- 1School for the Engineering of Matter, Transport, and Energy, Center for Biological Physics, Arizona State University, Tempe, AZ, United States
- 2BASF Corporation, Raleigh, NC, United States
- 3BASF SE, Ludwigshafen, Germany
- 4Molecular Foundry, Lawrence Berkeley National Laboratory, Berkeley, CA, United States
Calcium carbonate (CaCO3) is an incredibly abundant mineral on Earth, with over 90% of it being found in the lithosphere. To address the CO2 crisis and combat ocean acidification, it is essential to produce more CaCO3 using various synthetic methods. Additionally, this approach can serve as a substitute for energy-intensive processes like cement production. By doing so, we have the potential to not only reverse the damage caused by climate change but also protect biological ecosystems and the overall environment. The key lies in maximizing the utilization of CaCO3 in various human activities, paving the way for a more sustainable future for our planet.
Introduction
The significance of inorganic materials in the chemical industry cannot be overstated. These materials, derived from non-carbon-based compounds, play a foundational role in various processes, applications, and innovations (Gągol et al., 2020; Industrial inorganic chemistry, 2010; Wang and Wang, 2021; Wang et al., 2022; Waris et al., 2021) (Figure 1). As we navigate an era emphasizing sustainability, the importance of inorganic materials in fostering sustainable chemistry becomes increasingly apparent (Huang and Zhai, 2021; Lima et al., 2020; Van Soest et al., 2021). Inorganic materials serve as essential building blocks for countless chemical products, ranging from catalysts and reagents to structural components (Clark and Rhodes, 2000; Furukawa and Komatsu, 2017; Mitzi, 2009; Osterloh, 2008; Schubert and Hüsing, 2019; Song and Lee, 2002; Zheng et al., 2022). Their versatility extends into diverse sectors such as electronics, pharmaceuticals, energy, technology and materials science (Al Zoubi and Ko, 2020; Avouris and Martel, 2010; Boles et al., 2016; Chen and Park, 2018; Chen et al., 2015; Ebadi Jamkhaneh et al., 2021; Fadia et al., 2021; Fan et al., 2021; Moon et al., 2007; Niemeyer, 2001; Qi et al., 2020; Servin and White, 2016; Sun and Rogers, 2007; Vallet-Regí et al., 2007).
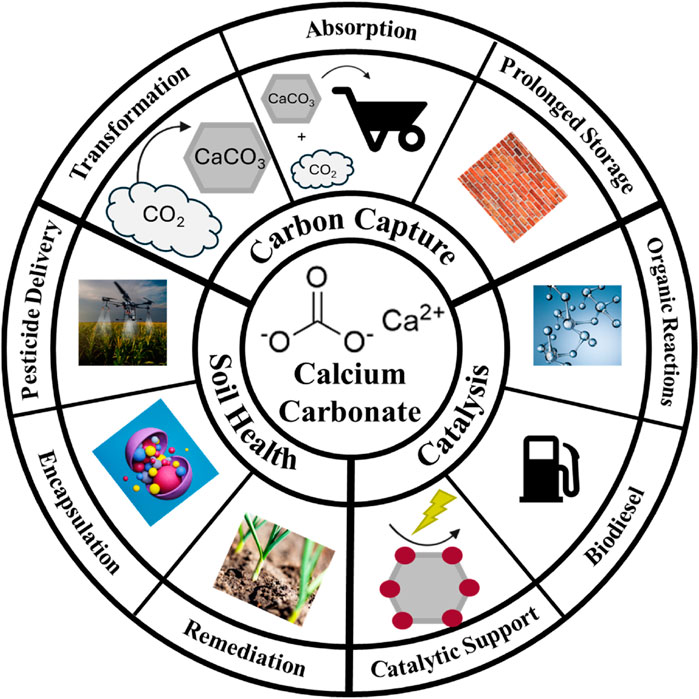
Figure 1. Schematic representation of current uses of calcium carbonate discussed in this mini review.
Crucially, in the context of sustainable chemistry, inorganic materials contribute to environmentally conscious practices (Caballero-Calero et al., 2021; Mazari et al., 2021; Nelson and Schelter, 2019; Pham et al., 2020). Their role in catalysts (Boles et al., 2016; K.-G; Liu et al., 2021; Shen et al., 2020) and processes designed for cleaner and more energy-efficient production and applications (Kitchen et al., 2014; Zheng et al., 2022) exemplifies their significance. Additionally, their use in energy storage (Cheng et al., 2021; Junaid et al., 2021; Liu et al., 2020; Piątek et al., 2021), renewable energy technologies (Chandrasekaran et al., 2011; Liang et al., 2017), and waste treatment (Goh and Ismail, 2018; Kayvani Fard et al., 2018; Manikandan et al., 2022; Xiang et al., 2022) underscores their pivotal role in addressing global sustainability challenges.
Calcium carbonate serves as a versatile reagent in inorganic chemistry, contributing to various reactions and processes (Al-Hosney and Grassian, 2004; Baltrusaitis et al., 2007; Lin et al., 2020; Salek et al., 2015; Suppes et al., 2001). Moreover, its unique properties such as its porous structure and high surface area (Durand et al., 2018), make it a suitable substrate for anchoring catalytically active components (García-Mota et al., 2011; Liu et al., 2013; Saetan et al., 2017; Schlägl et al., 1987). Calcium carbonate plays a role in the production of biodiesel, catalyzing transesterification of natural oils (Alonso et al., 2010; Chutia and Phukan, 2024; Kouzu et al., 2008; Ling et al., 2019; X; Liu et al., 2021; Ngamcharussrivichai et al., 2010; Suppes et al., 2001; Thangaraj et al., 2019). Calcium carbonate is employed in carbon capture applications as a sorbent for CO2 removal (Dou et al., 2016; Erans et al., 2016; Florin et al., 2010; Liu et al., 2010; Witoon, 2011). In a process called mineral carbonation, it reacts with carbon dioxide to form stable carbonates, contributing to carbon capture and storage efforts (Abanades, 2002; Bewernitz et al., 2024; Erans et al., 2020; Gadikota, 2021; Gambhir and Tavoni, 2019; Levey et al., 2024; Olajire, 2013; Sanna et al., 2014; Sanz-Pérez et al., 2016). Like in biological materials, various mineral phases of CaCO3 can be processed to obtain customized chemical reactivity and functionality (Briegel and Seto, 2012; Cho et al., 2019; Seto et al., 2014; 2013). This method aids in mitigating greenhouse gas emissions and addresses climate change concerns (Neeraj and Yadav, 2020; Snæbjörnsdóttir et al., 2020; Thonemann et al., 2022). Understanding these diverse properties unveils calcium carbonate’s significance in addressing environmental concerns.
This material emerges as a linchpin for fostering robust soil health, vibrant plant growth, and bountiful crop yields (Hamdan et al., 2017; Soon et al., 2014; Wang et al., 2015; Xie et al., 2024). From soil pH adjustment in acidic terrains (Neina, 2019) to serving as a vital calcium supplement for plants (Shabtai et al., 2023), calcium carbonate’s agricultural significance is underscored by its ability to rectify deficiencies that may impede the optimal development of crops. Furthermore, its impact extends beneath the surface, where it actively participates in enhancing soil structure (Dou et al., 2023). By promoting aggregation, calcium carbonate facilitates improved water retention and drainage, creating an environment conducive to the flourishing of roots (Figure 1).
As an additive in fertilizers, it takes on the role of a nourishing component, supplying essential calcium that supports the formation of robust cell walls and overall plant structure (Abo-Sedera, 2016; Hua et al., 2015). Acting as a buffering agent, calcium carbonate becomes a guardian of soil pH stability, curbing rapid fluctuations that could detrimentally affect plant health (McFarland et al., 2020; Zhang et al., 2016). Beyond the crop fields, its practical application even extends to dust control in agricultural settings, where it contributes to creating a more comfortable environment, particularly in livestock farming (Hamdan and Kavazanjian, 2016; Meyer et al., 2011; Song et al., 2020). In essence, the diverse applications of calcium carbonate in agriculture stand as a testament to its integral role in promoting soil fertility, sustaining healthy plant growth, and ultimately cultivating agricultural landscapes that thrive (Figure 1).
CaCO3 in carbon capture and mineralization applications
Carbon capture, utilization, and storage (CCUS) technologies aim to decrease the greenhouse gas effect by capturing emitted carbon and transforming it for long term storage or chemical utility (Chang et al., 2017). Industrial mineralization of carbon dioxide to produce calcium carbonate is a promising CCUS method with high economic potential (Chang et al., 2017; Teir et al., 2016). These reaction pathways valorize waste streams from processes such as steelmaking and cement production while reducing energy consumption (Jin et al., 2022; Katsuyama et al., 2005; Marin Rivera and Van Gerven, 2020; Teir et al., 2016). By utilizing chemicals in waste flue gas, steelmaking slag and cement powder, calcium carbonate production provides a green alternative to disposal and storage of carbon dioxide (Czaplicka and Konopacka-Łyskawa, 2020; Teir et al., 2016) (Figure 2). There are many methods to precipitate calcium carbonate from CO2 streams such as microbially induced precipitation, ultrasonication of supercritical carbon dioxide, and other methods, most of which are based on biomimetics or CO2 bubbling (Boyjoo et al., 2014; Chuo et al., 2020; López-Periago et al., 2010). When using sorbent technology, a compromise must be made between sorbent performance and the increasing cost (Erans et al., 2016). Modified materials and advanced chemical reactors increase sorbent utility, but can be vastly more expensive than their simpler counterparts. Also, the activity of sorbents decay over time due to sintering and attrition, further limiting utility (Erans et al., 2016).
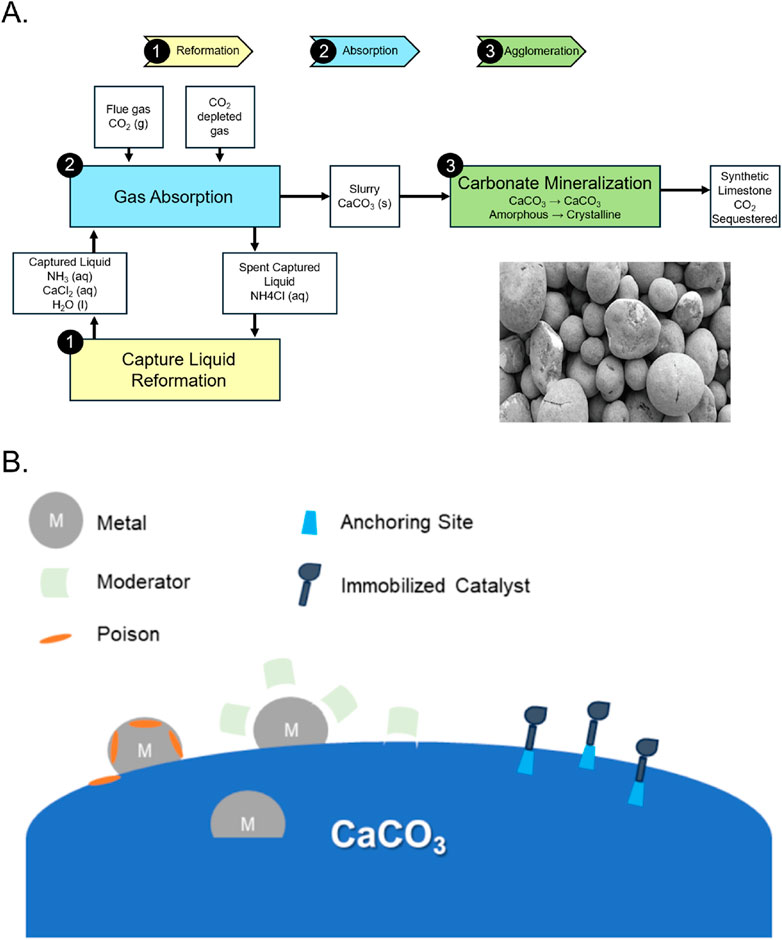
Figure 2. Diverse methods of utilizing CaCO3 at various length-scales. (A) By utilizing a biogeomimetic mineralization route, recycled geomass can be harvested to make tonnes of aggregates. [inset: CaCO3 aggregates formed] (images courtesy of Blue Planet Systems, Inc). (B) CaCO3 can serve as catalysts with specific functional groups to enhance the formation of chemical feedstocks. (conceptual graph inspired by, among others, Lindlar and Dubuis, 2003; Senra et al., 2008; Lizandara-Pueyo et al., 2021).
In recent years, chemical looping and mineralization has been gaining attention as a promising CCSU technology, with publications such as those from Jin, Z. et al. and Bewernitz, M.A. et al. (Table 1). CaCO3 aggregates can be used to replace cementitious products (Hargis et al., 2021; Pu et al., 2021). Through an exponential increase in the built environment, cement production has become an ever-increasing source of CO2 and dust pollution. It approximately accounts for 8% of the anthropogenic CO2 produced per year and is a process with very little technical improvement since its utilization from Roman times (Stefaniuk et al., 2023). Only through recent advances like replacements with clinker as well as new formulations with lower energy substitutes (Martinez et al., 2023) as well as aggregates (Hanifa et al., 2023). can we envision a world with more infrastructure, but without the pollution attached to building it (Figure 2).
It is indisputable that the indiscriminate emissions of greenhouse gas have resulted in increased surface temperature on Earth and environmental degradation (Yoro and Daramola, 2020). Given the tremendous amount of CO2 in Earth’s atmosphere, CCUS technology would have to make gigaton-scale changes to have a meaningful impact on the global scale (Figure 3). Through reduction of emissions and increasing global CCUS usage, humanity has been trying to reduce the impact of climate change caused by anthropogenic CO2 emissions (Dey and Dhal, 2019; Huisingh et al., 2015; Sanna et al., 2014).
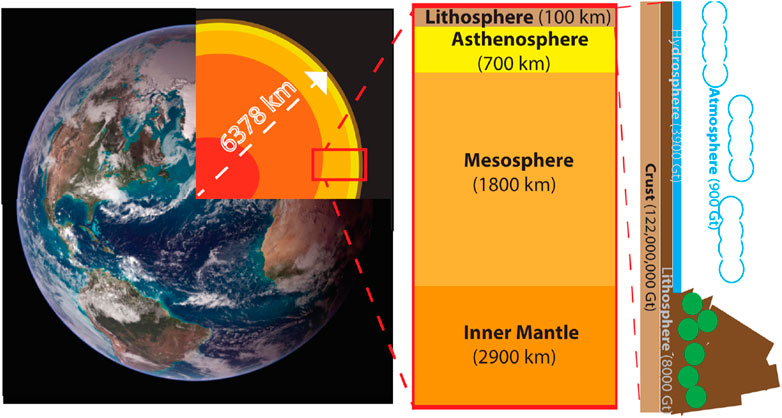
Figure 3. The geological composition of the Earth (A). The various segments from the core to the surface of the planet (B). The diverse composition of the Earth’s mantle (C). The majority of carbon (in the form of carbonates and CO2) stored in the diverse surface layers of Earth (image courtesy of NASA).
The relationship between average surface temperature and CO2 concentration is directly proportional (Humlum et al., 2013). Ice core records indicate that CO2 concentration has varied with temperature over long time scales for the past 420,000 years or even longer (Humlum et al., 2013; Lüthi et al., 2008). Also, it is suggested that rising atmospheric CO2 levels amplify or even precede global temperature changes initiated by Milankovitch cycles (Humlum et al., 2013; Shakun et al., 2012; Toggweiler and Lea, 2010). With these facts in mind, data from the National Center for Environmental Information (NOAA National Centers for Environmental Information, 2024; U.S. Global Change Research Program et al., 2017) was plotted and regression was performed to derive Equation 1, describing the relationship between global average surface temperature (as compared to the 1901–200 average), time, and atmospheric CO2 concentration.
The factor
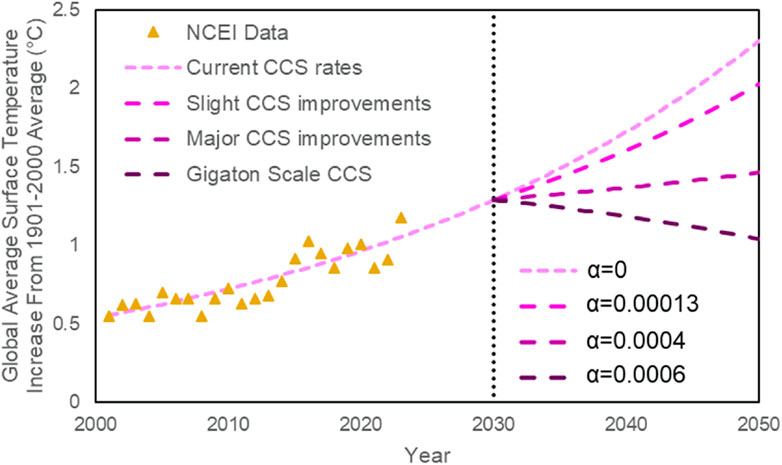
Figure 4. Global average surface temperature increase from 1901 to 2000 average based on data from the National Centers for Environmental Information (triangles) (NOAA National Centers for Environmental information, 2024).
Given Earth’s tremendously large surface area, and high water content, it takes an enormous amount of energy to raise the average surface temperature even by a small amount. Though 1°C may seem like an insignificant change, the global effects are drastic due to the tremendous amount of trapped heat (Lindsey and Dahlman, 2024; NOAA National Centers for Environmental Information, 2024; U.S. Global Change Research Program et al., 2017). If dramatic industrial and legal changes are not made, the disastrous effects may be irreversible (Armstrong McKay et al., 2022; Ridley et al., 2010).
CaCO3 in chemical catalysis
One of the most relevant processes in the chemical industry is the selective partial hydrogenation of alkynes to alkenes; this transformation is usually enabled by the Lindlar Catalyst (Lindlar and Dubuis, 2003). The use of calcium carbonate in the Lindlar Catalyst dates back to the 1980s when authors such as Schlägl, R. et al. released publications detailing its structure and activity (Table 1). This catalyst is based on palladium supported on calcium carbonate and passivated with lead acetate and quinoline. This catalytic system has a broad application in natural product synthesis (Ballesteros-Soberanas et al., 2022) or in the upgrading of vegetable oils (Laverdura et al., 2020). This catalyst allows the reaction to be performed at high temperatures (200°C), increasing conversion rates while simultaneously increasing the cost required to run such a system (Suppes et al., 2001). The versatility and inertness of calcium carbonate as support, allows the fine tuning of the surfaces. Accordingly, an efficient and selective catalytic system using hydroxypropylated cyclodextrins and palladium on calcium carbonate has been presented by Senra et al. (2008) for a ligand-free aqueous Heck reactions. In this case, the catalyst was not only active and selective but also immobilized. Most recently, calcium carbonate has been used as a support for the immobilization of asymmetric catalysts using biomineralization concepts and click chemistry. A calcium carbonate-supported α,α-diarylprolinol silyl ether prepared in this manner catalyzed Michael addition of aldehydes to trans-β-nitrostyrenes with very high diastereo- and enantioselectivity. By utilizing calcium carbonate as a heterogenous support, organocatalysis can be immobilized which reduces the cost and energy requirement of downstream separations (Lizandara-Pueyo et al., 2021). Additionally, this method allows for the used organocatalysis to be recycled and reused (Benaglia, 2009) (Figure 2). The catalyst can be recovered for reuse by simple decantation or used in a continuous flow reactor, increasing productivity five-fold as compared to batch processes (Lizandara-Pueyo et al., 2021).
Recycling catalysts is a sustainable process, but sometimes a certain portion of the catalyst cannot be recovered. A small amount of the catalyst can dissolve into the reaction mixture, and potentially escape into the environment. Calcium is extremely common in the environment as compared to other catalytic chemicals. The miniscule amounts of calcium carbonate which escape into the environment would generally cause no effect because calcium is already present (Suppes et al., 2001).
Remarkably, calcium carbonate is highly recyclable and utilization of recycled calcium carbonate enables a boost in the recycled content found in compounds and final polymer applications, thereby aiding customers in achieving their circular economy objectives. Utilization of pre-consumer and post-consumer recycled calcium carbonate is on rise thus adding another benefit of calcium carbonate as a part of a product (Cunningham et al., 2021).
CaCO3 in agricultural applications
With an increasing global population (Gu et al., 2021), food security is of the utmost importance (Molotoks et al., 2021). Between 2010 and 2050, there is an anticipated increase in global food demand ranging from 35% to 56%. Simultaneously, the population at risk of hunger is projected to undergo a change ranging from a reduction of 91% to an increase of 8% during the same period (Van Dijk et al., 2021). The looming threat of food scarcity heightens humanity’s need to protect and ensure a bountiful supply of healthy crops in the coming years (Anderson et al., 2020; Devaux et al., 2021; Fukase and Martin, 2020; Hasegawa et al., 2021; Zhao et al., 2021). Calcium carbonate offers various solutions to some issues farmers are facing (Liu et al., 2023; Patanè et al., 2018; Santos et al., 2020). By utilizing the unique chemical, physical and material properties of CaCO3, humanity can nourish the agricultural industry in a sustainable way, while potentially avoiding the projected food insecurities.
Soils play a vital role in meeting the food and feed requirements of an expanding global population. The addition of calcium carbonate to soil can have a beneficial effect on plant health. Traditionally, it is commonly used as a liming agent to reduce soil acidity, meaning that calcium carbonate acts as a neutralizing agent for acidic soils by increasing the soil’s pH level, making it more suitable for plant growth. Furthermore, it helps to enhance soil aggregation, water retention, and nutrient availability, making the soil more conducive to plant growth. Interestingly, doping soil with calcium carbonate has alleviated poisoning caused by heavy metal pollution in Hunan, China (Zeng et al., 2015). A field study conducted in Tehsil Lahore City, Punjab, Pakistan further confirmed that concentrations of heavy metals were negatively correlated with calcium carbonate concentrations (Bashir et al., 2019).
Calcium carbonate is often used as a filler in fertilizers to improve their physical properties. It helps to prevent caking and improve the flowability of granular fertilizers, making them easier to handle and apply (Abhiram et al., 2023). Calcium is an essential nutrient for plant growth and development. Calcium carbonate is used as a source of calcium to supplement the soil and provide plants with this vital nutrient. It helps in strengthening the cell walls of plants, improving their overall structure and resilience (Gao et al., 2023).
Plant diseases have a substantial effect on crop yields and quality, leading to considerable economic losses and requiring significant management inputs each year for crops, landscapes, and forests in the United States, amounting to billions of dollars (Fones et al., 2020; Ristaino et al., 2021). Plant sporopollenin has recently emerged as an environmentally friendly drug carrier (Mundargi et al., 2016). The sporopollenin capsule modified with calcium carbonate can be loaded with drugs or pesticides and used for controlled release of pesticide (Xiang et al., 2023). The engineered sporopollenin can then be mixed with soil used to grow plants. This process allows for the slow release of drugs, pesticides and calcium carbonate while remaining environmentally friendly and increasing crop yield.
Microcapsule based controlled release formulations are promising alternatives to conventional pesticide. By preparing double shelled calcium carbonate capsules, many adverse effects caused by traditional pesticide can be avoided. Approximately 90% of the liquid-based pesticide is lost to run-off and evaporation (Zhao et al., 2018). The controlled release of pesticides through microcapsules is a promising method to solve such issues (Zhou et al., 2022). Though environmentally favorable, the preparation of pesticide loaded calcium carbonate microcapsules is expensive, due to the large amount or organic substances required (Zhou et al., 2022). Therefore, developing a cheap and efficient production process is needed.
Starch doped porous calcium carbonate can also be used as a pesticide delivery mechanism. Calcium carbonate microspheres fabricated through coprecipitation regulated by soluble starch can be used as drug carriers for plants (Xiang et al., 2018). Porous calcium carbonate microspheres with intercalated soluble starch molecules were used as carriers for Prometryn, a typical herbicide (Xiang et al., 2018). Due to electrostatic attractions and hydrogen bonding, the herbicide is slowly released, controlling migration of the chemical while increasing its utility (Xiang et al., 2018). This method can potentially be expanded to include the use of other chemical herbicides as well.
For agricultural applications, the approval and regulations regarding calcium carbonate microcapsules depend on the specific application and the country in which it is being used. In general, calcium carbonate itself is commonly used and considered safe for various agricultural applications. However, if calcium carbonate is used as a carrier or encapsulating agent for other substances, such as pesticides or fertilizers, the regulations may vary. Nevertheless, this application will be more explored and it will gain more interest in the future in comparison to more traditional calcium carbonate applications (Table 1).
Regulatory bodies, such as the Environmental Protection Agency (EPA) in the United States or the European Chemicals Agency (ECHA) in the European Union, assess and approve the use of carriers and encapsulating technologies based on their specific applications and potential risks. These bodies evaluate factors such as the effectiveness, safety, and environmental impact of the carried or encapsulated substances. Calcium carbonate is a naturally occurring mineral that is not derived from plastic and does not pose the same environmental concerns as microplastics. Calcium carbonate is biologically and chemically distinct from plastics and is not classified as a microplastic.
Naturally, calcium carbonate exists in the form of limestone, a rock that contains a minimum of 50% calcium carbonate. Limestone deposits can be found worldwide and are extracted through quarrying or mining processes. The United States stands as a prominent producer of calcium carbonate, with minimal risk of supply disruption. Presently, the cost of lime for agricultural applications, like non-irrigated corn farming in South Georgia, stands at $55 per ton, amounting to $13.73 per acre or $0.16 per bushel. Although this may appear relatively high, investing in lime can yield returns within two to 3 years (University of Georgia, 2024). However, one should be careful in applying lime in the field as the pH of the soil is an important factor that can be regarded as a crucial variable because of its impact on various other soil properties and processes that ultimately affect the growth of plants. The activity of microorganisms, as well as the solubility and availability of nutrients, are among the vital processes that rely on soil pH. For example, pH has a significant influence on various plant characteristics or traits, including height, lateral spread, biomass, flower size and quantity, pollen production, and more (Gentili et al., 2018; Jiang et al., 2017).
Conclusion
CaCO3 is a mineral with a diversity of utilization throughout the built environment. With increasing atmospheric CO2 to ocean and soil acidification, methods to mitigate pollution are ever more required for a sustainable environment. Specifically, these activities include CO2 sequestration, chemical catalysis and utilization, precise agricultural applications and essential soil amendments, as well as formation of light weight aggregates in cement replacement applications; all of which will provide noticeable improvements for a more sustainable future. We show an evolution of diverse applications that have been and are using CaCO3 however, with time these applications with CaCO3 will also multiply and its utility will be invaluable in the near future.
Author contributions
JC: Conceptualization, Data curation, Formal Analysis, Funding acquisition, Investigation, Methodology, Project administration, Resources, Software, Supervision, Validation, Visualization, Writing–original draft, Writing–review and editing. EI: Conceptualization, Data curation, Formal Analysis, Funding acquisition, Investigation, Methodology, Project administration, Resources, Software, Supervision, Validation, Visualization, Writing–original draft, Writing–review and editing. CL-P: Conceptualization, Data curation, Formal Analysis, Funding acquisition, Investigation, Methodology, Project administration, Resources, Software, Supervision, Validation, Visualization, Writing–original draft, Writing–review and editing. JS: Conceptualization, Data curation, Formal Analysis, Funding acquisition, Investigation, Methodology, Project administration, Resources, Software, Supervision, Validation, Visualization, Writing–original draft, Writing–review and editing.
Funding
The author(s) declare that no financial support was received for the research, authorship, and/or publication of this article.
Conflict of interest
Author EI was employed by BASF Corporation. Author CL-P was employed by BASF SE.
The remaining authors declare that the research was conducted in the absence of any commercial or financial relationships that could be construed as a potential conflict of interest.
Publisher’s note
All claims expressed in this article are solely those of the authors and do not necessarily represent those of their affiliated organizations, or those of the publisher, the editors and the reviewers. Any product that may be evaluated in this article, or claim that may be made by its manufacturer, is not guaranteed or endorsed by the publisher.
References
Abanades, J. C. (2002). The maximum capture efficiency of CO2 using a carbonation/calcination cycle of CaO/CaCO3. Chem. Eng. J. 90, 303–306. doi:10.1016/S1385-8947(02)00126-2
Abhiram, G., Bishop, P., Jeyakumar, P., Grafton, M., Davies, C. E., and McCurdy, M. (2023). Formulation and characterization of polyester-lignite composite coated slow-release fertilizers. J. Coat. Technol. Res. 20, 307–320. doi:10.1007/s11998-022-00670-6
Abo-Sedera, F. (2016). Effect of organic fertilizer and foliar spray with some safety compounds on growth and productivity of snap bean. Ann. Agric. Sci. Moshtohor 54, 105–118. doi:10.21608/assjm.2016.103931
Ainsworth, E. A., Lemonnier, P., and Wedow, J. M. (2020). The influence of rising tropospheric carbon dioxide and ozone on plant productivity. Plant Biol. 22, 5–11. doi:10.1111/plb.12973
Al-Hosney, H. A., and Grassian, V. H. (2004). Carbonic acid: an important intermediate in the surface chemistry of calcium carbonate. J. Am. Chem. Soc. 126, 8068–8069. doi:10.1021/ja0490774
Alonso, D. M., Vila, F., Mariscal, R., Ojeda, M., Granados, M. L., and Santamaría-González, J. (2010). Relevance of the physicochemical properties of CaO catalysts for the methanolysis of triglycerides to obtain biodiesel. Catal. Today 158, 114–120. doi:10.1016/j.cattod.2010.05.003
Al Zoubi, W., and Ko, Y. G. (2020). Chemical stability of synergistic inorganic materials for enhancing electrochemical performance. Compos. Sci. Technol. 199, 108383. doi:10.1016/j.compscitech.2020.108383
Anderson, R., Bayer, P. E., and Edwards, D. (2020). Climate change and the need for agricultural adaptation. Curr. Opin. Plant Biol. 56, 197–202. doi:10.1016/j.pbi.2019.12.006
Armstrong McKay, D. I., Staal, A., Abrams, J. F., Winkelmann, R., Sakschewski, B., Loriani, S., et al. (2022). Exceeding 1.5°C global warming could trigger multiple climate tipping points. Science 377, eabn7950. doi:10.1126/science.abn7950
Avouris, P., and Martel, R. (2010). Progress in carbon nanotube electronics and photonics. MRS Bull. 35, 306–313. doi:10.1557/mrs2010.553
Ballesteros-Soberanas, J., Hernández-Garrido, J. C., Cerón-Carrasco, J. P., and Leyva-Pérez, A. (2022). Selective semi-hydrogenation of internal alkynes catalyzed by Pd–CaCO3 clusters. J. Catal. 408, 43–55. doi:10.1016/j.jcat.2022.02.020
Baltrusaitis, J., Usher, C. R., and Grassian, V. H. (2007). Reactions of sulfur dioxide on calcium carbonate single crystal and particle surfaces at the adsorbed water carbonate interface. Phys. Chem. Chem. Phys. 9, 3011. doi:10.1039/b617697f
Bashir, M. A., Rehim, A., Liu, J., Imran, M., Liu, H., Suleman, M., et al. (2019). Soil survey techniques determine nutrient status in soil profile and metal retention by calcium carbonate. CATENA 173, 141–149. doi:10.1016/j.catena.2018.10.015
Bewernitz, M. A., Ginder-Vogel, M., Wolf, S. E., Seto, J., and Constantz, B. R. (2024). A bicarbonate-rich liquid condensed phase in non-saturated solutions in the absence of divalent cations. Front. Bioeng. Biotechnol. 12, 1382047. doi:10.3389/fbioe.2024.1382047
Boles, M. A., Engel, M., and Talapin, D. V. (2016). Self-assembly of colloidal nanocrystals: from intricate structures to functional materials. Chem. Rev. 116, 11220–11289. doi:10.1021/acs.chemrev.6b00196
Boyjoo, Y., Pareek, V. K., and Liu, J. (2014). Synthesis of micro and nano-sized calcium carbonate particles and their applications. J. Mater Chem. A 2, 14270–14288. doi:10.1039/C4TA02070G
Briegel, C., and Seto, J. (2012). Single amino acids as additives modulating CaCO3 mineralization. Adv. Top. Biominer., 33–48.
Budinis, S., Krevor, S., Dowell, N. M., Brandon, N., and Hawkes, A. (2018). An assessment of CCS costs, barriers and potential. Energy Strategy Rev. 22, 61–81. doi:10.1016/j.esr.2018.08.003
Caballero-Calero, O., Ares, J. R., and Martín-González, M. (2021). Environmentally friendly thermoelectric materials: high performance from inorganic components with low toxicity and abundance in the Earth. Adv. Sustain. Syst. 5, 2100095. doi:10.1002/adsu.202100095
Chandrasekaran, J., Nithyaprakash, D., Ajjan, K. B., Maruthamuthu, S., Manoharan, D., and Kumar, S. (2011). Hybrid solar cell based on blending of organic and inorganic materials—an overview. Renew. Sustain. Energy Rev. 15, 1228–1238. doi:10.1016/j.rser.2010.09.017
Chang, R., Kim, S., Lee, S., Choi, S., Kim, M., and Park, Y. (2017). Calcium carbonate precipitation for CO2 storage and utilization: a review of the carbonate crystallization and polymorphism. Front. Energy Res. 5, 17. doi:10.3389/fenrg.2017.00017
Chen, J., and Park, N.-G. (2018). Inorganic hole transporting materials for stable and high efficiency perovskite solar cells. J. Phys. Chem. C 122, 14039–14063. doi:10.1021/acs.jpcc.8b01177
Chen, K., Song, S., and Xue, D. (2015). Beyond graphene: materials chemistry toward high performance inorganic functional materials. J. Mater. Chem. A 3, 2441–2453. doi:10.1039/C4TA06989G
Cheng, X., Liu, H., Yuan, H., Peng, H., Tang, C., Huang, J., et al. (2021). A perspective on sustainable energy materials for lithium batteries. SusMat 1, 38–50. doi:10.1002/sus2.4
Cho, K. R., Kulshreshtha, P., Wu, K. J. J., Seto, J., Qiu, S. R., and De Yoreo, J. J. (2019). The effects of different types of additives on growth of biomineral phases investigated by in situ atomic force microscopy. J. Cryst. Growth 509, 8–16. doi:10.1016/j.jcrysgro.2018.12.021
Chuo, S. C., Mohamed, S. F., Mohd Setapar, S. H., Ahmad, A., Jawaid, M., Wani, W. A., et al. (2020). Insights into the current trends in the utilization of bacteria for microbially induced calcium carbonate precipitation. Materials 13, 4993. doi:10.3390/ma13214993
Chutia, G. P., and Phukan, K. (2024). Biomass derived heterogeneous catalysts used for sustainable biodiesel production: a systematic review. Braz. J. Chem. Eng. 41, 23–48. doi:10.1007/s43153-023-00371-6
Clark, J., and Rhodes, C. (2000). “Clean synthesis using porous inorganic solid catalysts and supported reagents,” in RSC Clean technology monographs. Cambridge: Royal Society of Chemistry [distributor] RSC Distribution Services].
Cunningham, P. R., Green, P. G., and Miller, S. A. (2021). Utilization of post-consumer carpet calcium carbonate (PC4) from carpet recycling as a mineral resource in concrete. Resour. Conserv. Recycl. 169, 105496. doi:10.1016/j.resconrec.2021.105496
Czaplicka, N., and Konopacka-Łyskawa, D. (2020). Utilization of gaseous carbon dioxide and industrial Ca-rich waste for calcium carbonate precipitation: a review. Energies 13, 6239. doi:10.3390/en13236239
Davis, W. (2017). The relationship between atmospheric carbon dioxide concentration and global temperature for the last 425 million years. Climate 5, 76. doi:10.3390/cli5040076
Devaux, A., Goffart, J.-P., Kromann, P., Andrade-Piedra, J., Polar, V., and Hareau, G. (2021). The potato of the future: opportunities and challenges in sustainable agri-food systems. Potato Res. 64, 681–720. doi:10.1007/s11540-021-09501-4
Dey, S., and Dhal, G. C. (2019). Materials progress in the control of CO and CO2 emission at ambient conditions: an overview. Mater. Sci. Energy Technol. 2, 607–623. doi:10.1016/j.mset.2019.06.004
Dou, B., Wang, C., Song, Y., Chen, H., Jiang, B., Yang, M., et al. (2016). Solid sorbents for in-situ CO2 removal during sorption-enhanced steam reforming process: a review. Renew. Sustain. Energy Rev. 53, 536–546. doi:10.1016/j.rser.2015.08.068
Dou, X., Zhang, J., Zhang, C., Ma, D., Chen, L., Zhou, G., et al. (2023). Calcium carbonate regulates soil organic carbon accumulation by mediating microbial communities in northern China. CATENA 231, 107327. doi:10.1016/j.catena.2023.107327
Durand, N., Monger, H. C., Canti, M. G., and Verrecchia, E. P. (2018). “Calcium carbonate features,” in Interpretation of micromorphological features of soils and regoliths (Elsevier), 205–258. doi:10.1016/B978-0-444-63522-8.00009-7
Ebadi Jamkhaneh, M., Ahmadi, M., and Shokri Amiri, M. (2021). Sustainable reuse of inorganic materials in eco-friendly clay bricks: special focus on mechanical and durability assessment. J. Mater. Civ. Eng. 33, 04021111. doi:10.1061/(ASCE)MT.1943-5533.0003737
Erans, M., Manovic, V., and Anthony, E. J. (2016). Calcium looping sorbents for CO2 capture. Appl. Energy 180, 722–742. doi:10.1016/j.apenergy.2016.07.074
Erans, M., Nabavi, S. A., and Manović, V. (2020). Carbonation of lime-based materials under ambient conditions for direct air capture. J. Clean. Prod. 242, 118330. doi:10.1016/j.jclepro.2019.118330
Fadia, P., Tyagi, S., Bhagat, S., Nair, A., Panchal, P., Dave, H., et al. (2021). Calcium carbonate nano- and microparticles: synthesis methods and biological applications. 3 Biotech. 11, 457. doi:10.1007/s13205-021-02995-2
Fan, L.-Z., He, H., and Nan, C.-W. (2021). Tailoring inorganic–polymer composites for the mass production of solid-state batteries. Nat. Rev. Mater. 6, 1003–1019. doi:10.1038/s41578-021-00320-0
Florin, N. H., Blamey, J., and Fennell, P. S. (2010). Synthetic CaO-based sorbent for CO2 capture from large-point sources. Energy fuels. 24, 4598–4604. doi:10.1021/ef100447c
Fones, H. N., Bebber, D. P., Chaloner, T. M., Kay, W. T., Steinberg, G., and Gurr, S. J. (2020). Threats to global food security from emerging fungal and oomycete crop pathogens. Nat. Food 1, 332–342. doi:10.1038/s43016-020-0075-0
Frölicher, T. L., Winton, M., and Sarmiento, J. L. (2014). Continued global warming after CO2 emissions stoppage. Nat. Clim. Change 4, 40–44. doi:10.1038/nclimate2060
Fukase, E., and Martin, W. (2020). Economic growth, convergence, and world food demand and supply. World Dev. 132, 104954. doi:10.1016/j.worlddev.2020.104954
Furukawa, S., and Komatsu, T. (2017). Intermetallic compounds: promising inorganic materials for well-structured and electronically modified reaction environments for efficient catalysis. ACS Catal. 7, 735–765. doi:10.1021/acscatal.6b02603
Gadikota, G. (2021). Carbon mineralization pathways for carbon capture, storage and utilization. Commun. Chem. 4, 23. doi:10.1038/s42004-021-00461-x
Gągol, M., Cako, E., Fedorov, K., Soltani, R. D. C., Przyjazny, A., and Boczkaj, G. (2020). Hydrodynamic cavitation based advanced oxidation processes: studies on specific effects of inorganic acids on the degradation effectiveness of organic pollutants. J. Mol. Liq. 307, 113002. doi:10.1016/j.molliq.2020.113002
Gambhir, A., and Tavoni, M. (2019). Direct air carbon capture and sequestration: how it works and how it could contribute to climate-change mitigation. One Earth 1, 405–409. doi:10.1016/j.oneear.2019.11.006
Gao, Y., Chen, S., Li, Y., and Shi, Y. (2023). Effect of nano-calcium carbonate on morphology, antioxidant enzyme activity and photosynthetic parameters of wheat (Triticum aestivum L.) seedlings. Chem. Biol. Technol. Agric. 10, 31. doi:10.1186/s40538-023-00404-9
García-Mota, M., Gómez-Díaz, J., Novell-Leruth, G., Vargas-Fuentes, C., Bellarosa, L., Bridier, B., et al. (2011). A density functional theory study of the ‘mythic’ Lindlar hydrogenation catalyst. Theor. Chem. Acc. 128, 663–673. doi:10.1007/s00214-010-0800-0
Gentili, R., Ambrosini, R., Montagnani, C., Caronni, S., and Citterio, S. (2018). Effect of soil pH on the growth, reproductive investment and pollen allergenicity of ambrosia artemisiifolia L. Front. Plant Sci. 9, 1335. doi:10.3389/fpls.2018.01335
Goh, P. S., and Ismail, A. F. (2018). A review on inorganic membranes for desalination and wastewater treatment. Desalination 434, 60–80. doi:10.1016/j.desal.2017.07.023
Gu, D., Andreev, K., and Dupre, E. (2021). Major trends in population growth around the world, 3, 604–613. doi:10.46234/ccdcw2021.160
Hamdan, N., and Kavazanjian, E. (2016). Enzyme-induced carbonate mineral precipitation for fugitive dust control. Géotechnique 66, 546–555. doi:10.1680/jgeot.15.P.168
Hamdan, N., Kavazanjian, E., Rittmann, B. E., and Karatas, I. (2017). Carbonate mineral precipitation for soil improvement through microbial denitrification. Geomicrobiol. J. 34, 139–146. doi:10.1080/01490451.2016.1154117
Hanifa, M., Agarwal, R., Sharma, U., Thapliyal, P. C., and Singh, L. P. (2023). A review on CO2 capture and sequestration in the construction industry: emerging approaches and commercialised technologies. J. CO2 Util. 67, 102292. doi:10.1016/j.jcou.2022.102292
Hargis, C. W., Chen, I. A., Devenney, M., Fernandez, M. J., Gilliam, R. J., and Thatcher, R. P. (2021). Calcium carbonate cement: a carbon capture, utilization, and storage (CCUS) technique. Materials 14, 2709. doi:10.3390/ma14112709
Hasegawa, T., Sakurai, G., Fujimori, S., Takahashi, K., Hijioka, Y., and Masui, T. (2021). Extreme climate events increase risk of global food insecurity and adaptation needs. Nat. Food 2, 587–595. doi:10.1038/s43016-021-00335-4
Herzog, H. J. (2011). Scaling up carbon dioxide capture and storage: from megatons to gigatons. Energy Econ. 33, 597–604. doi:10.1016/j.eneco.2010.11.004
Hua, K.-H., Wang, H.-C., Chung, R.-S., and Hsu, J.-C. (2015). Calcium carbonate nanoparticles can enhance plant nutrition and insect pest tolerance. J. Pestic. Sci. 40, 208–213. doi:10.1584/jpestics.D15-025
Huang, M.-T., and Zhai, P.-M. (2021). Achieving Paris Agreement temperature goals requires carbon neutrality by middle century with far-reaching transitions in the whole society. Adv. Clim. Change Res. 12, 281–286. doi:10.1016/j.accre.2021.03.004
Huisingh, D., Zhang, Z., Moore, J. C., Qiao, Q., and Li, Q. (2015). Recent advances in carbon emissions reduction: policies, technologies, monitoring, assessment and modeling. J. Clean. Prod. 103, 1–12. doi:10.1016/j.jclepro.2015.04.098
Humlum, O., Stordahl, K., and Solheim, J.-E. (2013). The phase relation between atmospheric carbon dioxide and global temperature. Glob. Planet. Change 100, 51–69. doi:10.1016/j.gloplacha.2012.08.008
Jiang, Y., Li, Y., Zeng, Q., Wei, J., and Yu, H. (2017). The effect of soil pH on plant growth, leaf chlorophyll fluorescence and mineral element content of two blueberries. Acta Hortic., 269–276. doi:10.17660/ActaHortic.2017.1180.36
Jin, Z., Yu, C., Wang, R., Wei, W., Jing, Y., and Wang, Q. (2022). Preparation of precipitated calcium carbonate using wollastonite and CO2 from industrial exhaust. Braz. J. Chem. Eng. 39, 661–669. doi:10.1007/s43153-021-00200-8
Junaid, M. F., Rehman, Z. U., Čekon, M., Čurpek, J., Farooq, R., Cui, H., et al. (2021). Inorganic phase change materials in thermal energy storage: a review on perspectives and technological advances in building applications. Energy Build. 252, 111443. doi:10.1016/j.enbuild.2021.111443
Karl Heinz, B., Hans-Heinrich, M., and Dietmar, W. (2010). Industrial inorganic chemistry, 2nd, completed rev. Weinheim: Wiley VCH.
Karnosky, D. F. (2003). Impacts of elevated atmospheric CO2 on forest trees and forest ecosystems: knowledge gaps. Environ. Int. 29, 161–169. doi:10.1016/S0160-4120(02)00159-9
Katsuyama, Y., Yamasaki, A., Iizuka, A., Fujii, M., Kumagai, K., and Yanagisawa, Y. (2005). Development of a process for producing high-purity calcium carbonate (CaCO3) from waste cement using pressurized CO2. Environ. Prog. 24, 162–170. doi:10.1002/ep.10080
Kayvani Fard, A., McKay, G., Buekenhoudt, A., Al Sulaiti, H., Motmans, F., Khraisheh, M., et al. (2018). Inorganic membranes: preparation and application for water treatment and desalination. Materials 11, 74. doi:10.3390/ma11010074
Kitchen, H. J., Vallance, S. R., Kennedy, J. L., Tapia-Ruiz, N., Carassiti, L., Harrison, A., et al. (2014). Modern microwave methods in solid-state inorganic materials chemistry: from fundamentals to manufacturing. Chem. Rev. 114, 1170–1206. doi:10.1021/cr4002353
Kouzu, M., Kasuno, T., Tajika, M., Sugimoto, Y., Yamanaka, S., and Hidaka, J. (2008). Calcium oxide as a solid base catalyst for transesterification of soybean oil and its application to biodiesel production. Fuel 87, 2798–2806. doi:10.1016/j.fuel.2007.10.019
Laverdura, U. P., Rossi, L., Ferella, F., Courson, C., Zarli, A., Alhajyoussef, R., et al. (2020). Selective catalytic hydrogenation of vegetable oils on lindlar catalyst. ACS Omega 5, 22901–22913. doi:10.1021/acsomega.0c02280
Levey, C., Reed, J., Sanchez, C., Schneider, J., and Constantz, B. R. (2024). Calorimetry of amorphous calcium carbonate is correlated with its lithification and durability as synthetic stone—implications for CO2 storage and utilization. Front. Mater. 11, 1365217. doi:10.3389/fmats.2024.1365217
Liang, K., Li, L., and Yang, Y. (2017). Inorganic porous films for renewable energy storage. ACS Energy Lett. 2, 373–390. doi:10.1021/acsenergylett.6b00666
Lima, M. A., Mendes, L. F. R., Mothé, G. A., Linhares, F. G., De Castro, M. P. P., Da Silva, M. G., et al. (2020). Renewable energy in reducing greenhouse gas emissions: reaching the goals of the Paris agreement in Brazil. Environ. Dev. 33, 100504. doi:10.1016/j.envdev.2020.100504
Lin, P.-Y., Wu, H.-M., Hsieh, S.-L., Li, J.-S., Dong, C., Chen, C.-W., et al. (2020). Preparation of vaterite calcium carbonate granules from discarded oyster shells as an adsorbent for heavy metal ions removal. Chemosphere 254, 126903. doi:10.1016/j.chemosphere.2020.126903
Lindlar, H., and Dubuis, R. (2003). “Palladium catalyst for partial reduction of acetylenes,” in Organic syntheses. Editors A. S. Kende, and J. P. Freeman (Wiley), 89. doi:10.1002/0471264180.os046.27
Ling, J. S. J., Tan, Y. H., Mubarak, N. M., Kansedo, J., Saptoro, A., and Nolasco-Hipolito, C. (2019). A review of heterogeneous calcium oxide based catalyst from waste for biodiesel synthesis. SN Appl. Sci. 1, 810. doi:10.1007/s42452-019-0843-3
Liu, H., Cao, C.-Y., Wei, F.-F., Jiang, Y., Sun, Y.-B., Huang, P.-P., et al. (2013). Fabrication of macroporous/mesoporous carbon nanofiber using CaCO3 nanoparticles as dual purpose template and its application as catalyst support. J. Phys. Chem. C 117, 21426–21432. doi:10.1021/jp4078807
Liu, K.-G., Sharifzadeh, Z., Rouhani, F., Ghorbanloo, M., and Morsali, A. (2021). Metal-organic framework composites as green/sustainable catalysts. Coord. Chem. Rev. 436, 213827. doi:10.1016/j.ccr.2021.213827
Liu, L., Diao, X., He, Z., Yi, Y., Wang, T., Wang, M., et al. (2020). High-performance all-inorganic portable electrochromic Li-ion hybrid supercapacitors toward safe and smart energy storage. Energy Storage Mater. 33, 258–267. doi:10.1016/j.ensm.2020.08.023
Liu, Q., He, X., and Li, D. (2023). Unearthing the mechanisms underlying calcium carbonate therapies for eliminating pathogens during composting. Chem. Eng. J. 451, 139087. doi:10.1016/j.cej.2022.139087
Liu, W., Low, N. W., Feng, B., Wang, G., and Diniz Da Costa, J. C. (2010). Calcium precursors for the production of CaO sorbents for multicycle CO2 capture. Environ. Sci. Technol. 44, 841–847. doi:10.1021/es902426n
Liu, X., Xing, S., Yang, L., Fu, J., Lv, P., Zhang, X., et al. (2021). Highly active and durable Ca-based solid base catalyst for biodiesel production. Fuel 302, 121094. doi:10.1016/j.fuel.2021.121094
Lizandara-Pueyo, C., Fan, X., Ayats, C., and Pericàs, M. A. (2021). Calcium carbonate as heterogeneous support for recyclable organocatalysts. J. Catal. 393, 107–115. doi:10.1016/j.jcat.2020.11.013
López-Periago, A. M., Pacciani, R., García-González, C., Vega, L. F., and Domingo, C. (2010). A breakthrough technique for the preparation of high-yield precipitated calcium carbonate. J. Supercrit. Fluids 52, 298–305. doi:10.1016/j.supflu.2009.11.014
Lüthi, D., Le Floch, M., Bereiter, B., Blunier, T., Barnola, J.-M., Siegenthaler, U., et al. (2008). High-resolution carbon dioxide concentration record 650,000–800,000 years before present. Nature 453, 379–382. doi:10.1038/nature06949
Manikandan, S., Subbaiya, R., Saravanan, M., Ponraj, M., Selvam, M., and Pugazhendhi, A. (2022). A critical review of advanced nanotechnology and hybrid membrane based water recycling, reuse, and wastewater treatment processes. Chemosphere 289, 132867. doi:10.1016/j.chemosphere.2021.132867
Marin Rivera, R., and Van Gerven, T. (2020). Production of calcium carbonate with different morphology by simultaneous CO2 capture and mineralisation. J. CO2 Util. 41, 101241. doi:10.1016/j.jcou.2020.101241
Martinez, D. M., Horvath, A., and Monteiro, P. J. M. (2023). Comparative environmental assessment of limestone calcined clay cements and typical blended cements. Environ. Res. Commun. 5, 055002. doi:10.1088/2515-7620/acccd8
Mazari, S. A., Ali, E., Abro, R., Khan, F. S. A., Ahmed, I., Ahmed, M., et al. (2021). Nanomaterials: applications, waste-handling, environmental toxicities, and future challenges – a review. J. Environ. Chem. Eng. 9, 105028. doi:10.1016/j.jece.2021.105028
McFarland, C., Shiwakoti, S., Carpenter-Boggs, L., Schroeder, K., Brown, T., and Huggins, D. R. (2020). Evaluating buffer methods for determining lime requirement on acidified agricultural soils of the Palouse. Soil Sci. Soc. Am. J. 84, 1769–1781. doi:10.1002/saj2.20111
Meyer, F. D., Bang, S., Min, S., Stetler, L. D., and Bang, S. S. (2011). “Microbiologically-induced soil stabilization: application of sporosarcina pasteurii for fugitive dust control,” in Geo-frontiers 2011. Presented at the geo-frontiers congress 2011, American society of civil engineers (Dallas, Texas, United States), 4002–4011. doi:10.1061/41165(397)409
Molotoks, A., Smith, P., and Dawson, T. P. (2021). Impacts of land use, population, and climate change on global food security. Food Energy Secur 10, e261. doi:10.1002/fes3.261
Moon, H. Y., Shin, D. G., and Choi, D. S. (2007). Evaluation of the durability of mortar and concrete applied with inorganic coating material and surface treatment system. Constr. Build. Mater. 21, 362–369. doi:10.1016/j.conbuildmat.2005.08.012
Moore, C. E., Meacham-Hensold, K., Lemonnier, P., Slattery, R. A., Benjamin, C., Bernacchi, C. J., et al. (2021). The effect of increasing temperature on crop photosynthesis: from enzymes to ecosystems. J. Exp. Bot. 72, 2822–2844. doi:10.1093/jxb/erab090
Mundargi, R. C., Potroz, M. G., Park, S., Shirahama, H., Lee, J. H., Seo, J., et al. (2016). Natural sunflower pollen as a drug delivery vehicle. Small 12, 1167–1173. doi:10.1002/smll.201500860
Neeraj, D., and Yadav, S. (2020). Carbon storage by mineral carbonation and industrial applications of CO2. Mater. Sci. Energy Technol. 3, 494–500. doi:10.1016/j.mset.2020.03.005
Neina, D. (2019). The role of soil pH in plant nutrition and soil remediation. Appl. Environ. Soil Sci. 2019, 1–9. doi:10.1155/2019/5794869
Nelson, J. J. M., and Schelter, E. J. (2019). Sustainable inorganic chemistry: metal separations for recycling. Inorg. Chem. 58, 979–990. doi:10.1021/acs.inorgchem.8b01871
Ngamcharussrivichai, C., Nunthasanti, P., Tanachai, S., and Bunyakiat, K. (2010). Biodiesel production through transesterification over natural calciums. Fuel Process. Technol. 91, 1409–1415. doi:10.1016/j.fuproc.2010.05.014
Niemeyer, C. M. (2001). Nanoparticles, proteins, and nucleic acids: biotechnology meets materials science. Angew. Chem. Int. Ed. 40, 4128–4158. doi:10.1002/1521-3773(20011119)40:22<4128::aid-anie4128>3.3.co;2-j
NOAA National Centers for Environmental information (2024). Climate at a glance: global time series, published july. Available at: https://www.ncei.noaa.gov/access/monitoring/climate-at-a-glance/global/time-series.
Olajire, A. A. (2013). A review of mineral carbonation technology in sequestration of CO2. J. Pet. Sci. Eng. 109, 364–392. doi:10.1016/j.petrol.2013.03.013
Osterloh, F. E. (2008). Inorganic materials as catalysts for photochemical splitting of water. Chem. Mater. 20, 35–54. doi:10.1021/cm7024203
Patanè, C., Pellegrino, A., and Di Silvestro, I. (2018). Effects of calcium carbonate application on physiology, yield and quality of field-grown tomatoes in a semi-arid Mediterranean climate. Crop Pasture Sci. 69, 411. doi:10.1071/CP17424
Pham, P. T., Federico-Perez, R. A., Fine, K. L., Matzek, L. W., Carter, K. E., Palomino, A. M., et al. (2020). Sustained release of persulfate from inert inorganic materials for groundwater remediation. Chemosphere 259, 127508. doi:10.1016/j.chemosphere.2020.127508
Piątek, J., Afyon, S., Budnyak, T. M., Budnyk, S., Sipponen, M. H., and Slabon, A. (2021). Sustainable Li-ion batteries: chemistry and recycling. Adv. Energy Mater. 11, 2003456. doi:10.1002/aenm.202003456
Prakash, S. (2021). Impact of climate change on aquatic ecosystem and its biodiversity: an overview. Int. J. Biol. Innov. 03 03. doi:10.46505/IJBI.2021.3210
Pu, Y., Li, L., Wang, Q., Shi, X., Luan, C., Zhang, G., et al. (2021). Accelerated carbonation technology for enhanced treatment of recycled concrete aggregates: a state-of-the-art review. Constr. Build. Mater. 282, 122671. doi:10.1016/j.conbuildmat.2021.122671
Qi, W., Zhou, X., Li, J., Cheng, J., Li, Y., Ko, M. J., et al. (2020). Inorganic material passivation of defects toward efficient perovskite solar cells. Sci. Bull. 65, 2022–2032. doi:10.1016/j.scib.2020.07.017
Ridley, J., Gregory, J. M., Huybrechts, P., and Lowe, J. (2010). Thresholds for irreversible decline of the Greenland ice sheet. Clim. Dyn. 35, 1049–1057. doi:10.1007/s00382-009-0646-0
Ristaino, J. B., Anderson, P. K., Bebber, D. P., Brauman, K. A., Cunniffe, N. J., Fedoroff, N. V., et al. (2021). The persistent threat of emerging plant disease pandemics to global food security. Proc. Natl. Acad. Sci. 118, e2022239118. doi:10.1073/pnas.2022239118
Saetan, T., Lertvachirapaiboon, C., Ekgasit, S., Sukwattanasinitt, M., and Wacharasindhu, S. (2017). Palladium nanoparticles immobilized on individual calcium carbonate plates derived from mussel shell waste: an ecofriendly catalyst for the copper-free sonogashira coupling reaction. Chem. – Asian J. 12, 2221–2230. doi:10.1002/asia.201700537
Salek, S. S., Van Turnhout, A. G., Kleerebezem, R., and Van Loosdrecht, M. C. M. (2015). pH control in biological systems using calcium carbonate. Biotechnol. Bioeng. 112, 905–913. doi:10.1002/bit.25506
Sanna, A., Uibu, M., Caramanna, G., Kuusik, R., and Maroto-Valer, M. M. (2014). A review of mineral carbonation technologies to sequester CO2. Chem. Soc. Rev. 43, 8049–8080. doi:10.1039/C4CS00035H
Santos, C. A. D., Carmo, M. G. F. D., Bhering, A. D. S., Costa, E. S. P., and Amaral Sobrinho, N. M. B. D. (2020). Use of limestone and agricultural gypsum in cauliflower crop management and clubroot control in mountain farming. Acta Sci. Agron. 42, e42494. doi:10.4025/actasciagron.v42i1.42494
Sanz-Pérez, E. S., Murdock, C. R., Didas, S. A., and Jones, C. W. (2016). Direct capture of CO2 from ambient air. Chem. Rev. 116, 11840–11876. doi:10.1021/acs.chemrev.6b00173
Schlägl, R., Noack, K., Zbinden, H., and Reller, A. (1987). The microstructure of selective palladium hydrogenation catalysts supported on calcium carbonate and modified by lead (lindlar catalysts), studied by photoelectron spectroscopy, thermogravimetry, X-ray diffraction, and electron microscopy. Helv. Chim. Acta 70, 627–679. doi:10.1002/hlca.19870700317
Schubert, U., and Hüsing, N. (2019). Synthesis of inorganic materials. Fourth edition. Weinheim: Wiley VCH.
Senra, J. D., Malta, L. F. B., Souza, A. L. F., Aguiar, L. C. S., and Antunes, O. A. C. (2008). Palladium on calcium carbonate combined to 2-Hydroxypropyl-α/β-cyclodextrins: a selective catalytic system for aqueous Heck coupling and hydroarylation. Adv. Synth. Catal. 350, 2551–2558. doi:10.1002/adsc.200800472
Servin, A. D., and White, J. C. (2016). Nanotechnology in agriculture: next steps for understanding engineered nanoparticle exposure and risk. NanoImpact 1, 9–12. doi:10.1016/j.impact.2015.12.002
Seto, J., Azaı̈s, T., and Cölfen, H. (2013). Formation of aragonitic layered structures from kaolinite and amorphous calcium carbonate precursors. Langmuir 29, 7521–7528. doi:10.1021/la400442j
Seto, J., Picker, A., Chen, Y., Rao, A., Evans, J. S., and Cölfen, H. (2014). Nacre protein sequence compartmentalizes mineral polymorphs in solution. Cryst. Growth Des. 14, 1501–1505. doi:10.1021/cg401421h
Shabtai, I. A., Wilhelm, R. C., Schweizer, S. A., Höschen, C., Buckley, D. H., and Lehmann, J. (2023). Calcium promotes persistent soil organic matter by altering microbial transformation of plant litter. Nat. Commun. 14, 6609. doi:10.1038/s41467-023-42291-6
Shakun, J. D., Clark, P. U., He, F., Marcott, S. A., Mix, A. C., Liu, Z., et al. (2012). Global warming preceded by increasing carbon dioxide concentrations during the last deglaciation. Nature 484, 49–54. doi:10.1038/nature10915
Shen, Q., Li, X., Li, R., and Wu, Y. (2020). Application of metal–organic framework materials and derived porous carbon materials in catalytic hydrogenation. ACS Sustain. Chem. Eng. 8, 17608–17621. doi:10.1021/acssuschemeng.0c06849
Snæbjörnsdóttir, S. Ó., Sigfússon, B., Marieni, C., Goldberg, D., Gislason, S. R., and Oelkers, E. H. (2020). Carbon dioxide storage through mineral carbonation. Nat. Rev. Earth Environ. 1, 90–102. doi:10.1038/s43017-019-0011-8
Song, C. E., and Lee, S. (2002). Supported chiral catalysts on inorganic materials. Chem. Rev. 102, 3495–3524. doi:10.1021/cr0103625
Song, J. Y., Sim, Y., Jang, J., Hong, W.-T., and Yun, T. S. (2020). Near-surface soil stabilization by enzyme-induced carbonate precipitation for fugitive dust suppression. Acta Geotech. 15, 1967–1980. doi:10.1007/s11440-019-00881-z
Soon, N. W., Lee, L. M., Khun, T. C., and Ling, H. S. (2014). Factors affecting improvement in engineering properties of residual soil through microbial-induced calcite precipitation. J. Geotech. Geoenvironmental Eng. 140, 04014006. doi:10.1061/(ASCE)GT.1943-5606.0001089
Stefaniuk, D., Hajduczek, M., Weaver, J. C., Ulm, F. J., and Masic, A. (2023). Cementing CO2 into C-S-H: a step toward concrete carbon neutrality. PNAS Nexus 2, pgad052. doi:10.1093/pnasnexus/pgad052
Stone, E. J., Lowe, J. A., and Shine, K. P. (2009). The impact of carbon capture and storage on climate. Energy Env. Sci. 2, 81–91. doi:10.1039/B807747A
Sun, Y., and Rogers, J. A. (2007). Inorganic semiconductors for flexible electronics. Adv. Mater. 19, 1897–1916. doi:10.1002/adma.200602223
Suppes, G. J., Bockwinkel, K., Lucas, S., Botts, J. B., Mason, M. H., and Heppert, J. A. (2001). Calcium carbonate catalyzed alcoholysis of fats and oils. J. Am. Oil Chem. Soc. 78, 139–146. doi:10.1007/s11746-001-0234-y
Teir, S., Kotiranta, T., Pakarinen, J., and Mattila, H.-P. (2016). Case study for production of calcium carbonate from carbon dioxide in flue gases and steelmaking slag. J. CO2 Util. 14, 37–46. doi:10.1016/j.jcou.2016.02.004
Thangaraj, B., Solomon, P. R., Muniyandi, B., Ranganathan, S., and Lin, L. (2019). Catalysis in biodiesel production—a review. Clean. Energy 3, 2–23. doi:10.1093/ce/zky020
Thonemann, N., Zacharopoulos, L., Fromme, F., and Nühlen, J. (2022). Environmental impacts of carbon capture and utilization by mineral carbonation: a systematic literature review and meta life cycle assessment. J. Clean. Prod. 332, 130067. doi:10.1016/j.jclepro.2021.130067
Toggweiler, J. R., and Lea, D. W. (2010). Temperature differences between the hemispheres and ice age climate variability: ice age climate variability. Paleoceanography 25. doi:10.1029/2009PA001758
U.S. Global Change Research Program Wuebbles, D. J., Fahey, D. W., Hibbard, K. A., Dokken, D. J., Stewart, B. C., and Maycock, T. K. (2017). Climate science special report: fourth national climate assessment, volume I. U.S. Glob. Change Res. Program. doi:10.7930/J0J964J6
Vallet-Regí, M., Balas, F., Colilla, M., and Manzano, M. (2007). Bioceramics and pharmaceuticals: a remarkable synergy. Solid State Sci. 9, 768–776. doi:10.1016/j.solidstatesciences.2007.03.026
Valone, T. F. (2023). Gigatonne carbon dioxide removal can reverse global heating trend. . Environ. Ecol. 20, 42–58. doi:10.9734/ajee/2023/v20i2436
Van Dijk, M., Morley, T., Rau, M. L., and Saghai, Y. (2021). A meta-analysis of projected global food demand and population at risk of hunger for the period 2010–2050. Nat. Food 2, 494–501. doi:10.1038/s43016-021-00322-9
Van Soest, H. L., Den Elzen, M. G. J., and Van Vuuren, D. P. (2021). Net-zero emission targets for major emitting countries consistent with the Paris Agreement. Nat. Commun. 12, 2140. doi:10.1038/s41467-021-22294-x
Wang, C., Li, W., Yang, Z., Chen, Y., Shao, W., and Ji, J. (2015). An invisible soil acidification: critical role of soil carbonate and its impact on heavy metal bioavailability. Sci. Rep. 5, 12735. doi:10.1038/srep12735
Wang, J., and Wang, S. (2021). Effect of inorganic anions on the performance of advanced oxidation processes for degradation of organic contaminants. Chem. Eng. J. 411, 128392. doi:10.1016/j.cej.2020.128392
Wang, L., Zhang, J., Zhang, Y., Yu, H., Qu, Y., and Yu, J. (2022). Inorganic metal-oxide photocatalyst for H2O2 production. Small 18, 2104561. doi:10.1002/smll.202104561
Waris, A., Din, M., Ali, A., Ali, M., Afridi, S., Baset, A., et al. (2021). A comprehensive review of green synthesis of copper oxide nanoparticles and their diverse biomedical applications. Inorg. Chem. Commun. 123, 108369. doi:10.1016/j.inoche.2020.108369
Witoon, T. (2011). Characterization of calcium oxide derived from waste eggshell and its application as CO2 sorbent. Ceram. Int. 37, 3291–3298. doi:10.1016/j.ceramint.2011.05.125
Xiang, S., Kang, H., Chai, A., Shi, Y., Xie, X., Li, L., et al. (2023). Calcium carbonate-modified plant sporopollen capsule as an eco-friendly microvehicle for controlled release of pesticide. Pest Manag. Sci. 79, 1604–1614. doi:10.1002/ps.7333
Xiang, Y., Han, J., Zhang, G., Zhan, F., Cai, D., and Wu, Z. (2018). Efficient synthesis of starch-regulated porous calcium carbonate microspheres as a carrier for slow-release herbicide. ACS Sustain. Chem. Eng. 6, 3649–3658. doi:10.1021/acssuschemeng.7b03973
Xiang, Y., Liu, H., Zhu, E., Yang, K., Yuan, D., Jiao, T., et al. (2022). Application of inorganic materials as heterogeneous cocatalyst in Fenton/Fenton-like processes for wastewater treatment. Sep. Purif. Technol. 295, 121293. doi:10.1016/j.seppur.2022.121293
Xie, J., Gao, J., Cao, H., Li, J., Wang, X., Zhang, J., et al. (2024). Calcium carbonate promotes the formation and stability of soil macroaggregates in mining areas of China. J. Integr. Agric. 23, 1034–1047. doi:10.1016/j.jia.2023.09.015
Yoro, K. O., and Daramola, M. O. (2020). “CO2 emission sources, greenhouse gases, and the global warming effect,” in Advances in carbon capture (Elsevier), 3–28. doi:10.1016/B978-0-12-819657-1.00001-3
Zeng, W.-A., Li, F., Zhou, H., Qin, X.-L., Zou, Z.-J., Tian, T., et al. (2015). Effect of calcium carbonate on cadmium and nutrients uptake in tobacco (Nicotiana tabacum L.) planted on contaminated soil. J. Environ. Biol. 37, 163–168.
Zhang, Y., Zhang, S., Wang, R., Cai, J., Zhang, Y., Li, H., et al. (2016). Impacts of fertilization practices on pH and the pH buffering capacity of calcareous soil. Soil Sci. Plant Nutr. 62, 432–439. doi:10.1080/00380768.2016.1226685
Zhao, H., Chang, J., Havlík, P., Van Dijk, M., Valin, H., Janssens, C., et al. (2021). China’s future food demand and its implications for trade and environment. Nat. Sustain. 4, 1042–1051. doi:10.1038/s41893-021-00784-6
Zhao, X., Cui, H., Wang, Y., Sun, C., Cui, B., and Zeng, Z. (2018). Development strategies and prospects of nano-based smart pesticide formulation. J. Agric. Food Chem. 66, 6504–6512. doi:10.1021/acs.jafc.7b02004
Zheng, B., Fan, J., Chen, B., Qin, X., Wang, J., Wang, F., et al. (2022). Rare-earth doping in nanostructured inorganic materials. Chem. Rev. 122, 5519–5603. doi:10.1021/acs.chemrev.1c00644
Keywords: calcium carbonate, catalysis, carbon capture, agriculture, inorganic materials chemistry
Citation: Comes J, Islamovic E, Lizandara-Pueyo C and Seto J (2024) Improvements in the utilization of calcium carbonate in promoting sustainability and environmental health. Front. Chem. 12:1472284. doi: 10.3389/fchem.2024.1472284
Received: 23 August 2024; Accepted: 23 September 2024;
Published: 03 October 2024.
Edited by:
Bin Mu, Chinese Academy of Sciences (CAS), ChinaReviewed by:
Dimitrios Papoulis, University of Patras, GreeceYanfu Wei, Macau University of Science and Technology, Macao SAR, China
Copyright © 2024 Comes, Islamovic, Lizandara-Pueyo and Seto. This is an open-access article distributed under the terms of the Creative Commons Attribution License (CC BY). The use, distribution or reproduction in other forums is permitted, provided the original author(s) and the copyright owner(s) are credited and that the original publication in this journal is cited, in accordance with accepted academic practice. No use, distribution or reproduction is permitted which does not comply with these terms.
*Correspondence: Jong Seto, am9uZy5zZXRvQGFzdS5lZHU=; Emir Islamovic, ZW1pci5pc2xhbW92aWNAYmFzZi5jb20=; Carlos Lizandara-Pueyo, Y2FybG9zLmxpemFuZGFyYUBiYXNmLmNvbQ==