- 1Vilans, Centre of Expertise Long-Term Care, Utrecht, Netherlands
- 2Department of Industrial Engineering and Innovation Sciences, Eindhoven University of Technology, Eindhoven, Netherlands
Affective haptic devices (AHDs) have been developed with the aim of communicating touch acts, symbolic messages, emotions, and/or providing a sense of social awareness. Within AHDs, three categories can be distinguished: mediated social touch (MST), symbolic communication systems, and awareness systems. For each of these categories, prototypes have been developed and discussed in the literature. Each such prototype, however, describes but a small part of the design space of AHDs. What is lacking is a description of the design space itself—of all choices that can be considered during the design process. Such a description will allow for a more systematic exploration of AHD designs and provides a means of combining insights gained from individual point solutions (i.e., existing prototypes). Therefore, in this article, we provide a systematic description of the design space of AHDs and its underlying dimensions based on general (e.g., revisability or synchronicity) and AHD-specific (e.g., actuation type) communication system characteristics. This resulted in 17 design dimensions, each consisting of two or more categories (the design characteristics). Based on a systematic literature search from devices up to 2019, 89 AHD prototypes were identified, and each was classified on the design dimensions. The empirical analysis of where these AHDs are located in the design space revealed, first, that potentially interesting characteristics from mediated communication, such as revisability and reviewability, are underexplored in AHDs. Second, MST devices were found to often lack those system characteristics, such as real-time modalities, that seem crucial for providing the affordances needed to simulate social touch. In particular, when comparing symbolic and awareness devices to MST devices, we found the latter to more frequently lack some of the key characteristics of face-to-face communication (i.e., bi-directional and symmetric communication). Limitations and implications are discussed.
Introduction
Communication is an essential aspect of our human life. Over the past decades, communication media, from the physical letter and the telephone to modern-day social media, have drastically changed when, where, and how we communicate. In contrast to face-to-face communication, mediated forms of communication provide unique characteristics, such as the possibility of asynchronous communication (i.e., conversation that is not real-time) or adjusting a message before sending it to the other (revisability).
To date, most mediated forms of communication are not yet able to provide the richness of face-to-face communication, in which typically most of our sensory modalities are involved (Nadler, 2020). Past communication tools have largely focused on communication through the modalities of audio, text and/or video. Communication through a haptic modality has been less prominent in current communication technology. However, over the past years, there has been a rise in the development of so-called affective haptic devices (AHDs), which can be defined as devices aimed at communicating affective messages through a haptic modality (e.g., warmth, vibration and/or force). The promise of these AHDs is that they can enrich mediated communication in different ways. They can facilitate the communication of emotions, touch acts (e.g., a hug), symbolic messages (e.g., letting someone know you are thinking of them), or enhance social awareness. Currently, there are a wide range of AHD prototypes (e.g., Dodge, 1997; Teh et al., 2009; Pradana et al., 2015) as well as several commercial devices (e.g., Bond Touch, HB Ring1) that have been developed. Alongside these protypes, dedicated experimental devices have been developed (e.g., Haans et al., 2007; Cabibihan et al., 2012) with the purpose of investigating affective and/or behavioral responses toward affective haptic stimuli in controlled laboratory studies.
Three types of AHDs
The concept of AHDs is linked closely to that of social touch (or interpersonal physical contact). References to the importance of social touch for human wellbeing are abundant in the existing AHD literature, and the focus of the field has in earlier reviews been described as to be on mediated social touch (MST; Haans and IJsselsteijn, 2006) or social touch technologies (Huisman, 2017). While it is true that many articles in the field of AHDs focus on enabling social touch, such as hugs (Teh et al., 2012) or hand-holding (Erk et al., 2015), over a distance, close inspection of the available literature reveals that this is not the sole purpose for which AHDs are designed. At least three different types of AHDs, and thus three different design intentions, can be identified in the literature. First, there are AHDs aimed at facilitating an affective or playful touch over a distance. They focus on mimicking social touch for circumstances where skin-to-skin touch is not possible, for example, the Remote Handshake which facilitates handshaking during a videoconference (Nakanishi et al., 2014). Another example is the Huggy Pajama (Teh et al., 2012), a system build to enrich parent-child communication by facilitating parents to give their child a hug over a distance. This category of devices we refer to as Mediated Social Touch (MST), as they attempt to simulate, at least in part, the experience of real—unmediated—social touch acts. Second, there are systems aimed at facilitating the communication of affection (e.g., I love you, I'm thinking of you) in a symbolic manner, thus being more abstract in the communication. For example, the ForcePhone (Hoggan et al., 2012) or RingU (Pradana et al., 2015) allow users to send tactile messages by squeezing the AHD. Although these haptic signals are abstract in nature, users can use these devices to create their own haptic symbol systems, or “language”. Third and final, there are systems aimed at creating awareness of each other's activities, context, or status (Markopoulos et al., 2009), for example, via physiological- or location-based information. An example of such a system is CoupleVIBE (Blum and Cooperstock, 2016) which facilitates the sharing between two people of such information as activity level, distance to other person and physiological state. Another example is the air-pillow telephone (Iwaki et al., 2008), a pillow that provides force-feedback based on the head movement of one's partner.
Design space as a tool for thinking about design and design processes
One particular critique that has been expressed regarding the field of AHDs is that it lacks a systematic description of its design space, making it difficult to identify, describe, and validate the possible and essential parameters of mediated social touch systems (van Erp and Toet, 2015). Instead, the bulk of the work in the domain has focused on designing prototypical devices, including the ones mentioned above. Each such prototype has provided valuable lessons on what some of the choices are that can be made when designing AHDs, and on how these design choices may affect user evaluations. However, each study in isolation does not provide the type of systematic description of the design space of AHDs called for by van Erp and Toet (2015): They remain point solutions, each describing but a small part of the design space of AHDs. What is lacking is a systematic description of the design space itself—of all choices, good or bad, that can be considered during the design process.
Such a mapping of the design space is important for two reasons. First, it will provide a strong basis for research and design, allowing, for example, for more systematic consideration of potential design options. Second, it will provide a means of combining insights gained from individual point solutions (i.e., existing AHD prototypes). By locating each existing point solution (i.e., AHD prototypes) in this mapped design space, we can, for example, provide an overview of already explored design choices, but also detect parts of design space that are yet to be explored (i.e., terra incognita).
We adopt the term design space from Dennett (2013) and define it as the universe of all possible AHDs, which includes all existing and non-existing devices regardless of whether they provide any value to its user or not (i.e., design failures). The question central to the design process of course is: Where in this vast design space are the functional and valuable AHDs located? Answering this method requires exploring the design space in an efficient manner to discover which system functionalities are most suitable for a given context (Kang et al., 2011; Pimentel, 2017). The present article aims to contribute to this process, not by locating where in the design space the most valuable AHDs are located, but by describing the design space itself. Mapping the AHD design space will allow researchers and designers in the domain to search for value in a more structured fashion.
Research aims
The aim of this article is to map the design space of AHDs in a systematic manner, elucidating the design dimensions underlying these point solutions. For this purpose, we developed a classification system based on general (e.g., bi-directionality and synchrony) and AHD-specific (e.g., actuation type) communication system characteristics. Next, existing AHDs were identified via a systematic review of the literature2, using the following databases: Inspec and ACM Digital Libraries. The conducted search included AHD literature up to 2019. Subsequently, these existing point solutions were classified on each dimension of our classification system. Finally, we empirically explored where in the design space of AHDs, the devices included in our analysis are located. To our knowledge, such a systematic classification of AHDs has not yet been performed. However, similar systematic classifications exist for haptic and force feedback devices (see Haptipedia; Seifi et al., 2019).
By mapping the design space of AHDs, we aim to answer the following research questions:
RQ1: What design choices have to date been made with respect to the communication characteristics of AHDs?
RQ2: How do these design choices differ between AHD types (i.e., MST, symbolic devices, awareness systems)?
By answering these questions, we aim to get a better insight into not just what design choices have been made, but also which communication characteristics seem underused in the literature up to 2019—thus highlighting possible unexplored areas of the design space (i.e., terra incognita). Since the three types of AHDs represent different design intentions, we expect such design choices to differ between them. Hence, the empirical comparisons of the three AHD types on their communication system characteristics are expected to contribute to our understanding of the underlying design choices.
In this study, we will also include AHDs that have been developed as part of an experimental study (e.g., Haans and IJsselsteijn, 2009a) rather than developed as a design artifact (used in for example design research) or commercial device. We found it important to include them in our investigations as experimental devices may perhaps include new characteristics not typically included in design artifacts or commercial devices. However, we acknowledge that these experimental artifacts typically are not designed to be complete communication systems. Instead, they are typically designed to provide a haptic stimulus as part of an experimental manipulation and may lack many of the communication characteristics of design artifacts. For example, the device used in the study by Haans et al. (2014) was developed to test the efficacy of the Midas touch with haptic technology. Although the device facilitated a touch over a distance, it is not yet a complete system (e.g., only one user has the possibility of receiving a touch, purely for the experimental set-up of this study). In these cases, the device is not designed to be functional within a natural communication situation. Therefore, the analysis will be performed twice: Once on all devices (both experimental and design artifacts) and once with the experimental artifacts filtered out. This allows us to investigate how the inclusion of experimental artifacts affected the results.
Classification system based on communication characteristics
Development of the classification system
AHDs can, of course, be classified in a variety of difference ways, for example, according to their technology readiness, their intended use case (e.g., people in a long-distance relationship), or a combination of both. Since AHDs are the first most communication devices, we decided to map the design space of AHDs based on the system characteristics of communication technology (see Table 1). Such a classification system would allow for the kind of systematic investigation of possible design parameters that van Erp and Toet (2015) called for.
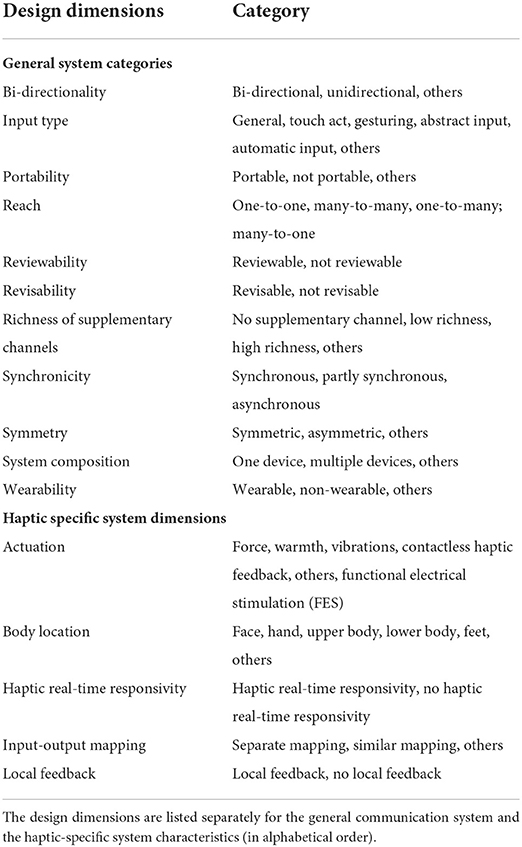
Table 1. Overview of the dimensions and corresponding categories used for mapping the design space of Affective Haptic Devices (AHDs), which were based on communication and system characteristics.
The structure of our classification system is comparable to that of Haptipedia (Seifi et al., 2019) and allows for a similar kind of faceted navigation through the AHD design. The development of the classification system was done through an iterative process. In the first step, we derived an initial set of so-called design dimensions, which represent the system characteristics and communication affordances onto which the AHDs will be mapped.
This initial list was based on the framework developed by Clark and Brennan (1991) and communication dimensions from the media synchronicity theory (Dennis and Valacich, 1999; Dennis et al., 2008), consisting of dimensions such as revisability and reviewability. This initial list was complemented with additional system dimensions available in current-mediated communication technology, such as portability and wearability and with haptic-specific dimensions available in AHDs, such as actuation type and body location. In the second step, we classified the prototypes that we identified through the systematic review on the list of design dimensions, and, while going back-and-forth between the literature on these AHDs and the design dimensions, several changes to the design dimensions and their descriptions were made in order to create clear and unambiguous definitions of the dimensions. This included for example the inclusion of dimensions such as input/output mapping and morphological congruency to better describe and distinguish AHDs.
The end result is 17 more or less exhaustive and mutually exclusive design dimensions, each consisting of two or more categories (the design characteristics; see Table 1). In the remainder of this article, we use the term system to refer to the AHD prototype as a whole and use the term device to indicate one part of this system, for example, the InTouch (Brave and Dahley, 1997) is a system consisting out of two interconnected devices. In the following section, we will provide a detailed description of each design dimension and associated categories.
General system categories
Bi-directional
A bi-directional communication implies that individual users are able to both send and receive a haptic message. This facilitates reciprocal communication where if someone sends a haptic message, then the receiver is able to respond by means of a haptic message (Chang et al., 2002). In determining whether or not a device could be labeled as bi-directional, we first must consider the intention of the design. For example, although the Feelybean (Kontaris et al., 2012) was user tested in a uni-directional context (i.e., one person sending, the other receiving), it was mapped as bi-directional in our design space because it was designed to function as such. In cases, where the design intentions were unclear, we classified the prototype according to the experimental context or use scenario in which the device was tested. For example, in Haans and IJsselsteijn (2009a), the system was mapped as unidirectional as the experiment did not allow for the participant to send a haptic message back after receiving one. An example of a system classified as bi-directional is InTouch (Brave and Dahley, 1997). InTouch consists of two connected devices, each with three rollers. If one user moves the rollers of his or her device, the other person will feel their rollers move in a similar fashion, and vice versa. Examples of systems classified as “uni-directional” include, for example, the device developed by Israr and Abnousi (2018) who in their experimental set-up did not offer all users to both send and receive a haptic message.
Systems that were designed to function both in a bi-directional and a uni-directional mode were classified as “other”. An example of such a system is the Hug Shirt3. In the uni-directional mode, one user can use his or her cell phone to activate the hug shirt worn by their communication partner. In the bi-directional mode, both communication partners wear a Hug Shirt which then acts as both input and output devices.
Input type
This dimension distinguished devices according to how the haptic message is transmitted. We identified six different categories: general, touch act, gesturing, abstract input, automatic input, and others. We classified systems as “general” when input can be provided in a general way, for example, by pressing a button (e.g., InTouch; Liang et al., 2013) or, in the case of the Thermal Hug (Gooch and Watts, 2010), by clicking a button with your mouse or typing a specific keyword and clicking send.
Systems were classified as “touch act” if the input type resembled a social touch act such as kissing (e.g., Kissenger; Zhang et al., 2016) or placing a hand on a hand imprint (e.g., Hotmits; Gooch and Watts, 2010). Moreover, systems could be classified under “gesturing” when input can be provided by performing gestures (e.g., Hand Shaking Model; Abe et al., 2008). Systems that did not fall under any of these categories and where input was more open to the interpretation of the user (in contrast to for example general input types that resemble well-known ways of providing input) were classified as “abstract”. These consisted of systems where input is provided by, for example, shaking a device (e.g., Strong and Gaver, 1996), moving a handle (e.g., Shanken, 2000), squeezing a device (e.g., Huggy; Teh et al., 2009), or by using a force-feedback device (e.g., Bailenson et al., 2007).
Systems were classified as “automatic” if the user does not have to provide an input consciously (e.g., by pressing a button), but rather output is sent automatically in the background through sensors in the system for example, through an accelerometer (e.g., FEELABUZZ; Tünnermann et al., 2014) or physiological measurements (e.g., AWE Goosebumps; Neidlinger et al., 2017). There are also systems that have multiple options for input type. We classified such systems as “other”. An example of such a system is the AHD used by Ipakchian Askari et al. (2019), as the aim of the study was to investigate the influence of the input device. A system was used where input could be provided by tapping a touchscreen (the touch screen contained buttons with different body locations as labels) or by stroking a morphologically congruent input device (i.e., a rubber hand), depending on the condition.
Portability
This dimension distinguishes devices according to their portability, meaning whether or not the users can easily take the device with them and use it outside of their homes. Examples of systems classified as “portable” are Bond touch (see text footnote 1) and POKE (Park et al., 2013). Both are wearables (which we define later as worn on the skin) facilitating the use of the AHD outside of their home. Although a portable device can be wearable, this is not a prerequisite (e.g., LoveBomb; Hansson and Skog, 2001). Systems lacking the characteristics of portability are classified as “not portable”. The Air-Pillow (Iwaki et al., 2008) and Telephonic Arm Wrestling (Shanken, 2000) are two examples of non-portable systems, both devices are not easily transported and require power cords, therefore preventing portable use.
Systems with a portable component as well as a non-portable one (e.g., a home station) are classified as “other”; an example of this is Cubble (Kowalski et al., 2013).
Reach
The design dimension reach represents how large the reach of a haptic message is, i.e., to how many recipients one can send a message to or receive a message from. This can be classified as “one-to-one”, as is the case with RingU (Pradana et al., 2015). RingU consists of a pair of rings used to send a symbolic haptic message between two persons. However, communication can also be classified as “many-to-many”, as is the case with Lovebomb (Hansson and Skog, 2001). With Lovebomb users can send anonymously a happy or a sad haptic message to fellow users located in the same radius of the sender of the message. Furthermore, Lovebomb users receive haptic messages from multiple users. Additionally, an AHD can also be classified as “one-to-many” or “many-to one”. However, none of the devices identified in our prototype search were classified as one-to-many4 or many-to-one.
Reviewability
Reviewability specifies whether the system offers the possibility to replay the haptic message after receiving it (Clark and Brennan, 1991; Dennis and Valacich, 1999). We classified systems with this feature as “reviewable” and systems without such a feature as “not reviewable”. Reviewability means that a message is stored and can be replayed multiple times by the receiver. Storing a received message for it to be played at a later, perhaps more convenient time (e.g., as is the case with the Hug; DiSalvo et al., 2003) is in itself not sufficient for a system to be reviewable, as reviewability requires the storing of the message for replaying it more than once. Rather, this is a feature of the design dimension synchronicity, which is described later in this section. Feel Messenger (Israr et al., 2015) is an example of a reviewable application. The Feel Messenger application facilitates users in sending textual and haptic messages to one another through the IM interface. The messages can be replayed after opening.
Revisability
The dimension revisability is defined as the possibility in an interface to modify a haptic message prior to sending it (Clark and Brennan, 1991; Dennis and Valacich, 1999). We classified systems with this feature as “revisable”. An example of such a system is FootIO (Rovers and van Essen, 2006). The FootIO is an instant messaging system in which text messages can be complemented with a haptic message by using so-called Hapticons (comparable to smileys), each of which represents a predefined set of vibration patterns. When composing the message users can select and revise their choice of these Hapticons before sending. Systems who do not facilitate this feature of revisability were classified as “not revisable” (e.g., SqueezeBands; Yarosh et al., 2017).
Richness of supplementary channels
The haptic communication channel can be accompanied by other modalities (e.g., a video or audio channel). The dimension “Richness of Supplementary Channels” specifies whether an additional communication channel exists beside the haptic one, and, if so, what level of richness is provided through these channels. Supplementary communication channels can provide contextual information that may aid in the interpretation of the tactile message, such as about the mood of one's communication partner. This seems particularly important for MST, as research has demonstrated that affective responses to MST are moderated by such contextual cues as facial expression (Harjunen et al., 2017) and the tone of a textual message (Ipakchian Askari et al., 2020). AHDs that provide supplementary channels high in richness (e.g., real-time video, audio, or a text chat) were classified under “high richness supplementary channel”. An example of such a system is The Remote Handshake (Nakanishi et al., 2014) which offers users, beside the haptic channel, also a video and audio channel. Another example is HaptiHug (Tsetserukou, 2010), an add-on for the virtual environment Second Life.
The tactile channel can also be supplemented by less rich channels, such as consisting of emoticons or animations. An example of a device providing such a type of supplementary channels is Cubble (Kowalski et al., 2013), where users can receive feedback in the form of light or animations, which do not provide rich contextual information. We classified such systems under “low richness supplementary channel”. Systems that offer a combination of high- and low-richness complementary channels were classified under “other”. An example of such a system is the Hug (DiSalvo et al., 2003) where the haptic message is accompanied by real-time audio as well as customizable lights and sounds that can communicate the status of the communication (indicating for example that one is not at home).” Systems that do not offer a supplementary communication channel were classified as “no supplementary channel”. An example of this is the TaSST (Huisman and Frederiks, 2013), which uses only a tactile channel for communication.
Synchronicity
In synchronous communication, the haptic message is received directly after the message is sent without any noteworthy delay (Dennis et al., 2008). A system is classified as “synchronous” when the system sends the message directly after input and is received directly by the user. Direct thus means that the message is intended to be directly received (disregarding unintended delays, or lag, in the communication, as for example due to network issues). An example of a synchronous system is Flex-N-Feel (Singhal et al., 2017). Flex-N-Feel consists of a pair of gloves developed for couples in a long-distance relationship. The gloves facilitate communication with their partner through a haptic channel. Partners can send a signal by flexing their hands. This signal is then directly sent to the receiving end. In our classification system, AHDs can also be classified as “partly synchronous”, i.e., systems allow for synchronous communication as long as the user is present and willing to accept the message. An example of such a system is Haptic Text Messaging (Mullenbach et al., 2014). Although the message is directly sent the receiving end can decide to open the message at a later moment of time. Another example of when a system is classified as “partly synchronous” is when a message is stored when the receiving end is not present at the time the haptic message is sent, for example, as possible with the Hug (DiSalvo et al., 2003). The Hug facilitates users in sending haptic messages in real-time. However, when the receiving end is not present, the haptic message is stored.
Under the classification “partly synchronous”, we also included systems where users have to grant permission before accepting the haptic message. For example with the system HKiss (Rahman and El Saddik, 2011). HKiss is a Second Life add-on enabling users to send one another a kiss. When a user sends a kiss, the receiving end first needs to accept this kiss. Other examples of systems classified as partly synchronous are systems where both users first need to hold the device before a haptic message is sent. An example is Hotmits (Gooch and Watts, 2012) where both users have to place their hand on a hand imprint, after which both imprints warm up. Other examples of partly synchronous devices are systems sending a message at a fixed time period, for example, every 12 s (Blum and Cooperstock, 2016).
We classified systems as “asynchronous”, when a haptic message is not directly sent but a recorded haptic message is always played back (e.g., in the case of using Mid-Air Haptics; Obrist et al., 2015). While partly synchronous systems could still facilitate a form of synchronous communication if answering the messages happens at a fast pace, this is not possible with an asynchronous system. Such systems have too big of a lag to facilitate such communication.
Symmetry
Symmetry refers to whether or not the sender and receiver have access to the same modalities (e.g., text, touch, audio) within the system. Consequently, for a device to be classified as “symmetric,” it needs to be bi-directional. An example of a symmetric device is POKE (Park et al., 2013), which enables users to enrich their phone conversations with haptic messages. The sender and the receiver have access to similar modalities (i.e., both can receive and send haptic messages during the conversation). While symmetric systems are by definition bi-directional, the opposite is not necessarily the case. Although no such systems were uncovered in our prototype search, a bi-directional system can be designed to be asymmetrical, for example, when only one of the two users has access to real-time video. However, we did not find such a system in our prototype search. Also note that symmetry does not mean equality in other functionalities. The Cubble (Kowalski et al., 2013) for example consists of a home station and an app on your phone. Depending on which of the two applications is used for sending/receiving a haptic message, the functionalities of the system differ. However, the system is symmetrical as the sender and the receiver have the same modalities at their disposal (in this case tactile modality in combination with light and animations).
Systems without the feature of symmetry are classified as “asymmetric” (e.g., Wikstrom et al., 2017). It should be noted that although symmetry allows both users to have access to the same modalities, this does not necessarily mean both users make use of similar modalities in a communication, for example, a person can decide to turn off the webcam while the person on the other side has turned it on.
Systems that are designed to support both asymmetric and symmetric modes of communication are labeled as “other”. An example of such a device is the system proposed by Guo and Sourin (2018). They designed a system for haptic interaction during video calls, which allows both users to send and receive haptic feedback. However, they also proposed a set-up where only one user received haptic feedback.
System composition
The design dimension system composition represents whether both input and output components are present on one and the same device, or whether the system consists of two separate devices (one for input and one for output). Systems composed of one device are classified as “one device”. InTouch (Brave and Dahley, 1997) is a system classified as being composed of one device. However, there are also systems that consist of separate components for sending and receiving. These are classified as “other” (e.g., the Huggy pajama; Teh et al., 2012).
We classified systems where input/output can be provided through one single device as well as multiple devices as “other”. Cubble (Kowalski et al., 2013) is an example of such a device. Cubble consists of two components: a home station and a phone application. Input and output can be provided/received on either one of these devices.
Wearability
The design dimension wearability represents whether users are able to wear the AHD on their body as a bracelet (e.g., FeelHey5, necklace (Wiedau et al., 2015), sleeve (Israr and Abnousi, 2018), jacket (e.g., HugME; Cha et al., 2009), scarf (Pfab and Willemse, 2015), etcetera. Systems containing this feature are classified as “wearable”. The difference between wearability and portability is the fact that the device is worn on the skin, for example, Comtouch (Chang et al., 2002) is classified as “portable” as it can easily be brought along. However, as the device is not worn on the body it is classified as “non-wearable”. Portability is not a prerequisite for wearability. It is possible that a system is not portable, while actuation is provided through a wearable component, for example in the case of SqueezeBands (Yarosh et al., 2017).
Systems consisting of a wearable and non-wearable element are classified as “other”. An example of such a device is Intimate Transactions (Hamilton, 2007), where users receive haptic feedback through a haptic pendant (i.e., a wearable) and a smart chair (a non-wearable component).
Haptic-specific system categories
Actuation
This dimension distinguished devices according to the type of actuation used for the haptic or tactile message. Systems providing a haptic message through kinesthetic feedback (e.g., using force-feedback as in Telephonic Arm Wrestling; Shanken, 2000) or pressure (e.g., by means of air inflation as in Huggy Pajama; Teh et al., 2009) are classified under “force”. Systems using warmth as an actuator are classified as “warmth” (e.g., Hothands and Hotmits; Gooch and Watts, 2012), and systems using vibrations motors are classified under “vibrations” (Darriba Frederiks et al., 2013). Systems where haptic feedback is provided through functional electrical stimulation (FES; e.g., Nishida et al., 2015) are classified as “FES”. AHDs can also provide haptic and/or tactile feedback without the actuator being attached or placed on the skin. These systems are classified as “contactless haptic feedback” for example, mid-air haptic (Makino et al., 2015) or a magnetic field (e.g., Wiedau et al., 2015). We classified systems that make use of multiple actuation types as “other”, as is, for example, the case with Vibrobod (Dobson et al., 2001). The Vibrobod uses both warmth and vibration.
Body location
Haptic messages can be provided on various body locations, and some devices may address more than one body location. For system addressing a single location on the body, the following labels were used: “The face” (e.g., CheekTouch; Park et al., 2012), “hand” [this also included touches experienced on the fingers; e.g., HB Ring (see text footnote 1)], “upper body” (this includes touches on the stomach, torso, shoulder or forearm; e.g., TaSST; Huisman and Frederiks, 2013), “lower body” (this includes abdomen and legs; e.g., Blum and Cooperstock, 2016) and “feet” (e.g., ComSlipper; Chen et al., 2006). However, some systems provide haptic stimulations on multiple body sections (e.g., Stitchies; Stenslie et al., 2013) or the body location is dependent on where the device is placed or hold (e.g., FEELABUZZ; Tünnermann et al., 2014). For a system that addressed more than a single location of the body, we used the label “other”.
Haptic real-time responsivity
When engaging in a naturalistic touch act (e.g., a handshake), one experiences immediate haptic feedback by means of the resistance of the skin or the response of the other. Such immediate haptic feedback can also be provided in a mediated setting and is defined as haptic real-time responsivity to haptic inputs. Systems where users feel real-time resistance to their own actions, thereby receiving feedback from the other are classified as “Haptic real-time responsivity”. An example of a system facilitating haptic real-time responsivity is Telephonic Arm Wrestling (Shanken, 2000), enabling users to arm-wrestling with one another over a distance. During arm-wrestling, both users feel resistance linked to the force that is exerted by their interaction partner.
Systems providing haptic real-time responsivity to only the sender of the touch, for example, a sender receiving feedback based on the body location of the receiver is also classified as haptic real-time responsivity. An example is HugMe (Cha et al., 2009), where only the sender of the touch received haptic real-time responsivity based on the body location that is touched. Systems do not need to apply haptic real-time responsivity through the actuation type “force” to be classified under haptic real-time responsivity. Systems using vibration to provide haptic real-time responsivity are also classified under haptic real-time responsivity. An example of such a system is Haptic Virtual Touch (Mullenbach et al., 2014), which is an application where users can remotely draw on a tablet. If both the fingers of both users intersect, then a haptic pattern is felt.
For a system to be classified as having haptic real-time responsivity, it does not require input and output to be present on one and the same device. Even though sending and receiving take place on two different devices the signal for sending and receiving between users can still be provided to users by haptic real-time responsivity. An example of a device with this feature is Hand Shaking Model (Abe et al., 2008), although the device has two separate devices for sending and receiving the user still experiences haptic real-time responsivity based on the input given by the other end. Systems that do not contain haptic real-time responsivity were labeled as “no haptic real-time responsivity.”
Input/output mapping
The design dimension Input/Output mapping represents whether or not the AHD uses the same haptic display to provide both input and output. Meaning, participants can both feel and send a signal through the same part of the device. In our classification, such systems are classified under “similar mapping”. InTouch (Brave and Dahley, 1997) is an example of such a system where input is provided by means of moving rollers, and output is also experienced through these rollers.
There are also systems where input/output mapping is at separate locations, these systems are classified as “separate mapping”. An example of such a system is Bond touch (see text footnote 1). Bond touch is a bracelet used to send haptic messages by tapping on the front of the bracelet. Output is provided through vibrations. In the case of Bond touch input and output is provided through separate parts of the system.
Systems where input/output mapping can be similar as well as dissimilar are classified as “other”. The article by Guo and Sourin (2018) describes an example of such a system. A force-feedback device provides the input and output for this device. However, the input can also be provided by making hand gestures, resulting in a system with separate input/output mapping.
Local feedback
The dimension of local feedback classifies whether systems provide the sender of the message with feedback on the intensity of the haptic signal they transmit (Chang et al., 2002). With some input types, such as a dial or slider, the user may already have some intuition as to the intensity of the haptic message they sent, as there is a visible minimum and maximum of the intensity range. Systems that offer real-time responsivity often give the user direct feedback as to the intensity or force they apply. An example of such a system is InTouch (Brave and Dahley, 1997). The InTouch consists of two devices, each with three physically coupled rollers. If a roller on one device is rotated, then the corresponding roller on the other device will rotate in the same way, thus offering the illusion of interacting on the same device. Providing real-time responsivity in this case, hence, also provides local feedback. For other input types, for example, those based on the force that a user applies to an input sensor, some form of local feedback may be required to determine the intensity of the haptic message that is being transmitted. An example is the ForcePhone (Hoggan et al., 2012), where the sender receives visual information about the relative pressure level of their haptic message. Devices that provide some form of local feedback—such as those described above—were classified as “local feedback.” AHDs which do not offer local feedback were classified as “no local feedback.” An example is the Vibrobod (Dobson et al., 2001). When applying pressure to the device to send the haptic message, the user has little to no information on how intense the tactile message will be. Devices such as the Vibrobod were classified as “no local feedback”.
Morphologically congruent input
The design dimension morphologically congruent input represents whether or not the system makes use of an input medium representing a human form (e.g., a hand or a mannequin)—a so-called morphologically congruent input (see Ipakchian Askari et al., 2019). Systems with such a feature are classified as “morphologically congruent” and those without as “morphologically incongruent”. HaptoClone (Makino et al., 2015) is an example of a system with a morphologically congruent input. HaptoClone enables users in sending a haptic message by touching a cloned image of the person at the other end. Under morphologically congruent input we do not include devices where users provide input through a wearable device placed on their body for example, as is the case with TaSST (Huisman and Frederiks, 2013), where input is provided on a sleeve attached to the user's arm. While these systems might facilitate an input that resembles a touch act, the form factor of the input device itself is not a human form (e.g., in the case of TaSST a sleeve). The input needs to be applied directly on a morphologically congruent input device. There are also systems that have the option of either a morphologically congruent or incongruent input (e.g., the system used in Ipakchian Askari et al., 2019). Such systems are classified as “other”.
Mapping the design space of AHDs
Method
Two scientific databases were used to find relevant AHDs: Inspec and ACM Digital Libraries. These two databases were selected since they include the publishers, such as Springer, ACM, and IEEE. Taken together these publishers ensure that we included in our search various mayor journals (Virtual Reality; IEEE Virtual Reality; Frontiers of Computer Science; IEEE transactions on Haptics; Presence; Journal of Nonverbal Behavior) and conference proceeding series (IEEE Haptics symposium, including WorldHaptics; the ACM conference on Computer-Human Interaction, or CHI; EuroHaptics; AsiaHaptics; International Conference on Multimodal Interfaces; Machine Learning for Multimodal Interaction; Intelligent User Interfaces; Tangible and Embedded Interaction; UBICOMP). Based on a screening of articles discussing AHDs it was found that the majority of the AHD prototypes are described in these sources. The reviews from Haans and IJsselsteijn (2006) and Huisman (2017) were scanned for additional devices. For both databases a query was composed to identify publications that include in the abstracts keywords related to three categories: remote (virtual OR tele* OR remote OR distance OR mediated) AND affective (interpersonal OR social OR affective OR communication OR intimate) AND haptic (touch OR haptic* OR tactile). As this article only focuses on AHDs for human-to-human communication the query was extended to exclude entries that contained the keyword robot* in the title.
The search was conducted on July 2, 2019. We did not set a time limit to the time span of the published research, and the oldest article included in our selection was dated 1996 (i.e., Strong and Gaver, 1996). For a visualization of the different categories of AHDs present plotted over time please see Figure B.1. The database search resulted in 786 entries for ACM and 859 entries for Inspec. This selection was further narrowed by manual inspection of the titles and where needed abstracts or full texts. The selection process used the following inclusion criteria:
1. Work that is focused on creating or testing a device allowing human-to-human affective communication through a haptic channel (e.g., warmth, vibration, and/or force).
a. For work regarding virtual collaboration systems, the abstract is read. Work is included if the device allows for affective interaction through a haptic channel.
b. Work discussing devices developed for enhancing communication for blind individuals is excluded.
c. Awareness systems were included if they explicitly mentioned a haptic channel and are aimed at personal purposes and not work-related purposes.
2. The article must describe enough detail about the AHD to allow it to be classified according to our classification scheme.
a. The article needs to discuss both the input and output sides of the device.
b. Articles should not be overly abstract or conceptual in describing the device.
When articles discussed the same or a highly similar device (e.g., a further iteration of the same design concept) only the most recent article was included in the analysis. Exceptions were made when the devices differed substantially. An example of such a case was the TaSST. Where in the earlier manuscript (Darriba Frederiks et al., 2013) the TaSST was described with one input possibility, this was extended to two possible inputs in a later manuscript (Darriba Frederiks et al., 2016). In the case that a manuscript described two different prototypes, both were included in the mapping exercise as separate devices. Figure 1 shows an overview of the selection process. The selection of articles was conducted in collaboration with a second rater. In case of doubt, the second rater took a second look. In case of disagreement, discrepancies were discussed, and a final decision was made together. Through this process, a total of 89 AHDs were uncovered that met the abovementioned criteria.
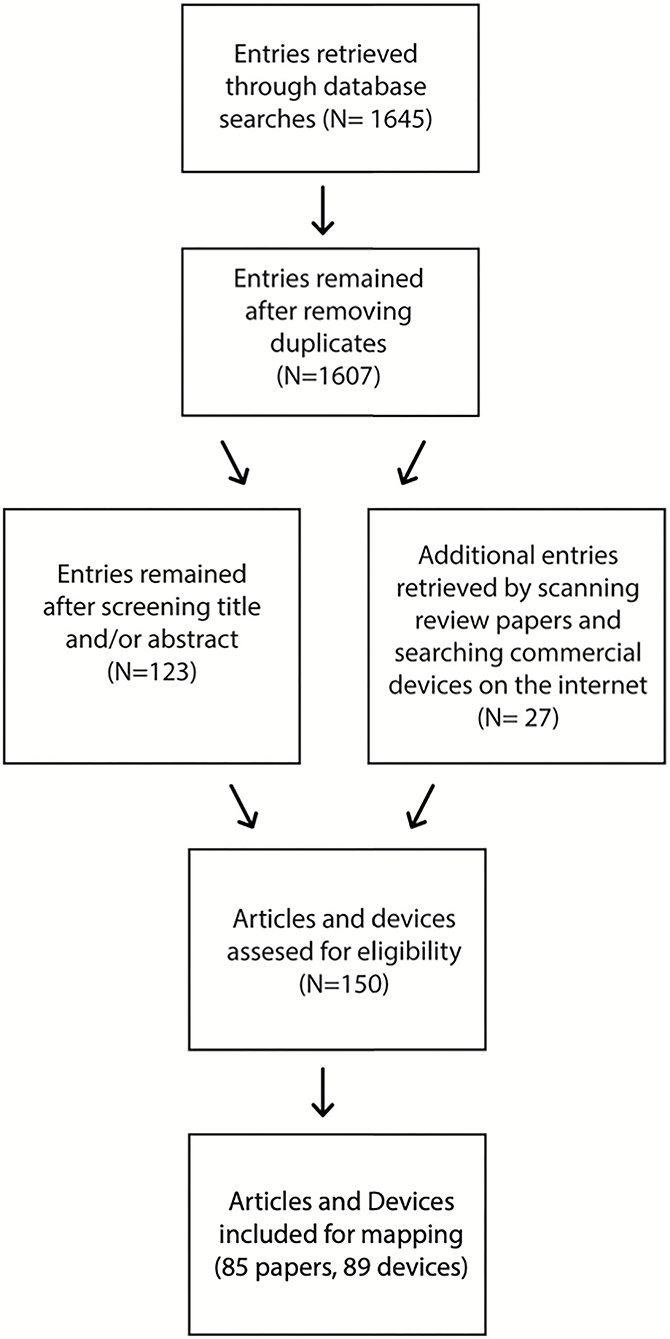
Figure 1. Overview of the selection process for devices to be used for mapping the design space of AHDs.
Mapping of the AHDs into the classification system was performed by the first author. Similar to the selection process, in case of doubt, the second rater took a second look. Disagreements and discrepancies were discussed, and a final decision was made together. In the case that it was not possible to completely classify an AHD (e.g., because information about a concerning design dimension was lacking) we contacted the authors of the articles. if the information was not found this was reported through a missing value. In the Supplementary materials, the final categorization of each of the 89 AHDs is provided as a searchable Excel sheet.
To divide AHDs into three categories, MST, symbolic, and awareness AHDs were sorted based on the primary aim and functionalities of the AHD. We classified AHDs as MTS if the authors of the articles/websites referred to the device as a simulation of natural social touch. AHDs consisting of an automatic input type and/or aimed to create a sense of awareness rather than the communication of haptic messages were classified as awareness. Devices facilitating haptic communication of a more abstract nature were classified as symbolic.
Results
Distribution of AHDs across each of the categories of the dimensions
The classification of the 89 AHDs on each of the dimensions of our classification system can be found in Figure A.2, and consist of the proportion of devices being classified in a certain category (e.g., symmetric) on each dimension (e.g., symmetry). In the remainder of this section, we will highlight only those proportions that we found to be of particular interest.
Out of the 89 devices, most are not revisable (87.5%) and not reviewable (92.0%; see Figure A.2 for an overview). The majority of the systems were synchronous (81.8%) or partly synchronous (15.9%). Regarding the dimension bi-directionally, we found most systems to be bi-directional (73.0%). With respect to reach, we found most (95.5%) systems to be one-to-one and 4.5% many-to-one. The design dimension symmetry consists out of 73.0% symmetric and 24.7% asymmetric systems. With respect to the dimension focused on supplementary information channels, 43.8% of the systems were classified as touch only, 23.6% to complement touch with low richness supplementary channels and 24.7% with high richness supplementary channels.
Regarding the input type most systems used a touch act (31.5%) or a general input type (30.3%), and only 19.1% of the systems had an abstract input and 11.2% an automatic input. Regarding actuation, the majority of the systems (44.9%) used vibrations, 30.3% force, 13.5% others, 6.7% warmth. Regarding the haptic real-time responsivity to haptic inputs dimension, we found 85.2% of the systems to have a no haptic real-time responsivity.
On the dimension body location, most systems had the hand (37.9%) or upper body (35.6%) as body location. On the dimension portability, we found the majority of the systems (57.5%) to be not portable. Finally, 50.6% of the systems were wearable.
The results of our classification systems suggest a typical AHD to be synchronous and bi-directional, to complement the haptic channel with some additional non-touch communication channel (e.g., audio), but lack real-time responsivity, reviewability, and revisability. Since the AHDs here categorized were designed with various functionalities in mind, it is perhaps more interesting to investigate and compare the design choices that are made for the three different types of AHDs introduced at the onset of this manuscript: mediated social touch, symbolic devices, and awareness systems.
Differences per dimension between the three AHD types
To explore for differences in design choices between MST, symbolic devices, and awareness systems, we ran a series of Fisher exact tests (Kim, 2017), one for each dimension of our classification system. In the remainder of this section, we will highlight only those proportions that we found to be of particular interest (for the complete analysis, see Table 2).
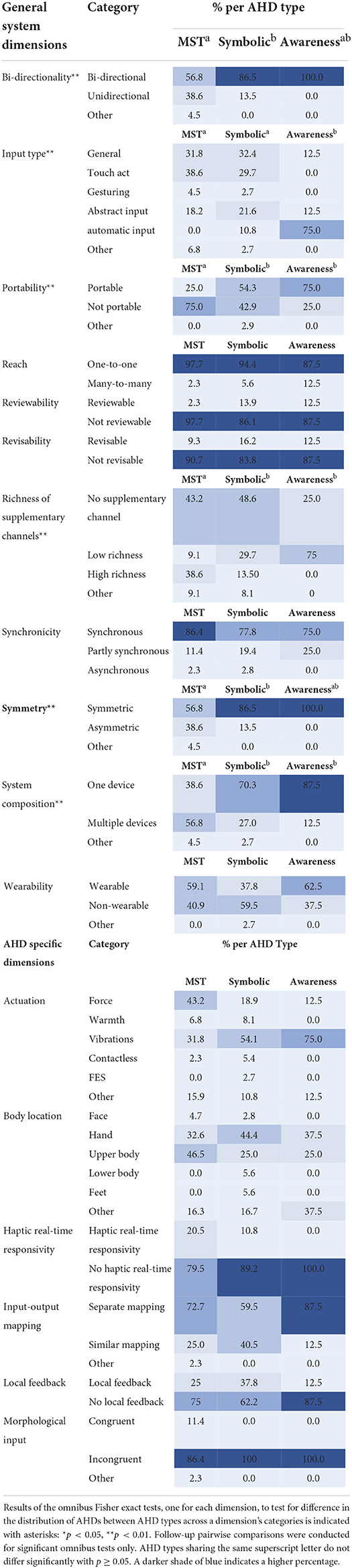
Table 2. Distribution of all 89 AHDs (in %) across the categories of each design dimension separated per AHD type: MST (n = 44), symbolic (n = 37), and awareness (n = 8).
The least common type of AHDs were awareness devices with only eight (i.e., 9.0%) of the devices classified as such. About half of the devices: 44 (49.4%) were classified as MST and 37 (41.6%) as symbolic. Results showed that for the design dimensions bi-directional, symmetry, the richness of supplementary channels, input type, system composition and portability there was a significant difference (p < 0.01) between the three AHD types (see Table 2 and Figure 2 for an overview of the results). A follow-up Fisher Exact test was conducted for each of these design dimensions to further explore differences using a pair wise comparison of two AHDs: MST vs. Symbolic, MST vs. Awareness, and Symbolic vs. Awareness.
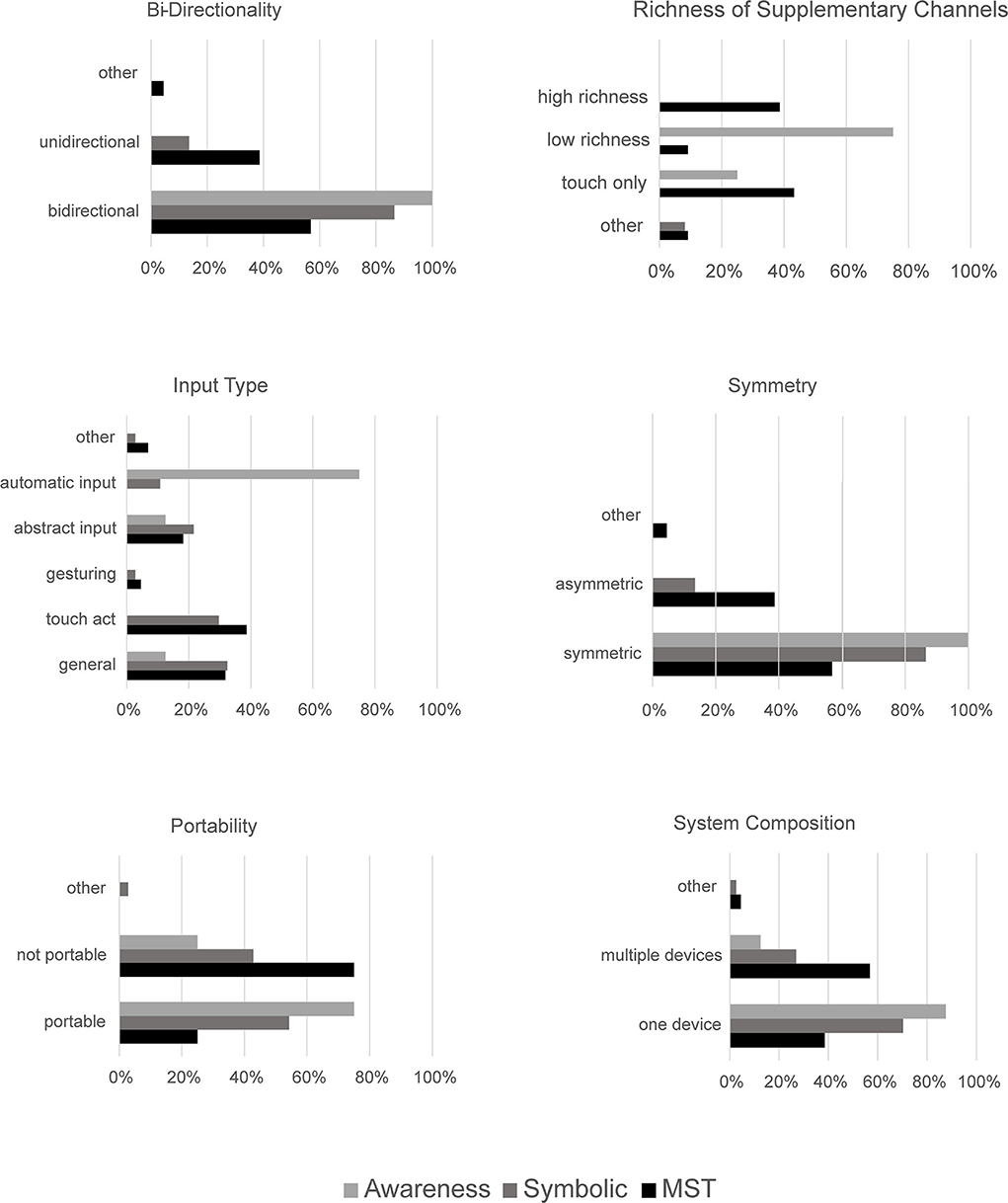
Figure 2. Bar graphs of the dimensions which were found to have significant differences between the types of AHDs. Each bar represents the percentage of devices within a certain AHD type classified to have that design characteristic.
For the dimension bi-directionality, there was a significant difference between MST and symbolic (p = 0.008). The characteristic bi-directionality occurred more often in symbolic devices (86.5%) than in MST devices (56.8%). Additionally, unidirectional devices were more prominent in MST (38.6%) than in symbolic devices (13.5%). Regarding the dimension, symmetry results showed a significant difference between MST and symbolic devices (p = 0.008): MST devices were more often asymmetric (38.6%) compared to symbolic devices (13.5%). Additionally, symbolic devices were more often symmetric (86.5%) compared to MST devices (56.8%).
On the richness of supplementary channels dimension, we found significant differences between MST vs. awareness (p = 0.001) and MST vs. symbolic (p = 0.02). More MST devices (43.2%) were touch only as compared to awareness devices (25%).
Complementing a touch channel with low-richness supplementary channels occurred more often in awareness (75%) and symbolic devices (29.7%) than in MST devices (9.1%). Complementing touch with high-richness supplementary channels, however, was more prominent in MST (38.6%) than in symbolic (13.5%) and awareness devices (0.0%). For the design dimension input type, there was a significant difference between awareness vs, symbolic (p = 0.005) and MST vs. awareness (p < 0.001). As is to be expected, automatic input occurred more often in awareness devices (75.0%) as compared to symbolic devices (10.8%) and MST devices (0.0%). Additionally, general input and touch input occur more often in symbolic (32.4% general input and 29.7% touch act) and MST devices (31.8% general input and 38.6% touch act) than in awareness devices (12.5% general input and 0.0% touch act).
On the dimension portability, results showed a significant difference between MST vs. symbolic (p = 0.007) and MST vs. awareness (p = 0.011). Not-portable devices occurred more often in MST devices (75.0%) than in symbolic (42.9%) and awareness devices (25.0%). Additionally, portability occurs more often in symbolic (54.3%) and awareness devices (75.0%) than in MST devices (25.0%).
To examine whether or not the inclusion of experimental devices in the analysis may have affected outcomes, we repeated the analysis with all experimental devices excluded (i.e., including only the design artifacts). With 20.2% of the devices in the original dataset being labeled as experimental, a sample of 71 design artifacts remained for these analyses. The exclusion of the experimental AHDs did not change the interpretation of the findings, except for the dimension system composition. With the experimental artifacts excluded the difference between MST and awareness devices was no longer significant with p = 0.17. The difference between MST and symbolic devices remained significant with p = 0.012 (see Table A.1 for an overview of these results).
Discussion
Over the past few years, there has been a rise in the development of AHDs, resulting in many prototypical devices described in the literature. As valuable as each of these point solutions is in exploring the possibilities when designing AHDs, they each describe but a small part of the AHD design space. As argued by van Erp and Toet (2015), the field lacks a systematic investigation of the possible parameters to consider when designing and researching AHDs. Therefore, the aims of the present study were (a) to develop a classification system based on general (e.g., reviewability) and AHD-specific communication characteristics (e.g., type of actuation) with which to map the design space of AHDs, (b) to identify existing AHDs in the literature, and classify these point solutions according to this classification system, (c) to empirically explore where in the design space of AHDs the devices included in our analysis are located—and thus to identify what design choices are most popular—, and (d) to elucidate differences in these design choices across different types of AHDs: MST, symbolic systems, and awareness systems.
The location of included devices in the design space of AHDs
Regarding RQ1, our analysis of the distribution of AHDs across each of the categories of the dimensions revealed that several design characteristics were proportionally underrepresented in our set of AHDs including, for example, devices employing unidirectionality or asymmetric communication. Similarly, various input modes and actuation types were underrepresented, including automatic input, gesturing, multiple input types, FES, and contactless haptic feedback. Additionally, the categories with multiple options for input/output mapping and similar mapping were underrepresented. AHDs which were both symmetric as well as asymmetric, in particular, were rarely observed. Communication via media technology allows for unique possibilities not present in face-to-face interaction, such as reviewability, revisability, and one-to-many reach. Still, relatively few AHDs were classified to possess such characteristics.
From the latter analysis, it is impossible to tell why certain design choices are underpopulated in the set of AHDs included in our study. One possible explanation may be that some of these design characteristics are deemed unsuitable for AHDs. In their review, Haans and IJsselsteijn (2006) argued that many designers of AHDs make reference to the importance of social touch in every day live. A naturalistic social touch is not revisable (it is to think before you act), not reviewable, and typically one-to-one (group hugs are an exception). It is perhaps because of this ever-present linkage between AHDs and naturalistic social touch, that categories such as reviewability, revisability, and one-to-many remain rare. However, if this is indeed the case, then we would expect differences in the use of these characteristics between AHD types as the linkage with natural social touch is probably stronger in MST that aims to simulate social touch. However, when comparing MST with symbolic and awareness systems, the latter two were not found to be more often reviewable or revisable.
A second possible explanation could be the technical difficulties that arise when certain design choices are made. Categories such as automatic input, gesturing as input or contactless haptic feedback, FES, similar input-output mapping, or hybrid in wearability add a considerable level of technical complexity to AHDs; resulting, for example in more sensors, actuators and/or larger online storage facilities, which might prevent designers in using them, especially when wanting to make low-fi prototypes that are easier to adjust based on user-feedback. Of course, the third explanation for these observations is that certain possibilities in the design space of AHDs have simply been overlooked by designers and researchers alike. Put differently, some of the observed underpopulated areas of the design space may have been ignored not because of rational decisions or technical limitations, but because these possibilities were never considered to begin with. Especially for these cases, a systematic mapping of design space, as performed here using communication media characteristics as a classification method, can be useful.
Insights on awareness type of AHDs
The least common type of AHDs was awareness devices. MST devices appeared to be the most common type of AHD, followed by symbolic devices, as can be found in section Differences per dimension between the three AHD types. This was to be expected as in contrast to MST devices, awareness devices are not restricted to the tactile or haptic modality; note that the same applies to symbolic devices. Moreover, awareness devices (whether affective haptic or not) appear in general to be a less explored type of media technology. When looking at the common characteristics for awareness AHDs the results are by and large in line with what one would expect for this type of device. Automatic input seems the appropriate input type for the information send by awareness devices (e.g., physiological signals), as according to our definition communication through such devices go often automatic, meaning users do not have to send a message themselves, rather these are sent automatically through the system.
Findings regarding MST and comparison to other types of AHDs
When focusing in particular on MSTs—which make-up the large majority of the AHDs—, it becomes obvious that certain design choices are, at least in our opinion, at odds with the goal of simulating naturalistic social touch. First, despite a significantly higher proportion of MST devices supplementing the haptic channel with a non-touch communication channel, the absolute number of MSTs classified as having a rich supplementary channel was rather low (i.e., 38.6%). This is surprising as social touch is more than tactile stimulation alone, and is accompanied by a rich set of multimodal cues, for example, physical closeness, facial expressions, and perhaps verbal communications that together establish the intention of the toucher (Haans et al., 2014). Second, and perhaps most strikingly, is that regarding RQ2 in comparison to symbolic and awareness devices—which do not intend to simulate social touch—it was the MST devices that more often lacked fundamental features of face-to-face interactions, such as bi-directionality and symmetry, as was found from the Fisher exact test. This is surprising given the reciprocal nature of social touch. MST was relatively overrepresented, as compared to awareness and symbolic devices, on only two of the more fundamental characteristics of face-to-face social touch: high richness supplementary channel and touch acting as input type.
Possible explanations for design choices made regarding MST devices
As mentioned above, we can only provide possible suggestions as to what explains these observed differences between MST on the one hand and awareness and symbolic AHDs on the other. One logical explanation is that mediated social touch—or any type of mediated communication that has many of the characteristics of a face-to-face interaction for that matter—remains technologically challenging. Simulating social touch in a realistic manner will possibly require more advanced technology in terms of sensors and actuators and therefore also larger installations. We do indeed see in our analysis that MST devices, compared to symbolic and awareness devices, are more often static, not portable and consist out of multiple devices. Apparently, there is a trade-off between portability and realism in designing MST. This may explain the popularity of vibrotactile actuators as they are more easily implemented in portable or wearable devices. Technical complexity may also explain why the use of a morphological congruent input medium is not common, despite previous work suggesting it can influence touch experience (Haans and IJsselsteijn, 2009b; Ipakchian Askari et al., 2019). It may also explain why non-sexual MSTs are currently limited to touches to the upper body and the hand, even though naturalistic social touch can be provided on multiple body locations.
Finally, the technological complexity of the realistic stimulation of touch can also explain why such fundamental characteristics of face-to-face communication, such as bi-directionality and symmetry, are less common in MST as compared to awareness and symbolic devices. It is already difficult enough to allow one person to remotely touch another convincingly, and it may still be too difficult to recreate a reciprocal social touch experience for both. In contrast to MST, symbolic and awareness devices can probably rely on less rich tactile or haptic output and hence can more easily be bi-directional and symmetrical. Another explanation could be that haptic stimulation is less abstract in MST than in symbolic devices. Given the more abstract nature of symbolic information, some of the symbolic devices identified in the literature (such as the InTouch; Brave and Dahley, 1997) require bi-directionality and symmetry as this supports the negotiation of meaning. It may of course also be the case that some designers of MST devices would argue that social touch over a distance is possible even with rather impoverished communication characteristics, such as vibrotactile stimulation, unidirectionality, and asymmetry. More research is needed to confirm whether this is the case, or whether such categories as unidirectionality and symmetry (and hence reciprocity) are fundamental characteristics without which the technological simulation of social touch becomes difficult. Our findings thus raise fundamental questions related to when and why AHDs should be labeled MST. Does the inclusion of a tactile or haptic display in a communication medium suffice? Should a device match all characteristics of social touch, including its reciprocal nature, or should other criteria, perhaps not related to the system characteristics be used (e.g., strong response similarities with naturalistic social touch; see Haans and IJsselsteijn, 2006)? Clearly, more research is needed to answer these questions and to uncover what the essential characteristics of social touch are that need to be reproduced by MST to turn tactile stimulation into a social touch.
Limitations
One limitation of the present study is that our database search has been conducted in 2019. After this period, new research has been published that contains additional AHD prototypes. Hence, the reported findings may not be representative of the state-of-the-art in design choices in the domain of AHDs. However, any type of review article is necessarily retrospective—describes only a fixed window of time in the past. Ours encompasses the beginning of research and design work on AHDs up to 2019, spanning over 23 years of published research. It is important for this type of research to be repeated in the future, as to illustrate how design choices have changed since 2019. The concept of a design space, the proposal to decompose AHDs in different types (i.e., MST, symbolic, and awareness), as well as the developed classification system as put forward in the present article, provides the necessary tools for doing so. This does not mean that this categorization of AHD types, or the developed classification system may not need changes in the future. The dimensions of the AHD design space presented in this study were based on existing communication literature and on an iterative process that aimed to optimize the dimensions and their categories to the differentiation between existing AHDs. This, however, does not guarantee that all communication characteristic dimensions have been uncovered. More and novel types of AHDs may emerge that our classification system may not be able to differentiate amongst. The number of categories per design dimension may need to be expanded, for example, to be able to differentiate between the perhaps increasing number of devices that would now be categorized as “other”. Similarly, additional design dimensions may need to be included. One example of such an additional dimension could be “morphological congruent output” which can differentiate between devices that do or do not provide actuation trough a haptic display that is congruent to the human body; a design choice that is becoming increasingly considered (see, e.g., the Future Affair installation; Dekker et al., 2021).
A second limitation of the present work is that we can neither guarantee that the set of AHDs uncovered in our literature search, although extensive and structured, is complete, nor that it is a representative (and thus unbiased) sample of all AHDs whether prototypical or commercial. Our search, for example, only focused on published literature and did, for example, not include white papers such as JoyHaptics (Tuovinen et al., 2022). It also did not include some of the more technical journals, such as the Journal of Dynamic Systems, Measurement, and Control, which sporadically publishes work on AHDs (e.g., Pedemonte et al., 2017). Similarly, we have not included the proceedings of early editions of such conferences as AsiaHaptics and EuroHaptics. Such omission may have affected the conclusion presented in this article.
A third limitation of our study is that we classified the AHDs on the basis of the communication characteristics that were implemented in the current version of the device, and did not take into account the possible envisioned end product. It may well be the case that various AHD prototypes, especially when in an early stage of development, may not yet have implemented all characteristics as envisioned for the final product. Consider, for example, the Air-Pillow (Iwaki et al., 2008). Being designed as a pillow to be used by geographically separated couples, it is obvious that the authors did intend for the final product to be portable. Nevertheless, we categorized it as non-portable as its current implementation does not easily allow the user to take it along with them. We opted for this manner of classifying the AHDs, as design intentions are not always made explicit in the literature; thus, requiring additional assumptions to be made on our part had we decided to take such intentions into account in the classification process.
A fourth limitation of the present work is that we focused only on communication system characteristics to classify AHDs within the design space. As mentioned in the method section, we could have used other systems, such as based on technology readiness, or the intended use case (e.g., simulating social touch between people in a long-distance relationship, or comforting a loved one in the hospital). Any such classification system would yield other insights than here presented. Future research should focus on other such classification systems, and when more data is available it would be of particular interest to investigate if and why AHDs designed for different use cases have different communication system characteristics. To do so, however, more data need to be available per intended use case than now is the case.
The fifth limitation of our work is that we have not yet provided insights into which combinations of design dimensions represented in our classification system are frequently co-occurring or incompatible with each other. Certain combinations of dimensions, for example, revisability and synchronicity are conflicting. An interesting analysis for future research is to explore such correlations between system characteristics. This, however, requires a larger dataset.
Finally, in the current article, we distinguished and made comparisons between three different types of AHDs, namely MST, symbolic devices, and awareness systems. We felt such distinction between different AHD types to be necessary as they present different design intentions that may lead to specific design choices. Although perhaps sufficient for the analysis here presented, we do acknowledge that the three proposed AHD types may be somewhat of an oversimplification, and that additional (sub-)categories, for example within the varied class of symbolic devices, may exist. More work is needed to further describe the different functionalities that AHDs can offer. The three types of AHDs here proposed are an important starting point, as it makes explicit that there is more to AHDs than the simulating of social touch, and thus that references to natural social touch are not always necessary when designing or commercializing an AHD.
Conclusion
The design space of AHDs is the infinite universe of possible AHD designs and includes amongst the non-functional designs those designs that can bring actual value to people. Over the years, various prototypical designs have been suggested in the literature, each of which is valuable for unveiling a small part of the design space—unveiling a small part of the possible design choices that can be made. In the present article, we aimed to locate these point solutions in the AHD design space, with the aim to provide a more systematic overview of the design choices considered up to 2019, but also to highlight those design possibilities that for various reasons have not yet been described. For this purpose, we proposed a multi-dimensional classification system based on media system characteristics. Our analysis pointed to various system characteristics being underrepresented in existing AHDs, in particular characteristics that are rather unique for mediated communication, such as revisability, reviewability, and one-to-many reach. Another finding was that, compared to symbolic and awareness AHDs, the MST devices in our dataset more often lacked some key characteristics of social touch in face-to-face interactions. Although we could only suggest potential explanations for these and other findings, our mapping exercise revealed various future research and design directions that can be addressed. Identifying and answering such questions is important to find value in the vast design space of AHDs. We hope that the current article contributes to this.
Data availability statement
The raw data supporting the conclusions of this article will be made available by the authors, without undue reservation.
Author contributions
SI conducted the prototype search, mapped the AHDs into the classification system, performed all the statistical analyses, and wrote the first draft of the manuscript. AH acted as the second coder for the mapping of the AHDs into the classification and contributed to writing the draft of the manuscript. SI and AH composed the selection and exclusion criteria for the prototype search. SI, AH, and WI developed the classification system. All authors contributed to the writing of the manuscript.
Conflict of interest
The authors declare that the research was conducted in the absence of any commercial or financial relationships that could be construed as a potential conflict of interest.
Publisher's note
All claims expressed in this article are solely those of the authors and do not necessarily represent those of their affiliated organizations, or those of the publisher, the editors and the reviewers. Any product that may be evaluated in this article, or claim that may be made by its manufacturer, is not guaranteed or endorsed by the publisher.
Supplementary material
The Supplementary Material for this article can be found online at: https://www.frontiersin.org/articles/10.3389/fcomp.2022.795772/full#supplementary-material
Footnotes
1. ^Bond Touch. (n.d.). Retrieved from: https://bond-touch.com/ (accessed March 25, 2021); HB Ring. (n.d.). Retrieved from: https://thetouchx.com/hbring/index.html (accessed February 5, 2021).
2. ^In our mapping we have disregarded haptic technologies developed for sexual interactions. Although such technologies can be regarded as AHDs, our focus was on creating a classification system of AHDs facilitating non-sexual forms of social touch for multiple relationship types (e.g., friend, partner and family). For interested readers, see Döring (2020) for examples of AHDs facilitating sexual interactions.
3. ^Hug Shirt. Available at: https://cutecircuit.com/hugshirt/ (accessed December 3, 2020).
4. ^Although not present in our database search output, there is an existing AHD that facilitates one-to-many, see TapTap (Bonanni et al., 2006).
5. ^FeelHey. (n.d.). Retrieved from: https://feelhey.com/collections/hey-touch#gref (accessed March 29, 2021).
References
Abe, T., Ogawa, T., Ogawara, M., Hirano, M., and Tanaka, K. (2008). “Tangible-3D,” in Proceeding of the Twenty-Sixth Annual CHI Conference Extended Abstracts on Human Factors in Computing Systems - CHI '08 (New York, NY: ACM Press). doi: 10.1145/1358628.1358674
Bailenson, J. N., Yee, N., Brave, S., Merget, D., and Koslow, D. (2007). Virtual interpersonal touch: expressing and recognizing emotions through haptic devices. Hum. Comp. Interact. 22, 325–352. doi: 10.1080/07370020701493509
Blum, J. R., and Cooperstock, J. R. (2016). “Expressing human state via parameterized haptic feedback for mobile remote implicit communication,” in Proceedings of the 7th Augmented Human International Conference 2016, Vol. 25 (New York, NY: ACM), 1–2. doi: 10.1145/2875194.2875225
Bonanni, L., Vaucelle, C., Lieberman, J., and Zuckerman, O. (2006). “TapTap,” in CHI '06 Extended Abstracts on Human Factors in Computing Systems (New York, NY: ACM), 580–585. doi: 10.1145/1125451.1125573
Brave, S., and Dahley, A. (1997). “inTouch,” in CHI '97 Extended Abstracts on Human Factors in Computing Systems Looking to the Future - CHI '97 (New York, NY: ACM Press), 363. doi: 10.1145/1120212.1120435
Cabibihan, J.-J., Zheng, L., and Cher, C. K. T. (2012). “Affective tele-touch,” in International Conference on Social Robotics, 348–356.
Cha, J., Eid, M., Barghout, A., Rahman, A. M., and El Saddik, A. (2009). “HugMe,”1 in Proceedings of the Seventeen ACM International Conference on Multimedia - MM '09 (New York, NY: ACM Press), 1135. doi: 10.1145/1631272.1631535
Chang, A., O'Modhrain, S., Jacob, R., Gunther, E., and Ishii, H. (2002). “Comtouch: design of a vibrotactile communication device,” in Proceedings of the Conference on Designing Interactive Systems Processes, Practices, Methods, and Techniques - DIS '02 (New York, NY: ACM Press), 312. doi: 10.1145/778712.778755
Chen, C.-Y., Forlizzi, J., and Jennings, P. (2006). “ComSlipper,” in CHI '06 Extended Abstracts on Human Factors in Computing Systems - CHI EA '06 (New York, NY: ACM Press), 369. doi: 10.1145/1125451.1125531
Clark, H. H., and Brennan, S. E. (1991). “Grounding in communication,” in Perspectives on Socially Shared Cognition, eds L. B. Resnick, J. M. Levine, and S. D. Teasley (American Psychological Association), 127–149. doi: 10.1037/10096-006
Darriba Frederiks, A., Heylen, D., and Huisman, G. (2013). “TaSST,” in Proceedings of the 15th ACM on International Conference on Multimodal Interaction - ICMI '13 (New York, NY: ACM Press), 315–316. doi: 10.1145/2522848.2531758
Darriba Frederiks, A., Kröse, B. J. A., and Huisman, G. (2016). “Internet of touch,” in Proceedings of the 2016 ACM International Joint Conference on Pervasive and Ubiquitous Computing: Adjunct (New York, NY: ACM), 273–276. doi: 10.1145/2968219.2971382
Dekker, A., den Dima, L. A., Huisman, G., and Shor, D. (2021). “Future affair: an artistic exploration of socially distanced affective touch via forearm stimulation,” in 2021 IEEE World Haptics Conference (WHC) (Montreal, QC: IEEE), 877–877. doi: 10.1109/WHC49131.2021.9517135
Dennett, D. (2013). Intuition Pumps and Other Tools for Thinking, ed Kindle. New York, NY: W. W. Norton & Company.
Dennis, A. R., Fuller, R. M., and Valacich, J. S. (2008). Media, tasks, and communication processes: a theory of media synchronicity. MIS Q. 32, 575. doi: 10.2307/25148857
Dennis, A. R., and Valacich, J. S. (1999). “Rethinking media richness: towards a theory of media synchronicity,” in Proceedings of the Hawaii International Conference on System Sciences, Vol. 99 (Maui: IEEE), 12. doi: 10.1109/hicss.1999.772701
DiSalvo, C., Gemperle, F., Forlizzi, J., and Montgomery, E. (2003). “The hug: an exploration of robotic form for intimate communication,” in The 12th IEEE International Workshop on Robot and Human Interactive Communication, 2003. Proceedings. ROMAN 2003 (Millbrae, CA: IEEE), 403–408. doi: 10.1109/ROMAN.2003.1251879
Dobson, K., Boyd, D., Ju, W., Donath, J., and Ishii, H. (2001). “Creating visceral personal and social interactions in mediated spaces,” in CHI '01 Extended Abstracts on Human Factors in Computer Systems - CHI '01 (New York, NY: ACM Press), 151. doi: 10.1145/634158.634160
Dodge, C. (1997). “The bed,” in CHI '97 Extended Abstracts on Human Factors in Computing Systems Looking to the Future - CHI '97 (New York, NY: ACM Press), 371. doi: 10.1145/1120212.1120439
Döring, N. (2020). How is the COVID-19 pandemic affecting our sexualities? An overview of the current media narratives and research hypotheses. Arch. Sex. Behav. 49, 2765–2778. doi: 10.1007/s10508-020-01790-z
Erk, S. M., Toet, A., and Van Erp, J. B. F. (2015). Effects of mediated social touch on affective experiences and trust. PeerJ 3, e1297. doi: 10.7717/peerj.1297
Gooch, D., and Watts, L. (2012). “YourGloves, hothands and hotmits,” in Proceedings of the 25th Annual ACM Symposium on User Interface Software and Technology - UIST '12 (New York, NY: ACM Press), 157. doi: 10.1145/2380116.2380138
Gooch, D., and Watts, L. A. (2010). “Communicating social presence through thermal hugs,” in Proc. Ubicomp 2010 SISSE Workshop (Copenhagen).
Guo, S., and Sourin, A. (2018). “Towards asynchronous video-haptic interaction in cyberspace,” in 2018 International Conference on Cyberworlds (CW) (Singapore: IEEE), 160–163. doi: 10.1109/CW.2018.00038
Haans, A., de Bruijn, R., and IJsselsteijn, W. A. (2014). A virtual midas touch? Touch, compliance, and confederate bias in mediated communication. J. Nonverb. Behav. 38, 301–311. doi: 10.1007/s10919-014-0184-2
Haans, A., de Nood, C., and IJsselsteijn, W. A. (2007). “Investigating response similarities between real and mediated social touch,” in CHI '07 Extended Abstracts on Human Factors in Computing Systems - CHI '07, 2405.
Haans, A., and IJsselsteijn, W. (2006). Mediated social touch: a review of current research and future directions. Virt. Real. 9, 149–159. doi: 10.1007/s10055-005-0014-2
Haans, A., and IJsselsteijn, W. A. (2009a). “I'm always touched by your presence, dear”: combining mediated social touch with morphologically correct visual feedback,” in Proceedings of the 12th Annual International Workshop on Presence Cleveland, 1–6.
Haans, A., and IJsselsteijn, W. A. (2009b). The virtual midas touch: helping behavior after a mediated social touch. IEEE Trans. Haptics 2, 136–140. doi: 10.1109/TOH.2009.20
Hamilton, J. (2007). “Embodied communication in the distributed network,” in Virtual Systems and Multimedia Vol. 4820 (Berlin; Heidelberg: Springer), 179–190. doi: 10.1007/978-3-540-78566-8_16
Hansson, R., and Skog, T. (2001). “The LoveBomb,” in CHI '01 Extended Abstracts on Human Factors in Computing Systems - CHI '01 (New York, NY: ACM Press), 433. doi: 10.1145/634067.634319
Harjunen, V. J., Spapé, M., Ahmed, I., Jacucci, G., and Ravaja, N. (2017). Individual differences in affective touch: behavioral inhibition and gender define how an interpersonal touch is perceived. Pers. Individ. Dif. 107, 88–95. doi: 10.1016/j.paid.2016.11.047
Hoggan, E., Stewart, C., Haverinen, L., Jacucci, G., and Lantz, V. (2012). “Pressages,” in Proceedings of the 25th Annual ACM Symposium on User Interface Software and Technology - UIST '12 (New York, NY: ACM Press), 555. doi: 10.1145/2380116.2380185
Huisman, G. (2017). Social touch technology: a survey of haptic technology for social touch. IEEE Trans. Haptics 10, 391–408. doi: 10.1109/TOH.2017.2650221
Huisman, G., and Frederiks, A. D. (2013). “Towards tactile expressions of emotion through mediated touch,” in Conference on Human Factors in Computing Systems - Proceedings 2013-April (New York, NY), 1575–1580. doi: 10.1145/2468356.2468638
Ipakchian Askari, S., Haans, A., Bos, P., Eggink, M., Lu, E. M., Kwong, F., et al. (2020). “Context matters: the effect of textual tone on the evaluation of mediated social touch,” in Lecture Notes in Computer Science (Including Subseries Lecture Notes in Artificial Intelligence and Lecture Notes in Bioinformatics) (Vol. 12272 LNCS) (Springer International Publishing). doi: 10.1007/978-3-030-58147-3_15
Ipakchian Askari, S., Haans, A., and IJsselsteijn, W. A. (2019). “Is seeing believing?,” in Extended Abstracts of the 2019 CHI Conference on Human Factors in Computing Systems (New York, NY: ACM), 1–6. doi: 10.1145/3290607.3312976
Israr, A., and Abnousi, F. (2018). “Towards pleasant touch,” in Extended Abstracts of the 2018 CHI Conference on Human Factors in Computing Systems (New York, NY: ACM), 1–6. doi: 10.1145/3170427.3188546
Israr, A., Zhao, S., and Schneider, O. (2015). “Exploring embedded haptics for social networking and interactions,” in Proceedings of the 33rd Annual ACM Conference Extended Abstracts on Human Factors in Computing Systems (New York, NY: ACM), 1899–1904. doi: 10.1145/2702613.2732814
Iwaki, S., Ueki, S., Nakamura, Y., Motegi, M., Ogawa, K., and Shimokura, K. (2008). “A basic study of a pillow-shaped haptic device using a pneumatic actuator,” in Proceeding of the 5th International Symposium on Mechatronics and Its Applications, ISMA 2008 (Amman: IEEE), 1–5. doi: 10.1109/ISMA.2008.4648859
Kang, E., Jackson, E., and Schulte, W. (2011). An Approach for Effective Design Space Exploration, Berlin: Springer. 33–54. doi: 10.1007/978-3-642-21292-5_3
Kim, H.-Y. (2017). Statistical notes for clinical researchers: chi-squared test and fisher's exact test. Restor. Dentist. Endodont. 42, 152. doi: 10.5395/rde.2017.42.2.152
Kontaris, D., Harrison, D., Patsoule, E.-E., Zhuang, S., and Slade, A. (2012). “Feelybean,” in Proceedings of the 2012 ACM Annual Conference Extended Abstracts on Human Factors in Computing Systems Extended Abstracts - CHI EA '12 (New York, NY: ACM Press), 1273. doi: 10.1145/2212776.2212439
Kowalski, R., Loehmann, S., and Hausen, D. (2013). “Cubble: a multi-device hybrid approach supporting communication in long-distance relationships,” in TEI 2013 - Proceedings of the 7th International Conference on Tangible, Embedded and Embodied Interaction (New York, NY: ACM Press), 201–204. doi: 10.1145/2460625.2460656
Liang, R.-H., Chung, W.-M., Kao, H.-L., and Lin, T.-Y. (2013). InTouch: Crossing Social Interaction with Perception. Springer, 306–315. doi: 10.1007/978-3-642-39238-2_34
Makino, Y., Furuyama, Y., and Shinoda, H. (2015). “HaptoClone (Haptic-Optical Clone),” in Proceedings of the 3rd ACM Symposium on Spatial User Interaction (New York, NY: ACM), 139–139. doi: 10.1145/2788940.2794354
Mullenbach, J., Shultz, C., Colgate, J. E., and Piper, A. M. (2014). “Exploring affective communication through variable-friction surface haptics,” in Proceedings of the SIGCHI Conference on Human Factors in Computing Systems (New York, NY: ACM), 3963–3972. doi: 10.1145/2556288.2557343
Nadler, R. (2020). Understanding “zoom fatigue”: theorizing spatial dynamics as third skins in computer-mediated communication. Comp. Comp. 58, 102613. doi: 10.1016/j.compcom.2020.102613
Nakanishi, H., Tanaka, K., and Wada, Y. (2014). “Remote handshaking,” in Proceedings of the SIGCHI Conference on Human Factors in Computing Systems (New York, NY: ACM), 2143–2152. doi: 10.1145/2556288.2557169
Neidlinger, K., Truong, K. P., Telfair, C., Feijs, L., Dertien, E., and Evers, V. (2017). “AWElectric,” in Proceedings of the Eleventh International Conference on Tangible, Embedded, and Embodied Interaction (New York, NY: ACM), 315–324. doi: 10.1145/3024969.3025004
Nishida, J., Takahashi, K., and Suzuki, K. (2015). “A wearable stimulation device for sharing and augmenting kinesthetic feedback,” in Proceedings of the 6th Augmented Human International Conference (New York, NY: ACM), 211–212. doi: 10.1145/2735711.2735775
Obrist, M., Subramanian, S., Gatti, E., Long, B., and Carter, T. (2015). “Emotions mediated through mid-air haptics,” in Proceedings of the 33rd Annual ACM Conference on Human Factors in Computing Systems (New York, NY: ACM), 2053–2062. doi: 10.1145/2702123.2702361
Park, Y.-W., Bae, S.-H., and Nam, T.-J. (2012). “How do couples use cheektouch over phone calls?,” in Proceedings of the 2012 ACM Annual Conference on Human Factors in Computing Systems - CHI '12 (New York, NY: ACM Press), 763. doi: 10.1145/2207676.2207786
Park, Y.-W., Baek, K.-M., and Nam, T.-J. (2013). “The roles of touch during phone conversations,” in Proceedings of the SIGCHI Conference on Human Factors in Computing Systems, 1679–1688.
Pedemonte, N., Laliberté, T., and Gosselin, C. (2017). A haptic bilateral system for the remote human–human handshake. J. Dyn. Syst. Meas. Control 139:044503. doi: 10.1115/1.4035171
Pfab, I., and Willemse, C. J. A. M. (2015). “Design of a wearable research tool for warm mediated social touches,” in 2015 International Conference on Affective Computing and Intelligent Interaction (ACII) (Xi'an: IEEE), 976–981. doi: 10.1109/ACII.2015.7344694
Pimentel, A. D. (2017). Exploring exploration: a tutorial introduction to embedded systems design space exploration. IEEE Design Test 34, 77–90. doi: 10.1109/MDAT.2016.2626445
Pradana, G. A., Zhang, E. Y., Cheok, A. D., and Morisawa, Y. (2015). “Delivering haptic sensations in mobile marketing,” in Proceedings of the 12th International Conference on Advances in Computer Entertainment Technology (New York, NY: ACM), 1–3. doi: 10.1145/2832932.2856223
Rahman, A. S. M. M., and El Saddik, A. (2011).” HKiss: real world based haptic interaction with virtual 3D Avatars,” in 2011 IEEE International Conference on Multimedia and Expo (Barcelona: IEEE), 1–6. doi: 10.1109/ICME.2011.6012223
Rovers, A. F., and van Essen, H. A. (2006). Guidelines for haptic interpersonal communication applications: an exploration of foot interaction styles. Virt. Reality 9, 177–191. doi: 10.1007/s10055-005-0016-0
Seifi, H., Fazlollahi, F., Oppermann, M., Sastrillo, J. A., Ip, J., Agrawal, A., et al. (2019). “Haptipedia,” in Haptipedia: Accelerating Haptic Device Discovery to Support Interaction and Engineering Design (New York, NY), 1–12. doi: 10.1145/3290605.3300788
Shanken, E. A. (2000). Tele-agency: telematics, telerobotics, and the art of meaning. Art J. 59, 64–77. doi: 10.1080/00043249.2000.10791997
Singhal, S., Neustaedter, C., Ooi, Y. L., Antle, A. N., and Matkin, B. (2017). “Flex-N-feel,” in Proceedings of the 2017 ACM Conference on Computer Supported Cooperative Work and Social Computing (New York, NY: ACM), 98–110. doi: 10.1145/2998181.2998247
Stenslie, S., Olsson, T., Göransson, A., and Cuartielles, D. (2013). “Stitchies,” in Proceedings of the 8th International Conference on Tangible, Embedded and Embodied Interaction - TEI '14 (New York, New York, NY: ACM Press), 327–329. doi: 10.1145/2540930.2555200
Strong, R., and Gaver, B. (1996). “Feather, scent and shaker: supporting simple intimacy,” in Proceedings of CSCW (Boston, MA), 29–30.
Teh, J. K. S., Cheok, A. D., Choi, Y., Fernando, C. L., Peiris, R. L., and Fernando, O. N. N. (2009). “Huggy pajama: a parent and child hugging communication system,” in Proceedings of IDC 2009 - The 8th International Conference on Interaction Design and Children (New York, NY: ACM Press),290–291. doi: 10.1145/1551788.1551861
Teh, J. K. S., Tsai, Z., Koh, J. T. K. V., and Cheok, A. D. (2012). “Mobile implementation and user evaluation of the Huggy Pajama System,” in 2012 IEEE Haptics Symposium (HAPTICS) (Vancouver, BC: IEEE), 471–478. doi: 10.1109/HAPTIC.2012.6183833
Tsetserukou, D. (2010). HaptiHug: A Novel Haptic Display for Communication of Hug Over a Distance. Springer, 340–347. doi: 10.1007/978-3-642-14064-8_49
Tünnermann, R., Leichsenring, C., and Hermann, T. (2014). Direct Tactile Coupling of Mobile Phones with the feelabuzz System Berlin, 74–83. doi: 10.1007/978-3-642-54325-8_8
Tuovinen, J., Martesuo, K., and Pasola, M. (2022). We All Need Touch - How Science and Technology are Helping Long-Distance Relationships and Beyond. Retrieved from: https://www.joyhaptics.com/whitepaper/
van Erp, J. B. F., and Toet, A. (2015). Social touch in human-computer interaction. Front. Dig. Human. 2, 1–14. doi: 10.3389/fdigh.2015.00002
Wiedau, A., Gilgen, D., Frankjær, R., Goerlich, T., and Wiedau, M. (2015). Commiticator: Enhancing Non-verbal Communication by Means of Magnetic Vision, Vol. 9187, ed A. Marcus (Cham: Springer International Publishing), 705–714. doi: 10.1007/978-3-319-20898-5_67
Wikstrom, V., Makkonen, T., and Saarikivi, K. (2017). “Exploring universal expression of emotion with vibrotactile patterns composed using dynamic features,” in 2017 Seventh International Conference on Affective Computing and Intelligent Interaction Workshops and Demos (ACIIW) (San Antonio, TX: IEEE), 77–78. doi: 10.1109/ACIIW.2017.8272590
Yarosh, S., Mejia, K., Unver, B., Wang, X., Yao, Y., Campbell, A., et al. (2017). “SqueezeBands,” in Proceedings of the ACM on Human-Computer Interaction, Vol. 1 (New York, NY), 1–18. doi: 10.1145/3134751
Keywords: mediated social touch, social touch technology, haptic technology, communication characteristics, classification, design space
Citation: Ipakchian Askari S, Haans A and IJsselsteijn WA (2022) Uncovering terra incognita in the AHD design space: A review of affective haptic devices. Front. Comput. Sci. 4:795772. doi: 10.3389/fcomp.2022.795772
Received: 15 October 2021; Accepted: 11 October 2022;
Published: 15 November 2022.
Edited by:
Karon E. MacLean, University of British Columbia, CanadaReviewed by:
Ulysses Bernardet, Aston University, United KingdomJan B. F. Van Erp, University of Twente, Netherlands
Karon E. MacLean, University of British Columbia, Canada
Copyright © 2022 Ipakchian Askari, Haans and IJsselsteijn. This is an open-access article distributed under the terms of the Creative Commons Attribution License (CC BY). The use, distribution or reproduction in other forums is permitted, provided the original author(s) and the copyright owner(s) are credited and that the original publication in this journal is cited, in accordance with accepted academic practice. No use, distribution or reproduction is permitted which does not comply with these terms.
*Correspondence: Sima Ipakchian Askari, s.ipakchian.askari@vilans.nl