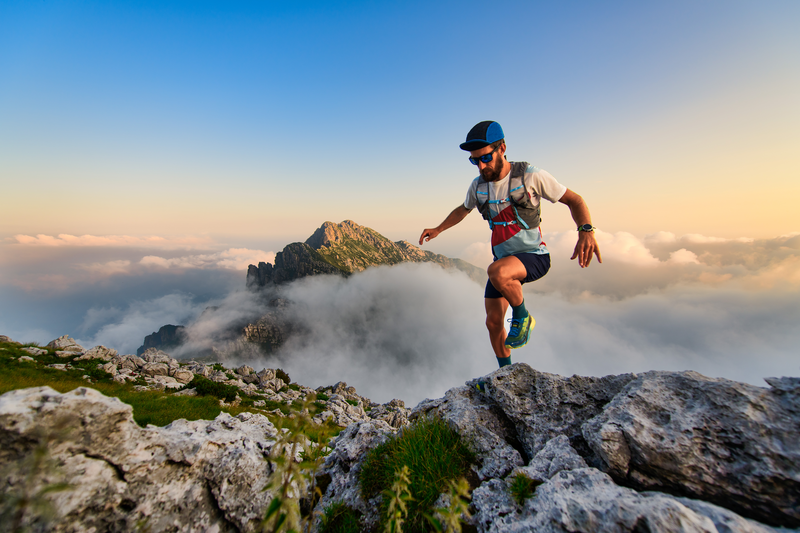
95% of researchers rate our articles as excellent or good
Learn more about the work of our research integrity team to safeguard the quality of each article we publish.
Find out more
ORIGINAL RESEARCH article
Front. Chem. , 31 March 2025
Sec. Catalytic Reactions and Chemistry
Volume 13 - 2025 | https://doi.org/10.3389/fchem.2025.1557628
The fabrication of core-shell structured magnetic resorcinol-formaldehyde composites has garnered considerable attention within the scientific community in recent years. A key area of focus has been the immobilization of homogeneous catalysts onto the surfaces of these materials and transforming them into heterogeneous catalysts. In this study, a novel quaternary ammonium salt catalyst was synthesized by immobilizing 1,4-diazabicyclo [2.2.2] octane (DABCO) on resorcinol-formaldehyde-modified Fe3O4 nanocomposite as a support (Fe3O4@RF/Pr-DABCO). The Fe3O4@RF/Pr-DABCO nanocomposite was characterized using various physicochemical techniques, including FT-IR, VSM, SEM, XRD, and TGA. The Fe3O4@RF/Pr-DABCO nanocomposite was employed as a power nanocatalyst in the Hantzsch reaction for synthesizing polyhydroquinoline derivatives using aromatic aldehydes, ammonium acetate, dimedone and ethyl acetoacetate. Various aromatic aldehydes were used as substrates in the presence of 0.003 g of Fe3O4@RF/Pr-DABCO under solvent-free condution at 60 °C, achieving high to excellent yields (90-99%) within short reaction times (5-15 min). Furthermore, this nanocatalyst showed excellent reusability and maintained its catalytic activity for at least eight consecutive cycles without a significant decrease in efficiency. These results demonstrate the potential of the Fe3O4@RF/Pr-DABCO nanocomposite as an efficient and sustainable catalyst for the synthesis of polyhydroquinoline derivatives via the Hantzsch reaction.
Aligned with the principles of green chemistry, the development of recyclable and reusable catalytic systems has garnered significant interest among researchers over the past few decades. This focus on sustainable catalyst design aims to address environmental concerns and promote resource efficiency in chemical processes (Saberi et al., 2020). In recent years, the design of magnetic nanocatalysts is of great interest due to their very easy recovery using a simple magnet without the need for conventional and time-consuming filtration and reactions catalyzed by magnetic nanocatalysts have been widely studied due to the easy purification of products and efficient recovery of the catalyst (Shabir et al., 2020; Yazdani et al., 2025). Various magnetic nanoparticles (NPs) have been investigated for the development of magnetic catalytic systems. Among magnetic NPs, Fe3O4 NPs has been widely considered due to its low toxicity, easy and low-cost synthesis from cheap and readily available starting materials. Despite the promising attributes of Fe3O4 NPs, their practical applications are hindered by their high sensitivity to oxidation and aggregation, as well as their chemically reactive nature due to their high surface area (Serenjeh et al., 2015; Shaker and Elhamifar, 2020; Barzkar and Beni, 2023). These limitations necessitate the development of strategies to overcome these challenges and unlock the full potential of Fe3O4 NPs. One effective approach involves the creation of a suitable organic or inorganic coating on the surface of the magnetic NPs, which can enhance their stability and mitigate the aforementioned issues, thus paving the way for broader applications in various fields. Various materials have been utilized for coating magnetic nanoparticles, including silica, metal oxides, and polymers (Gong et al., 2018; Kilic et al., 2019; Shaker and Elhamifar, 2020; 2021b; Kilic et al., 2022). Notably, resorcinol-formaldehyde (RF) resin has emerged as a particularly promising option due to its exceptional properties. These properties encompass high stability, cost-effectiveness, facile structural manipulation, superior mechanical and thermal characteristics, impressive electrical conductivity, and a large surface area. The unique combination of these properties makes RF resin an attractive choice for coating magnetic nanoparticles, with the potential to enhance their performance and broaden their applications in diverse fields (Barzkar and Beni, 2020). Some of the recently developed reports include Fe3O4@RF-Au (Shi et al., 2024), Fe3O4@RF@void@PMO (Yu et al., 2019), Fe3O4@RF@void@PMO(IL)/Cu (Shaker and Elhamifar, 2021a), Fe3O4@SiO2@RF–SO3H (Barzkar and Beni, 2020) and Fe3O4@RF/Cu2O (Wang et al., 2017).
On the other hand, 1,4- Diazabicyclo [2.2.2] octane, also recognized as DABCO is known as a valuable ligand and catalyst for organic reactions due to its cost-effectiveness, environmental friendliness, non-toxicity, and high reactivity. However, recovering and removing DABCO in chemical reactions is complex. This problem has been solved by immobilizing DABCO on solid substrates, which can be easily filtered and reused multiple times (Baharfar and Azimi, 2014). Recent developments in this area, including Fe3O4@DT-Au (Kardan et al., 2022), Pd–DABCO@SiO2 (Jadhav et al., 2019), Ni(II)–DABCO@SiO2 (Hajipour and Abolfathi, 2017), Pd-DABCO-γ-Fe2O3 (Sobhani and Pakdin-Parizi, 2014), SB-DABCO (Hasaninejad et al., 2011) and Fe3O4@SiO2@DABCO (Gupta et al., 2023).
Meanwhile, heterocycles are a crucial and valuable group of chemical compounds, forming the basis of many pharmaceutical molecules and antibiotics. Currently, Synthesis of nitrogen-containing heterocycles, such as polyhydroquinoline and its derivatives, is important due to their medicinal properties. Polyhydroquinolines possess anti-diabetic, anti-cancer, anti-tumor, liver-protective, and skin-protective properties and treat cardiovascular diseases and Alzheimer’s (Shinde and Thakur, 2023). Polyhydroquinoline derivatives can be synthesized using the Hantzsch reaction, which has garnered significant attention. This reaction is a four-component reaction and is one of the first and most well-known multi-component reactions (Sajjadifar and Azmoudehfard, 2019; Shaker and Beni, 2021; Norouzi and Beiranvand, 2023). Due to the widespread use of polyhydroquinoline derivatives, various catalysts have been introduced for this reaction such as GuHCl (Baghbanian et al., 2010), ceric ammonium nitrate (CAN) (Ko and Yao, 2006), Sc(OTf)3 (Donelson et al., 2006), urease (Zhu and Li, 2021), palladium (0) nanoparticles (Saha and Pal, 2011), nickel nanoparticles (Sapkal et al., 2009), SBA-15@AMPD-Co (Ghorbani-Choghamarani et al., 2019), [βCD/Im](OTs)2 (Moheiseni et al., 2021), NiFe2O4MNPs (Ahankar et al., 2016), Fe3O4adenineNi (Tamoradi et al., 2018), CuSPATB/Fe3O4 (Ghorbani-Choghamarani et al., 2016), Fe3O4@SiO2PEG/NH2 (Kardooni et al., 2017) and Fe3O4 @SiO2@(CH2)3Im}C(NO2)3 (Yarie et al., 2016). Although most of the reports mentioned have obvious advantages, they also come with problems, such as toxic catalysts, volatile and harmful solvents, expensive metal precursors, high temperatures, the formation of by-products, and contamination of the final product. In most methods, the catalysts get damaged during the reaction and cannot be separated and reused. Therefore, developing efficient, recyclable, and environmentally friendly catalysts for synthesizing polyhydroquinolines is crucial (Kazemi and Mohammadi, 2020; Sharma et al., 2021).
In light of the significance of heterogeneous catalysis and the unique properties of 1,4-diazabicyclo [2.2.2]octane (DABCO), this study aims to design and synthesize a novel core-shell structured Fe3O4@RF/Pr-DABCO catalyst (Scheme 1). DABCO, selected for its crucial role as an organic group in preparing quaternary ammonium salt catalysts, can be readily immobilized on the Fe3O4@RF support. This approach offers the potential to create a highly efficient and recyclable catalytic system that capitalizes on the benefits of both the magnetic core and the catalytic activity of DABCO. Also, Fe3O4@RF/Pr-DABCO is applied as a powerful catalyst for synthesizing polyhydroquinoline derivatives under mild conditions.
All chemicals are used, such as formaldehyde solution (37 wt%), resorcinol, triethylamine, (3-aminopropyl)-trimethoxysilane, dimedone, ethyl acetoacetate, toluene dried, and ammonium acetate, were purchased from Merck, hydrochloric acid (37%) and Benzaldehyde and its derivatives were purchased from Sigma-Aldrich. The morphology of the particles was evaluated by TESCAN-Vega 3 scanning electron microscope (SEM) (Czech republic). Energy-dispersive X-ray spectroscopy (EDX) was obtained by using TESCAN-Vega 3 apparatus (Czechrepublic). Fourier transform infrared (FT-IR) spectroscopy was recorded on a PerkinElmer Spectrum2 spectrometer (United States). Thermal gravimetric analysis (TGA) and Differential Scanning (DSC) Calorimetry were performed using a SDT 650 (United States). Thermal gravimetric analysis (TGA) and differential thermal analysis (DTA) were performed using a STA6000 (United States). X-ray diffraction (XRD) was obtained using a Rigaku Ultima IV diffractometer (Japan). The magnetic properties of the particles were investigated using a vibrating sample magnetometer (VSM) of MDKB. (Iran). Melting points were determined using a KSB1N, Kruss apparatus in open capillary tubes (Germany). TLC-Grade-silica Gel-G/UV 254 thin layer chromatography (TLC) was used. A mixture of ethyl acetate and normal hexane was used for the mobile phase in the TLC tank. Ultrasonic model Elmasonic P60H was used to disperse the particles and to perform the organic reactions (Germany).
In a 100 mL round-bottom flask, 25 mL HCl (2 M), FeCl3.6H2O (5.2 g), and FeCl2.4H2O (2 g) were combined with a magnetic stirrer under argon atmosphere for 30 min at room temperature to form nanoparticles of Fe3O4 magnetite. Next, NH4OH (25%, 30 mL), was slowly injected into the solution over 30 min at 25°C. The obtained black precipitate was separated by a magnet and washed with water before drying in an oven (Kargar et al., 2024).
In a 250 mL round-bottom flask, 1 g Fe3O4 particles were dispersed in water (20 mL) and ethanol (100 mL) using ultrasonic. Then (2 mL) of 25% ammonium hydroxide, formaldehyde (0.6 mL), and resorcinol (0.4 g) were added to the solution. The mixture was stirred for 10 h at 30°C under a magnetic stirrer and argon atmosphere, then was collected using a magnet and washed with water and ethanol three times, before finally drying at 60°C for 15 h (Mirbagheri et al., 2021).
For this aim, in a 100 mL round-bottom flask, Fe3O4@RF nanoparticles (50 mg) were added to 50 mL of dry toluene and dispersed under ultrasonic waves. After that, 2 mL of (3-chloropropyl) trimethoxysilane was injected into the reaction. After refluxing under a magnetic stirrer and argon atmosphere for 24 h at 110°C, an external magnet filtered the product. The resultant product was washed with toluene and dried at 70°C for 15 h (Zare et al., 2020).
In a 50 mL round-bottom flask, Fe3O4@RF/PrCl (1 g), DABCO (0.5 g), and triethylamine (0.4 mL) were dispersed under ultrasonic in 30 mL of dry toluene for 30 min. After 24 h of refluxing under a magnetic stirrer and argon atmosphere, the mixture was separated using a magnet, washed five times with dry toluene, and dried at 50°C for 5 h (Afsar et al., 2018).
For this, aldehyde (1 mmol), ammonium acetate (1.4 mmol), dimedone (1 mmol), ethyl acetoacetate (1 mmol), and Fe3O4@RF/Pr-DABCO nanocatalyst (0.003 g) were added into a 5 mL round-bottom flask. The reaction mixture was located in an oil bath at 60°C, the temperature of which was previously adjusted by a heater-stirrer advice, and stirred under solvent-free condition and air atmosphere. The progress of the reaction was monitored by TLC (eluent; n-hexane: ethyl acetate, 4:6). After completion of the reaction, hot EtOH (10 mL) was added in the reaction vessel and catalyst was removed by an external magnet. Finally, the solvent was evaporated, and pure products resulted after recrystallization in EtOH (Nikoorazm and Erfani, 2019).
The FT-IR spectra of Fe3O4, RF, Fe3O4@RF, Pr-Cl, Fe3O4@RF/Pr-Cl, DABCO, and Fe3O4@RF/Pr-DABCO are shown in Figure 1. For Fe3O4, Fe3O4@RF, Fe3O4@RF/Pr-Cl and Fe3O4@RF/Pr-DABCO, the observed peak at 574 cm−1 is related to the stretching vibrations of the Fe-O bonds (Figures 1d–g). The FT-IR spectrum of Fe3O4@ RF (Figure 1e) exhibits peaks at 3,010, 2,854–2,974 cm−1attributable to the vibrations of C-H aromatic and CH2 moieties of RF resin in Fe3O4@RF, respectively. Also, the absorption bands at 1,615 and 1,453 cm−1 correspond to the aromatic rings in the RF. These results are in perfect agreement with the RF spectrum (Figure 1c), thus confirming the successful formation of RF resin on the Fe3O4 surface. (Pol et al., 2014; Barzkar and Beni, 2020). The FT-IR spectrum of Fe3O4@RF/Pr-Cl (Figure 1f) shows the C-H stretching vibration at 2,950 and 2,826 cm−1, the C–Cl absorption band at 800 cm−1, and Si-O stretching at 1,000–1,100 cm−1, which perfectly match with the observed peaks in the pure Pr-Cl spectrum (Figure 1b). These results prove the successful formation of the Fe3O4@RF/Pr-Cl composite (Hamidinasab et al., 2020; Honari et al., 2022). FT-IR pure DABCO (Figure 1a) exhibits a peak at 1,250 cm−1 (C–N stretching), 1,475 cm−1 (CH2 bending), and 2,850–2,964 cm−1 (C-H stretching vibrations) (Tafti et al., 2024). Thus, for the Fe3O4@RF/Pr-DABCO, the presence of a peak at 1,250 cm−1 (C–N stretching) proved immobilization of DABCO groups on the of Fe3O4@RF/Pr-Cl composite (Figure 1g) (Hasaninejad et al., 2011; Nasseri and Sadeghzadeh, 2014).
Figure 1. FT-IR spectrum of (a) DABCO, (b) Pr-Cl, (c) RF, (d) Fe3O4, (e) Fe3O4@RF, (f) Fe3O4@RF/Pr-Cl, (g) Fe3O4@RF/Pr-DABCO.
The EDX spectrum of Fe3O4 nanoparticles demonstrated the presence of Fe and O elements, proving the successful production of these nanoparticles (Figure 2a). To confirm the successful modification of the Fe3O4 surface with RF resin, the Fe3O4@RF material was also characterized by EDX analysis. The appearance of C-signal in the latter analysis confirms successful-production of RF resin on Fe3O4 surface (Figure 2b). Furthermore, to prove the successful chemical immobilization of (3-chloropropyl) trimethoxysilane on Fe3O4@RF nanocomposite, the Fe3O4@RF/PrCl nanocomposite was also characterized by EDX analysis. The advent of Si and Cl elements proving successful-immobilization of (3-chloropropyl) trimethoxysilane species on Fe3O4@RF nanocomposite (Figure 2c). Finally, to confirm the successful immobilization of DABCO on Fe3O4@RF/PrCl nanocomposite, the final material was characterized by EDX and the appearance of N-signal confirmed immobilization of DABCO species (Figure 2d). The EDX mapping analysis was also done to display the dispensation of elements in the Fe3O4@RF/Pr-DABCO framework. This analysis demonstrated that all elements are uniformly distributed in the Fe3O4@RF/Pr-DABCO framework (Figure 3).
Figure 2. EDX spectrum of the (a) Fe3O4, (b) Fe3O4@RF, (c) Fe3O4@RF/Pr-Cl, and (d) Fe3O4@RF/Pr-DABCO.
The morphology of the particles at different steps of nanocomposite preparation was investigated by using SEM (Figure 4). The SEM analysis revealed a consistent spherical morphology with uniform particle size distribution at different stages of the process. Additionally, it was observed that the size of the nanoparticles (NPs) increased incrementally at each step compared to the previous stage.
The powder X-ray diffraction (PXRD) analysis of Fe3O4, Fe3O4@RF, Fe3O4@RF/Pr-Cl, and Fe3O4@RF/Pr-DABCO nanomaterials showed six sharp peaks at 2θ = 30.3, 35.6, 43.4, 53.7, 57.4, and 63.1°, corresponding to Miller indices of 220, 311, 400, 422, 511, and 440, respectively. The results of this analysis prove that the Fe3O4 crystalline structure is preserved during the synthesizing processes of Fe3O4@RF/Pr-DABCO nanocamposite (Figure 5) (Hashkavayi and Raoof, 2017).
Figure 5. XRD pattern of the (a) Fe3O4, (b) Fe3O4@RF, (c) Fe3O4@RF/Pr-Cl, and (d) Fe3O4@RF/Pr-DABCO.
The magnetic properties of Fe3O4, Fe3O4@RF, Fe3O4@RF, Fe3O4@RF/Pr-Cl, and Fe3O4@RF/Pr-DABCO nanomaterials were investigated by VSM \analysis at room temperature (Figure 6). The results of this analysis demonstrated that all samples have a superparamagnetic behavior. The magnetic saturation of Fe3O4, Fe3O4@RF, Fe3O4@RF/Pr-Cl, and Fe3O4@RF/Pr-DABCO nanomaterials were 61.98, 53.87, 42.23, and 41.96 emu/g, respectively. The decrease in saturation magnetization, after each step, confirms successful chemical immobilization of Resorcinol-Formaldehyde precursors and DABCO moieties on the surface of the Fe3O4 NPs. However, the magnetic property of Fe3O4@RF/Pr-DABCO is still sufficient, and it can be easily recovered using an external magnet.
To further investigate the compositional structure and thermal stability, thermogravimetric analysis (TGA), derivative thermogravimetry (DTG), differential thermal analysis (DTA), and Differential Scanning Calorimetry (DSC) were performed at different stages of nanocatalyst synthesis (Figures 7A–D). The TGA diagram of Fe3O4 (Figure 7Aa) shows two mass loss stages. The first stage, with a mass loss of 1.66%, at temperatures between 25°C and 200°C, is related to water evaporation, and the second stage, with a mass loss of 1.96%, at temperatures between 200°C and 600°C, is connected to the decomposition of hydroxyl groups on the Fe3O4 surface., the final mass loss is 3.62% (Zhang et al., 2021). The mass loss graph of Fe3O4@RF (Figure 7Ab) shows two stages of mass loss. In the first stage, at temperatures below 200°C, due to the evaporation of water or residual physically adsorbed solvent, the observed mass loss is 2.55%, While the second mass loss15.13% between 200°C and 469°C is related to the decomposition of the RF shell, the final mass loss is 17.68% (Zheng et al., 2016). The mass loss graph of Fe3O4@RF/Pr-Cl (Figures 7Ac) has two stages of mass loss. The first mass loss of about 2.49% is related to the residual other solvents or water on the surface of magnetic nanoparticles, which appears below 200°C. The main weight loss, with a mass loss of 18.59%, from 200°C to 600°C, is due to the decomposition of the chloropropyl group and RF shell; the final mass loss is 21.08% (Dehghani et al., 2025). TGA of Fe3O4@RF/Pr-DABCO shows two distinct stages of mass loss (Figures 7Ad). The first stage, with an onset of degradation at 50°C–200°C, with mass loss of 1.85%is caused by the evaporation of H2O or residual solvent on the catalyst’s surface. The second stage, between 200°C and 700°C, with mass loss of 20.78%, is related to continuous decomposition of the organic components (DABCO, propyl group and RF shell). The final mass loss is 22.63% (Rajabi-Salek et al., 2018; Jadidi Nejad et al., 2020; Jia et al., 2022).
Figure 7. (A) TGA (B) DTG, (C) DTA, and (D) DSC of the (A) Fe3O4, (B) Fe3O4@RF, (C) Fe3O4@RF/Pr-Cl, and (D) Fe3O4@RF/Pr-DABCO.
TGA and DTG (Figure 7B) data were supported by DTA and DSC curves. Based on DTA and DSC (Figures 7C, D), the separation process of organic materials attached to the surface of Fe3O4@RF/Pr-DABCO nanocatalyst is exothermic, and the different intensity of the peaks indicates the synthesis of different stages of the nanocatalyst.
Comparison of the mass loss curve and thermal stability (TGA) graph analysis of each step of the synthesis of the Fe3O4@RF/Pr-DABCO magnetic nanocatalyst confirmed that all four steps of the nanocatalyst synthesis were successfully completed and the alkyl group and DABCO was loaded onto the surface of the Fe3O4@RF particles. Also, the TGA thermograms of the product obtained at each stage of synthesis are parallel to the elemental composition obtained by EDX.
Following the successful characterization of Fe3O4@RF/Pr-DABCO, the effectiveness of the catalyst was assessed in the green production of polyhydroquinoline. The condensation between 1.4 mmol of NH4OAc, 1 mmol of benzaldehyde, 1 mmol of ethyl acetoacetate, and 1 mmol of dimedone was chosen as a model reaction. The findings indicate that the catalyst, solvent, and temperature significantly affect the reaction’s progression. Consequently, the amount of catalyst, choice of solvent, and temperature were evaluated to enhance the reaction conditions.
Initially, to evaluate the effectiveness of the Fe3O4@RF/Pr-DABCO catalyst, it was observed that the reaction did not occur in the absence of the catalyst, indicating its essential role in the process (Table 1, entry 1). To further examine the catalyst’s impact, we tested various amounts of the catalyst (1, 3, 5, 7, and 9 mg). As detailed in Table 1 entries 2–6, the reaction yield improved with increased catalyst quantity from 0.001 g to 0.003 g (Table 1, entry 3). The optimal yield was achieved with 0.003 g of the catalyst (Table 1, entry 3). Notably, a catalyst amount of 0.005 g produced the same yield as 0.003 g (Table 1, entry 4). Additionally, increasing the catalyst amount from 0.007 to 0.009 g resulted in a minor reduction in product yield. Studies have shown that excessive amounts of catalyst alter the reaction mechanism and increase side reactions, leading to the formation of unwanted by-products. This change reduces the selectivity towards the desired product and consequently reduces its yield (Chen et al., 2021; Platero et al., 2022).
Table 1. The reaction conditions optimized for the synthesis of polyhydroquinolines by Fe3O4@RF/Pr-DABCO
The model reaction was subsequently evaluated at various temperatures, specifically 25°C, 40°C, 60°C, 70°C, and 90°C (Table 1, entry 3 vs. entries 7–10). An increase in temperature from 25°C to 60°C resulted in enhanced reaction efficiency, with the optimal yield observed at 60°C (Table 1, entry 3). Additionally, satisfactory efficiency was noted at 70°C (Table 1, entry 9). However, as the temperature rose to 90°C, there was a decline in product yield (Table 1, entry 10). Studies have shown that by-products are formed at 90°C, and therefore the yield of the main product is reduced.
This study examined the impact of various solvents, such as dimethyl sulfoxide, toluene, water, acetonitrile, and ethanol, as well as solvent-free conditions (Table 1, entry 3 vs. entries 11–15). The findings indicated that the highest yield achieved was 97% under solvent-free conditions. Because DABCO is an organic compound and the solvents are all organic, the interaction between DABCO and the solvents increases, and the accumulation of substrate around the catalytic active site decreases, and the catalytic activity of DABCO decreases compared to solvent-free conditions. In the presence of ethanol and water solvents, although these solvents also interact with DABCO and reduce its catalytic activity, these solvents can have hydrogen interactions with the substrates, causing substrate activation and facilitating the reaction conditions. However, in general, solvent-free conditions are the best option for this process and do not reduce catalytic activity (Lu et al., 2012; Varghese and Mushrif, 2019).
To ascertain the pivotal role of DABCO groups in the catalytic cycle, the efficacy of DABCO-free Fe3O4, Fe3O4@RF, and Fe3O4@RF/PrCl nanomaterials was investigated under identical reaction conditions and time as the Fe3O4@RF/Pr-DABCO catalyst (Table 1, entry 3 vs. entries 16–18). Notably, the latter catalysts exhibited negligible product yields, unequivocally establishing the indispensable presence of DABCO species as crucial catalytic centers for the reaction.
A diverse range of polyhydroquinolines was synthesized under optimal conditions, utilizing 0.003 g of a solvent-free catalyst at 60°C with Fe3O4@RF/Pr-DABCO as a heterogeneous catalyst. As detailed in Table 2, various benzaldehydes featuring both electron-donating and electron-withdrawing groups were employed in the synthesis of polyhydroquinolines, resulting in products characterized by high yields, brief reaction times, and precise melting point measurements (Table 2, entries 1–7).
The recovery and reusability of the catalyst were tested for eco-friendly industrial and commercial applications. A test model evaluated the condensation of 0.003 mg of the catalyst with ethyl acetoacetate (1 mmol), dimedone (1 mmol) benzaldehyde (1 mmol), and NH4OAc (1.4 mmol). The catalyst was isolated from the solution following each run through an external magnet, washed with ethanol, dried, and then used again in another reaction to regenerate it. According to Figure 8, the recycling process can be conducted under identical conditions to the initial run at least eight times without experiencing any substantial loss.
In another experiment, the recoverability and reusability of the Fe3O4@RF/Pr-DABCO catalyst were investigated at a fixed time under optimal conditions. In this test, the reaction time for each run was 30 min. Following each run, the catalyst was separated using a magnetic field and subsequently reused in the next run, under the same conditions as the first run. As illustrated in Figure 9, this catalyst can be recovered and reused at least 8 times without a substantial decrease in its performance.
Scheme 2 presents a proposed mechanism for synthesizing polyhydroquinolines using the Fe3O4@RF/Pr-DABCO as a catalyst. Initially, the carbanion group (A) is generated through the deprotonation of the α-proton of dimedone by the basic nitrogen group of the catalyst, DABCO. Subsequently, intermediate (B) is formed via a Knoevenagel condensation reaction between carbanion (A) and an aldehyde. The formation of intermediate (C) occurs after the elimination of a water molecule from the reaction involving ammonia (derived from ammonium acetate) and ethyl acetoacetate. The Michael addition of intermediate (B) with (C) results in the formation of intermediate (D). Ultimately, the nanocatalyst facilitates the preparation of the final product by promoting the cyclization of (D) and the removal of a water molecule (Al Anazi et al., 2023).
Scheme 2. A suitable catalytic mechanism for the production of polyhydroquinolines using Fe3O4@RF/Pr-DABCO
We investigated the catalytic performance of magnetic Fe3O4@RF/Pr-DABCO in producing polyhydroquinoline derivatives and compared it to previously reported nanocatalysts (Table 3). The results show that our designed catalyst is cost-effective, easy to use, and highly efficient, yielding high amounts of polyhydroquinoline derivatives under typical reaction conditions. Therefore, this catalyst is comparable to or even better than previous catalysts in Hantzsch’s synthesis of polyhydroquinolines in terms of reaction conditions, yield, and ease of magnetic recovery.
In summary, a novel magnetic RF modified with DABCO (Fe3O4@RF/Pr-DABCO) was successfully synthesized. The EDX and FT-IR analyses confirmed successful chemical immobilization of resorcinol-formaldehyde precursors and DABCO moieties on the surface of the Fe3O4 NPs. Also, TGA analysis proved the good immobilization of resorcinol-formaldehyde resin and DABCO moieties onto Fe3O4 NPs and showed the high thermal stability of the Fe3O4@RF/Pr-DABCO nanocomposite. The SEM image showed that the Fe3O4@RF/Pr-DABCO nanostructures are spherical and regular. Also, The XRD demonstrated the structure of Fe3O4 is not changed under the conditions of the synthesis of Fe3O4@RF/Pr-DABCO nanocomposite. Finally, its catalytic application was studied as a catalyst for synthesizing polyhydroquinoline derivatives. This nanocatalyst, showed excellent catalytic activity with a minimum amount (0.003 g) at an optimum temperature of 60°C in only 15 min and effectively led to the formation of products with high yields (97%–99%). Additionally, the Fe3O4@RF/Pr-DABCO nanocatalyst can be easily recovered using an external magnet and could be reused up to eight times without any significant loss of its catalytic activity.
The original contributions presented in the study are included in the article/supplementary material, further inquiries can be directed to the corresponding authors.
ME: Formal Analysis, Investigation, Resources, Writing–original draft. AS: Conceptualization, Supervision, Visualization, Writing–review and editing.
The author(s) declare that no financial support was received for the research and/or publication of this article.
The authors thank the Yasouj University for supporting this work.
The authors declare that the research was conducted in the absence of any commercial or financial relationships that could be construed as a potential conflict of interest.
The author(s) declare that no Generative AI was used in the creation of this manuscript.
All claims expressed in this article are solely those of the authors and do not necessarily represent those of their affiliated organizations, or those of the publisher, the editors and the reviewers. Any product that may be evaluated in this article, or claim that may be made by its manufacturer, is not guaranteed or endorsed by the publisher.
Afsar, J., Zolfigol, M. A., Khazaei, A., Alonso, D. A., Khoshnood, A., Bayat, Y., et al. (2018). Synthesis and application of a novel nanomagnetic catalyst with Cl [DABCO-NO 2] C (NO 2) 3 tags in the preparation of pyrazolo [3, 4-b] pyridines via anomeric based oxidation. Res. Chem. Intermed. 44, 7595–7618. doi:10.1007/s11164-018-3576-9
Ahankar, H., Ramazani, A., and Joo, S. W. (2016). Magnetic nickel ferrite nanoparticles as an efficient catalyst for the preparation of polyhydroquinoline derivatives under microwave irradiation in solvent-free conditions. Res. Chem. Intermed. 42, 2487–2500. doi:10.1007/s11164-015-2163-6
Al Anazi, A. A., Satar, R., Jabbar, H. S., Sapaev, I., Altalbawy, F. M., Alameri, A. A., et al. (2023). Effectual and rapid synthesis of Hantzsch derivatives in solvent-free conditions catalyzed by a mesoporous basic silica-based nanomaterial. Silicon 15, 3453–3461. doi:10.1007/s12633-022-02275-5
Aute, D., Kshirsagar, A., Uphade, B., and Gadhave, A. (2020a). Aluminized polyborate-catalysed green and efficient synthesis of polyhydroquinolines under solvent-free conditions. Res. Chem. Intermed. 46, 3491–3508. doi:10.1007/s11164-020-04158-z
Aute, D., Kshirsagar, A., Uphade, B., and Gadhave, A. (2020b). Sulfated polyborate as an eco-compatible solid acid catalyst for efficient and facile solvent-free synthesis of polyhydroquinolines. J. Chem. Sci. 132, 147–210. doi:10.1007/s12039-020-01850-w
Baghbanian, S. M., Khaksar, S., Vahdat, S. M., Farhang, M., and Tajbakhsh, M. (2010). One-step, synthesis of Hantzsch esters and polyhydroquinoline derivatives using new organocatalyst. Chin. Chem. Lett. 21, 563–567. doi:10.1016/j.cclet.2009.12.011
Baharfar, R., and Azimi, R. (2014). Immobilization of 1, 4-diazabicyclo [2.2. 2] Octane (DABCO) over mesoporous silica SBA-15: an efficient approach for the synthesis of functionalized spirochromenes. Synth. Commun. 44, 89–100. doi:10.1080/00397911.2013.789527
Barzkar, A., and Beni, A. S. (2020). In situ synthesis of SO 3 H supported Fe 3 O 4@ resorcinol–formaldehyde resin core/shell and its catalytic evaluation towards the synthesis of hexahydroquinoline derivatives in green conditions. RSC Adv. 10, 41703–41712. doi:10.1039/d0ra06972h
Barzkar, A., and Beni, A. S. (2023). Fe3O4@ C@ MCM41-guanidine core–shell nanostructures as a powerful and recyclable nanocatalyst with high performance for synthesis of Knoevenagel reaction. Sci. Rep. 13, 10336. doi:10.1038/s41598-023-36352-5
Chen, S., Abdel-Mageed, A. M., Hauble, A., Ishida, T., Murayama, T., Parlinska-Wojtan, M., et al. (2021). Performance of Au/ZnO catalysts in CO2 reduction to methanol: varying the Au loading/Au particle size. Appl. Catal. A General 624, 118318. doi:10.1016/j.apcata.2021.118318
Chen, Y., Zhang, Z., Jiang, W., Zhang, M., and Li, Y. (2019). Ru III@ CMC/Fe 3 O 4 hybrid: an efficient, magnetic, retrievable, self-organized nanocatalyst for green synthesis of pyranopyrazole and polyhydroquinoline derivatives. Mol. Divers. 23, 421–442. doi:10.1007/s11030-018-9887-3
Cherkupally, S. R., and Mekala, R. (2008). P-TSA catalyzed facile and efficient synthesis of polyhydroquinoline derivatives through Hantzsch multi-component condensation. Chem. Pharm. Bull. 56, 1002–1004. doi:10.1248/cpb.56.1002
Dehghani, P., Elhamifar, D., and Kargar, S. (2025). Amine functionalized magnetic resorcinol formaldehyde as a green and reusable nanocatalyst for the Knoevenagel condensation. Sci. Rep. 15, 2873. doi:10.1038/s41598-025-85921-3
Dhengale, S. D., Naik, V. M., Kolekar, G. B., Rode, C. V., and Anbhule, P. V. (2021). Solvent free, environment benign synthesis of 1, 4-dihydropyridines and polyhydroquinolines by using heterogeneous Zn/MCM-41 catalyst. Res. Chem. Intermed. 47, 3263–3287. doi:10.1007/s11164-021-04473-z
Donelson, J. L., Gibbs, R. A., and De, S. K. (2006). An efficient one-pot synthesis of polyhydroquinoline derivatives through the Hantzsch four component condensation. J. Mol. Catal. A Chem. 256, 309–311. doi:10.1016/j.molcata.2006.03.079
Elhamifar, D., and Ardeshirfard, H. (2017). Phenyl and ionic liquid based bifunctional periodic mesoporous organosilica supported copper: an efficient nanocatalyst for clean production of polyhydroquinolines. J. colloid interface Sci. 505, 1177–1184. doi:10.1016/j.jcis.2017.07.010
Elhamifar, D., Badin, P., and Karimipoor, G. (2017). Alkyl-imidazolium based organosilica supported Fe/porphyrin complex: as novel, highly efficient and reusable catalyst for the unsymmetrical Hantzsch reaction. J. colloid interface Sci. 499, 120–127. doi:10.1016/j.jcis.2017.03.084
Ghorbani-Choghamarani, A., Mohammadi, M., Tamoradi, T., and Ghadermazi, M. (2019). Covalent immobilization of Co complex on the surface of SBA-15: green, novel and efficient catalyst for the oxidation of sulfides and synthesis of polyhydroquinoline derivatives in green condition. Polyhedron 158, 25–35. doi:10.1016/j.poly.2018.10.054
Ghorbani-Choghamarani, A., Tahmasbi, B., Moradi, P., and Havasi, N. (2016). Cu–S-(propyl)-2-aminobenzothioate on magnetic nanoparticles: highly efficient and reusable catalyst for synthesis of polyhydroquinoline derivatives and oxidation of sulfides. Appl. Organomet. Chem. 30, 619–625. doi:10.1002/aoc.3478
Gong, C., Zhou, Z., Li, J., Zhou, H., and Liu, R. (2018). Facile synthesis of ultra stable Fe3O4@ Carbon core-shell nanoparticles entrapped satellite au catalysts with enhanced 4-nitrophenol reduction property. J. Taiwan Inst. Chem. Eng. 84, 229–235. doi:10.1016/j.jtice.2018.01.026
Gupta, P., Rani, S., Sah, D., Shabir, J., Singh, B., Pani, B., et al. (2023). Basic ionic liquid grafted on magnetic nanoparticles: an efficient and highly active recyclable catalyst for the synthesis of β-nitroalcohols and 4H-benzo [b] pyrans. J. Mol. Struct. 1274, 134351. doi:10.1016/j.molstruc.2022.134351
Hajipour, A. R., and Abolfathi, P. (2017). Silica-supported ni (ii)–dabco complex: an efficient and reusable catalyst for the heck reaction. Catal. Lett. 147, 188–195. doi:10.1007/s10562-016-1880-9
Hamidinasab, M., Bodaghifard, M. A., and Mobinikhaledi, A. (2020). Green synthesis of 1H-pyrazolo [1, 2-b] phthalazine-2-carbonitrile derivatives using a new bifunctional base–ionic liquid hybrid magnetic nanocatalyst. Appl. Organomet. Chem. 34, e5386. doi:10.1002/aoc.5386
Hasaninejad, A., Shekouhy, M., Golzar, N., Zare, A., and Doroodmand, M. M. (2011). Silica bonded n-propyl-4-aza-1-azoniabicyclo [2.2. 2] octane chloride (SB-DABCO): a highly efficient, reusable and new heterogeneous catalyst for the synthesis of 4H-benzo [b] pyran derivatives. Appl. Catal. A General 402, 11–22. doi:10.1016/j.apcata.2011.04.012
Hashkavayi, A. B., and Raoof, J. B. (2017). Design an aptasensor based on structure-switching aptamer on dendritic gold nanostructures/Fe3O4@ SiO2/DABCO modified screen printed electrode for highly selective detection of epirubicin. Biosens. Bioelectron. 91, 650–657. doi:10.1016/j.bios.2017.01.025
Honari, M., Kiasat, A. R., Sanaeishoar, H., and Mohammadi, M. K. (2022). Fe3O4@ nSiO2@ mSiO2/DBU: a novel and effective basic magnetic nanocatalyst in the multicomponent one pot synthesis of polyhydroacridines and polyhydroquinolines. Polycycl. Aromat. Compd. 42, 1728–1746. doi:10.1080/10406638.2020.1804412
Jadhav, S., Patil, S., Kumbhar, A., Kamble, S., and Salunkhe, R. (2019). Mizoroki–Heck cross-coupling reactions using palladium immobilized on DABCO-functionalized silica. Transit. Metal. Chem. 44, 507–514. doi:10.1007/s11243-019-00308-4
Jadidi Nejad, M., Pazoki, F., Bagheri, S., Yazdani, E., and Heydari, A. (2020). 1, 4-Diazabicyclo [2.2. 2] octane-sulfonic acid immobilized on magnetic Fe 3 O 4@ SiO 2 nanoparticles: a novel and recyclable catalyst for the one-pot synthesis of 4-aryl-NH-1, 2, 3-triazoles. J. Chem. Sci. 132, 62–10. doi:10.1007/s12039-020-01761-w
Jia, X., Zhang, X., Wang, Z., and Zhao, S. (2022). Tertiary amine ionic liquid incorporated Fe3O4 nanoparticles as a versatile catalyst for the Knoevenagel reaction. Synth. Commun. 52, 774–786. doi:10.1080/00397911.2022.2053992
Kardan, M., Gholinejad, M., Saadati, F., Nayeri, S., Mirmohammad, S. S., and Sansano, J. M. (2022). DABCO-based ionic liquid-modified magnetic nanoparticles supported gold as an efficient catalyst for A3 coupling reaction in water. J. Iran. Chem. Soc. 19, 3417–3430. doi:10.1007/s13738-022-02534-7
Kardooni, R., Kiasat, A. R., and Motamedi, H. (2017). Designing of a novel dual-function silica-iron oxide hybrid based nanocomposite, Fe3O4@ SiO2PEG/NH2, and its application as an eco-catalyst for the solvent-free synthesis of polyhydroacridines and polyhydroquinolines. J. Taiwan Inst. Chem. Eng. 81, 373–382. doi:10.1016/j.jtice.2017.10.013
Kargar, P. G., Bagherzade, G., and Eshghi, H. (2024). Retraction: novel biocompatible core/shell Fe 3 O 4@ NFC@ Co (ii) as a new catalyst in a multicomponent reaction: an efficient and sustainable methodology and novel reusable material for one-pot synthesis of 4 H-pyran and pyranopyrazole in aqueous media. RSC Adv. 14, 1673. doi:10.1039/d3ra90127k
Kazemi, M., and Mohammadi, M. (2020). Magnetically recoverable catalysts: catalysis in synthesis of polyhydroquinolines. Appl. Organomet. Chem. 34, e5400. doi:10.1002/aoc.5400
Khazaei, A., Moosavi-Zare, A. R., Afshar-Hezarkhani, H., and Khakyzadeh, V. (2014). Nano-ferrous ferric oxide (nano-Fe 3 O 4): magnetite catalytic system for the one-pot four-component tandem imine/enamine formation-Knoevenagel–Michael-cyclocondensation reaction of dimedone, aldehydes, β-ketoesters and ammonium acetate under green media. RSC Adv. 4, 32142–32147. doi:10.1039/c4ra03980g
Kilic, A., Gezer, E., Durap, F., Aydemir, M., and Baysal, A. (2019). Pd (II) supported dioxime functionalized Fe3O4 nanoparticles as efficient, eco-friendly and reusable catalysts for the Suzuki-Miyaura cross-coupling reaction in water. J. Organomet. Chem. 896, 129–138. doi:10.1016/j.jorganchem.2019.06.007
Kilic, A., Karatas, M. E., Beyazsakal, L., and Okumus, V. (2022). Preparation and spectral studies of boronate ester modified magnetite iron nanoparticles (Fe3O4@ APTES-B) as a new type of biological agents. J. Mol. Liq. 361, 119602. doi:10.1016/j.molliq.2022.119602
Ko, S., and Yao, C.-F. (2006). Ceric ammonium nitrate (CAN) catalyzes the one-pot synthesis of polyhydroquinoline via the Hantzsch reaction. Tetrahedron 62, 7293–7299. doi:10.1016/j.tet.2006.05.037
Kumar, S., Sharma, P., Kapoor, K. K., and Hundal, M. S. (2008). An efficient, catalyst-and solvent-free, four-component, and one-pot synthesis of polyhydroquinolines on grinding. Tetrahedron 64, 536–542. doi:10.1016/j.tet.2007.11.008
Lu, C. S., Lu, J. H., Ma, L., Zhang, Q. F., and Li, X. N. (2012). Effect of solvent polarity properties on the selectivity and activity for 3, 4-dichloronitrobenzene hydrogenation over Pd/C catalyst. Adv. Mater. Res. 396, 2379–2383. doi:10.4028/www.scientific.net/amr.396-398.2379
Maleki, B., Alinezhad, H., Atharifar, H., Tayebee, R., and Mofrad, A. V. (2019). One-pot synthesis of polyhydroquinolines catalyzed by ZnCl2 supported on nano Fe3O4@ SiO2. Org. Prep. Proced. Int. 51, 301–309. doi:10.1080/00304948.2019.1600132
Mirbagheri, R., Elhamifar, D., and Shaker, M. (2021). Yolk–shell structured magnetic mesoporous silica: a novel and highly efficient adsorbent for removal of methylene blue. Sci. Rep. 11, 23259. doi:10.1038/s41598-021-02699-w
Moheiseni, F., Reza Kiasat, A., and Badri, R. (2021). Synthesis, characterization and application of β-cyclodextrin/imidazolium based dicationic ionic liquid supported on silica Gel as a novel catalyst in Hantzsch condensation reaction. Polycycl. Aromat. Compd. 41, 1094–1106. doi:10.1080/10406638.2019.1650784
Nasseri, M. A., and Sadeghzadeh, S. M. (2014). Diazabicyclo [2.2. 2] octane stabilized on Fe 3 O 4 as catalysts for synthesis of coumarin under solvent-free conditions. J. Iran. Chem. Soc. 11, 27–33. doi:10.1007/s13738-013-0270-0
Nikoorazm, M., and Erfani, Z. (2019). Core–shell nanostructure (Fe3O4@ MCM-41@ Cu-P2C) as a highly efficient and recoverable nanocatalyst for the synthesis of polyhydroquinoline, 5-substituted 1H-tetrazoles and sulfides. Chem. Phys. Lett. 737, 136784. doi:10.1016/j.cplett.2019.136784
Norouzi, M., and Beiranvand, S. (2023). Fe3O4@ SiO2@ BHA-Cu (II) as a new, effective, and magnetically recoverable catalyst for the synthesis of polyhydroquinoline and tetrazole derivatives. J. Chem. Sci. 135, 86. doi:10.1007/s12039-023-02206-w
Platero, F., Caballero, A., and Colón, G. (2022). Tuning the co-catalyst loading for the optimization of thermo-photocatalytic hydrogen production over Cu/TiO2. Appl. Catal. A General 643, 118804. doi:10.1016/j.apcata.2022.118804
Pol, V. G., Shrestha, L. K., and Ariga, K. (2014). Tunable, functional carbon spheres derived from rapid synthesis of resorcinol-formaldehyde resins. ACS Appl. Mater. and interfaces 6, 10649–10655. doi:10.1021/am502324m
Rajabi-Salek, M., Zolfigol, M. A., and Zarei, M. (2018). Synthesis of a novel DABCO-based nanomagnetic catalyst with sulfonic acid tags: application to the synthesis of diverse spiropyrans. Res. Chem. Intermed. 44, 5255–5269. doi:10.1007/s11164-018-3421-1
Saberi, F., Ostovar, S., Behazin, R., Rezvani, A., Ebrahimi, A., and Shaterian, H. R. (2020). Insight into 6-aminopenicillanic acid structure and study of the quantum mechanical calculations of the acid–base site on γ-Fe 2 O 3@ SiO 2 core–shell nanocomposites and as efficient catalysts in multicomponent reactions. New J. Chem. 44, 20688–20696. doi:10.1039/d0nj02942d
Saha, M., and Pal, A. K. (2011). Palladium (0) nanoparticles: an efficient catalyst for the one-pot synthesis of polyhydroquinolines. Tetrahedron Lett. 52, 4872–4877. doi:10.1016/j.tetlet.2011.07.031
Sajjadifar, S., and Azmoudehfard, Z. (2019). Application of [Fe3O4@ SiO2@(CH2) 3Py] HSO4− as heterogeneous and recyclable nanocatalyst for synthesis of polyhydroquinoline derivatives. Appl. Organomet. Chem. 33, e5101. doi:10.1002/aoc.5101
Sapkal, S. B., Shelke, K. F., Shingate, B. B., and Shingare, M. S. (2009). Nickel nanoparticle-catalyzed facile and efficient one-pot synthesis of polyhydroquinoline derivatives via Hantzsch condensation under solvent-free conditions. Tetrahedron Lett. 50, 1754–1756. doi:10.1016/j.tetlet.2009.01.140
Serenjeh, F. N., Hashemi, P., and Rasoolzadeh, F. (2015). A simple method for the preparation of spherical core–shell nanomagnetic agarose particles. Colloids Surfaces A Physicochem. Eng. Aspects 465, 47–53. doi:10.1016/j.colsurfa.2014.10.003
Shabir, J., Gupta, P., Sah, D., and Mozumdar, S. (2020). Magnetic core–shell dendritic mesoporous silica nanospheres anchored with diamine as an efficient and recyclable base catalyst. New J. Chem. 44, 21152–21166. doi:10.1039/d0nj04822d
Shaker, M., and Beni, A. S. (2021). Cu@ SB-MCM-41 composite as an efficient and recyclable nanocatalyst for the synthesis of polyhydroquinoline derivatives via unsymmetrical Hantzsch reaction. J. Porous Mater. 28, 435–449. doi:10.1007/s10934-020-01006-8
Shaker, M., and Elhamifar, D. (2020). Sulfonic acid supported on magnetic methylene-based organosilica as an efficient and recyclable nanocatalyst for biodiesel production via esterification. Front. Energy Res. 8, 78. doi:10.3389/fenrg.2020.00078
Shaker, M., and Elhamifar, D. (2021a). Cu-containing magnetic yolk-shell structured ionic liquid-based organosilica nanocomposite: a powerful catalyst with improved activity. Compos. Commun. 24, 100608. doi:10.1016/j.coco.2020.100608
Shaker, M., and Elhamifar, D. (2021b). Magnetic Ti-containing phenylene-based mesoporous organosilica: a powerful nanocatalyst with high recoverability. Colloids Surfaces A Physicochem. Eng. Aspects 608, 125603. doi:10.1016/j.colsurfa.2020.125603
Sharma, S., Singh, U. P., and Singh, A. (2021). Synthesis of MCM-41 supported cobalt (II) complex for the formation of polyhydroquinoline derivatives. Polyhedron 199, 115102. doi:10.1016/j.poly.2021.115102
Shi, C., Lin, C., Huang, X., Wu, Q., Ge, H., and Yang, Y. (2024). Facile synthesis of magnetic resorcinol–formaldehyde resin Fe3O4@ RF-Au composites for enhanced tetracycline photodegradation with simultaneous H2O2 production. J. Mater. Sci. Mater. Electron. 35, 1070. doi:10.1007/s10854-024-12841-9
Shinde, G., and Thakur, J. (2023). Core-shell structured Fe3O4@ MgO: magnetically recyclable nanocatalyst for one-pot synthesis of polyhydroquinoline derivatives under solvent-free conditions. J. Chem. Sci. 135, 14. doi:10.1007/s12039-023-02134-9
Singh, H., Garg, N., Arora, P., Rajput, J. K., and Jigyasa, (2018). Sucrose chelated auto combustion synthesis of BiFeO3 nanoparticles: magnetically recoverable catalyst for the one-pot synthesis of polyhydroquinoline. Appl. Organomet. Chem. 32, e4357. doi:10.1002/aoc.4357
Sobhani, S., and Pakdin-Parizi, Z. (2014). Palladium-DABCO complex supported on γ-Fe2O3 magnetic nanoparticles: a new catalyst for CC bond formation via Mizoroki– Heck cross-coupling reaction. Appl. Catal. A General 479, 112–120. doi:10.1016/j.apcata.2014.04.028
Surasani, R., Kalita, D., Rao, A. D., Yarbagi, K., and Chandrasekhar, K. (2012). FeF3 as a novel catalyst for the synthesis of polyhydroquinoline derivatives via unsymmetrical Hantzsch reaction. J. Fluor. Chem. 135, 91–96. doi:10.1016/j.jfluchem.2011.09.005
Tafti, A. D., Mirjalili, B. B. F., Salehi, N., and Bamoniri, A. (2024). Facile and efficient method for the synthesis of tetrahydrobenzo [b] pyrans and spirooxindoles catalysed by nano-Fe3O4@ dextrin/Si (CH2) 3/DABCO as a natural-based nanocatalyst in green media. Iran. J. Catal. 14. doi:10.57647/j.ijc.2024.1401.07
Tamoradi, T., Ghadermazi, M., and Ghorbani-Choghamarani, A. (2018). Ni (II)-Adenine complex coated Fe3O4 nanoparticles as high reusable nanocatalyst for the synthesis of polyhydroquinoline derivatives and oxidation reactions. Appl. Organomet. Chem. 32, e3974. doi:10.1002/aoc.3974
Varghese, J. J., and Mushrif, S. H. (2019). Origins of complex solvent effects on chemical reactivity and computational tools to investigate them: a review. React. Chem. and Eng. 4, 165–206. doi:10.1039/c8re00226f
Wang, L.-M., Sheng, J., Zhang, L., Han, J.-W., Fan, Z.-Y., Tian, H., et al. (2005). Facile Yb (OTf) 3 promoted one-pot synthesis of polyhydroquinoline derivatives through Hantzsch reaction. Tetrahedron 61, 1539–1543. doi:10.1016/j.tet.2004.11.079
Wang, M., Ni, Y., and Liu, A. (2017). Fe3O4@ resorcinol–formaldehyde resin/Cu2O composite microstructures: solution-phase construction, magnetic performance, and applications in antibacterial and catalytic fields. ACS omega 2, 1505–1512. doi:10.1021/acsomega.7b00064
Yarie, M., Zolfigol, M. A., Bayat, Y., Asgari, A., Alonso, D. A., and Khoshnood, A. (2016). Novel magnetic nanoparticles with ionic liquid tags as a reusable catalyst in the synthesis of polyhydroquinolines. RSC Adv. 6, 82842–82853. doi:10.1039/c6ra16459e
Yazdani, M., Elhamifar, D., and Shaker, M. (2025). Synthesis of 3, 4-dihydropyrimidinone derivatives by using mag@ TOS as a strong and magnetically recoverable nanocatalyst. J. Inorg. Organomet. Polym. Mater., 1–16. doi:10.1007/s10904-025-03617-7
Yu, L., Pan, P., Zhang, Y., Zhang, Y., Wan, L., Cheng, X., et al. (2019). Nonsacrificial self-template synthesis of colloidal magnetic yolk–shell mesoporous organosilicas for efficient oil/water interface catalysis. Small 15, 1805465. doi:10.1002/smll.201805465
Zare, A., Lotfifar, N., and Dianat, M. (2020). Preparation, characterization and application of nano-[Fe3O4@-SiO2@ R-NHMe2] [H2PO4] as a novel magnetically recoverable catalyst for the synthesis of pyrimido [4, 5-b] quinolines. J. Mol. Struct. 1211, 128030. doi:10.1016/j.molstruc.2020.128030
Zhang, X., He, X., and Zhao, S. (2021). Preparation of a novel Fe3O4@ SiO2@ propyl@ DBU magnetic core–shell nanocatalyst for Knoevenagel reaction in aqueous medium. Green Chem. Lett. Rev. 14, 85–98. doi:10.1080/17518253.2020.1862312
Zheng, D., Zhang, M., Ding, L., Zhang, Y., Zheng, J., and Xu, J. (2016). Facile synthesis of magnetic resorcinol–formaldehyde (RF) coated carbon nanotubes for methylene blue removal. RSC Adv. 6, 11973–11979. doi:10.1039/c5ra25738g
Keywords: quaternary ammonium salt, magnetic properties, 1,4-diazabicyclo [2.2.2] octane (DABCO), heterogeneous nanocatalyst, hantzsch reaction, resorcinol-formaldehyde
Citation: Esfandiari M and Salimi Beni A (2025) DABCO-modified magnetic core-shell as an efficient nanocatalyst for synthesizing polyhydroquinoline derivatives. Front. Chem. 13:1557628. doi: 10.3389/fchem.2025.1557628
Received: 08 January 2025; Accepted: 17 March 2025;
Published: 31 March 2025.
Edited by:
Ahmet Kilic, Harran University, TürkiyeReviewed by:
Ivaylo Tankov, Assen Zlatarov University, BulgariaCopyright © 2025 Esfandiari and Salimi Beni. This is an open-access article distributed under the terms of the Creative Commons Attribution License (CC BY). The use, distribution or reproduction in other forums is permitted, provided the original author(s) and the copyright owner(s) are credited and that the original publication in this journal is cited, in accordance with accepted academic practice. No use, distribution or reproduction is permitted which does not comply with these terms.
*Correspondence: Alireza Salimi Beni, YWxpcmV6YXNhbGltaTcxNzMyOTFAZ21haWwuY29t, c2FsaW1pYmVuaUB5dS5hYy5pcg==
Disclaimer: All claims expressed in this article are solely those of the authors and do not necessarily represent those of their affiliated organizations, or those of the publisher, the editors and the reviewers. Any product that may be evaluated in this article or claim that may be made by its manufacturer is not guaranteed or endorsed by the publisher.
Research integrity at Frontiers
Learn more about the work of our research integrity team to safeguard the quality of each article we publish.