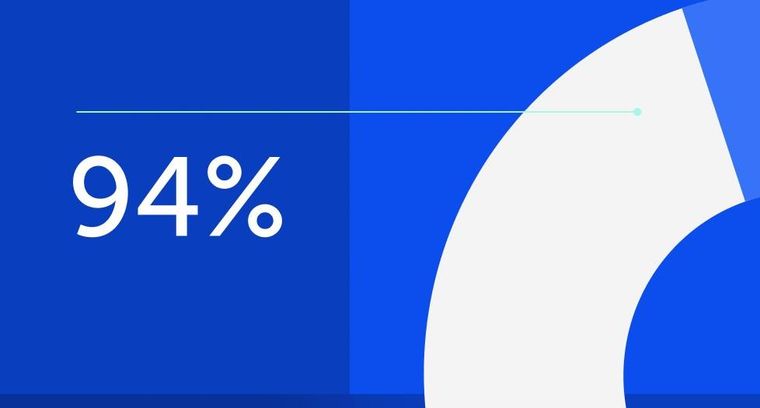
94% of researchers rate our articles as excellent or good
Learn more about the work of our research integrity team to safeguard the quality of each article we publish.
Find out more
ORIGINAL RESEARCH article
Front. Comput. Sci., 20 September 2021
Sec. Human-Media Interaction
Volume 3 - 2021 | https://doi.org/10.3389/fcomp.2021.673826
This article is part of the Research TopicCognitive InfocommunicationsView all 10 articles
Due to its constantly developing technological background, VR and AR technology has been gaining increasing popularity not just in industry or business but in education as well. Research in the field of Cognitive Infocommunications (CogInfoCom) shows that using existing digital technologies, online collaboration and cooperation technologies in 3D VR supports cognitive processes, including the finding, processing, memorization and recalling of information. 3D VR environments are also capable of providing users with a much higher level of comprehension when it comes to sharing and interpreting digital workflows. The paper presents a study carried out with the participation of 90 students. The aim of this study is to investigate how the application of 3D VR platforms as personalized educational environments can also increase VR learning efficiency. Besides considering participants’ test performance, metrics such as results on visual, auditory and reading-based learning tests for information acquisition, as well as responses on Kolb’s learning styles questionnaires are taken into consideration. The participants’ learning styles, information acquisition habits were also observed, allowing us to create and offer a variety of learning pathways based on a variety of content types in the 3D VR environment. The students within the study were divided into two groups: a test group receiving personalized training in the MaxWhere 3D VR classroom, and a control group that studied in a general MaxWhere 3D VR space. This research applies both quantitative and qualitative methods to report findings. The goal was to create adaptive learning environments capable of deriving models of learners and providing personalized learning experiences. We studied the correlation between effectiveness of the tasks and Kolb’s learning styles. The study shows the major importance of choosing the optimal task type regarding each Kolb learning style and personalized learning environment. The MaxWhere 3D spaces show a high potential for personalizing VR education. The non-intrusive guiding capabilities of VR environments and of the educational content integrated in the 3D VR spaces were very successful, because the students were able to score 20 percent higher on the tests after studying in VR than after using traditional educational tools. Students also performed the same tasks with 8-10 percent faster response times.
Research in the field of Cognitive Infocommunications (CogInfoCom) show that using existing digital technologies in 3D VR supports cognitive processes, including the finding, processing and memorization of information (Baranyi et al., 2015; Baranyi and Csapó, 2012).
In the past few years the VR Learning Center Research Lab at Széchenyi István University has been investigating the effects of 3D VR spaces on cognitive capabilities, based on the methodologies of CogInfoCom, and is actively working together with other institutions of higher education to test new ideas within VR-based training and to disseminate relevant results.
Our main studies have shown that:
In the area of effectiveness:
• Digital workflows can be reduced by at least 50 percent in 3D VR spaces Lampert et al. (2018).
• Workflows can be reduced with 30 percent fewer user operations and 80 percent fewer machine operations in 3D VR Horváth and Sudár (2018).
• The rate of information recall is 50 percent higher Berki (2018a).
• The MaxWhere 3D platform increases the effectiveness of online collaborative project management software–requiring 72 percent less elementary user operations, and 80 percent less time spent on overview-related tasks Horváth (2019d).
In the area of visual attention and memory:
• The fixation parameters of eye and hand motion are in negative correlation with visual attention, while the distance between the focus of visual attention and the mouse cursor’s motion are not correlated to each other Kovari et al. (2020).
• The effectiveness of VR advertisements was higher than classic web-based ads as demonstrated by a questionnaire based free recall test in a 3D space Berki (2018a).
• There is no significant relationship between the effectiveness of completing a task in MaxWhere VR and the spatial memory (measured by the Corsi-task) and the mental rotation ability Berki (2019).
There have been a number of positive results regarding the capabilities of 3D VR for enhancing teaching activities (Csapó et al., 2018; Kvasznicza, 2017; Kvasznicza et al., 2019; Kövecses-Gõsi, 2019; Katona and Kovari, 2018; Horvath, 2016; Kuczmann and Budai, 2019; Markopoulos et al., 2020; Botha-Ravyse et al., 2020; Biró et al., 2017; Orosz et al., 2019) the effectiveness of new teaching methodologies (Horváth, 2019b; Kövecses-Gõsi, 2019; Kővári, 2019; Horváth, 2019c; Sztahó et al., 2019; Vicsi et al., 2000, Mathability Baranyi and Gilányi, 2013; Chmielewska, 2019) or eye-tracking studies (Hercegfi et al., 2019; Hercegfi and Köles, 2019; Hercegfi et al., 2019) as well.
Most traditional teaching techniques share information through visual or auditory channels. At the same time, multi-sensory educational techniques and strategies are utilized to engage students at multiple levels (Shams and Seitz, 2008). Using a multisensory teaching technique means that students are capable of learning in multiple ways. The great advantage of multisensory teaching methods is that they activate more regions of both hemisphere so linking to more senses makes knowledge more memorable and durable.
VR education basically applies a multisensory teaching strategy and exploits the great advantages of 3D. It provides visual, audiovisual and multisensory websites for students besides text based 1st order workflows (Lampert et al., 2018; Horváth and Sudár, 2018) to a multidirectional VR learning content. The goal of multisensory stimulation is to decrease cognitive load.
Our experience in the world incorporates multisensory stimulation. Visual and auditory information are integrated in performing many tasks that involve localizing and tracking moving objects (Shams and Seitz, 2008). Csapo and colleagues 2012 article unfolds how to design multi-sensory signals, which are capable of communicating high-level information to users (Csapó et al., 2012).
VR education can utilize the simultaneous use of visual, auditory, and indirectly (use of mouse, keyboard, touchpad, touch screen and CogiNav technology) kinesthetic (motion-related) channels in multisensory teaching.
3D VR technology provides interactivity by responding to movements. Interactivity is the ability to control events in the simulation by using ones body or several input peripheral devices movements which in turn initiates responses in the simulation as a result of these movements (Christou, 2010).
The content of the smart boards can be selected as desired in the MaxWhere 3D VR spaces. Content can be static or dynamic, and can include text, 3D models, online graphic organizers, calendars, project management software, and more. By highlighting and marking up words, modifying the colors of the smartboard frames, or by changing colors from static to blinking can provide visual support for focus of attention and memorization (Figure 1). In this case, an involuntary action activates the user’s attention and the visual memorization. This activity is built up piece by piece in order to facilitate the synthesis of the student’s learning. The videos related to the educational topic, which have the advantage of being multisensory on the one hand because they contain both visual and verbal elements and on the other hand presenting information holistically rather than analytically, as in most textbooks and teaching materials.
The 3D objects give students a chance to become more visually immersed and engaged in educational content. 3D objects can be walked around, you can also look inside the object with a movement that is not possible in reality. Disassembled, stackable 3D objects also provide a kinesthetic effect. The reception and retention of information gained by vision may be affected by difficulties in tracking or visual processing. It is important to distinguish between textual and pictorial information. For most users, processing the information they read represents a greater cognitive strain. On the other hand, there are those for whom texts are is a more explicit source of information.
In MaxWhere spaces, auditive information can also be a source of information. Its exclusive use is not typical, as VR spaces add more to the user’s work or learning process with a 3D visual experience. As part of multisensory training, VR also provides auditory information. Sometimes, the subject’s auditory processing may be more stressful. Considering students ‘information acquisition preferences and using multiple senses together can provide a solution to individual problems.
The feeling of natural movement in VR space is provided by MaxWhere 3D VR cognitive navigation (CogiNav) technology. The CogiNav technology operates by taking into consideration the context of the camera (or more generally, the avatar) and the object that it is looking at, using those two pieces of information to deduce the intentions of the user, and finally mapping those intentions onto the limited degrees of freedom of the input device and the characteristics of the camera movements. Thus, although the input dimensions of the input device are limited, their function changes through context in a way that fits naturally with users’ expectations from the physical world. The seamless controls for spatial navigation allows it to be used as a communication tool built primarily on 3D interactions and 3D metaphors. Spatial awareness helps to connect visual, auditory, and movement memories to a place, piece of equipment, environment, and involves the delivery of an accurate workflow.
The user’s having to expend less effort means that the user feels less disturbed in his or her primary activities while still being able to perceive new information that he or she may find useful. More specifically, in a 3D space with smartboards all along the walls of the environment, users will recognize key changes in the contents of those smartboards even when they are not actively looking at them. A previous study showed, that users could benefit more from the supplementary information in the desktop VR than in a 2D browser if it appeared in their visual field Berki (2018b).
In addition to cognitive factors (Vogel and Esposito, 2020), it is important to consider that people are complex individuals with different personality types, learning preferences, aptitudes and attitudes toward learning. Each student has different and consistent preferred ways of perception, organization and retention. Some people tend to pick up information better when it is presented verbally, others when it is presented visually and still others prefer reading. These learning styles are good indicators of how students respond to their learning environment.
There are many theories - some of them are really critically for learning styles (Pashler et al., 2008; Coffield et al., 2004)- a move to an active experiential based education explicitly acknowledging different learning styles - has been called transformational learning de Jesus et al. (2006) has been promoted as a more effective alternative to traditional pedagogy (Jacoby, 1996).
Coffield et al. (2004) identified more than 70 learning style models (Coffield et al., 2004). The most influential learning style model is Kolb’s model (Kayes et al., 2005; Kayes, 2005). An appeal of Kolb’s Experiential Learning Model is its focus on the experiential learning process rather than on fixed learning traits (Turesky and Gallagher, 2011).
However, while there are many theories, little empirical evidence exists for linking such learning styles to 3D VR education.
Students’ learning styles never show a unified picture, so if we teach with only one channel, it favors some students while not others. A learning style is also built on the three main channels, which describes the form in which the student can most effectively process the curriculum. Although we are able to receive information through all three channels, the use of a dominant channel and a dominant learning style provides the best results.
In 3D VR learning the kind of analytical thinking and step-by-step information processing representative of earlier times in 2D e-Learning is being replaced by a more comprehensive spatial-visual kind of information processing. It is necessary to select the most effective technology and methodology to support education. However, it is important to realize that learning is not just about memorizing facts, it is also about learning styles and skills. VR learning activates all learning skills at once.
If we want to personalize VR education, we must pay attention to personalizing the VR learning environment and learning content as well, so learners can choose to engage with the learning materials in the manner that interests them the most. MaxWhere 3D VR platform (www.maxwhere.com) has several opportunity to personalizing the education:
• Providing personal check-in. All students can make the course “personal” in MaxWhere’s learning spaces. They can capture their name as part of the registration process. Moreover, they can find their own name on the control panel without personal login if the lecturers create personalized educational projects for students (Figure 2). Personalize the learner helps the learning motivation, mainly when we use the name throughout the course.
• The developed Smartboard Sharing technology connecting to 3D VR spaces, which enables users to create personalized learning spaces by scaling, moving, turning on and off the boards. (Figure 3).
Personalizing learning sequences. Creating “nonlinear” content - Digital Workflows of the 4th order Lampert et al. (2018); Horváth and Sudár (2018) - in 3D VR allows learners to pick and choose how they will learn. Students can optimize their learning path and make personalized story in 3D VR. To implement personalization to suit students ’learning styles and preferences, the research presented in the next chapter was conducted.
This study looks to address this issue, considering not only test performance - visual, auditory and reading-based learning test and Kolb’s learning styles questionnaire - but also other outcomes of using VR for personalized learning. This paper emphasizes that cooperation of humans in virtual spaces enhances affective mental behaviors and motivations in addition to cognitive abilities. The survey was based on an empirical survey and direct measurements with the participation of 90 students (45 students in an experimental group and 45 students in a control group), in September 2019. The study was carried out with the participation of BSc electrical engineering students at the Faculty of Engineering and Information Technology of the University of Pécs, and BsC students of educational sciences at the Faculty of Apáczai Csere János at the Széchenyi István University. Participation in this research was voluntary and conducted with informed consent of the participants.
The paper is structured as follows. The first part draws attention to MaxWhere’s multisensory educational capabilities and the concept of personalization. In the second section, materials and methods are presented. In section 3, the research results of this paper are summarized. Finally, a discussion is provided on how a framework for personalized affective learning in VR education could be implemented.
During the COVID era, the need for personalized learning in online education has intensified. The goal is to ensure independent and successful learning. At the same time, independence does not mean the absence of consultation or space to face meetings, but it means the creation of the possibility of independent or interactive knowledge acquisition without continuous supervision. VR education could be designed several ways on the MaxWhere 3D VR platform, which includes multiple learning preferences so students can choose to relate to the curriculum in a way that interests them. Personalized learning is goals to facilitate the academic success of each student by first determining the learning needs, preferences of individual students, and then providing learning space, contents and methods that are customized for each student. To accomplish this goal we observe the students learning styles, information acquisition habits, create and offer students a variety of learning pathways and contents. Since the 1970s, educational researchers have attempted to categorize the different ways in which people learn and retain information and concepts. “Cognitive styles” or “learning styles” have been defined as “self-consistent, enduring individual differences in cognitive organization and functioning” (Ausubel et al., 1968).
There are multiple factors that impact students’ ability to learn, including age, digital and cultural background beside the learning styles and habits. The results for these factors have been published previously (Horváth, 2019a; Komlósi and Waldbuesser, 2015). In this study a test identifying preferred information acquisition habits and the Kolb’s Learning Style test (Kolb, 1981) were applied.
The learning style is made up of a combination of several factors, such as:
• What is the easiest way to receive information?
• How we organize and process information?
• What conditions do we need to help pick up and store information?
• How can we retrieve information?
The goal of this test was to created in order to assess the students for preferred information acquisition habits. The relevance of this test in this experiment was that the students were grouped by visual, auditive and reading categories based on this test results.
To test the visual skills, students saw 10 pictures in a row. They did not take notes. This was followed by a task. This task diverted their thoughts from the images they saw. The task was to answer the question: “If Anna’s birthday was on the 60th day of the year, and the year was not a leap year, then on which day was she born?”
After answering the task, the words the students remembered had to be written down based on the pictures they saw. They had 1 min to complete the task.
The auditive test was similar. In this case 10 words were heard, followed by a logical task, after which the words had to be recalled from memory.
In the case of the reading test, the students saw 10 words. Next task was: “Yesterday was the 3rd day after Monday. What day will be the day after tomorrow?”
After answering, participants had to write down the words they memorized.
Mapping the kinesthetic learning style was not part of the study. This is because the user can move in 3D using a mouse or touchpad. Its seamless controls for spatial navigation allows it to be used as a communication tool built primarily on 3D interactions and 3D metaphors.
David A Kolb an American social psychologist and learning researcher, developed a test to determine learning style. Kolb separated four different learning style. According to his theory learning is a cyclically repetitive process, where experience, observation, thinking and application stages are separated. The learning cycle depends on a double basis: the recording, obtaining and processing the information. Kolb identified four different learning styles (Kolb, 1981):
• Accommodating: The information that is retained is based on experience and is processed by an active experimenter. This is an action oriented, flexible, risk taking and quickly applied way of learning.
• Diverging: Specific information is gathered through experiences of a reflective observer. Information in this case is processed through observation and understanding.
• Assimilating: Through observational experience, this learning style processes abstract information. The assimilating style is logical, theory oriented and creates coordinated models.
• Converging: This style uses abstract information in a practical, action-oriented, purposeful, and planning-oriented way.
Kolb created a 12 question test to determine the learning style, which can be an important step in our work of self-knowledge. We can become aware of the characteristic way of obtaining and processing information during learning and working. This awareness helps us balance the educational goal and learning materials and methods or understand and accept the learning style of students. The positive outcome of acceptance, understanding and apprehension of conflicts is that we are more aware of our own learning style.
We were looking for opportunities to implement personalized education in 3D VR spaces.
Before completing the test, students were asked what type of learning style they classified themselves. The aim of the question was to find out whether they had prior knowledge of their own learning processes and cognitive abilities.
To fulfill the test all students received the curriculum in MaxWhere 3D VR space and all students were given equal time to learn. The students in the focus group received a personalized curriculum, while the control group got multisensory curriculum.
In terms of gathering information for visual type learners, the curriculum was created with graphs, flowcharts, images, videos, and 3D objects. Keywords are highlighted in the text information. Tasks always included, “Make a picture/graph etc.!” instruction as well.
For the auditory type of students, we provided the lectures with audio recording, online meeting applications, they had the opportunity to discuss the topic, request additional oral information and audio videos were added to the theoretical material. Tasks included, “Tell/Summarize!” instructions. The recordings could also be uploaded using Smartboard Sharing, so there was also an opportunity for teacher feedback.
The educational content for students who preferred to read written text included presentations, additional notes, articles, and written web content. Their job was to create and upload their own notes and summary on the Smartboard Sharing.
In the case of mixed-type students, the curriculum was created by blending the above. VR activities could be designed to include multiple learning methods, so according to the results of the Kolb learning style test, students of the Accomodating type were given more interactive contents an activity task with quick feedback. We gave a large number of articles and websites to students belonging to the Diverging type. Their task is with instructions: ”collect, organize, formulate somethings” we have given. Students who belong to the Assimilating type received more information based on the principle or rule, than what the curriculum focuses. In addition to receiving presentations, articles, or visions, their tasks were to create models, other types of uses. The students belonging to Converging learning style are action oriented and purposeful, so the optimal educational methods are the project based educational or Edu-Coaching methods. They were asked to provide a learning goal before beginning the learning process. Why is it important for them to acquire that knowledge? For Converging learning style students, we can often use Laboratory spaces that also included simulations that worked with 3D objects (Figure 4).
This research was reviewed and approved by both the ethical review committee for Doctoral School of Multidisciplinary Engineering Sciences (MMTDI) at the Széchenyi István University and the ethical review committee for University of Pécs, Faculty of Engineering and Information Technology, Department of Electrical Network.
At the first lesson of the course the participants were informed in detail about the purpose, conditions and background of the research. This detailed information was: “The aim of the study is to observe the categorization of learning preferences and styles. During the task, participants see pictures, hear and read words, and then describe what they remember. After the examination, a questionnaire (Kolb’s learning styles questionnaire) is also completed. The test above has no adverse consequences. The test takes approximately 30 min. The study can be stopped at any time without consequences, in which case the data already recorded will not be evaluated. After the study, participants learn in a personalized educational environment and curricula. Researchers measure their learning outcomes.”
Participants were volunteers. Consenting participants made assertions that they have no known neurological or psychiatric disorders. They declared that their vision and hearing is intact or corrected to normal. I have no dyslexia, dysgraphia or other learning difficulties. The manuscript has the English text of the consent form as an Supplementary Appendix. Participants had declared consent bellow:
“I declare that I have received satisfactory information about the goals and nature of the study.
I declare that I am participating in this study voluntarily.
Neither I nor my relatives requested or received financial compensation for my contribution to the investigations.
I acknowledge that the personally identifiable information will be treated confidentially by the study manager and will not be disclosed to anyone other than those involved in the conduct of the experiment.
I agree that non-identifiable data included in the study should be made available to other researchers.
I acknowledge that the test data are for research and non-diagnostic purposes only.
I do not request such an opinion after the tests have been performed.
I, the undersigned (name), agree that I will participate in the examinations of Széchenyi István University on (day) September 2019.”
After developing personalized VR educational spaces and learning content, the 45 students (in experimental group) included in the study continued their personalized learning. The control group also included 45 students from the same age group. They also received the educational contents in 3D VR space, but their learning style was not determined and applied.
90 (56 male and 34 female) students participated in the study. The average age of participants was 20.188 (between 19 and 24 years).
Levene’s test was applied to assess the equality of variances for a variable calculated for experimental and control group, before we perform the independent t-test. The null hypothesis formed in the Levene’s test is that the groups we are comparing have equal variance.
The Independent Samples t Test compares the means of two independent groups - the experimental and the control group - in order to determine whether there is statistical evidence that the means of total score and response time are significantly different in our two groups.
In terms of visual, auditory and reading-based learning test, 42 percent of students preferred visual information, 24 percent of them the auditory, 13 percent of them the reading based and 21 percent the mixed (visual and reading, visual and auditory, visual-auditory and reading) information (Figure 5).
A surprisingly high proportion of the students were unable to answer the question (47 percent), or gave a wrong answer the question (15.5 percent): “What kind of learning style do you prefer?” The answer was wrong if the student did not name the own real learning test. Students stated that so far they have not had the opportunity to choose between types of educational materials, so they have not addressed this issue.
According to Kolb’s learning test results, the distribution of students’ learning styles is shown in Figure 6.
Logical thinking, modelling and design are connected with the assimilating learning type, which is mainly present in the field of engineering.
The learning performance of the two groups were examined. To test the hypothesis that the experimental group (N = 45), who got the personalized curriculum for learning and the control group (N = 45) were associated with statistically significant different mean response time and total score, an independent samples t-test was performed. Additionally, the assumption of homogeneity of variances was tested and satisfied via Levene’s Test F = 0.375, p = 0.542 to total Score and F = 0.387, p = 0.532 to response time.
Based on statistical analysis, we accept the null hypothesis of Levene’s test and conclude that the variance in total score and in response time variance are accepted as equal. Levene’s statistic response time is shown in Figure 7 and the total score is shown in Figure 8.
To compare the results of the experimental and control group an independent sample t-test was used. The null hypothesis of the independent samples t-test is that the means of total score and response time are equal in the two groups. The mean total score in the control group was 38.73 (SD = 8,29) and 48,44 in the experimental group (SD = 7.3). The difference between these means are statistically significant based on the results of the t-test: t (88) = −5,898, p < 0.01. The means response time in the control group was 29.58 min (SD = 1.69) and 27.02 min (SD = 1.29) in the experimental group. This difference is statistically significant based on the results of the t-test: t (88) = 8,082, p <0 .01. This means that the test score in the experimental group, who received personalized educational content, was significantly higher, also their response times were significantly faster.
The correlation between the test score and the characteristic score was examined. The characteristic score of Kolb’s test represents the strength of group membership. There was a positive correlation between personalized educational content and tests’ score (r = 0.784 p <0 .01). Correlation is significant on the level of 0.01. The test score in the focus group (personalized education) was on average 20 percent higher than in the control group, with 8–10 percent faster response times. There was a significant performance improvement for memory recall type tasks. Students in the focus group remembered more than 30 percent more factual data. In case the question contains the initial information (facts), and the task was to process them. The difference here was not significant. We studied the correlation between effectiveness of the tasks and Kolb’s learning styles. The study shows the major importance of choosing the optimal task type regarding each Kolb learning style and personalized learning environment. According to the results of the correctional investigations, the Accomodating learning style shows a negative significant correlation (r = −, 783; p < 0.01) with learning results of long descriptive or long and tedious explanatory tasks. This result concerns students who are a part of the control group, so they studied in a non-personalized learning environment.
The other definitive result was found for the students with a Diverging learning style. In this case, the active presentations were less successful and caused difficulties for these students (r = −0.688; p < 0.01).
Traditional educational methods and their tools and sources, such as presentations or textbooks typically guide the students to follow fixed sequences to related to their learning process. In order to integrate the information in the knowledge system, the students have to go through the process of information processing which cannot eliminate one or the other phase. However, the inappropriate course ware leads to learner cognitive overload or disorientation during learning processes, thus reducing learning performance (Chen, 2008). We see, that the Web-based instruction researchers have given considerable attention to flexible curriculum sequencing control to provide adaptable, personalized learning programs (Brusilovsky and Vassileva, 2003; Hübscher, 2000; Papanikolaou et al., 2002; Lee, 2001) In an educational adaptive hypermedia system, an optimal learning path aims to maximize a combination of the learner’s understanding of course ware and the efficiency of learning the course ware Hübscher (2000). Nowadays, most adaptive/personalized tutoring systems (Lee, 2001; Papanikolaou et al., 2002; Tang and McCalla, 2003) consider student preferences, when investigating learner behaviors for personalized services.
However, while there are many theories little empirical evidence exists for linking such learning styles to 3D VR education. Our previous papers focuses for information finding, filtering, reception, processing and applications. This paper investigates the opportunity of personalization in 3D VR. The main point here is that in order for learning to be effective. Learning is by necessity a multi-faceted process. Students must be able to recall facts, definitions, the relationships and to create new relevant information.3D environment is absolutely effective because the human brain was formed in an evolutionary environment where the recognition and recollection of spatial relationships and locations was of paramount importance (much more so than the rote memorization of non-spatial concepts) (Logie, 1986; Rodrıguez et al., 2002; Csapó et al., 2018).
If we want to personalize VR education the best way is the categorization and optimizing of VR educational environments and contents. The results of the two types of learning style tests helped us to design this. At first the results of the two types of learning tests were analyzed and individual educational content was developed for each participant in experimental group\enleadertwodots Learning style test for preferred information acquisition habits provided us with relevant information for creating individually preferred content, Kolb learning test results were used in connection with information processing. For example, regarding the Accomodating learning style in the case of students in the personalized group, the most successful tasks were the ones which were short, substantial and concise. The students with a Diverging learning type were most efficient in tasks where they could do analytical work and work alone. There isn’t such a strong correlation between the other two learning styles and the several tasks.
To implement an automated and personalized VR educational system, we first need a database in which the curriculum contents are stored in the form of visual, audio visual and text based data. After that we have to determine the individual’s primary information gathering preference by using sensors or other techniques. For user interaction there are several options: head tracking, eye tracking, motion tracking etc. If the information obtained by eye movement tracking or other sensory based device is the same as the results of classical information acquisition preference tests, then starting from a mixed information base, after a few minutes the personalized information content can be loaded into the smart boards automatically, from the database behind MaxWhere VR system.
Our original idea was based on the examination based on the tracking of eye movement. However, due to the pandemic, we were unable to perform this measurement, which is part of our work for further research and development.
With a personalized learning experience, every student can enjoy an unique educational approach in 3D VR that is fully tailored to his or her individual abilities and needs. This can directly increase students’ motivation and reduce their likelihood of dropping out. It could also help achieve a better understanding of each student’s learning process. Therefore, the education system could benefit from the application of tests for different types of learning styles. This paper examined learning effectiveness as it relates to the use personalized learning content. The goal was to create adaptive learning environments capable of deriving models of learners and providing personalized learning experiences.
The MaxWhere 3D spaces show a high potential for personalizing VR education. The non-intrusive guiding capabilities of VR environments and of the educational content integrated in the spaces were successful, because the students were able to score 20 percent higher on the tests after studying in VR than after using traditional educational tools.
The original contributions presented in the study are included in the article/Supplementary Material, further inquiries can be directed to the corresponding author.
This research was reviewed and approved by both the ethical review committee for Doctoral School of Multidisciplinary Engineering Sciences (MMTDI) at the Szechenyi Istvan University and the ethical review committee for University of Pecs, Faculty of Engineering and Information Technology, Department of Electrical Network. Written informed consent to participate in the study was provided by the participants
IH contributed to conception and design of the study. IH organized the database. IH performed the statistical analysis. IH wrote the manuscript. The author read, and approved the submitted version I have an Agreement between IH (“Me”) and IEEE (“IEEE”) consists of my license details and the terms and conditions provided by IEEE and Copyright Clearance Center.
The author declares that the research was conducted in the absence of any commercial or financial relationships that could be construed as a potential conflict of interest.
The reviewer BB declared a shared affiliation with the author IH to the handling editor at time of review.
All claims expressed in this article are solely those of the authors and do not necessarily represent those of their affiliated organizations, or those of the publisher, the editors and the reviewers. Any product that may be evaluated in this article, or claim that may be made by its manufacturer, is not guaranteed or endorsed by the publisher.
The content of this manuscript has been Horváth (2020) presented in part at the 11th IEEE International Conference on Cognitive InfoCommunications. I would like to thank the IEEE for permission to reuse the published conference paper. This work was supported by the FIEK program (Center for cooperation between higher education and the industries at the Széchenyi István University, GINOP-2.3.4-15-2016-00003).
The Supplementary Material for this article can be found online at: https://www.frontiersin.org/articles/10.3389/fcomp.2021.673826/full#supplementary-material
Ausubel, D. P., Novak, J. D., and Hanesian, H. (1968). Educational Psychology: A Cognitive View. New York: Holt Rinehart & Winston.
Baranyi, P., and Csapó, Á. (2012). Definition and Synergies of Cognitive Infocommunications. Acta Polytechnica Hungarica 9, 67–83.
Baranyi, P., Csapo, A., and Sallai, G. (2015). Cognitive Infocommunications (CogInfoCom). New York: Springer.
Baranyi, P., and Gilányi, A. (2013). “Mathability: Emulating and Enhancing Human Mathematical Capabilities,” in 2013 IEEE 4th international conference on cognitive Infocommunications (CogInfoCom) (New Jersey: IEEE), 555–558. doi:10.1109/coginfocom.2013.6719309
Berki, B. (2018a). “Better Memory Performance for Images in Maxwhere 3d Vr Space Than in Website,” in 2018 9th IEEE International Conference on Cognitive Infocommunications (CogInfoCom) (New Jersey: IEEE), 000281–000284. doi:10.1109/coginfocom.2018.8639956
Berki, B. (2018b). “Desktop Vr and the Use of Supplementary Visual Information,” in 2018 9th IEEE International Conference on Cognitive Infocommunications (CogInfoCom) (New Jersey: IEEE), 000333–000336. doi:10.1109/coginfocom.2018.8639925
Berki, B. (2019). Does Effective Use of Maxwhere Vr Relate to the Individual Spatial Memory and Mental Rotation Skills? Acta Polytechnica Hungarica 16, 41–53. doi:10.12700/aph.16.6.2019.6.4
Biró, K., Molnár, G., Pap, D., and Szűts, Z. (2017). “The Effects of Virtual and Augmented Learning Environments on the Learning Process in Secondary School,” in 2017 8th IEEE International Conference on Cognitive Infocommunications (CogInfoCom) (New Jersey: IEEE), 000371–000376. doi:10.1109/coginfocom.2017.8268273
Botha-Ravyse, C., Fr, T. H., Luimula, M., Markopoulos, P., Bellalouna, F., et al. (2020). “Towards a Methodology for Online Vr Application Testing,” in 2020 11th IEEE International Conference on Cognitive Infocommunications (CogInfoCom) (New Jersey: IEEE), 000295–000298. doi:10.1109/coginfocom50765.2020.9237893
Brusilovsky, P., and Vassileva, J. (2003). Course Sequencing Techniques for Large-Scale Web-Based Education. Int. J. Contin. Eng. Edu. Lifelong Learn. 13, 75–94. doi:10.1504/ijceell.2003.002154
Chen, C.-M. (2008). Intelligent Web-Based Learning System with Personalized Learning Path Guidance. Comput. Edu. 51, 787–814. doi:10.1016/j.compedu.2007.08.004
Chmielewska, K. (2019). “From Mathability to Learnability,” in 2019 10th IEEE International Conference on Cognitive Infocommunications (CogInfoCom) (New Jersey: IEEE), 513–516. doi:10.1109/coginfocom47531.2019.9089891
Christou, C. (2010). “Virtual Reality in Education,” in Affective, interactive and cognitive methods for e-learning design: creating an optimal education experience (Pennsylvania: IGI Global), 228–243.
Coffield, F., Ecclestone, K., Hall, E., and Moseley, D. (2004). Learning Styles and Pedagogy in post-16 Learning: A Systematic and Critical Review. New Delhi: Learning and Skills Research Council.
Csapó, Á., Baranyi, P., and Várlaki, P. (2012). “A Taxonomy of Coginfocom Trigger Types in Practical Use Cases,” in 2012 IEEE 3rd International Conference on Cognitive Infocommunications (CogInfoCom) (New Jersey: IEEE), 21–25. doi:10.1109/coginfocom.2012.6421990
Csapó, Á. B., Horvath, I., Galambos, P., and Baranyi, P. (2018). “Vr as a Medium of Communication: from Memory Palaces to Comprehensive Memory Management,” in 2018 9th IEEE International Conference on Cognitive Infocommunications (CogInfoCom) (New Jersey: IEEE), 000389–000394. doi:10.1109/coginfocom.2018.8639896
de Jesus, H. T. P., Almeida, P. A., Teixeira-Dias, J. J., and Watts, M. (2006). Students’ Questions: Building a Bridge between Kolb’s Learning Styles and Approaches to Learning. Edu. Train. 48, 97. doi:10.1108/00400910610651746
Hercegfi, K., and Köles, M. (2019). Temporal Resolution Capabilities of the Mid-frequency Heart Rate Variability-Based Human-Computer Interaction Evaluation Method. Acta Polytechnica Hungarica 16, 95–114. doi:10.12700/aph.16.6.2019.6.7
Hercegfi, K., Komlódi, A., Köles, M., and Tóvölgyi, S. (2019). Eye-tracking-based Wizard-Of-Oz Usability Evaluation of an Emotional Display Agent Integrated to a Virtual Environment. Acta Polytechnica Hungarica 16, 145–162. doi:10.12700/aph.16.2.2019.2.9
Horváth, I. (2019a). “Behaviors and Capabilities of Generation Ce Students in 3d Vr,” in 2019 10th IEEE International Conference on Cognitive Infocommunications (CogInfoCom) (New Jersey: IEEE), 491–494. doi:10.1109/coginfocom47531.2019.9089988
Horváth, I. (2019c). “How to Develop Excellent Educational Content for 3d Vr,” in 2019 10th IEEE International Conference on Cognitive Infocommunications (CogInfoCom) (New Jersey: IEEE), 483–490. doi:10.1109/coginfocom47531.2019.9089916
Horvath, I. (2016). “Innovative Engineering Education in the Cooperative Vr Environment,” in 2016 7th IEEE International Conference on Cognitive Infocommunications (CogInfoCom) (New Jersey: IEEE), 000359–000364. doi:10.1109/coginfocom.2016.7804576
Horváth, I. (2019d). Maxwhere 3d Capabilities Contributing to the Enhanced Efficiency of the Trello 2d Management Software. Acta Polytechnica Hungarica 16, 55–71. doi:10.12700/aph.16.6.2019.6.5
Horváth, I. (2020). “Personalized Learning Opportunity in 3d Vr,” in 2020 11th IEEE International Conference on Cognitive Infocommunications (CogInfoCom) (New Jersey: IEEE), 000425–000430. doi:10.1109/coginfocom50765.2020.9237895
Horváth, I., and Sudár, A. (2018). Factors Contributing to the Enhanced Performance of the Maxwhere 3d Vr Platform in the Distribution of Digital Information. Acta Polytechnica Hungarica 15, 149–173. doi:10.12700/aph.15.3.2018.3.9
Horváth, I. (2019b). “The Edu-Coaching Method in the Service of Efficient Teaching of Disruptive Technologies,” in Cognitive Infocommunications, Theory and Applications (New York: Springer), 349–363. doi:10.1007/978-3-319-95996-2_16
Hübscher, R. (2000). “Logically Optimal Curriculum Sequences for Adaptive Hypermedia Systems,” in International conference on adaptive hypermedia and adaptive web-based systems (New York: Springer), 121–132. doi:10.1007/3-540-44595-1_12
Jacoby, B. (1996). “Service-learning in Today’s Higher Education,” in Service-Learning in Higher Education: Concepts and Practices. Editors B. Jacoby and Associates (San Francisco, CA: Jossey-Bass), 3–25.
Katona, J., and Kovari, A. (2018). Examining the Learning Efficiency by a Brain-Computer Interface System. Acta Polytechnica Hungarica 15, 251–280. doi:10.12700/aph.15.3.2018.3.14
Kayes, A. B., Kayes, D. C., and Kolb, D. A. (2005). Experiential Learning in Teams. Simulation & Gaming 36, 330–354. doi:10.1177/1046878105279012
Kayes, D. C. (2005). Internal Validity and Reliability of Kolb's Learning Style Inventory Version 3 (1999). J. Bus Psychol. 20, 249–257. doi:10.1007/s10869-005-8262-4
Komlósi, L. I., and Waldbuesser, P. (2015). “The Cognitive Entity Generation: Emergent Properties in Social Cognition,” in 2015 6th IEEE International Conference on Cognitive Infocommunications (CogInfoCom) (New Jersey: IEEE), 439–442. doi:10.1109/coginfocom.2015.7390633
Kovari, A., Katona, J., and Costescu, C. (2020). Quantitative Analysis of Relationship between Visual Attention and Eye-Hand Coordination. Acta Polytech Hung 17, 77–95. doi:10.12700/aph.17.2.2020.2.5
Kövecses-Gõsi, V. (2019). “The Pedagogical Project of Education for Sustainable Development in 3d Virtual Space,” in 2019 10th IEEE International Conference on Cognitive Infocommunications (CogInfoCom) (New Jersey: IEEE), 000539–000544. doi:10.1109/coginfocom47531.2019.9090000
Kuczmann, M., and Budai, T. (2019). Linear State Space Modeling and Control Teaching in Maxwhere Virtual Laboratory. Acta Polytechnica Hungarica 16, 27–39. doi:10.12700/aph.16.6.2019.6.3
Kvasznicza, Z., Kovács, J., Maza, G., and Péli, M. (2019). “Vr based duale education at e. on the win-win-win situation for companies, graduates and universities,” in 2019 10th IEEE International Conference on Cognitive Infocommunications (CogInfoCom) (New Jersey: IEEE), 479–482. doi:10.1109/coginfocom47531.2019.9089961
Kvasznicza, Z. (2017). “Teaching Electrical Machines in a 3d Virtual Space,” in 2017 8th IEEE International Conference on Cognitive Infocommunications (CogInfoCom) (New Jersey: IEEE), 000385–000388. doi:10.1109/coginfocom.2017.8268276
Kővári, A. (2019). Adult Education 4.0 and Industry 4.0 Challenges in Lifelong Learning. PedActa 9, 9–16.
Lampert, B., Pongrácz, A., Sipos, J., Vehrer, A., and Horvath, I. (2018). Maxwhere Vr-Learning Improves Effectiveness over Clasiccal Tools of E-Learning. Acta Polytechnica Hungarica 15, 125–147. doi:10.12700/aph.15.3.2018.3.8
Lee, M.-G. (2001). Profiling Students' Adaptation Styles in Web-Based Learning. Comput. Edu. 36, 121–132. doi:10.1016/s0360-1315(00)00046-4
Logie, R. H. (1986). Visuo-spatial Processing in Working Memory. The Q. J. Exp. Psychol. Section A 38, 229–247. doi:10.1080/14640748608401596
Markopoulos, E., Markopoulos, P., Laivuori, N., Moridis, C., and Luimula, M. (2020). “Finger Tracking and Hand Recognition Technologies in Virtual Reality Maritime Safety Training Applications,” in 2020 11th IEEE International Conference on Cognitive Infocommunications (CogInfoCom) (New Jersey: IEEE), 000251–000258. doi:10.1109/coginfocom50765.2020.9237915
Orosz, B., Kovács, C., Karuović, D., Molnár, G., Major, L., Vass, V., et al. (2019). Digital Education in Digital Cooperative Environments. J. Appl. Tech. Educ. Sci. 9, 55–69.
Papanikolaou, K. A., Grigoriadou, M., Magoulas, G. D., and Kornilakis, H. (2002). Towards New Forms of Knowledge Communication: the Adaptive Dimension of a Web-Based Learning Environment. Comput. Edu. 39, 333–360. doi:10.1016/s0360-1315(02)00067-2
Pashler, H., McDaniel, M., Rohrer, D., and Bjork, R. (2008). Learning Styles. Psychol. Sci. Public Interest 9, 105–119. doi:10.1111/j.1539-6053.2009.01038.x
Rodrıguez, F., López, J. C., Vargas, J. P., Broglio, C., Gómez, Y., and Salas, C. (2002). Spatial Memory and Hippocampal Pallium through Vertebrate Evolution: Insights from Reptiles and Teleost Fish. Brain Res. Bull. 57, 499–503.
Shams, L., and Seitz, A. R. (2008). Benefits of Multisensory Learning. Trends Cognitive Sciences 12, 411–417. doi:10.1016/j.tics.2008.07.006
Sztahó, D., Szaszák, G., and Beke, A. (2019). Deep Learning Methods in Speaker Recognition: a Review. arXiv preprint arXiv:1911.06615.
Tang, T. Y., and McCalla, G. (2003). “Smart Recommendation for an Evolving E-Learning System,” in Workshop on Technologies for Electronic Documents for Supporting Learning, International Conference on Artificial Intelligence in Education, Sydney, Australia, 699–710.
Turesky, E. F., and Gallagher, D. (2011). Know Thyself: Coaching for Leadership Using Kolb’s Experiential Learning Theory. Coaching Psychol. 7, 5–14.
Vicsi, K., Roach, P., Öster, A., Kacic, Z., Barczikay, P., Tantos, A., et al. (2000). A Multimedia, Multilingual Teaching and Training System for Children with Speech Disorders. Int. J. speech Technol. 3, 289–300. doi:10.1023/a:1026563015923
Keywords: cognitive infocommunications, learning efficiency, affective learning, personalization, multi-sensory education, VR learning
Citation: Horváth I (2021) An Analysis of Personalized Learning Opportunities in 3D VR. Front. Comput. Sci. 3:673826. doi: 10.3389/fcomp.2021.673826
Received: 28 February 2021; Accepted: 24 August 2021;
Published: 20 September 2021.
Edited by:
Carl Vogel, Trinity College Dublin, IrelandReviewed by:
Borbála Berki, Széchenyi István University, HungaryCopyright © 2021 Horváth. This is an open-access article distributed under the terms of the Creative Commons Attribution License (CC BY). The use, distribution or reproduction in other forums is permitted, provided the original author(s) and the copyright owner(s) are credited and that the original publication in this journal is cited, in accordance with accepted academic practice. No use, distribution or reproduction is permitted which does not comply with these terms.
*Correspondence: Ildikó Horváth, aG9ydmF0aC5pbGRpa29AZ2Euc3plLmh1
Disclaimer: All claims expressed in this article are solely those of the authors and do not necessarily represent those of their affiliated organizations, or those of the publisher, the editors and the reviewers. Any product that may be evaluated in this article or claim that may be made by its manufacturer is not guaranteed or endorsed by the publisher.
Research integrity at Frontiers
Learn more about the work of our research integrity team to safeguard the quality of each article we publish.