- MIT Media Lab, Massachusetts Institute of Technology, Cambridge, MA, United States
Technologies on the body that require explicit awareness to be operated or monitored often risk disrupting human awareness and induce stress and excessive cognitive load. With the increasing interest in body-centric technologies, it is thus essential to understand how to build technologies that interface with human awareness without disrupting or requiring too many cognitive resources. In this paper, we build and evaluate a wearable system that uses different feedback types to alter human awareness (of the device). We further demonstrate how this awareness impacts cognitive load, sense of body-ownership, and sense of agency, which are often essential antecedents to successful and continued use. Moreover, we further investigate physiological signals, such as physiological synchrony, as well as qualitative reports in a multimodal analysis. Our results show that devices that provide feedback that deviate from expected behavior tend to generate higher amounts of explicit awareness, and that such increased awareness correlates with increased cognitive load, lower sense of agency and lower sense of body-ownership. Moreover, we find that interoceptive acuity correlates with diminished sense of agency. We discuss their implications for designing wearable body-centric systems that induce or disrupt different levels of awareness to deliver or diminish a sense of body-ownership and agency over the system.
1 Introduction
Wearable technologies have emerged as an essential interface between humans and computational augmentation, offering utility in fields ranging from health and wellness to entertainment (Park and Jayaraman, 2003; Mueller et al., 2020). However, despite rapid advancements, a significant barrier to long-term adoption exists: a large percentage of users discontinue the use of these wearables over time. For instance, as many as 40% of individuals fitted with a prosthesis either rarely use it or abandon it altogether (Biddiss and Chau, 2007; Brandebusemeyer et al., 2021). Preliminary evidence suggests that one key reason for this attrition is the increased cognitive load and explicit device awareness imposed by these devices (Slater et al., 2003; Murray, 2004; Li et al., 2019; Danry et al., 2022) such as increased attention, concentrates or focalization of the devices in our immediate experience (see Figure 1). However, it is still unclear what factors in wearable devices generate this cognitive load and explicit awareness, as well as how wearable devices can operate to minimize such influences cognitive load and awareness.
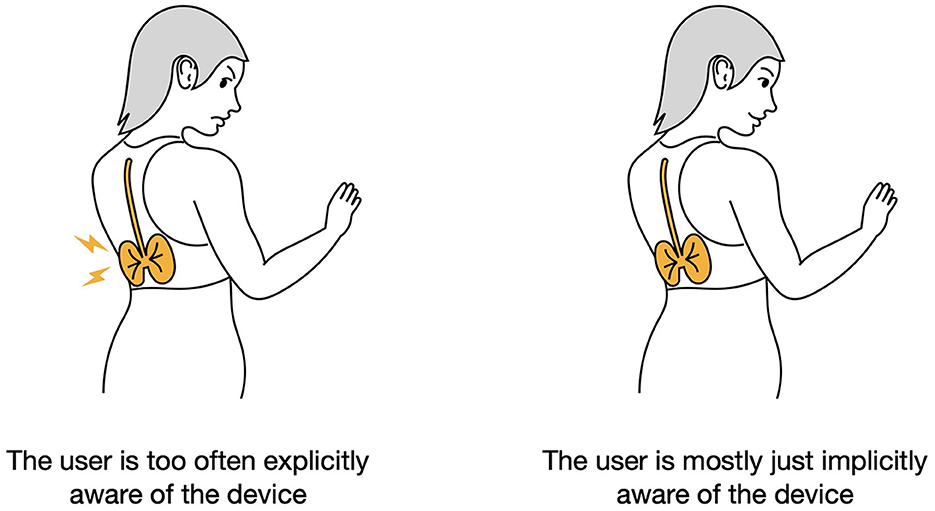
Figure 1. Wearable devices will have to consider their influence on user awareness (of the device) to facilitate long-term integration, adoption and satisfactory use.
Recent studies have focused on sense of agency and sense of body ownership as predictors for successful use where the actions performed with a system are either attributed with a feeling of “I did that” instead of “the device did that” (Kasahara et al., 2019; Cornelio et al., 2022) (Sense of Agency) or a feeling like the device has become part of the user; “I did that with my body” rather than “I did that with the device” (Sense of Body Ownership) (Lopes et al., 2021; Danry et al., 2022). There is evidence that suggests that wearable devices that require or cause more frequent explicit awareness lead to lower adoption due to a diminished sense of agency and sense of device-body ownership (Murray, 2004). Some prior work has hinted at sudden changes in device parameters such as an abrupt increase in simulated heart rate to generate explicit awareness (Morris et al., 2022). However, the existing literature remains mostly anecdotal, and has not quantitatively investigated how these variations contribute to unintentional explicit awareness of wearable devices. Moreover, the subsequent effects of such awareness on both sense of agency and sense of device body-ownership remain inadequately explored.
It is also unclear if physiological markers can act as predictors of explicit awareness, sense of agency, sense of body ownership or other phenomenological states. For instance, there has lately been an increased interest around understanding phenomenological states through their correlating bodily states (Damasio, 1994; Clore and Ortony, 2008). Interoception, or the awareness of internal bodily states, has been shown to play a crucial role in emotional experiences and cognitive decision-making (Craig, 2009; Barrett, 2017). For instance, interoceptive cues such as heart rate and respiratory rate has been shown to significantly impact appraised emotions and cognitive states such as “fear” (Schreuder et al., 2016; Schoeller et al., 2019) which has been shown as not the cause but, rather, the result of physiological changes such as increased heart rate or rapid breathing.
Existing literature has identified a significant correlation between increased empathy levels, as measured by the Interpersonal Reactivity Index (IRI) (Davis, 1983), and heightened interoceptive acuity—the ability to sense and interpret internal bodily sensations (Seifert, 2018). Given this established connection, individuals with higher IRI scores might more frequently become explicitly aware of a wearable device creating or influencing bodily sensations due to such heightened acuity. This elevated awareness is likely to inversely correlate with feelings of device body-ownership, such that people who are more aware of the device may feel less like it is an integrated part of their body. This understanding has implications for the design and application of wearable technologies across a natural variance of people, particularly in contexts that require seamless human-device interaction.
In this paper, we aim to contribute insights for the development of attention-free wearable technologies that are both effective and user-friendly, without causing disruption, to serve as a foundation for future bodily integrated or worn systems.
In particular, our primary research goals are the following:
1. To understand how variation in wearable device parameters may affect explicit awareness and cognitive load.
2. To investigate the relationship between explicit device awareness and the user's sense of agency and body ownership.
3. To explore the potential of physiological signals, such as physiological synchrony, as predictors for explicit awareness, sense of agency, sense of body ownership and other phenomenological states.
4. To understand the impact of user's interoceptive acuity, as measured by increased empathy levels, on the explicit awareness of wearable device and its effects on device-body ownership.
5. To generate insights that can guide the design of wearables to better incorporate integration with the human body, minimize disruption and foster long term usage.
In order to achieve these goals, we uses both qualitative and quantitative methods to collect and analyze data from participants using a wearable breath simulation device.
2 Experiential factors of wearable systems
2.1 Bodily awareness
The human consciousness is often categorized into two principal modes of awareness: explicit (reflective) and implicit (pre-reflective). Explicit awareness enables detailed attention and cognitive control over the tasks we consciously direct our focus toward, generating rich experiences (Damasio, 1999; Baars, 2005; Legrand, 2007; Breedlove and Watson, 2018; Gallagher and Zahavi, 2020). Often used in problem-solving, judgment-making and learning, this mode of consciousness enhances the precision of sensory inputs and aids in allocating cognitive resources. On the other hand, implicit awareness essentially constitutes a lower attention, automatic functioning of our bodies and thoughts, which do not demand constant attention or explicit control (Dourish, 2001; Gallagher and Zahavi, 2020). This implicit mode of awareness enables us to delegate less complex tasks to our body with only minimal attention while reflectively focusing on other things, facilitating multitasking. These two types of consciousness dynamically interact, allowing us to bring implicit senses into explicit awareness, a capability which aids in monitoring the body's automatic processes and resolving ambiguities in gists of sensory information (Gallagher and Zahavi, 2019).
2.2 Sense of device body-ownership
Sense of body-ownership is a minimal sense of awareness that the body or body part moving or the thoughts formed in one's mind are one's own (and not anyone else's) (Damasio, 1999; Gallagher and Zahavi, 2020). Studies have shown that this sense can be extended beyond our biological bodies to artificial limbs or tools due to the plasticity of our brain (Botvinick and Cohen, 1998; Lewis and Lloyd, 2010; Ponzo et al., 2018). To distinguish this notion from sense of body-ownership over one's biological limbs, we refer to this phenomenon as the “sense of device body-ownership,” where one develops a feeling of ownership toward an external device when it is sensed through multisensory correlation and fit within the user's body-model (Tsakiris et al., 2007; Danry et al., 2022). For humans, the sense of body-ownership can sometimes be disrupted in certain neurological or psychiatric conditions, where distortions in the sense of body-ownership have been linked to disorders like schizophrenia and depersonalization (Sass and Parnas, 2003; Sass, 2014). Similarly, some integration systems like brain-computer interfaces have also been shown to distort the sense of self of some users (Gilbert et al., 2017; Gilbert, 2018). For prosthetic users, the sense of body-ownership often equates to sensations being felt on the prosthesis. For instance, prosthetic users often speak of being able to “feel my [prosthetic] heel land,” feel “the [prosthetic] foot move forward,” or feel the “things that come into contact with it [the prosthesis]” but that these feelings are minimal and that the users do not pay much attention to them when using the prosthetic as it has become pre-reflective (Murray, 2004). Being able to elicit a sense of body-ownership of an integration would allow researchers to create technology that is non-disruptive and feels like a part of the user. Research has shown that in order to generate a sense of body-ownership, technology must be able to be sensed through multisensory correlation and fit within the user's body-model (Tsakiris et al., 2007; Danry et al., 2022). There is also some evidence that a diminished sense of agency decreases sense of body-ownership (Tsakiris et al., 2007).
2.3 Eliciting a sense of agency
The sense of agency is the minimal awareness or sense that I am the one causing the action of an object (Tsakiris et al., 2007). The sense of agency is perhaps the most common minimal sense that we have of objects: when skillfully eating with a fork, driving a car or using a tool, we do not need to think about how we are using them—they just do what we intend them to—and we only become explicitly aware of them when they hinder our intentions. Similarly, in the literature, we find that a sense of agency correlates with feeling that intended actions are done “intuitively,” without the continuous explicit monitoring and “awareness of [the integration]” (Murray, 2004). As one prosthetic wearer says: “[when using my prosthetic] it's pretty much a matter of well I want to go from here to there. I just walk” (Murray, 2004). Additionally, losing a sense of agency has also been shown to diminish senses of body-ownership. Research on the rubber hand illusion shows that after a sense of body-ownership is elicited, moving the rubber hand involuntarily (while the participant's real hand does not move) disrupts sense of agency and leads to a diffused or weak sense of body-ownership over the rubber hand (Tsakiris et al., 2007; Lewis and Lloyd, 2010; De Vignemont, 2011). For designers to effectively elicit a sense of agency in their designs, research shows that congruences between intended sensory outcomes (e.g., expecting a sensation of moving my robot arm to the right) and real sensory outcomes (e.g., feeling the sensation of moving my robot arm to the right) are essential (Tsakiris et al., 2007). For instance, I expect that moving an integration in a specific way will lead to a certain proprioceptive, tactile, or visual sensation (e.g., seeing that the integration has moved from A to B), and not having these sensory outcomes removes my sense of agency. We all know this from when our devices or even our body breaks down and we feel that we can no longer control them as skillfully as we expect. Further, not only must the outcome (e.g., movement) closely match the intention, but the match should also not happen after around 200 ms of initiation (Kasahara et al., 2019).
2.4 Physiological synchrony
Physiological synchrony is the matching in time of bodily rhythms and patterns, from movement of the body (e.g., walking in step) to the matching of internal processes such as heart rate and breathing rate. Synchrony is associated with self-other overlap in neural representation, and is therefore associated with feelings of affiliation toward another person or object with synchronous, time-matched behaviors (Hove and Risen, 2009). Physical co-presence can also cause a contagion effect of synchrony without direct interaction during a shared activity, suggesting that the mere presence of multiple individuals together can allow for the sharing of physiological information such as heart rate and electrodermal activity (Golland et al., 2015). This effect has been used to generate device-person synchrony, inducing individuals to unconsciously match their physiological states to the rhythm of a device, for purposes of altering one's affective state (e.g., to encourage calmness or focus) (Ghandeharioun and Picard, 2017; Frey et al., 2018). As the occurrence of synchrony correlates with strength of relationship affiliation or neural self-other overlap, induced device-person synchrony can provide information about the degree of one's sense of device-body ownership at a physiological level (Bizzego et al., 2019). In this work, we employ the term synchrony to refer to whether a participant's physiological states (heart rate, heart rate variability, breathing) are influenced to match the control parameters of the device (i.e., show parallel increases or decreases), indicating a sense of self-other overlap.
3 System design
3.1 Software
To achieve these objectives, we developed and assessed a wearable system that employs various simulated breathing characteristics to modulate human awareness of the device (Figure 2). The interactive component of the study was orchestrated through custom state machine software, which acted as a comprehensive input-output controller. This software facilitated hardware and pneumatic device calibration, manipulation of timed interval settings, administration of the screen-based Trail Making Test, logging of participant awareness, synchronization of Lab Streaming Layer (LSL) markers with the on-body physiological sensor, and data capture.
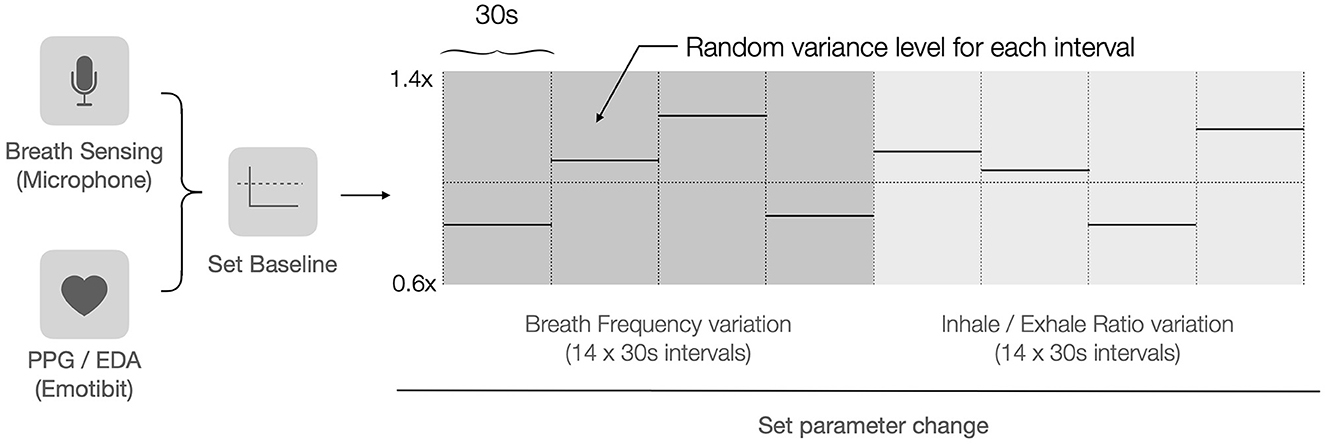
Figure 2. Overview of system design for simulated breath frequency and inhale/exhale ratio levels. Emotibit and microphone sensor inputs are synced with control software to establish a baseline respiration rate for each participant, setting a normalized baseline for randomly generated variance steps. In the first condition, breath frequency is varied randomly at 30-s intervals within limits of absolute value and maximum change (20%) per interval step. In the second condition, inflate-deflate ratio is varied randomly at 30-s intervals within the same constraints.
3.2 Pneumatic apparatus and setup
To test our hypotheses about low-attention-level on-body sensations, we engineered a custom garment device featuring soft pneumatics, designed to simulate a subtle, non-intrusive breathing sensation. The device was designed and fabricated in the style of a typical clothing garment to improve perceptual adoption and wearability, as compared to devices which feel inherently foreign or medical. Within the adjustable vest garment, interior chambers contain subdivided pneumatic pouches and an air tubing exit port to connect to the control electronics. Nylon webbing at the sides and shoulders of the vest can be changed in length via strap adjusters to accommodate a wide range of body sizes and seated positions. The inflatable pouches are strategically located at the front diaphragm area, just beneath the ribcage, aligning with the region where individuals commonly perceive belly breathing to occur on the body's surface.
The inflatable pouches are made of PVC vinyl, a soft fabric-like material, which is divided into air chambers using a heat sealing method, allowing for a more distributed inflation with less air volume exchange. The pneumatic interface is controlled by two 5V motors, one operating as a pump for inflation and one as a vacuum for deflation, mediated by a pneumatic valve relay. The motors are driven by an nRF52840 Cortex M4 processor with individual drivers for control of the pump, vacuum, and relay (Figure 3). The electronic control and motor pump equipment are mounted in a control box with foam rubber lining for noise isolation, and connected to the wearable vest by air tubing ~6' in length, such that the controls are out of line of sight of the participant. During the study, a white noise machine placed on the participant's computer table was used to mask any remaining perceptible sound from the motors (Figure 4).
3.3 Device control and calibration
We calibrated the inflation and pressure level of the pneumatic devices based on the subjective proprioceptive perception threshold level of each participant, using step-wise psychophysics calibration methods. Participants watched a short video of a nature scene, while air was injected into the pneumatic chambers at a low fill rate. Participants were instructed to indicate to the facilitator when they could feel any perceptible sensation from the air fill. This perceptible threshold level was marked in software, and air was then sequentially extracted and injected to test perception of inflation and deflation at levels below and above the original marked point, repeated across multiple trials to determine a consistent average.
Baseline respiration rates for participants were established during both the initial Interpersonal Reactivity Index (IRI) survey and the pneumatic device calibration phase. Based on these measurements, we set an individualized average simulated respiration rate for each participant, which ranged from ~16.5 to 19.5 breaths per minute. This individualized rate served as the normalized baseline value of 1.0 for all subsequent calculations per participant, with stepwise variation in settings calculated relative to this baseline.
3.4 Interval-based design
Within the timed sequence portion of the study, participants experienced a sequence of stepwise changes in either the simulated respiration rate (first portion, 14 rate intervals) or simulated inflate-deflate ratio (second portion, 14 ratio intervals) from the device (Figure 2). Each step change was generated at a randomized step difference from the previous interval setting, within constraints of a maximum step size of +/− 0.2, with minimum and maximum absolute values of 0.6/1.4 relative to the normalized 1.0 baseline. Each frequency or ratio setting stayed constant across a fixed-length interval of 30 s. We use the term “breath frequency” to refer to the frequency (speed) of the simulated device inflate-deflate cycle, which varied during the “breath frequency” experimental section. We use the term “inflate/deflate ratio” to refer to the ratio of inflate (simulating an inhalation) to deflate (simulating an exhalation) in each cycle, which varied during the “ratio” experimental section. For example, at an average breathing frequency of 15 breaths per minute, each breath cycle is 4,000 ms; with a 1.0 ratio, both the inflate and deflate will be 2,000 ms each. The delta of each respective condition (e.g., delta breath frequency) refers to the difference of the parameter in a given 30-s interval compared to the same parameter in the previous 30-s interval.
3.5 Participants
Twenty-eight participants (12 male, 15 female, one unspecified; mean age = 35.4, SD = 13.5, between 19 and 64) were recruited through MIT's Behavioral Research Lab, via a mailing list to a geographically local participant pool without specific academic affiliations who had registered their interest in participant studies. Eight participants noted that they had no prior experience with breath-related awareness such as yoga or meditation experience, 18 participants noted some slight prior experience, and two participants noted significant prior experience. All participants were fluent in English and had normal or corrected-to-normal vision. Participants signed an informed consent form before their participation in the study, and received a gift card as compensation.
4 Multimodal assessment
Assessing individual perceptions of attention, awareness, and sensory experience presents inherent challenges. To address this, our approach integrates real-time performance data from a well-established task designed to measure attention and cognitive load [the Trail-Making Test (Reitan, 1955)], with multi-channel physiological measurements (such as heart rate, heart rate variability, electrodermal activity, and breathing rate). We also incorporate survey responses and conduct a post-study phenomenological interview. These diverse assessment metrics are coherently linked through time synchronization and time-based reference points, enabling a strong integration of quantitative and qualitative data types. In the scope of this work, our primary focus lies on examining the effects on synchrony, cognitive load, as well as sense of ownership and agency. In our discussion of future directions, we outline the potential for advancing methods to cross-analyze objective and subjective data, thereby enabling a deeper understanding of personalized experiences.
4.1 Cognitive load measurement
4.1.1 Procedure
Cognitive load is evaluated using the Trail Making Test, a well-established neuropsychological assessment tool for visual attention and task switching (Sánchez-Cubillo et al., 2009). In this task, participants are directed to connect 25 dots in numerical order as swiftly and accurately as possible. The test offers insights into visual search speed, attention, and cognitive processing speed. While the complete Trail Making Test often includes a second component to assess mental flexibility and executive functioning (i.e., connecting lines in a 1-A-2-B-3-C pattern instead of 1-2-3), our study focuses solely on the first segment, where participants connect dots numerically to assess attention and cognitive load.
Each Trail Making Test trial had a time limit of 25 s for its completion. Before initiating the timed tasks, participants engaged in several practice rounds to become familiar with the task interface and the keyboard and mouse ergonomics. This phase also allowed for an adaptation period for the pneumatic device, which remained active throughout the practice rounds. During this adaptation phase, both the simulated breathing rate and the inflate-deflate ratio were maintained at a normalized level of 1.0, based on established baselines.
4.1.2 Stimuli
To achieve our measurement objectives, we developed a custom open-source software program that generates patterns for the Trail Making Test. These patterns appear random but form generally recognizable shapes, such as animals, vehicles, or plants. This modification serves dual purposes: it maintains the original goals of assessing cognitive load and also introduces reference points into the otherwise non-referential task, thereby facilitating participant reflections during post-study interviews.
The customized Trail Making Test process uses a three-step pipeline: (1) an image generator, (2) a free online image-to-dot-pattern generator, and (3) software to generate coordinate data based on the dot patterns. This approach allows raw images, such as sample pictures or drawings, to be transformed into specified coordinate points, creating a low-resolution representation of the original image (Figure 5). During the task, these ordered dots are displayed on the participant's screen, allowing us to capture accuracy relative to each coordinate point. The code will be made available after approval of this manuscript.
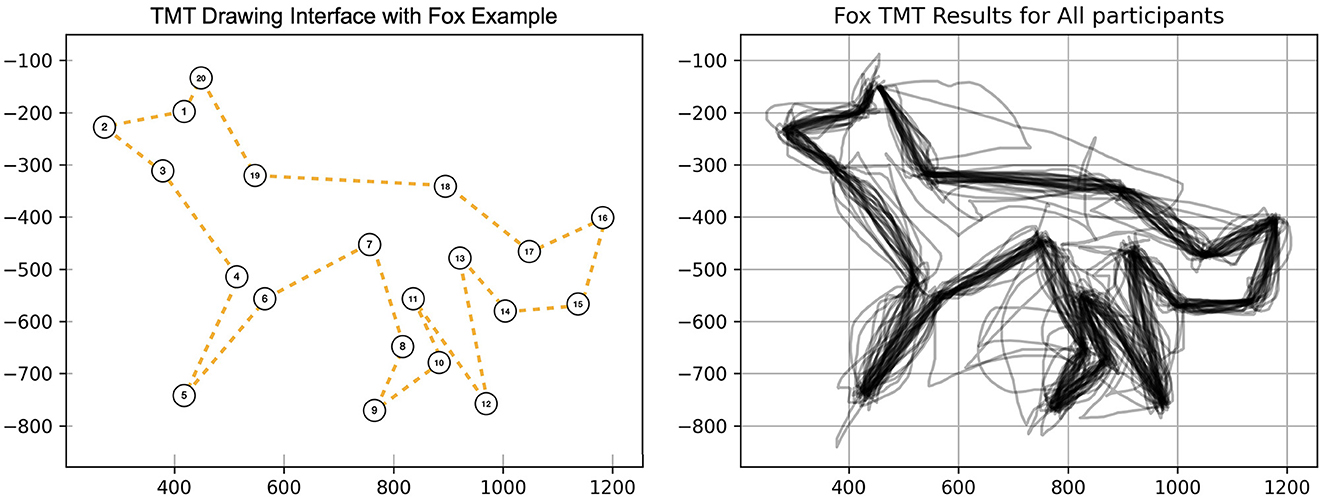
Figure 5. (Left) Trail Making Test prompt with numerically ordered dots. Dashed line is shown for representative purposes and was not visible for participant interaction. (Right) All participant line drawings for the sample Trail Making Test prompt, showing a variation in accuracy and drawing speed.
4.2 Physiological data capture
We collected physiological data—including heart rate (HR), photoplethysmogram (PPG), and electrodermal activity (EDA)—from participants using an Emotibit device placed on the upper arm. Emotibit is an open-source multimodal sensor designed for capturing research-grade physiological signals (Montgomery et al., 2023). To ensure the quality of the recorded signals, we verified sensor positioning and data integrity using a real-time oscilloscope tool available within the Emotibit platform. Data was directly stored onto an SD card within the device. At the beginning of each session, we synchronized time with the device. Important events in the control software—such as parameter interval changes, progression of prompts in the Trail Making Test (TMT), and markers indicating participant awareness—were timestamped according to this synchronized time. These timestamps were sent to the device as lab streaming layer (LSL) markers and also locally recorded for time-sync validation.
For the calculation of Heart Rate Variability (HRV), we used photoplethysmogram (PPG) signals captured by the Emotibit device and processed them using the scipy library. The raw PPG data underwent preprocessing to remove artifacts and outliers, using a z-score-based filtering technique. Subsequently, we identified the intervals between successive peaks of the PPG waveform, analogous to RR-intervals in ECG. HRV was quantified using the Root Mean Square of Successive Differences (RMSSD) method over a 30-s moving window.
Breathing rate was assessed using an audio-based signal analysis, taking the audio signal from a microphone worn under the nostril, enabling participants to breathe naturally through either the nose or mouth with good signal clarity. The audio signal was filtered into FFT bands to capture breathing events while reducing external noise. We analyzed the selected FFT bands in our control software using a running average of peak-peak intervals in the signal, using interquartile range (IQR) filtration for noise reduction, and averaging over a moving window of 20 s to achieve a reliable estimate of breathing rate.
4.3 Empathy and interoceptive acuity
Participants completed a pre-study survey using the Interpersonal Reactivity Index (IRI) measurement tool, a common study for assessing empathy-related qualities with measures for self-esteem, social functioning, emotionality, and sensitivity to others. This questionnaire was selected because of the established links between other-sensitivity and individual interoceptive acuity (Seifert, 2018; Angioletti and Balconi, 2020); our hypotheses explore whether a higher IRI score (indicating greater empathy and other-sensitivity) can predict higher device awareness or sense of ownership.
4.4 Explicit awareness
Participants used the spacebar on the computer keyboard to denote instances of explicit awareness of the wearable device. They were instructed to press the spacebar whenever their attention shifted to the device, either due to a discernible change in sensation or a redirection of focus from their primary computer-based task. Participants used the computer mouse for the Trail Making Task with their right hand (all participants were right-hand dominant), keeping their left hand resting on the keyboard. This setup enabled them to register awareness of the device by pressing the spacebar without disrupting their ongoing engagement with the Trail Making Test. Timestamps for these explicit awareness markers were recorded locally and also transmitted as Lab Streaming Layer (LSL) markers to the Emotibit device, ensuring accurate time synchronization for subsequent analysis. For the analysis, explicit awareness for normalized across participants and intervals, where 1.0 is the highest number of times one participant pressed spacebar within an interval and 0 is no presses.
4.5 Sense of agency and ownership
Post-study survey measures include a Sense of Agency score and Sense of Body Ownership score. The Sense of Agency measure followed the questionnaire proposed by Tapal et al. (2017), with seven items rated on a Likert scale from “1-Strongly Disagree” to “5-Strongly Agree”. We modified the Sense of Agency Score questionnaire to be more specific about breathing by inserting “associated to the breathing device” in every item. For example, “My experiences and actions were under my control” became “My experiences and actions associated with the breathing device were under my control. Moreover, we removed item 7 and 9 since they did not refer to feelings of control (agency) of the device.
The Sense of Body Ownership followed the questionnaire proposed by Lewis and Lloyd (2010), with nine items rated on a Likert scale from “−3” to “3”. We modified the Sense of Body Ownership Score questionnaire to be more specific to a sensation of breathing, for example “I felt as if the device breathing was my breathing” rather than “I felt as if the body was my body.” We encountered several limitations around systematically measuring ownership and agency of a sensation at the threshold of perceivable awareness, explored more fully in the Discussion section.
4.6 Interview methods
After completing the closing surveys, each participant engaged in a structured phenomenological interview with the study facilitator to understand their perceived experience with the wearable device. These interviews, lasting between 10 and 15 min, were informed by established phenomenological methodologies designed to collaboratively generate knowledge about the participant's experience during the experiment (Høffding and Martiny, 2016). The interviewing technique is designed to elicit precise, nuanced descriptions of the participant's experiences during the study. This was achieved by employing open-ended “how“ questions, aimed at encouraging participants to articulate the foundational elements of their experiences in as much detail as feasible (Høffding and Martiny, 2016; Zahavi and Martiny, 2019).
5 Data analysis
We pre-registered our analysis at https://aspredicted.org/2Y7_XJF. Unless noted otherwise, the results are pre-registered.
5.1 Cognitive load analysis
Given that most of the participants normally fail to reach more than 12 points (Linari et al., 2022), a criterion of 12 correctly connected points was set, starting from the first point, to declare the trial completed and define the response time as the time needed to concatenate the first 12 points. A similar criterion was used for the percentage of completion: the percentage of trials that had been successfully completed until the final point “12.” Please note that this 12-point “successful trial” criterion was not pre-registered.
Correct trials were those that fulfilled the completeness criterion and also presented a correctly connected sequence of points. To that end, the drawn trajectory of the mouse should enter all the targets only in the correct order (e.g., 1-2-3-4, etc.) at a minimum of 10 px distance from the center of each coordinate point dot.
To examine the data, we employed an ordinary-least squared (OLS) regression model. We used z-scores for the variables, which is a statistical technique to measure a value's relationship to the mean of a group of values. It is measured in terms of standard deviations from the mean. Previous meditation experience was another factor we controlled for because it might have influenced the participant's performance. The information about prior meditation experience was gathered as part of the participant's background information before the trial began. We sought to specifically ask if they practiced meditation regularly or if they had any experience with similar attention-focused activities prior to the study.
5.2 Synchrony analysis
To conduct synchrony analysis, we assessed whether different features of each participant's physiological data fluctuate relative to the device input (e.g., whether increases in the participant's breathing rate or HRV are time-matched with increases in the device frequency or ratio, demonstrating synchronous behavior). To measure HRV and breath synchrony, we calculated a delta HRV and delta breath rate for each interval per person. These calculated scores were then each subtracted from delta breath frequency to arrive at a synchrony score for each interval. Since the score had 0 as the value for synchrony and negative and positive values as deviations from synchrony, we normalized the data to between 0 and 1, and subtracted the score from 1 to have 1 be the indicated of synchrony and 0 the indicator of no synchrony.
The study implemented OLS regression models to test for the effects of the independent variables (inflate/deflate ratio and breath frequency).
6 Results
6.1 Greater deviation in parameters from baseline leads to increased explicit awareness
In evaluating the effects of parameter deviation on explicit awareness using an ordinary least squares (OLS) regression model, factors such as delta inflate-deflate, delta breath frequency, along with control variables such as interval order, and prior meditation experience were taken into account. See Figure 6.
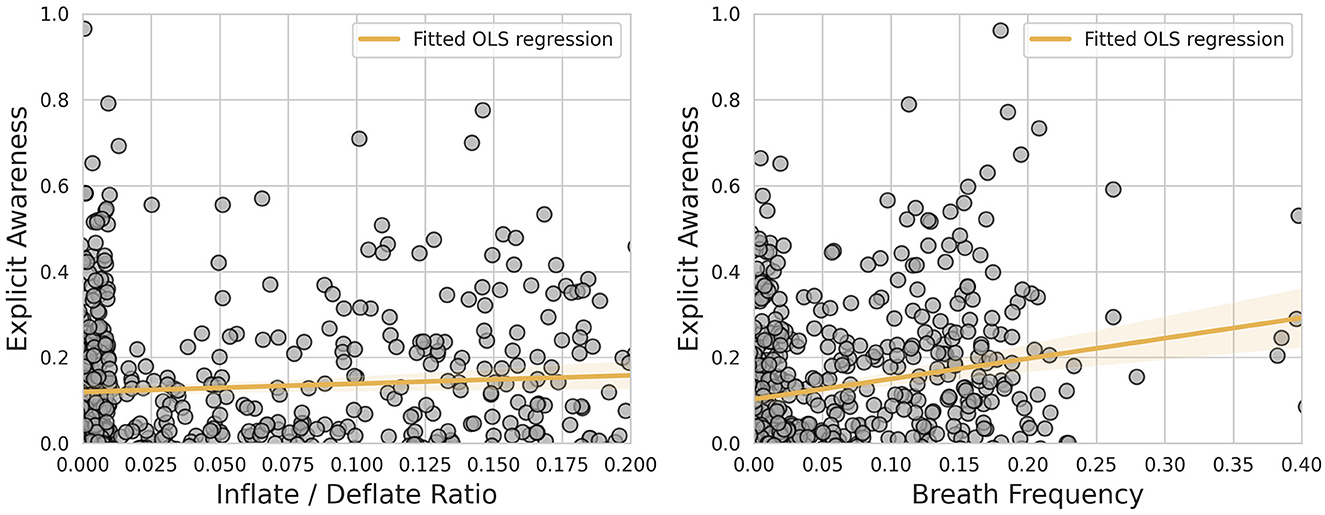
Figure 6. OLS regression models, showing the influence of device breath frequency and device inflate/deflate ratio on explicit awareness.
The model's results highlight that delta inflate-deflate (amount of change in the inflate-deflate ratio of the simulated breathing pattern, relative to the previous state) and delta breath frequency (amount of change in the frequency of the simulated breathing pattern, relative to the previous state) are statistically significant predictors of explicit awareness (p < 0.001 and p < 0.001, respectively). A unit increase in delta inflate-deflate leads to a rise of β = 0.30 in explicit awareness where 0 is no awareness and 1 is fully aware, assuming all other variables remain constant. Similarly, a unit increase in delta breath frequency engenders an increment of β = 0.38 in explicit awareness.
Conversely, the control variables, interval order and prior meditation experience, did not significantly impact explicit awareness. The interval order yielded an estimated coefficient of β = 0.02 but with a high p-value of 0.7, indicating it is not statistically significant at conventional levels. The prior meditation experience demonstrated an estimated coefficient of β = −0.06 with a p-value of 0.12, suggesting it is also not statistically significant at conventional levels.
The R-squared value for the model stands at 0.08, implying that ~8% of the variation in explicit awareness is accounted for by the predictors in this model (delta inflate-deflate, delta breath frequency, interval order, and prior meditation experience). This means that other variables not included in our model also contribute to explicit awareness. Meanwhile, the adjusted R-squared value is slightly lower at 0.076, considering the number of predictors in the model.
The F-statistic value is 12.28, significant at p < 0.001 level, suggesting the overall fit of the model is statistically significant. Hence, the model is adequate in explaining the relationship between these selected predictors and explicit awareness even though the explained variance is relatively limited at ~8.3%.
6.2 Deviation in parameters may impact sense of device body-ownership but not sense of agency
To evaluate the effects of deviation in parameters on Sense of Agency and Sense of Device-Body Ownership, an Ordinary Least Squares (OLS) regression model incorporating delta inflate-deflate and delta breath frequency as predictors, with interval order, and prior meditation experience as control variables was implemented. See Figure 7.
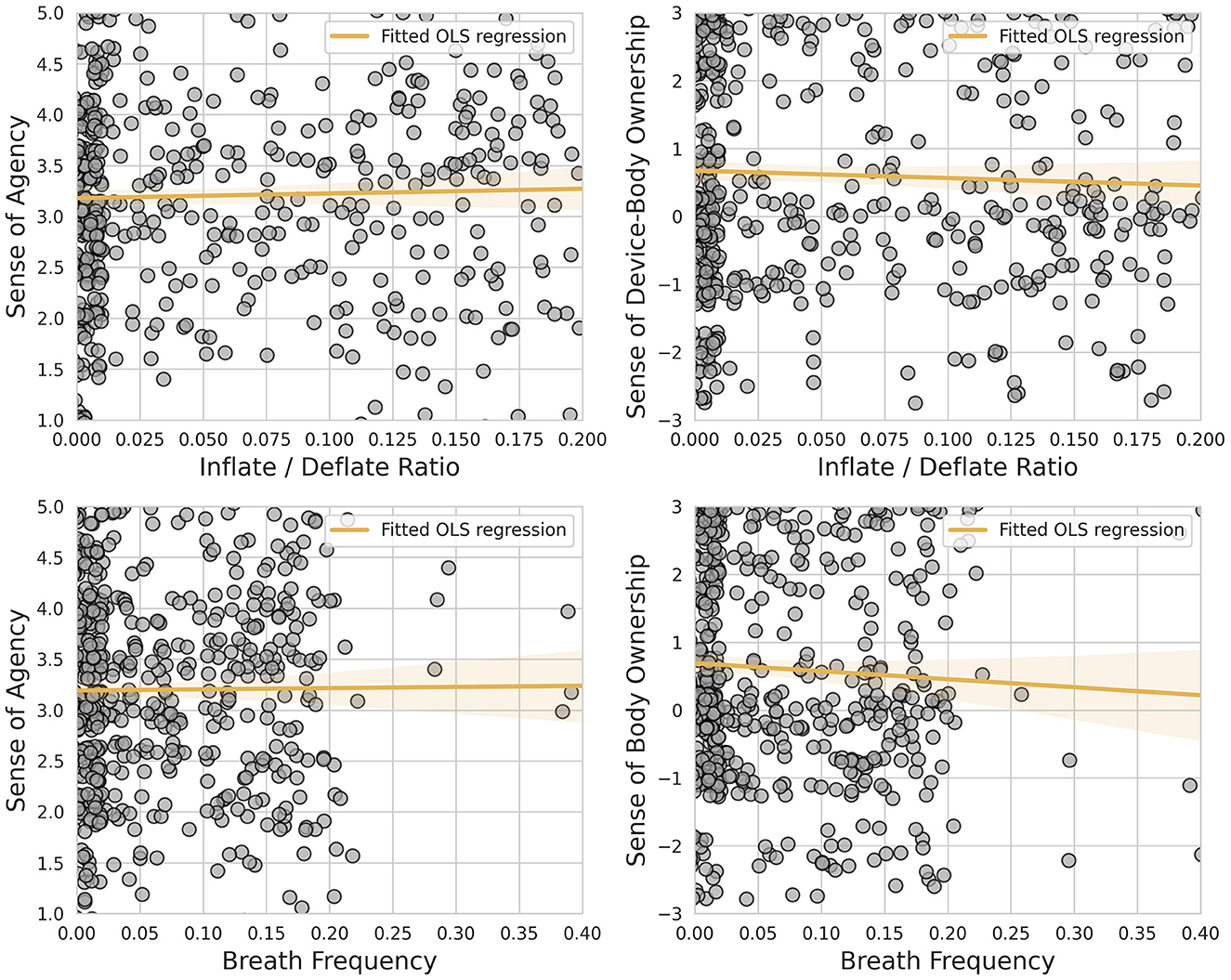
Figure 7. OLS regression models, showing the influence of parameter changes on sense of agency and sense of body-ownership.
Among the predictors, neither changes in inflate-deflate ratio nor changes in breath frequency demonstrated statistically significant influence on the sense of agency (β = 0.03, p = 0.61 and β = 0.06, p = 0.30, respectively) or sense of device-body ownership (β = −0.08, p = 0.22 and β = −0.11, p = 0.09). However, for sense of body ownership the changes in breath frequency is close to being significant at a 10% level with a p-value of 0.09, suggesting that variation in breath frequency may be associated with a change in sense of device-body ownership, though this relationship is moderately weak.
Among the control variables, prior meditation experience had a significant effect on both sense of agency (β = 0.34, p < 0.001) and sense of device-body ownership (β = −0.11, p = 0.01). However, the interval order variable was not statistically significant (p = 0.34) with an estimated coefficient of β = 0.05.
The model's R-squared and adjusted R-squared values are 0.118 and 0.112 respectively, indicating that ~11.2-11.8% of the variability in sense of agency can be attributed the variables included in this model.
6.3 Increases in breath frequency leads to a decrease in both HRV and breath synchrony
To test whether deviations in the parameters from the baseline results in increased HRV/breath synchrony while larger deviations diminish synchrony, we employed an OLS linear regression analysis. Instead of assessing the absolute values of deviation from baseline for measures such as delta inflate-deflate and delta breath frequency, this analysis probed the directionality of these changes. See Figure 8.
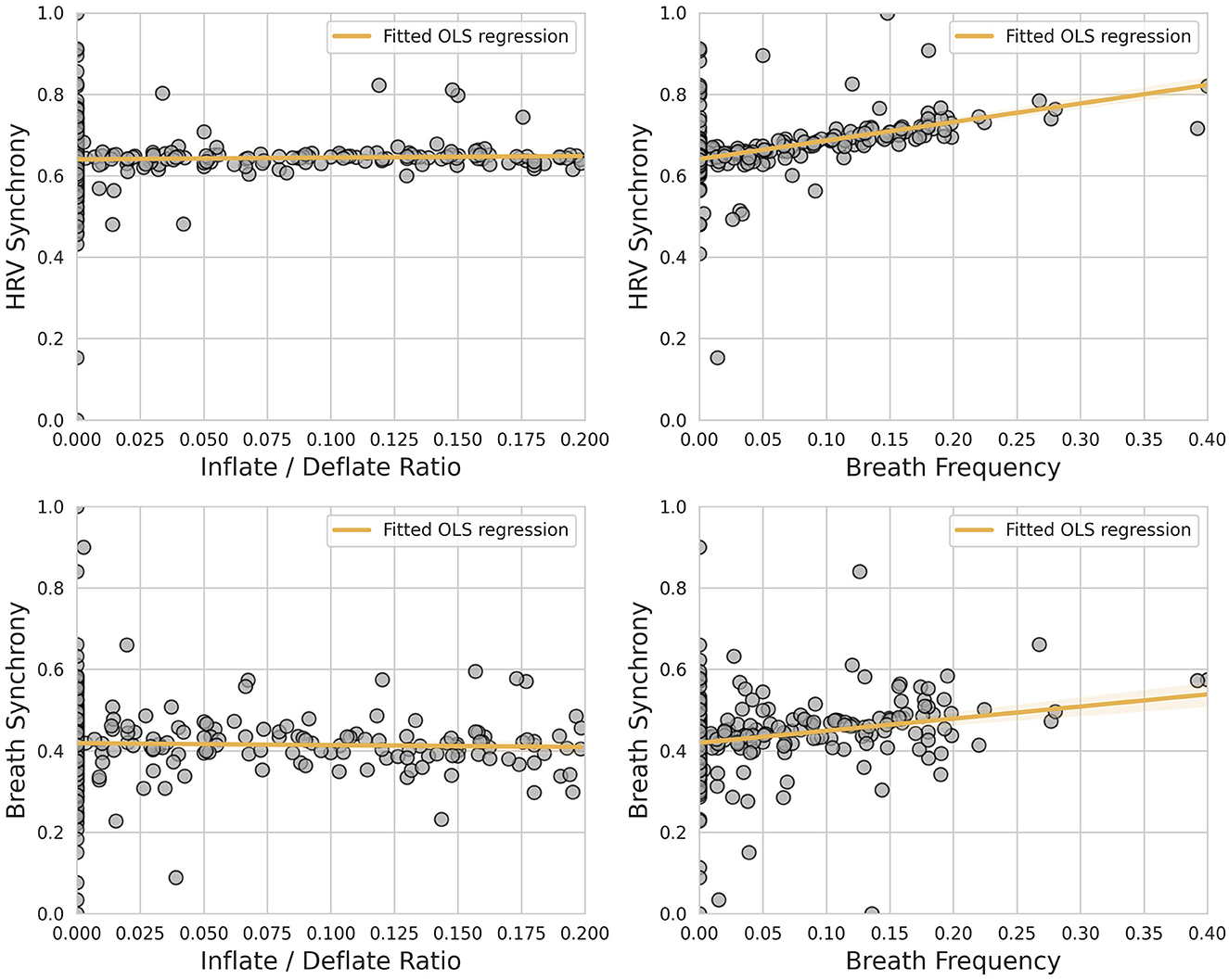
Figure 8. OLS regression models, showing the influence of parameter changes on heart rate variability synchrony and breath rate synchrony.
Upon analyzing the HRV Synchrony model, a significant negative relationship emerged between delta breath frequency and HRV synchrony (β = −0.59, p < 0.001, not pre-registered). This suggests that with every unit increase in deviation from the baseline breath frequency, a corresponding decrease is noted in HRV synchrony. The other factors, namely delta inflate-deflate, interval order, and prior meditation experience, demonstrated non-significant effects, indicating their limited role in explaining variability in HRV synchrony (β = 0.05, p = 0.14 and β = 0.06, p = 0.09 and β = 0.03, p = 0.39, respectively, not pre-registered). The R-squared value in the model explained ~35.7% of the variation in HRV synchrony (Adjusted R2 = 0.35).
A similar pattern of findings emerged in the breath synchrony model. Delta breath frequency was a significant predictor (β = −0.32, p < 0.001, not pre-registered), indicating a negative relationship between changes in breath frequency and breath rate synchrony. Other variables—delta inflate-deflate, interval order, and prior meditation experience—did not demonstrate statistical significance, suggesting that their influence on breath synchrony was limited (β = −0.04, p = 0.29 and β = 0.02, p = 0.65 and β = 0.00, p = 0.91, respectively, not pre-registered). The R-squared for this model was relatively less in comparison to the HRV synchrony model, accounting for about 10.7% of the variation in breath synchrony (adjusted R-squared = 0.100).
In both cases, the direction of change in breath frequency, rather than just the magnitude of change, significantly influenced synchrony levels. Specifically, increases in breath frequency from the baseline corresponded to decreases in both HRV and breath synchrony. Thus, utilizing non-absolute delta values allowed the examination of impact directionality of the predictors, providing a more nuanced understanding of their relationships with HRV and breath synchrony.
Notably, despite the statistical significance observed, the R-squared values suggest that other variables not included in this model might also play a significant role in explaining variations in HRV synchrony and breath synchrony. Therefore, future studies should explore other potential predictors for a more comprehensive view.
6.4 Increased cognitive load correlates with increased explicit awareness of the device and lower sense of agency and lower sense of device body-ownership
A Pearson correlation coefficients test was run to evaluate the hypothesis that higher levels of IRI scores and cognitive load correlate with increased explicit awareness of the device, and a reduction in the sense of agency and sense of device body-ownership (Figure 9).
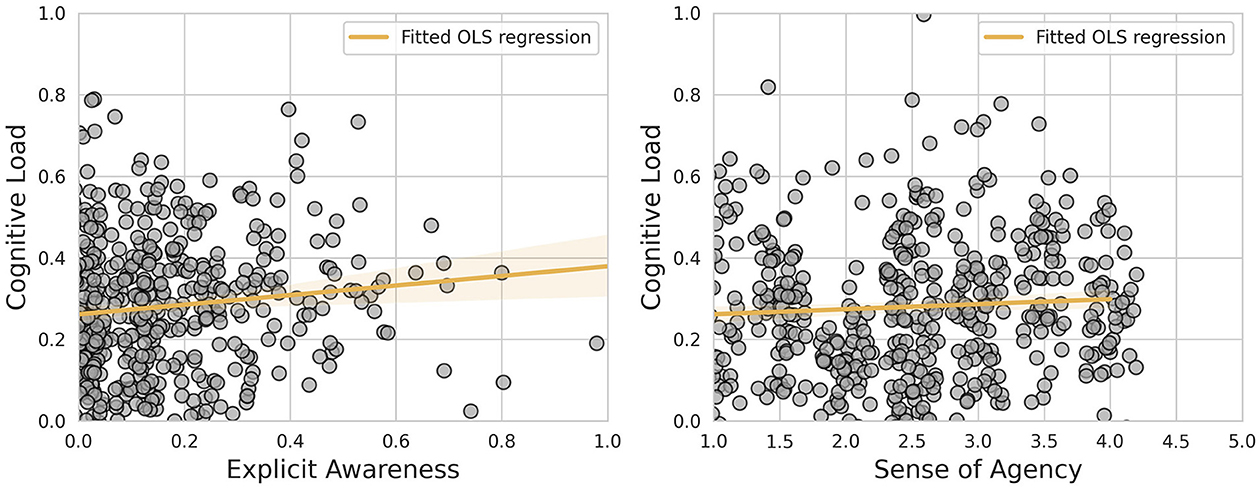
Figure 9. Pearson correlations between explicit awareness and cognitive, and sense of agency and cognitive load.
Correlation between cognitive load score and explicit awareness showed not significant (r = −0.05, p = 0.23), suggesting no meaningful correlation. A significant negative correlation was observed between cognitive load score and sense of agency (r = −0.10, p = 0.02), suggesting that higher cognitive load scores are associated with diminished sense of agency. On the other hand, the correlation of cognitive load score with sense of device body-ownership was not significant at conventional levels but trended toward negative (r = −0.08, p = 0.06). This suggests that with higher cognitive load scores there may be a tendency toward reduced sense of device body-ownership although this effect did not quite reach statistical significance.
Lastly, a negative correlation was observed between sense of device body-ownership and sense of agency (r = −0.07, p = 0.09). While not statistically significant at conventional levels, it suggests a possible trend that as sense of device body-ownership decreases, sense of agency also decreases.
6.5 High IRI scores correlate with lower sense of agency
Lastly, to evaluate the hypothesis that higher levels of IRI scores correlate with increased explicit awareness of the device, and a reduction in the sense of agency and sense of device body-ownership, a Pearson correlation coefficients test was run.
Correlation between the IRI score and explicit awareness turned out to be not statistically significant, (r = 0.04, p = 0.41), indicating no substantial association. However, the IRI score was found to be significantly negatively correlated with sense of agency (r = −0.37, p < 0.001), suggesting higher IRI scores are associated with lower sense of agency. However, no significant correlation was found between the IRI score and sense of device body-ownership (r = 0.025, p = 0.56), suggesting no substantial relationship (see Figure 10).
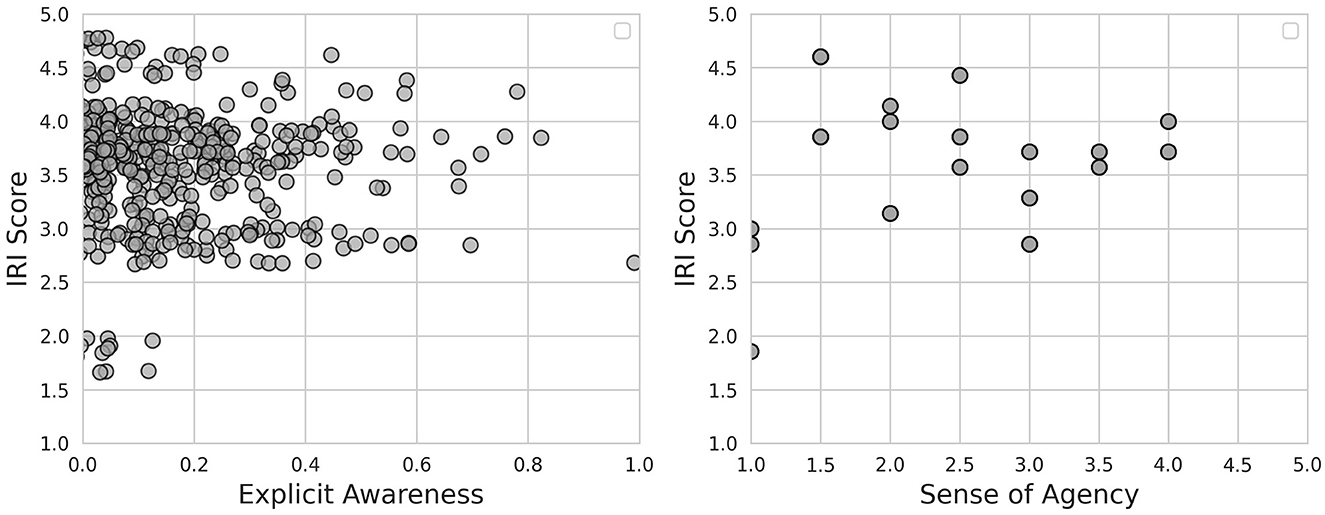
Figure 10. Scatter plots showing the correlation of explicit awareness and sense of agency with IRI scores.
7 Discussion
The primary aim of the current study was to explore how manipulating certain parameters related to the respiration rate of a wearable device translated to different user perceptions on aspects such as explicit awareness, sense of agency, and sense of device body-ownership. We also investigated the interactions between these parameters and heart rate variability and breath synchrony.
7.1 Devices that deviate from “expected” behavior increases explicit awareness
The research found strong evidence supporting the predicted increase in explicit awareness, resulting from larger deviations in parameters from baseline. This is consistent with prior research, which shows that alterations in a physiological signal that deviates from an individual's “normal” or “expected” state can increase the conscious attention paid to it.
Descriptions of the physical sensation of the device, when participants noted awareness of the device presence, included a variety of metaphors and physical descriptions of the simulated breathing sensation. Most descriptors identified the sensation as a perceived external entity, e.g., an animal or an external object, in moments when they were consciously aware of the sensation. One participant described the following: “It felt like breathing on breathing... like a puppy or a cat or something, but just something small breathing on you, just enough where you feel the motion on your tummy, but not full on balloons inflating or an inflatable vest or something like that, very subtle” (P146833). Some participants identified the sensation as a modified perception of their own body relative to the vest: “I wasn't sure if… the fitted vest was inflating or I myself was inflating, as though I was breathing and my abdomen was expanding” (P144349).
7.2 Devices that deviate from “expected” behavior was not associated with sense of agency and ownership
Testing for the effects of deviations in inhale/exhale ratio and breath frequency on sense of agency and body ownership, our models did not reveal any significance and presented a weak predictive power, with only breath frequency being close to significance on sense of device-body ownership. This contradicts previous reports by Murray (2004) and could be caused by limitations in our measurements of sense of agency and sense of body ownership which was only calculated once per participant rather than somehow assessed continuously after each parameter change. Previous research reveals sense of agency and sense of body-ownership to operate very dynamically in-the-moment (Lewis and Lloyd, 2010; Kasahara et al., 2019).
Future research should investigate the use of alternative variables or measures that might be more appropriate or insightful in predicting sense of agency and sense of body-ownership. For instance, more “local” measurements of sense of agency during the task as seen in Kasahara et al. (2019) rather than cumulatively at the end could be able to account for more dynamic changes.
7.3 Prior meditation experience influences reported sense of agency and sense of device body-ownership
Prior meditation experience was found to significantly correlate with both sense of agency and sense of body ownership. For sense of agency, the coefficient for prior meditation experience is positive and significant, implying that participants with more meditation experience had a higher sense of agency. This connection could be attributed to the fact that meditation practices often involve focusing on one's body and breath, which might enhance a person's ability to perceive the agency. However, in the ownership model, the relationship between prior meditation experience and sense of ownership is negative and significant, suggesting that individuals with more meditation experience had a lower sense of device-body ownership. This outcome may be due to experienced meditators having a better ability to distinguish between their own body and external devices. In both cases meditators might have an increased capability to introspect and analyze their interoceptive signals potentially leading to stronger ratings in agency and body-ownership.
7.4 Body-ownership and sense of agency not correlated
We did not find sense of body device body-ownership to correlate with sense of agency, contrary to findings in previous literature. One likely explanation for this divergence can be found in the participants' self-reported focus on the Trail Making Test except in moments of perceived unnatural sensations from the device. Many noted that they were largely unaware of the pneumatic device except in moments when its characteristics notably diverged from their own baseline state (as supported by H1). This lack of reflective awareness, despite demonstrated physiological synchrony with the device, suggests that the device most commonly operated below the threshold for conscious perception yet still influenced physiological states. This is supported by other literature around unconscious interfaces (Amores and Maes, 2017; Jain et al., 2020). This phenomenon may indicate that the scales we used to measure body-ownership and agency were not adequately tailored to capture the unique experiences participants had with this device. This could additionally count for the anomalous discovery in our findings that Interpersonal Reactivity Index (IRI) scores were negatively correlated with sense of agency, but showed no correlation with explicit awareness or sense of body-ownership.
P146833 noted this limitation in the specific descriptions of the survey: “As I was answering the survey questions, I also realized I wasn't aware that sensation was taking place.” The same participant independently described that: “I feel like in the beginning, it felt like my breath was catching up to a different rhythm. And then toward the end of the tasks, I think it started to feel like it too.” This self-report seems to indicate an experience of change within the participant's self-owned body that was not adequately assessed in the survey measures. Similarly, P128956 noted regarding the survey measures: “I know some of the survey questions was like, did it feel a part of your breathing? But for me, it felt very external.” Yet in the phenomenological interview, that participant also described adopting the device characteristic internally: “there were actually stretches when I became very aware of the fact that there was a cadence. And then it made me follow that cadence in my head and maybe my breath and expect the cadence.” These outcomes suggest the limitations of conventional measurements in capturing the complex interplay between conscious and unconscious interactions with wearable technology.
7.5 Self-report of body-ownership vs. body non-ownership
Participants reported that they primarily became consciously aware of the device when they felt an asynchronous relationship between their own breath and their perception of the device, causing an attention shift to the device, and biasing their perception of body-ownership toward the moments of a more externalized relationship to the sensation. Participants noted that they had little awareness of the device when it seems to be synchronized to their own breathing (and perhaps more aligned with potential body-ownership), despite the trends in synchrony, indicating that physiological synchrony—a trend toward matching the characteristics of the device, even when not consciously perceived—may be a more genuine assessment measure as compared to self-reported ownership. Participants noted: “It was only those points where my mind shifted focus from the task and moving the mouse that I noticed the device going. And it may not have been in sync with me, but it just wasn't so out of sync that I could feel it. And when I did notice it, it felt not in sync. It felt... out of sync. But that may also be that my breathing might have adjusted and I was out of sync with the breathing that I had been breathing. And that's where the out of sync felt” (P32008). “I don't know if that's what it was doing, but it felt like it was in sync with me. So I never really noticed it” (P128857). “Sometimes it felt a little bit out of sync, as though I were supposed to be breathing more in time with the device. And I sort of wondered if maybe over time, I was matching it without thinking about it, but that was hard to tell” (P129238).
7.6 Opportunities for mapping subjective-objective data
A common theme which emerged in interviews was that participants' explicit awareness of the device increased when they were between tasks in the Trail Making Test, or in sections where the TMT prompt had more adjacent consecutive numbers and was easier to complete: “I also feel like when I was solving the puzzles, I could have also paid more attention to the device. I would allocate my attention to my tummy for the device, for easier puzzles, but when the puzzles got harder, I sort of just filtered it out” (P124528). “I would finish a puzzle, for example, and I would observe [the breathing] more, or toward the beginning of puzzles. More so than like, the middle. In the middle, I was more into finding the numbers and paying less attention” (P128857). The current methods do not take into account the current state of the Trail-Making Test (e.g., beginning, middle, or end of a prompt) as a predictor for explicit awareness marking, physiological state, or other variables. Future research would potentially discover more nuanced relationships between these factors by cross-mapping these phenomenological reports to the objective moments of occurrence within the task, using cues such as repeated awareness marking with the space bar, reported memory of identifiable shapes, and other markers.
8 Conclusion
This research advanced our understanding of the complex interplay between wearable devices and human perception, particularly around awareness, sense of agency, and body-device ownership. Our results confirm that greater deviations in device parameters from baseline levels (increase/decrease in device breath frequency or inflate-deflate ratio) increase explicit awareness, and also that smaller variations are more likely to produce physiological synchrony, as shown by participants' physiological rhythms (e.g., breathing rate) fluctuating in parallel with changes in the device parameters. Our results also raise compelling questions about traditional methods used to measure sense of agency and body-device ownership, especially at a low perceptual threshold and under attentional demands. Furthermore, our findings contribute to emerging research about individual variances, as indicated by correlations with Interpersonal Reactivity Index (IRI) scores. As wearable devices become increasingly ubiquitous in various aspects of health and wellbeing—from health monitoring to augmenting social interaction—it is crucial to understand the nuanced ways in which these devices are perceived and interacted with. Our work aims lay the foundation for future research in this area, offering insights that could inform the design of more intuitively integrated and widely adopted wearable technologies.
Data availability statement
The datasets presented in this study can be found in online repositories. The names of the repository/repositories and accession number(s) can be found at: https://github.com/mitmedialab/breath-simulation.
Ethics statement
The studies involving humans were approved by Massachusetts Institute of Technology (MIT) Committee on the Use of Humans as Experimental Subjects. The studies were conducted in accordance with the local legislation and institutional requirements. The participants provided their written informed consent to participate in this study.
Author contributions
CM: Conceptualization, Investigation, Methodology, Project administration, Software, Visualization, Writing – original draft, Writing – review & editing. VD: Conceptualization, Formal analysis, Investigation, Methodology, Software, Visualization, Writing – original draft, Writing – review & editing. PM: Supervision, Writing – review & editing.
Funding
The author(s) declare financial support was received for the research, authorship, and/or publication of this article. CM was partially funded by a Meta Research Fellowship in AR/VR Future Technologies.
Acknowledgments
We are grateful for support in methods design and data analysis from consultants at the Harvard-MIT Data Center (HMDC). We are also grateful for support in participant recruitment and study management from MIT's Behavioral Research Lab (BRL).
Conflict of interest
The authors declare that the research was conducted in the absence of any commercial or financial relationships that could be construed as a potential conflict of interest.
Publisher's note
All claims expressed in this article are solely those of the authors and do not necessarily represent those of their affiliated organizations, or those of the publisher, the editors and the reviewers. Any product that may be evaluated in this article, or claim that may be made by its manufacturer, is not guaranteed or endorsed by the publisher.
References
Amores, J., and Maes, P. (2017). “Essence: olfactory interfaces for unconscious influence of mood and cognitive performance,” in Proceedings of the 2017 CHI Conference on Human (New York, NY: ACM). doi: 10.1145/3025453.3026004
Angioletti, L., and Balconi, M. (2020). Interoceptive empathy and emotion regulation: the contribution of neuroscience. Neuropsychol. Trends 27, 85–100. doi: 10.7358/neur-2020-027-ang2
Baars, B. J. (2005). Global workspace theory of consciousness: toward a cognitive neuroscience of human experience. Prog. Brain Res. 150, 45–53. doi: 10.1016/S0079-6123(05)50004-9
Biddiss, E., and Chau, T. (2007). Upper-limb prosthetics: critical factors in device abandonment. Am. J. Phys. Med. Rehabil. 86, 977–987. doi: 10.1097/PHM.0b013e3181587f6c
Bizzego, A., Azhari, A., Campostrini, N., Truzzi, A., Ng, L. Y., Gabrieli, G., et al. (2019). Strangers, friends, and lovers show different physiological synchrony in different emotional states. Behav. Sci. 10, 11. doi: 10.3390/bs10010011
Botvinick, M., and Cohen, J. (1998). Rubber hands “feel” touch that eyes see. Nature 391, 756. doi: 10.1038/35784
Brandebusemeyer, C., Luther, A. R., König, S. U., König, P., and Kärcher, S. M. (2021). Impact of a vibrotactile belt on emotionally challenging everyday situations of the blind. Sensors 21, 7384. doi: 10.3390/s21217384
Breedlove, S. M., and Watson, N. V. (2018). Behavioral Neuroscience. Sunderland, MA: Sinauer Associates, Incorporated, Publishers.
Clore, G. L., and Ortony, A. (2008). “Appraisal theories: how cognition shapes affect into emotion,“ in Handbook of Emotions, 3rd ed., eds M. Lewis, J. M. Haviland-Jones, and L. F. Barrett (New York, NY: The Guilford Press), 628–642.
Cornelio, P., Haggard, P., Hornbaek, K., Georgiou, O., Bergström, J., Subramanian, S., et al. (2022). The sense of agency in emerging technologies for human-computer integration: a review. Front. Neurosci. 16, 949138. doi: 10.3389/fnins.2022.949138
Craig, A. B. (2009). How do you feel – now? The anterior insula and human awareness. Nat. Rev. Neurosci. 10, 59–70. doi: 10.1038/nrn2555
Damasio, A. R. (1994). Descartes' error and the future of human life. Sci. Am. 271, 144. doi: 10.1038/scientificamerican1094-144
Damasio, A. R. (1999). The Feeling of What Happens: Body and Emotion in the Making of Consciousness. Boston, MA: Houghton Mifflin Harcourt.
Danry, V., Pataranutaporn, P., Mueller, F., Maes, P., and Leigh, S.-w. (2022). “On eliciting a sense of self when integrating with computers,” in Proceedings of the Augmented Humans International Conference 2022 (New York, NY: ACM), 68–81. doi: 10.1145/3519391.3519414
Davis, M. H. (1983). Measuring individual differences in empathy: evidence for a multidimensional approach. J. Pers. Soc. Psychol. 44, 113–126. doi: 10.1037/0022-3514.44.1.113
De Vignemont, F. (2011). Embodiment, ownership and disownership. Conscious. Cogn. 20, 82–93. doi: 10.1016/j.concog.2010.09.004
Dourish, P. (2001). Where the Action is. Cambridge, MA: MIT press. doi: 10.7551/mitpress/7221.001.0001
Frey, J., Grabli, M., Slyper, R., and Cauchard, J. R. (2018). “Breeze: sharing biofeedback through wearable technologies,” in Proceedings of the 2018 CHI Conference on Human Factors in Computing Systems, 645. doi: 10.1145/3173574.3174219
Gallagher, S., and Zahavi, D. (2019). “Phenomenological approaches to self-consciousness,” in The Stanford Encyclopedia of Philosophy, summer 2019 edition, ed. E. N. Zalta (Stanford, CA: Metaphysics Research Lab, Stanford University).
Gallagher, S., and Zahavi, D. (2020). The Phenomenological Mind. London: Routledge. doi: 10.4324/9780429319792
Ghandeharioun, A., and Picard, R. (2017). “Brightbeat: effortlessly influencing breathing for cultivating calmness and focus,” in Proceedings of the 2017 CHI Conference Extended Abstracts on Human Factors in Computing Systems, CHI EA '17 (New York, NY: Association for Computing Machinery), 1624–1631. doi: 10.1145/3027063.3053164
Gilbert, F. (2018). Deep brain stimulation: inducing self-estrangement. Neuroethics 11, 157–165. doi: 10.1007/s12152-017-9334-7
Gilbert, F., Goddard, E., Viaña, J. N. M., Carter, A., and Horne, M. (2017). I miss being me: phenomenological effects of deep brain stimulation. AJOB Neurosci. 8, 96–109. doi: 10.1080/21507740.2017.1320319
Golland, Y., Arzouan, Y., and Levit-Binnun, N. (2015). The mere co-presence: synchronization of autonomic signals and emotional responses across co-present individuals not engaged in direct interaction. PLoS ONE 10, e0125804. doi: 10.1371/journal.pone.0125804
Høffding, S., and Martiny, K. (2016). Framing a phenomenological interview: what, why and how. Phenomenol. Cognitive Sci. 15, 539–564. doi: 10.1007/s11097-015-9433-z
Hove, M. J., and Risen, J. L. (2009). It's all in the timing: interpersonal synchrony increases affiliation. Soc. Cogn. 27, 949–960. doi: 10.1521/soco.2009.27.6.949
Jain, A., Horowitz, A. H., Schoeller, F., Leigh, S-. W., Maes, P., Sra, M., et al. (2020). Designing interactions beyond conscious control: a new model for wearable interfaces. Proc. ACM Interact. Mob. Wearable Ubiquitous Technol. 4, 1–23. doi: 10.1145/3411829
Kasahara, S., Nishida, J., and Lopes, P. (2019). “Preemptive action: accelerating human reaction using electrical muscle stimulation without compromising agency,” in Proceedings of the 2019 CHI Conference on Human Factors in Computing Systems (New York, NY: ACM), 1–15. doi: 10.1145/3290605.3300873
Legrand, D. (2007). Pre-reflective self-as-subject from experiential and empirical perspectives. Conscious. Cogn. 16, 583–599. doi: 10.1016/j.concog.2007.04.002
Lewis, E., and Lloyd, D. M. (2010). Embodied experience: a first-person investigation of the rubber hand illusion. Phenomenol. Cogn. Sci. 9, 317–339. doi: 10.1007/s11097-010-9154-2
Li, Z., Wang, Y., Wang, W., Chen, W., Hoang, T., Greuter, S., et al. (2019). “Heatcraft: designing playful experiences with ingestible sensors via localized thermal stimuli,” in Proceedings of the 2019 CHI Conference on Human Factors in Computing Systems (New York, NY: ACM), 1–12. doi: 10.1145/3290605.3300806
Linari, I., Juantorena, G. E., Ibáñez, A., Petroni, A., and Kamienkowski, J. E. (2022). Unveiling trail making test: visual and manual trajectories indexing multiple executive processes. Sci. Rep. 12, 14265. doi: 10.1038/s41598-022-16431-9
Lopes, P., Andres, J., Byrne, R., Semertzidis, N., Li, Z., Knibbe, J., et al. (2021). Towards understanding the design of bodily integration. Int. J. Human-Comput. Stud. 152, 102643. doi: 10.1016/j.ijhcs.2021.102643
Montgomery, S. M., Nair, N., Chen, P., and Dikker, S. (2023). Introducing EmotiBit, an open-source multi-modal sensor for measuring research-grade physiological signals. Sci. Talks 6, 100181. doi: 10.1016/j.sctalk.2023.100181
Morris, C., Danry, V., and Maes, P. (2022). “EmbER: a system for transfer of interoceptive sensations to improve social perception,” in Designing Interactive Systems Conference, DIS '22 (New York, NY: Association for Computing Machinery), 277–287. doi: 10.1145/3532106.3533550
Mueller, F. F., Lopes, P., Strohmeier, P., Ju, W., Seim, C., Weigel, M., et al. (2020). “Next steps for human-computer integration,” in Proceedings of the 2020 CHI Conference on Human Factors in Computing Systems (New York, NY: Association for Computing Machinery), 1–15. doi: 10.1145/3313831.3376242
Murray, C. D. (2004). An interpretative phenomenological analysis of the embodiment of artificial limbs. Disabil. Rehabil. 26, 963–973. doi: 10.1080/09638280410001696764
Park, S., and Jayaraman, S. (2003). Enhancing the quality of life through wearable technology. IEEE Eng. Med. Biol. Mag. 22, 41–48. doi: 10.1109/MEMB.2003.1213625
Ponzo, S., Kirsch, L. P., Fotopoulou, A., and Jenkinson, P. M. (2018). Balancing body ownership: Visual capture of proprioception and affectivity during vestibular stimulation. Neuropsychologia 117, 311–321. doi: 10.1016/j.neuropsychologia.2018.06.020
Reitan, R. M. (1955). The relation of the trail making test to organic brain damage. J. Consult. Psychol. 19, 393–394. doi: 10.1037/h0044509
Sánchez-Cubillo, I., Periáñez, J. A., Adrover-Roig, D., Rodríguez-Sánchez, J. M., Ríos-Lago, M., Tirapu, J., et al. (2009). Construct validity of the trail making test: role of task-switching, working memory, inhibition/interference control, and visuomotor abilities. J. Int. Neuropsychol. Soc. 15, 438–450. doi: 10.1017/S1355617709090626
Sass, L. A. (2014). Self-disturbance and schizophrenia: structure, specificity, pathogenesis (current issues, new directions). Schizophr. Res. 152, 5–11. doi: 10.1016/j.schres.2013.05.017
Sass, L. A., and Parnas, J. (2003). Schizophrenia, consciousness, and the self. Schizophr. Bull. 29, 427–444. doi: 10.1093/oxfordjournals.schbul.a007017
Schoeller, F., Haar, A. J. H., Jain, A., and Maes, P. (2019). Enhancing human emotions with interoceptive technologies. Phys. Life Rev. 31, 310–319. doi: 10.1016/j.plrev.2019.10.008
Schreuder, E., van Erp, J., Toet, A., and Kallen, V. L. (2016). Emotional responses to multisensory environmental stimuli: a conceptual framework and literature review. SAGE Open 6, 2158244016630591. doi: 10.1177/2158244016630591
Seifert, T. (2018). The Relationship between Proprioceptive Acuity, Emotion Recognition and Emotional Empathy (PhD thesis). Haifa University, Israel.
Slater, M., Brogni, A., and Steed, A. (2003). “Physiological responses to breaks in presence: a pilot study,” in Presence 2003: The 6th Annual International Workshop on Presence, Vol. 157 (Aalborg). Citeseer.
Tapal, A., Oren, E., Dar, R., and Eitam, B. (2017). The sense of agency scale: a measure of consciously perceived control over one's mind, body, and the immediate environment. Front. Psychol. 8, 1552. doi: 10.3389/fpsyg.2017.01552
Tsakiris, M., Schütz-Bosbach, S., and Gallagher, S. (2007). On agency and body-ownership: phenomenological and neurocognitive reflections. Conscious. Cogn. 16, 645–660. doi: 10.1016/j.concog.2007.05.012
Keywords: wearable device, human-computer integration, soft robotics, cognitive load, awareness, sense of body-ownership, sense of agency
Citation: Morris C, Danry V and Maes P (2023) Wearable systems without experiential disruptions: exploring the impact of device feedback changes on explicit awareness, physiological synchrony, sense of agency, and device-body ownership. Front. Comput. Sci. 5:1289869. doi: 10.3389/fcomp.2023.1289869
Received: 06 September 2023; Accepted: 24 November 2023;
Published: 21 December 2023.
Edited by:
Zhuying Li, Southeast University, ChinaReviewed by:
Diego Vilela Monteiro, ESIEA University, FranceJoan Llobera, University of Barcelona, Spain
Josh Andres, Australian National University, Australia
Copyright © 2023 Morris, Danry and Maes. This is an open-access article distributed under the terms of the Creative Commons Attribution License (CC BY). The use, distribution or reproduction in other forums is permitted, provided the original author(s) and the copyright owner(s) are credited and that the original publication in this journal is cited, in accordance with accepted academic practice. No use, distribution or reproduction is permitted which does not comply with these terms.
*Correspondence: Caitlin Morris, Y2Ftb3JyaXNAbWVkaWEubWl0LmVkdQ==