- 1Department of Biodiversity and Ecosystem Management, Environmental Sciences Research Institute, Shahid Beheshti University, Tehran, Iran
- 2Department of Plant Science and Biotechnology, Faculty of Life Science and Biotechnology, Shahid Beheshti University, Tehran, Iran
- 3Departments of Environmental Planning and Design, Environmental Sciences Research Institute, Shahid Beheshti University, Tehran, Iran
- 4Department of Environment, Mazandaran Provincial Office, Nowshahr, Mazandaran, Iran
Introduction: Climate warming, combined with grazing pressures, poses significant challenges to vegetation cover and ecosystem stability. In particular, livestock grazing is a primary factor in vegetation degradation, adversely affecting plant diversity, soil health, and key ecological interactions.
Methods: This study investigates the impact of grazing on vegetation in the Alborz region of Iran, focusing on the comparison between native herbivores (Artiodactyla) and livestock in the Central Alborz Protected Area (CAPA). We utilized 30 years of NDVI (Normalized Difference Vegetation Index) data from May to June (1994–2024) to assess vegetation dynamics and evaluate the influence of climate and grazing. ANOVA, Pearson correlation, and regression analyses were performed to identify relationships between vegetation cover, climate variables (temperature and rainfall), and grazing intensity.
Results: Results show a significant increase in vegetation cover, with Golestanak (without livestock) exhibiting a stronger positive response to climate variables (R² = 65%), while Zānoos (higher livestock density) showed greater variability (R² = 58%). Vegetation in Golestanak demonstrated a more significant positive correlation with temperature and rainfall, reflecting resilience to climatic stressors and the beneficial effect of moderate grazing by native Artiodactyla. In contrast, Zānoos showed more fluctuation in vegetation trends due to high livestock grazing pressure.
Discussion: This study highlights that while climate warming can positively affect vegetation cover, intensive livestock grazing can impede these gains, underlining the importance of adaptive management strategies for maintaining ecosystem health.
1 Introduction
Livestock grazing is widely recognized as a primary driver of vegetation degradation, particularly in natural ecosystems rich in diverse native plant species. Overgrazing significantly alters plant community structures, reduces diversity, and disrupts interactions essential for ecosystem resilience (Crovo et al., 2021; Pelliza et al., 2021; Macheroum and Chenchouni, 2022; Abebe et al., 2024). Beyond altering vegetation directly, livestock grazing can cause physical and biochemical changes to soil. The trampling and grazing activities of livestock often result in soil compaction and physical damage, diminishing soil porosity and aeration (Jones et al., 2022; Song et al., 2023; Xu et al., 2023; Blanco-Sepúlveda et al., 2024; Ma et al., 2024; Mushinski et al., 2024). These processes disrupt nutrient cycles, impair biogeochemical functions, and negatively impact soil biota, ultimately reducing ecological health and productivity.
The ecological impact of livestock grazing extends to the disruption of biological community balance and stability, affecting both interspecific and intraspecific relationships within ecosystems (Tian et al., 2019; Öllerer et al., 2019; Filazzola et al., 2020). For instance, overgrazing can interfere with pollination dynamics, altering the mutualistic interactions between native plants and their pollinators (Chen et al., 2022; Kral-O’Brien et al., 2023; SStevenson, 2023). It also disrupts natural herbivory patterns and seed dispersal mechanisms, which can even indirectly affect carnivores by altering the availability of prey species reliant on healthy plant communities (Filazzola et al., 2020; Ugarte et al., 2019; Thapa-Magar et al., 2020; Wilkinson et al., 2020; Pozo et al., 2021).
The severity of grazing impacts is influenced by factors such as livestock density, the duration of grazing practices, and the effectiveness of grazing management strategies. In particular, a lack of seasonal timing and control of grazing events exacerbates resource overuse, further degrading vegetation and soil quality (Narantsetseg et al., 2018; Pulido et al., 2018). These pressures become especially concerning in the context of climate change, where warming temperatures and altered precipitation patterns add additional stressors. As habitats experience shifts in temperature and moisture, the resilience of native plant communities becomes increasingly compromised (Oñatibia et al., 2020; Wei et al., 2022). This synergistic effect of climate-induced stress and unmanaged grazing accelerates vegetation loss, posing a severe threat to the long-term sustainability of these ecosystems. Consequently, there is a critical need for adaptive management strategies that mitigate the combined impacts of climate change and grazing pressures to promote ecosystem stability and resilience.
Seed dispersal through animal ingestion is a critical ecological process that supports the persistence and regeneration of plant populations (Karimi et al., 2018; Pires, 2024; Ghasemi et al., 2024). Large native mammals, in particular, play a crucial role in facilitating seed dispersal, which is essential for maintaining plant diversity and ecosystem functionality. However, these animals are increasingly threatened by anthropogenic pressures such as habitat destruction, poaching, and fragmentation, which can disrupt their dispersal activities and, in turn, impact vegetation dynamics (Gelmi-Candusso and Hämäläinen, 2019; Leclerc et al., 2020; Nield et al., 2020; Draper et al., 2022; Estrada-Villegas et al., 2023). The loss of these ecological services highlights the urgent need for conservation efforts to protect these key species, whose roles in maintaining ecological balance are indispensable.
In contrast, livestock grazing, which is prevalent in northern Iran’s natural pastures, can exert considerable pressure on vegetation, competing with the ecosystem services provided by native herbivores. Livestock grazing has been shown to disrupt plant communities, especially when grazing intensity exceeds the land’s ecological capacity (Gonzalez and Ghermandi, 2021; Shakeri et al., 2021; Salmanpour et al., 2021; Pearson et al., 2022). In many regions, traditional pastoral practices rely heavily on the grazing of livestock in natural meadows, which are often located in areas rich in native plant species. These grazing areas, particularly in the high-altitude pastures on the northern slopes of the Alborz Mountains, are shared by both domestic livestock and native wildlife (Soofi et al., 2018; Hojjati et al., 2021; Savari, 2023; Zandebasiri et al., 2023). Despite the legal framework that permits grazing outside protected zones, few studies have comprehensively examined the long-term impacts of domestic livestock grazing compared to the effects of native artiodactyls, especially in relation to vegetation dynamics and climate change. Understanding these interactions is crucial for informing management strategies aimed at balancing the needs of both livestock and wildlife while mitigating the potential ecological consequences of climate warming.
The findings from this study aim to provide valuable insights into the complex interplay between climate change, grazing pressure, and vegetation dynamics in the Alborz Protected Area. In Zanoos, livestock grazing is carefully managed to prevent entry into the strictly protected Golestanak core zone, where grazing is prohibited under ranger enforcement. This research highlights the importance of such measures in minimizing the impact of grazing on vegetation cover and native herbivore populations. By addressing the effects of climate change alongside varying land-use practices, this study contributes to the growing body of knowledge on ecosystem functioning in diverse landscapes. Ultimately, the outcomes will inform strategies for managing both domestic and wild herbivore populations in the face of climate change and may provide valuable guidance for conservation efforts in similar ecosystems worldwide.
2 Materials and methods
2.1 Study area
The Central Alborz Protected Area (CAPA) in Mazandaran Province, Iran, is a vital biodiversity hotspot. Located at the junction of the eastern and western Alborz Mountains and the Hyrcanian forests, a UNESCO World Heritage site in this area, plays a crucial role in preserving biodiversity (Noroozi et al., 2019, 2020). Spanning 2,950 km², CAPA includes diverse landscapes ranging from the Alborz Mountains’ northern slopes, reaching elevations above 4,000 meters, down to the Caspian Sea’s coastal plains at −16 meters below sea level (Figure 1). Vegetation varies from dense Hyrcanian forests at lower altitudes to sparse high-altitude flora unique to the Alborz region (Darvishsefat, 2008). The CAPA critical habitat for threatened species such as the Caspian red deer (Cervus elaphus maral), Persian leopard (Panthera pardus tulliana), brown bear (Ursus arctos), and roe deer (Capreolus capreolus), alongside other native large mammals like the gray wolf (Canis lupus), wild goat (Capra aegagrus), and wild boar (Sus scrofa) (Nezami and Farhadinia, 2011; Karami et al., 2016; Salmanpour et al., 2022; Nezami et al., 2024). Managed by 13 ranger stations, including three inactive, three seasonal, three semi-active, and four fully operational sites, CAPA faces conservation challenges due to human-wildlife interactions. Conflicts from carnivore predation on livestock, poaching, grazing, beekeeping, and habitat modification continue to pose significant threats to the area’s biodiversity (Soofi et al., 2018; Salmanpour et al., 2021 and 2023; Shakoori and Salmanpour, 2024; Salmanpour et al., 2024).
Within CAPA, the 70.5 km² Zānoos zone (h= 1900–3700 m) permits controlled livestock grazing, while the 46.2 km² Golestanak core zone (h= 2100–3900 m) enforces a complete prohibition on grazing and unauthorized human activities since 1994. Before these restrictions, Golestanak experienced widespread grazing and frequent human disturbances, which significantly strained its ecosystem. The implementation of these protections has been critical in curbing ecological damage, allowing for the habitat’s gradual recovery and regeneration over the past few decades (Unpublished data).
2.2 Data collection
2.2.1 Normalized Difference Vegetation Index (NDVI)
Data from Landsat satellites and the Google Earth Engine platform were utilized to analyze the trends in the Normalized Difference Vegetation Index (NDVI) for the study area. The datasets from Landsat 5, Landsat 7, and Landsat 8 were loaded for the study area and filtered based on the specified region of interest (ROI) and dates. Then, using the mask Clouds function, cloudy pixels were masked using the QA_PIXEL band. The cloud cover percentage for each image was calculated using the calculate Cloud Cover function, and images with cloud cover less than or equal to 50% were filtered out. To calculate NDVI, the calculateNDVI57 and calculateNDVI8 functions were used, and the NDVI datasets from Landsat 5, 7, and 8 were merged. The median NDVI for each August (May–August) from 1994 to 2024 was calculated, and a time series chart was created to display the NDVI trend for August (May–August). Due to its high accuracy and ability to process large volumes of data, this method is widely used in environmental and agricultural studies and can help better understand environmental changes and improve natural resource management.
2.2.2 Native species population size
For data collection on native artiodactyl populations, we relied on official census data for two key species: The Caspian red deer and the wild goat, both of which are native to the CAPA. These population figures were obtained from annual surveys conducted by wildlife management authorities (Department of Environment), which employed systematic monitoring techniques to ensure accuracy and consistency.
2.2.3 Domestic livestock population size
The census data for livestock, including both heavy (cattle) and light (sheep) livestock, were gathered from field censuses conducted at Zānoos farms.
2.2.4 Weather data
Meteorological data were collected daily from the Siah Bisheh synoptic meteorological station, located at an altitude of 2200 meters, approximately 12 km southwest of the study area. This station was selected as it is the closest meteorological site to the Golestanak core zone, sharing a similar elevation and climatic conditions. The climatic parameters analyzed included mean daily temperature (tm, °C), relative humidity (um, %), mean daily precipitation (rrr24, mm), and maximum snowfall (essmax, mm). These data were crucial for evaluating the influence of climate variables on vegetation cover and grazing pressure in the Alborz Protected Zones.
2.3 Statistical analysis
2.3.1 ANOVA
An ANOVA test was performed to analyze changes in the Normalized Difference Vegetation Index (NDVI) across different environmental conditions (May et al., 2020; Hossain and Li, 2021). This statistical test was used to assess whether significant differences existed in NDVI values across varying levels of grazing pressure, climate conditions, and other relevant factors within the study area. The results from the ANOVA test provided a comprehensive understanding of how these variables influenced vegetation cover in the CAPA, offering insights into the potential impacts of climate warming on ecosystem dynamics.
2.3.2 Pearson correlation coefficient
A paired Pearson correlation test was employed to measure the relationship between NDVI values and weather parameters across different months of the year. This statistical approach was used to assess the strength and direction of the linear relationship between vegetation cover (as represented by NDVI) and climatic variables such as temperature, humidity, precipitation, and snowfall (Yan et al., 2022). By analyzing these correlations, the test helped to determine how seasonal weather variations influenced vegetation dynamics in the Alborz Protected Zones, offering insights into the ecological responses to climate change.
2.3.3 Regression
Regression analysis was employed to measure the trends in NDVI changes over the past years, as well as to assess the influence of weather parameters on NDVI. This method allowed us to quantify the relationship between vegetation cover (NDVI) and key climatic factors such as temperature, precipitation, humidity, and snowfall. By examining the trends of these weather parameters over time, we were able to evaluate their impact on vegetation dynamics and identify how fluctuations in climate variables have influenced NDVI trends in the study area.
3 Results
3.1 Temporal trends in vegetation cover across protected zones
The analysis of NDVI across designated time intervals reveals a statistically significant fluctuation in vegetation cover in both the Golestanak and Zānoos protected zones, reflecting a marked enhancement in vegetation density over the study period (Figure 2). For Golestanak, ANOVA results indicate a highly significant temporal effect on NDVI (F = 9.3, df = 30, p < 0.001), with time accounting for 65% of the observed variance (R² = 65%). Mean NDVI values in this zone show a progressive increase from approximately 0.15 during 1994–1999 to 0.18 in the 2020–2024 interval, with error bars denoting the within-period variation. This trend underscores a steady improvement in vegetation cover over time, suggesting a potential relationship with long-term ecological or climatic changes.
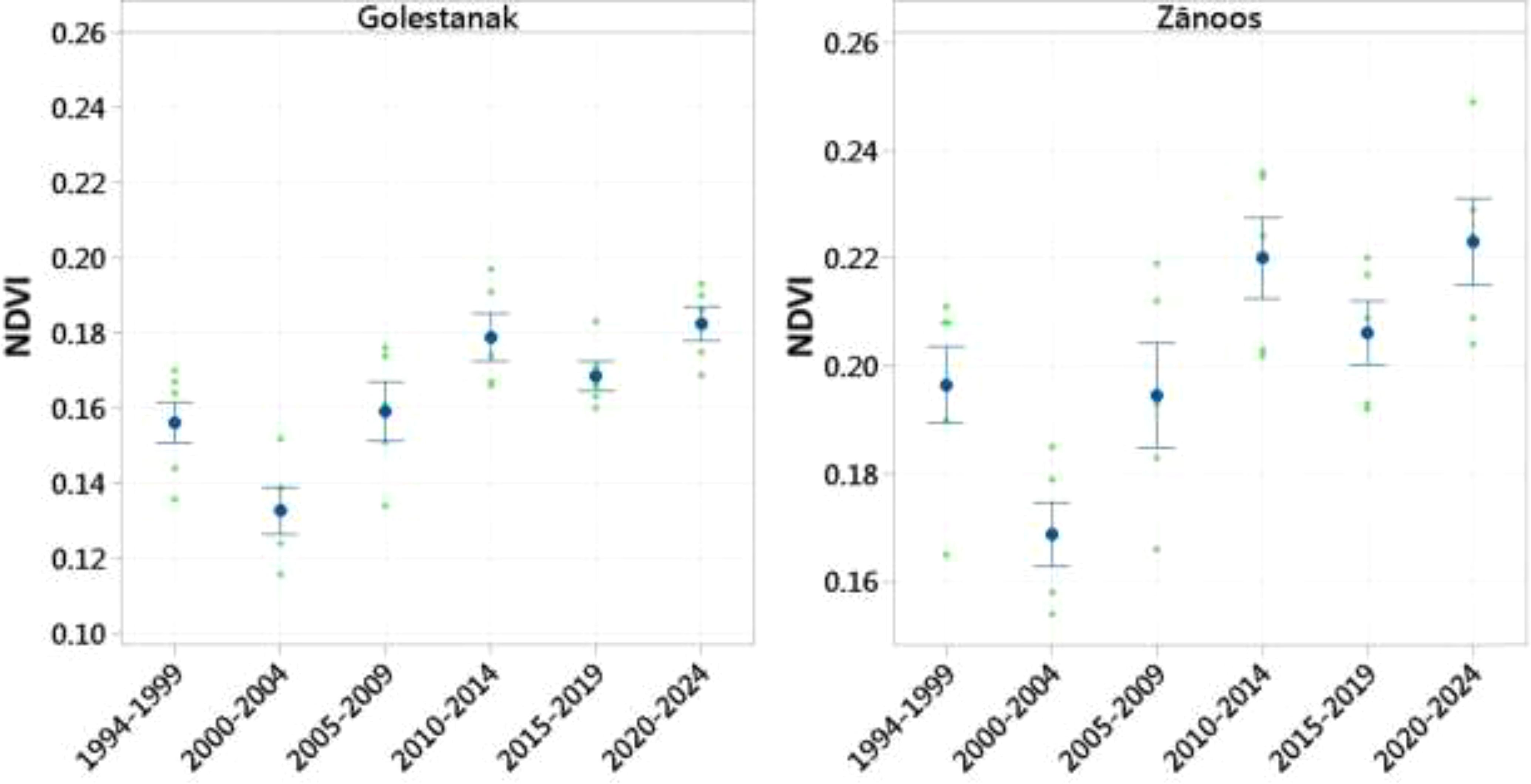
Figure 2. The ANOVA test results show the fluctuations of Golestanak vegetation index compared to Zānoos.
In Zānoos, NDVI exhibited a similar positive trajectory, with ANOVA results demonstrating a significant temporal effect (F = 6.9, df = 30, p < 0.001), and an R² of 58%, indicating moderate explanatory power. Initial NDVI values were recorded at approximately 0.18 in 1994–1999, gradually rising to around 0.23 in the most recent interval (2020–2024). Despite some within-group variability, as illustrated by the error bars, the overall upward trend is consistent and suggests an increase in vegetation cover.
The stronger R² value in Golestanak (65%) compared to Zānoos (58%) implies a more pronounced effect of temporal changes on vegetation dynamics in Golestanak. This discrepancy may be attributable to differences in ecological responses, habitat characteristics, or specific management interventions in the two zones.
3.2 Temporal and spatial changes in vegetation cover in Golestanak and Zānoos
The analysis of NDVI across Golestanak core zone and Zānoos reveals significant temporal and spatial shifts in vegetation cover from 1994 to 2024, suggesting a positive response to conservation efforts and possible climate influences.
In Golestanak, there is a clear upward trend in vegetation density over the study period (Figure 3). In 1994, low NDVI values (mostly below 0.16) indicate sparse vegetation cover. By 2004, areas with higher NDVI values (0.20–0.33) begin to appear, particularly in the northern and central parts of the zone. This trend continues through 2014, with substantial increases in vegetation density observed in several regions. By 2024, a more extensive distribution of high NDVI values is evident, indicating a consistent expansion of vegetative cover over the past three decades. Statistical analysis supports these observations, as the ANOVA results for Golestanak show a significant temporal effect on NDVI (F = 9.3, df = 30, p < 0.001), with time explaining a considerable proportion of the variance (R² = 65%).
Zānoos exhibits a similar but less pronounced pattern. The 1994 NDVI map shows low vegetation density, with values mostly below 0.14. Over time, vegetation cover gradually increases, with NDVI values above 0.20 emerging by 2004 and expanding further in 2014. In 2024, there is a modest increase in NDVI values, though the extent of high-density vegetation remains smaller than in Golestanak. ANOVA results confirm a significant temporal effect on NDVI in Zānoos (F = 6.9, df = 30, p < 0.001), with time explaining 58% of the observed variation (R² = 58%). These patterns suggest that both zones are benefiting from protective measures, with Golestanak showing a more pronounced increase in vegetation cover, possibly due to differences in habitat management, grazing pressure, or native mammal activity.
This graph illustrates the year-over-year percentage changes in NDVI for Golestanak and Zānoos, highlighting shifts in vegetation density and health in response to annual environmental conditions (Figure 4). Given the similar climate in both regions, the observed fluctuations are likely a response to other than prevailing climate patterns, including changes in precipitation, extreme temperatures, and seasonal droughts.
Despite the shared climate, Golestanak and Zānoos show distinct responses in their NDVI trends. Golestanak generally demonstrates more robust and positive NDVI changes, indicating greater vegetation resilience and faster recovery in favorable years. This trend suggests that Golestanak vegetation may have structural or compositional characteristics that allow it to better withstand and recover from climatic stressors, possibly due to variations in species composition, soil quality, or conservation practices.
Conversely, Zānoos experiences more frequent and severe negative NDVI changes, particularly during years with challenging weather conditions, suggesting that its vegetation may be more vulnerable to stress factors like drought or temperature variability. The higher variability in Zānoos’ vegetation response might be indicative of a less resilient ecosystem, where vegetation is more sensitive to common climatic fluctuations, leading to larger declines in vegetation health during adverse conditions.
The error bars indicate variability within each year’s NDVI change, with wider margins observed in years with extreme fluctuations. This variability highlights possible habitat differences within each zone, though the overall trend suggests that Golestanak vegetation benefits from inherent or managed resilience factors that allow it to respond more positively to similar environmental conditions. In summary, although Golestanak and Zānoos experience comparable climate conditions, Golestanak vegetation consistently shows stronger resilience and recovery.
The NDVI analysis reveals interesting contrasts between Golestanak and Zānoos. Although Zānoos currently exhibits a higher NDVI, with values consistently above 0.19 and reaching over 0.23 in recent years, the trend in Golestanak shows a more rapid increase in NDVI over time. In Golestanak, the NDVI values have risen from approximately 0.15 to nearly 0.18 by 2023, demonstrating a statistically significant upward trend (p = 0.001, F = 9, df = 30, R² = 39.15%) compared to Zānoos (p = 0.003, F = 7.3, df = 30, R² = 34.31%).
To quantify this trend difference, I extrapolated the regression equations provided for each site. If the current growth rates continue, Golestanak NDVI is projected to intersect with that of Zānoos probably around the year 2045. This projection underscores the robust vegetation recovery occurring in Golestanak, despite the pressures of grazing, and suggests that, in the long term, Golestanak may reach or even exceed Zānoos in NDVI values if the observed trends persist (Figure 5).
The pairwise Pearson correlation analysis reveals significant relationships between climate variables (temperature and rainfall) and NDVI in both Golestanak and Zānoos. In Golestanak, a significant positive correlation is observed between May temperature (tm – May) and NDVI (r = 0.424, p = 0.039), and a stronger correlation with May rainfall (rrr24 – May) and NDVI (r = 0.461, p = 0.023). This suggests that both temperature and rainfall during May have a noticeable influence on vegetation growth in Golestanak.
In Zānoos, however, while the correlation between May temperature and NDVI is positive, it does not reach statistical significance (r = 0.345, p = 0.099), indicating that May temperatures may have a more limited impact on vegetation compared to Golestanak. Conversely, May rainfall shows a significant positive correlation with NDVI (r = 0.485, p = 0.016), suggesting that precipitation may be a key driver of vegetation growth in Zānoos during this period.
Further, June temperature (tm – Jun) shows a significant positive correlation with NDVI in both regions: Golestanak (r = 0.483, p = 0.017) and Zānoos (r = 0.408, p = 0.048). This indicates that rising temperatures in June are likely to enhance vegetation growth across both sites, supporting the notion that warmer late-spring conditions favor increased NDVI levels.
In the previous section, climate parameters affecting NDVI were identified, but June temperature is the only variable that has shown significant changes over the past years. In Golestanak, where livestock grazing is prohibited and only large native mammals are present, NDVI exhibits a significant positive correlation with June temperature (p = 0.017, F = 6.7, R² = 23.4). The regression model indicates that a 1°C increase in June temperature results in a 12.8%–13.2% increase in NDVI, highlighting a strong positive response in vegetation growth. In contrast, Zānoos, which is heavily impacted by domestic animal grazing, shows a positive relationship between June temperature and NDVI (p = 0.048, F = 4.4, R² = 16.6), but with a more moderate increase of 6.5%–7% in NDVI for the same 1°C temperature rise. Although both regions show a positive correlation between temperature and vegetation growth, the intensity of the response is notably stronger in Golestanak, suggesting that factors such as grazing pressure may influence the magnitude of vegetation response across the regions (Figure 6).
The Golestanak region’s census data indicate that native artiodactyla (red deer and wild goats) exert variable grazing impacts based on their population densities and estimated grazing pressures. Red deer exhibit a mean density of approximately 324 individuals, with a standard deviation spanning from 300 to 348, while the wild goat population maintains a mean density of around 700 individuals, fluctuating between 600 and 800. These densities suggest a moderate grazing impact from wild ungulates, potentially beneficial for vegetation management. This grazing pressure may help enhance biodiversity and control dominant plant species, offering an adaptive advantage under warming climate conditions.
In contrast, livestock census data from the nearby Zānoos region reveal considerably higher grazing pressures exerted by domesticated animals. Sheep populations average around 6,000 individuals (with a standard deviation of 5,500 to 6,500), while cows show a mean density of approximately 550 individuals, ranging from 500 to 600. These elevated densities of domesticated grazers are likely placing significant pressure on local vegetation, potentially leading to overgrazing and a reduction in plant diversity within this protected area.
The spatial analysis of habitat availability in the Golestanak and Zānoos protected zones reveals significant contrasts in habitat distribution per individual for native and domesticated Artiodactyla species, reflecting their natural versus human-influenced distributions. In Golestanak, where native species like red deer and wild goats are present, each individual occupies approximately 42,000–40,000 square meters of habitat (Figure 7). This naturally expansive distribution allows for sustainable grazing, which promotes vegetation recovery and fosters ecosystem stability. Conversely, in Zānoos, where domesticated livestock (primarily sheep and cows) are concentrated, each animal is allocated only about 10,000–12,000 square meters of habitat, within grid cells of 104.8 × 104.8 meters. This artificial, dense distribution resulting from livestock management practices significantly intensifies grazing pressure per unit area, increasing the likelihood of overgrazing and subsequent vegetation degradation.
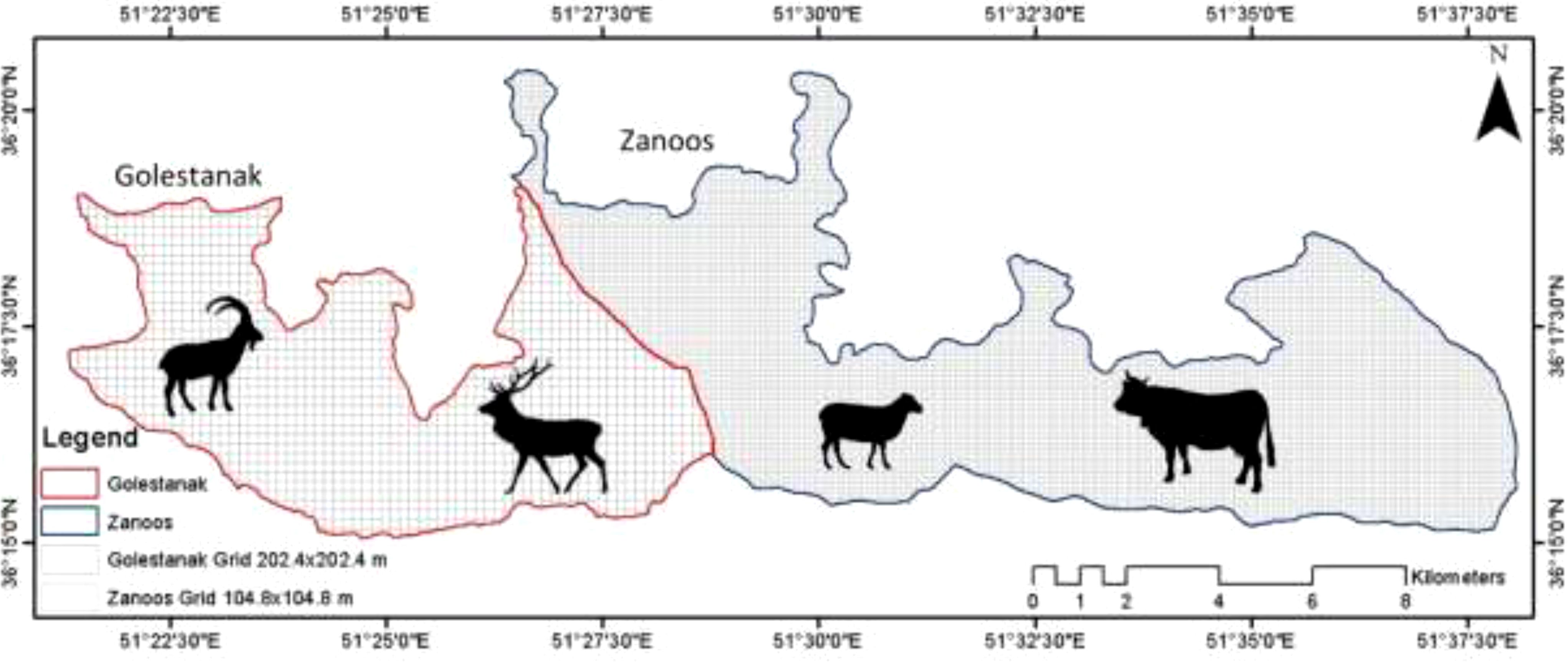
Figure 7. Amount of potential habitat for native artiodactyla in Golestanak compared to domesticated livestock in Zānoos.
4 Discussion
This study sheds valuable light on the complex interplay between climate warming and land-use practices, notably livestock grazing, in shaping vegetation dynamics in the Alborz Protected Area over the past 30 years (1994–2024). The significant upward NDVI trend observed in both study sites, Zānoos and Golestanak, underscores the role of increasing temperatures especially the pronounced June warming and seasonal precipitation in enhancing vegetation productivity in high-altitude ecosystems. However, the slower NDVI growth rate in Zānoos compared to Golestanak highlights the dampening effect of intensive livestock grazing (1–1.2 hectares per head) versus the lighter grazing pressure exerted by native artiodactyls in Golestanak (4.2–4 hectares per individual). This research is crucial, demonstrating that while climate change positively influences vegetation cover, high densities of domestic livestock may offset these gains, which has important implications for conservation and land management. The study provides an essential foundation for understanding how warming and grazing pressures interact, offering insights that could guide policy adjustments aimed at balancing biodiversity conservation with sustainable grazing practices under shifting climate regimes.
The results of this study provide compelling evidence of a significant upward trend in NDVI over the past three decades, with annual increases of 0.7–0.75% in Zānoos and 0.92–0.98% in Golestanak. These findings align with previous studies, including Piao et al. (2006), Pang et al. (2017), Xu et al. (2024), and Tuoku et al. (2024), which identify climate change as a primary driver of increasing NDVI. Furthermore, studies by Eskandari Dameneh et al. (2021) and Ghorbanian et al. (2022) confirm that the NDVI increases observed in Iran are closely linked to climate variability. Our results specifically highlight May and June temperature and precipitation as the most significant climate parameters influencing NDVI, consistent with findings from Kiapasha et al. (2017a, b), Eskandari Dameneh et al. (2021), Ghorbanian et al. (2022), and Zhang et al. (2024). While other studies have indicated that the relative importance of climatic factors can vary by region, with drier habitats showing stronger influences of rainfall on NDVI, our work reinforces the complexity of these interactions. This study underscores the importance of considering both long-term climate trends and short-term climatic fluctuations in understanding vegetation dynamics, particularly in climate-sensitive regions such as Iran, where ongoing climate change is likely to have profound impacts on ecosystem productivity and resilience.
Our results provide compelling evidence of the differential impacts of grazing by domestic livestock (cattle and sheep) compared to native artiodactyls (Caspian red deer and wild goats) on vegetation dynamics. Despite relatively similar ecological conditions in Zānoos and Golestanak, the response of NDVI to temperature increases exhibited a divergent pattern between the two areas. While Zānoos consistently displayed 0.3 higher NDVI values than Golestanak, the rate of NDVI increase in Golestanak over recent years was 30–32% greater than in Zānoos. This disparity is likely attributed to the higher grazing pressure in Zānoos, where domestic livestock densities are significantly higher (about 4 times denser than Golestanak). The negative effects of livestock grazing on vegetation cover have been well-documented in the literature, with studies by Ndungu et al. (2019), Balata et al. (2022), Li et al. (2022), and Velamazán et al. (2023) highlighting the degradation of plant communities under domestic grazing. These findings emphasize the urgent need for regulating grazing intensity, particularly in areas experiencing both grazing pressure and climate change, to protect ecosystem productivity. Our study underscores the critical importance of sustainable land-use practices to mitigate the combined effects of overgrazing and climate change on vegetation health and ecosystem resilience. Furthermore, our findings align with the results of Skidmore and Ferwerda (2008), Searle et al. (2015), Garroutte et al. (2016), Navarro et al. (2020), and Zhang et al. (2024), which demonstrate the positive influence of protected areas where livestock grazing is prohibited, and only native herbivores are present. This highlights that the distribution of herbivores, particularly native species, is strongly associated with resource availability and reinforces the importance of conservation strategies aimed at promoting native herbivore populations to enhance vegetation regeneration and maintain ecosystem stability.
This study provides compelling evidence for the critical role of effective grazing management in safeguarding Iran’s high-altitude ecosystems, which are increasingly important as climate refuges for vulnerable species (Martin et al., 2018; Peters et al., 2019; Wen et al., 2022; Sayahnia et al., 2024). With rising temperatures, these elevated habitats offer essential seasonal refuge for native large mammals allowing them to escape thermal stress in lower-altitude zones. Research by Millon et al. (2019), Kelt et al. (2019), and Cantor et al. (2021) similarly emphasizes the value of high-altitude habitats for supporting biodiversity under shifting climates, while studies by Teitelbaum and Mueller (2019), Shaw (2020), and Shizuka and Johnson (2020) illustrate how species mobility serves as an adaptive response to dynamic and fragmented environments.
Nevertheless, the encroachment of domestic livestock into these habitats presents a significant challenge, leading to vegetation degradation and reduced habitat availability for native herbivores (Soofi et al., 2018), which are then forced into limited protected areas like Golestanak. This concentration intensifies interspecies competition and risks overgrazing, potentially destabilizing the ecosystem’s delicate balance. In response, adaptive land management strategies that restrict livestock access to critical habitats are essential. Practical measures could include the acquisition of high-pressure grazing lands in areas such as Zānoos and the establishment of ranger stations to reinforce habitat protection. These actions would not only reduce livestock encroachment but also secure habitat corridors essential for the seasonal migrations of native species. Such targeted interventions are crucial for mitigating the compounded impacts of climate change and grazing pressure, ensuring the resilience and long-term sustainability of Iran’s unique biodiversity and high-altitude ecosystems.
5 Conclusion
This study provides crucial insights into the complex interactions between climate warming and grazing pressures in shaping vegetation dynamics within Iran’s Alborz Protected Area over the past three decades. Our findings demonstrate a significant increase in vegetation cover, driven primarily by temperature increases and seasonal rainfall, with particularly strong responses observed in high-altitude ecosystems. However, the positive effects of climate change on vegetation productivity are tempered by the higher grazing pressures exerted by domestic livestock, as seen in the Zānoos region. In contrast, the more sustainable grazing practices of native herbivores in Golestanak contribute to a stronger and more resilient vegetation response, highlighting the benefits of maintaining native herbivore populations in promoting ecosystem stability. These results underscore the urgent need for sustainable land-use and grazing management strategies, particularly in climate-sensitive regions, to protect ecosystem health and biodiversity. Effective management, including the regulation of grazing intensity and the protection of critical habitats, is essential for mitigating the adverse impacts of climate change and overgrazing. This research provides a foundation for policy adjustments that balance biodiversity conservation with sustainable land-use practices, ensuring the long-term resilience of Iran’s unique high-altitude ecosystems amidst the challenges posed by climate change.
Data availability statement
The original contributions presented in the study are included in the article/supplementary material. Further inquiries can be directed to the corresponding authors.
Author contributions
FS: Conceptualization, Data curation, Formal analysis, Investigation, Methodology, Project administration, Resources, Software, Supervision, Validation, Visualization, Writing – original draft, Writing – review & editing. ZS: Conceptualization, Data curation, Formal analysis, Investigation, Methodology, Project administration, Resources, Software, Validation, Visualization, Writing – original draft, Writing – review & editing. MKe: Software, Writing – review & editing. MKi: Data curation, Writing – review & editing. RS: Software, Writing – review & editing.
Funding
The author(s) declare that no financial support was received for the research, authorship, and/or publication of this article.
Conflict of interest
The authors declare that the research was conducted in the absence of any commercial or financial relationships that could be construed as a potential conflict of interest.
Generative AI statement
The author(s) declare that no Generative AI was used in the creation of this manuscript.
Publisher’s note
All claims expressed in this article are solely those of the authors and do not necessarily represent those of their affiliated organizations, or those of the publisher, the editors and the reviewers. Any product that may be evaluated in this article, or claim that may be made by its manufacturer, is not guaranteed or endorsed by the publisher.
References
Abebe B., Ashagrie M., Eshete G. (2024). Effects of livestock grazing on herbaceous species composition and biomass in the Simien Mountains National Park, Ethiopia. Israel J. Ecol. Evol. 1, 1–0. doi: 10.1163/22244662-bja10087
Balata D., Gama I., Domingos T., Proença V. (2022). Using satellite NDVI time-series to monitor grazing effects on vegetation productivity and phenology in heterogeneous Mediterranean forests. Remote Sens. 14, 2322. doi: 10.3390/rs14102322
Blanco-Sepúlveda R., Gómez-Moreno M. L., Lima F. (2024). An approach to the key soil physical properties for assessing soil compaction due to livestock grazing in mediterranean mountain areas. Sustainability 16, 4279. doi: 10.3390/su16104279
Cantor M., Maldonado-Chaparro A. A., Beck K. B., Brandl H. B., Carter G. G., He P., et al. (2021). The importance of individual-to-society feedbacks in animal ecology and evolution. J. Anim. Ecol. 90, 27–44. doi: 10.1111/1365-2656.13336
Chen M., Zhao X. Y., Yue P., Guo X. X., Qiao J. J., Li X. Y. (2022). Effect of grazing disturbance on floral display, pollen limitation and plant pollination efficiency in the desert steppe. BMC Plant Biol. 22, 514. doi: 10.1186/s12870-022-03899-w
Crovo O., Aburto F., da Costa-Reidel C., Montecino F., Rodríguez R. (2021). Effects of livestock grazing on soil health and recovery of a degraded Andean Araucaria forest. Land Degradation Dev. 32, 4907–4919. doi: 10.1002/ldr.v32.17
Draper J. P., Young J. K., Schupp E. W., Beckman N. G., Atwood T. B. (2022). Frugivory and seed dispersal by carnivorans. Front. Ecol. Evol. 10, 864864. doi: 10.3389/fevo.2022.864864
Eskandari Dameneh H., Gholami H., Telfer M. W., Comino J. R., Collins A. L., Jansen J. D. (2021). Desertification of Iran in the early twenty-first century: assessment using climate and vegetation indices. Sci. Rep. 11, 548. doi: 10.1038/s41598-021-99636-8
Estrada-Villegas S., Stevenson P. R., López O., DeWalt S. J., Comita L. S., Dent D. H. (2023). Animal seed dispersal recovery during passive restoration in a forested landscape. Philos. Trans. R. Soc. B 378, 20210076. doi: 10.1098/rstb.2021.0076
Filazzola A., Brown C., Dettlaff M. A., Batbaatar A., Grenke J., Bao T., et al. (2020). The effects of livestock grazing on biodiversity are multi-trophic: a meta-analysis. Ecol. Lett. 23, 1298–1309. doi: 10.1111/ele.13527
Garroutte E. L., Hansen A. J., Lawrence R. L. (2016). Using NDVI and EVI to map spatiotemporal variation in the biomass and quality of forage for migratory elk in the Greater Yellowstone Ecosystem. Remote Sens. 8, 404. doi: 10.3390/rs8050404
Gelmi-Candusso T. A., Hämäläinen A. M. (2019). Seeds and the city: the interdependence of zoochory and ecosystem dynamics in urban environments. Front. Ecol. Evol. 7, 41. doi: 10.3389/fevo.2019.00041
Ghasemi A., Hemami M. R., Karimi S., Iravani M., Senn J. (2024). Potential seed dispersal by persian wild ass in south central Iran. Rangeland Ecol. Manage. 92, 73–79. doi: 10.1016/j.rama.2023.09.007
Ghorbanian A., Mohammadzadeh A., Jamali S. (2022). Linear and non-linear vegetation trend analysis throughout Iran using two decades of MODIS NDVI imagery. Remote Sens. 14, 3683. doi: 10.3390/rs14153683
Gonzalez S. L., Ghermandi L. (2021). Overgrazing causes a reduction in the vegetation cover and seed bank of Patagonian grasslands. Plant Soil 464, 75–87. doi: 10.1007/s11104-021-04931-y
Hojjati S. M., Darzi A., Asadi H., Tafazoli M. (2021). Changes in soil properties and plant biodiversity after 12 years of rehabilitating livestock farms in the Hyrcanian Forests. Agroforestry Syst. 95, 1493–1503. doi: 10.1007/s10457-021-00658-y
Hossain M. L., Li J. (2021). NDVI-based vegetation dynamics and its resistance and resilience to different intensities of climatic events. Global Ecol. Conserv. 30, e01768. doi: 10.1016/j.gecco.2021.e01768
Jones C. S., Duncan D. H., Rumpff L., Robinson D., Vesk P. A. (2022). Permanent removal of livestock grazing in riparian systems benefits native vegetation. Global Ecol. Conserv. 33, e01959. doi: 10.1016/j.gecco.2021.e01959
Karami M., Ghadirian T., Faizolahi K. (2016). The atlas of mammals of Iran. Jahad daneshgahi, kharazmi Branch.
Karimi S., Hemami M. R., Esfahani M. T., Akhani H., Baltzinger C. (2018). Complementary endozoochorous seed dispersal by large mammals in the Golestan National Park, Iran. Seed Sci. Res. 28, 294–302. doi: 10.1017/S0960258518000351
Kelt D. A., Heske E. J., Lambin X., Oli M. K., Orrock J. L., Ozgul A., et al. (2019). Advances in population ecology and species interactions in mammals. J. Mammalogy 100, 965–1007. doi: 10.1093/jmammal/gyz017
Kiapasha K., Darvishsefat A. A., Julien Y., Sobrino J. A., Zargham N., Attarod P., et al. (2017b). Trends in phenological parameters and relationship between land surface phenology and climate data in the Hyrcanian Forests of Iran. IEEE J. Selected Topics Appl. Earth Observations Remote Sens. 10, 4961–4970. doi: 10.1109/JSTARS.4609443
Kiapasha K., Darvishsefat A., Zargham N., Attarod P., Nadi M., Schaepman M. (2017a). Greening trend in the Hyrcanian forests using NOAA NADVI time series during 1981-2012. For. Wood Products 70, 09–420. doi: 10.1109/JSTARS.2017.2736938
Kral-O’Brien K. C., Roberton B., Duquette C. A., Hovick T. J., Harmon J. P. (2023). A mechanistic framework for studying indirect effects of large vertebrate herbivores on pollinators. Arthropod-Plant Interact. 17, 263–274. doi: 10.1007/s11829-023-09964-x
Leclerc C., Villéger S., Marino C., Bellard C. (2020). Global changes threaten functional and taxonomic diversity of insular species worldwide. Diversity Distributions 26, 402–414. doi: 10.1111/ddi.13024
Li Y., Gong J., Zhang Y., Gao B. (2022). NDVI-based greening of alpine steppe and its relationships with climatic change and grazing intensity in the Southwestern Tibetan Plateau. Land 11, 975. doi: 10.3390/land11070975
Ma Q., Zhu Y., Wang Y., Liu T., Qing X., Liu J., et al. (2024). Livestock grazing modifies soil nematode body size structure in mosaic grassland habitats. J. Environ. Manage. 351, 119600. doi: 10.1016/j.jenvman.2023.119600
Macheroum A., Chenchouni H. (2022). Short-term land degradation driven by livestock grazing does not affect soil properties in semiarid steppe rangelands. Front. Environ. Sci. 10, 846045. doi: 10.3389/fenvs.2022.846045
Martin J., Tolon V., Morellet N., Santin-Janin H., Licoppe A., Fischer C., et al. (2018). Common drivers of seasonal movements on the migration–residency behavior continuum in a large herbivore. Sci. Rep. 8, 7631. doi: 10.1038/s41598-018-25777-y
May J. L., Hollister R. D., Betway K. R., Harris J. A., Tweedie C. E., Welker J. M., et al. (2020). NDVI changes show warming increases the length of the green season at Tundra communities in Northern Alaska: a fine-scale analysis. Front. Plant Sci. 11, 1174. doi: 10.3389/fpls.2020.01174
Millon A., Lambin X., Devillard S., Schaub M. (2019). Quantifying the contribution of immigration to population dynamics: A review of methods, evidence and perspectives in birds and mammals. Biol. Rev. 94, 2049–2067. doi: 10.1111/brv.12549
Mushinski R. M., Zhou Y., Hyodo A., Casola C., Boutton T. W. (2024). Interactions of long-term grazing and woody encroachment can shift soil biogeochemistry and microbiomes in savanna ecosystems. Geoderma 441, 116733. doi: 10.1016/j.geoderma.2023.116733
Narantsetseg A., Kang S., Ko D. (2018). Livestock grazing and trampling effects on plant functional composition at three wells in the desert steppe of Mongolia. J. Ecol. Environ. 42, 1–8. doi: 10.1186/s41610-018-0075-2
Navarro C. J., Izquierdo A. E., Aráoz E., Foguet J., Grau H. R. (2020). Rewilding of large herbivore communities in high elevation Puna: geographic segregation and no evidence of positive effects on peatland productivity. Regional Environ. Change 20, 1–11. doi: 10.1007/s10113-020-01704-8
Ndungu L., Oware M., Omondi S., Wahome A., Mugo R., Adams E. (2019). Application of MODIS NDVI for monitoring Kenyan rangelands through a web based decision support tool. Front. Environ. Sci. 7, 475659. doi: 10.3389/fenvs.2019.00187
Nezami B., Farhadinia M. S. (2011). Litter sizes of brown bears in the central alborz protected area, Iran. Bio One 22 (2), 167–171. doi: 10.2192/URSUS-D-10-00026.1
Nezami B., Rahmani M., Faghihi H., Shams-Esfandabad B. (2024). Habitat selection and identification of potential breeding habitats of red deer cervus elaphus maral in mazandaran province. Environ. Interdiscip. Dev. 8, 108–121. doi: 10.22034/envj.2024.450806.1362
Nield A. P., Nathan R., Enright N. J., Ladd P. G., Perry G. L. (2020). The spatial complexity of seed movement: animal-generated seed dispersal patterns in fragmented landscapes revealed by animal movement models. J. Ecol. 108, 687–701. doi: 10.1111/1365-2745.13287
Noroozi J., Naqinezhad A., Talebi A., Doostmohammadi M., Plutzar C., Rumpf S. B., et al. (2019). Hotspots of vascular plant endemism in a global biodiversity hotspot in Southwest Asia suffer from significant conservation gaps. Biol. Conserv. 237, 299–307. doi: 10.1016/j.biocon.2019.07.005
Noroozi J., Talebi A., Doostmohammadi M. (2020). The alborz mountain range. Plant Biogeography Vegetation High Mountains Cent. South-West Asia, 117–149. doi: 10.1007/978-3-030-45212-4
Öllerer K., Varga A., Kirby K., Demeter L., Biró M., Bölöni J., et al. (2019). Beyond the obvious impact of domestic livestock grazing on temperate forest vegetation–A global review. Biol. Conserv. 237, 209–219. doi: 10.1016/j.biocon.2019.07.007
Oñatibia G. R., Amengual G., Boyero L., Aguiar M. R. (2020). Aridity exacerbates grazing-induced rangeland degradation: A population approach for dominant grasses. J. Appl. Ecol. 57, 1999–2009. doi: 10.1111/1365-2664.13704
Pang G., Wang X., Yang M. (2017). Using the NDVI to identify variations in, and responses of, vegetation to climate change on the Tibetan Plateau from 1982 to 2012. Quaternary Int. 444, 87–96. doi: 10.1016/j.quaint.2016.08.038
Pearson D. E., Ortega Y. K., Cimino H. E., Mummy D. L., Ramsey P. W. (2022). Does active plant restoration passively restore native fauna community structure and function? Restor. Ecol. 30, e3481. doi: 10.1111/rec.13481
Pelliza Y. I., Fernandez A., Saiz H., Tadey M. (2021). Together we stand, divided we fall: Effects of livestock grazing on vegetation patches in a desert community. J. Vegetation Sci. 32, e13015. doi: 10.1111/jvs.13015
Peters W., Hebblewhite M., Mysterud A., Eacker D., Hewison A. M., Linnell J. D., et al. (2019). Large herbivore migration plasticity along environmental gradients in Europe: life-history traits modulate forage effects. Oikos 128, 416–429. doi: 10.1111/oik.2019.v128.i3
Piao S., Mohammat A., Fang J., Cai Q., Feng J. (2006). NDVI-based increase in growth of temperate grasslands and its responses to climate changes in China. Global Environ. Change 16, 340–348. doi: 10.1016/j.gloenvcha.2006.02.002
Pires M. M. (2024). The restructuring of ecological networks by the pleistocene extinction. Annu. Rev. Earth Planetary Sci. 52. doi: 10.1146/annurev-earth-040722-104845
Pozo R. A., Cusack J. J., Acebes P., Malo J. E., Traba J., Iranzo E. C., et al. (2021). Reconciling livestock production and wild herbivore conservation: challenges and opportunities. Trends Ecol. Evol. 36, 750–761. doi: 10.1016/j.tree.2021.05.002
Pulido M., Schnabel S., Lavado Contador J. F., Lozano-Parra J., González F. (2018). The impact of heavy grazing on soil quality and pasture production in rangelands of SW Spain. Land Degradation Dev. 29, 219–230. doi: 10.1002/ldr.v29.2
Salmanpour F., Ahmadzadeh F., Sayyahnia R., Hasanzade Kiabi B. (2022). The effect of climate change on the distribution of Caspian red deer (Cervus elaphus maral) in Central Alborz Protected Area. Environ. Sci. 20, 229–242. doi: 10.48308/envs.2023.1260
Salmanpour F., Shakoori Z., Kia M., Eshaghi R., Ghaderi M., Ghomi S., et al. (2023). Mineral lick use by a community of large herbivores in northern Iran. Ecol. Evol. 13, e9731. doi: 10.1002/ece3.v13.1
Salmanpour F., Shakoori Z., Kia M., Ghomi S. (2021). Investigating the factors affecting leopard (Panthera pardus tulliana) conflict with domestic livestock compared to other large carnivores in Nowshahr Hyrcanian forests. Anim. Environ. J. 13, 1–8. doi: 10.22034/aej.2020.135105
Salmanpour F., Shakoori Z., Salmanpour M., Tizrouyan M., Kia M., Eshaghi R., et al. (2024). The effect of ranger patrols on the sighting of large mammals by local herders in northern Iran. Heliyon 11 (1), e41452. doi: 10.1016/j.heliyon.2024.e41452
Savari M. (2023). Explaining the ranchers’ behavior of rangeland conservation in western Iran. Front. Psychol. 13, 1090723. doi: 10.3389/fpsyg.2022.1090723
Sayahnia R., Ommi S., Khoshnamvand H., Salmanpour F., Sadeghi S. M. M., Ahmadzadeh F. (2024). Fire protection priorities in the oak forests of Iran with an emphasis on vertebrate habitat preservation. Sci. Rep. 14, 15624. doi: 10.1038/s41598-024-65355-z
Searle K. R., Rice M. B., Anderson C. R., Bishop C., Hobbs N. T. (2015). Asynchronous vegetation phenology enhances winter body condition of a large mobile herbivore. Oecologia 179, 377–391. doi: 10.1007/s00442-015-3348-9
Shakeri Z., Simberloff D., Bernhardt-Römermann M., Eckstein R. L. (2021). The impact of livestock grazing and canopy gaps on species pool and functional diversity of ground flora in the Caspian beech forests of Iran. Appl. Vegetation Sci. 24, e12592. doi: 10.1111/avsc.12592
Shakoori Z., Salmanpour F. (2024). Nutritional position of managed honey bees during pollination of native plants by the melissopalynology method. Sci. Rep. 14, 21563. doi: 10.1038/s41598-024-73086-4
Shaw A. K. (2020). Causes and consequences of individual variation in animal movement. Movement Ecol. 8, 12. doi: 10.1186/s40462-020-0197-x
Shizuka D., Johnson A. E. (2020). How demographic processes shape animal social networks. Behav. Ecol. 31, 1–11. doi: 10.1093/beheco/arz083
Skidmore A. K., Ferwerda J. G. (2008). “Resource distribution and dynamics: mapping herbivore resources,” in Resource ecology: spatial and temporal dynamics of foraging (Springer Netherlands), 57–77.
Song Y., Xu M., Xu T., Zhao X., Yue Y., Yu H., et al. (2023). Changes in plant community assembly from patchy degradation of grasslands and grazing by different-sized herbivores. Ecol. Appl. 33, e2803. doi: 10.1002/eap.v33.2
Soofi M., Ghoddousi A., Zeppenfeld T., Shokri S., Soufi M., Jafari A., et al. (2018). Livestock grazing in protected areas and its effects on large mammals in the Hyrcanian forest, Iran. Biol. Conserv. 217, 377–382. doi: 10.1016/j.biocon.2017.11.020
Stevenson E. (2023). The Beef and the Bees: How Livestock Grazing Affects Pollinator Feeding and Nesting Resources Over time. Northern Arizona University. Master's thesis.
Teitelbaum C. S., Mueller T. (2019). Beyond migration: causes and consequences of nomadic animal movements. Trends Ecol. Evol. 34, 569–581. doi: 10.1016/j.tree.2019.02.005
Thapa-Magar K. B., Davis T. S., Kondratieff B. (2020). Livestock grazing is associated with seasonal reduction in pollinator biodiversity and functional dispersion but cheatgrass invasion is not: Variation in bee assemblages in a multi-use shortgrass prairie. PloS One 15, e0237484. doi: 10.1371/journal.pone.0237484
Tian C., Liao P. C., Dayananda B., Zhang Y. Y., Liu Z. X., Li J. Q., et al. (2019). Impacts of livestock grazing, topography and vegetation on distribution of wildlife in Wanglang National Nature Reserve, China. Global Ecol. Conserv. 20, e00726. doi: 10.1016/j.gecco.2019.e00726
Tuoku L., Wu Z., Men B. (2024). Impacts of climate factors and human activities on NDVI change in China. Ecol. Inf. 81, 102555. doi: 10.1016/j.ecoinf.2024.102555
Ugarte C. S., Moreira-Arce D., Simonetti J. A. (2019). Ecological attributes of carnivore-livestock conflict. Front. Ecol. Evol. 7, 433. doi: 10.3389/fevo.2019.00433
Velamazán M., Sánchez-Zapata J. A., Moral-Herrero R., Jacquemin E. G., Sáez-Tovar J. A., Barbosa J. M. (2023). Contrasting effects of wild and domestic ungulates on fine-scale responses of vegetation to climate and herbivory. Landscape Ecol. 38, 3463–3478. doi: 10.1007/s10980-023-01676-0
Wei Y., Lu H., Wang J., Wang X., Sun J. (2022). Dual influence of climate change and anthropogenic activities on the spatiotemporal vegetation dynamics over the Qinghai-Tibetan plateau from 1981 to 2015. Earth's Future 10, e2021EF002566. doi: 10.1029/2021EF002566
Wen Z., Feijó A., Ke J., He X., Cheng J., Ge D., et al. (2022). Altitudinal dispersal process drives community assembly of montane small mammals. Ecography 2022, e06318. doi: 10.1111/ecog.v2022.i9
Wilkinson C. E., McInturff A., Miller J. R., Yovovich V., Gaynor K. M., Calhoun K., et al. (2020). An ecological framework for contextualizing carnivore–livestock conflict. Conserv. Biol. 34, 854–867. doi: 10.1111/cobi.13469
Xu Y., Dai Q. Y., Lu Y. G., Zhao C., Huang W. T., Xu M., et al. (2024). Identification of ecologically sensitive zones affected by climate change and anthropogenic activities in Southwest China through a NDVI-based spatial-temporal model. Ecol. Indic. 158, 111482. doi: 10.1016/j.ecolind.2023.111482
Xu H., You C., Tan B., Xu L., Liu Y., Wang M., et al. (2023). Effects of livestock grazing on the relationships between soil microbial community and soil carbon in grassland ecosystems. Sci. Total Environ. 881, 163416. doi: 10.1016/j.scitotenv.2023.163416
Yan J., Zhang G., Ling H., Han F. (2022). Comparison of time-integrated NDVI and annual maximum NDVI for assessing grassland dynamics. Ecol. Indic. 136, 108611. doi: 10.1016/j.ecolind.2022.108611
Zandebasiri M., Azadi H., Shirmardi H., Jahanbazi Goujani H., Iranmanesh Y., Shamsoddini S., et al. (2023). The economic valuation of ecosystem services: economic value-based management in a case study of protected areas in Iran. Int. J. Environ. Res. 17, 53. doi: 10.1007/s41742-023-00536-8
Keywords: ecosystem resilience, restoration, biodiversity conservation, ecosystem management, temperature, rainfall, plant ecology
Citation: Salmanpour F, Shakoori Z, Keshtkar M, Kia M and Sayahnia R (2025) Impact of climate warming on vegetation cover: positive effects of native artiodactyla vs. grazing pressure in Alborz Protected Area. Front. Conserv. Sci. 6:1534034. doi: 10.3389/fcosc.2025.1534034
Received: 25 November 2024; Accepted: 09 January 2025;
Published: 28 January 2025.
Edited by:
Xuhui Dong, Guangzhou University, ChinaReviewed by:
Jing Yang, Chinese Academy of Sciences (CAS), ChinaEmin Ugurlu, Bursa Technical University, Türkiye
Copyright © 2025 Salmanpour, Shakoori, Keshtkar, Kia and Sayahnia. This is an open-access article distributed under the terms of the Creative Commons Attribution License (CC BY). The use, distribution or reproduction in other forums is permitted, provided the original author(s) and the copyright owner(s) are credited and that the original publication in this journal is cited, in accordance with accepted academic practice. No use, distribution or reproduction is permitted which does not comply with these terms.
*Correspondence: Farid Salmanpour, ZmEuc2FsbWFucG91ckBnbWFpbC5jb20=; Romina Sayahnia, cl9zYXlhaG5pYUBzYnUuYWMuaXI=
†ORCID: Farid Salmanpour, orcid.org/0000-0002-1517-9061
Zahra Shakoori, orcid.org/0000-0003-3057-8981
Romina Sayahnia, orcid.org/0000-0001-7844-3758