- 1Department for Geology & Palaeontology, Universalmuseum Joanneum, Graz, Austria
- 2Institute of Earth Sciences, University of Graz, NAWI Graz Geocenter, Graz, Austria
Before the onset of the modern Amazon River system, northwestern South America was shaped by an extensive wetland during the Miocene. This “Pebas mega-wetland” kept a well-renowned endemic mollusk and ostracod fauna, which initiated a persisting debate about marine ingressions reaching the center of Amazonia at that time. Due to high endemism, uniformitarian principles are hardly applicable to this biota, but also other paleontological, sedimentological, and geochemical information led to ambiguous paleoenvironmental interpretations. Here, we investigate the ostracod and foraminifer assemblages and the oxygen and carbon stable isotope compositions of their biogenic calcite from an outcrop at the cutbank of the Amazon River (NE-Peru, ∼55 km S of Iquitos). While ostracods (e.g., the genus Cyprideis) are able to calcify their carapaces along the entire salinity range, at least low saline conditions are a prerequisite for the biomineralization of calcareous foraminiferan tests. Hence, the finding of calcareous foraminifers (Ammonia, Elphidium), associated mainly with brackish water ostracods indicates the presence of saline waters. In contrast, δ18O and δ13C analyses performed on co-occurring ostracod valves and foraminiferan tests yielded constantly very light ratios. Such values refer to a pure freshwater environment and are incompatible with the interference of isotopically heavier, marine waters or a stable isotope enrichment by evaporation. Based on these contrasting data, we hypothesize that the Pebas mega-wetland was episodically influenced by saline but isotopically light groundwater discharge. Possibly, the resulting specific hydrochemistry not only contributed to the evolution of the endemic Pebasian fauna but also facilitated the sporadic settlement of euryhaline foraminifers, which mimics short-lived marine incursions.
Introduction
Since Gabb (1869, p. 197) recognized “a marine, or rather a brackish water [mollusk] fauna” close to the village Pebas (∼160 km ENE Iquitos, NE-Peru; Figure 1A), the debate about the presence of marine ingressions reaching the heart of Amazonia in “a very recent era” continues (e.g., Jaramillo et al., 2017). Currently, the existence of a huge, long-lived wetland (i.a., “Lake Pebas” or “Pebas mega-wetland”) covering western Amazonia during the Miocene is widely accepted (e.g., Wesselingh et al., 2002; Hoorn et al., 2010a, b; Figure 1A). However, sedimentological (tidalites; e.g., Hovikoski et al., 2010), paleontological (ichnofossils, marine plant, protist, mollusk, crustacean, vertebrate fossils; e.g., Boonstra et al., 2015), and geochemical observations (Vonhof et al., 1998, 2003) arguing for or against marine influxes on this wetland remain a matter of debate (e.g., Kaandorp et al., 2006; Wesselingh et al., 2006b; Westaway, 2006; Latrubesse et al., 2010; Gross et al., 2011, 2013, 2016). Accordingly, the resulting paleoenvironmental interpretations for the Pebas wetland range from shallow marine (e.g., Räsänen et al., 1995; Webb, 1995), to brackish lacustrine (e.g., Whatley et al., 1998) and fluviolacustrine with short-lived marine incursions (e.g., Wesselingh et al., 2002, 2006b; Hoorn et al., 2010a) to an entirely fluvial system (Latrubesse et al., 2010).
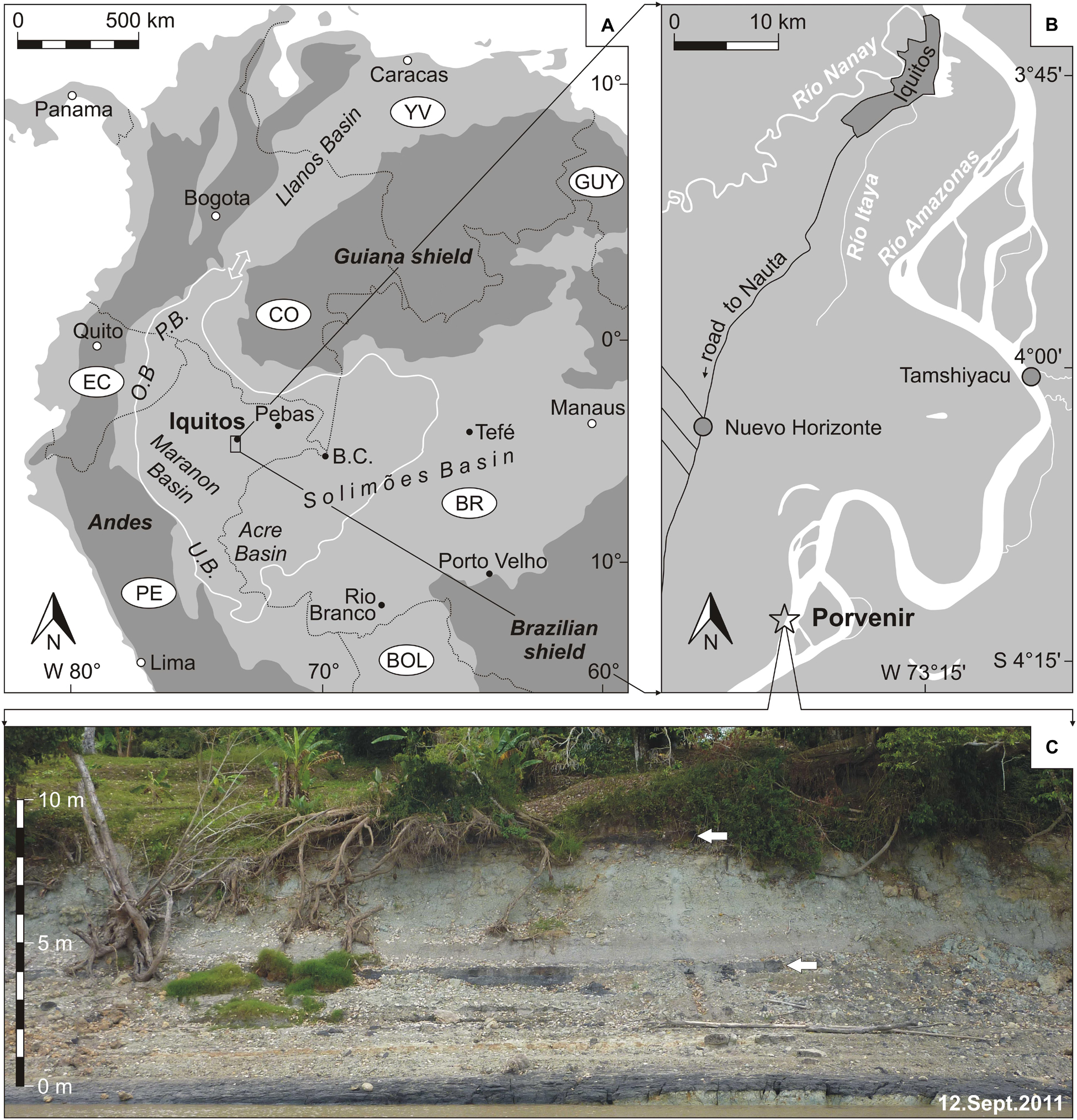
Figure 1. Location of the investigated outcrop Porvenir in NE-Peru. (A) Overview map of northern South America (white line marks the maximal extend of the Pebas wetland during the middle Miocene; double arrow indicates the most favored marine connection through the Llanos Basin; BC = Benjamin Constant, OB = Oriente Basin, PB = Putumayo Basin, UB = Ucayali Basin; adapted from Wesselingh, 2008). (B) Location of the village Porvenir, south of Iquitos (modified from Gross and Piller, 2019). (C) Outcrop photograph of the Porvenir section [field of view ∼40 m; arrows indicate lignite layers (beds 5 and 10), see Figure 2].
The conflict about the salinity regime of the Pebas wetland is well exemplified by Wesselingh et al. (2006b). Within one outcrop (Santa Rosa de Pichana; ∼39 km SSE Pebas; Figure 1A), mollusk assemblages and O/C isotopes from mollusk shells indicate a freshwater environment, whereas ichnofossils point to mesohaline conditions (compare Gingras et al., 2002 and Hovikoski et al., 2007 for Pebasian ichnofossils). These authors proposed two explanations: (i) ichnofossils and mollusks lived in separate time intervals and environments, not resolved by the samples or by the preserved body fossils; or (ii) the producers of ichnofossils have adapted to freshwater like an array of other marine-derived biota (e.g., Bloom and Lovejoy, 2017).
Here, we investigate for the first time the δ18O- and δ13C-isotopy of calcareous ostracod and foraminifer shells co-occurring within the same layer of one outcrop (Porvenir, ∼55 km S Iquitos; Figure 1B). By this bed-by-bed analysis of microfossils, we avoid potential sampling gaps (Wesselingh et al., 2006b; Hovikoski et al., 2007, 2010). While ostracods (bivalved crustaceans) settle almost every aquatic habitat, calcareous foraminifers (unicellular eukaryotes) are restricted to marine habitats or saline inland lakes (e.g., Horne et al., 2002; Iglikowska and Pawłowska, 2015). Hence, the assumption of an adaptation of calcified test bearing foraminifers (“supraliminal evolution”; Myers, 1960; Wesselingh, 2007) to a freshwater environment is much more challenging than for ostracods (Gross et al., 2013, 2016).
Based on our again puzzling paleontological and O/C-isotope data, we explore the possibility of marine incursions affecting the Pebas wetland as well as alternative explanations for the occurrence of foraminifers more than 1,000 km away from the next assumed paleocoast (Boonstra et al., 2015).
Geological Setting and Age
The sampled outcrop Porvenir (S 4.226361°, W 73.36994°) locates on the left cutbank of the Amazon River (Figures 1B,C) in the area of the “Iquitos arch.” This NW–SE-trending anteclise separates the Marañon basin from the Solimões basin (e.g., Roddaz et al., 2005a, b; Rebata et al., 2006a; Wesselingh and Salo, 2006; Wesselingh et al., 2006a; Zamora and Gil, 2018).
Räsänen et al. (1998) described the outcrop sequence along the village Porvenir over a distance of about 2 km. These authors distinguished between the lignite and fossil bearing Pebas formation and the overlying lignite and mollusk lacking “Unidad Canalizada (Unidad B)” (= uppermost Pebas Fm. or Ipururo Fm.; Rebata et al., 2006b). According to the provided section and geological map, our outcrop belongs to the “lacustrine, occasionally marine influenced” Pebas Fm. (Räsänen et al., 1998; Vonhof et al., 2003; Wesselingh et al., 2006a; Hovikoski et al., 2007).
Biostratigraphically, these deposits are attributed to mollusk zone MZ9 and the Grimsdalea pollen zone, respectively (Wesselingh et al., 2006a). Recent palynostratigraphical recalibrations for the nearby site Nuevo Horizonte (∼20 km NNW Porvenir; also MZ9; Figure 1B) suggest a late middle Miocene age (∼14.2–12.7 Ma; pollen zone T-15 sensu Jaramillo et al., 2011) for the Porvenir section (Boonstra et al., 2015; Gross and Piller, 2019).
Materials and Methods
In the field, we logged the Porvenir section vertically in respect of bed thickness, color, sedimentary structures, grain size, and macrofossil content. Micropaleontological samples (∼1–2 kg; stratigraphical resolution: ∼0.1 m) were taken from nine beds or parts of beds (Figure 2). For micropaleontological analyses 500 g of dried sediment per sample (40°C, 24 h) were disintegrated by using diluted hydrogen peroxide (H2O2:H2O = 1:5) and washed through standard sieves (63/125/250/500 μm). Before drying (40°C, 24 h), wet sieve residues were washed with ethanol (70%; Mischke et al., 2008; Roberts et al., 2018). Residues ≥250 μm were picked completely; half of the residue ≥125 μm was screened for microfossils. Determinations on species level are based on Purper (1979); Sheppard and Bate (1980), Muñoz-Torres et al. (1998); Whatley et al. (2000), Gross et al. (2013; 2014; 2016), and Boonstra et al. (2015). All fossils are housed in the collection of the Department for Geology and Paleontology, Universalmuseum Joanneum, Graz (inventory number UMJG&P 217.218). Prior to stable isotope analysis (δ18O, δ13C), ostracod valves and foraminiferan tests were washed once more with distilled water and finally rinsed in ethanol. Adult valves of four ostracod and two foraminifer species were analyzed with a Thermo-Finnigan Kiel II automated reaction system and a Thermo-Finnigan Delta Plus isotope-ratio mass spectrometer (University of Graz; standard deviation = 0.1‰ relative to NBS-19; results in per mille relative to Vienna Pee Dee Belemnite). The number of specimens needed per analysis (>50 μg) varied between 2 (ostracods) and 10 (foraminifers) specimens. Scanning electron microscope photographs were taken with a JEOL (JSM-6610LV; Universalmuseum Joanneum).
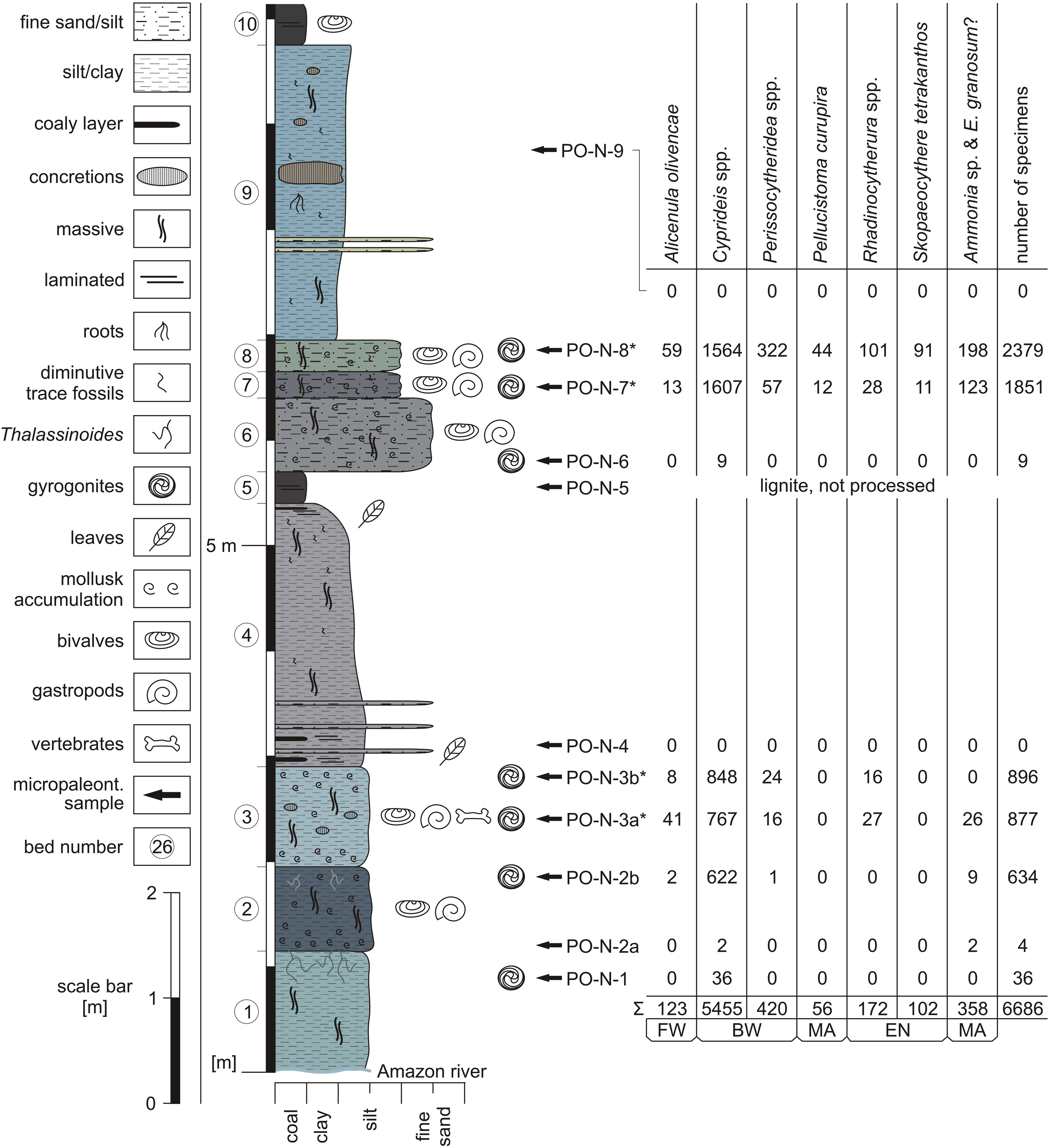
Figure 2. Lithological section of the outcrop north of Porvenir and fossil content (FW = freshwater, BW = brackish water, MA = marine, EN = endemic taxa; *only 1/4 of available specimens determined and counted; modified from Gross and Piller, 2019).
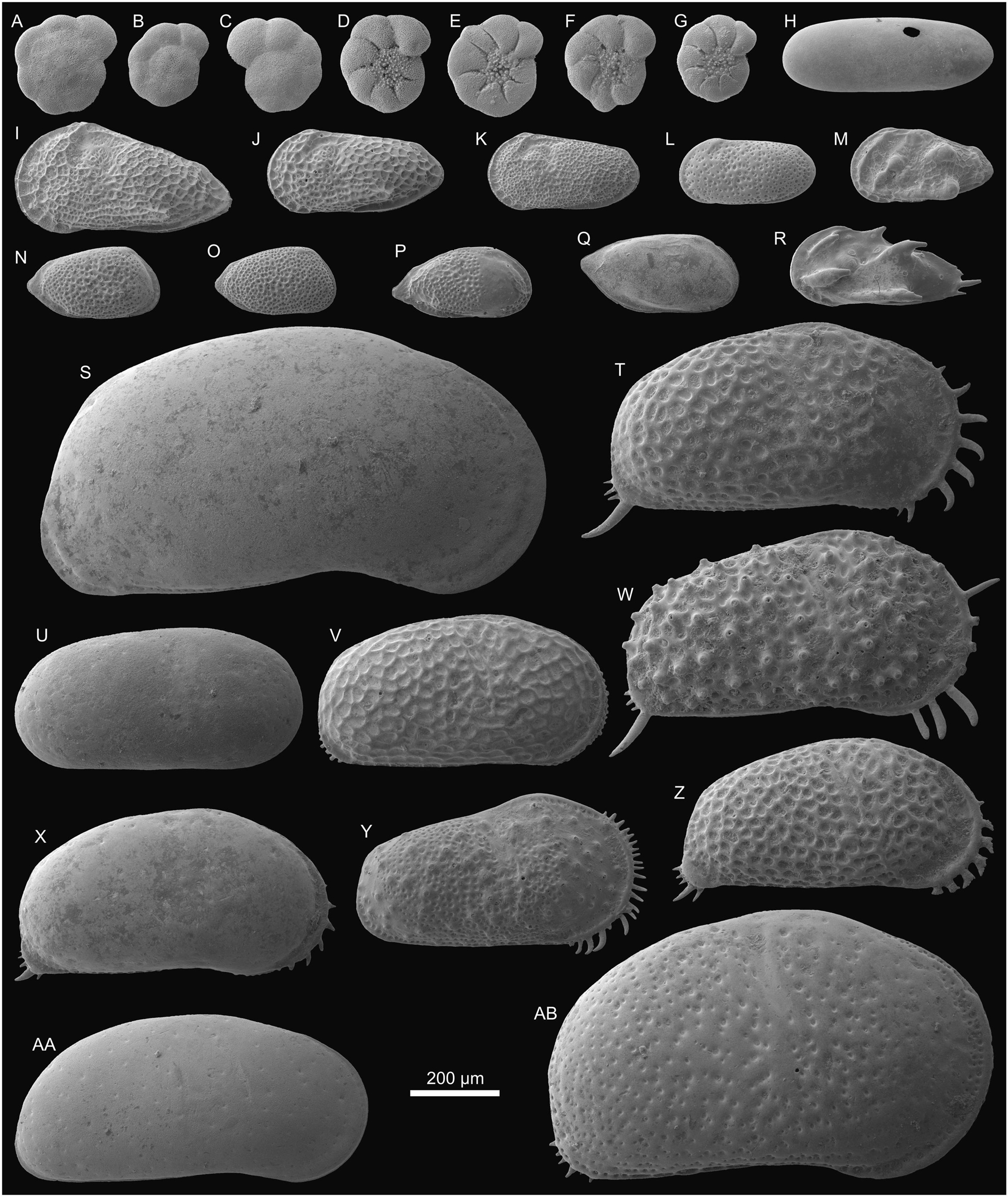
Figure 3. Foraminifers and ostracods from Porvenir (sample PO-N-8). (A–C) Ammonia sp., (A) Tsp (0.25), (B) Tsp (0.20), and (C) Tsp (0.23). (D–G) E. granosum?, (D) Tsi (0.22), (E) Tsi (0.23), (F) Tsi (0.22), and (G) Tsi (0.19). (H) Alicenula olivencae (Rje, 0.47/0.17). (I) Perissocytheridea acuminata (L♀e, 0.49/0.25). (J) P. akistron (L♂e, 0.40/0.19). (K) P. ornellasae (L♂e, 0.34/0.18). (L) Perissocytheridea sp. 1 (L♂e, 0.31/0.15). (M) ?Perissocytheridea sp. 2 (L♀?e, 0.32/0.17). (N) Rhadinocytherura amazonensis (R♂?e, 0.30/0.16). (O) Rhadinocytherura sp. 1 (R♂?e, 0.27/0.15). (P) Rhadinocytherura sp. 2 (Re, 0.32/0.16). (Q) Pellucistoma curupira (Re, 0.36/0.18). (R) Skopaeocythere tetrakanthos (L♂e, 0.38/0.18). (S) Cyprideis machadoi (R♀e, 1.14/0.61). (T) C. aff. graciosa (R♀e, 0.75/0.42). (U) C. paralela (R♀e, 0.65/0.32). (V) C. munoztorresi (R♀e, 0.65/0.34). (W) C. curucae (R♀e, 0.78/0.42). (X) C. cyrtoma (R♀e, 0.70/0.37). (Y) C. inversa (R♀e, 0.62/0.35). (Z) C. ituiae (R♀e, 0.68/0.34). (AA) C. multiradiata (R♀e, 0.79/0.38). (AB) C. sulcosigmoidalis (R♀e, 1.00/0.60). L = left valve; R = right valve; T = test; e = external view; si = side view; sp = spiral view; ♀ = female; j = juvenile; ♂ = male. In brackets the diameter (foraminifers) and length/height (ostracods) are indicated in millimeters; the length of spines on ostracods is not included.
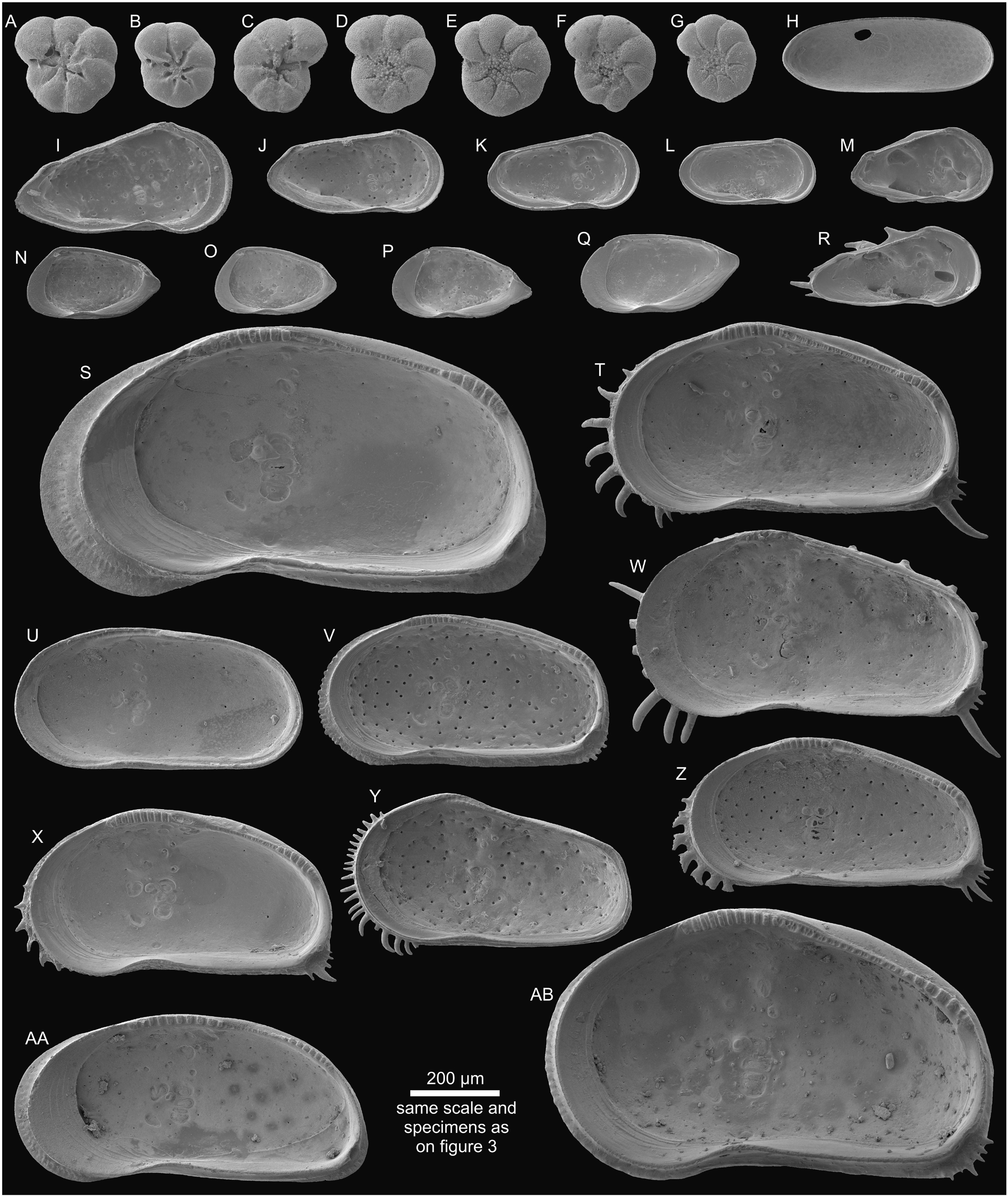
Figure 4. Foraminifers and ostracods from Porvenir (sample PO-N-8; same specimens as in Figure 3). (A–C) Tests in umbilical view. (D–G) Tests in side view. (H–AB) Valves in internal view.
Results
Description of the Porvenir Section
Hovikoski et al. (2007) already described this outcrop (location 9 therein) in detail, which coincides largely with our observations (Gross and Piller, 2019; Figure 2).
Bed 1 consists of more than 1.15-m-thick, green–gray, massive clayey silt. Centimeter-thick Thalassinoides burrows occur in the upper 0.30 m and are filled with dark gray pelite and mollusk shells. Bed 2 comprises 0.80-m-thick, mollusk-rich, dark gray, massive pelite, which is burrowed by Thalassinoides in the uppermost part. Above (bed 3) follow 0.95 m of green gray, partly brown mottled, massive fine sandy pelite with abundant mollusk shells at the top and base. Centimeter-scale calcic concretions and rare turtle remains have been observed. Bed 4 is formed by 2.50 m of dark brown to gray, massive clay with coaly (i.a., leaf remains) and silty fine sand layers in the lower 0.80 m. The uppermost 0.10 m contain coaly layers (i.a., leaf fragments) and merge gradually into a brown, laminated lignite (bed 5). Up-section (bed 6), 0.70 m of mollusk-rich, gray to gray brown, massive silty fine sand and 0.25 m of brown gray, massive fine sandy silt (bed 7), rich in mollusks, follow; 0.30 m of mollusk-rich, green gray, massive fine sandy silt form bed 8. Bed 9 comprises 2.80 m of blue gray, massive clay with some discontinuous fine sand layers, centimeter-scale concretions and root traces. Meter-scale (dm-thick) calcic concretions are developed at section-meter 8.50. The uppermost part of the outcrop (bed 10) consists of 0.40-m-thick, brown, laminated lignite with bivalves.
The Ostracod and Foraminifer Fauna of Porvenir
Eight samples (two were barren; one lignite sample was not processed) from Porvenir furnished 21 ostracod and 3 foraminifer species in total (Figures 2–4; Supplementary Table S1). Several species are left in open nomenclature due to limited material or represent presumably new species. A more detailed treatment of these specimens is beyond the scope of the current study.
Systematic Notes on Ostracods and Foraminifers
Alicenula olivencae (Purper, 1979) has been described originally as Darwinula fragilis by Purper (1979, 1984), was later renamed as Darwinula olivencae (Purper, 1984), and was attributed to the genera Alicenula Rossetti and Martens, 1998 (Wesselingh and Ramos, 2010) or Penthesilenula Rossetti and Martens, 1998 (Gross et al., 2013). Here we follow the opinion of Pinto et al. (2013), who place this species to Alicenula. Basically, this genus is a freshwater ostracod (e.g., lakes, rivers, springs, wet moss) but has been found in oligohaline to mesohaline swamps too (Keyser, 1975; Higuti et al., 2009; Karanovic, 2012).
Ten species of Cyprideis Jones, 1857 have been encountered at Porvenir: Cyprideis machadoi (Purper, 1979); C. aff. graciosa (Purper, 1979); C. paralela (Purper, 1979); C. munoztorresi Gross et al., 2014; C. curucae Gross et al., 2014; C. cyrtoma Muñoz-Torres et al., 1998; C. inversa (Purper and Pinto, 1983); C. ituiae Gross et al., 2014; C. multiradiata (Purper, 1979); and C. sulcosigmoidalis (Purper, 1979). For a review of western Amazonian Cyprideis species, see Gross et al. (2014). Cyprideis is a holoeuryhaline (freshwater to hypersaline) ostracod. It is especially common in shallow, oligohaline to mesohaline marginal marine environments (e.g., lagoons, estuaries, marshes) but also settles lowland rivers, as well as saline and freshwater lakes (e.g., Sandberg, 1964; Meisch, 2000; Gross et al., 2013; Meyer J. et al., 2017; Bright et al., 2018a).
The genus Perissocytheridea Stephenson, 1938, is represented by Perissocytheridea acuminata (Purper, 1979), Perissocytheridea akistron Muñoz-Torres et al., 1998, Perissocytheridea ornellasae (Purper, 1979), Perissocytheridea sp. 1, and ?Perissocytheridea sp. 2. The first three species match with original and subsequent descriptions (e.g., Muñoz-Torres et al., 1998; Gross et al., 2013; Linhares et al., 2017). Perissocytheridea sp. 1 of Porvenir resembles Perissocytheridea sp. 1 in Gross et al. (2013) and Perissocytheridea sp. A in Medeiros (2017) and is certainly a new species for western Amazonia. ?Perissocytheridea sp. 2 is to some degree similar to ?Paracytheridea sp. of Purper (1979). However, due to limited material, we refrain from an assured generic attribution and the description of a new species as well. Perissocytheridea is a euryhaline (oligohaline to hyperhaline) ostracod. It is most common in mesohaline, marginal marine environments (e.g., lagoons) but is found in slightly saline inland lakes too (e.g., Keyser, 1977; Pérez et al., 2011; Gross et al., 2013; Nogueira and Ramos, 2016).
Specimens of Pellucistoma Coryell and Fields, 1937, from Porvenir are identical with Pellucistoma curupira Gross et al., 2016 from late middle Miocene of Brazil (Sucuriju, ∼62 km SW Benjamin Constant). This genus is a euhaline taxon (Gross et al., 2016).
Three species of Rhadinocytherura Sheppard and Bate, 1980 have been found at Porvenir among them one could be determined on species level (Rhadinocytherura amazonensis Sheppard and Bate, 1980). Rhadinocytherura sp. 1 is similar to R. amazonensis but displays a distinct difference in ornament and is probably a new species. Muñoz-Torres et al. (1998, pl. 6, Fig. 10) figured valves under R. amazonensis, which are identical with Rhadinocytherura sp. 2 herein. However, because of marked divergences in outline and ornament, we consider Rhadinocytherura sp. 2 as a separate species, which will be described elsewhere. Rhadinocytherura, a genus endemic for western Amazonia, is supposed to be a marine to brackish water taxon (Sheppard and Bate, 1980).
Skopaeocythere tetrakanthos Whatley et al., 2000 corresponds to the specimens from Porvenir and those in Linhares et al. (2017). It is the only species of this genus, endemic for western Amazonian and considered to be a brackish water form (Whatley et al., 2000).
The rotaliid foraminifers Ammonia Brünnich, 1771 and Elphidium Montfort, 1808 develop various morphotypes, leading to different taxonomical views (e.g., Hayward et al., 2004; Schweizer et al., 2011; Bright et al., 2018b and discussion therein). Our specimens coincide with the material of Boonstra et al. (2015) from Nuevo Horizonte, attributed to Ammonia tepida (Cushman, 1926), Ammonia parkinsoniana (d’Orbigny, 1839) and Elphidium granosum? (d’Orbigny, 1846). In the current work, we do not further differentiate between both Ammonia morphotypes. Ammonia and Elphidium occur in equal numbers at Porvenir, and repeatedly aberrant tests of both have been found. These euryhaline genera dwell typically marginal marine environments (e.g., estuaries, lagoons, mangroves) but also colonize saline inland lakes (e.g., Almogi-Labin et al., 1992; Murray, 2006; Gennari et al., 2011; Boonstra et al., 2015). Ammonia and Elphidium tolerate very low saline (< 1 PSU) waters where specific solute compositions may be the critical factor for their occurrence and not “simply” salinity (e.g., Boltovskoy and Lena, 1971; Wennrich et al., 2007; Flako-Zaritsky et al., 2011; Bright et al., 2018b). Test anomalies are mainly associated to strong salinity fluctuations or hyposaline conditions, but other parameters may trigger or suppress abnormalities (e.g., oxygenation, ion composition of the host water, organic matter input; Flako-Zaritsky et al., 2011; Melis and Covelli, 2013; Boonstra et al., 2015; Pint et al., 2017).
Calcareous Microfossil Content of the Samples
For detailed counts of ostracod valves and foraminiferan tests at species level, see Supplementary Table S1 (very rare = 1–5 specimens, rare = 6–20 specimens, abundant = 21–100 specimens, very abundant ≥ 100 specimens).
Sample PO-N-1: abundant ostracods (only Cyprideis species); rare gyrogonites (charophyte).
Sample PO-N-2a: very rare ostracods (only Cyprideis species); very rare foraminifers.
Sample PO-N-2b: very abundant ostracods; rare foraminifers; rare gyrogonites.
Sample PO-N-3a: very abundant ostracods; abundant foraminifers; abundant gyrogonites.
Sample PO-N-3b: very abundant ostracods; abundant gyrogonites.
Sample PO-N-4: no calcareous microfossils.
Sample PO-N-5: lignite, not processed.
Sample PO-N-6: rare ostracods (only C. sulcosigmoidalis); rare gyrogonites.
Sample PO-N-7: very abundant ostracods; very abundant foraminifers; very abundant gyrogonites.
Sample PO-N-8: very abundant ostracods; very abundant foraminifers; abundant gyrogonites; abundant glochidia (unionid bivalve larvae; Gross and Piller, 2019).
Sample PO-N-9: no calcareous microfossils.
Results of Stable Isotope (δ18O- and δ13C) Analyses
Well-preserved, translucent (foraminifers) or translucent to slightly milky (adult ostracods) specimens were selected for geochemical analyses from layer PO-N-7 and PO-N-8 (Table 1, Figure 5). Both layers contain the richest, most diverse, and best-preserved calcareous microfossil fauna with an admixture of typically freshwater, brackish water, and marine taxa. All measurements furnished very light δ18O and δ13C ratios (for details, see Table 1):
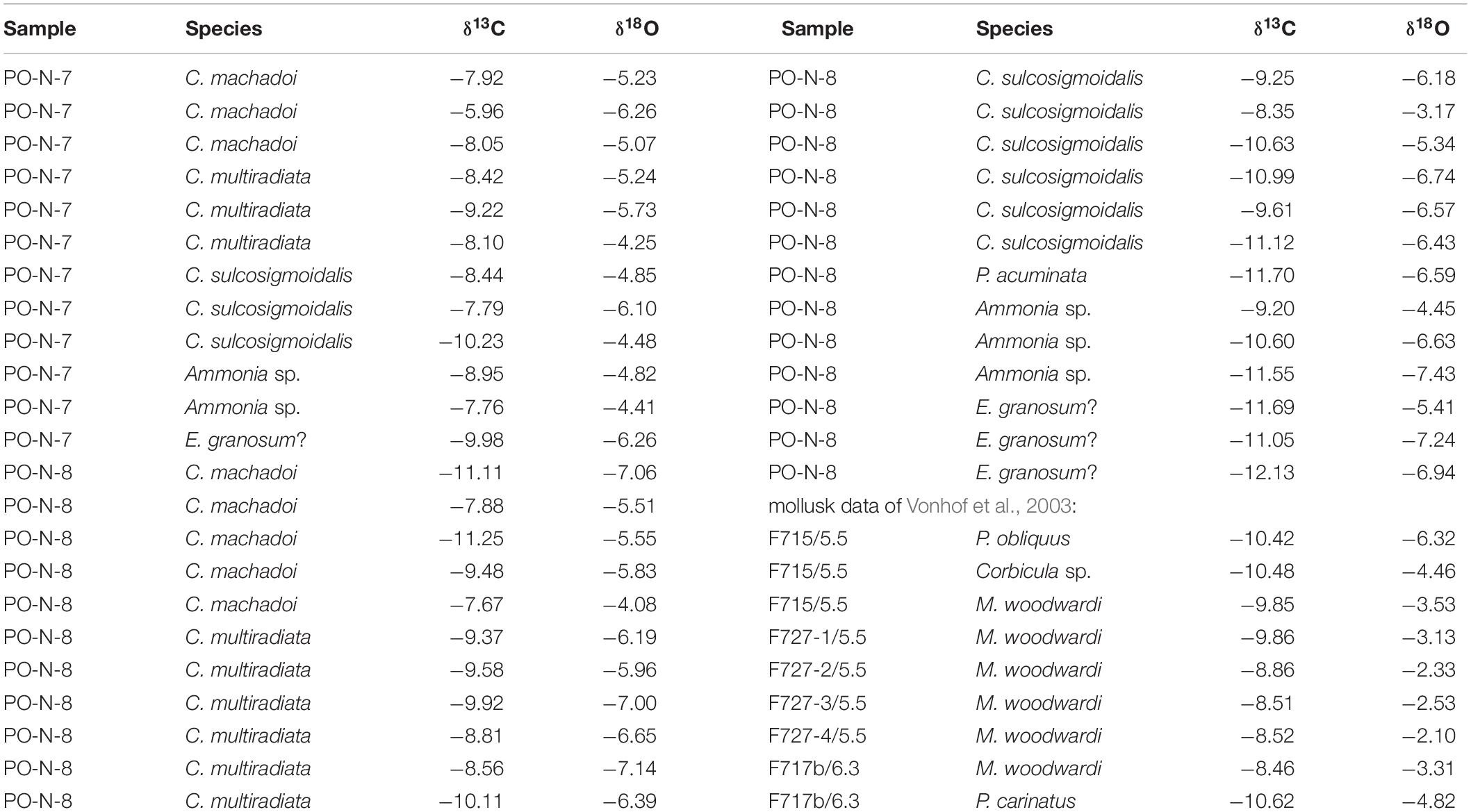
Table 1. δ18O- and δ13C-isotopic ratios of foraminiferan tests, ostracod valves, and mollusk shells from Porvenir (samples PO-N-7 and 8; mollusk data from Vonhof et al., 2003; section meters 5.5 and 6.3 of Vonhof et al., 2003, correspond approximately to PO-N-7 and 8).
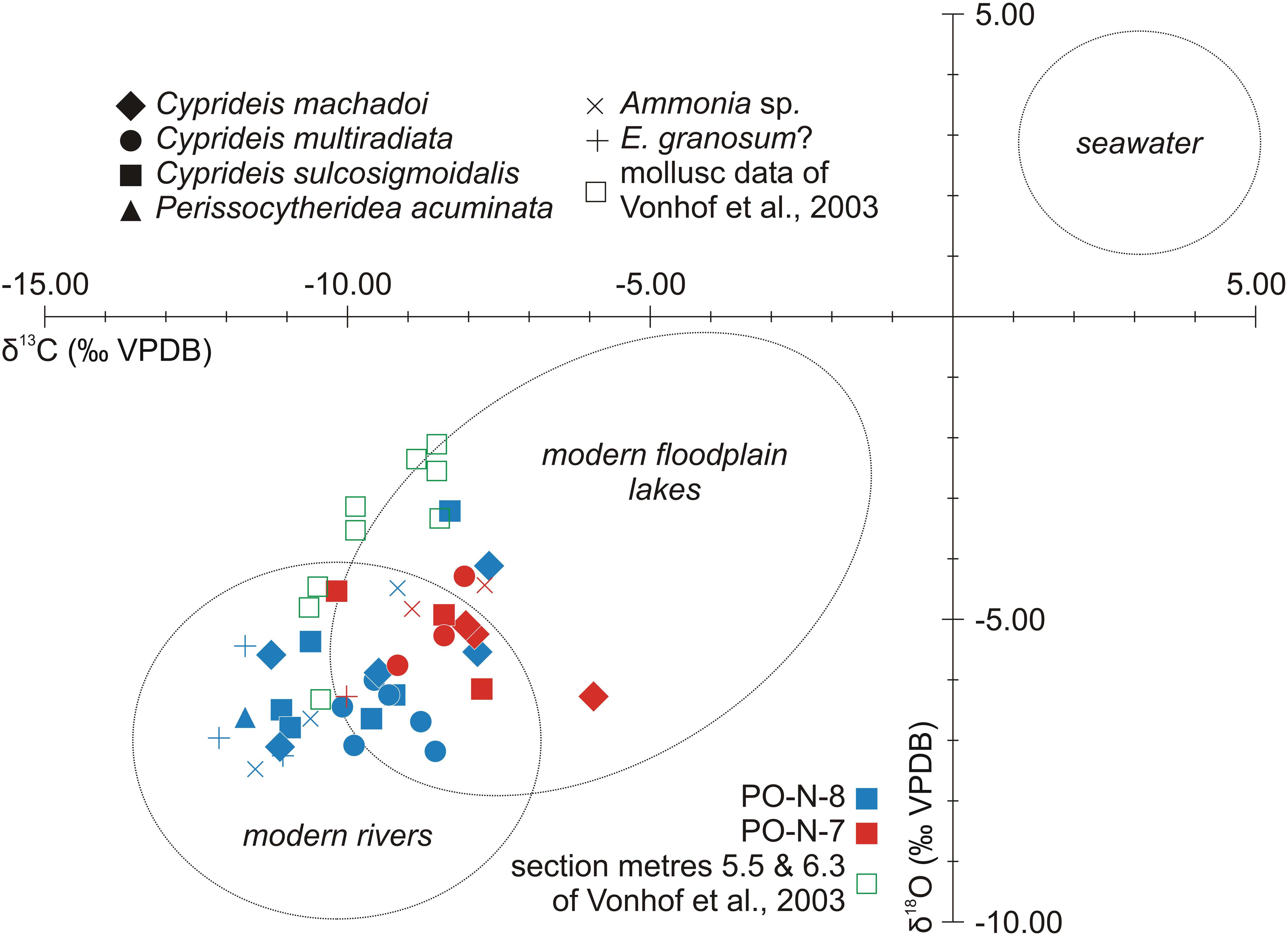
Figure 5. δ18O- and δ13C-isotopic ratios of foraminiferan tests, ostracod valves, and mollusk shells from Porvenir (for data, see Table 1). Ellipses indicate the range of modern Amazonian river and floodplain lake dwelling mollusks (modified from Wesselingh et al., 2006b; note: mollusk aragonite furnish slightly heavier ratios than ostracod calcite within the same environment; Grossman and Ku, 1986).
Sample PO-N-7: C. machadoi (δ18O: −5.07 to −6.26‰, δ13C: −5.96 to −8.05‰), C. multiradiata (δ18O: −4.25 to −5.73‰, δ13C: −8.10 to −9.22‰), C. sulcosigmoidalis (δ18O: −4.48 to −6.10‰, δ13C: −7.79 to −10.23‰), Ammonia species (δ18O: −4.41 to 4.82‰, δ13C: −7.76 to −8.95‰), and E. granosum? (δ18O: −6.26‰, δ13C: −9.98‰).
Sample PO-N-8: C. machadoi (δ18O: −4.08 to −7.06‰, δ13C: −7.67 to −11.25‰), C. multiradiata (δ18O: −5.96 to −7.14‰, δ13C: −8.56 to −10.11‰), C. sulcosigmoidalis (δ18O: −3.17 to −6.74‰, δ13C:−8.35 to −11.12‰), P. acuminata (δ18O: −6.59‰, δ13C: −11.70‰), Ammonia species (δ18O: −4.45 to 7.43‰, δ13C: −9.20 to −11.55‰), and E. granosum? (δ18O: −5.41 to −7.24‰, δ13C: −11.05 to −12.13‰).
Discussion
Paleoenvironmental Inferences From the Paleontological Record
Calcareous Microfossils
Charophyte gyrogonites, found at Porvenir in all productive samples, normally point to shallow freshwater bodies (Gennari et al., 2011). However, a few stoneworts live in (or tolerate) brackish, marine, and also hypersaline waters, which limits their paleoecological indication without a more detailed determination (e.g., Soulié-Märsche, 2008; Soulié-Märsche and García, 2015).
Species of the genus Cyprideis strongly dominate the ostracod faunas of Porvenir (Figure 2). Other taxa occur in minor amounts. All recovered ostracod species and the extinct genera Rhadinocytherura and Skopaeocythere are endemic for western Amazonia. Extant Cyprideis and Perissocytheridea are typical brackish water elements; darwinulids (here Alicenula) dwell usually freshwaters, and Pellucistoma is a full marine taxon (for details, see Systematic Notes on Ostracods and Foraminifers). Rhadinocytherura and Skopaeocythere are allegedly marine to brackish water ostracods (Sheppard and Bate, 1980; Whatley et al., 2000).
Based on the predominance of Cyprideis, oligohaline to mesohaline waters have been assumed (lagoon/estuary: Purper, 1979; Sheppard and Bate, 1980; saline inland lake: Whatley et al., 1998). Perissocytheridea, the second most frequent ostracod at Porvenir, hints at the same direction (Nogueira and Ramos, 2016). Sporadically found freshwater (darwinulids) and marine taxa (here: Pellucistoma, Ammonia, Elphidium) were interpreted as (par-)allochthonous elements or indicators of short-lived marine incursions, respectively (e.g., Purper, 1979; Sheppard and Bate, 1980; Whatley et al., 1998; Boonstra et al., 2015).
Deduced from sedimentological and geochemical data, Gross et al. (2011, 2013) proposed the occurrence of Cyprideis, Perissocytheridea, and Rhadinocytherura in fluvial (freshwater), post-Pebasian deposits. Also an exceptional adaptation of Pellucistoma (and consequently of the co-occurring Skopaeocythere) to freshwater conditions has been discussed (Gross et al., 2016). Hence, none of the recorded ostracod species provides a doubtless clue for the presence of brackish waters or marine incursions, which is, however, clearly contested by the finding of calcareous foraminifers.
Mollusks
The mollusk fauna of Porvenir comprises mainly endemic cochliopid gastropods and corbulid bivalves (for detailed information about the mollusk content, see van Aartsen and Wesselingh, 2000; Wesselingh et al., 2002, 2006a; Vonhof et al., 2003; van Aartsen and Wesselingh, 2005; Wesselingh, 2006). Only Aylacostoma browni (Etheridge, 1879), Corbicula sp., Diplodon amygdalaeformis Wesselingh, 2006, Diplodon longulus (Conrad, 1874), Glabertryonia glabra Wesselingh, 2006, and Neritina roxoi de Greve, 1938 are assumed to be freshwater forms. Remarkably, Gross and Piller (2019) reported very fragile glochidia of the exclusively freshwater unionid Diplodon Spix, 1827. Putative marginal marine taxa are Iolaea amazonica van Aartsen and Wesselingh, 2005, Melongena woodwardi Roxo and de Oliveira, 1924, Odostomia cotuhensis van Aartsen and Wesselingh, 2000, and Odostomia nuttalli van Aartsen and Wesselingh, 2000 (Wesselingh and Ramos, 2010).
Hence, among a wealth of endemic forms, few freshwater and marine taxa co-occur at Porvenir (Figure 2). This is well comparable to the microfossil record and a common pattern for Pebasian strata (e.g., Wesselingh et al., 2006b; Hovikoski et al., 2007, 2010).
As already emphasized by Wesselingh et al. (2002; 2006b; Wesselingh, 2007), the endemic mollusk (and ostracod) faunas indicate a long-lived, somehow isolated aquatic setting and conflicts with regularly open, marine connections (e.g., estuaries). A generally brackish water interpretation (e.g., lagoons, saline inland lake) is at odds with the highly diverse endemic faunas, the frequent occurrence of in situ stenohaline freshwater taxa, and the lack of otherwise common marginal marine mollusks. “Simple” floodplain environments (like modern Amazon’s “várzeas”) mismatch by their low diverse, obligate freshwater and terrestrial faunas too (e.g., Wesselingh et al., 2002; Wesselingh, 2007). These authors concluded a dynamic, overall freshwater mega-wetland, influenced by brief episodes of marine ingressions (see also Hoorn et al., 2010a; Jaramillo et al., 2017).
Ichnofossils
Hovikoski et al. (2007) studied the trace fossil assemblages of Porvenir. The beds containing herein reported foraminifers were interpreted as deltaic and lagoonal deposits within a bay-margin succession. Among others, the ichnofossil Thalassinoides, related to thalassinid shrimp burrows, has been inferred to indicate mesohaline to marine salinity conditions (Gingras et al., 2002; Hovikoski et al., 2007). These authors refused other decapods as producers of these Thalassinoides traces, as well as an adaptation of their constructors to freshwater (Wesselingh et al., 2006b).
However, the only more thoroughly known decapod remains from the Pebas/Solimões Fm. belong to today exclusively freshwater-dwelling trichodactylid crabs (Klaus et al., 2017; see also Antoine et al., 2016). Whether such decapods (or others) could be the producers of Pebasian Thalassinoides requires, of course, further investigations but a reappraisal of this ichnofossil seems appropriate (compare similar discussions about marine vs. fluvial sedimentary structures; e.g., Westaway, 2006; Latrubesse et al., 2007, 2010).
Paleoenvironmental Inferences From the Geochemical Record
In contrast to the paleontological record, geochemical data display a uniform pattern. Consistently, O/C-stable isotope analyses performed on mollusk, ostracod, and foraminiferan shells resulted in very light values (e.g., Vonhof et al., 1998, 2003; Kaandorp et al., 2005, 2006; Wesselingh et al., 2006b; Gross et al., 2013, 2016; and Figure 5 herein).
Significantly diverging O/C-isotopic compositions of rainwater, as, for example, modeled for the Eocene of the Tibetan Plateau (Botsyun et al., 2019) are not projected for the Miocene of western Amazonia. The impact of slightly differing paleolatitude or higher Mid-Miocene temperatures and atmospheric CO2-content are assumed negligible or irresolvable to date. The source of vapor (Atlantic Ocean), its distance from the ocean (causing “continental effects”), and amount (despite probably lower Andes) of precipitation are considered comparable to modern ones (Kaandorp et al., 2005, 2006; see also the modeling of Insel et al., 2010 and Sepulchre et al., 2010). Nevertheless, the effect of the enormous Pebas wetland itself to the Amazonian hydrological cycle remains incalculable so far due to vague boundary conditions (i.e., water surface area and water depth at a specific stratigraphic interval; Kaandorp et al., 2006 and S. Richoz, pers. comm.).
A diagenetic alteration of the isotopic signal is improbable for the mineralogically pristine material and due to the preserved seasonal δ18O-cycles (Vonhof et al., 1998, 2003; Kaandorp et al., 2006). Biologically induced offsets (“vital effects”) of δ18O-values from isotope equilibrium may range in the order of about + 2‰ but will not substantially affect the general isotope signal (Marco-Barba et al., 2012; Holmes and De Deckker, 2017; Meyer J. et al., 2017; Bright et al., 2018a). Variations of δ18O recorded in the growth increments of Pebasian mollusks were related to distinct annual changes in precipitation (Kaandorp et al., 2005, 2006), which might have also affected the chemistry of ostracod valves. Because the isotopy of foraminifers corresponds well with that of the ostracods, the measured O/C ratios could reflect the dry season with the most evaporated and most 16O-depleted waters. All here and previously measured biogenic carbonates furnished well-comparable O/C-values. This indicates that all biota calcified within the same ambient water and precludes reworking or faunal mixing.
An extensive 18O depletion of marine waters reaching western Amazonia by incursions due to strong freshwater input (e.g., Andean runoff, precipitation) is possible. Although such diluted marine waters will be low saline, the amount of essential solutes (e.g., Ca+2) could be sufficient for euryhaline organisms to survive (Bright et al., 2018a, b). However, 87Sr/86Sr-isotope ratios of Pebasian mollusks do not suggest the influence of seawater (except arguable results from Buenos Aires/Colombia; Vonhof et al., 1998, 2003).
Hence, the obtained light O/C ratios are incompatible with the influx of marine, isotopically heavier waters and exclude substantial evaporative effects (18O-enrichment) as well (Wesselingh et al., 2002; Leng and Marshall, 2004; Hovikoski et al., 2010). From O/C-isotopic point of view, the analyzed taxa dwelled in a pure freshwater environment.
To sum up, within the same layers an admixture of usually freshwater, brackish-water, and marine-water biota occurs at Porvenir. This is probably best explained by a brackish (oligohaline) salinity regime, like in an estuarine system (Boonstra et al., 2015). However, a fully freshwater O/C-signal, earlier Sr-isotope analyses (Vonhof et al., 2003) and the faunal composition conflict with this interpretation and render marine incursions questionable. Short-term salinity oscillations not resolved by sampling, an alteration (including vital effects) of the geochemical signal, and mixing of faunas can be excluded (Wesselingh et al., 2006b; Hovikoski et al., 2007, 2010). Therefore, other interpretations about the nature of the Pebas system with its unique fauna and freshwater geochemical signature are discussed below.
An Alternative Hypothesis to the Marine Incursion Model
Calcareous foraminifers, like euryhaline Ammonia and Elphidium, are well known from continental settings (inland lakes, rivers). Yet, all records are related to elevated salinity due to (i) episodic marine influx, (ii) evaporative solute concentration, or (iii) saline groundwater discharge (e.g., Boltovskoy and Boltovskoy, 1968; Boltovskoy and Lena, 1971; Cann and De Deckker, 1981; Patterson, 1987; Patterson et al., 1997; Boudreau et al., 2001; Wennrich et al., 2007; Flako-Zaritsky et al., 2011; Gennari et al., 2011; Pint et al., 2017). At least slightly saline (>0.5 PSU) conditions seem to be a prerequisite for foraminiferan test calcification with the availability of calcium as a critical factor (Toyofuku et al., 2008; Iglikowska and Pawłowska, 2015; Charrieau et al., 2018a, b).
Miocene foraminifers from western Amazonian show a high degree of aberrant tests, which is probably caused by very low salinity and pH (Boonstra et al., 2015; Charrieau et al., 2018a, b) and agrees with geochemical paleosalinity calculations (<1 PSU; Vonhof et al., 2003). Most specimens have megalospherical tests referring to sexual reproduction under fluctuating conditions (Colburn and Baskin, 1998; Flako-Zaritsky et al., 2011). Similarly, otherwise marine ostracods (Pellucistoma) are miniaturized and poorly calcified referring to very low salinity too (Gross et al., 2016).
Notably, western Amazonian Cyprideis consistently lack hollow protuberances (“nodes”) on their shells and bear round sieve pores (e.g., Purper, 1979; Muñoz-Torres et al., 1998; Gross et al., 2014; for extremely rare exceptions, see Linhares et al., 2011). Whereas a dominance of unnoded Cyprideis valves usually points to mesohaline (>14 PSU) salinity regimes, round sieve pores occur characteristically in oligohaline (<1 PSU) waters (Frenzel et al., 2012, 2017 and references therein). This discrepancy could be taxon-specific or related to a peculiar ion composition of the ambient water (e.g., van Harten, 2000; Pint et al., 2012; Grossi et al., 2015). For instance, adequate Ca2+ supply has been demonstrated to reduce the proportion of noded Cyprideis valves (Frenzel et al., 2012; compare Keyser, 2005; Keatings et al., 2007). Perhaps, also the SO42– content of the host water plays an additional role in node formation as the sulfate (and chloride) concentration is negatively correlated to node frequency (Meyer J. et al., 2017).
Hence, not salinity—the total amount of dissolved solids—per se but the ready presence of specific ions could be the controlling parameter for the occurrence of marine ostracods and foraminifers in the Pebas system (Forester, 1983, 1986; Flako-Zaritsky et al., 2011; Pint et al., 2015; Bright et al., 2018b).
If we assume a continental freshwater environment, shaped by precipitation and Andean runoff, and exclude marine ingressions and significant evaporative processes, possibly the inflow of saline, Andean, and/or basement-derived waters accounts for the solute composition of the Pebas wetland.
Today, abundant evaporite-bearing formations (largely gypsum/anhydrite but also halite) crop out west and southwest of the Marañon basin and contribute significantly to the dissolved load of the modern Marañon and Ucayali River systems (Benavides, 1968; Stallard and Edmond, 1983; McClain and Naiman, 2008; Moquet et al., 2011, 2014, 2016). Locally, in proximity to salt diapirs, rivers and lakes with considerably raised salinities are known (e.g., Río Mishquiyacu: ∼4.4 PSU or Lago Limón: ∼24.9 PSU, NW and SE of Tarapoto/Peru, respectively; Stallard and Edmond, 1983; García, 2003; Ortega et al., 2007).
Until the late Miocene, the Huallaga basin, for example, was open to the Pebas system (Hermoza et al., 2005; Roddaz et al., 2005b). It is possible that saline surface waters, originating from Andean evaporite dissolution, affected the shallow Pebas wetland. Nevertheless, high freshwater input (rainwater, surface runoff) probably diluted such waters quickly over long distances (e.g., distance Huallaga evaporite domes–Iquitos > 450 km) and obliterated any effect on Pebasian environments.
Another possibility is that mineralized but O-isotopically light subsurface waters (Jørgensen and Holm, 1994; Wennrich, 2005) have influenced the Pebas wetland. Synsedimentary faults due to forebulge dynamics (e.g., Iquitos arch; Roddaz et al., 2005a, b; Meyer C. M. C. et al., 2017) could have enabled the ascent of ion-rich groundwater during deposition of the Pebas Fm. An upward migration of brackish to hypersaline waters through faults can be still observed in western Amazonia nowadays (Stallard and Edmond, 1983; Rosário et al., 2016). Presumably, such groundwater inferences would be rather local phenomena. However, they could have occurred repeatedly and widespread in the Pebas system, mimicking short-lived marine incursions.
Possibly, the mixture of groundwater and freshwater resulted in a distinct hydrochemistry of Pebasian waters suitable only for few euryoecious biota. For example, athalassic ostracod distributions are sensitive to specific ion compositions (e.g., Ca2+, Cl–, SO42–; Stout, 1981; Keatings et al., 2007; Pint et al., 2015). Especially, Cyprideis seems to thrive in sulfate- and chloride-rich continental waters (Mezquita et al., 1999; Keatings et al., 2007; Bright et al., 2018a). The episodic influx of dissolved CaSO4 (and NaCl) could have favored the occurrence of Cyprideis but hampered the settlement of cypridoid freshwater ostracods and ordinary freshwater mussels (Whatley et al., 1998; Keatings et al., 2007; Wesselingh, 2007). Perhaps, an unusual Ca2+, Cl–, and SO42– supply not only prevented the formation of noded Cyprideis valves but also facilitated the erratic occurrence of marine ostracods and foraminifers.
We assume the Pebas system as a huge, spatial, and temporal highly dynamic tropical wetland consisting of shallow lakes, swamps, and rivers. Climatically driven fluctuations in precipitation and fluvial runoff (water, clasts, solutes) affected this patchy structured freshwater ecosystem in manifold ways (rhythmic sedimentation, oxygenation, turbulence, nutrients, etc.; e.g., Kaandorp et al., 2006; Wesselingh et al., 2006b; Hoorn et al., 2010a; Latrubesse et al., 2010; Gross et al., 2013). In addition, saline groundwater inflow caused an (episodically) aberrant water chemistry with an O/C-isotope freshwater signature but specific ion composition. This contributed to a further habitat diversification within but also to an ecological separation beyond the system (aside other factors; e.g., frequent dysoxia, muddy substrate, predation pressure; Wesselingh et al., 2002; Wesselingh, 2007). The Pebas wetland communicated not necessarily by marine (e.g., Jaramillo et al., 2017; Albert et al., 2018) but rather by fluvial pathways with the Llanos basin and Caribbean Sea, respectively (Nuttall, 1990). Nektic biota of marine origin (e.g., fishes, aquatic mammals) might have migrated actively upstream into the Pebas wetland. Less mobile organisms (e.g., ostracods, foraminifers, mollusks, marine plants) could have introduced phoretically, either by aquatic or aerial transport means (e.g., attached to fishes or birds). After successful colonization, niche occupation triggered the radiation of some marine “arrivals” (e.g., Cyprideis, corbulid bivalves), as well as a gradual, long-term adaptation to post-Pebasian, fluvial environments (Gross et al., 2013, 2014, 2016; Linhares et al., 2017). On occasion, euryhaline foraminifers were introduced by avian transport and reproduced for a short period in groundwater influenced lakes and ponds (e.g., Flako-Zaritsky et al., 2011).
Conclusion
In accordance to earlier studies on the mollusk contents, the microfossil fauna of Porvenir is by far dominated by endemic species of the “brackish water” ostracod Cyprideis. Ordinary freshwater and marine taxa (including foraminifers) occur in minor proportions. Solely based on actualistic evidence, a brackish water paleoenvironment is indicated, which agrees with repeatedly suggested, brief marine connections of the Pebas wetland to the Llanos basin and further to the Caribbean Sea.
Nonetheless, a simple application of uniformitarian principals to the highly endemic Pebasian fauna (and trace fossils) remains ambiguous. Geochemical data (O/C- and Sr isotopes) clearly point to a non–marine-influenced, freshwater environment.
Admittedly, the herein studied Porvenir section covers only a very small portion of the entire spatiotemporal extent of the Pebas Fm. and Pebas system, respectively (∼10 m vs. several hundreds of meters’ thickness; several thousands to few hundred thousands of years vs. several millions of years; e.g., Hoorn et al., 2010a; Jaramillo et al., 2017). However, similar observations were reported from a variety of sites distributed over a wide area of the Pebas system. Thus, our interpretation of such opposing data could be a clue for the whole system or, at least, a novel perspective.
We hypothesize that the Pebas wetland received some influx of “saline” but isotopically “fresh” groundwaters resulting in a complex water chemistry. Such scenario would explain (i) the high endemicity of the Pebasian mollusk and ostracod fauna, (ii) the dominance and diversification of only few groups, (iii) the lack of other common marginal marine taxa, (iv) the low abundance of otherwise widespread freshwater elements, (v) the freshwater isotope signature of calcareous shells, and (vi) the sporadic existence of marine ostracods and foraminifers close to their tolerance limits far inland.
Data Availability Statement
All datasets generated for this study are included in the article/Supplementary Material.
Author Contributions
MG and WP investigated the stratigraphical section. MG studied the fossil content, prepared all figures and wrote the manuscript. WP performed stable isotope analyses and contributed by discussions to the final version of the manuscript.
Conflict of Interest
The authors declare that the research was conducted in the absence of any commercial or financial relationships that could be construed as a potential conflict of interest.
Acknowledgments
The Austrian Science Fund financed this study (project number P21748-N21). Claudia Wrozyna (University of Leipzig) kindly performed a part of the stable isotope analyses. For discussion and for stable isotope analyses at the Institute of Earth Sciences, University of Graz, we thank Sylvain Richoz (Lund University).
Supplementary Material
The Supplementary Material for this article can be found online at: https://www.frontiersin.org/articles/10.3389/feart.2020.00116/full#supplementary-material
References
Albert, J. S., Val, P., and Hoorn, C. (2018). The changing course of the Amazon River in the Neogene: center stage for Neotropical diversification. Neotrop. Ichthyol. 16:e180033. doi: 10.1590/1982-0224-20180033
Almogi-Labin, A., Perelis-Grossovicz, L., and Raab, M. (1992). Living Ammonia from a hypersaline inland pool, Dead Sea, Israel. J. Foramin. Res. 22, 257–266. doi: 10.2113/gsjfr.22.3.257
Antoine, P.-O., Abello, M. A., Adnet, S., Altamirano Sierra, A. L., Baby, P., Billet, G., et al. (2016). A 60-million-year Cenozoic history of western Amazonian ecosystems in Contamana, eastern Peru. Gondwana Res. 31, 30–59. doi: 10.1016/j.gr.2015.11.001
Benavides, V. (1968). Saline deposits of South America. Geol. Soc. Am. Spec. P. 88, 249–290. doi: 10.1130/SPE88-p249
Bloom, D. D., and Lovejoy, N. R. (2017). On the origins of marine-derived freshwater fishes in South America. J. Biogeogr. 44, 1927–1938. doi: 10.1111/jbi.12954
Boltovskoy, E., and Boltovskoy, A. (1968). Foraminíferos y tecamebas de la parte inferior del Río Quequén Grande (Sistemática, distribución, ecología). Rev. Mus. Argent. Cienc. Nat. B. Rivadavia Hidrobiol. 2, 127–164.
Boltovskoy, E., and Lena, H. (1971). The foraminifera (except family Allogromiidae) which dwell in fresh water. J. Foramin. Res. 1, 71–76. doi: 10.2113/gsjfr.1.2.71
Boonstra, M., Ramos, M. I. F., Lammertsma, E. I., Antoine, P.-O., and Hoorn, C. (2015). Marine connections of Amazonia: evidence from foraminifera and dinoflagellate cysts (early to middle Miocene, Colombia/Peru). Palaeogeogr. Palaeoclimatol. Palaeoecol. 417, 176–194. doi: 10.1016/j.palaeo.2014.10.032
Botsyun, S., Sepulchre, P., Donnadieu, Y., Risi, C., Licht, A., and Rugenstein, J. K. C. (2019). Revised paleoaltimetry data show low Tibetan Plateau elevation during the Eocene. Science 363:eaaq1436. doi: 10.1126/science.aaq1436
Boudreau, R. E. A., Patterson, R. T., Dalby, A., and McKillop, W. B. (2001). Non-marine occurrence of the foraminifer Cribroelphidium gunteri in Northern Lake Winnipegosis, Manitoba, Canada. J. Foramin. Res. 31, 108–119. doi: 10.2113/0310108
Bright, J., Cohen, A. S., Dettman, D. L., and Pearthree, P. A. (2018a). Freshwater plumes and brackish lakes: integrated microfossil and O-C-Sr isotopic evidence from the late Miocene and early Pliocene Bouse Formation (California-Arizona) supports a lake overflow model for the integration of the lower Colorado River corridor. Geosphere 14, 1875–1911. doi: 10.1130/GES01610.1
Bright, J., Cohen, A. S., and Starratt, S. W. (2018b). Distinguishing brackish lacustrine from brackish marine deposits in the stratigraphic record: a case study from the late Miocene and early Pliocene Bouse Formation, Arizona and California, USA. Earth Sci. Rev. 185, 974–1003. doi: 10.1016/j.earscirev.2018.08.011
Cann, J. H., and De Deckker, P. (1981). Fossil quaternary and living foraminifera from athalassic (non-marine) saline lakes, Southern Australia. J. Paleontol. 55, 660–670.
Charrieau, L. M., Filipsson, H. L., Ljung, K., Chierici, M., Knudsen, K. L., and Kritzberg, E. (2018a). The effects of multiple stressors on the distribution of coastal benthic foraminifera: a case study from the Skagerrak-Baltic Sea region. Mar. Micropaleontol. 139, 42–56. doi: 10.1016/j.marmicro.2017.11.004
Charrieau, L. M., Filipsson, H. L., Nagai, Y., Kawada, S., Ljung, K., Kritzberg, E., et al. (2018b). Decalcification and survival of benthic foraminifera under the combined impacts of varying pH and salinity. Mar. Environ. Res. 138, 36–45. doi: 10.1016/j.marenvres.2018.03.015
Colburn, D. F., and Baskin, J. A. (1998). A morphological study of Ammonia parkinsoniana from laguna madre and Baffin Bay, Texas. Gulf Coast Ass. Geol. Soc. T. 48, 11–19.
Conrad, T. A. (1874). Remarks on the tertiary clay of the upper amazon with descriptions of new shells. Proc. Acad. Nat. Sci. USA. 1874, 25–32.
de Greve, L. (1938). Eine molluskenfauna aus dem neogen von iquitos am oberen amazonas in peru. Abh. Schweizer. Palaeontol. Ges. 61, 1–133.
d’Orbigny, A. D. (1839). “Foraminifères,” in Histoire Physique, Politique et Naturelle de l’ile de Cuba, ed. R. de la Sagra (Paris: A. Bertrand), 1–224.
d’Orbigny, A. D. (1846). Die Fossilen Foraminiferen des Tertiären Beckens von Wien. Paris: Gide et Comp, 1–312.
Etheridge, R. (1879). Notes on the Mollusca collected by C. Barrington Brown, Esq., A.R.S.M., from the Tertiary deposits of Solimoes and Javary Rivers, Brazil. Quart. J. Geol. Soc. Lond. 35, 82–88. doi: 10.1144/GSL.JGS.1879.035.01-04.126
Flako-Zaritsky, S., Almogi-Labin, A., Schilman, B., Rosenfeld, A., and Benjamini, C. (2011). The environmental setting and microfauna of the oligohaline Timsah pond, Israel: the last remnant of the Kabara swamps. Mar. Micropaleontol. 80, 74–88. doi: 10.1016/j.marmicro.2011.06.002
Forester, R. M. (1983). Relationship of two lacustrine ostracode species to solute composition and salinity: implications for paleohydrochemistry. Geology 11, 435–438. doi: 10.1130/0091-7613198311<435:ROTLOS<2.0.CO;2
Forester, R. M. (1986). Determination of the dissolved anion composition of ancient lakes from fossil ostracodes. Geology 14, 796–799. doi: 10.1130/0091-7613198614<796:DOTDAC<2.0.CO;2
Frenzel, P., Ewald, J., and Pint, A. (2017). Salinity-dependent sieve pore variability in Cyprideis torosa: an experiment. J. Micropaleontol. 36, 57–62. doi: 10.1144/jmpaleo2016-009
Frenzel, P., Schulze, I., and Pint, A. (2012). Noding of Cyprideis torosa valves (Ostracoda) – a proxy for salinity? New data from field observations and a long-term microcosm experiment. Int. Rev. Hydrobiol. 97, 314–329. doi: 10.1002/iroh.201211494
Gabb, W. M. (1869). Description of fossils from the clay deposits of the upper Amazon. Am. J. Conchol. 4, 197–200.
García, J. M. (2003). Hidrografía de la region San Martín. Zonificación ecológica económica de la region San Martín. Estudio temático preliminar. San Martín: Instituto de Investigaciones de la Amazonia Peruana.
Gennari, G., Rosenberg, T., Spezzaferri, S., Berger, J.-P., Fleitmann, D., Preusser, F., et al. (2011). Faunal evidence of a Holocene pluvial phase in Southern Arabia with remarks on the morphological variability of Helenina anderseni. J. Foramin. Res. 41, 248–259. doi: 10.2113/gsjfr.41.3.248
Gingras, M. J., Räsänen, M. E., Pemberton, S. G., and Romero, L. P. (2002). Ichnology and sedimentology reveal depositional characteristics of bay-margin parasequences in the Miocene Amazonian foreland basin. J. Sediment. Res. 72, 871–883. doi: 10.1306/052002720871
Gross, M., and Piller, W. E. (2019). Fossil glochidia (Bivalvia: Unionida: Hyriidae) from the middle Miocene of western Amazonia (Peru). J. Syst. Palaeontol. 17, 897–908. doi: 10.1080/14772019.2018.1498034
Gross, M., Piller, W. E., Ramos, M. I., and Paz, J. D. S. (2011). Late Miocene sedimentary environments in south-western Amazonia (Solimões Formation; Brazil). J. S. Am. Earth Sci. 32, 169–181. doi: 10.1016/j.jsames.2011.05.004
Gross, M., Ramos, M. I., Caporaletti, M., and Piller, W. E. (2013). Ostracods (Crustacea) and their palaeoenvironmental implication for the Solimões Formation (Late Miocene; Western Amazonia/Brazil). J. S. Am. Earth Sci. 42, 216–241. doi: 10.1016/j.jsames.2012.10.002
Gross, M., Ramos, M. I. F., and Piller, W. E. (2014). On the Miocene Cyprideis species flock (Ostracoda; Crustacea) of Western Amazonia (Solimões Formation): refining taxonomy on species level. Zootaxa 3899, 1–69. doi: 10.11646/zootaxa.3899.1.1
Gross, M., Ramos, M. I. F., and Piller, W. E. (2016). A minute ostracod (Crustacea: Cytheromatidae) from the Miocene Solimões Formation (western Amazonia, Brazil): evidence for marine incursions? J. Syst. Palaeontol. 14, 581–602. doi: 10.1080/14772019.2015.1078850
Grossi, F., Gliozzi, E., Anadón, P., Castorina, F., and Voltaggio, M. (2015). Is Cyprideis agrigentina Decima a good paleosalinometer for the Messinian Salinity Crisis? Morphometrical and geochemical analyses from the Eraclea Minoa section (Sicily). Palaeogeogr. Palaeoclimatol. Palaeoecol. 419, 75–89. doi: 10.1016/j.palaeo.2014.09.024
Grossman, E. L., and Ku, T.-L. (1986). Oxygen and carbon isotope fractionation in biogenic aragonite: temperature effects. Chem. Geol. 59, 59–74. doi: 10.1016/0168-9622(86)90057-6
Hayward, B. W., Holzmann, M., Grenfell, H. R., Pawlowski, J., and Triggs, C. M. (2004). Morphological distinction of molecular types in Ammonia – towards a taxonomic revision of the world’s most commonly misidentified foraminifera. Mar. Micropaleontol. 50, 237–271. doi: 10.1016/S0377-8398(03)00074-4
Hermoza, W., Brusset, S., Baby, P., Gil, W., Roddaz, M., Guerrero, N., et al. (2005). The Huallaga foreland basin evolution: thrust propagation in a deltaic environment, northern Peruvian Andes. J. S. Am. Earth Sci. 19, 21–34. doi: 10.1016/j.jsames.2004.06.005
Higuti, J., Lansac-Tôha, F. A., Velho, L. F. M., Pinto, R. L., Vieira, L. C. G., and Martens, K. (2009). Composition and distribution of Darwinulidae (Crustacea, Ostracoda) in the alluvial valley of the upper Paraná River, Brazil. Braz. J. Biol. 69, 253–262. doi: 10.1590/S1519-69842009000200004
Holmes, J. A., and De Deckker, P. (2017). Trace-element and stable-isotope composition of the Cyprideis torosa (Crustacea, Ostracoda) shell. J. Micropalaeontol. 36, 38–49. doi: 10.1144/jmpaleo2015-024
Hoorn, C., Wesselingh, F. P., Hovikoski, J., and Guerrero, J. (2010a). “The development of the Amazonian mega-wetland (Miocene; Brazil, Colombia, Peru, Bolivia),” in Amazonia, Landscape and Species Evolution: A Look into the Past, eds C. Hoorn and F. P. Wesselingh (Oxford: Wiley-Blackwell), 123–142. doi: 10.1002/9781444306408.ch8
Hoorn, C., Wesselingh, F. P., ter Steege, H., Bermudez, M. A., Mora, A., Sevink, J., et al. (2010b). Amazonia through time: andean uplift, climate change, landscape evolution, and biodiversity. Science 330, 927–931. doi: 10.1126/science.1194585
Horne, D. J., Cohen, A., and Martens, K. (2002). Taxonomy, morphology and biology of quaternary and living ostracoda. Am. Geophys. Union Monogr. 131, 5–36. doi: 10.1029/131GM02
Hovikoski, J., Gingras, M., Räsänen, M., Rebata, L. A., Guerrero, J., Ranzi, A., et al. (2007). The nature of Miocene Amazonian epicontinental embayment: high-frequency shifts of the low-gradient coastline. Geol. Soc. Am. Bull. 119, 1506–1520.
Hovikoski, J., Wesselingh, F. P., Räsänen, M., Gingras, M., and Vonhof, H. B. (2010). “Marine influence in Amazonia: evidence from the geological record,” in Amazonia, Landscape and Species Evolution: A Look into the Past, eds C. Hoorn and F. P. Wesselingh (Oxford: Wiley-Blackwell), 143–161. doi: 10.1002/9781444306408.ch9
Iglikowska, A., and Pawłowska, J. (2015). “The adaptations of the foraminifera and ostracoda to fresh water colonisation,” in Impact of Climate Changes on Marine Environments, eds T. Zielinski, M. Weslawski, and K. Kuliñski (Cham: Springer International Publishing), 91–113. doi: 10.1007/978-3-319-14283-8_8
Insel, N., Poulsen, C. J., and Ehlers, T. A. (2010). Influence of the andes mountains on South American moisture transport, convection, and precipitation. Clim. Dyn. 35, 1477–1492. doi: 10.1007/s00382-009-0637-1
Jaramillo, C., Romero, I., D’Apolito, C., Bayona, G., Duarte, E., Louwye, S., et al. (2017). Miocene flooding events of western Amazonia. Sci. Adv. 3:e1601693. doi: 10.1126/sciadv.1601693
Jaramillo, C. A., Rueda, M., and Torres, V. (2011). A palynological zonation for the Cenozoic of the Llanos and Llanos Foothills of Colombia. Palynology 35, 46–84. doi: 10.1080/01916122.2010.515069
Jørgensen, N. O., and Holm, P. M. (1994). “Isotope studies (18O/16O, D/H and 87Sr/86Sr) of saline groundwater in Denmark,” in Future Groundwater Resources at Risk, eds J. Soveri and T. Suokko (Wallingford: International Association of Hydrological Sciences), 231–238.
Kaandorp, R. J. G., Vonhof, H. B., Wesselingh, F. P., Romero Pittman, L., Kroon, D., and van Hinte, J. E. (2005). Seasonal Amazonian rainfall variation in the miocene climate optimum. Palaeogeogr. Palaeoclimatol. Palaeoecol. 221, 1–6. doi: 10.1016/j.palaeo.2004.12.024
Kaandorp, R. J. G., Wesselingh, F. P., and Vonhof, H. B. (2006). Ecological implications from geochemical records of Miocene Western Amazonian bivalves. J. S. Am. Earth Sci. 21, 54–74. doi: 10.1016/j.jsames.2005.07.012
Karanovic, I. (2012). Recent Freshwater Ostracods of the World. Heidelberg: Springer, doi: 10.1007/978-3-642-21810-1
Keatings, K. W., Hawkes, I., Holmes, J. A., Flower, R. J., Leng, M. J., Abu-Zied, R. H., et al. (2007). Evaluation of ostracod-based palaeoenvironmental reconstruction with instrumental data from the arid Faiyum Depression, Egypt. J. Paleolimnol. 38, 261–283. doi: 10.1007/s10933-006-9074-x
Keyser, D. (1975). Ostracoden aus den Mangrovegebieten von Südwest-Florida) (Crustacea: Ostracoda, Podocopida). Abh. Verh. Nat. Ver. Hamburg N. F. 18–19, 255–290.
Keyser, D. (1977). “Ecology and zoogeography of recent brackish-water ostracoda (Crustacea) from south-west Florida,” in Aspects of Ecology and Zoogeography of Recent and Fossil Ostracoda, eds H. Löffler and D. Danielopol (The Hague: Junk), 207–222.
Keyser, D. (2005). Histological peculiarities of the noding process in Cyprideis torosa (Jones) (Crustacea, Ostracoda). Hydrobiologia 538, 95–106. doi: 10.1007/s10750-004-4940-x
Klaus, S., Magalhães, C., Salas-Gismondi, R., Gross, M., and Antoine, P.-O. (2017). Palaeogene and Neogene brachyurans of the Amazon Basin: a revised first appearance date for primary freshwater crabs (Brachyura, Trichodactylidae). Crustaceana 90, 953–967. doi: 10.1163/15685403-00003629
Latrubesse, E., Silva, S. A. F., Cozzuol, M., and Absy, M. L. (2007). Late Miocene continental sedimentation in the southwestern Amazonia and its regional significance: biotic and geological evidence. J. S. Am. Earth Sci. 23, 61–80. doi: 10.1016/j.jsames.2006.09.021
Latrubesse, E. M., Cozzuol, M., Silva-Caminha, S. A. F., Rigsby, C. A., Absy, M. L., and Jaramillo, C. (2010). The late miocene paleogeography of the Amazon Basin and the evolution of the Amazon River system. Earth Sci. Rev. 99, 99–124. doi: 10.1016/j.earscirev.2010.02.005
Leng, M. J., and Marshall, J. D. (2004). Palaeoclimate interpretation of stable isotope data from lake sediment archives. Quaternary Sci. Rev. 23, 811–831. doi: 10.1016/j.quascirev.2003.06.012
Linhares, A. P., Ramos, M. I. F., Gross, M., and Piller, W. E. (2011). Evidence for marine influx during the Miocene in southwestern Amazonia, Brazil. Geol. Colombiana 36, 91–103.
Linhares, A. P., Souza Gaia, V. C., and Ramos, M. I. F. (2017). The significance of marine microfossils for paleoenvironmental reconstruction of the Solimões Formation (Miocene), western Amazonia, Brazil. J. S. Am. Earth Sci. 79, 57–66. doi: 10.1016/j.jsames.2017.07.007
Marco-Barba, J., Ito, E., Carbonell, E., and Mesquita-Joanes, F. (2012). Empirical calibration of shell chemistry of Cyprideis torosa (Jones, 1850) (Crustacea: Ostracoda). Geochim. Cosmochim. Ac. 93, 143–163. doi: 10.1016/j.gca.2012.06.019
McClain, M., and Naiman, R. J. (2008). Andean influences on the Biogeochemistry and Ecology of the Amazon River. BioScience 58, 325–338. doi: 10.1641/B580408
Medeiros, C. G. (2017). Ostracodes da formacão Solimões, Brasil: Contribução à bioestratigrafia do Neógeno da Amazônia. thesis, Universidade de Brasília, Brasília.
Meisch, C. (2000). “Freshwater ostracoda of western and central europe,” in Süßwasserfauna von Mitteleuropa, 8/3, eds J. Schwoerbel and P. Zwick (Heidelberg: Spektrum Akademischer Verlag), 1–522.
Melis, R., and Covelli, S. (2013). Distribution and morphological abnormalities of recent foraminifera in the Marano and Grado Lagoon (North Adriatic Sea, Italy). Mediterr. Mar. Sci. 14, 432–450. doi: 10.12681/mms.351
Meyer, J., Wrozyna, C., Gross, M., Leis, A., and Piller, W. E. (2017). Morphological and geochemical variations of Cyprideis (Ostracoda) from modern waters of the northern Neotropics. Limnology 18, 251–273. doi: 10.1007/s10201-016-0504-9
Meyer, C. M. C., Rodríguez, J. M., Carpio, E. A., García, P. A., Stengel, C., and Berg, M. (2017). Arsenic, manganese and aluminum contamination in groundwater resources of Western Amazonia (Peru). Sci. Total Environ. 607–608, 1437–1450. doi: 10.1016/j.scitotenv.2017.07.059
Mezquita, F., Tapia, G., and Roca, J. R. (1999). Ostracoda from springs on the eastern Iberian Peninsula: ecology, biogeography and palaeolimnological implications. Palaeogeogr. Palaeoclimatol. Palaeoecol. 148, 65–85. doi: 10.1016/S0031-0182(98)00176-X
Mischke, S., Zhang, C., and Börner, A. (2008). Bias of ostracod stable isotope data caused by drying of sieve residues from water. J. Paleolimnol. 40, 567–575. doi: 10.1007/s10933-007-9160-8
Moquet, J.-S., Crave, A., Viers, J., Seyler, P., Armijos, E., Bourrel, L., et al. (2011). Chemical weathering and atmospheric/soil CO2 uptake in the Andean and foreland Amazon basins. Chem. Geol. 287, 1–26. doi: 10.1016/j.chemgeo.2011.01.005
Moquet, J.-S., Guyot, J.-L., Crave, A., Viers, J., Filizola, N., Martinez, J.-M., et al. (2016). Amazon River dissolved load: temporal dynamics and annual budget from the Andes to the ocean. Environ. Sci. Pollut. Res. 23, 11405–11429. doi: 10.1007/s11356-015-5503-6
Moquet, J.-S., Maurice, L., Crave, A., Viers, J., Arevalo, N., Lagane, C., et al. (2014). Cl and Na Fluxes in an Andean Foreland Basin of the Peruvian Amazon: an anthropogenic impact evidence. Aquat. Geochem. 20, 613–637. doi: 10.1007/s10498-014-9239-6
Muñoz-Torres, F., Whatley, R. C., and van Harten, D. (1998). The endemic non-marine Miocene ostracod fauna of the Upper Amazon Basin. Rev. Esp. Micropaleontol. 30, 89–105.
Murray, J. W. (2006). Ecology and Applications of Benthic Foraminifera. Cambridge: Cambridge University Press, doi: 10.1017/CBO9780511535529
Myers, G. S. (1960). The endemic fish fauna of Lake Lanao and the evolution of higher taxonomic categories. Evolution 14, 323–333. doi: 10.2307/2405975
Nogueira, A. A. E., and Ramos, M. I. F. (2016). The genus Perissocytheridea Stephenson, 1938 (Crustacea: Ostracoda) and evidence of brackish water facies along the Oligo-Miocene, Pirabas Formation, eastern Amazonia, Brazil. J. S. Am. Earth Sci. 65, 101–121. doi: 10.1016/j.jsames.2015.11.007
Nuttall, C. P. (1990). A review of the Tertiary non-marine molluscan faunas of the Pebasian and other inland basins of north-western South America. Bull. Brit. Mus. Nat. Hist. Geol. 45, 165–371.
Ortega, H., Guerra, H., and Ramírez, R. (2007). “The introduction of nonnative fishes into freshwater systems of Peru,” in Ecological and Genetic Implications of Aquaculture Activities. Methods and Technologies in Fish Biology and Fisheries, v. 6, ed. T. M. Bert (Dordrecht: Springer), 247–278. doi: 10.1007/978-1-4020-6148-6_14
Patterson, R. T. (1987). Arcellaceans and foraminifera from Pleistocene Lake Tecopa, California. J. Foramin. Res. 17, 333–343. doi: 10.2113/gsjfr.17.4.333
Patterson, R. T., McKillop, W. B., Kroker, S., Nielsen, E., and Reinhardt, E. G. (1997). Evidence for rapid avian-mediated foraminiferal colonization of Lake Winnipegosis, Manitoba, during the Holocene Hypsithermal. J. Paleolimnol. 18, 131–143. doi: 10.1023/A:1007927622654
Pérez, L., Frenzel, P., Brenner, M., Escobar, J., Hoelzmann, P., Scharf, B., et al. (2011). Late Quaternary (24–10 ka BP) environmental history of the Neotropical lowlands inferred from ostracodes in sediments of Lago Petén Itzá, Guatemala. J. Paleolimnol. 46, 59–74. doi: 10.1007/s10933-011-9514-0
Pint, A., Engel, M., Melzer, S., Frenzel, P., Plessen, B., and Brückner, H. (2017). How to discriminate athalassic and marginal marine microfaunas: foraminifera and other fossils from an early Holocene continental lake in northern Saudi Arabia. J. Foramin. Res. 47, 175–187. doi: 10.2113/gsjfr.47.2.175
Pint, A., Frenzel, P., Fuhrmann, R., Scharf, B., and Wennrich, V. (2012). Distribution of Cyprideis torosa (Ostracoda) in quaternary athalassic sediments in Germany and its application for palaeoecological reconstructions. Int. Rev. Hydrobiol. 97, 330–355. doi: 10.1002/iroh.201111495
Pint, A., Frenzel, P., Horne, D. J., Franke, J., Daniel, T., Burghardt, A., et al. (2015). Ostracoda from inland waterbodies with saline influence in Central Germany: implications for palaeoenvironmental reconstruction. Palaeogeogr. Palaeoclimatol. Palaeoecol. 419, 37–46. doi: 10.1016/j.palaeo.2014.07.042
Pinto, R. L., Rocha, C. E. F., Rossetti, G., and Martens, K. (2013). Contribution to the knowledge of the genus Vestalenula Rossetti & Martens, 1998 (Crustacea, Ostracoda, Darwinulidae), with the description of a new species, V. carinata s. sp., from the island of Florianópolis, Brazil. Zootaxa 3666, 62–72. doi: 10.11646/zootaxa.3666.1.6
Purper, I. (1979). Cenozoic ostracodes of the Upper Amazon Basin, Brazil. Pesquisas 12, 209–281. doi: 10.22456/1807-9806.21765
Purper, I., and Pinto, I. D. (1983). New genera and species of ostracodes of the Upper Amazon Basin. Brasil Pesquisas 15, 113–126. doi: 10.22456/1807-9806.21726
Räsänen, M., Linna, A., Irion, G., Hernani Rebata, L., Vargas Huaman, R., and Wesselingh, F. (1998). Geología y geoformas de la zona de Iquitos. Ann. Univ. Turkuensis AII 114, 59–137.
Räsänen, M. E., Linna, A. M., Santos, J. C. R., and Negri, F. R. (1995). Late Miocene tidal deposits in the Amazonian Foreland Basin. Science 269, 386–390. doi: 10.1126/science.269.5222.386
Rebata, L. A. H., Gingras, M. K., Räsänen, M. E., and Barberi, M. (2006). Tidal-channel deposits on a delta plain from the Upper Miocene Nauta Formation, Marañón Foreland Sub-basin, Peru. Sedimentology 53, 971–1013. doi: 10.1111/j.1365-3091.2006.00795.x
Rebata, L. A. H., Räsänen, M. E., Gingras, M. K., Vieira, V. Jr., Barberi, M., and Irion, G. (2006b). Sedimentology and ichnology of tide-influenced Late Miocene successions in western Amazonia: the gradual transition between the Pebas and Nauta formations. J. S. Am. Earth Sci. 21, 96–119. doi: 10.1016/j.jsames.2005.07.011
Roberts, L. R., Holmes, J. A., Leng, M. J., Sloane, H. J., and Horne, D. J. (2018). Effects of cleaning methods upon preservation of stable isotopes and trace elements in shells of Cyprideis torosa (Crustacea, Ostracoda): implications for palaeoenvironmental reconstruction. Quaternary Sci. Rev. 189, 197–209. doi: 10.1016/j.quascirev.2018.03.030
Roddaz, M., Baby, P., Brusset, S., Hermoza, W., and Darrozes, J. M. (2005a). Forebulge dynamics and environmental control in Western Amazonia: the case study of the Arch of Iquitos (Peru). Tectonophysics 399, 87–108. doi: 10.1016/j.tecto.2004.12.017
Roddaz, M., Viers, J., Brusset, S., Baby, P., and Hérail, G. (2005b). Sediment provenances and drainage evolution of the Neogene Amazonian foreland basin. Earth Planet. Sci. Lett. 239, 57–78. doi: 10.1016/j.epsl.2005.08.007
Rosário, F. F., Custodio, E., and Silva, G. C. Jr. (2016). Hydrogeology of the Western Amazon Aquifer System (WAAS). J. S. Am. Earth Sci. 72, 375–386. doi: 10.1016/j.jsames.2016.10.004
Roxo, M. G., and de Oliveira (1924). Breve noticea sobre os fosseis terciario do Alto Amazonas. Bol. Serv. Geol. Mineral. Brasil 11, 41–52.
Sandberg, P. A. (1964). The ostracod Genus Cyprideis in the Americas. Acta Univ. Stockholmiensis 12, 1–178.
Schweizer, M., Polovodova, I., Nikulina, A., and Schönfeld, J. (2011). Molecular identification of Ammonia and Elphidium species (Foraminifera, Rotaliida) from the Kiel Fjord (SW Baltic Sea) with rDNA sequences. Helgoland Mar. Res. 65, 1–10. doi: 10.1007/s10152-010-0194-3
Sepulchre, P., Sloan, L. C., and Fluteau, F. (2010). “Modelling the response of Amazonian climate to the uplift of the Andean mountain range,” in Amazonia, Landscape and Species Evolution: A Look into the Past, eds C. Hoorn and F. P. Wesselingh (Oxford: Wiley-Blackwell), 212–222. doi: 10.1002/9781444306408.ch13
Sheppard, L. M., and Bate, R. H. (1980). Plio-Pleistocene ostracods from the Upper Amazon of Colombia and Peru. Palaeontology 23, 97–124.
Soulié-Märsche, I. (2008). Charophytes, indicators for low salinity phases in North African sebkhet. J. Afr. Earth Sci. 51, 69–76. doi: 10.1016/j.jafrearsci.2007.12.002
Soulié-Märsche, I., and García, A. (2015). Gyrogonites and oospores, complementary viewpoints to improve the study of the charophytes (Charales). Aquat. Bot. 120, 7–17. doi: 10.1016/j.aquabot.2014.06.003
Spix, J. B. (1827). “Diplodon,” in Testacea Fluviatilia Brasiliensia, eds J. B. Spix and J. A. Wagner (Munich: Wolf), 33.
Stallard, R. F., and Edmond, J. M. (1983). Geochemistry of the Amazon. 2. The influence of geology and weathering environment on the dissolved load. J. Geophys. Res. 88, 9671–9688. doi: 10.1029/JC088iC14p09671
Stout, L. N. (1981). An unusual occurrence of the brackish-water ostracode Cyprideis salebrosa in central Missouri. J. Paleontol. 55, 898–900.
Toyofuku, T., de Nooijer, L. J., Yamamoto, H., and Kitazato, H. (2008). Real-time visualization of calcium ion activity in shallow benthic foraminiferal cells using the fluorescent indicator Fluo-3 AM. Geochem. Geophys. Geosyst. 9, 1–6. doi: 10.1029/2007GC001772
van Aartsen, J. J., and Wesselingh, F. P. (2000). New Odostomia species (Gastropoda, Heterobranchia, Pyramidellidae) from the Miocene Pebas Formation of Western Amazonia (Peru, Colombia). Basteria 64, 165–170.
van Aartsen, J. J., and Wesselingh, F. P. (2005). Iolaea amazonica sp. nov., another pyramidellid (Gastropoda, Heterobranchia) from the Miocene Pebas Formation of Western Amazonia (Peru). Basteria 69, 21–24.
van Harten, D. (2000). Variable noding in Cyprideis torosa (Ostracoda, Crustacea): an overview, experimental results and a model from Catastrophe Theory. Hydrobiologia 419, 131–139. doi: 10.1023/A:1003935419364
Vonhof, H. B., Wesselingh, F. P., and Ganssen, G. M. (1998). Reconstruction of the Miocene western Amazonian aquatic system using molluscan isotopic signatures. Palaeogeogr. Palaeoclimatol. Palaeoecol. 141, 85–93. doi: 10.1016/S0031-0182(98)00010-8
Vonhof, H. B., Wesselingh, F. P., Kaandorp, R. J. G., Davies, G. R., van Hinte, J. E., Guerrero, J., et al. (2003). Paleogeography of Miocene Western Amazonia: isotopic composition of molluscan shells constrains the influence of marine incursions. Geol. Soc. Am. Bull. 115, 983–993. doi: 10.1130/B25058.1
Webb, D. S. (1995). Biological implications of the middle miocene Amazon Seaway. Science 269, 361–362. doi: 10.1126/science.269.5222.361
Wennrich, V. (2005). Die spätweichselglaziale und holozäne Klima- und Umweltgeschichte des Mansfelder Landes/Sachsen-Anhalt, abgeleitet aus Seesedimenten des ehemaligen Salzigen Sees. Dissertation, University of Leipzig, Leipzig.
Wennrich, V., Meng, S., and Schmiedl, G. (2007). Foraminifers from Holocene sediments of two inland brackish lakes in Central Germany. J. Foramin. Res. 37, 318–326. doi: 10.2113/gsjfr.37.4.318
Wesselingh, F. P. (2006). Molluscs from the Miocene Pebas Formation of Peruvian and Colombian Amazonia. Scripta Geol. 133, 19–290.
Wesselingh, F. P. (2007). “Long-Lived Lake Molluscs as Island Faunas: a bivalve perspective,” in Biogeography, Time, and Place: Distributions, Barriers, and Islands, ed. W. Renema (Dordrecht: Springer), 275–314. doi: 10.1007/978-1-4020-6374-9_9
Wesselingh, F. P. (2008). Molluscan radiations and landscape evolution in Miocene Amazonia. Ann. Univ. Turkuensis Biol.Geogr. Geol. 232, 1–41.
Wesselingh, F. P., Hoorn, M. C., Guerrero, J., Räsänen, M. E., Romero Pittmann, L., and Salo, J. (2006a). The stratigraphy and regional structure of Miocene deposits in western Amazonia (Peru, Colombia and Brazil), with implications for late Neogene landscape evolution. Scripta Geol. 133, 291–322.
Wesselingh, F. P., Kaandorp, R. J. G., Vonhof, H. B., Räsänen, M. E., Renema, W., and Gingras, M. (2006b). The nature of aquatic landscapes in the Miocene of western Amazonia: an integrated palaeontological and geochemical approach. Scripta Geol. 133, 363–393.
Wesselingh, F. P., and Ramos, M. I. F. (2010). “Amazonian aquatic invertebrate faunas (Mollusca, Ostracoda) and their development over the past 30 million years,” in Amazonia, Landscape and Species Evolution: A Look into the Past, eds C. Hoorn and F. P. Wesselingh (Oxford: Wiley-Blackwell), 302–316. doi: 10.1002/9781444306408.ch18
Wesselingh, F. P., Räsänen, M. E., Irion, G., Vonhof, H. B., Kaandorp, R., Renema, W., et al. (2002). Lake Pebas: a palaeoecological reconstruction of a Miocene, long-lived lake complex in western Amazonia. Cainozoic Res. 1, 35–81.
Wesselingh, F. P., and Salo, J. A. (2006). A Miocene perspective on the evolution of the Amazonian biota. Scripta Geol. 133, 439–458.
Westaway, R. (2006). Late Cenozoic sedimentary sequences in Acre state, southwestern Amazonia: fluvial or tidal? Deductions from the IGCP 449 fieldtrip. J. S. Am. Earth Sci. 21, 120–134. doi: 10.1016/j.jsames.2005.08.004
Whatley, R. C., Muñoz-Torres, F., and van Harten, D. (1998). The Ostracoda of an isolated Neogene saline lake in the western Amazon Basin, Peru. Bull. Cent. Rech. Elf. Explor. Prod. Mem. 20, 231–245.
Whatley, R. C., Muñoz-Torres, F., and van Harten, D. (2000). Skopaeocythere: a minute new limnocytherid (Crustacea, Ostracoda) from the Neogene of the Amazon Basin. Ameghiniana 37, 163–167.
Keywords: Pebas formation, Iquitos region, calcareous microfossils, stable isotopes, paleoenvironments, saline groundwater influx
Citation: Gross M and Piller WE (2020) Saline Waters in Miocene Western Amazonia – An Alternative View. Front. Earth Sci. 8:116. doi: 10.3389/feart.2020.00116
Received: 06 December 2019; Accepted: 27 March 2020;
Published: 12 May 2020.
Edited by:
Michaël Hermoso, UMR 8187 Laboratoire d’Océanologie et de Géosciences (LOG), FranceReviewed by:
José Darrozes, UMR 5563 Géosciences Environnement Toulouse (GET), FranceCarlos Jaramillo, Smithsonian Tropical Research Institute (SI), Panama
Copyright © 2020 Gross and Piller. This is an open-access article distributed under the terms of the Creative Commons Attribution License (CC BY). The use, distribution or reproduction in other forums is permitted, provided the original author(s) and the copyright owner(s) are credited and that the original publication in this journal is cited, in accordance with accepted academic practice. No use, distribution or reproduction is permitted which does not comply with these terms.
*Correspondence: Martin Gross, bWFydGluLmdyb3NzQG11c2V1bS1qb2FubmV1bS5hdA==