from the Gulf Coast Basin: brine genesis and evolution. Chem. Geology. 176, 343–360. doi:10.1016/s0009-2541(00)00374-0
Eastoe, C. J., Peryt, T. M., Petrychenko, O. Y., and Geisler-Cussey, D. (2007). Stable chlorine isotopes in Phanerozoic evaporites. Appl. Geochem. 22, 575–588. doi:10.1016/j.apgeochem.2006.12.012
Eggenkamp, H. G. M. (2015). Comment on "stable isotope fractionation of chlorine during the precipitation of single chloride minerals" by Luo, C.-g., Xiao, Y.-k., wen, H.-j., Ma, H.-z., Ma, Y.-q., Zhang, Y.-l., Zhang, Y.-x. And He, M.-y. [Applied geochemistry 47 (2014) 141-149]. Appl. Geochem. 54, 111–116. doi:10.1016/j.apgeochem.2014.11.018
Eggenkamp, H. G. M., Kreulen, R., and Koster Van Groos, A. F. (1995). Chlorine stable isotope fractionation in evaporites. Geochimica et. Cosmochimica Acta. 59, 5169–5175. doi:10.1016/0016-7037(95)00353-3
Eggenkamp, H. G. M., Louvat, P., Agrinier, P., Bonifacie, M., Bekker, A., Krupenik, V., et al. (2019a). The bromine and chlorine isotope composition of primary halite deposits and their significance for the secular isotope composition of seawater. Geochimica et. Cosmochimica Acta. 264, 13–29. doi:10.1016/j.gca.2019.08.005
Eggenkamp, H. G. M., Louvat, P., Griffioen, J., and Agrinier, P. (2019b). Chlorine and bromine isotope evolution within a fully developed Upper Permian natural salt sequence. Geochimica et Cosmochimica Acta 245, 316–326. doi:10.1016/j.gca.2018.11.010
Eggenkamp, H., Bonifacie, M., Ader, M., and Agrinier, P. (2016). Experimental determination of stable chlorine and bromine isotope fractionation during precipitation of salt from a saturated solution. Chem. Geol. 433, 46–56.
Eggenkamp, H., Griffioen, J., Louvat, P., and Agrinier, P. (2018). “Bromine stable isotope fractionation in evaporites,” in 20th EGU General Assembly, EGU2018, Proceedings from the conference, Vienna, Austria, April 4–13, 2018, 2536.
Ercan, H. Ü., Karakaya, M. Ç., Bozdağ, A., Karakaya, N., and Delikan, A. (2019). Origin and evolution of halite based on stable isotopes (δ37Cl, δ81Br, δ11B and δ7Li) and trace elements in Tuz Gölü Basin, Turkey. Appl. Geochem. 105, 17–30. doi:10.1016/j.apgeochem.2019.04.008
Fatmi, A. N. (1973). Lithostratigraphic units of the Kohat-Potwar province, Indus basin. Pakistan: Geological Survey of Pakistan.
Gardezi, S. a. H. (2016). “Geological constraints on central KOHAT FORELAND basin, Khyber PAKHTUNKHWA, Pakistan: implication from 2d and 3d structural modeling,” in International conference and exhibition Barcelona, Spain: Society of Exploration Geophysicists and American Association of Petroleum, 3–6.
Gee, E. (1938). The economic geology of the northern Punjab, with notes on adjoining portions of the North-West Frontier Province: India Mining Geol. Metall. Inst. Trans. 33, 263–350.
Gee, E. (1945). The age of the Saline Series of the Punjab and of Kohat. Proceedings of the National Academy of Science India 14, 269–310.
Grandia, F., Canals, A., Cardellach, E., Banks, D. A., and Perona, J. (2003). Origin of ore-forming brines in sediment-hosted Zn-Pb deposits of the Basque-Cantabrian Basin, Northern Spain. Econ. Geology. 98, 1397–1411. doi:10.2113/gsecongeo.98.7.1397
Guo, P., Liu, C., Huang, L., Wang, P., Wang, K., Yuan, H., et al. (2017). Genesis of the late Eocene bedded halite in the qaidam basin and its implication for paleoclimate in east Asia. Palaeogeogr. Palaeoclimatol. Palaeoecol. 487, 364–380. doi:10.1016/j.palaeo.2017.09.023
Han, J-L., Hussain, S-A., and Han, F-Q. (2019). Stable chlorine isotopes in saline springs from the Nangqen basin, Qinghai–Tibet Plateau: brine genesis and evolution. J. Earth Syst. Sci. 128, 206. doi:10.1007/s12040-019-1236-0
Hanif, M., Hart, M. B., Grimes, S. T., and Leng, M. J. (2014). Integrated stratigraphy and palaeoenvironment of the P/E boundary interval, Rakhi Nala section, Indus Basin (Pakistan). Arab J. Geosci. 7, 323–339. doi:10.1007/s12517-012-0812-2
Hanif, M., Sabba, M., Ali, N., Rahman, M. U., Ali, F., and Swati, M. a. F. (2020). A multi‐proxy based high‐resolution stratigraphical analysis of the possible Palaeocene–Eocene boundary interval, Salt Range, Pakistan. Geol. J. 56, 434–456. doi:10.1002/gj.3912
Hanif, M. (2011). Stratigraphy and palaeoenvironment of the paleocene/eocene boundary interval in the Indus basin. Pakistan: University of Plymouth.
Hanlon, C., Stotler, R., Frape, S., and Gwynne, R. (2017). Comparison of δ81Br and δ37Cl composition of volatiles, salt precipitates, and associated water in terrestrial evaporative saline lake systems. Isotopes Environ. Health Stud. 53, 446–465. doi:10.1080/10256016.2017.1324856
Hansen, J., Sato, M., Russell, G., and Kharecha, P. (2013). Climate sensitivity, sea level and atmospheric carbon dioxide. Phil. Trans. R. Soc. A. 371, 20120294. doi:10.1098/rsta.2012.0294
Hardie, L. A., and Lowenstein, T. K. (2004). Did the Mediterranean Sea dry out during the Miocene? A reassessment of the evaporite evidence from DSDP Legs 13 and 42A cores. J. Sediment. Res. 74, 453–461. doi:10.1306/112003740453
Heinrich, C. A., Bain, J. H. C., Fardy, J. J., and Waring, C. L. (1993). Br/Cl geochemistry of hydrothermal brines associated with Proterozoic metasediment-hosted copper mineralization at Mount Isa, northern Australia. Geochimica et. Cosmochimica Acta. 57, 2991–3000. doi:10.1016/0016-7037(93)90288-8
Holland, H. D. (2005). Sea level, sediments and the composition of seawater. Am. J. Sci. 305, 220–239. doi:10.2475/ajs.305.3.220
Holland, H. D. (1984). The chemical evolution of the atmosphere and oceans. New Jersey: Princeton University Press.
Holland, H. D., and Zimmermann, H. (2000). The dolomite problem Revisited1. Int. Geology. Rev. 42, 481–490. doi:10.1080/00206810009465093
Holland, O. B., Brown, H., Kuhnert, L., Fairchild, C., Risk, M., and Gomez-Sanchez, C. E. (1984). Further evaluation of saline infusion for the diagnosis of primary aldosteronism. Hypertension 6, 717–723. doi:10.1161/01.hyp.6.5.717
Holser, W. T. (1979). Trace elements and isotopes in evaporites. Marine Minerals. Mineralogical Society of America Short Course Notes Washington, DC doi:10.1515/9781501508646-013
Hsü, K. J., and Bernoulli, D. (1978). Genesis of the Tethys and the mediterranean. Boston, MA: US Government Printing Office.
Hsü, K. J., Ryan, W. B. F., and Cita, M. B. (1973). Late Miocene desiccation of the mediterranean. Nature 242, 240–244. doi:10.1038/242240a0
Hussain, H., and Shuangxi, Z. (2018). Structural evolution of the Kohat Fold and thrust Belt in the shakardarra area (south eastern Kohat, Pakistan). Geosciences 8, 311. doi:10.3390/geosciences8090311
Hussain, M., and Warren, J. K. (1991). Source rock potential of shallow-water evaporites: an investigation in holocenepleistocene Salt Flat sabkah (playa), west Texas-New Mexico. Carbonates Evaporites 6, 217–224. doi:10.1007/bf03174424
Hussain, S. A., Feng-Qing, H., Yunqi, M., Khan, H., Jian, Y., Hussain, G., et al. (2020a). An overview of Pakistan rock salt resources and their chemical characterisation. Pakistan Journal of Scientific and Industrial Research
Hussain, S. A., Han, F-Q., Han, J., Khan, H., and Widory, D. (2020b). Chlorine isotopes unravel conditions of formation of the Neoproterozoic rock salts from the Salt Range Formation, Pakistan. Can. J. Earth Sci. doi:10.1139/cjes-2019-0149
Hussain, S. A., Han, F-Q., Han, W., Rodríguez, A., Han, J-L., Han, J., et al. (2019). Climate change impact on the evolution of the saline lakes of the soan-sakaser valley (central salt range; Pakistan): evidences from hydrochemistry and water (δD, δ18O) and chlorine (δ37Cl) stable isotopes. Water 11, 912. doi:10.3390/w11050912
Hussain, S. A., Han, F-Q., Ma, Z., Hussain, A., Mughal, M. S., Han, J., et al. (2021). Unraveling sources and climate conditions prevailing during the deposition of neoproterozoic evaporites using coupled chemistry and boron isotope compositions (δ11B): the example of the salt range, Punjab, Pakistan. Minerals 11, 161. doi:10.3390/min11020161
Katz, B. G., Eberts, S. M., and Kauffman, L. J. (2011). Using Cl/Br ratios and other indicators to assess potential impacts on groundwater quality from septic systems: a review and examples from principal aquifers in the United States. J. Hydrol. 397, 151–166. doi:10.1016/j.jhydrol.2010.11.017
Kazmi, A. H., and Rana, R. A. (1982). Tectonic map of Pakistan 1: 2 000 000: map showing structural features and tectonic stages in Pakistan. Quetta: Geological Survey of Pakistan.
Khan, K., Khan, I., Leghari, A., and Khan, M. (1987). Geology along the karakoram highway from hasan abdal to khunjerab pass. Quetta: Geological Survey of Pakistan, 39–43.
Kovalevych, V., Marshall, T., Peryt, T., Petrychenko, O., and Zhukova, S. (2006). Chemical composition of seawater in neoproterozoic: results of fluid inclusion study of halite from salt range (Pakistan) and amadeus basin (Australia). Precambrian Res. 144, 39–51. doi:10.1016/j.precamres.2005.10.004
Krishnan, M. S. (1966). Salt tectonics in the Punjab salt range, Pakistan1. Geol. Soc. America Bull. 77, 115–122. doi:10.1130/0016-7606(1966)77[115:stitps]2.0.co;2
Le Fort, P. (1975). Himalayas: the collided range. Present knowledge of the continental arc. Am. J. Sci. 275, 1–44.
Luo, C., Wen, H., Xiao, Y., Ma, H., Fan, Q., Ma, Y., et al. (2016). Chlorine isotopes in sediments of the Qarhan Playa of China and their paleoclimatic significance. Geochemistry. 76, 149–156. doi:10.1016/j.chemer.2016.01.004
Luo, C., Xiao, Y., Ma, H., Ma, Y., Zhang, Y., and He, M. (2012). Stable isotope fractionation of chlorine during evaporation of brine from a saline lake. Chinese Science Bulletin 57 (15), 1833–1843.
Luo, C., Xiao, Y., Wen, H., Ma, H., Ma, Y., Zhang, Y., et al. (2014). Stable isotope fractionation of chlorine during the precipitation of single chloride minerals. Appl. Geochem. 47, 141–149. doi:10.1016/j.apgeochem.2014.06.005
Matray, J. (1988). Hydrochimie et géochimie isotopique des eaux de réservoir pétrolier du Trias et du Dogger dans le Bassin de Paris. Tbbse de 36me cycle, Orsay: Universit6 de Paris-Sud. 118.
Mcinerney, F. A., and Wing, S. L. (2011). The Paleocene-Eocene Thermal Maximum: a perturbation of carbon cycle, climate, and biosphere with implications for the future. Annu. Rev. Earth Planet. Sci. 39, 489–516. doi:10.1146/annurev-earth-040610-133431
Meissner, C. R., Hussain, M., Rashid, M., and Sethi, U. (1975). Geology of the parachinar quadrangle. Pakistana: US Govt. Print. Off.
Meissner, C. R., Master, J., Rashid, M., and Hussain, M. (1974). Stratigraphy of the Kohat quadrangle. Pakistan: US Govt. Print. Off.
Moretto, R. (1986). Etude sédimentologique et géochimique des dépôts de la série salifère paléogène du bassin de Bourg‐en‐Bresse (France). Nancy 1.
Najman, Y., Jenks, D., Godin, L., Boudagher-Fadel, M., Millar, I., Garzanti, E., et al. (2017). The Tethyan Himalayan detrital record shows that India-Asia terminal collision occurred by 54 Ma in the Western Himalaya. Earth Planet. Sci. Lett. 459, 301–310. doi:10.1016/j.epsl.2016.11.036
Paracha, W. (2004). Kohat plateau with reference to Himalayan tectonic general study. CSEG recorder.
Pivnik, D. A., and Khan, M. J. (1996). Transition from foreland- to piggyback-basin deposition, plio-pleistocene upper siwalik group, shinghar range, NW Pakistan. Sedimentology. 43, 631–646. doi:10.1111/j.1365-3091.1996.tb02018.x
Pivnik, D. A., and Sercombe, W. J. (1993a). Transpression-and compression-related, evaporite-controlled faulting and folding in the Kohat Plateau, NW Pakistan. Himalayan Tectonics: Geol. Soc. Lond. Spec. Publications.
Pivnik, D. A., and Sercombe, W. J. (1993b). Compression-and transpression-related deformation in the Kohat Plateau, NW Pakistan. Geol. Soc. Lond. Spec. Publications. 74(1), 559–580. doi:10.1144/gsl.sp.1993.074.01.37
Pivnik, D. A., and Wells, N. A. (1996). The transition from Tethys to the Himalaya as recorded in northwest Pakistan. Geol. Soc. America Bull. 108, 1295–1313. doi:10.1130/0016-7606(1996)108<1295:ttfttt>2.3.co;2
Potter, P. E., and Szatmari, P. (2009). Global Miocene tectonics and the modern world. Earth-Science Rev. 96, 279–295. doi:10.1016/j.earscirev.2009.07.003
Salvany, J. M., GARCÍA‐VEIGAS, J., and OrtI, F. (2007). Glauberite–halite association of the Zaragoza Gypsum Formation (Lower Miocene, Ebro Basin, NE Spain). Sedimentology 54 (2), 443–467.
Shah, I. (1977). Stratigraphy of Pakistan: geological survey of Pakistan memoirs. Islamabad, Pakistan: Geol Surv Pakistan, 12.
Shah, S. (2009). Stratigraphy of Pakistan: geological survey of Pakistan memoir. Quetta: Pakistan: Geol Surv Pakistan., 22.
Shearman, D. J. (1970). Recent halite rock, Baja California. Mexico: Instition of Mining & Metallurgy.
Shouakar-Stash, O. (2008). Evaluation of stable chlorine and bromine isotopes in sedimentary formation fluids. PhD thesis. (Waterloo (Canada): University of Waterloo).
Sonney, R., Vuataz, F.-D., and Cattin, S. (2010). “Use of Cl/Br ratio to decipher the origin of dissolved mineral components in deep fluids from the Alps range and neighbouring areas”, in Proceedings World Geothermal Congress: Institut des sciences du langage et de la communication. Bali, Indonesia: Université de Neuchâtel, 1–13.
Timofeeff, M. N., Lowenstein, T. K., Da Silva, M. A. M., and Harris, N. B. (2006). Secular variation in the major-ion chemistry of seawater: evidence from fluid inclusions in Cretaceous halites. Geochimica et. Cosmochimica Acta. 70, 1977–1994. doi:10.1016/j.gca.2006.01.020
Tomascak, P. B., Magna, T., and Dohmen, R. (2016). Advances in lithium isotope geochemistry. New York: Springer.
Vengosh, A., Chivas, A. R., and Mcculloch, M. T. (1989). Direct determination of boron and chlorine isotopic compositions in geological materials by negative thermal-ionization mass spectrometry. Chem. Geology. Isotope Geosci. Section. 79, 333–343. doi:10.1016/0168-9622(89)90039-0
Walter, L. M., Stueber, A. M., and Huston, T. J. (1990). Br-Cl-Na systematics in Illinois basin fluids: constraints on fluid origin and evolution. Geol. 18, 315–318. doi:10.1130/0091-7613(1990)018<0315:bcnsii>2.3.co;2
Warren, J. K. (2010). Evaporites through time: tectonic, climatic and eustatic controls in marine and nonmarine deposits. Earth-Science Rev. 98, 217–268. doi:10.1016/j.earscirev.2009.11.004
Warren, J. K. (2006). Evaporites: sediments, resources and hydrocarbons. Heidelberg, Germany: Springer Science & Business Media.
Wells, N. (1984). Marine and continental sedimentation in the early Cenozoic Kohat Basin and adjacent northwestern Indo-Pakistan. Ph. D. dissertation. (Ann Arbor: University of Michiga).
Wynne, A. (1875). The Trans-Indus salt region in the Kohat district. New Delhi, India: Government of India.
Wynne, A. (1880). On the Trans-Indus extension of the Punjab salt Range, Calcutta, India: Geological Survey of India, 17. 1–95.
Xiao, Y., Liu, W., Zhou, Y., and Sun, D. (1997). Isotopic compositions of chlorine in brine and saline minerals. Chin.Sci.Bull. 42, 406–409. doi:10.1007/bf02884233
Keywords: kohat-plateau, marine halite, geochemistry, paleoclimate, Cl stable isotopes
Citation: Hussain SA, Han FQ, Ma Z, Hussain A, Mughal MS, Han J, Alhassan A and Widory D (2021) Origin and Evolution of Eocene Rock Salts in Pakistan and Implications for Paleoclimate Studies: Insights From Chemistry and Cl Stable Isotopes. Front. Earth Sci. 9:644485. doi: 10.3389/feart.2021.644485
Received: 21 December 2020; Accepted: 18 February 2021;
Published: 08 April 2021.
Edited by:
Xiangjun Liu, Northwest Normal University, ChinaReviewed by:
Tadeusz Marek, Polish Geological Institute, PolandXiangru Zhang, Guangxi Normal University, China
Copyright © 2021 Hussain, Han, Ma, Hussain, Mughal, Han, Alhassan and Widory. This is an open-access article distributed under the terms of the Creative Commons Attribution License (CC BY). The use, distribution or reproduction in other forums is permitted, provided the original author(s) and the copyright owner(s) are credited and that the original publication in this journal is cited, in accordance with accepted academic practice. No use, distribution or reproduction is permitted which does not comply with these terms.
*Correspondence: Syed Asim Hussain, s.asim_110@yahoo.com; Feng Qing Han, hanfq@isl.ac.cn